- 1Department of Infectious Disease, The Second Affiliated Hospital and Yuying Children’s Hospital, Wenzhou Medical University, Wenzhou, China
- 2Key Laboratory of Medical Genetics of Zhejiang Province, Key Laboratory of Laboratory Medicine, Ministry of Education, School of Laboratory Medicine and Life Sciences, Wenzhou Medical University, Wenzhou, China
- 3Department of Pediatric Respiratory Disease, The Second Affiliated Hospital and Yuying Children’s Hospital, Wenzhou Medical University, Wenzhou, China
- 4Medical Molecular Biology Laboratory, School of Medicine, Jinhua Polytechnic, Jinhua, China
- 5Institute of Translational Medicine, Baotou Central Hospital, Baotou, China
Pseudomonas aeruginosa can cause infections in the blood, lungs (pneumonia), or other parts of the body after surgery. To investigate the molecular characteristics of β-lactam antibiotic resistance of P. aeruginosa isolated from a hospital population between 2015 and 2017, in this study, the antimicrobial susceptibility and the resistance gene profile of the bacteria were determined. The Pulsed-field gel electrophoresis (PFGE) was used to characterize the clonal relatedness and sequencing and comparative genomic analysis were performed to analyze the structure of the resistance gene-related sequences. As a result, of the 260 P. aeruginosa strains analyzed, the resistance rates for 6 β-lactam antibiotics ranged from 4.6 to 9.6%. A total of 7 genotypes of 44 β-lactamase genes were identified in 23 isolates (8.9%, 23/260). Four transconjugants from different donors carrying blaCARB-3 exhibited a phenotype of reduced susceptibility to piperacillin–tazobactam, ceftazidime, and cefepime, and 2 transconjugants harboring blaIMP-45 exhibited a phenotype of reduced susceptibility to carbapenems. blaCARB positive isolates (n = 12) presented six PFGE patterns, designated groups A to F. Two bla genes (blaIMP-45 and blaOXA-1) in PA1609 related to a class 1 integron (intI1-blaIMP-45-blaOXA-1-aac(6′)-Ib7-catB3-qacE∆1-sul1) were encoded on a plasmid (pPA1609-475), while the blaCARB-3 gene of PA1616 also related to a class 1 integron was located on the chromosome. The results suggest that β-lactam antibiotic resistance and clonal dissemination exist in this hospital population. It indicates the necessity for molecular surveillance in tracking β-lactamase-producing strains and emphasizes the need for epidemiological monitoring.
Introduction
The treatment of Pseudomonas aeruginosa (P. aeruginosa) infection has become a clinical problem because of the emergence of multidrug-resistant bacteria (Subedi et al., 2018). P. aeruginosa is prevalent in healthcare settings and is commonly found in patients under medical care and surviving on biotic and abiotic surfaces, such as medical equipment (Mohammadpour et al., 2019). It is largely associated with hospital-acquired infections including ventilator-associated pneumonia, central line-associated bloodstream infection, and so on (Philippon et al., 1986; Barnes et al., 2018).
Pseudomonas aeruginosa is intrinsically resistant to a variety of antimicrobials, such as β-lactams (Keith, 2011). One of the main drug resistance mechanisms is the production of β-lactamase, an enzyme that hydrolyses β-lactam antibiotics and leads to their inactivation (Livermore and Woodford, 2006). There are many classifications of β-lactamases (Bush and Jacoby, 2010). The β-lactamases classified under the Ambler classification are divided into four classes: Class A ESBLs, Class B MBLs, Class C AmpC, and Class D (OXA type). Two of them (Class A and B) are reported as rapidly developing enzymes in clinical isolates of P. aeruginosa (Al Dawodeyah et al., 2018).
In P. aeruginosa, the overexpression of the naturally occurring AmpC is associated with a decreased susceptibility or resistance to expanded spectrum cephalosporins, such as ceftazidime (Mikhail et al., 2019). Plasmid-mediated AmpC was first identified in the 1980s in Gram-negative bacteria (Fallah et al., 2013). However, unlike Enterobacteriaceae, very few reports describe plasmid-borne AmpC in P. aeruginosa. The sulfhydryl reagent variable (SHV) family of Class A β-lactamases was derived from the chromosome of Klebsiella spp., but later incorporated into plasmids (Wolter and Lister, 2013). Variants of SHV have been isolated from P. aeruginosa. The TEM (Temoneira) type β-lactamases are functionally similar to SHV and were first identified in Escherichia coli. Later, many TEM variants were found in P. aeruginosa with different enzymatic capacities (Livermore and Woodford, 2006; Bush and Jacoby, 2010). Carbapenem resistance in clinical isolates of A. baumannii is mediated by overexpression of either OXA-23 or OXA-51 through insertion of ISAba1 in their promoter region (Wong et al., 2019). Several variants of OXA enzymes with extended spectrum have been identified in P. aeruginosa (Shaikh et al., 2015). Other extended-spectrum β-lactamases (ESBL) include PER (penicillins and cephalosporins), GES (penicillins and extended-spectrum cephalosporins), and KPC (carbapenems). Metallo-β-lactamases are unique due to the presence of zinc in their active site. They are plasmid-mediated and highly transferable; however, chromosomal integration is also common. The first metallo-β-lactamase (IMP-1) in P. aeruginosa was detected in Japan and since then the incidence of these agents has been increasing (Watanabe et al., 1991). Other MGE (mobile genetic elements)-associated metallo-β-lactamase families include SPM, VIM, and GIM (Ghamgosha et al., 2015). These also have been identified in P. aeruginosa and their prevalence rates are gradually increasing (Queenan and Bush, 2007). Since mutations in the ESBL genes produce enzymes with different specificities for substrates or susceptibilities to β-lactam inhibitors, the numbers of variants are growing.
In this study, the molecular resistance mechanisms of β-lactam antibiotics and the characteristics of the resistance gene-related sequences of 260 clinical P. aeruginosa isolates were analyzed.
Materials and Methods
Bacterial Strains
A total of 260 non-duplicate clinical P. aeruginosa (hereinafter referred to as PA in strain numbers of P. aeruginosa) were isolated from an affiliated hospital of Wenzhou Medical University, Zhejiang, China between 2015 and 2017. The strain was identified using the Vitek-60 microorganism auto analysis system (BioMerieux Corporate, Craponne, France). Further verification was performed using homologous comparisons of the sequences of 16S rRNA genes.
Antimicrobial Susceptibility Test
Antimicrobial susceptibility test was performed using the agar dilution method and interpreted according to the Clinical and Laboratory Standards Institute (CLSI, 2019) guidelines. P. aeruginosa ATCC 27853 was used as the quality control strain.
DNA Extraction and Sequencing
Each isolate was incubated overnight in 5 ml of Luria-Bertani (LB) broth (Yamamoto et al., 2021) at 37°C for 16 h, and the genomic DNA was extracted using an AxyPrep Bacterial Genomic DNA Miniprep kit (Axygen Scientific, Union City, CA, United States). For the mixed genomic DNA sequencing, the cultured LB broth of all 260 isolates was mixed and the DNA was extracted. A library with an average insert size of 400 bp was prepared using the NEBNext Ultra II DNA library preparation kit and subsequently sequenced by the Illumina Novaseq (paired-end run; 2 × 150 bp with sequencing depth of about 100X). In addition, for the whole-genome sequencing of a certain bacterium, a 10- to 20-kb insert library was prepared and sequenced (with sequencing depth of about 200X, coverage 100%) by the Pacific Bioscience RSII sequencer at Annoroad Gene Technology Co., Ltd. (Beijing, China).
Genome Assembly and Annotation
Genome assembly of the mixed DNA sequencing data was performed using megahit.1 The complete genome of a P. aeruginosa isolate was assembled using Canu2 with long reads obtained from PacBio sequencing and the error correction of the tentative complete circular sequence was performed using Pilon3 with short read sets of the same isolate derived from Illumina sequencing. Open reading frames (ORFs) of the mixed DNA sequences were predicted using Prodigal4 with default parameters. Using the antibiotic resistance genes of the CARD5 and ResFinder databases6 as a query, a BLASTN search was performed against the assembled sequences of the mixed DNA with thresholds of >70% nucleotide identity and > 80% alignment coverage. Gene prediction and annotation of the genome were initially performed with RAST7 and then verified by BLASTP searches against the UniProtKB/Swiss-Prot8 and RefSeq databases.9 Annotation of mobile genetic elements was carried out using online databases including ISfinder,10 INTEGRALL,11 and the Tn Number Registry.12 Gene organization diagrams were generated using R script13 and modified with Inkscape 1.0.14
Screening of the β-Lactamase Resistance Determinants
β-lactamase resistance genes including Amber class A (blaCARB-3, blaGES-1, and blaTEM-1), class B (blaIMP-45), class C (blaDHA-1, blaPDC-3), and class D (blaOXA-23, blaOXA-50, blaOXA-66) were screened by the polymerase chain reaction (PCR). The primers were designed according to the corresponding publications and synthesized by Shanghai Sunny Biotechnology Co., Ltd. (Shanghai, China; Table 1). The PCR products were verified by sequencing and compared with those in the public database by the BLASTN program.15
Plasmid Conjugation Experiments
The conjugation experiment of biparental mating on the sterile nitrocellulose filter was performed using the multiple resistant P. aeruginosa as the donor cell and the rifampin-resistant Escherichia coli C600 (EC600) as the recipient (He et al., 2021). The transconjugants were selected on Mueller–Hinton agar plates containing 600 μg/ml rifampin and 32 μg/ml ceftazidime or 8 μg/ml meropenem. The target resistance gene in the transformant was verified by PCR with primers of the corresponding β-lactamase resistance genes (Table 1). The PCR product was sequenced and the sequence was compared with those in the public database by the BLASTN program (see footnote 15). The plasmid of the transformant was extracted with an AxyPrep Plasmid Miniprep Kit (Axygen Scientific, Union City, CA, United States), and the target resistance gene on the plasmid was also verified by PCR and PCR product sequencing as mentioned above.
Pulsed-Field Gel Electrophoresis
Pulsed-field gel electrophoresis was performed after digestion of DNA samples with 20 U of Spe I restriction enzyme (Takara, Dalian, China). The DNA fragments were separated using a CHEF-Mapper XA PFGE system (Bio-Rad, Hercules, California, United States) for 18 h at 6 V/cm for 14°C, with a pulse angle of 120° and pulse duration of 5 s to 25 s in 1% Seakem Gold agarose with cell suspension buffer (10 ml 1 M Tris–HCl, pH 8.0, 20 ml 0.5 M EDTA, pH 8.0, diluted to 100 ml with sterilized pure water) and cell lysis buffer (25 ml 1 M Tris–HCl, pH 8.0, 50 ml 0.5 M EDTA, pH 8.0, 50 ml 10% Sarcosyl, diluted to 100 ml with sterilized pure water). The restriction patterns were analyzed and interpreted according to the criteria proposed by Tenover, et al. (Bannerman et al., 1995).
Comparative Genomic Analysis of the β-Lactamase Gene-Related Sequences
For the comparative genomic analysis of the blaCARB-3, blaIMP45, and blaPDC3 gene-related fragments, sequences containing these bla genes were obtained from the NCBI nucleotide database using the blaCARB-3 gene (S46063.1), the blaIMP45 gene (NG_049209) or the blaPDC3 gene (NG_04990) as a query by Blastn searching. The results were retained only if sequences containing a complete blaCARB-3 (a total of 13 kb with approximately 6 kb both upstream and downstream of the gene), blaIMP45 (a total of 22 kb with approximately 18 kb upstream and 3 kb downstream of the gene) or blaPDC3 (a total of 10 kb with approximately 4 kb upstream and 5 kb downstream of the gene). Multiple sequence alignments were performed by MAFFT2417 using each of the bla gene-related fragments of this work as a reference, and the sequences were clustered and the sequences flanking the resistance gene with an identity of ≥80% was retained. The sequence sharing the highest similarity to the other sequences in each cluster was chosen as the candidate for ortholog analysis. Orthologous groups of the genes from the candidate sequences were identified using BLASTP and InParanoid.18 The sequence retrieval, statistical analysis, and other bioinformatics tools used in this study were applied with Python and Biopython scripts.19
Data Availability Statement
The nucleotide sequences of the chromosomes and plasmids of the bacteria of this work have been deposited in GenBank under accession numbers CP090649 (PA1609), CP090650 (pPA1609-475), CP090651 (pPA1609-47), CP090648 (PA1616), and CP090647 (PA1681).
Results
Antimicrobial Susceptibility
In this study, the MIC levels of the 12 antibiotics (including oxacillin, piperacillin–tazobactam, cefuroxime, ceftazidime, cefepime, aztreonam, imipenem, meropenem, gentamicin, amikacin, levofloxacin, and polymyxin B) were determined for 260 P. aeruginosa isolates. A small part of the isolates showed resistance to 6 β-lactams with the resistance rates ranging from 4.6% (piperacillin–tazobactam) to 9.2% (meropenem). They also showed lower resistance rates for other classes of the antibiotics, such as gentamicin (5.4%, 14/260), amikacin (5.4%, 14/260), and levofloxacin (9.2%, 24/260), and the lowest rate of resistance (1.2%, 3/260) for polymyxin B (Table 2).
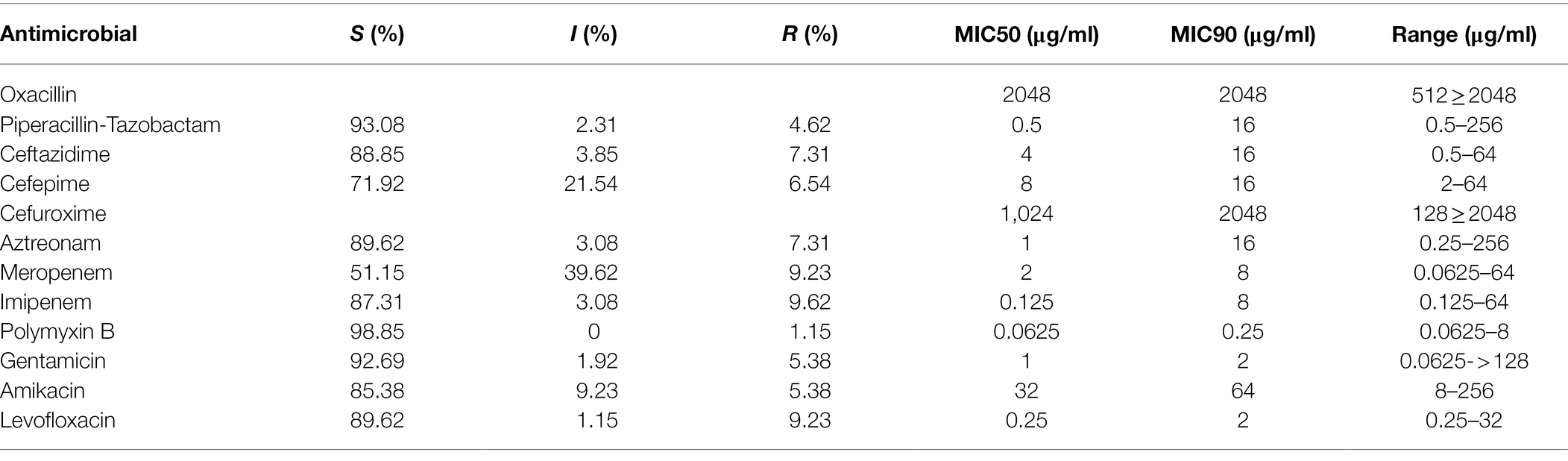
Table 2. Antimicrobial susceptibility of the 260 P. aeruginosa isolates against 12 antimicrobials tested.
Distribution of β-Lactamase Genes
Among the bla genes screened, a total of 44 resistance genes of 7 genotypes from 23 isolates were identified in the 260 P. aeruginosa isolates with blaOXA showing the highest positive rate of 38.6% (17/44). blaCARB ranged the second (27.3%, 12/44), and then blaGES-1 (13.6%, 6/44). The rest 4 (blaTEM, blaIMP, blaPDC, and blaDHA) showed lower positive rates of 4.5% (2/44), 4.5% (2/44), 9.1% (4/44) and 2.3% (1/44), respectively. Among the 23 β-lactamase gene-positive strains, more than a half (52.2%, 12/23) carried 2 resistance genes. Three strains carried 3 resistance genes each and one contained 4 resistance genes, while the rest 7 harbored one each (Table 3). The β-lactamase gene harboring isolates showed higher MIC values for the β-lactam antibiotics, especially for the two carbapenem antibiotics (meropenem and imipenem). All (23/23) of these isolates showed MIC levels ≥8 μg/ml for meropenem or imipenem, with 56.5% (13/23) and 60.9% (14/23) showing MIC levels ≥16 μg/ml for cefepime and ceftazidime, respectively (Table 3). In the β-lactamase gene negative strains, there were 11 strains demonstrating resistance to β-lactam antibiotics including 4 strains (1.7%, 4/237) with MIC levels ≥16 μg/ml for meropenem and/or imipenem.
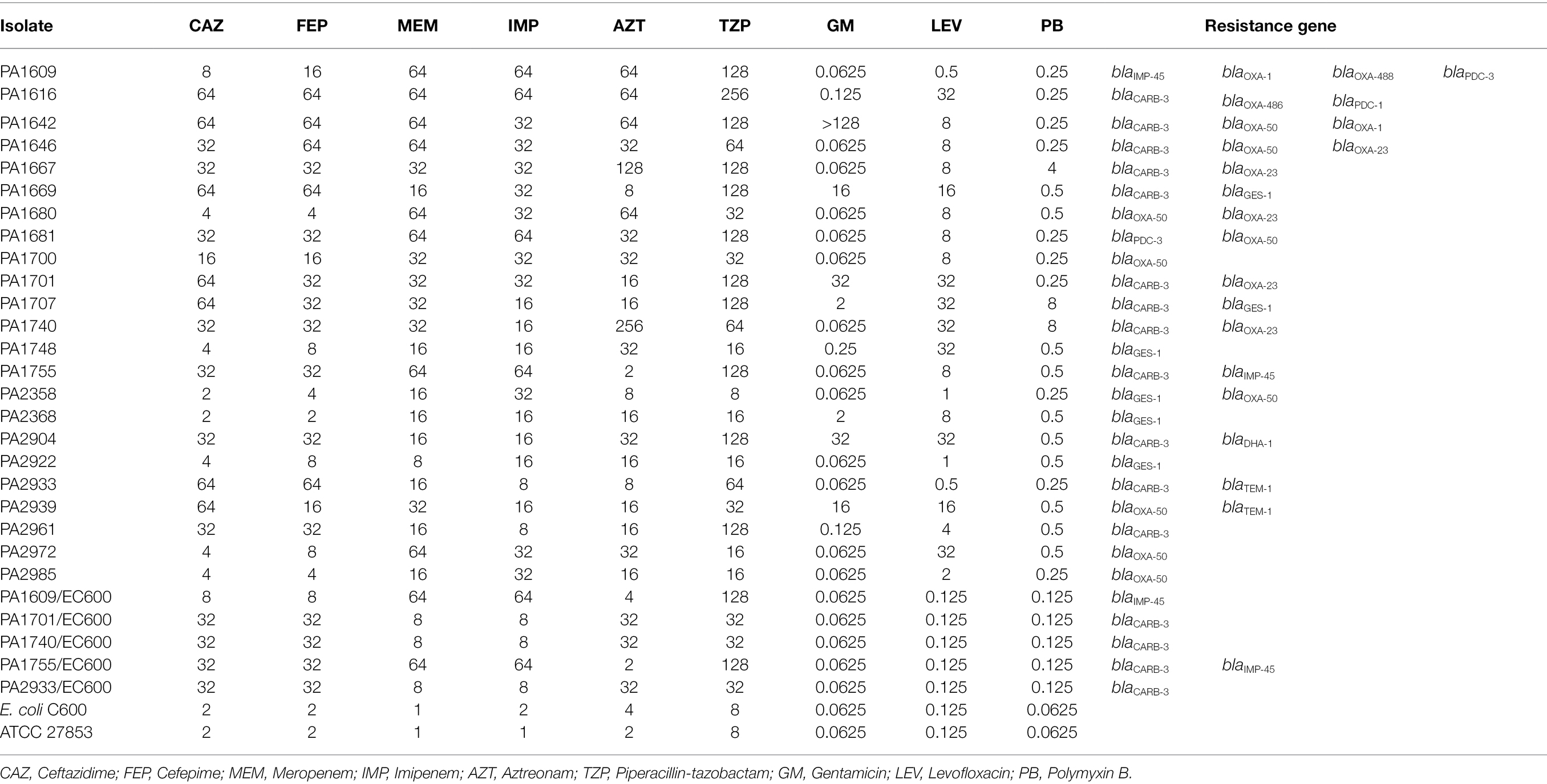
Table 3. Antimicrobial susceptibility and β-lactam resistance gene distribution of the 23 β-lactamase gene-positive P. aeruginosa isolates.
Resistant Plasmid Transferability and Resistance Characteristics
In order to identify the transferability of the β-lactamase genes, we performed conjugation experiments for 5 isolates with high MIC levels to the β-lactam antibiotics. Plasmid detection revealed that they carried one or more large plasmids of more than 100 kb in size. The conjugative plasmids of five strains (PA1609, PA1701, PA1740, PA1755, and PA2933) were successfully transferred to the recipient Escherichia coli C600 (EC600). PCR amplification of the β-lactamase genes of the plasmids extracted from the transconjugants revealed that four (PA1701/EC600, PA1740/EC600, PA1755/EC600, and PA2933/EC600) of them carried a blaCARB-3 gene (including one, PA1755/EC600, co-carrying a blaIMP-45 gene) and the other one (PA1609/EC600) harbored a blaIMP-45 gene which was further confirmed by the whole-genome sequencing of the conjugative plasmid of PA1609 (pPA1609-475; Table 4). The 4 transconjugants with blaCARB-3 exhibited a phenotype of reduced susceptibility to ceftazidime and cefepime (MIC levels of each transconjugant increased up to 16 folds compared to the recipients, respectively), and 2 blaIMP-45 positive transconjugants exhibited a phenotype of reduced susceptibility to carbapenems (MIC levels increased up to 64 folds compared to the recipient; Table 3).
Clonal Relatedness of the 12 blaCARB-3 Carrying Pseudomonas aeruginosa Strains
To investigate possible outbreaks of the bacteria carrying the β-lactamase genes, we used PFGE to perform molecular typing for the 12 blaCARB-3 gene-positive strains. The result showed that the 12 isolates were typable and grouped into 6 clusters designated A to F (Figure 1). The dominant cluster A and B contained 5 and 3 strains, respectively, while the other 4 clusters contained only one strain each.
General Features of the Pseudomonas aeruginosa Genomes
To analyze the molecular structure of the β-lactamase gene-related sequences, the whole-genome sequences of three P. aeruginosa isolates carrying different β-lactamase genes with a wider resistance spectrum and higher MIC levels to β-lactam antibiotics were sequenced. It turned out that the three isolates PA1609, PA1616, and PA1681 carried four, three, and two bla genes, respectively. All the three carried one blaPDC and one or two blaOXA genes and an extra bla genotype was found in PA1609 (blaIMP) and PA1616 (blaCARB), respectively. Besides the β-lactam resistance genes, the three strains also carried several resistance genes of other classes of antibiotics. More resistance genes in the PA1609 genome were encoded on the plasmid. The resistance genes and their locations of the sequenced isolates are shown in Table 4. PA1609 harbored 2 plasmids (a 475 kb plasmid designated pPA1609-475 with blaIMP-45, blaOXA-1, aac(6′)-Ib7, and so on, and a 47 kb plasmid free of the resistance gene). PA1616 and PA1681 were free of the plasmid.
Comparative Genomic Analysis of blaIMP-45, blaCARB-3, and blaPDC-3 Related Sequences
Searching the nucleotide sequence data in the NCBI database, four plasmids with the highest percentage of nucleotide sequence similarities with pPA1609-475 from two Pseudomonas species, namely, plasmids of pBM413, pPAG5, pSY153-MDR, and pA681-IMP were retrieved (Figure 2). The blaIMP-45 encoding plasmid pPA1609-475 harbored a total of 14 resistance genes which clustered in two regions with region A of 25 kb in length (position 265–290 kb) and region B of 25 kb in length (position 310–335 kb). These resistance genes were related to the mobile genetic elements including two class 1 integrons and several transposons or insertion sequences. One class 1 integron which contained blaIMP-45 encoded 6 resistance genes (265–290 kb), and the other one contained 5 resistance genes (310–335 kb; Figures 1, 3).
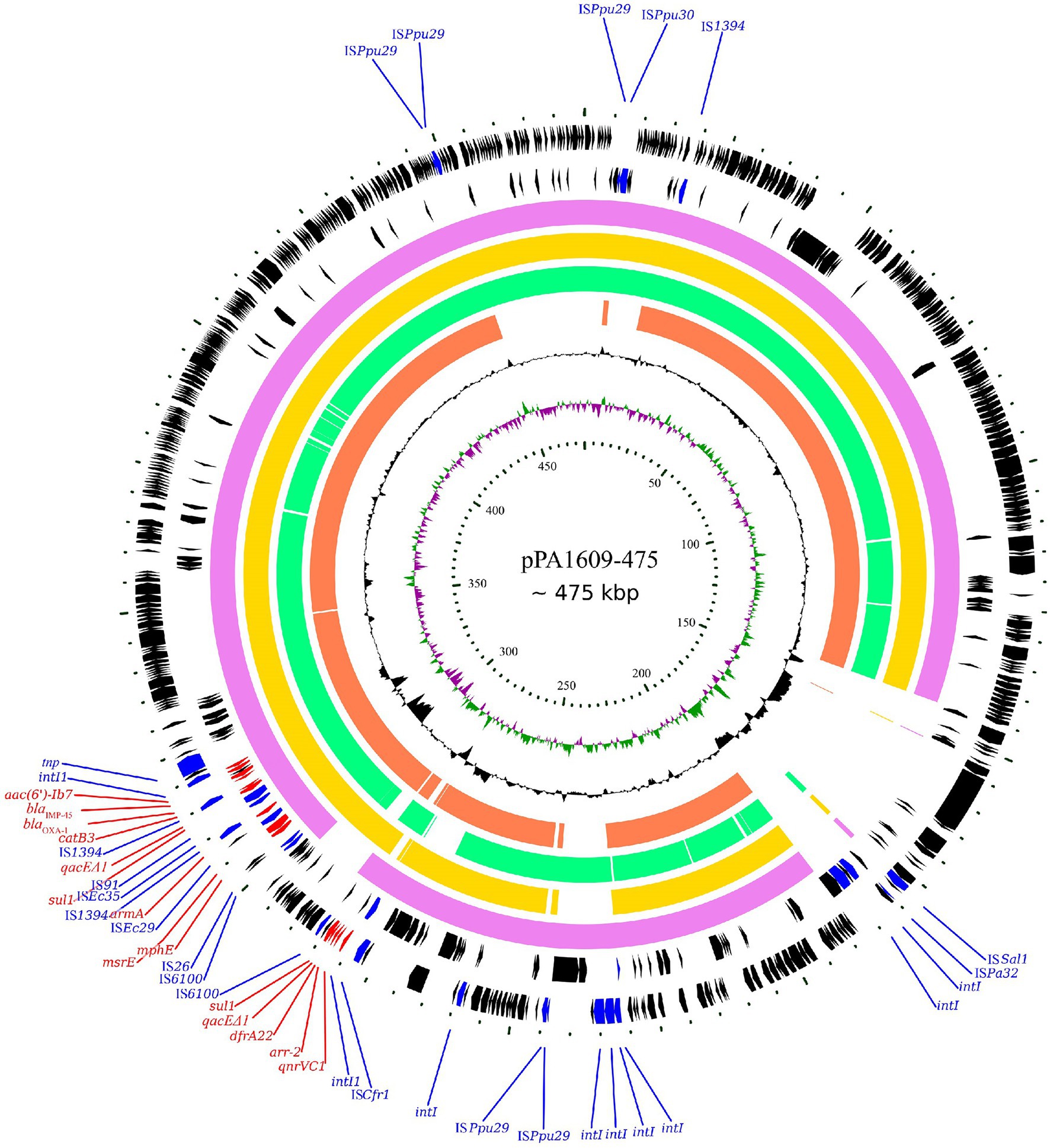
Figure 2. Complete genome sequence of the pPA1609-475 plasmid and comparative genomic analysis of the pPA1609-475 plasmid sequence with other homologous sequences. The circles (from innermost to outermost) represent (i) the scale in kb; (ii) the cumulative GC skew of pPA1609-475; (iii) the GC content of pPA1609-475; (iv) four homologous plasmids pA681-IMP (MF344570.1 from Pseudomonas aeruginosa), pPAG5 (CP045003.1 from P. aeruginosa), pBM413 (CP016215.1 from P. aeruginosa) and pSY153-MDR (KY883660.1 from P. putida), respectively; (v) the annotated coding sequences of pPA1609-475 with selected genes indicated according to the gene function: antimicrobial resistance genes in red arrows, transposase genes and IS elements in blue arrows.
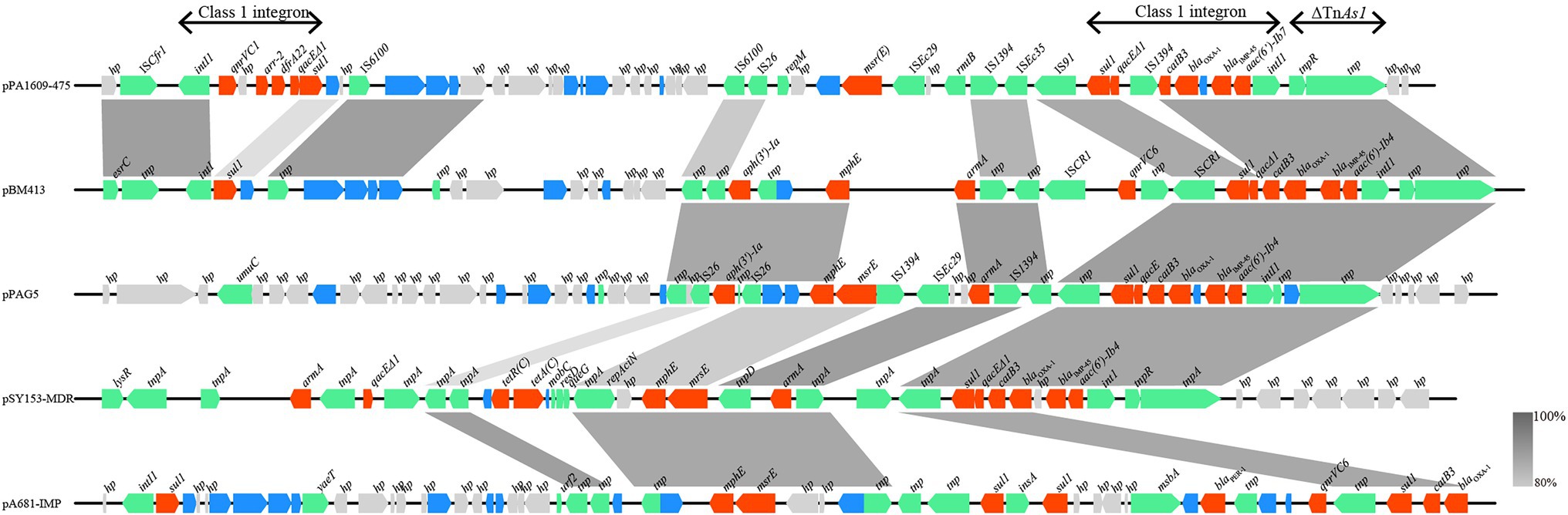
Figure 3. Comparative genomic analysis of the resistance gene regions of pPA1609-475 with other sequences from different bacteria. The homologous gene clusters among the plasmids pPA1609-475, pBM413 (CP016215.1), pPAG5 (CP045003.1), pSY153-MDR (KY883660.1), and pA681-IMP (MF344570.1) with the resistance gene clusters in red. pPA1609-475 contained two class 1 integrons with the blaIMP-45 encoding one carrying 6 resistance genes (intI1-blaIMP-45-blaOXA-1-aac(6′)-Ib7-catB3-qacE∆1-sul1), and the other one contained 5 resistance genes (intI1-qnrVC1-aar-2-dfrA22-qacE∆1-sul). Annotated coding sequences are displayed as arrows. Coding sequences are colored based on their assigned gene functions. The genes in blue are those without direct gene names. The grey shading areas indicate homologous regions (identity >80%). hp., hypothetical protein.
As mentioned above, the blaCARB-3 of P. aeruginosa PA1616 and blaPDC-3 of P. aeruginosa PA1681 were in the chromosomes. The blaCARB-3 gene was related to a class 1 integron. The comparative genomic analysis of the area of about 65 kb in length with blaCARB-3 carrying integron at the center showed that only two other sequence fragments carrying the integrons and with the sequence identity 100% and coverage ≥96% to that of PA1616 were available in the NCBI nucleotide database. The sequence of the clone fosmid AMO9 of Achromobacter xylosoxidans X02736 (JX448550.1) contained the same integron structure as that of P. aeruginosa PA1616, while the integron encoded in the sequence of P. aeruginosa strain SE5443 chromosome (CP046405.1) contained a VIM-2 gene inserted between grol and ant(2”)Ia (Figures 4, 5).
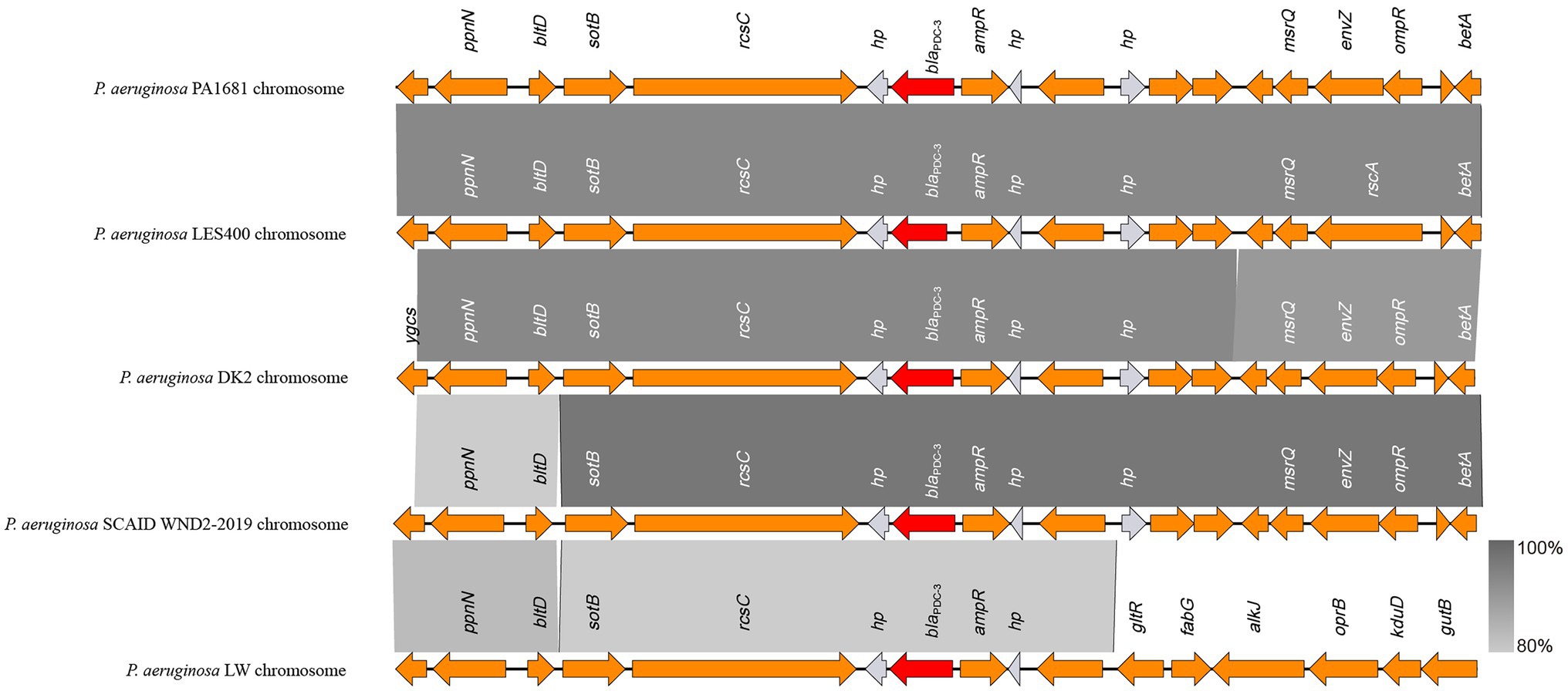
Figure 4. Comparative genomic analysis of the blaPDC-3-encoding fragment of PA1681 with other sequences from Pseudomonas aeruginosa strains: P. aeruginosa LES400 (CP006982.1), P. aeruginosa DK2 (CP003149.1), P. aeruginosa SCAID WND2-2019 (CP041786.1), and P. aeruginosa LW (CP022478.1). Annotated coding sequences are displayed as arrows. The grey shading areas indicate homologous regions (identity >80%). hp., hypothetical protein.
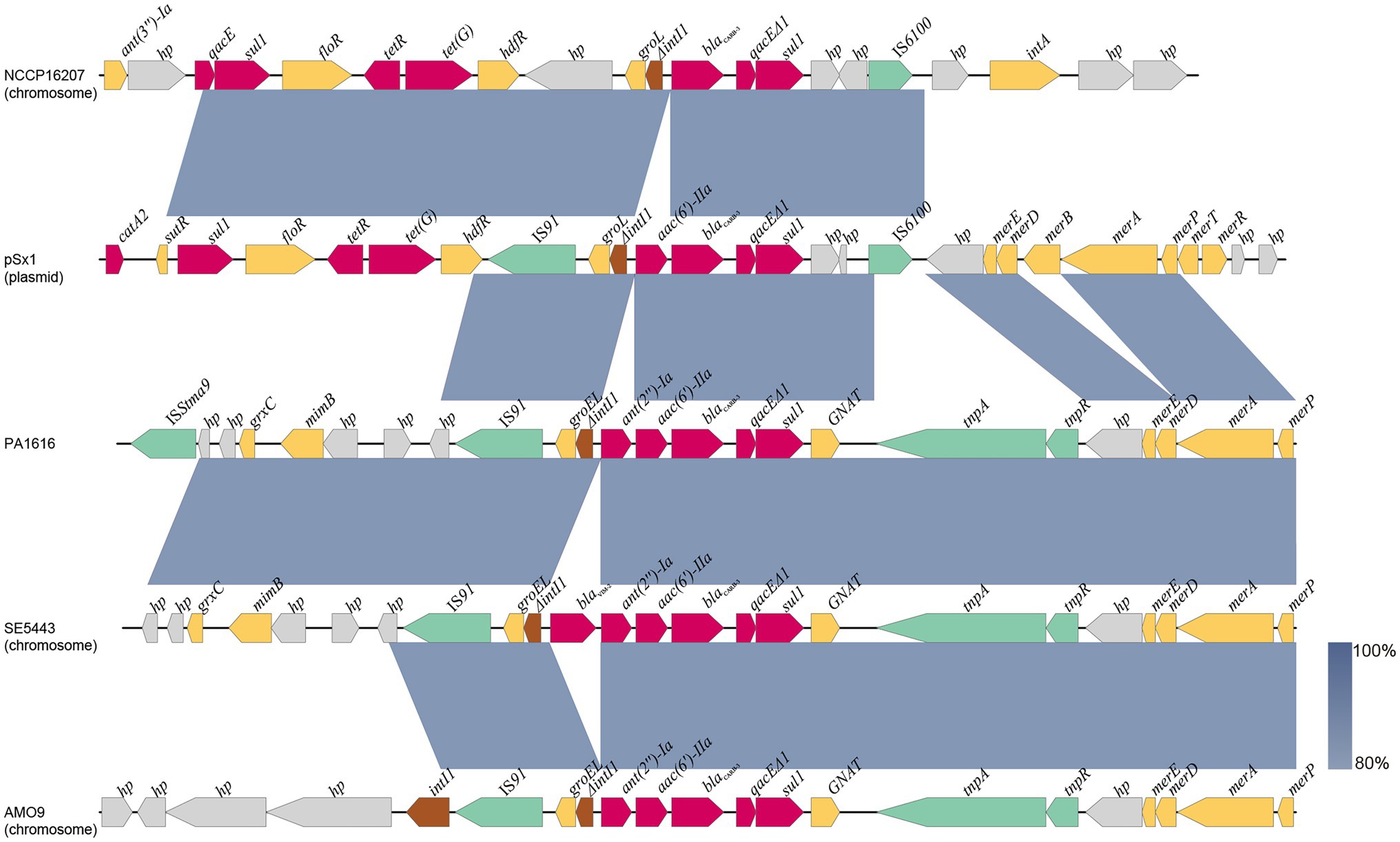
Figure 5. Comparative analysis of the blaCARB-3 of PA1616 encoding region with other sequences from different bacteria: Achromobacter xylosoxidans AMO9 (JX448550.1), P. aeruginosa SE5443 (CP046405.1), Salmonella enterica NCCP16207 (CP041976.1), and Shewanella xiamenensis plasmid pSx1 (CP013115.1). The blaCARB-3 genes were all related to class 1 integrons. Coding sequences are colored based on their assigned gene functions. The blue shading areas indicate homologous regions (identity >80%). hp., hypothetical protein.
Discussion
In this study, a total of 7 bla genotypes of all Amber classes including classes A, B, C, and D genes were identified in 23 of 260 clinical P. aeruginosa isolates, of which blaCARB-3 was the most prevalent with 4.6% (12/260) of the isolates carrying it. The PFGE data indicated that all 12 P. aeruginosa strains bearing blaCARB-3 in this study were divided into 6 groups. Except two groups included 5 and 3 strains, respectively, the other four groups contain only one isolate each. This result not only demonstrated the distribution of the blaCARB-3 gene over the pathogens of different genetic backgrounds but also the potential epidemic threat of blaCARB-3 positive P. aeruginosa isolates over the hospital populations.
In this study, two strains (0.8%, 2/260) carrying blaIMP45 showed higher MIC levels to meropenem and imipenem (both 64 μg/ml). As less frequently, but much powerful carbapenem-hydrolyzing enzyme in P. aeruginosa, IMP45 belongs to the class B β-lactamase (a metallo-β-lactamase, MBLs). On the other hand, seven strains carrying blaGES-1 showed weak carbapenem resistance. blaGES-1 was first found in a Klebsiella pneumoniae strain isolated from a patient hospitalized in French Guiana (GES stands for Guiana ESBL) in 1998, which has been identified in Enterobacteriaceae (K. pneumoniae, Enterobacter cloacae, or Escherichia coli) and P. aeruginosa. The other variant of the GES has been found to have strong hydrolyzing activities against the β-lactams including blaGES-2, blaGES-5, blaGES-24, and blaGES-11, especially blaGES-5 (Liu et al., 2018b; Xu et al., 2018).
Besides 12 isolates with 2 β-lactamase genotypes, 4 strains carried three or four different β-lactamase genotypes or subgenotypes of blaCARB, blaOXA, blaIMP, and blaPDC (all isolates containing 1 or 2 blaOXA genes, 3 with a blaCARB gene, 2 with a blaPDC gene, and 1 carrying a blaIMP-45 gene). Until recently, no similar drug resistance gene combinations, particularly co-occurrence with blaCARB-3 were reported. These strains showed a wider resistance spectrum and higher resistance levels to the β-lactam antibiotics tested in this study. Co-occurrence of β-lactamase genes displays high rates of resistance to broad-spectrum cephalosporins owing to induced expression of ampC β-lactamase gene, and an increasing number of organisms harboring plasmid-encoded extended-spectrum b-lactamase (ESBL) or/and carbapenemase genes have been described (Jahan et al., 2020). Co-occurrence of β-lactamase genes has been reported worldwide. The most common is the co-occurrence of two different β-lactamase genes, in particular, the combination of two different blaOXA variants is very common. Co-occurrence of three or more β-lactamase genes is very rare. The emergence of multidrug-resistant K. pneumoniae isolates, which produces blaVIM-4, blaCTX-M-15, blaTEM-1, blaCMY-4 have been reported from France (Ktari et al., 2006). Co-occurrence of blaNDM-1 with blaOXA-23 or blaOXA-58 in clinical multidrug-resistant Acinetobacter baumannii isolates was also found in Algeria (Ramoul et al., 2016).
The whole-genome sequencing of three isolates (PA1609, PA1616, and PA1681) demonstrated that most of the resistance genes were related to the mobile genetic elements and might be located on the plasmids. The resistance genes of pTL1609-475 (an IncFII-like plasmid) were mainly clustered in two MDR (multidrug-resistant) regions, while in the other plasmids with the most similarity with that of pTL1609-475, only one MDR region was identified. The MDR region of PA1609 encoding a class 1 integron which carried both blaIMP-45 and blaOXA-1 were similar to those on the plasmid pBM413 (Liu et al., 2018a), pPAG5, and pSY153. It has been reported that the IncFII-like plasmid had superiority to capture blaIMP45 by mobile gene elements, resulting in gradual acquisition or accumulation of carbapenem resistance in ST847 E. coli (Wang et al., 2020). Compared with pBM413 and other plasmids with similar MDR regions, MDR of pTL1609-475 had undergone much more massive insertions of foreign resistance genetic contents and showed a higher degree of genomic plasticity.
The blaPDC genes of PA1616 and PA1681 were located in the chromosomes, of which the one of PA1681 was carried by a class 1 integron, while the other in PA1616 was related to a TnAs2 transposon. TnAs2, a derivative of Tn3, was first identified in Aeromonas salmonicida (Zamora-Lagos et al., 2015), and then Escherichia coli, which has also been reported to carry other resistance genes, such as aacA4 and qnrVC6 in addition to blaIMP45 in a region derived from a class 1 integron. More interestingly, we identified the complete structure of IntI1 coupled with TnAs2 transposon in plasmid genomes rather than only the IntI1-derived ARGs. Our results indicated the high possibility of horizontal transmission of ARGs by IntI1 through plasmids. In addition, compared with SE5443, an ISStma9 element adjacent to blaCARB-3 was identified in PA1616, which might indicate the high possibility of horizontal transmission of ARGs by IntI1 from different DNA molecules.
Conclusion
In this work, among the 23 strains that were positive for the β-lactamase gene, most carried two or more β-lactam resistance genes. The blaCARB-3 gene is the most common β-lactam resistance gene in those isolates and some blaCARB-3 positive isolates presented clonal relatedness. The β-lactamase gene was related to the mobile genetic elements, such as class 1 integron, located both on plasmids and chromosomes. The β-lactamase gene carrying plasmid was transferable and the transconjugant with blaCARB-3 exhibited the phenotype of reduced susceptibility to piperacillin–tazobactam, ceftazidime, and cefepime, while the two co-carrying blaIMP-45 also exhibited reduced susceptibility to carbapenems. These results suggest that resistance to β-lactam antibiotics and clonal dissemination is prevalent in the hospital population of P. aeruginosa.
Data Availability Statement
The datasets presented in this study can be found in online repositories. The names of the repository/repositories and accession number(s) can be found in the article/supplementary material.
Ethics Statement
Individual patient data were not involved, and only anonymous clinical residual samples during routine hospital laboratory procedures were used in this study. This study followed the principles stated in the Declaration of Helsinki (https://www.wma.net/policies-post/wma-declaration-of-helsinki-ethical-principles-for-medical-research-involving-human-subjects/) and was approved by the Second Affiliated Hospital and Yuying Children’s Hospital of Wenzhou Medical University.
Author Contributions
TX, CL, and QB designed the study. HL, CF, TZ, QL, and XZ acquired the data. HL, CF, AL, SL, LZ, LL, and JL performed the results analysis and interpreted the data. HL and QB wrote the first draft of the paper. KL, HZ, and TX revised it critically for important intellectual content. All authors contributed to the article and approved the submitted version.
Funding
This study was supported by the Science & Technology Project of Wenzhou City, China (N20210001 and Y20210003), Zhejiang Provincial Natural Science Foundation of China (LY19C060002 and LQ17H190001), and the National Natural Science Foundation of China (81973382, 81960381, and 81700011).
Conflict of Interest
The authors declare that the research was conducted in the absence of any commercial or financial relationships that could be construed as a potential conflict of interest.
Publisher’s Note
All claims expressed in this article are solely those of the authors and do not necessarily represent those of their affiliated organizations, or those of the publisher, the editors and the reviewers. Any product that may be evaluated in this article, or claim that may be made by its manufacturer, is not guaranteed or endorsed by the publisher.
Acknowledgments
The authors thank all the colleagues and the reviewers who helped this work.
Footnotes
1. ^https://github.com/voutcn/megahit
2. ^https://github.com/marbl/canu
3. ^https://github.com/broadinstitute/pilon
4. ^https://github.com/hyattpd/Prodigal
5. ^http://arpcard.mcmaster.ca
6. ^https://cge.cbs.dtu.dk/services/ResFinder/
8. ^https://www.expasy.org/resources/uniprotkb-swiss-prot
9. ^https://www.ncbi.nlm.nih.gov/refseq/
10. ^https://github.com/thanhleviet/ISfinder-sequences
11. ^http://integrall.bio.ua.pt/
12. ^http://transposon.lstmed.ac.uk/
13. ^https://www.r-project.org/
15. ^http://blast.ncbi.nlm.nih.gov/Blast.cgi
17. ^https://mafft.cbrc.jp/alignment/software/
References
Al Dawodeyah, H. Y., Obeidat, N., Abu-Qatouseh, L. F., and Shehabi, A. A. (2018). Antimicrobial resistance and putative virulence genes of Pseudomonas aeruginosa isolates from patients with respiratory tract infection. GERMS 8, 31–40. doi: 10.18683/germs.2018.1130
Bannerman, T. L., Hancock, G. A., Tenover, F. C., and Miller, J. M. (1995). Pulsed-field gel electrophoresis as a replacement for bacteriophage typing of Staphylococcus aureus. J. Clin. Microbiol. 33, 551–555. doi: 10.1128/JCM.33.3.551-555.1995
Barnes, M. D., Bethel, C. R., Alsop, J., Becka, S. A., Rutter, J. D., Papp-Wallace, K. M., et al. (2018). Inactivation of the pseudomonas-derived Cephalosporinase-3 (PDC-3) by relebactam. Antimicrob. Agents Chemother. 62, e02406–e02417. doi: 10.1128/AAC.02406-17
Bush, K., and Jacoby, G. A. (2010). Updated functional classification of β-lactamases. Antimicrob. Agents Chemother. 54, 969–976. doi: 10.1128/AAC.01009-09
Codjoe, F. S., Brown, C. A., Smith, T. J., Miller, K., and Donkor, E. S. (2019). Genetic relatedness in carbapenem-resistant isolates from clinical specimens in Ghana using ERIC-PCR technique. PLoS One 14:e0222168. doi: 10.1371/journal.pone.0222168
Culebras, E., González-Romo, F., Head, J., Gómez, M., Morales, G., and Picazo, J. J. (2010). Outbreak of Acinetobacter baumannii producing OXA-66 in a Spanish hospital: epidemiology and study of patient movements. Microb. Drug Resist. 16, 309–315. doi: 10.1089/mdr.2009.0113
Fallah, F., Borhan, R. S., and Hashemi, A. (2013). Detection of bla (IMP) and bla (VIM) metallo-β-lactamases genes among Pseudomonas aeruginosa strains. Int. J. Burns Trauma 3, 122–124.
Ghamgosha, M., Shahrekizahedani, S., Kafilzadeh, F., Bameri, Z., Taheri, R. A., and Farnoosh, G. (2015). Metallo-β-lactamase VIM-1, SPM-1, and IMP-1 genes among clinical Pseudomonas aeruginosa species isolated in Zahedan. Iran. Jundishapur J. Microbiol. 8:e17489. doi: 10.5812/jjm.8(4)2015.17489
He, J., Sun, L., Zhang, L., Leptihn, S., Yu, Y., and Hua, X. (2021). A Novel SXT/R391 Integrative and Conjugative Element Carries Two Copies of theblaNDM-1Gene in Proteus mirabilis. mSphere. 6:e0058821. doi: 10.1128/mSphere.00588-21
Jahan, M. I., Rahaman, M. M., Hossain, M. A., and Sultana, M. (2020). Occurrence of intI1-associated VIM-5 carbapenemase and co-existence of all four classes of β-lactamase in carbapenem-resistant clinical Pseudomonas aeruginosa DMC-27b. J. Antimicrob. Chemother. 75, 86–91. doi: 10.1093/jac/dkz426
Keith, P. (2011). Pseudomonas aeruginosa: resistance to the max. Front. Microbiol. 2:65. doi: 10.3389/fmicb.2011.00065
Ktari, S., Arlet, G., Mnif, B., Gautier, V., Mahjoubi, F., Ben Jmeaa, M., et al. (2006). Emergence of multidrug-resistant Klebsiella pneumoniae isolates producing VIM-4 metallo-β-lactamase, CTX-M-15 extended-spectrum β-lactamase, and CMY-4 AmpC β-lactamase in a Tunisian university hospital. Antimicrob. Agents Chemother. 50, 4198–4201. doi: 10.1128/AAC.00663-06
Lachapelle, J., Dufresne, J., and Levesque, R. C. (1991). Characterization of the blaCARB-3 gene encoding the carbenicillinase-3 beta-lactamase of Pseudomonas aeruginosa. Gene 102, 7–12. doi: 10.1016/0378-1119(91)90530-O
Lee, C.-H., Liu, J.-W., Li, C.-C., Chien, C.-C., Tang, Y.-F., and Su, L.-H. (2011). Spread of ISCR1 elements containing blaDHA-1 and multiple antimicrobial resistance genes leading to increase of flomoxef resistance in extended-Spectrum-β-lactamase-producing Klebsiella pneumoniae. Antimicrob. Agents Chemother. 55, 4058–4063. doi: 10.1128/AAC.00259-11
Liu, J., Li, L., Peters, B. M., Li, B., Chen, D., Xu, Z., et al. (2018a). Complete genomic analysis of multidrug-resistance Pseudomonas aeruginosa Guangzhou-Pae617, the host of megaplasmid pBM413. Microb. Pathog. 117, 265–269. doi: 10.1016/j.micpath.2018.02.049
Liu, J., Yang, L., Chen, D., Peters, B. M., Li, L., and Li, B. (2018b). Complete sequence of pBM413, a novel multidrug resistance megaplasmid carrying qnrVC6 and Bla IMP-45 from Pseudomonas aeruginosa. Int. J. Antimicrob. Agents 51, 145–150. doi: 10.1016/j.ijantimicag.2017.09.008
Livermore, D. M., and Woodford, N. (2006). The β-lactamase threat in Enterobacteriaceae, pseudomonas and Acinetobacter. Trends Microbiol. 14, 413–420. doi: 10.1016/j.tim.2006.07.008
Mikhail, S., Singh, N. B., Kebriaei, R., Rice, S. A., Stamper, K. C., Castanheira, M., et al. (2019). Evaluation of the synergy of Ceftazidime-Avibactam in combination with Meropenem, Amikacin, Aztreonam, Colistin, or Fosfomycin against well-characterized multidrug-resistant Klebsiella pneumoniae and Pseudomonas aeruginosa. Antimicrob. Agents Chemother. 63, e00779–e00719. doi: 10.1128/AAC.00779-19
Mohammadpour, B., Rouhi, S., Moradi, M., Ramazanzadeh, R., Saniyi, E., Zandi, S., et al. (2019). Prevalence of metallo-β-lactamases in Acinetobacter baumannii in Iran: A review and meta-analysis. Infect. Disord. Drug Targets 19, 350–361. doi: 10.2174/1871526518666181016101430
Philippon, A. M., Paul, G. C., Thabaut, A. P., and Jacoby, G. A. (1986). Properties of a novel carbenicillin-hydrolyzing β-lactamase (CARB-4) specified by an IncP-2 plasmid from Pseudomonas aeruginosa. Antimicrob. Agents Chemother. 29, 519–520. doi: 10.1128/AAC.29.3.519
Poirel, L., Le Thomas, I., Naas, T., Karim, A., and Jacoby, P. (2000). Biochemical sequence analyses of GES-1, a novel class A extended-Spectrum β-lactamase, and the class 1 integron In52 from Klebsiella pneumoniae. Antimicrob. Agents Chemother. 44, 622–632. doi: 10.1128/AAC.44.3.622-632.2000
Queenan, A. M., and Bush, K. (2007). Carbapenemases: the versatile β-lactamases. Clin. Microbiol. Rev. 20, 440–458. doi: 10.1128/CMR.00001-07
Ramoul, A., Loucif, L., Bakour, S., Rolain, J. M., Dekhil, M., and Rolain, J. M. (2016). Co-occurrence of blaNDM-1 with blaOXA-23 or blaOXA-58 in clinical multidrug-resistant Acinetobacter baumannii isolates in Algeria. J. Glob. Antimicrob. Resist. 6, 136–141. doi: 10.1016/j.jgar.2016.05.003
Rodríguez-Martínez, J. M., Poirel, L., and Nordmann, P. (2009). Extended-spectrum cephalosporinases in Pseudomonas aeruginosa. Antimicrob. Agents Chemother. 53, 1766–1771. doi: 10.1128/AAC.01410-08
Shaikh, S., Fatima, J., Shakil, S., Rizvi, S. M., and Kamal, M. A. (2015). Antibiotic resistance and extended spectrum β-lactamases: types, epidemiology and treatment. Saudi J. Biol. Sci. 22, 90–101. doi: 10.1016/j.sjbs.2014.08.002
Subedi, D., Vijay, A. K., and Willcox, M. (2018). Overview of mechanisms of antibiotic resistance in Pseudomonas aeruginosa: an ocular perspective. Clin. Exp. Optom. 101, 162–171. doi: 10.1111/cxo.12621
Sun, H., Xiao, G., Zhang, J., Pan, Z., Chen, Y., and Xiong, F. (2019). Rapid simultaneous detection of Bla (oxa-23), Ade-B, int-1, and ISCR-1 in multidrug-resistant Acinetobacter baumannii using single-tube multiplex PCR and high resolution melting assay. Infect. Drug Resist. 12, 1573–1581. doi: 10.2147/IDR.S207225
Wang, Y., Wang, X., Schwarz, S., Zhang, R., Lei, L., Liu, X., et al. (2014). IMP-45-producing multidrug-resistant Pseudomonas aeruginosa of canine origin. J. Antimicrob. Chemother. 69, 2579–2581. doi: 10.1093/jac/dku133
Wang, M., Zeng, Z., Jiang, F., Zheng, Y., Shen, H., Macedo, N., et al. (2020). Role of enterotoxigenic Escherichia coli prophage in spreading antibiotic resistance in a porcine-derived environment. Environ. Microbiol. 22, 4974–4984. doi: 10.1111/1462-2920.15084
Watanabe, M., Iyobe, S., Inoue, M., and Mitsuhashi, S. (1991). Transferable imipenem resistance in Pseudomonas aeruginosa. Antimicrob. Agents Chemother. 35, 147–151. doi: 10.1128/AAC.35.1.147
Wolter, D. J., and Lister, P. D. (2013). Mechanisms of β-lactam resistance among Pseudomonas aeruginosa. Curr. Pharm. Des. 19, 209–222. doi: 10.2174/138161213804070311
Wong, M. H.-Y., Chan, B. K.-W., Chan, E. W.-C., and Chen, S. (2019). Over-expression of ISAba1-linked intrinsic and exogenously acquired OXA type Carbapenem-hydrolyzing-class D-ß-lactamase-encoding genes is key mechanism underlying Carbapenem resistance in Acinetobacter baumannii. Front. Microbiol. 10:2809. doi: 10.3389/fmicb.2019.02809
Xu, T., Wang, J., Ying, J., Zhu, T., Liu, Y., Xu, L., et al. (2018). Characterisation of a class 1 integron associated with the formation of quadruple bla GES-5 cassettes from an IncP-1β group plasmid in Pseudomonas aeruginosa. Int. J. Antimicrob. Agents 52, 485–491. doi: 10.1016/j.ijantimicag.2018.07.002
Yamamoto, K., Toya, S., Sabidi, S., Hoshiko, Y., and Maeda, T. (2021). Diluted Luria-Bertani medium vs. sewage sludge as growth media: comparison of community structure and diversity in the culturable bacteria. Appl. Microbiol. Biotechnol. 105, 3787–3798. doi: 10.1007/s00253-021-11248-4
Zamora-Lagos, M. A., Pfeiffer, F., Habermann, B., and Gruber, S. (2015). Blettinger M. ISfinder. Available at: https://www-is.biotoul.fr/scripts/ficheIS.php?name=TnAs2 (Accessed July 30, 2020).
Keywords: Pseudomonas aeruginosa, resistance, antimicrobial susceptibility test, β-lactamase gene, pulsed-field gel electrophoresis
Citation: Lin H, Feng C, Zhu T, Li A, Liu S, Zhang L, Li Q, Zhang X, Lin L, Lu J, Lin X, Li K, Zhang H, Xu T, Li C and Bao Q (2022) Molecular Mechanism of the β-Lactamase Mediated β-Lactam Antibiotic Resistance of Pseudomonas aeruginosa Isolated From a Chinese Teaching Hospital. Front. Microbiol. 13:855961. doi: 10.3389/fmicb.2022.855961
Edited by:
Yuji Morita, Meiji Pharmaceutical University, JapanReviewed by:
Iain Lamont, University of Otago, New ZealandTaha Menasria, University of Tébessa, Algeria
Copyright © 2022 Lin, Feng, Zhu, Li, Liu, Zhang, Li, Zhang, Lin, Lu, Lin, Li, Zhang, Xu, Li and Bao. This is an open-access article distributed under the terms of the Creative Commons Attribution License (CC BY). The use, distribution or reproduction in other forums is permitted, provided the original author(s) and the copyright owner(s) are credited and that the original publication in this journal is cited, in accordance with accepted academic practice. No use, distribution or reproduction is permitted which does not comply with these terms.
*Correspondence: Teng Xu, eHV0ZW5nQHdtdS5lZHUuY24=; Changchong Li, d3psaWNoY2hAMTYzLmNvbQ==; Qiyu Bao, YmFvcXlAZ2Vub21pY3MuY24=
†These authors have contributed equally to this work