- 1State Key Laboratory of Animal Nutrition, Institute of Animal Science, Chinese Academy of Agricultural Sciences, Beijing, China
- 2State Key Laboratory of Animal Nutrition, College of Animal Science and Technology, China Agricultural University, Beijing, China
Calcium propionate is one kind of good source for preventing and treating hypocalcemia and ketosis for dairy cows in early lactation. However, little is known about the effects of different feeding levels of calcium propionate on the ruminal bacterial community of early lactation dairy cows. This study aimed to explore the effects of different calcium propionate feeding levels on the ruminal fermentation and bacterial community composition of early lactation dairy cows. Twenty-four multiparous cows were randomly allocated into control (CON), low calcium propionate (LCaP), medium calcium propionate (MCaP), and high calcium propionate (HCaP) groups with six cows per group after calving. The CON group cows were fed the normal total mixed ration (TMR), and the cows of the LCaP, MCaP, and HCaP groups were fed TMR supplemented with 200, 350, and 500 g/day calcium propionate for 35 days after calving, respectively. The rumen fermentation parameters were measured every week, and the ruminal bacterial community composition of the last week was analyzed by 16S rRNA gene sequencing. Under the same diet, the rumen pH showed no difference among the four groups, but the content of microbial crude protein (MCP) and ammonia nitrogen quadratically decreased and linearly increased with calcium propionate supplementation, respectively. The feeding of calcium propionate linearly increased the concentrations of total volatile fatty acid (VFA), acetate, propionate, butyrate, iso-valerate, and valerate in the rumen. In all the treatment groups, Bacteroidetes, Firmicutes, and Proteobacteria were the dominant phyla, and Prevotella_1 and Succiniclasticum were the dominant genera in the rumen. Compared with the CON group, the addition of calcium propionate to the early lactation dairy cows quadratically improved the alpha diversity index of Chao1 estimator and observed species, but had little effect on the relative abundance of the major bacterial at phyla and genera level. These results suggested different levels of calcium propionate supplementation improved the rumen fermentation and the ruminal bacterial diversity but had little impact on the major ruminal bacterial community composition of dairy cows in early lactation.
Introduction
The most important challenge for early lactation dairy cows is the difficulty of nutrient intake to meet the nutritional requirements of rapidly increasing milk production. Dairy cows in this period presented complicated metabolic challenges with blood calcium defects and negative energy balance (NEB), especially high-performing dairy cows. Hypocalcemia is a known risk for many diseases, including milk fever, dystocia, uterine prolapse, retained placenta, metritis, displaced abomasum, ketosis, and mastitis (Couto Serrenho et al., 2021). Cows with severe NEB are susceptible to fatty liver, ketosis, and other metabolic disorders. Therefore, hypocalcemia and NEB play etiological roles in the reduction in dry matter (DM) intake and milk production, reproductive disorders development, fertility problems, and infectious diseases (Kara, 2013), which should be prevented and treated immediately for the periparturient cows. Increasing dietary calcium and energy feeding levels is a common method to prevent and treat hypocalcemia and ketosis for early lactation dairy cows. In ruminants, propionate serves as an energy source that contributes to 60–74% of hepatic gluconeogenesis (Aschenbach et al., 2010). Calcium propionate, which can be hydrolyzed into propionic acid and calcium ions in the rumen, is widely used to correct the metabolic problem in dairy cows (Zhang et al., 2017). Goff et al. (1996) observed dairy cows administered with one calcium propionate tube (each containing 37 g of calcium) respectively at calving and again 12 h after calving was beneficial in reducing subclinical hypocalcemia and the incidence of milk fever. Liu et al. (2010) reported that different amounts of calcium propionate (100, 200, and 300 g/day) supplementation improved the nutrient digestibility and energy status of dairy cows in early lactation. In the study conducted by Martins et al. (2019), Holstein cows fed calcium propionate (200 g/day) increased milk yield during early lactation. In addition, we reported that dietary supplementation with calcium propionate to the dairy cows in early lactation quadratically increased milk yield and DM intake, and the optimum amount of calcium propionate was 350 g/day (Zhang et al., 2022). We speculated that the benefit of improved lactation performance after feeding calcium propionate might be related to the process of rumen microbial fermentation.
In ruminants, a wide range of microorganisms carry out extensive and complex metabolic activities, fermenting plant proteins and polysaccharides to produce nutrients for ruminant maintenance, growth, and lactation (Liu et al., 2019). The rumen microbiome directly or indirectly contributes to animal production and is susceptible to changes in feed types and components of the diet (Kataev et al., 2020). The profile of the rumen microbial community and the abundance of metabolites (including the rumen fermentation index) play key roles in the health status and production performance of dairy cows (Wang et al., 2021b). A better understanding of the changes of rumen microbial community compositions is crucial to invest the impact of calcium propionate feed additive in dairy cows. Many studies about the application of calcium propionate in dairy cows focused on lactation performance and metabolic properties, whereas the effect of calcium propionate on rumen fermentation and ruminal bacterial community composition had received less attention (Goff and Horst, 1993; Pehrson et al., 1998; Liu et al., 2010). As one of the major fermentation productions of rumen microbiota from carbohydrates, the supplementation of propionate-related production might affect the rumen fermentation profiles and ruminal bacterial communities. The 16S rRNA sequencing is a well-tested, fast and cost-effective method for analyzing the differential abundance of microbial communities (Liu et al., 2019). Therefore, this study aimed to evaluate the application of calcium propionate as a calcium source and glycogenic precursor during early lactation on rumen fermentation and ruminal bacterial community composition in high-yielding dairy cows.
Materials and Methods
The animals used in the study were approved by the Animal Care Committee of the Chinese Academy of Agricultural Sciences (Beijing, China; No. IAS2020-93), and all experimental procedures were following the guidelines of the academy for animal research.
Animals and Diets
This study was performed at the China-Israel demonstration dairy farm (Beijing, China) from September to December 2020. Twenty-four multiparous Holstein cows in early lactation were randomly divided into four blocks (n = 6 per treatment) based on parity (2, n = 4; 3, n = 12; and 4, n = 8), previous lactation corrected 305-days milk yield (12,672 ± 312 kg), and anticipated calving date. All the dairy cows were fed the same basal total mixed ration diet (TMR), which was formulated according to the National Research Council (NRC, 2001) recommendation. The ingredients and chemical composition of the diet are shown in Table 1. The four treatments of control (CON), low calcium propionate (LCaP), medium calcium propionate (MCaP), and high calcium propionate (HCaP) groups were oral drenched with 0, 200, 350, and 500 g/day calcium propionate (Jiangsu Runpu Food Technology Co. LTD., Lianyungang, Jiangsu, China) per cow from calving to days 35 postpartum, respectively. The calcium propionate was oral drenched three times a day in equal amounts after milking. During the experiment, the cows were milked three times a day at 6:00, 14:00, and 22:00, and the TMR was fed ad libitum after milking. The TMR was adjusted according to the orts and made sure there were 5% ~ 10% orts every day. The cows were housed in individual stalls and had free access to water.
The TMR samples were collected from the trough weekly on 2 consecutive days, composited to get one sample per week. The obtained samples were dried at 55°C for 48 h in an oven, and ground through a 1-mm screen for chemical composition analysis. The composition and nutrient levels of TMR were analyzed according to the methods of the Association of Official Analytical Chemists (AOAC, 2005). The DM was measured by drying the samples at 105°C for 3 h (method 934.01; AOAC, 2005). The nitrogen (N) content was determined by the Kjeldahl method and crude protein (CP) was calculated as 6.25 × N (method 954.01; AOAC, 2005). Ether extract (EE) was measured by the weight loss of DM after extraction with diethyl ether in a Soxhlet extraction apparatus for 8 h (method 920.39; AOAC, 2005). The content of ash was measured in a muffle furnace for 6 h at 550°C (method 942.05; AOAC, 2005). The calcium (Ca) was measured by an M9W-700 atomic absorption spectrophotometer (Perkin-Elmer Corp, Norwalk, CT, United States; method 968.08; AOAC, 2005). Total phosphorus (P) was analyzed by the molybdovanadate colorimetric method (method 946.06; AOAC, 2005). The neutral detergent fiber (NDF) was analyzed with heat-stable α-amylase and expressed inclusive of residual ash (Van Soest et al., 1991). The acid detergent fiber (ADF) content in TMR was determined according to the method 973.18 of AOAC (2005).
Ruminal Sample Collection and Chemical Analyses
Ruminal fluid was collected from six cows of each group at days 7, 14, 21, 28, and 35 of parturition. Cows were sampled by an oral stomach tube and 200 ml syringe before morning feeding. The initial 150 ml was discarded to minimize saliva contamination. The ruminal pH value was measured immediately using a pH meter (Model 144 PB-10, Sartorius Co., Germany). The rumen fluid was filtered through four layers. The filtered liquid sample of about 10 ml was collected in a 15 ml centrifuge tube, acidified with 25% (w/v) metaphosphoric acid (5:1), and stored at −20°C for analysis of ruminal fermentation parameters. The concentrations of volatile fatty acids (VFA) profile were measured by the Agilent 6890 N gas chromatograph (Agilent Technologies Inc., Santa Clara, CA, United States) fitted with a capillary column (HP-FFAP19095F-123, 30 m × 0.53 mm diameter and 1 mm thickness, Agilent Technologies, Inc., Santa Clara, CA, United States; Jia et al., 2018). The ammonia nitrogen (NH3-N) was measured by the colorimetric method described by Lv et al. (2019) and microbial crude protein (MCP) was analyzed according to the method of Makkar et al. (1982). Then, 2 ml of the rumen fluid for each cow in the last time (days 35 postpartum) was collected in one sterile Eppendorf tube and stored in liquid nitrogen for DNA extraction.
DNA Extraction, Amplification, and 16S rRNA Gene Sequencing
The microbial DNA of the ruminal fluid samples (days 35 postpartum) was extracted using a commercial DNA Isolation Kit (MoBio Laboratories, Carlsbad, CA, United States) according to the manufacturer’s instructions. The quality and purity of the genomic DNA were checked on 1% agarose gels and a NanoDrop 2000 UV spectrophotometer (ThermoFisher, Waltham, MA, United States). The V3-V4 hypervariable region of the bacterial 16S rRNA gene was amplified with the primers 338F (5′-ACTCCTACGGGAGGCAGCAG-3′) and 806R (5′-GGACTACNNGGGTATCTAAT-3′; Cui et al., 2019). For each ruminal sample, an eight-digit barcode sequence was added to the 5′ end of the forward and reverse primers (Allwegene Company, Beijing, China). The PCR was performed in a 25 μl reaction volumes, containing 12.5 μl 2 × Taq PCR Master Mix, 3 μl BSA (2 ng/μl), 1 μl Forward Primer (5 μM), 1 μl Reverse Primer (5 μM), 2 μl template DNA, and 5.5 μl dd H2O. The PCR cycling conditions were used as follows: 95°C for 5 min, followed by 28 cycles of 95°C for 45 s, 55°C for 50 s, and 72°C for 45 s with a final extension at 72°C for 10 min. The PCR amplicons products were extracted from 2% agarose gels and purified using an Agencourt AMPure XP Kit (Beckman Coulter, Brea, CA, United States). The purified amplicons were pooled in equimolar and paired-end sequenced (2 × 300) on an Illumina MiSeq platform (Illumina, San Diego, CA, United States).
Statistical and Bioinformatics Analysis
The raw sequences data were first screened and the sequences were discarded if they were shorter than 120 bp, had a quality score (≤20) over a 50 bp sliding window, any contained ambiguous bases, or did not exactly match to primer sequences and barcode tags. Only sequences that overlapped >10 bp and had <10% mismatches were assembled (Bi et al., 2018). The assembled sequences were clustered into operational taxonomic units (OTUs) at a similarity level of 97% (Edgar, 2013). After splicing, we use VSEARCH (v2.7.1) software to remove tags with a length of less than 230 bp. The Ribosomal Database Project (RDP) Classifier tool was used to classify all sequences into different taxonomic groups against the SILVA128 database (Cole et al., 2009). Alpha diversity values including observed species, Chao1, PD_whole_tree, Shannon index, and Simpson index of the ruminal bacterial communities were calculated using the QIIME software (v1.8.0) based on the OTU information. Based on the results of taxonomic annotation and relative abundance, R (v3.6.0) software was used for bar-plot diagram analysis. The principal coordinate analysis (PCoA) and nonmetric multidimensional scaling analysis (NMDS) were performed based on Bray-Curtis distance by QIIME1 (V1.8.0) and R packages vegan (v3.6.0) software (Ran et al., 2021). The raw sequencing data of this study were available in the NCBI SRA database with the BioProject ID of PRJNA791506.1
Statistical Analysis
Data about rumen fermentation parameters, the alpha diversity indices, the relative abundance of phylum and genus were analyzed using the MIXED procedure in SAS 9.4 (SAS Institute Inc., Cary, NC, United States). The repeated measures about rumen fermentation parameters included the fixed effects of treatment, lactation week, the interaction between diet treatment and lactation week, and the random effect of the block. The model about ruminal microbial data was analyzed with treatment as a fixed effect, and block as a random effect. The compound symmetry was used as the variance–covariance error structure in the process of analysis. Duncan’s multiple range tests were used to examine the significance among treatments. Orthogonal polynomial contrasts were used to determine the linear and quadratic responses to the increasing calcium propionate supplementation levels. The IML procedure of SAS was used to generate coefficients adjusted for the unequal spacing of calcium propionate supplementation in the diet. The data were presented as the least square mean and SEM. Statistical significance was assumed at p ≤ 0.05 and 0.05 < p ≤ 0.10 was designated as a tendency for all analyses.
Results
Rumen Fermentation Parameters of Different Dietary Treatments
The effects of calcium propionate supplementation on rumen fermentation of the 5 weeks were shown in Table 2. The calcium propionate supplementation did not affect the rumen fermentation of rumen pH, iso-butyrate, and the ratio of acetate to propionate. The concentration of MCP decreased quadratically (p = 0.02) with the increasing supplementation of calcium propionate. With the increasing feeding of calcium propionate, the content of NH3-N (p = 0.01) and total VFA (p = 0.05) increased linearly. Dietary supplementation with calcium propionate linearly increased the concentration of rumen acetate (p = 0.05), iso-valerate (p = 0.03), and valerate (p = 0.007), with the highest values in the HCaP group. Furthermore, a linear trend increase appeared in the concentration of propionate (p = 0.07) and butyrate (p = 0.07) as the calcium propionate dose increased. The molar proportions of individual rumen fluid VFA were shown in Supplementary Table S1. The molar proportions of acetate, propionate, and butyrate were not changed by the calcium propionate addition. However, the valerate proportion increased linearly (p = 0.004) with the dietary addition of calcium propionate.
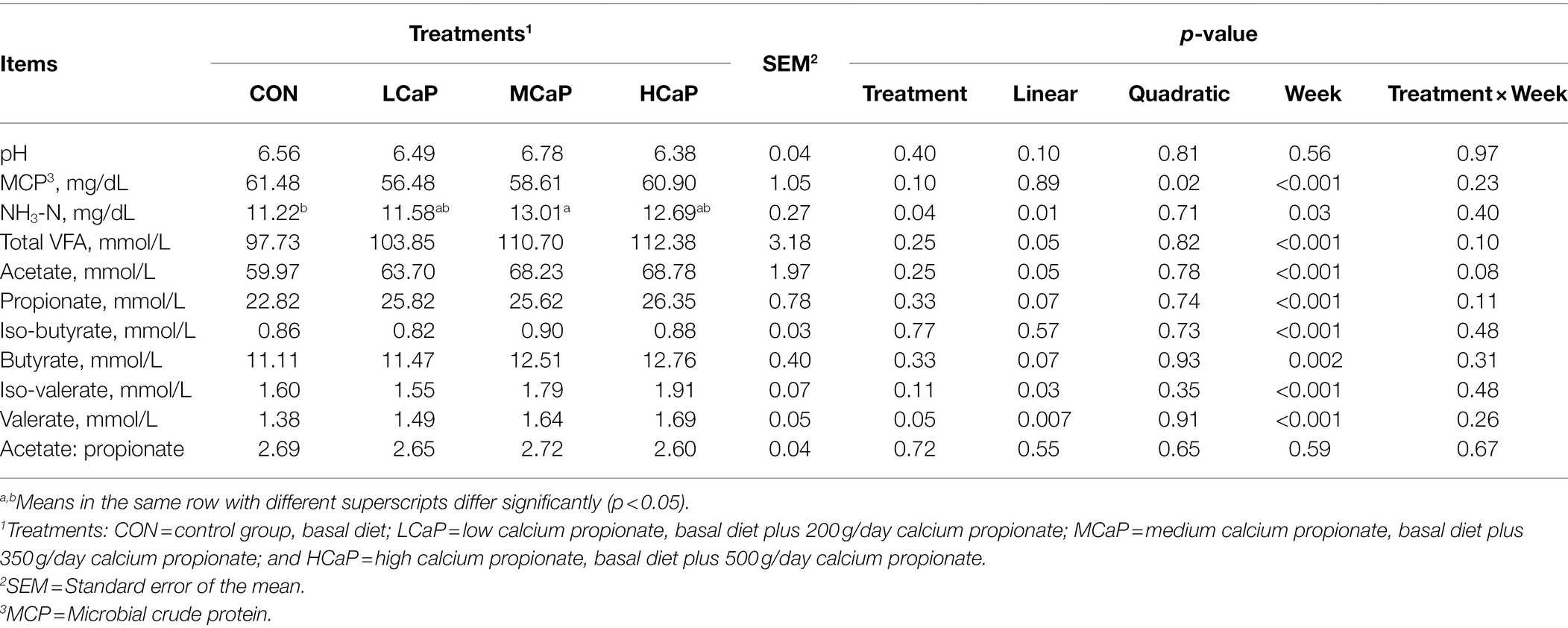
Table 2. Effects of dietary supplementation with calcium propionate on ruminal pH and fermentation parameters of dairy cows in early lactation.
Sequencing Depth, Coverage, and Index of Bacterial Community
A total of 1,642,279 high quality V3-V4 16S rRNA sequences were obtained from the 24 rumen samples, with an average of 68,428 sequence reads per sample (minimum 35,721; maximum, 163,397) after data quality checking, filtering, and removing of primers, chimeras, short sequences, and ambiguous bases. The length of the sequence reads was major distributed in 420–440 bp (Supplementary Table S2). In total, 3,442 OTUs were detected based on a similarity level ≥ 97%. The OUT numbers of CON, LCaP, MCaP, and HCaP groups were 2,938, 3,096, 3,025, and 2,928, respectively. The detailed common and unique OTUs numbers of ruminal bacteria among the four groups were shown in the Venn diagram (Supplementary Figure S1). The rarefaction curves (Supplementary Figure S2) of the rumen sample showed that the sequencing depth was sufficient and accurate to assess the diverse bacterial communities. The alpha diversity index analysis indicated that the supplementation of calcium propionate quadratically increased the Chao1 estimator (p = 0.02) and observed species (p = 0.03), but had no effect on the PD_whole_tree, Shannon index, and Simpson index (Figure 1; Supplementary Table S3).
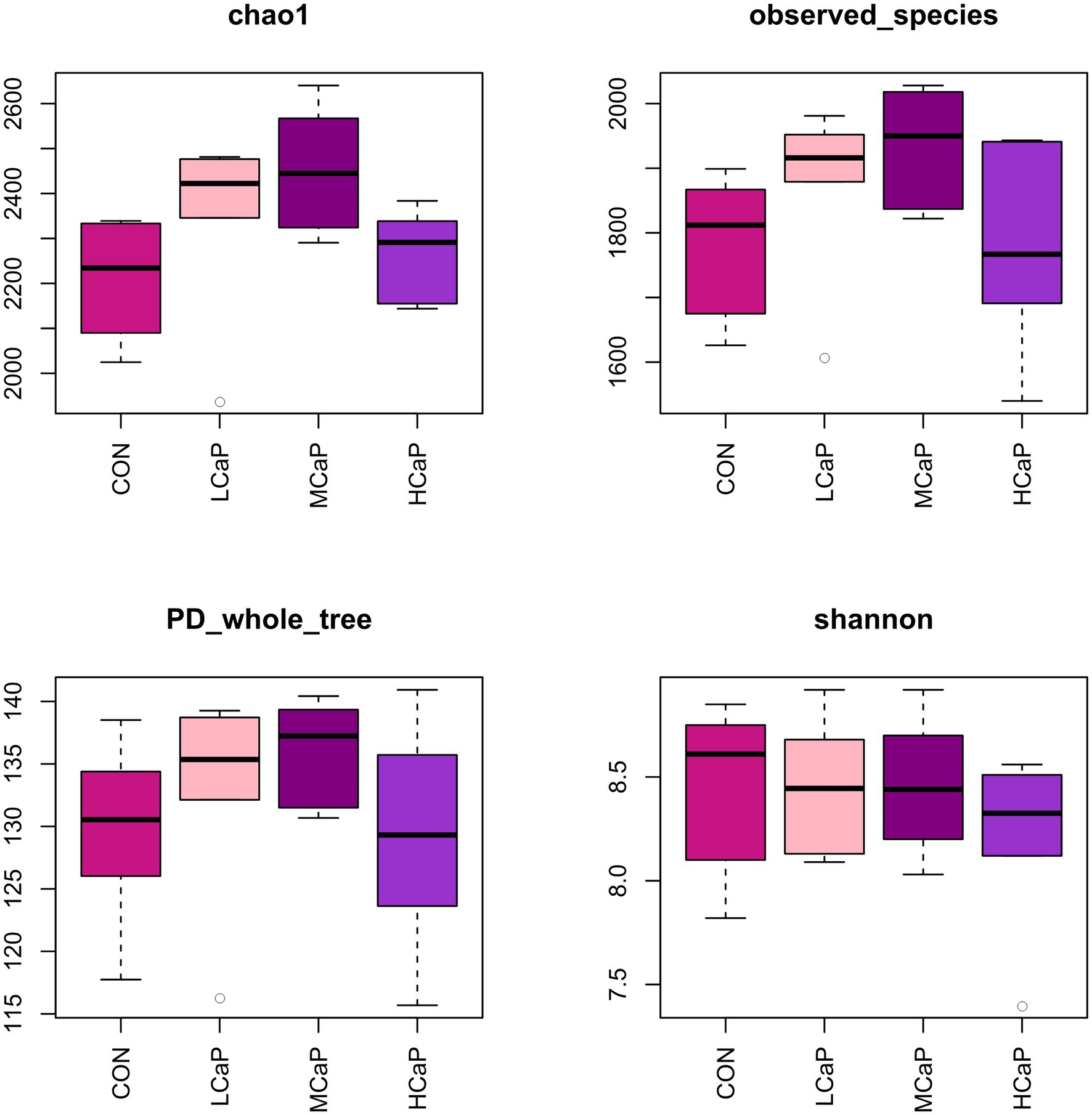
Figure 1. Alpha diversity of the rumen bacterial communities in dairy cows given different calcium propionate levels in early lactation. CON: control group, basal diet; LCaP: low calcium propionate, basal diet plus 200 g/day calcium propionate; MCaP: medium calcium propionate, basal diet plus 350 g/day calcium propionate; and HCaP: high calcium propionate, basal diet plus 500 g/day calcium propionate.
The β-diversity was performed to compare the differences in microbial diversity among the treatments (Supplementary Figure S3). The PCoA and NMDS plots showed that the separation among the treatments of microbial diversity was not obvious at the OUT level.
Rumen Bacterial Community Composition of Different Dietary Treatments
A comparison of the effects of calcium propionate supplementation on the rumen bacterial composition of early lactation dairy cows was performed by the taxonomic analysis. A total of 19 bacterial phyla were obtained from the 24 rumen samples. The most predominant phyla were Bacteroidetes (55.41% ± 1.56%, mean ± the SEM), Firmicutes (35.75% ± 1.34%), Proteobacteria (3.95% ± 0.58%), and Tenericutes (1.20% ± 0.17%) among the four groups (Figure 2A).
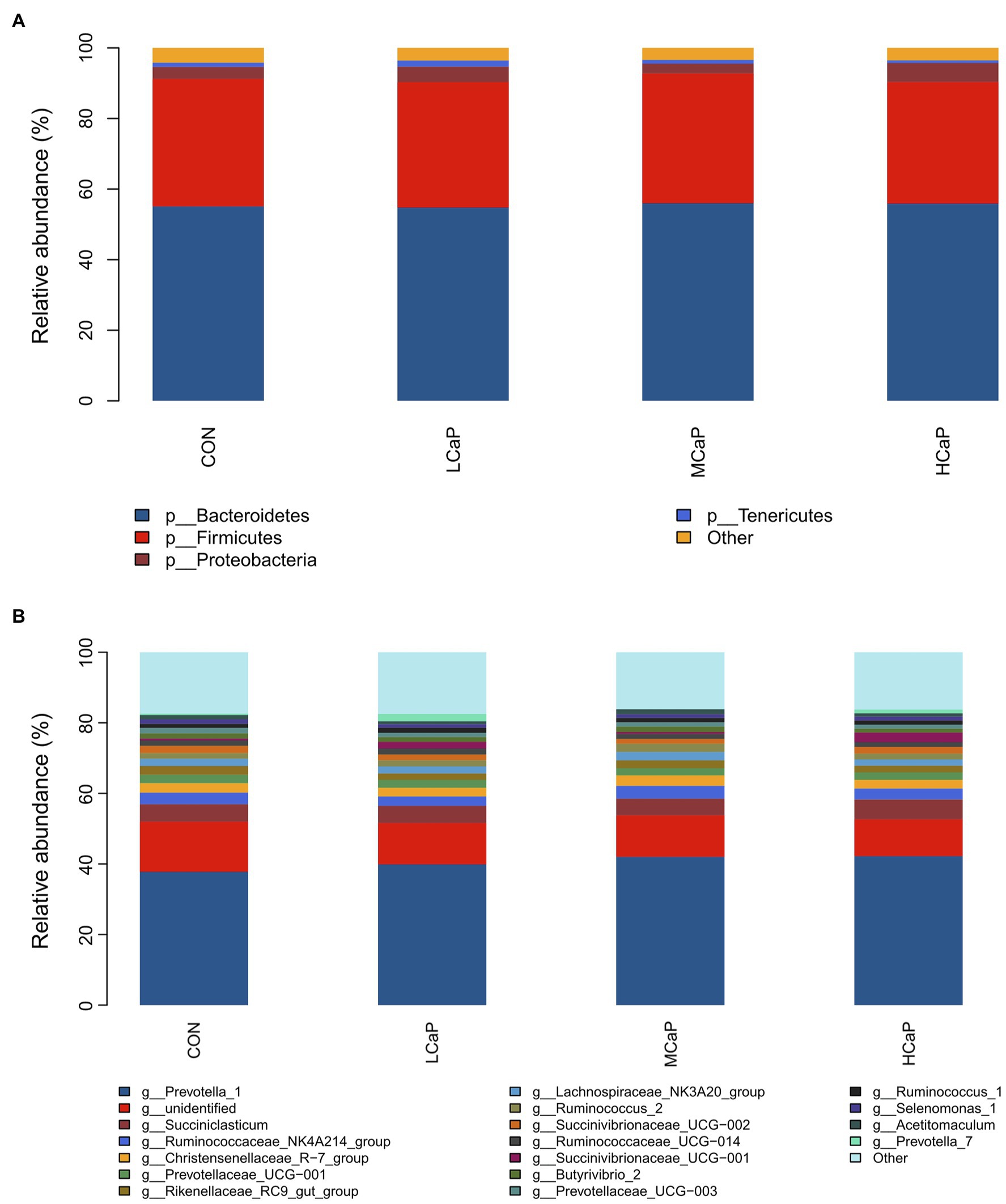
Figure 2. Distribution of the ruminal bacterial community composition across the four treatments. (A) Phylum level; (B) genus level. CON: control group, basal diet; LCaP: low calcium propionate, basal diet plus 200 g/day calcium propionate; MCaP: medium calcium propionate, basal diet plus 350 g/day calcium propionate; and HCaP: high calcium propionate, basal diet plus 500 g/day calcium propionate.
At the genus level, 254 genera belonging to 19 phyla were deleted in the samples. There were 16 most abundant shared genera with a relative abundance >1% in all the samples of the different treatments. Among these genera, Prevotella_1 (40.46% ± 1.76%), Succiniclasticum (5.03% ± 0.42%), Ruminococcaceae_NK4A214_group (3.15% ± 0.21%), Christensenellaceae_R-7_group (2.65% ± 0.21%), Prevotellaceae_UCG-001 (2.19% ± 0.16%), Rikenellaceae_RC9_gut_group (2.11% ± 0.16%), Lachnospiraceae_NK3A20_group (2.04% ± 0.16%), Ruminococcus_2 (1.84% ± 0.19%), Succinivibrionaceae_UCG-002 (1.74% ± 0.32%), Ruminococcaceae_UCG-014 (1.50% ± 0.11%), Succinivibrionaceae_UCG-001 (1.40% ± 0.48%), Butyrivibrio_2 (1.40% ± 0.14%), Prevotellaceae_UCG-003 (1.26% ± 0.08%), Ruminococcus_1 (1.25% ± 0.10%), Selenomonas_1 (1.10% ± 0.10%), and Acetitomaculum (1.10% ± 0.11%) were predominant across the four groups (Figure 2B).
As shown in Tables 3 , 4, different dietary calcium propionate supplement levels had little impact on the relative abundance of the major rumen bacteria compositions at phylum and genus level under the same basal dietary condition. The relative abundance of Prevotellaceae_UCG-003 (p = 0.09) showed a trend linear decrease with the increasing calcium propionate supplementation to the early lactation dairy cows.
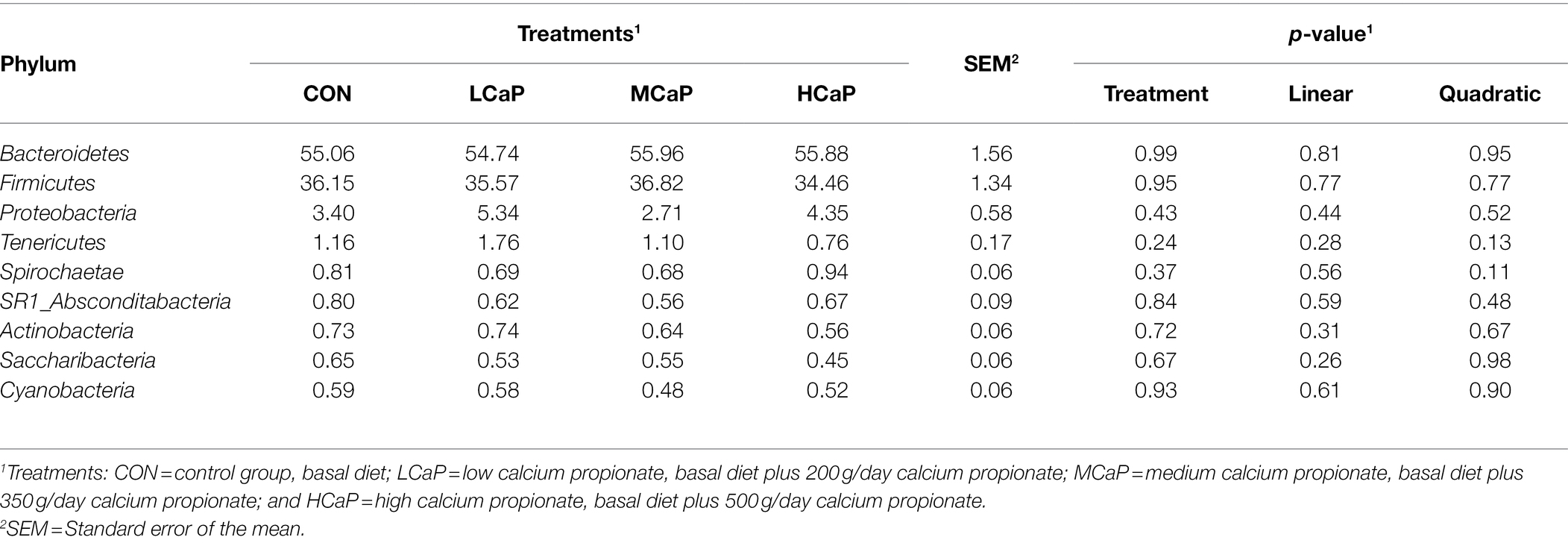
Table 3. Effects of dietary supplementation with calcium propionate on the relative abundance of ruminal bacterial communities at phylum level (average relative abundance ≥ 0.5% for at least one treatment) of dairy cows in early lactation.
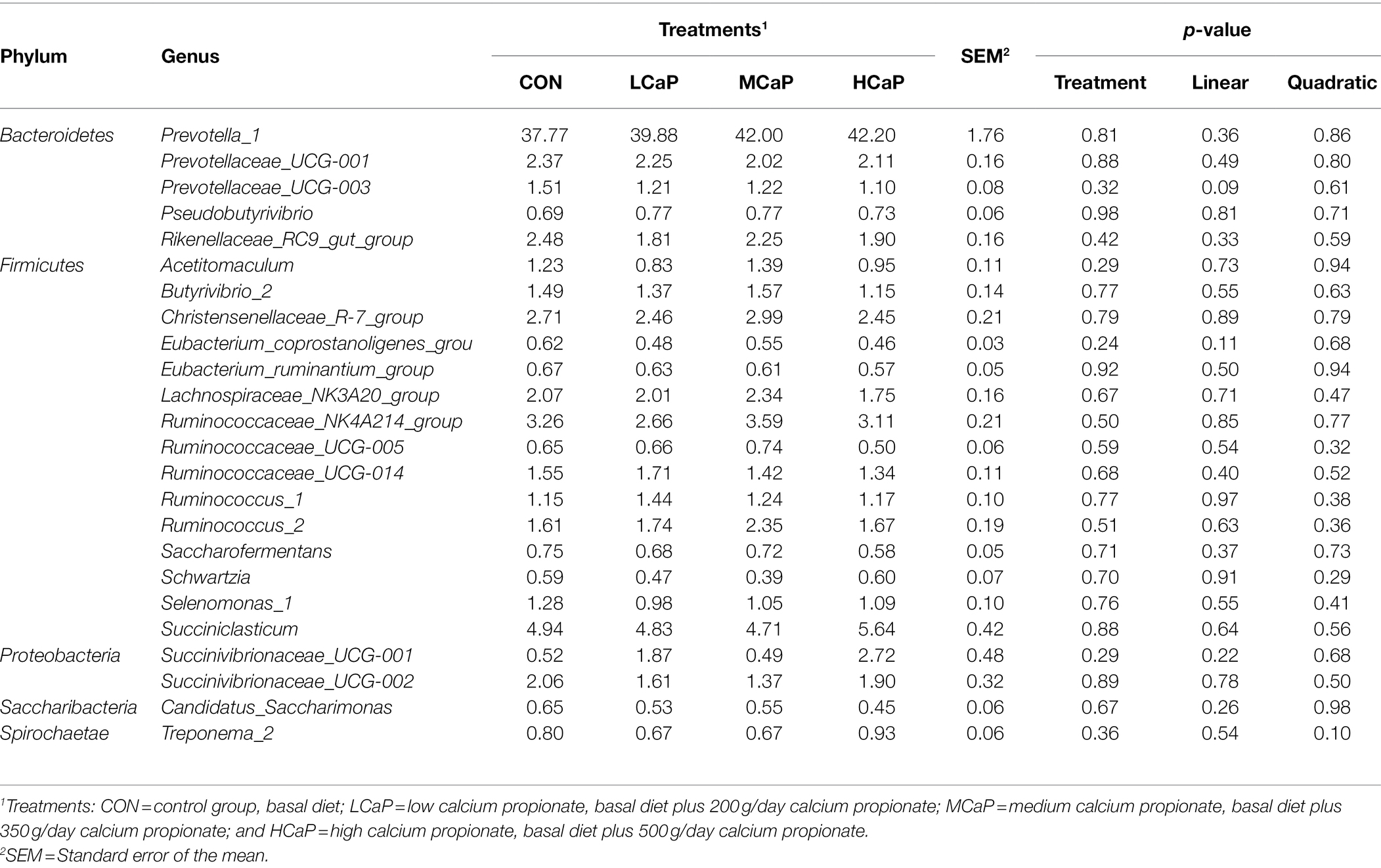
Table 4. Effects of dietary supplementation with calcium propionate on the relative abundance of ruminal bacterial communities at genera level (average relative abundance ≥ 0.5% for at least one treatment) of dairy cows in early lactation.
Discussion
Calcium propionate is widely used as a food and feed additive to inhibit mold or as a nutrition additive. Ruminal fermentation and rumen bacterial community play key roles in the production performance and health of dairy cows. The purpose of the study was to evaluate the effects of different dietary calcium propionate feeding levels, with the same TMR, on ruminal fermentation parameters and bacterial community composition in Holstein dairy cows by 16S rRNA high-throughput sequencing technology.
The fermentation characteristics of ruminal pH, MCP, NH3-N, and VFA concentration can reflect the function and the stability of the internal environment in the rumen. The ruminal pH is an important indicator of proper rumen fermentation and health (Zhang et al., 2018). Lee-Rangel et al. (2012) and Zhang et al. (2018) reported similar results that rumen pH was not affected by calcium propionate supplementation. The increased total VFA concentration is commonly associated with lower rumen pH (Fregulia et al., 2021). But in this study, the increasing total VFA concentration did not change the rumen pH may be related to the weakly alkaline properties of calcium propionate aqueous solution (Zhang et al., 2020). The unchanged rumen pH indicated that the calcium propionate supplementation did not affect the rumen normal function.
Ruminal NH3-N concentration is the balance between dietary protein degradation, and microbial utilization (Jiao et al., 2021). Liu et al. (2009) found that the ruminal CP degradability of concentration mix was linearly and quadratically increased with greater calcium propionate supplementation in finishing steers. The increased CP degradation resulted in a higher ruminal NH3-N concentration (Unnawong et al., 2021). The linearly increased concentration of ruminal NH3-N and the quadratically decreased MCP concentration in the calcium propionate treatment groups indicated that less ruminal NH3-N was incorporated into MCP or the MCP consumption increased in the treatments. Propionate is a great contributor to gluconeogenesis and supports milk synthesis for dairy cows (Reynolds et al., 2003). Miettinen and Huhtanen (1996) and Sheperd and Combs (1998) observed propionate infusion improved the milk and protein yield. The dairy cows fed calcium propionate had more milk yield and milk protein levels (Martins et al., 2019; Zhang et al., 2022), which improved the protein requirement during early lactation. Therefore, it was speculated that improving milk and protein yield might result in increasing MCP consumption by improving MCP flow to small intestines.
The VFAs, also known as short-chain fatty acids, are produced by microbial fermentation of carbohydrates and endogenous substrates and supply approximately 70% of the energy required to ruminants (Bergman, 1990). The increased ruminal VFAs concentration in the calcium propionate treatment groups stimulated the capacity for VFA absorption (Dieho et al., 2017), which was beneficial to alleviate NEB for early lactation dairy cows. It was reported that calcium propionate supplementation in calves could increase the rumen acetate, propionate, and butyrate concentrations (Zhang et al., 2018). Similarly, in the present study, it was found that the concentrations of acetate, propionate, butyrate, iso-valerate, and valerate were increasing in the calcium propionate supplementation groups. As a precursor of glucose, the advantage of propionate feeding to dairy cows is that it can improve milk production and feed intake (McNamara and Valdez, 2005). Cifuentes-Lopez et al. (2021) also observed the rumen total VFA increased linearly as dietary calcium propionate increased in fishing lambs. Liu et al. (2009) indicated that the calcium propionate supplementation improved the rumen fermentation and the in situ ruminal degradation of organic matter, NDF, and CP in beef cattle. Our previous study also showed that the dry matter intake of dairy cows showed a quadratic increase with the increasing supplementation of calcium propionate (Zhang et al., 2022). It was accepted that the increased feed intake and rumen nutrient digestibility improved the ruminal VFAs concentration of dairy cows in early lactation.
Calcium propionate is dissociated to Ca2+ and propionic acid in rumen aqueous solutions (Zhang et al., 2018). In this study, the exogenous supplementation of calcium propionate did not improve the molar proportion of propionate. Propionate acted as a gluconeogenic precursor (Kennedy et al., 2020) and a signaling molecule to stimulate rumen development (Sakata and Tamate, 1979; Zhang et al., 2018). The growth and integrity of the rumen epithelium were important for the absorption of VFAs (Zebeli et al., 2015). Therefore, it was speculated that the unchanged molar proportion of propionate may be related to the sampling time and the improvement of rumen epithelium absorption capacity. The rapid ruminal propionate absorption resulted in a brief increase in propionate molar proportion after feeding, but not changed at the sampling time (before morning feeding). With the increasing absorption of propionate as glucose precursors, we had found that feeding calcium propionate improves milk production of dairy cows in the previous study (Zhang et al., 2022). Lee-Rangel et al. (2012) also indicated the proportion of rumen acetate, propionate, and butyrate were not affected by the addition of calcium propionate in the diet of lambs. The increased total concentration of VFA and the unchanged molar proportion of acetate, propionate, and butyrate indicated that calcium propionate supplementation can improve rumen VFA production, but had minimal effects on fermentation mode.
There was little reported previously about the effects of calcium propionate feeding levels on the ruminal bacterial community of dairy cows in early lactation by pyrosequencing of the 16S RNA gene. The alpha diversity of bacterial communities was evaluated by quantitative methods. It showed that the calcium propionate feeding quadratically increased the Chao 1 index and the observed_species, which suggested that the 350 g/day calcium propionate feeding level may improve bacterial diversity and richness in the rumen. However, compared with the MCaP group, the Chao 1 index and the observed_species in the HCaP group were decreasing, indicating the feeding level of 500 g/day exceeded the optimal feeding level. The relative abundance of rumen bacterial genera has a significant correlation with the apparent digestibility of nutrients and rumen fermentation characteristics (He et al., 2018). Feeding calcium propionate improved the alpha diversity of the rumen bacterial community, which explained the increases in rumen NH3-N and the total VFA concentration of the calcium propionate treatment groups.
The PCoA and NMDS results also verified no significant differences in ruminal bacterial community structures among the four groups. In this study, the relative abundance of dominant phyla and the majority of the genera with the relative abundance ≥ 0.5% were not affected by the calcium propionate treatments. He et al. (2018) found that calcium salt of long-chain fatty acids increased microbial diversity index, but no significant difference in bacteria abundance at the genus level was found in Holstein bulls. The data of Yao et al. (2017) suggested that the ruminal bacterial community composition was nearly unchanged by calcium propionate supplementation in finishing bulls. The dietary changes have important impacts on rumen bacterial communities, but the dietary forage to concentrate ratio was the main factor affecting the rumen microbial population structure under the same diet ingredients and processing conditions (Bi et al., 2018). It was reported the rapid transition to a high-grain diet resulted in dynamic changes in the sheep rumen microbiome (Seddik et al., 2019). Bi et al. (2018) reported that an 8% difference in dietary energy levels had little impact on rumen bacterial community composition in heifers. In contrast, Cao et al. (2020) indicated calcium propionate supplementation decreased the diversity of bacteria and altered the ruminal microbiota in calves both pre- and postweaning. The difference may be related to the experimental animals and basal diet. Propionic acid and calcium ions were normally present in rumen. The early lactation dairy cows required large amounts of propionic acid and calcium ions for alleviating NEB and hypocalcemia, respectively. After calcium propionate was rapidly absorbed by the rumen of dairy cows for milk synthesis, the composition of rumen fluid remained relatively balanced. Therefore, the relative abundance of the majority rumen microbiota composition was not affected by the different calcium propionate feeding levels. This study showed no difference was found in the relative abundance of ruminal bacterial community composition among the different calcium propionate feeding levels, which was also consistent with the similar molar proportions of VFA among the treatments.
The Bacteroidetes, Firmicutes, and Proteobacteria were the dominant phyla in the rumen of the study, which was similar to the previous study (Wang et al., 2019, 2021c). The sequence analysis of the reads from the four treatments showed the phylum Bacteroidetes was the dominant bacteria, which was mainly composed of the genus Prevotella_1. Many reports suggested that the Prevotella genus was the dominant ruminal genus in the abundance of dairy cows (He et al., 2018; Mu et al., 2021), which was similar to the results of ours. Prevotella, which can degrade starch and protein, had many OTUs strongly associated with feed efficiency (Fregulia et al., 2021). In our study, the relative abundance of Prevotella_1 increased with the supplementation of calcium propionate, but there were no significant differences among the four groups. The NH3-N concentration was significantly positively correlated with the relative abundance of Prevotella_1 (Bi et al., 2018; Cao et al., 2020), and the numerical increase in relative abundance was likely linked to the higher degradation of protein and greater NH3-N production (He et al., 2018). Succiniclasticum was another prevalent genus in this study. And the high relative abundance of Succiniclasticum was commonly observed in dairy cows fed high levels of concentration (Bi et al., 2018; Wang et al., 2021a). The results of the present study were similar to the report of Yao et al. (2017), in which, the relative abundance of Succiniclasticum was also not affected by calcium propionate supplementation. The dominant rumen microorganisms were not significantly different among the four groups eventually led to no changed molar proportions of VFA.
In general, there was no significant change in the abundance of microbial community composition at phyla and genus levels, which may be due to the same dietary TMR, indicating that dietary calcium propionate feeding level did not affect the rumen bacterial community.
Conclusion
This study demonstrated that the supplementation of calcium propionate to the dairy cows in early lactation had no significant impact on major rumen bacterial community composition under the same basal TMR diet. Furthermore, Bacteroidetes, Firmicutes, and Proteobacteria were the dominant phyla in all the treatments. However, the calcium propionate supplementation increased the alpha diversity of the rumen bacterial communities and improved rumen fermentation of the dairy cows. This study is useful to guide future research on investigating the calcium propionate application in alleviating NEB and hypocalcemia of early lactation dairy cows.
Data Availability Statement
The datasets presented in this study can be found in online repositories. The names of the repository/repositories and accession number(s) can be found in the article/Supplementary Material.
Ethics Statement
The animal study was reviewed and approved by Animal Care Committee of the Chinese Academy of Agricultural Sciences (Beijing, China).
Author Contributions
FZ, YG, and BX conceived and designed the experiment. FZ and YW were involved in the animal experiment and sample collection. FZ analyzed the data and completed the initial manuscript. HW and XN completed the language editing and revised the manuscript. BX provided funding support. All authors contributed to the article and approved the submitted version.
Funding
This study was financially supported by the Central Public-Interest Scientific Institution Basal Research Fund (No. 2019-YWF-YTS-10), State Key Laboratory of Animal Nutrition (2004DA125184G2104), and Beijing Dairy Industry Innovation Team (bjcystx-ny-1).
Conflict of Interest
The authors declare that the research was conducted in the absence of any commercial or financial relationships that could be construed as a potential conflict of interest.
Publisher’s Note
All claims expressed in this article are solely those of the authors and do not necessarily represent those of their affiliated organizations, or those of the publisher, the editors and the reviewers. Any product that may be evaluated in this article, or claim that may be made by its manufacturer, is not guaranteed or endorsed by the publisher.
Acknowledgments
The authors would like to thank the crew of the China-Israel demonstration dairy farm in Beijing for their help in animal management.
Supplementary Material
The Supplementary Material for this article can be found online at: https://www.frontiersin.org/articles/10.3389/fmicb.2022.847488/full#supplementary-material
Footnotes
References
AOAC (2005). Official Methods of Analysis of AOAC International. 18th Edn. Arlington, VA, USA: Association of Official Analytical Chemist.
Aschenbach, J. R., Kristensen, N. B., Donkin, S. S., Hammon, H. M., and Penner, G. B. (2010). Gluconeogenesis in dairy cows: the secret of making sweet milk from sour dough. IUBMB Life 62, 869–877. doi: 10.1002/iub.400
Bergman, E. N. (1990). Energy contributions of volatile fatty acids from the gastrointestinal tract in various species. Physiol. Rev. 70, 567–590. doi: 10.1152/physrev.1990.70.2.567
Bi, Y. L., Zeng, S. Q., Zhang, R., Diao, Q. Y., and Tu, Y. (2018). Effects of dietary energy levels on rumen bacterial community composition in Holstein heifers under the same forage to concentrate ratio condition. BMC Microbiol. 18:69. doi: 10.1186/s12866-018-1213-9
Cao, N., Wu, H., Zhang, X. Z., Meng, Q. X., and Zhou, Z. M. (2020). Calcium propionate supplementation alters the ruminal bacterial and archaeal communities in pre- and postweaning calves. J. Dairy Sci. 103, 3204–3218. doi: 10.3168/jds.2019-16964
Cifuentes-Lopez, O., Lee-Rangel, H. A., Mendoza, G. D., Delgado-Sanchez, P., Guerrero-Gonzalez, L., Chay-Canul, A., et al. (2021). Effects of dietary calcium propionate supplementation on hypothalamic neuropeptide messenger RNA expression and growth performance in finishing Rambouillet lambs. Life 11:566. doi: 10.3390/life11060566
Cole, J. R., Wang, Q., Cardenas, E., Fish, J., Chai, B., Farris, R. J., et al. (2009). The ribosomal database project: improved alignments and new tools for rRNA analysis. Nucleic Acids Res. 37, D141–D145. doi: 10.1093/nar/gkn879
Cui, K., Qi, M. L., Wang, S. Q., Diao, Q. Y., and Zhang, N. F. (2019). Dietary energy and protein levels influenced the growth performance, ruminal morphology and fermentation and microbial diversity of lambs. Sci. Rep. 9:16612. doi: 10.1038/s41598-019-53279-y
Dieho, K., van Baal, J., Kruijt, L., Bannink, A., Schonewille, J. T., Carreno, D., et al. (2017). Effect of supplemental concentrate during the dry period or early lactation on rumen epithelium gene and protein expression in dairy cattle during the transition period. J. Dairy Sci. 100, 7227–7245. doi: 10.3168/jds.2016-12403
Edgar, R. C. (2013). UPARSE: highly accurate OTU sequences from microbial amplicon reads. Nat. Methods 10, 996–998. doi: 10.1038/Nmeth.2604
Fregulia, P., Neves, A. L. A., Dias, R. J. P., and Campos, M. M. (2021). A review of rumen parameters in bovines with divergent feed efficiencies: what do these parameters tell us about improving animal productivity and sustainability? Livest. Sci. 254:104761. doi: 10.1016/j.livsci.2021.104761
Goff, J. P., and Horst, R. L. (1993). Oral administration of calcium salts for treatment of hypocalcemia in cattle. J. Dairy Sci. 76, 101–108. doi: 10.3168/jds.S0022-0302(93)77328-2
Goff, J. P., Horst, R. L., Jardon, P. W., Borelli, C., and Wedam, J. (1996). Field trials of an oral calcium propionate paste as an aid to prevent milk fever in periparturient dairy cows. J. Dairy Sci. 79, 378–383. doi: 10.3168/jds.S0022-0302(96)76375-0
He, Y., Niu, W. J., Qiu, Q. H., Xia, C. Q., Shao, T. Q., Wang, H. B., et al. (2018). Effect of calcium salt of long-chain fatty acids and alfalfa supplementation on performance of Holstein bulls. Oncotarget 9, 3029–3042. doi: 10.18632/oncotarget.23073
Jia, P., Cui, K., Ma, T., Wan, F., Wang, W. Y., Yang, D., et al. (2018). Influence of dietary supplementation with Bacillus licheniformis and Saccharomyces cerevisiae as alternatives to monensin on growth performance, antioxidant, immunity, ruminal fermentation and microbial diversity of fattening lambs. Sci. Rep. 8:16712. doi: 10.1038/s41598-018-35081-4
Jiao, P. X., Hu, G. H., Liang, G. G., Chen, M. M., An, N., Wang, Z. W., et al. (2021). Dietary supplementation with Macleaya cordata extract inclusion affects growth performance, rumen fermentation, bacterial communities, and immune responses of weaned lambs. Anim. Feed Sci. Technol. 282:115127. doi: 10.1016/j.anifeedsci.2021.115127
Kara, C. (2013). Physiological and metabolic changes during the transition period and the use of calcium propionate for prevention or treatment of hypocalcemia and ketosis in periparturient cows. J. Biol. Environ. Sci. 7, 9–17.
Kataev, V. Y., Sleptsov, I., Martynov, A., Aduchiev, B. K., Khlopko, Y. A., Miroshnikov, S. A., et al. (2020). Data on rumen and faeces microbiota profiles of Yakutian and Kalmyk cattle revealed by high-throughput sequencing of 16S rRNA gene amplicons. Data Brief 33:106407. doi: 10.1016/j.dib.2020.106407
Kennedy, K. M., Donkin, S. S., and Allen, M. S. (2020). Effects of propionate concentration on short-term metabolism in liver explants from dairy cows in the postpartum period. J. Dairy Sci. 103, 11449–11460. doi: 10.3168/jds.2020-18914
Lee-Rangel, H. A., Mendoza, G. D., and Gonzalez, S. S. (2012). Effect of calcium propionate and sorghum level on lamb performance. Anim. Feed Sci. Technol. 177, 237–241. doi: 10.1016/j.anifeedsci.2012.08.012
Liu, Q., Wang, C., Guo, G., Yang, W. Z., Dong, K. H., Huang, Y. X., et al. (2009). Effects of calcium propionate on rumen fermentation, urinary excretion of purine derivatives and feed digestibility in steers. J. Agric. Sci. 147, 201–209. doi: 10.1017/S0021859609008429
Liu, Q., Wang, C., Yang, W. Z., Guo, G., Yang, X. M., He, D. C., et al. (2010). Effects of calcium propionate supplementation on lactation performance, energy balance and blood metabolites in early lactation dairy cows. J. Anim. Physiol. Anim. Nutr. 94, 605–614. doi: 10.1111/j.1439-0396.2009.00945.x
Liu, C., Wu, H., Liu, S. J., Chai, S. T., Meng, Q. X., and Zhou, Z. M. (2019). Dynamic alterations in yak rumen bacteria community and metabolome characteristics in response to feed type. Front. Microbiol. 10:1116. doi: 10.3389/fmicb.2019.01116
Lv, X. K., Chai, J. M., Diao, Q. Y., Huang, W. Q., Zhuang, Y. M., and Zhang, N. F. (2019). The signature microbiota drive rumen function shifts in goat kids introduced to solid diet regimes. Microorganisms 7:516. doi: 10.3390/microorganisms7110516
Makkar, H. P., Sharma, O. P., Dawra, R. K., and Negi, S. S. (1982). Simple determination of microbial protein in rumen liquor. J. Dairy Sci. 65, 2170–2173. doi: 10.3168/jds.S0022-0302(82)82477-6
Martins, W. D. C., Cunha, S. H. M., Boscarato, A. G., De Lima, J. S., Junior, J. D. E., Uliana, G. C., et al. (2019). Calcium propionate increased milk parameters in Holstein cows. Acta Sci. Vet. 47:1691. doi: 10.22456/1679-9216.97154
McNamara, J. P., and Valdez, F. (2005). Adipose tissue metabolism and production responses to calcium propionate and chromium propionate. J. Dairy Sci. 88, 2498–2507. doi: 10.3168/jds.S0022-0302(05)72927-1
Miettinen, H., and Huhtanen, P. (1996). Effects of the ratio of ruminal propionate to butyrate on milk yield and blood metabolites in dairy cows. J. Dairy Sci. 79, 851–861. doi: 10.3168/jds.S0022-0302(96)76434-2
Mu, Y. Y., Qi, W. P., Zhang, T., Zhang, J. Y., Mei, S. J., and Mao, S. Y. (2021). Changes in rumen fermentation and bacterial community in lactating dairy cows with subacute rumen acidosis following rumen content transplantation. J. Dairy Sci. 104, 10780–10795. doi: 10.3168/jds.2021-20490
NRC (2001). Nutrient Requirements of Dairy Cattle. 7th Edn. Washington, DC: National Academies Press.
Pehrson, B., Svensson, C., and Jonsson, M. (1998). A comparative study of the effectiveness of calcium propionate and calcium chloride for the prevention of parturient paresis in dairy cows. J. Dairy Sci. 81, 2011–2016. doi: 10.3168/jds.S0022-0302(98)75775-3
Ran, T., Tang, S. X., Yu, X., Hou, Z. P., Hou, F. J., Beauchemin, K. A., et al. (2021). Diets varying in ratio of sweet sorghum silage to corn silage for lactating dairy cows: feed intake, milk production, blood biochemistry, ruminal fermentation, and ruminal microbial community. J. Dairy Sci. 104, 12600–12615. doi: 10.3168/jds.2021-20408
Reynolds, C. K., Aikman, P. C., Lupoli, B., Humphries, D. J., and Beever, D. E. (2003). Splanchnic metabolism of dairy cows during the transition from late gestation through early lactation. J. Dairy Sci. 86, 1201–1217. doi: 10.3168/jds.S0022-0302(03)73704-7
Sakata, T., and Tamate, H. (1979). Rumen epithelium cell proliferation accelerated by propionate and acetate. J. Dairy Sci. 62, 49–52. doi: 10.3168/jds.S0022-0302(79)83200-2
Seddik, H., Xu, L., Wang, Y., and Mao, S. Y. (2019). A rapid shift to high-grain diet results in dynamic changes in rumen epimural microbiome in sheep. Animal 13, 1614–1622. doi: 10.1017/S1751731118003269
Serrenho, R. C., DeVries, T. J., Duffield, T. F., and LeBlanc, S. J. (2021). Graduate student literature review: what do we know about the effects of clinical and subclinical hypocalcemia on health and performance of dairy cows? J. Dairy Sci. 104, 6304–6326. doi: 10.3168/jds.2020-19371
Sheperd, A. C., and Combs, D. K. (1998). Long-term effects of acetate and propionate on voluntary feed intake by midlactation cows. J. Dairy Sci. 81, 2240–2250. doi: 10.3168/jds.S0022-0302(98)75803-5
Unnawong, N., Cherdthong, A., and So, S. (2021). Influence of supplementing Sesbania grandiflora pod meal at two dietary crude protein levels on feed intake, fermentation characteristics, and methane mitigation in Thai purebred beef cattle. Vet. Sci. 8:35. doi: 10.3390/vetsci8020035
Van Soest, P. J., Robertson, J. B., and Lewis, B. A. (1991). Methods for dietary fiber, neutral detergent fiber, and nonstarch polysaccharides in relation to animal nutrition. J. Dairy Sci. 74, 3583–3597. doi: 10.3168/jds.S0022-0302(91)78551-2
Wang, B., Ma, M. P., Diao, Q. Y., and Tu, Y. (2019). Saponin-induced shifts in the rumen microbiome and metabolome of young cattle. Front. Microbiol. 10:356. doi: 10.3389/fmicb.2019.00356
Wang, Y., Nan, X., Zhao, Y., Jiang, L., Wang, H., Zhang, F., et al. (2021b). Dietary supplementation of inulin ameliorates subclinical mastitis via regulation of rumen microbial community and metabolites in dairy cows. Microbiol. Spectr. 9:e0010521. doi: 10.1128/Spectrum.00105-21
Wang, K., Nan, X. M., Zhao, Y. G., Tong, J. J., Jiang, L. S., and Xiong, B. H. (2021a). Effects of propylene glycol on in vitro ruminal fermentation, methanogenesis, and microbial community structure. J. Dairy Sci. 104, 2924–2934. doi: 10.3168/jds.2020-18974
Wang, Y. P., Nan, X. M., Zhao, Y. G., Wang, Y., Jiang, L. S., and Xiong, B. H. (2021c). Ruminal degradation of rumen-protected glucose influences the ruminal microbiota and metabolites in early-lactation dairy cows. Appl. Environ. Microbiol. 87, e01908–e01920. doi: 10.1128/AEM.01908-20
Yao, Q., Li, Y., Meng, Q., and Zhou, Z. (2017). The effect of calcium propionate on the ruminal bacterial community composition in finishing bulls. Asian-Australas J. Anim. Sci. 30, 495–504. doi: 10.5713/ajas.16.0469
Zebeli, Q., Ghareeb, K., Humer, E., Metzler-Zebeli, B. U., and Besenfelder, U. (2015). Nutrition, rumen health and inflammation in the transition period and their role on overall health and fertility in dairy cows. Res. Vet. Sci. 103, 126–136. doi: 10.1016/j.rvsc.2015.09.020
Zhang, X. Z., Chen, W. B., Wu, X., Zhang, Y. W., Jiang, Y. M., Meng, Q. X., et al. (2018). Calcium propionate supplementation improves development of rumen epithelium in calves via stimulating G protein-coupled receptors. Animal 12, 2284–2291. doi: 10.1017/S1751731118000289
Zhang, F., Nan, X. M., Wang, H., Guo, Y. M., and Xiong, B. H. (2020). Research on the applications of calcium propionate in dairy cows: a review. Animals 10:1336. doi: 10.3390/ani10081336
Zhang, X. Z., Wu, X., Chen, W. B., Zhang, Y. W., Jiang, Y. M., Meng, Q. X., et al. (2017). Growth performance and development of internal organ, and gastrointestinal tract of calf supplementation with calcium propionate at various stages of growth period. PLoS One 12:e0179940. doi: 10.1371/journal.pone.0179940
Keywords: calcium propionate, dairy cows, early lactation, rumen fermentation, negative energy balance, ruminal bacterial community composition
Citation: Zhang F, Wang Y, Wang H, Nan X, Guo Y and Xiong B (2022) Calcium Propionate Supplementation Has Minor Effects on Major Ruminal Bacterial Community Composition of Early Lactation Dairy Cows. Front. Microbiol. 13:847488. doi: 10.3389/fmicb.2022.847488
Edited by:
Franck Carbonero, Washington State University Health Sciences Spokane, United StatesReviewed by:
Anusorn Cherdthong, Khon Kaen University, ThailandSonia Andrés, Spanish National Research Council (CSIC), Spain
Copyright © 2022 Zhang, Wang, Wang, Nan, Guo and Xiong. This is an open-access article distributed under the terms of the Creative Commons Attribution License (CC BY). The use, distribution or reproduction in other forums is permitted, provided the original author(s) and the copyright owner(s) are credited and that the original publication in this journal is cited, in accordance with accepted academic practice. No use, distribution or reproduction is permitted which does not comply with these terms.
*Correspondence: Yuming Guo, guoyum@cau.edu.cn; Benhai Xiong, xiongbenhai@caas.cn