- Department of Biotechnology, Thapar Institute of Engineering and Technology, Patiala, India
Soil heavy metal (HM) pollution, which arises from natural and anthropogenic sources, is a prime threat to the environment due to its accumulative property and non-biodegradability. Ectomycorrhizal (ECM) symbiosis is highly efficient in conferring enhanced metal tolerance to their host plants, enabling their regeneration on metal-contaminated lands for bioremediation programs. Numerous reports are available regarding ECM fungal potential to colonize metal-contaminated lands and various defense mechanisms of ECM fungi and plants against HM stress separately. To utilize ECM–plant symbiosis successfully for bioremediation of metal-contaminated lands, understanding the fundamental regulatory mechanisms through which ECM symbiosis develops an enhanced metal tolerance in their host plants has prime importance. As this field is highly understudied, the present review emphasizes how plant’s various defense systems and their nutrient dynamics with soil are affected by ECM fungal symbiosis under metal stress, ultimately leading to their host plants ameliorated tolerance and growth. Overall, we conclude that ECM symbiosis improves the plant growth and tolerance against metal stress by (i) preventing their roots direct exposure to toxic soil HMs, (ii) improving plant antioxidant activity and intracellular metal sequestration potential, and (iii) altering plant nutrient uptake from the soil in such a way to enhance their tolerance against metal stress. In some cases, ECM symbiosis promotes HM accumulation in metal stressed plants simultaneous to improved growth under the HM dilution effect.
Introduction
The advancements in human technologies such as industrialization and urbanization increase the soil heavy metal (HM) pollution. HMs can also leach down into groundwater or can be transferred to the successive levels of the food chain (Khosla and Reddy, 2014), causing a significant threat to living organisms and the environment (Vaclavikova et al., 2008; Nagajyoti et al., 2010). Both anthropogenic sources (such as industrialization, agriculture, sewage sludge, traffic emissions, and untreated wastewater) and natural sources (such as volcanic eruptions, rock weathering, and windblown dust) can contribute to the soil HM pollution (Srivastava et al., 2017). HMs are mainly categorized as essential or non-essential based on their role in various biological functions such as cell structure stabilization and enzyme catalysis (Bruins et al., 2000). The non-essential HMs are not required for cell metabolisms and are highly toxic for cells even in trace amounts (Haferburg and Kothe, 2007). HM toxicity in plants can reduce plant biomass, seed germination, fruit yield, nutrition content, and root and shoot length and induce chlorosis and mortality (Rai et al., 2021). The plant immune system, production of photosynthetic pigments such as carotenoids, chlorophylls, and reactive oxygen species (ROS) scavenging systems is also predominantly affected in the plants subjected to the high concentrations of HMs (Rastgoo and Alemzadeh, 2011; Ghnaya et al., 2013). HM toxicity can induce oxidative stress in plants (Khator and Shekhawat, 2020), further damaging cellular biomolecules such as protein and nucleic acids (Romero-Puertas et al., 2019). HM stress in plants leads to the impaired growth of primary root and root hairs (Broadley et al., 2007; Hayat et al., 2012; Feleafel and Mirdad, 2013), thus resulting in reduced water uptake efficiency of host plants (Rucińska-Sobkowiak, 2016). The content of HMs uptake varies with plant species and depends on environmental factors such as temperature, pH, nutrients, and moisture. For example, the accumulation of metal ions in Beta vulgaris is higher in summers than in winter due to the relatively high transpiration rates (Sharma et al., 2007). From plants, the metal can enter into higher trophic levels of food chains such as insects, herbivores, and humans, resulting in the ecosystem and food chains imbalance (Zhang et al., 2017). HM toxicity reduces the growth of plants in terms of dry weight and height, which can be improved by plant symbiotic association with ectomycorrhizal (ECM) fungi. The transmission of HMs from soil to plants is highly influenced by the presence of ECM fungal partners in the symbiosis with plant roots (Tang et al., 2019; Sun et al., 2021).
Ectomycorrhizal fungi are ubiquitous symbionts of plants, predominately found in Boreal and Temperate biomes. They colonize the roots of a wide range of woody plants such as Eucalyptus, Pinus, Acacia, and Picea (Smith and Read, 2010). ECM fungi play a critical role in nutrient dynamics of the terrestrial ecosystem by facilitating the mobilization of soil unavailable nutrients and water to host plants in return to their photosynthesis driven carbon (Smith and Read, 2010; Van der Heijden et al., 2015; Hodge, 2017). ECM fungi possess highly efficient and diverse defense mechanisms against HM stress, allowing them to thrive on metal-polluted lands (Khullar and Reddy, 2018). They enhance the tolerance of host plants against metal stress by various mechanisms and play a critical role in the bioremediation of metal-contaminated lands (Jourand et al., 2014; Reddy et al., 2016; Liu B. et al., 2020).
Ectomycorrhizal fungi in symbiosis develop into extramatrical mycelia growing in the soil surrounding the rhizosphere, aggregated fungal hyphae to ensheath lateral roots called as a mantle, and hyphae penetrating the apoplastic zones of cortical and epidermal cells of the host roots named as Hartig net (Figure 1; Martin et al., 2016). The extramatrical hyphae, the potential sinks for host plant-derived carbon, act as an essential candidate for delivering the carbon to the soil. They also play a significant function in N dynamics (Wu, 2011), P uptake (Cairney, 2011), and mineral weathering (Landeweert et al., 2001; Rosling, 2009). The immense networks of ECM fungal mycelia in the soil can also link the root tips of different tree species. The labeled carbon (13C) derived from tree Picea can transfer to the surrounding trees through ECM mycelia networks, where the exchange is found to be higher with phylogenetically related trees. The ECM communities among the phylogenetically related trees are very similar in composition (Rog et al., 2020). The diversity of ECM fungi is significantly determined by edaphic factors such as (i) soil moisture (Jarvis et al., 2015), pH, carbon, and N and P content (Veach et al., 2018); (ii) type of host (Tedersoo et al., 2014; Saitta et al., 2018), host age (Zhang et al., 2014), and host genotype (Wang et al., 2018); and (iii) environmental factors such as climatic gradients (Steidinger et al., 2020), location coordinates on mountain slopes (Wei et al., 2020), light availability (Kummel and Lostroh, 2011), and canopy and terrestrial soils (Nilsen et al., 2020).
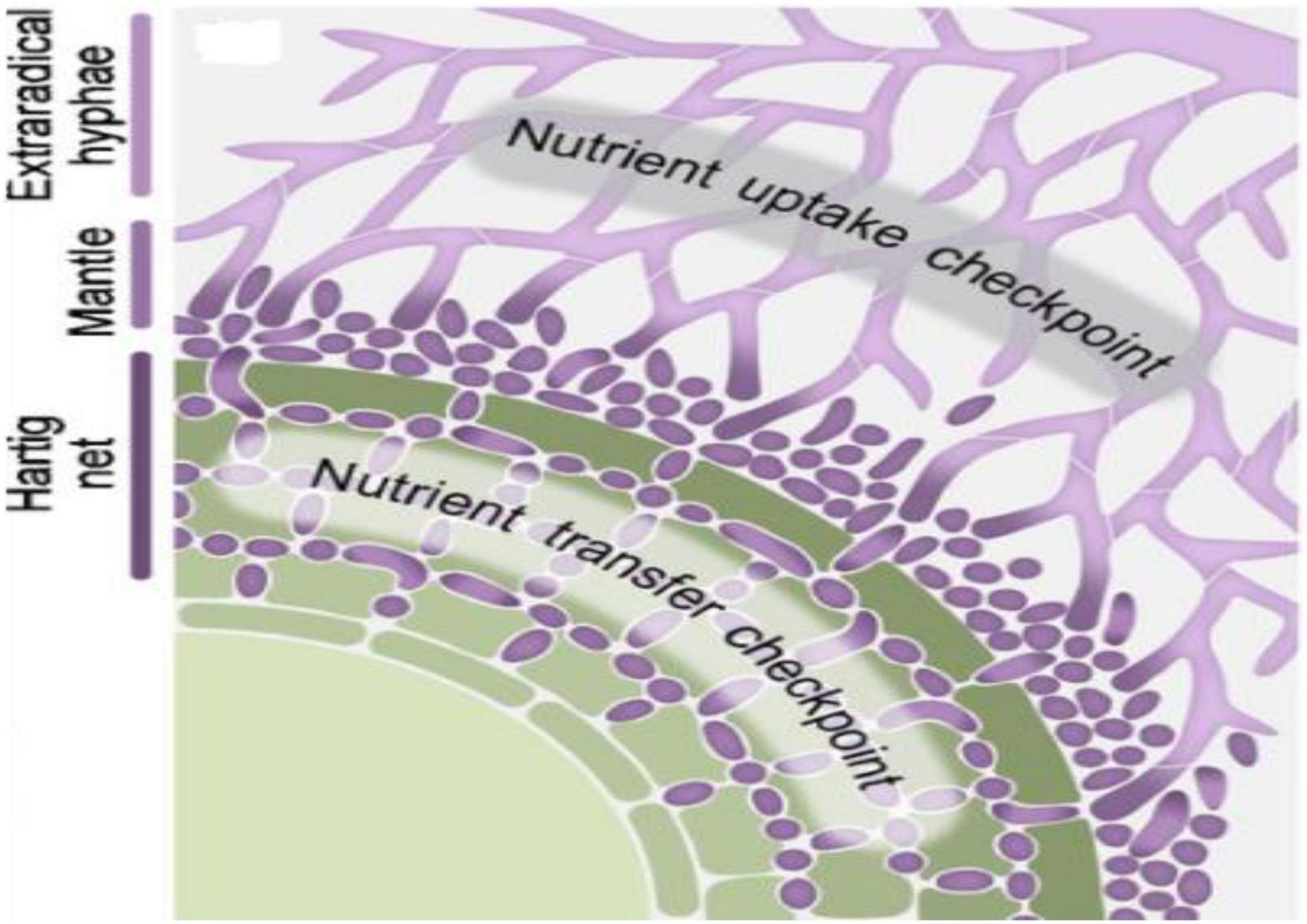
Figure 1. Schematic view depicting various parts (Hartig net, mantle, and extraradical hyphae) of established Ectomycorrhizal (ECM) symbiosis with plant root cells and regulatory checkpoints of nutrient transfer that occurs between ECM fungi and plant in established symbiosis. Reproduced from the work of Garcia et al. (2015) with permission of John Wiley and Sons.
It is well-known fact that ECM fungal symbiosis improves their host plants tolerance against metal stress (Jourand et al., 2010, 2014; Sun et al., 2021) and that ECM symbiosis application in plant regeneration on metal contaminated land has received considerable focus in global research (Wen et al., 2017; Shi et al., 2019; Dagher et al., 2020). Various reports concerning HM defense mechanisms in ECM fungi (Kalsotra et al., 2018; Khullar and Reddy, 2018; Shi et al., 2018, 2020) or plants (Hasan et al., 2017; Li et al., 2019; Schat et al., 2020) are available separately. Thus, understanding how ECM symbiosis affects and regulates the different plant defense mechanisms against metal stress has prime importance. This field is not extensively studied and reviewed but requires more focus to reinforce ECM symbiosis as a bioremediation tool for rehabilitating metal contaminated lands with plants. The present review mainly focused on understanding how ECM symbiosis affects the various host plant defense systems against metal stress, which thus results in their enhanced metal tolerance.
Ectomycorrhizal Symbiosis–Driven Mechanisms Behind Enhanced Metal Tolerance of Their Host Plants
Plants have diverse molecular and physiological mechanisms to counteract the HM stress, which broadly includes HM exclusion, compartmentalization, chelation, sequestration, and mitigation of HM-induced oxidative stress (Joshi et al., 2019; Zhu et al., 2021). Various plant defense mechanisms against HM stress have been reported to be positively affected by ECM symbiosis for their improved tolerance against HM stress, which are discussed in the following (Figure 2).
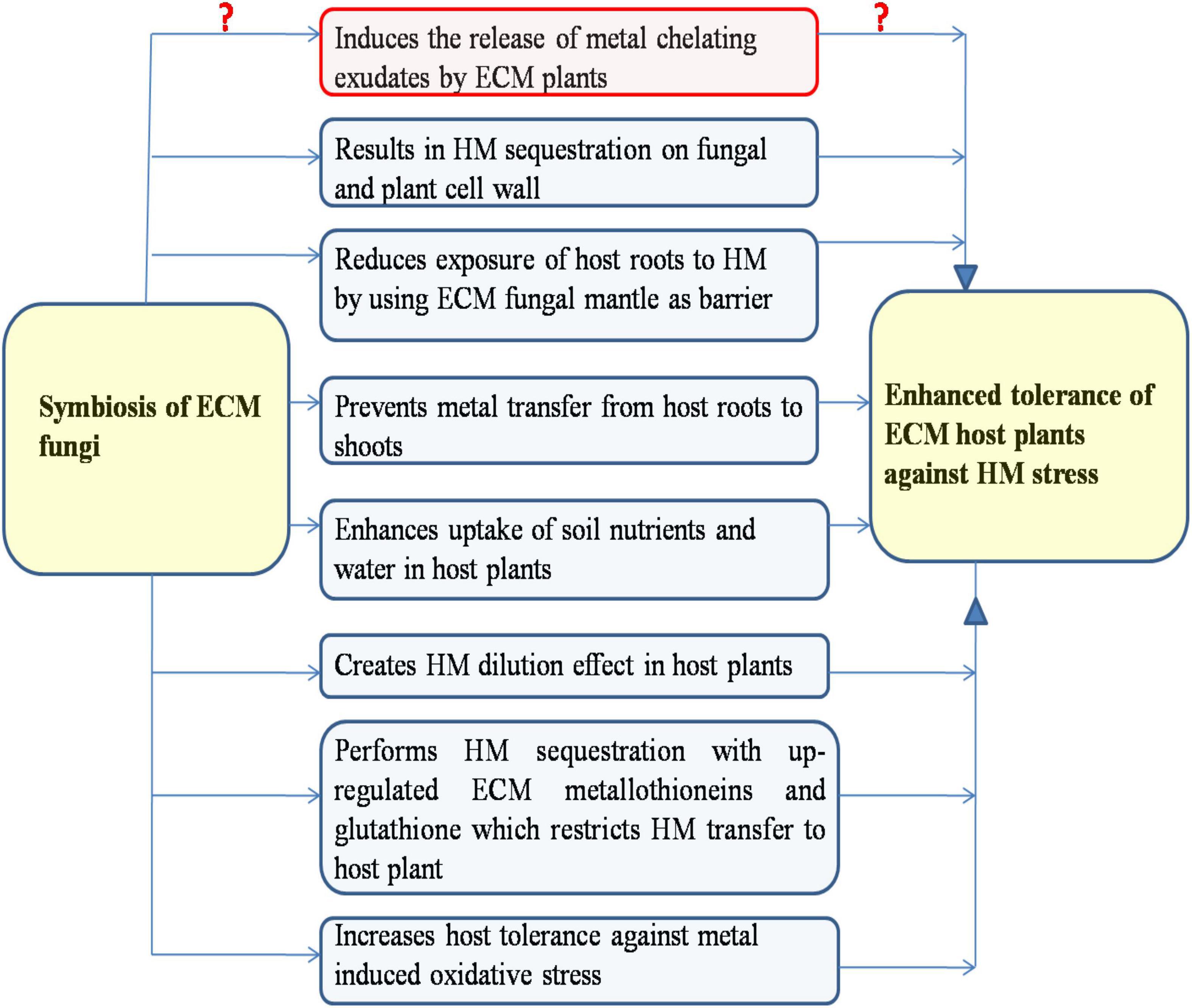
Figure 2. Ectomycorrhizal (ECM) symbiosis mediated different mechanisms conferring their host plants an increased tolerance against heavy metal stress (ECM symbiosis induces or represses the release of organic acids from ECM plants remain unclear).
Extracellular Secretion of Organic Acids by Ectomycorrhizal Plants Under Heavy Metal Stress
The assessment of bioavailable content forms a solid basis for predicting soil pollution and risk (Gonzalez-Chavez et al., 2004). Several environmental factors such as soil pH strongly affect the HM availability (Fässler et al., 2010; Bolan et al., 2014; Liu B. et al., 2020), with alkaline soil pH favoring metal unavailability (Fernández-Fuego et al., 2017). ECM-mediated reduction in bio-available or exchangeable soil HM content decreases metal toxicity on their host plants (Shi et al., 2019). The exudates of mycorrhizal fungi contain organic acids such as oxalic acid, succinic acid, malic acid, and formic acid, which chelate the metals and play an important role in metal detoxification (Meharg, 2003; Ray and Adholeya, 2009; Colpaert et al., 2011). The trivalent ions such as Fe3+ and Al3+ can form strong complexes with chelating agents like oxalate, citrate, and malate (Jones, 1998). The ECM fungal symbiosis induces the secretion of root exudates by host plants, which, in soil, chelates metal ions, thereby reducing their toxicity on plants (Gonzalez-Guerrero et al., 2008; Acosta et al., 2014). Many fungal species generally produce oxalic acid in large amounts (Dutton and Evans, 1996). Higher levels of oxalic acid production were reported in mycorrhizal plants of Pinus sylvestris in the presence of Al (Ahonen-Jonnarth et al., 2000). Contrary to this, a lower amount of oxalic acid production was recorded in mycorrhizal plants under high Ni stress, thus suggesting fungal sheath as a barrier restricting HM uptake in ECM roots, leading to reduced HM accumulation (Jourand et al., 2010). Although reduced organic acid production in metal stressed ECM plants relative to non-mycorrhizal plants has been reported, the ECM symbiosis enhances their host plant growth under metal stress (Fernández-Fuego et al., 2017). Plants have to pay high metabolic costs to survive the HM-induced oxidative stress, which could cause the reduced production of organic acids in plants under high HM stress (Iori et al., 2012). In support of this, Fernández-Fuego et al. (2017) showed that ECM-mediated enhanced HM accumulation in their metal stressed host plants reduces organic acid production compared to non-mycorrhizal control and vice versa. The utilization of plant high energy in expressing metallothioneins (MTs) (see section “Intracellular Heavy Metal Sequestration With Metal-Chelating Compounds”) reduces the growth of Willow plants upon ECM inoculation in Betulapubescens (Lanfranco, 2007). Besides the variations in the conclusion of the different studies described above, Ahonen-Jonnarth et al. (2000) also reported the varying potential of ECM symbiotic Pinus sylvestris to produce organic acids under metal stress depending upon the different ECM fungal species, HM type, and its concentration. Other ECM fungal species–based variations suggest their different metal tolerance strategies (Ahonen-Jonnarth et al., 2000; Courbot et al., 2004), which could also be possible due to varying fungal cell wall efficiencies to bind with HMs (Johansson et al., 2008). Hence, on the basis of these contradictory results, the impact of ECM symbiosis on the secretion of plant root exudates under metal stress cannot be precisely commented on and requires more studies.
Ectomycorrhizal-Associated Plant Roots Against Heavy Metal Toxicity
The ECM fungus, Paxillus involutus, inoculated to Pinus sylvestris showed the localization of Pb aggregates in epidermis and cortex of roots but not in stem or roots endodermis. These results suggest that roots are significant plant defense operators against metal stress (Bizo et al., 2017). The number of electronegative sites present on the cell wall of fungal mycelium for HM binding and, further, the fungal potential of intracellular HM binding forms the firm basis of varying efficiency of different ECM fungi in providing HM tolerance to their host plants (Shi et al., 2019). HMs binding to the sulfhydryl and phosphate compounds intracellularly or by binding with electronegative sites on fungal cell wall confers that ECM fungi provide high tolerance toward HM stress (Bizo et al., 2017). Several past studies focused on determining the impact of ECM inoculation on HM transfer from soil to host plants had generated diverse and contradictory results from each other. (i) Some studies reported ECM fungi as a physical barrier between soil HM content and plants, thereby reducing metal accumulation in plants (Colpaert and van Assche, 1993; Baum et al., 2006; Reddy et al., 2016). Under increasing Pb stress, ECM plants accumulate comparatively fewer Pb than non-mycorrhizal plants due to the fungal mantle-mediated reduction in roots exposure with Pb, eliminating the requirement of high energy-consuming plant defense metabolism against HM in ECM plants (Szuba et al., 2020). (ii) In contrast, other studies showed an increase of HM absorption and accumulation in plants upon ECM fungal inoculation (Fernández et al., 2008; Wen et al., 2017; Tang et al., 2019). Furthermore, ECM fungi can enhance the HM accumulation in plant roots but alleviate its transport into shoots (Kozdrój et al., 2007; Mrnka et al., 2012; Yu et al., 2020). Zong et al. (2015) reported that the extent of HM uptake by ECM roots and further transfer to shoots varies according to different ECM species inoculated with the same host separately and with other host plants. The soil HMs taken up by mycorrhizal fungi are loaded from their Hartig net to host roots. Still, the restricted apoplastic pathway of root endoderm due to Casparian strips causes the higher HM accumulation in roots than plant other tissues (Luo et al., 2014). (iii) A few studies have reported that ECM fungi could both increase and inhibit HM transport to the host plants (Marschner et al., 1996; Zimmer et al., 2009). In the case of plant nutrient metals such as Zn, the ECM fungi can perform the dual function of promoting and inhibiting HM accumulation in host plants depending upon its low and high toxic concentration present in an external environment, respectively (Zhang et al., 2021). The enhanced uptake of essential and non-essential HM by ECM roots was reported under low HM concentration, whereas the metal uptake got restricted under high HM concentrations, keeping plants healthy in both cases (Bojarczuk and Kieliszewska-Rokicka, 2010; Fernández-Fuego et al., 2017). The variability, in conclusions, derived from various studies could be due to several factors such as differences in culture medium used, period of HM treatment, and mycorrhization rates (Tang et al., 2019). While increasing the HM content of their host plants under HM stress, ECM fungi also improve plant growth, which the HM dilution effect can describe. The inoculation of ECM fungi promotes the nutrients, water, and HM uptake in host plants, which results in improved plant growth and dilution of HM content in metal stressed plants, thus reducing the HM-mediated toxicity in plants (Shi et al., 2019; Tang et al., 2019). Under Cd stress, the ECM plants can couple the net Cd influx with net H+ efflux through H+-ATPases, causing a higher Cd influx than non-ECM plant roots. Although Cd accumulates higher in ECM plant roots and leaves than non-ECM plants, the improved C assimilation, growth, and nutrition status of ECM plants provide them an enhanced growth compared to non-ECM plants under Cd stress (Table 1; Ma et al., 2014). The ECM inoculation increases the host plant biomass on metal-contaminated land. It improves the phytoextraction of HM in the host plant, resulting in reduced soil content compared to non-mycorrhizal plants (Dagher et al., 2020). To enhance the phytoremediation of HM pollution, the increase in HM mobilization can also lead to HM leaching to groundwater, which thus requires careful monitoring (Bolan et al., 2014).
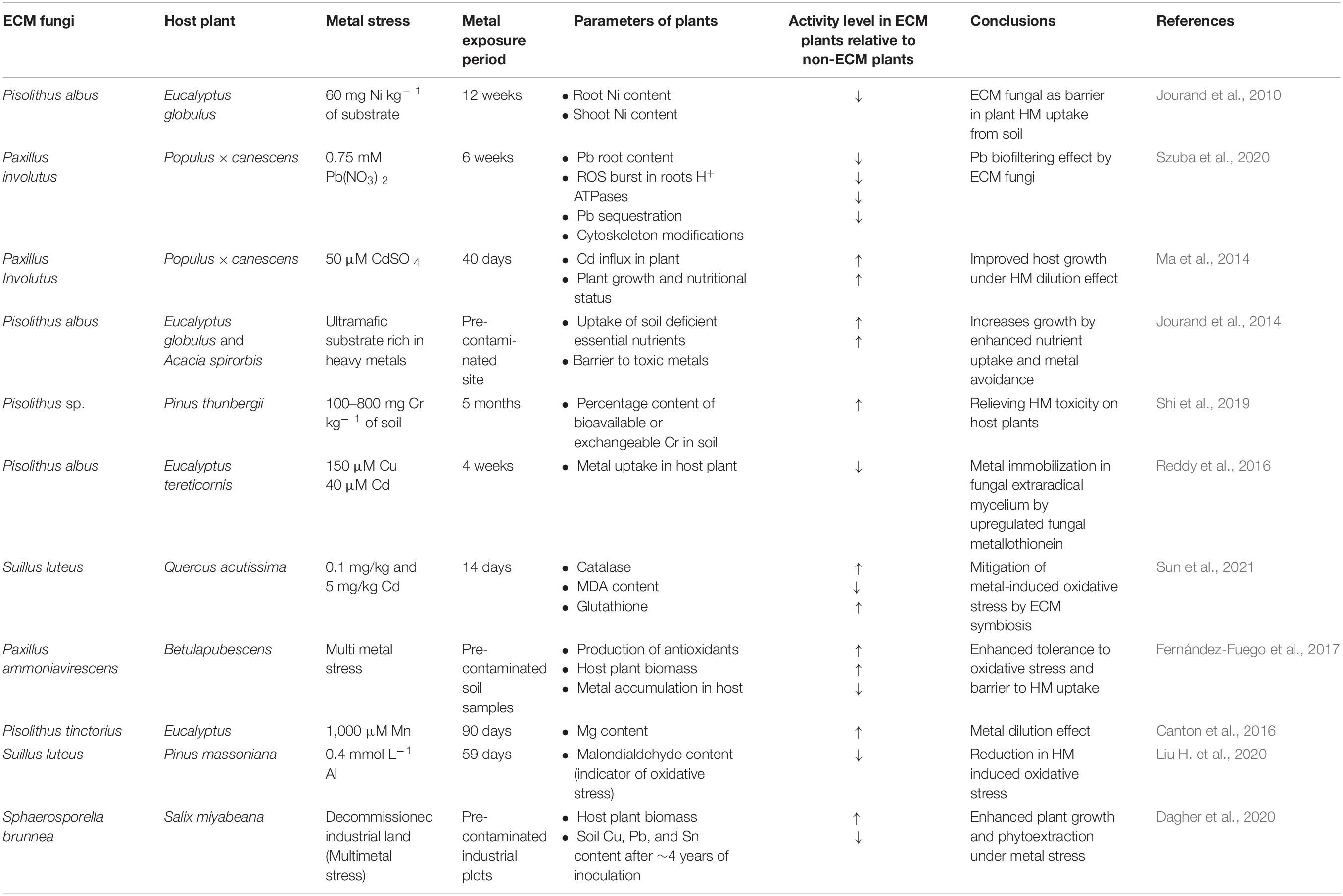
Table 1. Ectomycorrhizal (ECM) symbiosis–based altered parameters of metal stressed host plants, which confers them a better HM tolerance than non-ECM plants [upward (↑) and downward (↓) arrows show activity increase and decrease, respectively].
Intracellular Heavy Metal Sequestration With Metal-Chelating Compounds
Metallothioneins are cysteine-rich low–molecular weight proteins that strongly bind to metal ions through their thiol groups of cysteine residues, thus playing an essential role in metal sequestration and detoxification (Cobbett and Goldsbrough, 2002; Zhu et al., 2009). Besides the metal chelation, plant MTs function in scavenging accumulated ROS under oxidative stress (Xue et al., 2009: Hu et al., 2011; Ansarypour and Shahpiri, 2017; Malekzadeh and Shahpiri, 2017). The plant MT activity in metal detoxification varies with different valence states of metal (Zeng et al., 2011; Yu et al., 2019) and various plant tissues such as roots or shoots (Yu et al., 2019). Numerous reports about the differential expression of plant MTs under metal stress are available, which functions in metal detoxification; for example, Oryza sativa OsMT1b and OsMT2c under Cr stress (Yu et al., 2019); Oryza sativa OsMT1e under Cd stress (Rono et al., 2021); Physcomitrella patens PpMT2 under Cd and Cu stress (Liu Y. et al., 2020); and Phytolacca americana PaMT3-1 under Cd stress (Zhi et al., 2020). On the other hand, in the case of ECM fungi, the differential expression of MTs: LbMT1 and LbMT2 in Laccaria bicolor under Cd and Cu stress, respectively (Reddy et al., 2014); PaMT1 in Pisolithus albus under excess Cd and Cu stress (Reddy et al., 2016); HmMT3 in Hebeloma mesophaeum under Cd stress; and SuiMT1 and SuiMT2 in Suillus indicus under Cu stress (Shikha et al., 2019), leads to the detoxification of respective metal ions. The increased expression of symbiotic ECM MTs under metal stress causes the immobilization of metal ions in their extraradical mycelium and reduces the metal content in ECM roots compared to non-ECM roots (Reddy et al., 2016). Although not many studies are available regarding ECM effects on MT content of metal stressed host plants, past reports have determined the enhanced expression of host plant MTs upon their inoculation with arbuscular mycorrhizal (AM) fungi as compared to non-mycorrhizal plants under metal stress, ultimately leading to the host ameliorated metal tolerance (Cicatelli et al., 2010; Shabani and Sabzalian, 2016).
Glutathiones are well known to prevent oxidative stress and xenobiotics detoxification in cells (Sheehan et al., 2001). The upregulation of ECM fungal genes associated with glutathione biosynthesis under metal stress causes enhanced complexing of HM ions with glutathione and further sequestration of HM-glutathione complexes in their vacuoles. This process limits the HM transfer to their host plants, reducing HM toxicity (Khullar and Reddy, 2020). Similarly, the enhanced glutathione in ECM plants has been reported under metal stress compared to non-ECM plants, as shown in Table 1.
Host Plant Tolerance Against Heavy Metal Stress-Induced Oxidative Burst
The excess concentration of plant nutrient Zn can lead to increased production of ROS, which, if it breaks its balance with ROS destruction, results in oxidative stress (Bartoli et al., 2013; Mohammadhasani et al., 2017). With evolution, plants acquire native defense mechanisms against oxidative stress, including antioxidant enzymes such as superoxide, catalase, glutathione peroxidase, ascorbate peroxidase, and guaiacol peroxidase (Wu et al., 2014). Plants under HM stress can increase their antioxidant activities as a defense mechanism, and mycorrhizal symbiosis further enhances this activity (Fernández-Fuego et al., 2017; Mohammadhasani et al., 2017). Catalase, peroxidase, ascorbate peroxidase, and superoxide dismutase are the antioxidants that play an essential role in alleviating the HM-induced oxidative stress in plants. The superoxide dismutase mediates the ROS species conversion to H2O2, whereas peroxidase and catalase mediate the H2O2 conversion to H2O (Li et al., 2015). The activities of these antioxidants in ECM fungi rise with increasing HM concentration upto certain levels, after which their activities decreases (Dachuan and Jinyu, 2021). The ECM symbiosis mediates the increase in antioxidant activities in their host plants against HM stress as shown by enhanced catalase, glutathione activity, and reduced malondialdehyde (MDA) content in metal stressed Quercus acutissima roots inoculated with Suillus luteus compared to non-ECM plants (Table 1; Sun et al., 2021). The lower MDA content corresponds to the stronger antioxidant activities of an organism. The reduction in MDA content of host Pinus massoniana under Al stress has also been reported upon their inoculation with Suillus luteus compared to non-ECM plants (Liu H. et al., 2020). It thus suggests the ECM-mediated improved antioxidant machinery of the host plant as one of the essential mechanisms for their enhanced HM tolerance.
Alterations in the Nutrient Status of the Host Plants
The resource allocation between ECM fungal and plant partners strongly regulates the maintenance of long-term symbiotic association among both the partners. This long-term cooperation of nutrient transfer is maintained by transcriptional and translational regulations of transporter systems at regulatory checkpoints as shown in Figure 1 (Garcia et al., 2015). The presence of ECM symbiosis alters the transfer of nutrients from the soil to their host plants under HM stress (Table 2). The plant minerals such as Fe, Ca, N, P, and K content in roots and shoots get improved by ECM symbiosis in Pb-, Zn-, and Cd-contaminated soils (Hachani et al., 2020). The mycorrhiza-mediated N and P uptake in plants provides an ameliorated tolerance against oxidative stress (Begum et al., 2019). The P content is well correlated with the Cd concentration. The enhanced P content increases Cd accumulation in plants roots and significantly reduces Cd translocation upward in plants (Kong et al., 2020). At low Cd stress, the P content decreased in leaves and got doubled in the roots of ECM fungal plants. At high Cd stress, P content doubled in leaves and reduced in roots significantly compared with non-mycorrhizal plants under Cd stress, suggesting the vital role of ECM fungi in Cd retention of their host plants by regulating their P content (Sun et al., 2021). N and P enrichment can relieve Cd-induced oxidative stress in plants, possibly by increasing proline content (Kong et al., 2020). Proline acts as a potent non-enzymatic antioxidant and metal chelating agent (Sharma and Dietz, 2006).
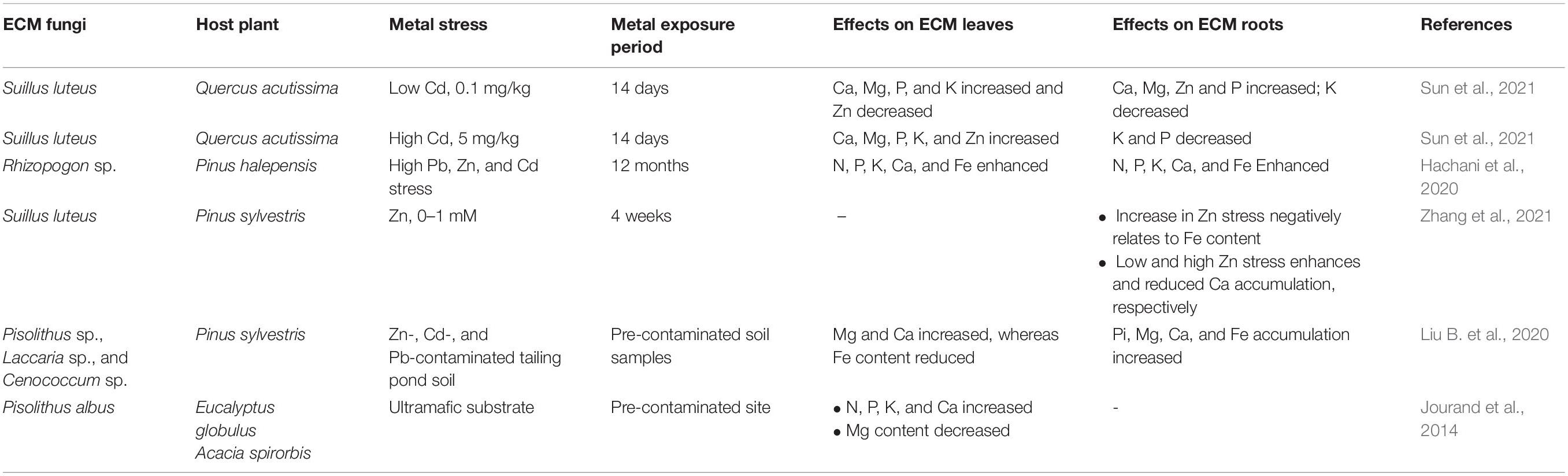
Table 2. Alteration in the nutrition status of host plants by Ectomycorrhizal (ECM) symbiosis under metal stress.
Further, the Na and P enrichment highly promotes Cd uptake and its sequestration with proline in plant roots which further decreases Cd transfer from sources to stem and helps to enhance the phytoextraction potential of the plant (Kong et al., 2020). On the other hand, the high external concentration of Zn in ECM plants negatively correlates with Fe accumulation in ECM roots. Ca uptake was enhanced under initial low external Zn and reduced under high Zn stress (Zhang et al., 2021). The antagonism between external Zn concentrations and Fe accumulation could be due to Fe displacement by Zn on ligand binding sites of metal transporters or siderophores (Suzuki et al., 2008; Hussein and Joo, 2019). Similarly, Langer et al. (2012) showed that the Fe uptake by ECM plants reduces under Zn stress compared to non-mycorrhizal plants, suggesting the competitive uptake among Fe, Zn, and Cd based on congruent ionic radii (Marschner, 1995). Among HM defense systems of ECM plants, the ion dilution effect is the mechanism under which the uptake and accumulation of nutrient minerals such as P and Mg got enhanced in plants to counteract the toxicity of harmful HMs to create HM dilution effects (Malcová et al., 2002; Canton et al., 2016). The increase in Ca and Mg content in ECM plants under HM stress suggests their role in improved plant biomass and further HM dilution effect in ECM plants as their tolerance mechanism (Sun et al., 2021).
On the other hand, P. albus symbiosis reduces Mg uptake in their host plants growing on ultramafic substrate rich in HMs to check excess Mg transfer. In contrast, host uptake for ultramafic soil deficient in N, P, K, and Ca increased, thus improving plant growth and creating a barrier for HMs present in excess (Jourand et al., 2014). The enhanced content of P, Mg, Ca, and Fe in Pinus sylvestris roots inoculated with different ECM fungal species under HM stress was reported compared to non-mycorrhizal plants. In shoots, reduced Fe content and increased Mg and Ca content were observed upon ECM symbiosis compared to non-mycorrhizal plants under metal stress (Liu B. et al., 2020). The varying uptake capacity of ECM plants for different nutrients could be due to the other kind of ECM fungi and their host plant species used and their tolerance potential for excess nutrients (Teotia et al., 2017).
At present, the restoration of abandoned mining lands is highly required to improve soil quality, microorganisms and plants growth for ecological rehabilitation (Wani et al., 2017). The use of traditional physical and chemical technologies for restoration programs can result in secondary pollution and high cost (Ayangbenro and Babalola, 2017).
The different species of ECM fungi affect host tolerance efficiency against metal stress differently (Sousa et al., 2012), thereby highlighting the need to optimize the best ECM fungal partner for the host before large-scale afforestation programs. In the reforestation of mine wastelands, the ECM infection rates in ECM fungal inoculated plant seedlings reduce after 6 months of their growth in mining lands (Chappelka et al., 1991; Hartley-Whitaker et al., 2000; Huang et al., 2012; Zong et al., 2015). The decline in ECM fungal colonization rate on land with high HM concentrations is associated with poor soil properties such as low organic matter, soil texture (Guo et al., 2007), macronutrients deficiency, and high processed residues content (Huang et al., 2012). The different species combination of ECM fungi and host plants gives different mycorrhization rates (Zong et al., 2015). On Pb-, Zn-, and Cd-polluted land, ECM fungal community richness and diversity have been correlated with soil N content but not with Pb, Zn, and Cd concentrations. For example, the dominant ECM fungal species obtained on N deficient tailing are mostly found on N-poor soils (Huang et al., 2012). By contrast, the diversity of ECM fungal community associated with white oak and Mason pine on Mn mine site correlated with less toxic Mn concentration (Huang et al., 2014). The Cd hyperaccumulator ecotypes of Sedum alfredii have more Cd accumulation in roots than non-hyperaccumulators (Sun et al., 2013). Among the different strains of the same ECM fungal species, the HM tolerant ecotypes of species are reported to be more potent for enhancing metal tolerance and growth in their host plants. Hence, this depicts ECM fungal selection’s importance for the phytoremediation programs of HM contaminated lands (Szuba et al., 2017). It is necessary to focus future studies on determining the ECM-mediated antagonist or synergistic effects of external HM stress with the dynamics of different nutrients in host plants, which may participate in plant response to HM stress. As different species of ECM fungi affect plant HM defense systems differently, further studies are required to explore the factors playing a role behind this. Furthermore, plant phytohormones such as auxin, ethylene, and abscisic acid are widely reported for their important roles in plant defense response against HM stress conditions (Emamverdian et al., 2021). Inoculating AM fungi to Robinia pseudoacacia seedlings under As stress enhanced the Indole-3-acetic acid and abscisic acid content, decreasing the zeatin riboside gibberellic acid concentrations and altering the ratios of various phytohormones in the host plant. These results suggest that the mycorrhiza-mediated phytohormones are essential factors behind host-alleviated metal toxicity (Zhang et al., 2020). Although, exogenously applying phytohormones and manipulating the plant’s endogenous level of phytohormones are reported as promising ways to enhance plants tolerance against metal stress (Saini et al., 2021). Several past studies reported that the ECM symbiosis significantly alters the hormonal status of their host plants (Felten et al., 2009; Basso et al., 2020). It would be interesting to explore the impact of ECM inoculation on hormones derived metal tolerance in host plants, which is not yet extensively reported. Besides the few lighter elements possessing naturally occurring radioisotopes, all elements with an atomic number more than 83 are considered radioactive (Thompson et al., 1949). To these natural radionuclides, several anthropogenic activities, such as nuclear industries, mining, and nuclear weapon trials, further add up radionuclides concentration in environment (Hain et al., 2020). The bioavailability and mobility of radionuclides significantly influence their deleterious impacts on environment and human health (Lopez-Fernandez et al., 2021). The AM symbiosis significantly affects the transfer of soil ribonuclides to their host plants. When inoculated with the same host plant, further ribonuclease retention in roots or shoot transfer varies depending upon different AM fungal species. For bioremediation purpose, the utilization of mycorrhizal symbiosis in enhancing phytoaccumulation of ribonuclides requires further studies (Rosas-Moreno et al., 2021). Similarly, the impact of ECM symbiosis over radionuclides accumulation in their host plants is reported in a few studies but remains unclear and needs focus studies (Ogo et al., 2018).
Conclusion
The ECM symbiosis improves the HM tolerance and growth of their metal stressed host plants through several mechanisms, which thus help in regenerating the metal-contaminated lands with plants. First, the ECM fungi can either act as a physical barrier between soil HM and plant roots or enhance HM accumulation in host plants depending on several factors such as HM type, external concentrations, and plant and fungal species. ECM fungi can also prevent HM transfer to plant roots by sequestering them on fungal cell walls or intracellularly with MTs and glutathione. ECM symbiosis ameliorates plant growth and their tolerance to oxidative stress under HM stress. The ECM fungi can change the nutrition dynamic of plants with soil in such a way to create HM dilution effects and to prevent HM transfer from roots to shoots. The role of ECM promoted or inhibited release plant root exudates in HM stress tolerance needs more studies for clarification. On the basis of the collected evidences, the ECM symbiosis proves to be beneficial for promoting plant growth on metal-contaminated lands and enhancing soil HM phytoextraction in host plants to reduce soil HM content. This field requires more extensive studies to understand the nutrient dynamics of ECM plants under metal stress and how it affects their tolerance.
Author Contributions
EC conducted the literature review and designed and wrote the manuscript. MR supervised, corrected, improved, and accepted the final version of the manuscript. Both authors contributed to the article and approved the submitted version.
Conflict of Interest
The authors declare that the research was conducted in the absence of any commercial or financial relationships that could be construed as a potential conflict of interest.
Publisher’s Note
All claims expressed in this article are solely those of the authors and do not necessarily represent those of their affiliated organizations, or those of the publisher, the editors and the reviewers. Any product that may be evaluated in this article, or claim that may be made by its manufacturer, is not guaranteed or endorsed by the publisher.
Abbreviations
Al, Aluminium; Fe, Iron; Ni, Nickel; Pb, Lead; Zn, Zinc; Cd, Cadmium; H, Hydrogen; C, Carbon; Cu, Copper; Cr, Chromium; Ca, Calcium; K, Potassium; P, Phosphorus; N, Nitrogen; Na, Sodium; Mg, Magnesium; Mn, Manganese.
References
Acosta, J. A., Faz, A., Kalbitz, K., Jansen, B., and Martínez-Martínez, S. (2014). Partitioning of heavy metals over different chemical fraction in street dust of Murcia (Spain) as a basis for risk assessment. J. Geochem. Explor. 144, 298–305. doi: 10.1016/j.gexplo.2014.02.004
Ahonen-Jonnarth, U. L. L. A., Van Hees, P. A., Lundström, U. S., and Finlay, R. D. (2000). Organic acids produced by mycorrhizal Pinus sylvestris exposed to elevated aluminium and heavy metal concentrations. New Phytol. 146, 557–567. doi: 10.1046/j.1469-8137.2000.00653.x
Ansarypour, Z., and Shahpiri, A. (2017). Heterologous expression of a rice metallothionein isoform (OsMTI-1b) in Saccharomyces cerevisiae enhances cadmium, hydrogen peroxide and ethanol tolerance. Braz. J. Microbiol. 48, 537–543. doi: 10.1016/j.bjm.2016.10.024
Ayangbenro, A. S., and Babalola, O. O. (2017). A new strategy for heavy metal polluted environments: a review of microbial biosorbents. Int. J. Environ. Res. Public Health 14:94. doi: 10.3390/ijerph14010094
Bartoli, C. G., Casalongué, C. A., Simontacchi, M., Marquez-Garcia, B., and Foyer, C. H. (2013). Interactions between hormone and redox signalling pathways in the control of growth and cross tolerance to stress. Environ. Exp. Bot. 94, 73–88. doi: 10.1016/j.envexpbot.2012.05.003
Basso, V., Kohler, A., Miyauchi, S., Singan, V., Guinet, F., Šimura, J., et al. (2020). An ectomycorrhizal fungus alters sensitivity to jasmonate, salicylate, gibberellin, and ethylene in host roots. Plant Cell Environ. 43, 1047–1068. doi: 10.1111/pce.13702
Baum, C., Hrynkiewicz, K., Leinweber, P., and Meißner, R. (2006). Heavy-metal mobilization and uptake by mycorrhizal and nonmycorrhizal willows (Salix× dasyclados). J. Plant Nutr. Soil Sci. 169, 516–522. doi: 10.1002/jpln.200521925
Begum, N., Qin, C., Ahanger, M. A., Raza, S., Khan, M. I., Ashraf, M., et al. (2019). Role of arbuscular mycorrhizal fungi in plant growth regulation: implications in abiotic stress tolerance. Front. Plant Sci. 10:1068. doi: 10.3389/fpls.2019.01068
Bizo, M. L., Nietzsche, S., Mansfeld, U., Langenhorst, F., Majzlan, J., Göttlicher, J., et al. (2017). Response to lead pollution: mycorrhizal Pinus sylvestris forms the biomineral pyromorphite in roots and needles. Environ. Sci. Pollut. Res. Int. 24, 14455–14462. doi: 10.1007/s11356-017-9020-7
Bojarczuk, K., and Kieliszewska-Rokicka, B. (2010). Effect of ectomycorrhiza on Cu and Pb accumulation in leaves and roots of silver birch (Betula pendula Roth.) seedlings grown in metal-contaminated soil. Water Air Soil Pollut. 207, 227–240. doi: 10.1007/s11270-009-0131-8
Bolan, N., Kunhikrishnan, A., Thangarajan, R., Kumpiene, J., Park, J., Makino, T., et al. (2014). Remediation of heavy metal (loid) s contaminated soils–to mobilize or to immobilize? J. Hazard. Mater. 266, 141–166. doi: 10.1016/j.jhazmat.2013.12.018
Broadley, M. R., White, P. J., Hammond, J. P., Zelko, I., and Lux, A. (2007). Zinc in plants. New Phytol. 173, 677–702. doi: 10.1111/j.1469-8137.2007.01996.x
Bruins, M. R., Kapil, S., and Oehme, F. W. (2000). Microbial resistance to metals in the environment. Ecotoxicol. Environ. Saf. 45, 198–207. doi: 10.1006/eesa.1999.1860
Cairney, J. W. (2011). Ectomycorrhizal fungi: the symbiotic route to the root for phosphorus in forest soils. Plant Soil 344, 51–71. doi: 10.1007/s11104-011-0731-0
Canton, G. C., Bertolazi, A. A., Cogo, A. J., Eutrópio, F. J., Melo, J., de Souza, S. B., et al. (2016). Biochemical and ecophysiological responses to manganese stress by ectomycorrhizal fungus Pisolithus tinctorius and in association with Eucalyptus grandis. Mycorrhiza 26, 475–487. doi: 10.1007/s00572-016-0686-3
Chappelka, A. H., Kush, J. S., Runion, G. B., Meier, S., and Kelley, W. D. (1991). Effects of soil-applied lead on seedling growth and ectomycorrhizal colonization of loblolly pine. Environ. Pollut. 72, 307–316. doi: 10.1016/0269-7491(91)90004-G
Cicatelli, A., Lingua, G., Todeschini, V., Biondi, S., Torrigiani, P., and Castiglione, S. (2010). Arbuscular mycorrhizal fungi restore normal growth in a white poplar clone grown on heavy metal-contaminated soil, and this is associated with upregulation of foliar metallothionein and polyamine biosynthetic gene expression. Ann. Bot. 106, 791–802. doi: 10.1093/aob/mcq170
Cobbett, C., and Goldsbrough, P. (2002). Phytochelatins and metallothioneins: roles in heavy metal detoxification and homeostasis. Annu. Rev. Plant Biol. 53, 159–182. doi: 10.1146/annurev.arplant.53.100301.135154
Colpaert, J. V., and van Assche, J. A. (1993). The effects of cadmium on ectomycorrhizal Pinus sylvestris L. New Phytol. 123, 325–333. doi: 10.1111/j.1469-8137.1993.tb03742.x
Colpaert, J. V., Wevers, J. H., Krznaric, E., and Adriaensen, K. (2011). How metal-tolerant ecotypes of ectomycorrhizal fungi protect plants from heavy metal pollution. Ann. Sci. 68, 17–24. doi: 10.1007/s13595-010-0003-9
Courbot, M., Diez, L., Ruotolo, R., Chalot, M., and Leroy, P. (2004). Cadmium-responsive thiols in the ectomycorrhizal fungus Paxillus involutus. Appl. Environ. Microbiol. 70, 7413–7417. doi: 10.1128/AEM.70.12.7413-7417.2004
Dachuan, Y., and Jinyu, Q. (2021). The physiological response of Ectomycorrhizal fungus Lepista sordida to Cd and Cu stress. PeerJ 9:e11115. doi: 10.7717/peerj.11115
Dagher, D. J., Pitre, F. E., and Hijri, M. (2020). Ectomycorrhizal fungal inoculation of sphaerosporella brunnea significantly increased stem biomass of Salix miyabeana and decreased lead, tin, and zinc, soil concentrations during the phytoremediation of an industrial landfill. J. Fungi. 6:87. doi: 10.3390/jof6020087
Dutton, M. V., and Evans, C. S. (1996). Oxalate production by fungi: its role in pathogenicity and ecology in the soil environment. Can. J. Microbiol. 42, 881–895. doi: 10.1139/m96-114
Emamverdian, A., Ding, Y., Mokhberdoran, F., and Ahmad, Z. (2021). Mechanisms of selected plant hormones under heavy metal stress. Pol. J. Environ. Stud. 30, 497–508. doi: 10.15244/pjoes/122809
Fässler, E., Robinson, B. H., Stauffer, W., Gupta, S. K., Papritz, A., and Schulin, R. (2010). Phytomanagement of metal-contaminated agricultural land using sunflower, maize and tobacco. Agric. Ecosyst. Environ. 136, 49–58. doi: 10.1016/j.agee.2009.11.007
Feleafel, M. N., and Mirdad, Z. M. (2013). Hazard and effects of pollution by lead on vegetable crops. J. Agric. Environ. Ethics. 26, 547–567. doi: 10.1007/s10806-012-9403-1
Felten, J., Kohler, A., Morin, E., Bhalerao, R. P., Palme, K., Martin, F., et al. (2009). The ectomycorrhizal fungus Laccaria bicolor stimulates lateral root formation in poplar and Arabidopsis through auxin transport and signaling. Plant Physiol. 151, 1991–2005. doi: 10.1104/pp.109.147231
Fernández, R., Bertrand, A., Casares, A., García, R., González, A., and Tamés, R. S. (2008). Cadmium accumulation and its effect on the in vitro growth of woody fleabane and mycorrhized white birch. Environ. Pollut. 152, 522–529. doi: 10.1016/j.envpol.2007.07.011
Fernández-Fuego, D., Bertrand, A., and González, A. (2017). Metal accumulation and detoxification mechanisms in mycorrhizal Betula pubescens. Environ. Pollut. 231, 1153–1162. doi: 10.1016/j.envpol.2017.07.072
Garcia, K., Delaux, P. M., Cope, K. R., and Ané, J. M. (2015). Molecular signals required for the establishment and maintenance of ectomycorrhizal symbioses. New Phytol. 208, 79–87. doi: 10.1111/nph.13423
Ghnaya, T., Zaier, H., Baioui, R., Sghaier, S., Lucchini, G., Sacchi, G. A., et al. (2013). Implication of organic acids in the long-distance transport and the accumulation of lead in Sesuvium portulacastrum and Brassica juncea. Chemosphere 90, 1449–1454. doi: 10.1016/j.chemosphere.2012.08.061
Gonzalez-Chavez, M. C., Carrillo-Gonzalez, R., Wright, S. F., and Nichols, K. A. (2004). The role of glomalin, a protein produced by arbuscular mycorrhizal fungi, in sequestering potentially toxic elements. Environ. Pollut. 130, 317–323. doi: 10.1016/j.envpol.2004.01.004
Gonzalez-Guerrero, M., Melville, L. H., Ferrol, N., Lott, J. N., Azcon-Aguilar, C., and Peterson, R. L. (2008). Ultrastructural localization of heavy metals in the extraradical mycelium and spores of the arbuscular mycorrhizal fungus Glomus intraradices. Can. J. Microbiol. 54, 103–110. doi: 10.1139/W07-119
Guo, J. P., Wu, F. C., Xie, S. R., Yao, L. L., and Xie, Y. H. (2007). Environmental conditions and exploitation of lead–zinc tailings in Linxiang County, Hunan Province. Chin. J. Soil Sci. 38, 553–557.
Hachani, C., Lamhamedi, M. S., Cameselle, C., Gouveia, S., Zine El Abidine, A., Khasa, D. P., et al. (2020). Effects of ectomycorrhizal fungi and heavy metals (Pb, Zn, and Cd) on growth and mineral nutrition of Pinus halepensis seedlings in North Africa. Microorganisms 8:2033. doi: 10.3390/microorganisms8122033
Haferburg, G., and Kothe, E. (2007). Microbes and metals: interactions in the environment. J. Basic Microbiol. 47, 453–467. doi: 10.1002/jobm.200700275
Hain, K., Steier, P., Froehlich, M. B., Golser, R., Hou, X., Lachner, J., et al. (2020). 233U/236U signature allows to distinguish environmental emissions of civil nuclear industry from weapons fallout. Nat. Commun. 11, 1–11. doi: 10.1038/s41467-020-15008-2
Hartley-Whitaker, J., Cairney, J. W., and Meharg, A. A. (2000). Sensitivity to Cd or Zn of host and symbiont of ectomycorrhizal Pinus sylvestris L.(Scots pine) seedlings. Plant Soil 218, 31–42. doi: 10.1023/A:1014989422241
Hasan, M., Cheng, Y., Kanwar, M. K., Chu, X. Y., Ahammed, G. J., and Qi, Z. Y. (2017). Responses of plant proteins to heavy metal stress—a review. Front. Plant Sci. 8:1492. doi: 10.3389/fpls.2017.01492
Hayat, S., Khalique, G., Irfan, M., Wani, A. S., Tripathi, B. N., and Ahmad, A. (2012). Physiological changes induced by chromium stress in plants: an overview. Protoplasma 249, 599–611. doi: 10.1007/s00709-011-0331-0
Hodge, A. (2017). Accessibility of inorganic and organic nutrients for mycorrhizas in Mycorrhizal mediation of soil. Amsterdam: Elsevier, 129–148.
Hu, L., Liang, W., Yin, C., Cui, X., Zong, J., Wang, X., et al. (2011). Rice MADS3 regulates ROS homeostasis during late anther development. Plant Cell 23, 515–533. doi: 10.1105/tpc.110.074369
Huang, J., Nara, K., Lian, C., Zong, K., Peng, K., Xue, S., et al. (2012). Ectomycorrhizal fungal communities associated with Masson pine (Pinus massoniana Lamb.) in Pb–Zn mine sites of central south China. Mycorrhiza 22, 589–602. doi: 10.1007/s00572-012-0436-0
Huang, J., Nara, K., Zong, K., Wang, J., Xue, S., Peng, K., et al. (2014). Ectomycorrhizal fungal communities associated with Masson pine (Pinus massoniana) and white oak (Quercus fabri) in a manganese mining region in Hunan Province. China Fungal Ecol. 9, 1–10. doi: 10.1016/j.funeco.2014.01.001
Hussein, K. A., and Joo, J. H. (2019). Zinc ions affect siderophore production by fungi isolated from the Panax ginseng rhizosphere. J. Microbiol. Biotechnol. 29, 105–113. doi: 10.4014/jmb.1712.12026
Iori, V., Pietrini, F., Massacci, A., and Zacchini, M. (2012). Induction of metal binding compounds and antioxidative defence in callus cultures of two black poplar (P. nigra) clones with different tolerance to cadmium. Plant Cell Tissue Organ. Cult. 108, 17–26. doi: 10.1007/s11240-011-0006-8
Jarvis, S. G., Woodward, S., and Taylor, A. F. (2015). Strong altitudinal partitioning in the distributions of ectomycorrhizal fungi along a short (300 m) elevation gradient. New Phytol. 206, 1145–1155. doi: 10.1111/nph.13315
Johansson, E. M., Fransson, P. M., Finlay, R. D., and van Hees, P. A. (2008). Quantitative analysis of root and ectomycorrhizal exudates as a response to Pb, Cd and as stress. Plant Soil 313, 39–54. doi: 10.1007/s11104-008-9678-1
Jones, D. L. (1998). Organic acids in the rhizosphere–a critical review. Plant Soil 205, 25–44. doi: 10.1023/A:1004356007312
Joshi, R., Dkhar, J., Singla-Pareek, S. L., and Pareek, A. (2019). Molecular mechanism and signaling response of heavy metal stress tolerance in plants in Plant-metal interactions. Cham: Springer, 29–47. doi: 10.1007/978-3-030-20732-8_2
Jourand, P., Ducousso, M., Reid, R., Majorel, C., Richert, C., Riss, J., et al. (2010). Nickel-tolerant ectomycorrhizal Pisolithus albus ultramafic ecotype isolated from nickel mines in New Caledonia strongly enhance growth of the host plant Eucalyptus globulus at toxic nickel concentrations. Tree Physiol. 30, 1311–1319. doi: 10.1093/treephys/tpq070
Jourand, P., Hannibal, L., Majorel, C., Mengant, S., and Ducousso, M. (2014). Ectomycorrhizal Pisolithus albus inoculation of Acacia spirorbis and Eucalyptus globulus grown in ultramafic topsoil enhances plant growth and mineral nutrition while limits metal uptake. J. Plant Physiol. 171, 164–172. doi: 10.1016/j.jplph.2013.10.011
Kalsotra, T., Khullar, S., Agnihotri, R., and Reddy, M. S. (2018). Metal induction of two metallothionein genes in the ectomycorrhizal fungus Suillus himalayensis and their role in metal tolerance. Microbiology 164, 868–876. doi: 10.1099/mic.0.000666
Khator, K., and Shekhawat, G. S. (2020). Cd-and Cu-induced phytotoxicity on 2–3 leaf stage of Cyamopsis tetragonoloba and its regulation by nitrate reductase and ROS quenching enzyme. Acta Physiol. 42, 1–14. doi: 10.1007/s11738-020-03105-0
Khosla, B., and Reddy, M. S. (2014). Mycorrhizal fungi in extreme environments and their impact on plant growth. Kavaka 42, 123–130.
Khullar, S., and Reddy, M. S. (2018). Ectomycorrhizal fungi and its role in metal homeostasis through metallothionein and glutathione mechanisms. Curr. Biotechnol. 7, 231–241. doi: 10.2174/2211550105666160531145544
Khullar, S., and Reddy, M. S. (2020). Arsenic toxicity and its mitigation in ectomycorrhizal fungus Hebeloma cylindrosporum through glutathione biosynthesis. Chemosphere 240:124914. doi: 10.1016/j.chemosphere.2019.124914
Kong, X., Zhao, Y., Tian, K., He, X., Jia, Y., He, Z., et al. (2020). Insight into nitrogen and phosphorus enrichment on cadmium phytoextraction of hydroponically grown Salix matsudana Koidz cuttings. Environ. Sci. Pollut. Res. Int. 27, 8406–8417. doi: 10.1007/s11356-019-07499-4
Kozdrój, J., Piotrowska-Seget, Z., and Krupa, P. (2007). Mycorrhizal fungi and ectomycorrhiza associated bacteria isolated from an industrial desert soil protect pine seedlings against Cd (II) impact. Ecotoxicology 16, 449–456. doi: 10.1007/s10646-007-0149-x
Kummel, M., and Lostroh, P. (2011). Altering light availability to the plant host determined the identity of the dominant ectomycorrhizal fungal partners and mediated mycorrhizal effects on plant growth. Botany 89, 439–450. doi: 10.1139/b11-033
Landeweert, R., Hoffland, E., Finlay, R. D., Kuyper, T. W., and van Breemen, N. (2001). Linking plants to rocks: ectomycorrhizal fungi mobilize nutrients from minerals. Trends Ecol. Evol. 16, 248–254. doi: 10.1016/S0169-5347(01)02122-X
Lanfranco, L. (2007). The fine-tuning of heavy metals in mycorrhizal fungi. New Phytol. 174, 3–6. doi: 10.1111/j.1469-8137.2007.02029.x
Langer, I., Santner, J., Krpata, D., Fitz, W. J., Wenzel, W. W., and Schweiger, P. F. (2012). Ectomycorrhizal impact on Zn accumulation of Populus tremula L. grown in metalliferous soil with increasing levels of Zn concentration. Plant Soil 355, 283–297. doi: 10.1007/s11104-011-1098-y
Li, D. Q., Chen, G. K., Zheng, H., Li, H. S., and Li, X. B. (2015). Effects of cadmium on growth and antioxidant enzyme activities of two kidney bean (Phaseolus vulgaris L.) cultivars. J. Agro-Environ. Sci. 34, 221–226.
Li, J., Jia, Y., Dong, R., Huang, R., Liu, P., Li, X., et al. (2019). Advances in the mechanisms of plant tolerance to manganese toxicity. Int. J. Mol. Sci. 20:5096. doi: 10.3390/ijms20205096
Liu, B., Wang, S., Wang, J., Zhang, X., Shen, Z., Shi, L., et al. (2020). The great potential for phytoremediation of abandoned tailings pond using ectomycorrhizal Pinus sylvestris. Sci. Total Environ. 719:137475. doi: 10.1016/j.scitotenv.2020.137475
Liu, H., Chen, H., Ding, G., Li, K., and Ren, Q. (2020). Identification of candidate genes conferring tolerance to aluminum stress in Pinus massoniana inoculated with ectomycorrhizal fungus. BMC Plant Biol. 20:1–13. doi: 10.1186/s12870-020-02719-3
Liu, Y., Kang, T., Cheng, J. S., Yi, Y. J., Han, J. J., Cheng, H. L., et al. (2020). Heterologous expression of the metallothionein PpMT2 gene from Physcomitrella patens confers enhanced tolerance to heavy metal stress on transgenic Arabidopsis plants. Plant Growth Regul. 90, 63–72. doi: 10.1007/s10725-019-00558-3
Lopez-Fernandez, M., Jroundi, F., Ruiz-Fresneda, M. A., and Merroun, M. L. (2021). Microbial interaction with and tolerance of radionuclides: underlying mechanisms and biotechnological applications. Microb. Biotechnol. 14, 810–828. doi: 10.1111/1751-7915.13718
Luo, Z. B., Wu, C., Zhang, C., Li, H., Lipka, U., and Polle, A. (2014). The role of ectomycorrhizas in heavy metal stress tolerance of host plants. Environ. Exp. Bot. 108, 47–62. doi: 10.1016/j.envexpbot.2013.10.018
Ma, Y., He, J., Ma, C., Luo, J., Li, H., Liu, T., et al. (2014). Ectomycorrhizas with Paxillus involutus enhance cadmium uptake and tolerance in Populus× canescens. Plant Cell Environ. 37, 627–642. doi: 10.1111/pce.12183
Malcová, R., Gryndler, M., and Vosátka, M. (2002). Magnesium ions alleviate the negative effect of manganese on Glomus claroideum BEG23. Mycorrhiza 12, 125–129. doi: 10.1007/s00572-002-0161-1
Malekzadeh, R., and Shahpiri, A. (2017). Independent metal-thiolate cluster formation in C-terminal Cys-rich region of a rice type 1 metallothionein isoform. Int. J. Biol. Macromol. 96, 436–441. doi: 10.1016/j.ijbiomac.2016.12.047
Marschner, P., Godbold, D. L., and Jentschke, G. (1996). Dynamics of lead accumulation in mycorrhizal and non-mycorrhizal Norway spruce (Picea abies (L.) Karst.). Plant Soil 178, 239–245. doi: 10.1007/BF00011589
Martin, F., Kohler, A., Murat, C., Veneault-Fourrey, C., and Hibbett, D. S. (2016). Unearthing the roots of ectomycorrhizal symbioses. Nat. Rev. Microbiol. 14:760. doi: 10.1038/nrmicro.2016.149
Meharg, A. A. (2003). The mechanistic basis of interactions between mycorrhizal associations and toxic metal cations. Mycol. Res. 107, 1253–1265. doi: 10.1017/S0953756203008608
Mohammadhasani, F., Ahmadimoghadam, A., Asrar, Z., and Mohammadi, S. Z. (2017). Effect of Zn toxicity on the level of lipid peroxidation and oxidative enzymes activity in Badami cultivar of pistachio (Pistacia vera L.) colonized by ectomycorrhizal fungus. Indian J. Plant. Physiol. 22, 206–212. doi: 10.1007/s40502-017-0300-5
Mrnka, L., Kuchár, M., Cieslarová, Z., Matčjka, P., Száková, J., Tlustoš, P., et al. (2012). Effects of endo-and ectomycorrhizal fungi on physiological parameters and heavy metals accumulation of two species from the family Salicaceae. Water Air Soil Pollut. 223, 399–410. doi: 10.1007/s11270-011-0868-8
Nagajyoti, P. C., Lee, K. D., and Sreekanth, T. V. M. (2010). Heavy metals, occurrence and toxicity for plants: a review. Environ. Chem. Lett. 8, 199–216. doi: 10.1007/s10311-010-0297-8
Nilsen, A. R., Teasdale, S. E., Guy, P. L., Summerfield, T. C., and Orlovich, D. A. (2020). Fungal diversity in canopy soil of silver beech, Nothofagus menziesii (Nothofagaceae). PLoS One 15:e0227860. doi: 10.1371/journal.pone.0227860
Ogo, S., Yamanaka, T., Akama, K., Nagakura, J., and Yamaji, K. (2018). Influence of ectomycorrhizal colonization on cesium uptake by Pinus densiflora seedlings. Mycobiology 46, 388–395. doi: 10.1080/12298093.2018.1538074
Rai, G. K., Bhat, B. A., Mushtaq, M., Tariq, L., Rai, P. K., Basu, U., et al. (2021). Insights into decontamination of soils by phytoremediation: a detailed account on heavy metal toxicity and mitigation strategies. Physiol. Plant 173, 287–304. doi: 10.1111/ppl.13433
Rastgoo, L., and Alemzadeh, A. (2011). Biochemical responses of Gouan (’Aeluropus littoralis’) to heavy metals stress. Aust. J. Crop Sci. 5:375. doi: 10.3316/informit.281334528451448
Ray, P., and Adholeya, A. (2009). Correlation between organic acid exudation and metal uptake by ectomycorrhizal fungi grown on pond ash in vitro. Biometals 22:275. doi: 10.1007/s10534-008-9163-6
Reddy, M. S., Kour, M., Aggarwal, S., Ahuja, S., Marmeisse, R., and Fraissinet Tachet, L. (2016). Metal induction of a Pisolithus albus metallothionein and its potential involvement in heavy metal tolerance during mycorrhizal symbiosis. Environ. Microbiol. 18, 2446–2454. doi: 10.1111/1462-2920.13149
Reddy, M. S., Prasanna, L., Marmeisse, R., and Fraissinet-Tachet, L. (2014). Differential expression of metallothioneins in response to heavy metals and their involvement in metal tolerance in the symbiotic basidiomycete Laccaria bicolor. Microbiology 160, 2235–2242. doi: 10.1099/mic.0.080218-0
Rog, I., Rosenstock, N. P., Körner, C., and Klein, T. (2020). Share the wealth: Trees with greater ectomycorrhizal species overlap share more carbon. Mol. Ecol. 29, 2321–2333. doi: 10.1111/mec.15351
Romero-Puertas, M. C., Terron-Camero, L. C., Pelaez-Vico, M. A., Olmedilla, A., and Sandalio, L. M. (2019). Reactive oxygen and nitrogen species as key indicators of plant responses to Cd stress. Environ. Exp. Bot. 161, 107–119. doi: 10.1016/j.envexpbot.2018.10.012
Rono, J. K., Le Wang, L., Wu, X. C., Cao, H. W., Zhao, Y. N., Khan, I. U., et al. (2021). Identification of a new function of metallothionein-like gene OsMT1e for cadmium detoxification and potential phytoremediation. Chemosphere 265:129136. doi: 10.1016/j.chemosphere.2020.129136
Rosas-Moreno, J., Pittman, J. K., and Robinson, C. H. (2021). Specific arbuscular mycorrhizal fungal–plant interactions determine radionuclide and metal transfer into Plantago lanceolata. Plants People Planet 3, 667–678. doi: 10.1002/ppp3.10185
Rosling, A. (2009). Trees, mycorrhiza and minerals–field relevance of in vitro experiments. Geomicrobiol. J. 26, 389–401. doi: 10.1080/01490450902929324
Rucińska-Sobkowiak, R. (2016). Water relations in plants subjected to heavy metal stresses. Acta Physiol. 38, 1–13. doi: 10.1007/s11738-016-2277-5
Saini, S., Kaur, N., and Pati, P. K. (2021). Phytohormones: Key players in the modulation of heavy metal stress tolerance in plants. Ecotoxicol. Environ. Saf. 223:112578. doi: 10.1016/j.ecoenv.2021.112578
Saitta, A., Anslan, S., Bahram, M., Brocca, L., and Tedersoo, L. (2018). Tree species identity and diversity drive fungal richness and community composition along an elevational gradient in a Mediterranean ecosystem. Mycorrhiza 28, 39–47. doi: 10.1007/s00572-017-0806-8
Schat, H., Llugany, M., and Bernhard, R. (2020). Metal-specific patterns of tolerance, uptake, and transport of heavy metals in hyperaccumulating and nonhyperaccumulating metallophytes in Phytoremediation of contaminated soil and water. Boca Raton: CRC Press, 171–188.
Shabani, L., and Sabzalian, M. R. (2016). Arbuscular mycorrhiza affects nickel translocation and expression of ABC transporter and metallothionein genes in Festuca arundinacea. Mycorrhiza 26, 67–76. doi: 10.1007/s00572-015-0647-2
Sharma, R. K., Agrawal, M., and Marshall, F. (2007). Heavy metal contamination of soil and vegetables in suburban areas of Varanasi. India Ecotoxicol. Environ. Saf. 66, 258–266. doi: 10.1016/j.ecoenv.2005.11.007
Sharma, S. S., and Dietz, K. J. (2006). The significance of amino acids and amino acid-derived molecules in plant responses and adaptation to heavy metal stress. J. Exp. Bot. 57, 711–726. doi: 10.1093/jxb/erj073
Sheehan, D., Meade, G., and Foley, V. M. (2001). Structure, function and evolution of glutathione transferases: implications for classification of non-mammalian members of an ancient enzyme superfamily. Biochem. J. 360, 1–16. doi: 10.1042/bj3600001
Shi, L., Deng, X., Yang, Y., Jia, Q., Wang, C., Shen, Z., et al. (2019). A Cr (VI)-tolerant strain, Pisolithus sp1, with a high accumulation capacity of Cr in mycelium and highly efficient assisting Pinus thunbergii for phytoremediation. Chemosphere 224, 862–872. doi: 10.1016/j.chemosphere.2019.03.015
Shi, L., Dong, P., Song, W., Li, C., Lu, H., Wen, Z., et al. (2020). Comparative transcriptomic analysis reveals novel insights into the response to Cr (VI) exposure in Cr (VI) tolerant ectomycorrhizal fungi Pisolithus sp. 1 LS-2017. Ecotoxicol. Environ. Saf. 188:109935. doi: 10.1016/j.ecoenv.2019.109935
Shi, L., Xue, J., Liu, B., Dong, P., Wen, Z., Shen, Z., et al. (2018). Hydrogen ions and organic acids secreted by ectomycorrhizal fungi, Pisolithus sp1, are involved in the efficient removal of hexavalent chromium from waste water. Ecotoxicol. Environ. Saf. 161, 430–436. doi: 10.1016/j.ecoenv.2018.06.004
Shikha, K., Anuja, S., Radhika, A., and Reddy, M. S. (2019). Metallothioneins mediated intracellular copper homeostasis in Ectomycorrhizal fungus Suillus indicus. Kavaka 53, 48–54. doi: 10.36460/Kavaka/53/2019/48-54
Sousa, N. R., Ramos, M. A., Marques, A. P., and Castro, P. M. (2012). The effect of ectomycorrhizal fungi forming symbiosis with Pinus pinaster seedlings exposed to cadmium. Sci. Total Environ. 414, 63–67. doi: 10.1016/j.scitotenv.2011.10.053
Srivastava, V., Sarkar, A., Singh, S., Singh, P., de Araujo, A. S., and Singh, R. P. (2017). Agroecological responses of heavy metal pollution with special emphasis on soil health and plant performances. Front. Environ. Sci. 5:64. doi: 10.3389/fenvs.2017.00064
Steidinger, B. S., Bhatnagar, J. M., Vilgalys, R., Taylor, J. W., Qin, C., Zhu, K., et al. (2020). Ectomycorrhizal fungal diversity predicted to substantially decline due to climate changes in North American Pinaceae forests. J. Biogeogr. 47, 772–782. doi: 10.1111/jbi.13802
Sun, J., Wang, R., Liu, Z., Ding, Y., and Li, T. (2013). Non-invasive microelectrode cadmium flux measurements reveal the spatial characteristics and real-time kinetics of cadmium transport in hyperaccumulator and nonhyperaccumulator ecotypes of Sedum alfredii. J. Plant Physiol. 170, 355–359. doi: 10.1016/j.jplph.2012.10.014
Sun, W., Yang, B., Zhu, Y., Wang, H., Qin, G., and Yang, H. (2021). Ectomycorrhizal fungi enhance the tolerance of phytotoxicity and cadmium accumulation in oak (Quercus acutissima Carruth.) seedlings: modulation of growth properties and the antioxidant defense responses. Environ. Sci. Pollut. Res. Int. 2021, 1–12. doi: 10.1007/s11356-021-16169-3
Suzuki, M., Tsukamoto, T., Inoue, H., Watanabe, S., Matsuhashi, S., Takahashi, M., et al. (2008). Deoxymugineic acid increases Zn translocation in Zn-deficient rice plants. Plant Mol. Biol. 66, 609–617. doi: 10.1007/s11103-008-9292-x
Szuba, A., Karliński, L., Krzesłowska, M., and Hazubska-Przybył, T. (2017). Inoculation with a Pb-tolerant strain of Paxillus involutus improves growth and Pb tolerance of Populus× canescens under in vitro conditions. Plant Soil 412, 253–266. doi: 10.1007/s11104-016-3062-3
Szuba, A., Marczak, L., and Kozłowski, R. (2020). Role of the proteome in providing phenotypic stability in control and ectomycorrhizal poplar plants exposed to chronic mild Pb stress. Environ. Pollut. 264:114585. doi: 10.1016/j.envpol.2020.114585
Tang, Y., Shi, L., Zhong, K., Shen, Z., and Chen, Y. (2019). Ectomycorrhizal fungi may not act as a barrier inhibiting host plant absorption of heavy metals. Chemosphere 215, 115–123. doi: 10.1016/j.chemosphere.2018.09.143
Tedersoo, L., Bahram, M., Põlme, S., Kõljalg, U., Yorou, N. S., Wijesundera, R., et al. (2014). Global diversity and geography of soil fungi. Science 346:1256688. doi: 10.1126/science.1256688
Teotia, P., Kumar, M., Prasad, R., Kumar, V., Tuteja, N., and Varma, A. (2017). Mobilization of micronutrients by mycorrhizal fungi in Mycorrhiza-Function, Diversity, State of the Art. Cham: Springer. 9–26. doi: 10.1007/978-3-319-53064-2_2
Thompson, S. G., Ghiorso, A., Rasmussen, J. O., and Seaborg, G. T. (1949). Alpha-decay in isotopes of atomic number less than 83. Phys. Rev. 76:1406. doi: 10.1103/PhysRev.76.1406
Vaclavikova, M., Gallios, G. P., Hredzak, S., and Jakabsky, S. (2008). Removal of arsenic from water streams: an overview of available techniques. Clean Technol. Environ. Policy 10, 89–95. doi: 10.1007/s10098-007-0098-3
Van der Heijden, M. G., Martin, F. M., Selosse, M. A., and Sanders, I. R. (2015). Mycorrhizal ecology and evolution: the past, the present, and the future. New Phytol. 205, 1406–1423. doi: 10.1111/nph.13288
Veach, A. M., Stokes, C. E., Knoepp, J., Jumpponen, A., and Baird, R. (2018). Fungal communities and functional guilds shift along an elevational gradient in the southern Appalachian Mountains. Microb. Ecol. 76, 156–168. doi: 10.1007/s00248-017-1116-6
Wang, X., Agathokleous, E., Qu, L., Fujita, S., Watanabe, M., Tamai, Y., et al. (2018). Effects of simulated nitrogen deposition on ectomycorrhizae community structure in hybrid larch and its parents grown in volcanic ash soil: The role of phosphorous. Sci. Total Environ. 618, 905–915. doi: 10.1016/j.scitotenv.2017.08.283
Wani, R. A., Ganai, B. A. M., Shah, A., and Uqab, B. (2017). Heavy metal uptake potential of aquatic plants through phytoremediation technique–a review. J. Bioremediat. Biodegrad. 8:2. doi: 10.4172/2155-6199.1000404
Wei, S., Song, Y., and Jia, L. (2020). Influence of the slope aspect on the ectomycorrhizal fungal community of Quercus variabilis Blume in the middle part of the Taihang Mountains, North China. J. Res. 2020, 1–16. doi: 10.1007/s11676-019-01083-9
Wen, Z., Shi, L., Tang, Y., Shen, Z., Xia, Y., and Chen, Y. (2017). Effects of Pisolithus tinctorius and Cenococcum geophilum inoculation on pine in copper-contaminated soil to enhance phytoremediation. Int. J. Phytoremed. 19, 387–394. doi: 10.1080/15226514.2016.1244155
Wu, Q. S., Zou, Y. N., and Abd-Allah, E. F. (2014). Mycorrhizal association and ROS in plants in Oxidative damage to plants. Cambridge: Academic Press, 453–475. doi: 10.1016/B978-0-12-799963-0.00015-0
Wu, T. (2011). Can ectomycorrhizal fungi circumvent the nitrogen mineralization for plant nutrition in temperate forest ecosystems? Soil Biol. Biochem. 43, 1109–1117. doi: 10.1016/j.soilbio.2011.02.003
Xue, T., Li, X., Zhu, W., Wu, C., Yang, G., and Zheng, C. (2009). Cotton metallothionein GhMT3a, a reactive oxygen species scavenger, increased tolerance against abiotic stress in transgenic tobacco and yeast. J. Exp. Bot. 60, 339–349. doi: 10.1093/jxb/ern291
Yu, P., Sun, Y., Huang, Z., Zhu, F., Sun, Y., and Jiang, L. (2020). The effects of ectomycorrhizal fungi on heavy metals’ transport in Pinus massoniana and bacteria community in rhizosphere soil in mine tailing area. J. Hazard Mater. 381:121203. doi: 10.1016/j.jhazmat.2019.121203
Yu, X. Z., Lin, Y. J., and Zhang, Q. (2019). Metallothioneins enhance chromium detoxification through scavenging ROS and stimulating metal chelation in Oryza sativa. Chemosphere 220, 300–313. doi: 10.1016/j.chemosphere.2018.12.119
Zeng, F., Zhou, W., Qiu, B., Ali, S., Wu, F., and Zhang, G. (2011). Subcellular distribution and chemical forms of chromium in rice plants suffering from different levels of chromium toxicity. J. Plant Nutr. Soil Sci. 174, 249–256. doi: 10.1002/jpln.200900309
Zhang, C., Wang, X., Ashraf, U., Qiu, B., and Ali, S. (2017). Transfer of lead (Pb) in the soil-plant-mealybug-ladybird beetle food chain, a comparison between two host plants. Ecotoxicol. Environ. Saf. 143, 289–295. doi: 10.1016/j.ecoenv.2017.05.032
Zhang, J., Taniguchi, T., Xu, M., Du, S., Liu, G. B., and Yamanaka, N. (2014). Ectomycorrhizal fungal communities of Quercus liaotungensis along different successional stands on the Loess Plateau. China J. Res. 19, 395–403. doi: 10.1007/s10310-013-0433-y
Zhang, K., Tappero, R., Ruytinx, J., Branco, S., and Liao, H. L. (2021). Disentangling the role of ectomycorrhizal fungi in plant nutrient acquisition along a Zn gradient using X-ray imaging. Sci. Total Environ. 801:149481. doi: 10.1016/j.scitotenv.2021.149481
Zhang, Q., Gong, M., Liu, K., Chen, Y., Yuan, J., and Chang, Q. (2020). Rhizoglomus intraradices improves plant growth, root morphology and Phytohormone balance of Robinia pseudoacacia in arsenic-contaminated soils. Front. Microbiol. 11:1428. doi: 10.3389/fmicb.2020.01428
Zhi, J., Liu, X., Yin, P., Yang, R., Liu, J., and Xu, J. (2020). Overexpression of the metallothionein gene PaMT3-1 from Phytolacca americana enhances plant tolerance to cadmium. Plant Cell Tissue Organ. Cult. 143, 211–218. doi: 10.1007/s11240-020-01914-2
Zhu, T., Li, L., Duan, Q., Liu, X., and Chen, M. (2021). Progress in our understanding of plant responses to the stress of heavy metal cadmium. Plant Signal. Behav. 16:1836884. doi: 10.1080/15592324.2020.1836884
Zhu, W., Zhao, D. X., Miao, Q., Xue, T. T., Li, X. Z., and Zheng, C. C. (2009). Arabidopsis thaliana metallothionein, AtMT2a, mediates ROS balance during oxidative stress. J. Plant Biol. 52:585. doi: 10.1007/s12374-009-9076-0
Zimmer, D., Baum, C., Leinweber, P., Hrynkiewicz, K., and Meissner, R. (2009). Associated bacteria increase the phytoextraction of cadmium and zinc from a metal-contaminated soil by mycorrhizal willows. Int. J. Phytoremed. 11, 200–213. doi: 10.1080/15226510802378483
Keywords: ectomycorrhizal fungi, heavy metal stress, host plants, metal tolerance, symbiosis, metal defense mechanisms
Citation: Chot E and Reddy MS (2022) Role of Ectomycorrhizal Symbiosis Behind the Host Plants Ameliorated Tolerance Against Heavy Metal Stress. Front. Microbiol. 13:855473. doi: 10.3389/fmicb.2022.855473
Received: 15 January 2022; Accepted: 15 February 2022;
Published: 28 March 2022.
Edited by:
Alok Kumar Srivastava, National Bureau of Agriculturally Important Microorganisms (ICAR), IndiaReviewed by:
Simone Cantamessa, Council for Agricultural and Economics Research (CREA), ItalyHayssam M. Ali, King Saud University, Saudi Arabia
Copyright © 2022 Chot and Reddy. This is an open-access article distributed under the terms of the Creative Commons Attribution License (CC BY). The use, distribution or reproduction in other forums is permitted, provided the original author(s) and the copyright owner(s) are credited and that the original publication in this journal is cited, in accordance with accepted academic practice. No use, distribution or reproduction is permitted which does not comply with these terms.
*Correspondence: Mondem Sudhakara Reddy, bXNyZWRkeUB0aGFwYXIuZWR1