- 1Department of Microbiology, Biological Sciences Center, State University of Londrina, Londrina, Brazil
- 2Department of Preventive Veterinary Medicine, Avian Medicine Laboratory, State University of Londrina, Londrina, Brazil
- 3Department of Pathology, Clinical Analysis and Toxicology, Health Sciences Center, State University of Londrina, Londrina, Brazil
Considering the worrying emergence of multidrug resistance, including in animal husbandry and especially in food-producing animals, the need to detect antimicrobial resistance strains in poultry environments is relevant, mainly considering a One Health approach. Thus, this study aimed to conduct longitudinal monitoring of antimicrobial resistance in broiler chicken farms, with an emphasis on evaluating the frequency of resistance to fosfomycin and β-lactams. Escherichia coli was isolated from broiler chicken farms (cloacal swabs, meconium, poultry feed, water, poultry litter, and Alphitobius diaperinus) in northern Paraná from 2019 to 2020 during three periods: the first period (1st days of life), the second period (20th to 25th days of life), and third period (40th to 42nd days of life). Antibiogram tests and the detection of phenotypic extended-spectrum β-lactamase (ESBL) were performed, and they were confirmed by seaching for genes from the blaCTX–M group. The other resistance genes searched were mcr-1 and fosA3. Some ESBL blaCTX–M–1 group strains were selected for ESBL identification by sequencing and enterobacterial repetitive intergenic consensus-polymerase chain reaction analysis. To determine the transferability of the blaCTX–M–1– and fosA3-carrying plasmids, strains were subjected to conjugation experiments. A total of 507 E. coli were analyzed: 360 from cloacal swabs, 24 from meconium samples, 3 from poultry feed samples, 18 from water samples, 69 from poultry litter samples, and 33 from A. diaperinus samples. Among the strain isolate, 80% (406/507) were multidrug-resistant (MDR), and 51% (260/507) were ESBL-positive, with the blaCTX–M–1 group being the most frequent. For the fosA3 gene, 68% (344/507) of the strains isolated were positive, deserves to be highlighted E. coli isolated from day-old chickens (OR 6.34, CI 2.34–17.17), when compared with strains isolated from other origins (poultry litter, A. diaperinus, water, and poultry feed). This work alerts us to the high frequency of the fosA3 gene correlated with the CTX-M-1 group (OR 3.57, CI 95% 2.7–4.72, p < 0.05), especially the blaCTX–M–55 gene, in broiler chickens. This profile was observed mainly in day-old chicken, with a high percentage of E. coli that were MDR. The findings emphasize the importance of conducting longitudinal monitoring to detect the primary risk points during poultry production.
Introduction
Antimicrobial resistance is a global public health threat, as infections with multiresistant bacteria are predicted to become the leading cause of death 30 years from now (O’Neill, 2014; European Commission, 2018). Approximately 33,000 deaths/year are estimated to occur in the European Union due to bacterial resistance to antibiotics (Cassini et al., 2019). Currently, strains with a high resistance profile (Avershina et al., 2021a,b; Zalewska et al., 2021), which could be aggravated by the increased use of antimicrobials during the SARS Cov-2 pandemic, are being increasingly detected (Abelenda-Alonso et al., 2020; Huttner et al., 2020; Lobie et al., 2021; Nieuwlaat et al., 2021).
The One Health concept allows for a greater understanding of the scope of this issue (Thanner et al., 2016; Aslam et al., 2021; Gazal et al., 2021), given the connection that exists between human, animal, and environmental health (Aslam et al., 2018; Aarestrup et al., 2021). Through this, the World Health Organization (WHO), together with the United Nations Food and Agriculture Organization and the World Organization for Animal Health, have promoted the monitoring and surveillance of resistance to antimicrobials, aiming to detect the possible causes and main points responsible for the selection of multiresistant strains (Poirel et al., 2018). Therefore, establishing programs to monitor resistance and control the use of antimicrobials are strategies that can minimize the rapid spread of antimicrobial resistance genes (Schnall et al., 2019).
Some studies have already shown that the poultry environment (Cunha et al., 2017; Saharan et al., 2020; Gazal et al., 2021) and its products (Koga et al., 2015; Davis et al., 2018; Cyoia et al., 2019) are responsible for harboring and transmitting multidrug-resistant (MDR) strains that produce extended-spectrum β-lactamase (ESBL) or other mechanisms with resistance to antimicrobials, that are considered the last resort in human medicine, such as fosfomycin and colistin (WHO, 2018, 2019).
Among the microorganisms present in the poultry environment, Escherichia coli stands out and is even considered a bioindicator of antimicrobial resistance (Poirel et al., 2018; Manges et al., 2019; Büdel et al., 2020). MDR strains facilitate the spread of resistance genes and enhance the phenomenon of antimicrobial resistance (Cazares et al., 2020). Among the genes that encode ESBL, the CTX-M group is predominant and the most widespread (Paterson and Bonomo, 2005; Laube et al., 2013; De Oliveira et al., 2020; Song et al., 2020). The blaCTX–M–1 group is the most prevalent gene detected from the CTX-M group, and the CTX-M-55 enzyme has already been described in animal production in countries from Europe, China, and Brazil (Cunha et al., 2017; Lukman et al., 2017; Lupo et al., 2018; Park et al., 2019; Gazal et al., 2021; Lay et al., 2021).
Fosfomycin is used in human medicine in cases of urinary tract infections caused by MDR strains in Brazil (Huttner et al., 2018; Camposda et al., 2020) and cases of bacterial prostatitis (Kwan and Beahm, 2020), with the fosA3 gene being the most frequently associated with fosfomycin resistance.
Brazil is the world’s largest exporter and the second-largest chicken meat producer. Paraná is the most productive state, and when its production is added to the other states of the southern region of Brazil, it represents 60% of the country’s chicken meat production (Aquino, 2021). In the state, the broiler breeding system works by integrators. Companies provide food, birds, and technical assistance, and farmers provide labor and installations, such as poultry sheds (Frost et al., 2003; Arikan et al., 2017). Thus, common poultry nuclei supply day-old broilers to large geographic regions, constituting the broiler production pyramid (Zurfluh et al., 2014).
Due to high productivity, there is heterogeneity in the size of the farms, the type of poultry sheds, and the number of birds housed, with an average of 14 birds/m2 (Frost et al., 2003; Arikan et al., 2017; De Oliveira Sidinei et al., 2021).
Therefore, this study aimed to conduct longitudinal monitoring of antimicrobial resistance in broiler chicken farms, emphasizing the frequency of resistance to fosfomycin and β-lactams.
Materials and Methods
This project was approved by the Ethics Committee on the Use of Animals of the State University of Londrina – CEUA/UEL, processing number 13142.2019.51.
Poultry Farm Characteristics
Samples (cloacal swabs, meconium, water, poultry feed, poultry litter, and Alphitobius diaperinus) were collected from four broiler farms in the northern region of Paraná – from March 2019 to July 2020, no farms were sampled simultaneously.
The all-in/all-out system was adopted in all farms evaluated, with an interval between batches of 7 to 12 days and a housing capacity from 10,000 to 30,000 chickens. Some sheds were technified (dark house), and others were conventional sheds. The poultry litter (composed of shavings) was reused for up to seven cycles, with fermentation conducted by canvas covering between them (De Oliveira Sidinei et al., 2021).
The animals evaluated came from three different hatcheries, but all were from the same agroindustry.
All farms adopted the use of organic acids in water within 20–25 days of life (dol), and on farms 1, 2, and 3, the supply of antimicrobials was reported, such as tiamulin (before the prohibition of its use) and enrofloxacin (EN; Brasil, 2020).
Regarding water, all the farms provided chlorinated water (1–3 ppm), and the feed was provided by the integrator company, respecting the stages of chicken rearing.
Poultry Farm Samples
Sample collections (cloacal swabs, meconium, water, poultry feed, poultry litter, and A. diaperinus) were collected at three different periods: the first period (1st dol or day-old), the second period (20th to 25th dol), and the third period (40th to 42nd dol).
The cloacal swabs were collected on the first day of life before housing the birds, and meconium was collected from the bottom of the transport boxes. Thirty cloacal swabs were collected per farm, placed in Cary Blair medium (Absorb), and sent under refrigeration for processing. Swabs (n = 30) were grouped into five pools, totaling 60 samples.
From the environment, samples were collected from poultry litter (n = 12) with sterile boot swabs (Brasil, 2016) using two pairs of props, each sampling 50% of the shed. Feed samples (2 kg/silo per moment, totaling 12 samples) and A. diaperinus (100 adult beetles per house, totaling 12 samples) were collected. Water samples were collected at the beginning (initial water) and end (final water) of the drinking fountain line for a total of 24 samples. In total, 124 samples of materials from different sources were processed.
Processing and Selection of the Isolates
The water samples were analyzed by a multiple-tube fermentation technique according to Eckner (1998) and (Brasil, 2013). After incubation in buffered peptone water, at 37°C for 18–24 h, the other samples were seeded on MacConkey agar without supplementation (MC) and supplemented with the antimicrobials, ciprofloxacin (CIP), cefotaxime (CTX), and ciprofloxacin + cefotaxime (CIP/CTX), at a final concentration of 8 μg/ml. The lactose-fermenting colonies were subjected to identification by biochemical screening using triple-sugar iron agar, indole production, Simmons citrate, urease production, lysine decarboxylation, and sorbitol and cellobiose fermentation tests (Merck, Darmstadt, Alemanha; Awogbemi et al., 2018; Moeinizadeh and Shaheli, 2021; Thomrongsuwannakij et al., 2021).
Up to three E. coli colonies were selected from each culture medium and stored at -20°C in brain heart infusion broth (Himedia Laboratories Pvt. Ltd., Mumbai, Índia) supplemented with 20% glycerol until processing. For the subsequent analyses, six bacterial isolates from each origin were used, per farm, per period.
Antimicrobial Resistance and Extended-Spectrum β-Lactamase Production
Antimicrobial sensitivity was determined using the disk diffusion method, following the Clinical and Laboratory Standards Institute (CLSI, 2018). Nineteen antimicrobials belonging to seven different classes were used, β-lactams: amoxicillin-clavulanic acid (AMC, 10/20 μg), ampicillin (AMP, 10 μg), cefazolin (CFZ, 30 μg), cefoxitin, (CFO, 30 μg), ceftiofur (CTF, 30 μg), ceftriaxone (CRO, 30 μg), ceftazidime (CAZ, 30 μg), CTX (30 μg), CRO (30 μg), aztreonam (ATM, 30 μg), and imipenem (IPM, 30 μg); quinolones: CIP (5 μg), EN (10 μg), and nalidixic acid (NAL, 30 μg); sulfonamides: sulfamethoxazol + trimethoprim (SXT, 1.25/23.75 μg); tetracyclines: tetracycline (TET, 30 μg); aminoglycosides: gentamicin (CN, 10 μg); amphenicols: chloramphenicol (C, 30 μg); and fosfomycin: fosfomycin/trometamol (FOT, 200 μg; Oxoid Ltd., Basingstoke, Hants, Reino Unido, United Kingdom). E. coli ATCC 25922 was used as a standard control for the antibiogram test. The results were interpreted according to CLSI (2019) and BRCAST (2019) except for CTF and EN, which followed CLSI (2008).
For ESBL detection, the double synergism technique was performed (Jarlier et al., 1988) with an AMC disk (10/20 μg), placed at 20 mm disks of ATM (30 μg), CAZ (30 μg), CTF (30 μg), and cefepime (FEP, 30 μg).
Antimicrobial Resistance Survey
Bacterial DNA was extracted using the Pure Link Genomic DNA Mini Kit (Invitrogen).
We searched for genes that conferred resistance to β-lactams (blaCTX–M–1, blaCTX–M–2, blaCTX–M–8, blaCTX–M–9, and blaCTX–M–25; Woodford et al., 2006), colistin resistance coding genes (mcr-1; Liu et al., 2016), and fosfomycin (fosA3; Sato et al., 2013).
All PCR amplicons were visualized on 1.5% agarose gels stained with GelRed (Biotium, Hayward, CA, United States). After gel electrophoresis, the images were captured using Image Capture Systems (LPixImageHE).
Analysis of the Genetic Similarity Profile by Enterobacterial Repetitive Intergenic Consensus-Polymerase Chain Reaction
The genetic similarity profile was evaluated by enterobacterial repetitive intergenic consensus-polymerase chain reaction (ERIC-PCR) analysis, in accordance with Versalovic et al. (1991). The PCR products were subjected to 2% agarose gel electrophoresis for 4 h in TBE buffer, stained with ethidium bromide (0.5 μg/mL), and visualized in a UV transilluminator (Vilbert Loumart). The similarity dendrogram was constructed using Gel J 2.0 software (Heras et al., 2015), using the unweighted pair group method with arithmetic mean (UPGMA) and the data similarity coefficient for cluster analysis (Jaccard), with a tolerance index of 1.0. The standard cutoff level to define the clusters was 85% (Daga et al., 2019).
Sequencing
Sequencing was performed from the PCR product amplified for ISEcp (Zurita et al., 2016). When negative for this insertion sequence, the amplified product for the gene using blaCTX–M–1 (Fei Tian et al., 2011; Cantón et al., 2012; Dierikx et al., 2013) was characterized for bidirectional Sanger sequencing on ABI-PRISM 3500 XL (Applied Biosystems) following the manufacturer’s recommendations. For the interpretation and alignment of the sequencing results, Chromas was used to evaluate the electropherogram, and ClustalW was used to achieve alignment of the sequences. Using BLAST, the sequences were compared with the NCBI database.
Conjugation Experiment
Conjugation was performed to assess the horizontal transfer capacity of the blaCTX–M–55 gene and fosA3 (Gonçalves et al., 2021). Two E. coli blaCTX–M–55 and fosA3 from Group B1 (negative for chuA and YjaA and positive for TspE4 and ArpA) were used as donors, and one E. coli from Group D (negative for TspE4 and YjaA and positive for chuA and ArpA) resistant to gentamicin was used as the recipient. All isolates were from day-old chickens (Clermont et al., 2013).
Statistical Evaluation
Statistical analysis was performed using R version 3.5.1. To assess the relationship between the studied variables, multivariate logistic regression analysis was used. The odds ratio (OD) calculation of the prediction model (α = 5%) was used to identify the set of information that best explained the relationship of risk factors associated with the occurrence of fosA3- and ESBL-producing E. coli (Menard, 2002).
Results
Escherichia coli Phenotypic and Genotypic Profile
A total of 507 E. coli were isolated: 360 from cloacal swabs, 24 from meconium, 3 from poultry feed, 18 from water, 69 from poultry litter, and 33 from A. diaperinus (Table 1).
Among the 507 E. coli isolated, fosfomycin (41% – 202/507) and AMP (68% – 347/507) are the antimicrobials that deserve the most attention due to their high percentage of resistance. Therefore, more than 80.1% (406/507) were MDR, and more than 51% (260/507) were ESBL producers. Regarding resistance to β-lactams, the blaCTX–M–1 group, was present in 40% (202/507) of the strains, the most frequent blaCTX–M group, followed by the blaCTX–M–2 group at 17% (86/507; Table 2). The strains that presented some gene from the blaCTX–M group had greater resistance to CAZ (98.9%, 268/271), CFZ (94.5%, 256/271), and AMP (92.3%, 250/271).
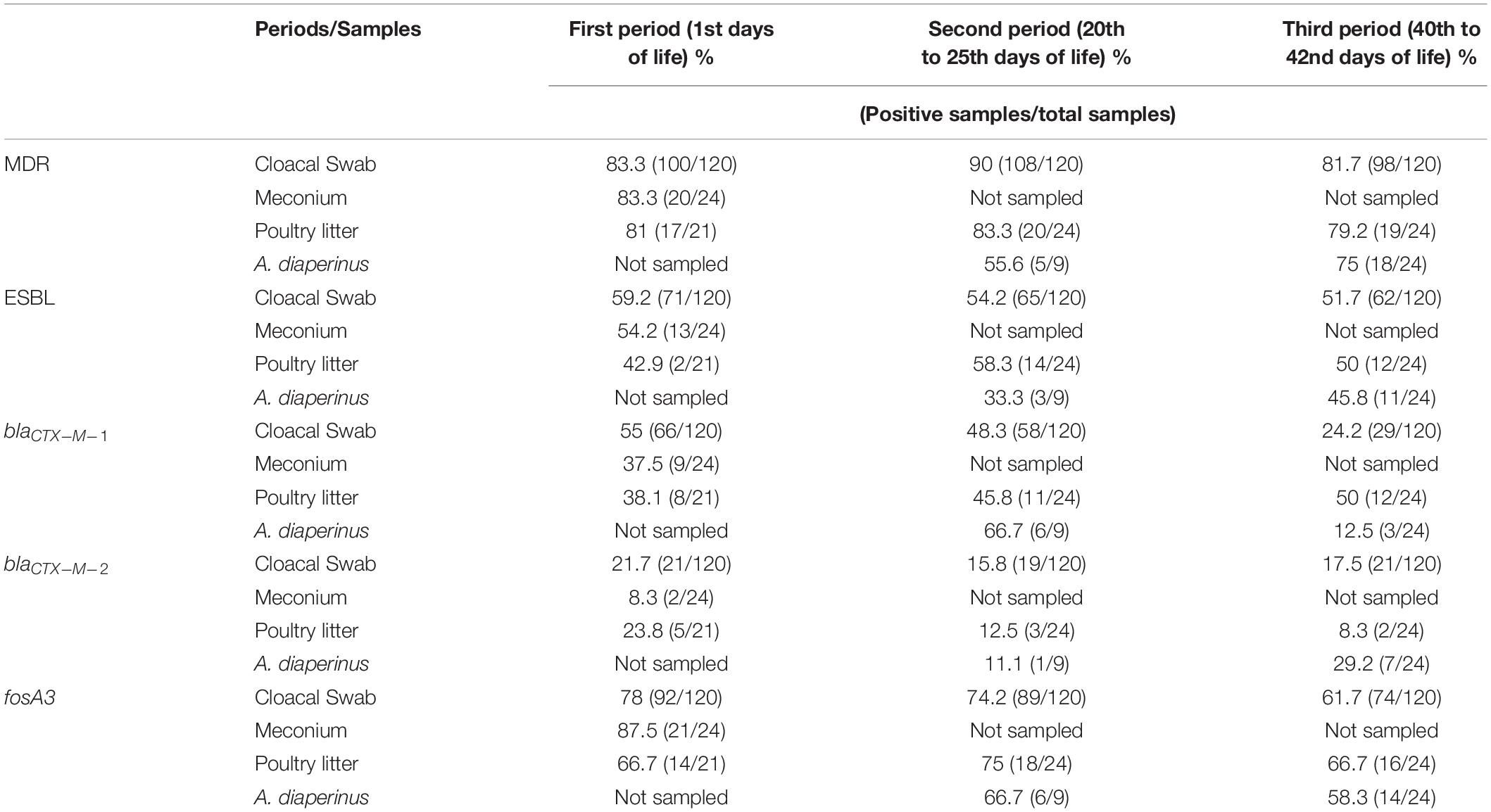
Table 2. Percentage (%) of isolates with MDR, ESBL, blaCTX–M–1 group, blaCTX–M–2 group, or fosA3 gene from the cloacal swab, meconium, poultry litter, and A. diaperinus samples, per period.
Comparing the resistance profiles of the strains, no significant difference was observed between the sampled periods regarding the presence of MDR and fosA3 and the occurrence of ESBL. For the simultaneous presence of the fosA3 and blaCTX–M genes, the strain that had fosA3 was 3.57 (CI 2.7–4.72) times more likely to also have a gene in the blaCTX–M–1 group.
Escherichia coli Isolated From Cloacal Swab and Meconium Samples
Twenty-four E. coli samples from meconium and 360 E. coli samples from cloacal swabs were isolated, totaling 384 E. coli from chickens (Table 1). On all farms surveyed and, in all periods, E. coli ESBL-production was detected.
In the first period, E. coli resistant to CTX (70% – 101/144) and fosfomycin (47.9% – 69/144) was isolated from meconium and cloacal swab samples, and the first period was 1.64 (CI 1.01–2.65) times more likely to harbor the fosA3 gene, than other periods. In the second period, the highest percentage of MDR was observed (90% – 108/120). In the third period, there was a decrease in strains resistant to some antimicrobials, such as fosfomycin (34% – 41/120), quinolones, and third and fourth generation cephalosporins, and it was the period with the lowest number of strains harboring the fosA3 gene (OR 0.49, CI 0.31–0.77), compared to the first and second periods (Figure 1).
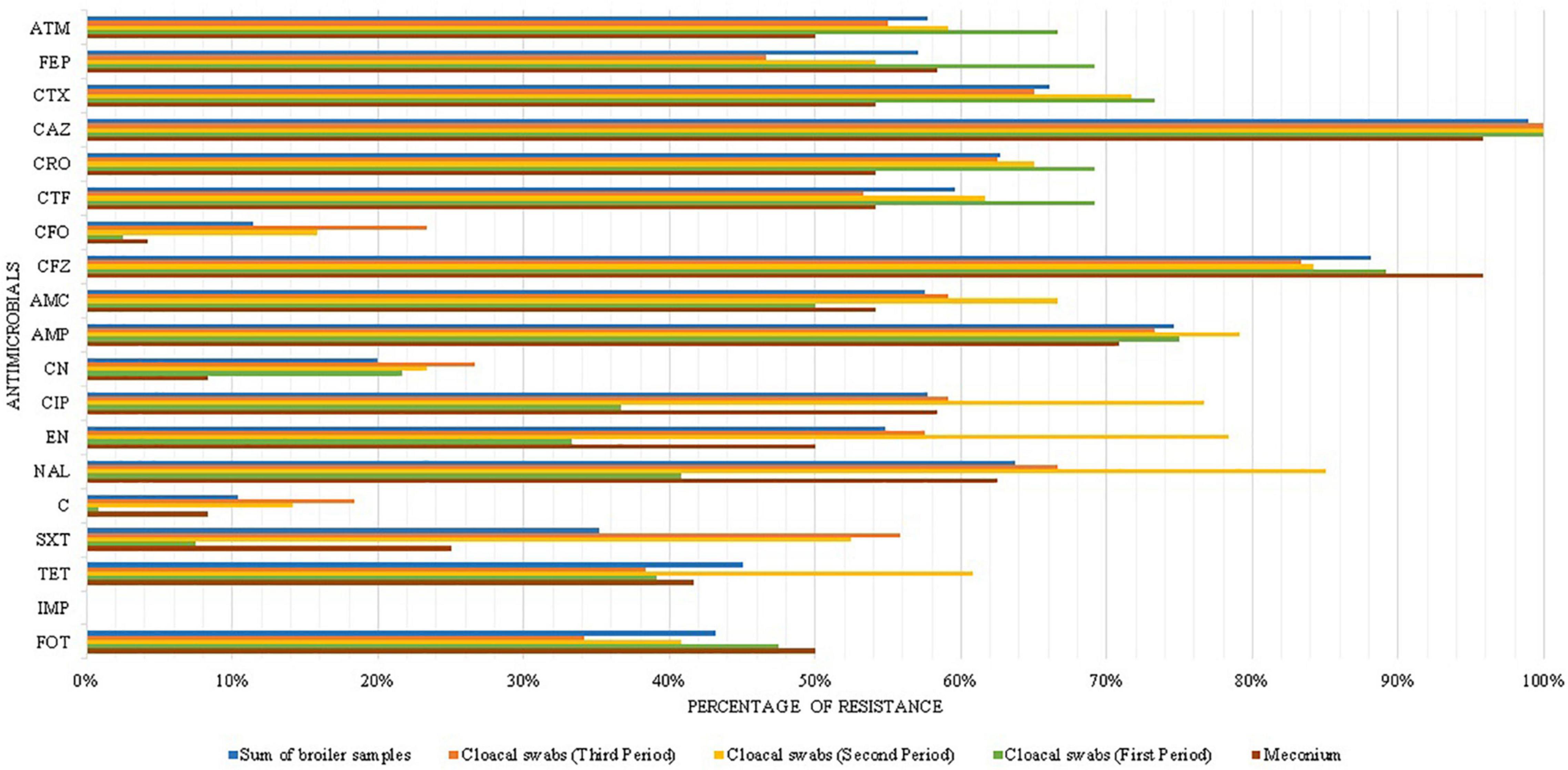
Figure 1. Phenotypic resistance profile of broiler samples by periods. FOT, Fosfomycin-trometamol; TET, tetracycline; SXT, trimethoprim-sulfamethoxazole; C, chloramphenicol; CN, gentamicin; CIP, ciprofloxacin; NAL, nalidixic acid; EN, enrofloxacin; AMC, amoxicillin-clavulanic acid; AMP, ampicillin; CFZ, cefazolin; CFO, cefoxitin; CTF, ceftiofur; CRO, ceftriaxone; CAZ, ceftazidime; CTX, cefotaxime; FEP, cefepime; ATM, aztreonam; and IMP, imipenem.
Strains isolated from chickens (meconium + cloacal swabs) were 1.62 (CI 1.19–2.2) times more likely to be ESBL producers and present the fosA3 gene than the other samples. Cloacal swab samples were 2.53 (CI 1.61–3.97) times more likely to be resistant to three or more classes of antimicrobials.
None of the strains isolated were positive for the mcr-1 gene or blaCTX–M–9 and blaCTX–M–25 groups. However, five strains isolated from cloacal swabs had the blaCTX–M–8 group, and they were from different periods and cloacal swab samples but belonged to the same farm.
Escherichia coli Isolated From Poultry Litter and A. diaperinus Samples
A total of 69 E. coli isolated from poultry litter were obtained (Table 1). In one of the farms, no strains isolated from poultry litter were resistant to CIP or CTX in the first period. In the second period, the frequency of the isolation of ESBL-producing E. coli was higher than that of the others, so the MDR profile prevailed in this evaluation period (83.3% – 20/24). Notably, a gradual increase in resistance to fosfomycin and CTX was found from the first period to the second (Figure 2). Strains isolated from poultry litter were more likely to be ESBL producers (OR 2.07, CI 1.31–3.27); additionally, they were more likely (OR 1.64, CI 1.08–2.48) to have the fosA3 and blaCTX–M–1 genes than other samples.
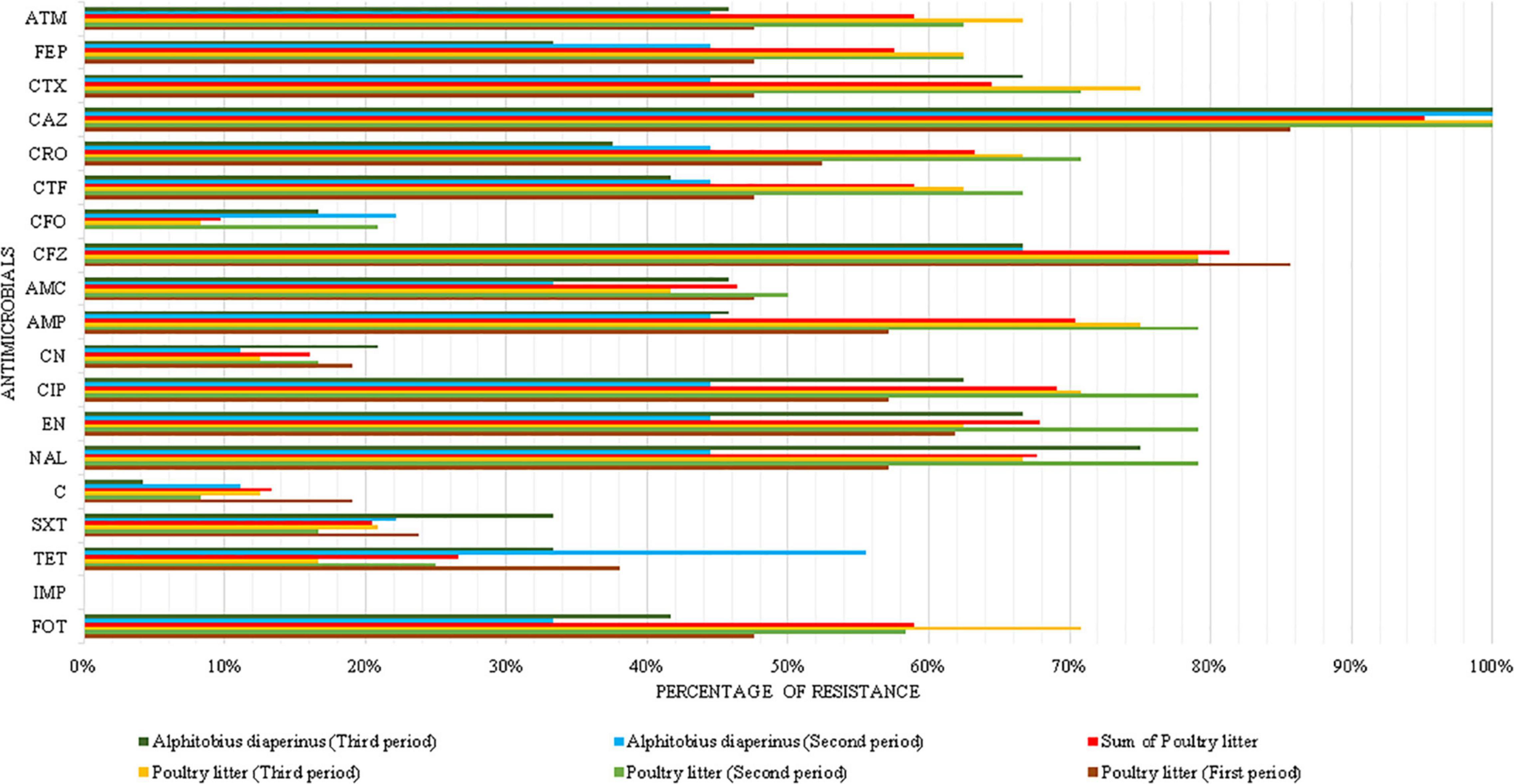
Figure 2. Phenotypic resistance profile of poultry litter and A. diaperinus by periods. FOT, Fosfomycin-trometamol; TET, tetracycline; SXT, trimethoprim-sulfamethoxazole; C, chloramphenicol; CN, gentamicin; CIP, ciprofloxacin; NAL, nalidixic acid; EN, enrofloxacin; AMC, amoxicillin-clavulanic acid; AMP, ampicillin; CFZ, cefazolin; CFO, cefoxitin; CTF, ceftiofur; CRO, ceftriaxone; CAZ, ceftazidime; CTX, cefotaxime; FEP, cefepime; ATM, aztreonam; and IMP, imipenem.
Regarding, only one strain was positive (5% – 1/21) to the mcr-1 gene, and it came from the first period; in this strain, the presence of the fosA3 and blaCTX–M–2 genes was also observed (Table 2).
A total of 33 E. coli strains isolated from A. diaperinus were collected in the second and third periods (Table 1). The highest percentage of MDR- and ESBL-producing isolates was observed in the third period (Table 2). A. diaperinus was not collected in the first period since these beetles were not present on the farms.
Escherichia coli Isolated From Poultry Feed and Water Samples
In the feed samples, E. coli were isolated on only one farm (farm 3 – Table 1), which showed 100% resistance to NAL, CIP, and CFZ, and were sensitive to some antimicrobials, such as fosfomycin. Poultry feed-isolated strains tended not to be MDR-, ESBL- or fosA3-positive (OR < 0.01).
Regarding the water samples, 18 strains were obtained, and a more significant recovery of E. coli occurred in the first period (Table 1).
None of the strains isolated from the water were MDR or ESBL positive, nor did they present any resistance genes studied. Strains isolated from start water and end water tended not to be MDR-, ESBL-, or fosA3-positive (OR < 0.01).
Enterobacterial Repetitive Intergenic Consensus Sequence (ERIC-PCR) and Sequencing
Forty-three bacterial isolates from poultry litter, cloacal swabs, and meconium, positive for the blaCTX–M–1 and fosA3 genes, were selected for ERIC-PCR analysis and blaCTX–M–1 sequencing. The sequenced isolates carried the enzyme blaCTX–M–55.
Regarding ERIC-PCR, ten clonal groups with more than 85% similarity were observed. Some of these groups were represented by E. coli isolated from cloacal swabs in the first periods but from different farms (clonal group III), E. coli isolated from the same farm but at different periods (clonal group I), and E. coli isolated from different farms, periods, and sources (clonal groups I, VI, VIII, and IX), as shown in Figure 3.
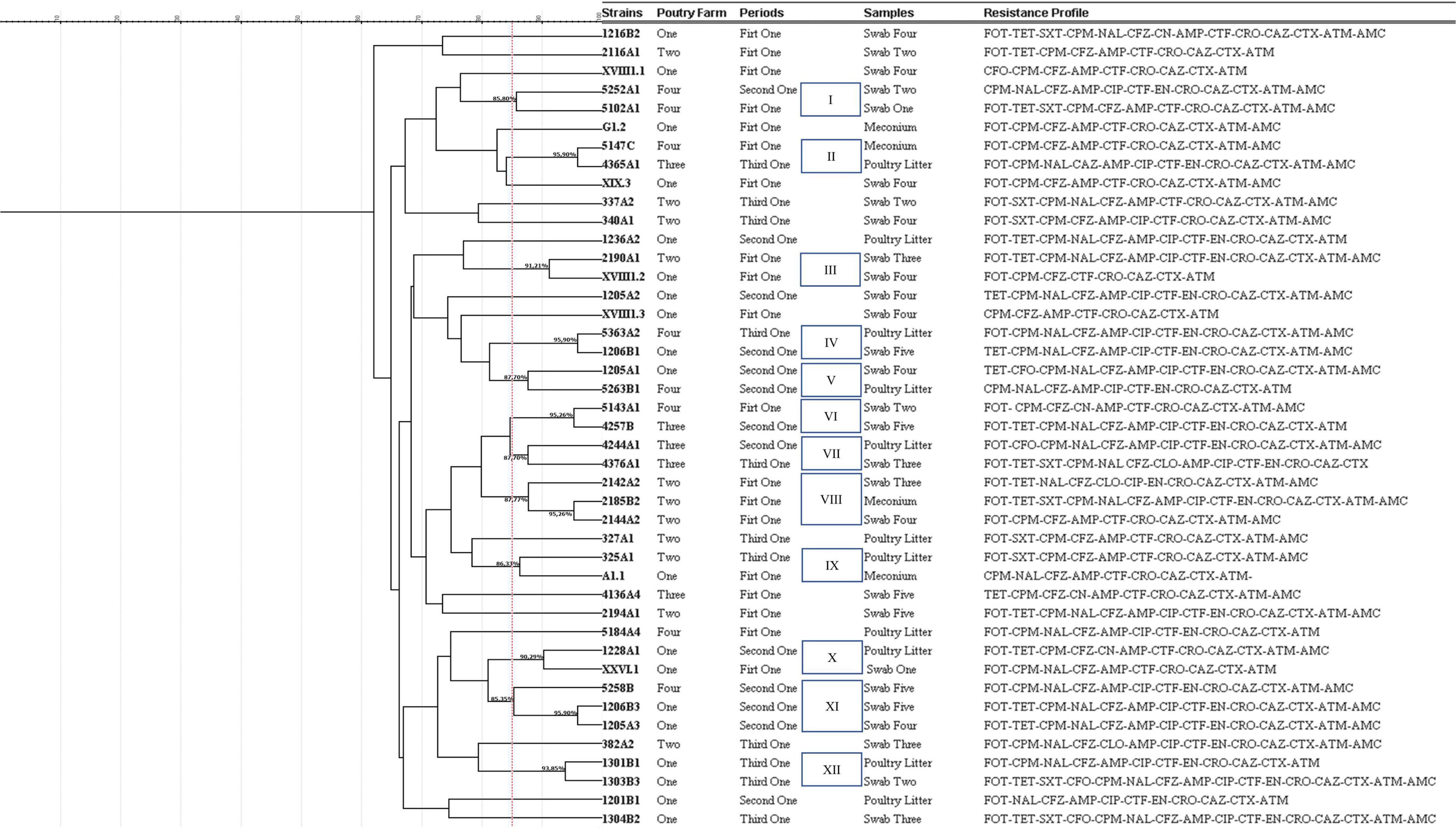
Figure 3. Dendrogram with the characteristics of E. coli harboring fosA3/CTX-M-55 by ERIC-PCR. FOT, Fosfomycin-trometamol; TET, tetracycline; SXT, trimethoprim-sulfamethoxazole; C, chloramphenicol; CN, gentamicin; CIP, ciprofloxacin; NAL, nalidixic acid; EN, enrofloxacin; AMC, amoxicillin-clavulanic acid; AMP, ampicillin; CFZ, cefazolin; CFO, cefoxitin; CTF, ceftiofur; CRO, ceftriaxone; CAZ, ceftazidime; CTX, cefotaxime; FEP, cefepime; ATM, aztreonam; and IMP, imipenem.
Conjugation Experiment Results
Two transconjugants were obtained in the conjugation assay; these isolates belonged to phylogenetic group D, confirming that they are the recipient strains and were positive for the blaCTX–M–55 and fosA3 groups, demonstrating the horizontal transfer capacity of these genes.
Discussion
Emerging resistance to antimicrobials is a concern and needs to be investigated. According to PanBr-Agro, broiler farms are important places for monitoring resistance to antimicrobials (Brasil, 2018) due to the sanitary challenges in the poultry production system and the consequent use of antimicrobials for different purposes. Thus, poultry production is one of the segments responsible for the selection of isolates resistant to antimicrobials (Cuong et al., 2019; Hedman et al., 2020; Zalewska et al., 2021), and the control of some antimicrobials can minimize zoonotic risks of multiresistant transmission bacteria from animals to humans (Magnusson, 2020). Our study carried out a longitudinal monitoring, researching multiresistant bacteria from different samples in three life periods of broilers, to investigate possible risk points in the spread of antimicrobial resistance.
Our results demonstrate that the main critical point for the spread of antimicrobial resistance in broiler chicken farms was the chickens, which arrived colonized with E. coli harboring the fosA3 and blaCTX–M–1 genes, especially the blaCTX–M–55. We detected 68% (344/507) of the isolates positive for the fosA3 gene. Our fosA3 positive strains were from cloacal swab, meconium, A. diaperinus, and poultry litter samples.
According to the WHO, fosfomycin is in the AWaRe classification group, and it acts against strains resistant to carbapenems (Martirosov and Lodise, 2016; Pauwels et al., 2021). Cottell and Webber (2017) analyzed 676 E. coli isolated from urine in a hospital in the United Kingdom, and 12% were resistant to fosfomycin. The authors demonstrated that E. coli isolated from infections in humans have a low resistance to fosfomycin and highlighted the importance of keeping this antimicrobial for human use only. Derington et al. (2020) tested fosfomycin as a therapeutic option for treating complicated urinary tract infections, leading to the resolution of the evaluated conditions. Based on these results, they, and other authors report that fosfomycin is an essential human therapeutic option (Wachino et al., 2010; Neuner et al., 2012; Jean et al., 2016; Cao et al., 2017; Abbott et al., 2020; Loras et al., 2020; Seok et al., 2020), against urinary infections for example, the second most important bacterial infection affecting humans (Moeinizadeh and Shaheli, 2021).
It is an antimicrobial that should only be used against multiresistant microorganisms (WHO, 2019); therefore, its use should be restricted to human medicine. Notably, Brazil does not have a ban on using this antimicrobial in animal production, being a country that uses fosfomycin in poultry production (Roth et al., 2019). The use of antimicrobials leads to selective pressure and consequently the selection of resistant strains (WHO, 2018, 2019); thus, the monitoring and surveillance of antimicrobials in animal production should promote the rational use of the same.
We report that both meconium and cloacal swab samples from day-old chickens (first period) had a 1.64 (CI 1.01–2.65) times greater chance of harboring the fosA3 gene than those from other periods. Vertical transmission can occur at any point in poultry production before the chickens reach the farms. In a longitudinal study, Poulsen et al. (2017) demonstrated that broiler breeders could transmit microorganisms to broilers. Although vertical transmission of E. coli to day-old chickens has already been shown by other authors (Lu et al., 2003; Dierikx et al., 2013; Fischer et al., 2014; Zurfluh et al., 2014; Osman et al., 2018; Apostolakos et al., 2019, 2020; Dame-Korevaar et al., 2019, Dame-Korevaar et al., 2020; Zhao et al., 2019), this is the first report of the presence of fosfomycin-resistant E. coli in day-old chickens.
Enterobacterial repetitive intergenic consensus-polymerase chain reaction detected clonal groups formed by strains from different farms isolated from day-old chickens (first period) and groups with E. coli isolated from the same farm but at different periods (clonal group I), suggesting possible vertical transmission and the perpetuation of fosA3/CTX-M-55 samples, respectively. This finding serves as a warning about the potential spread of resistance within the broiler production chain.
We also showed that strains isolated from poultry litter were more likely to be ESBL producers (OR 2.07, CI 1.31–3.27) than other samples, an important point in disseminating and perpetuating ESBL strains in poultry farming. Curiously in our research, poultry litter did not have the high number of resistance when compared with day-old chicken sample. The use of the same poultry litter in subsequent production cycles, when subjected to a sanitary vacuum and a good fermentation process, may result in a low microbial load (Wei et al., 2013; Vieira et al., 2015; Waziri and Kaltungo, 2017; Gurmessa et al., 2021; Moffo et al., 2021; Yévenes et al., 2021). In Brazil, poultry litter is reused for more than one consecutive batch, and this practice is allowed if sanitary problems have not occurred with the batches housed on the poultry litter to be reused (Waziri and Kaltungo, 2017).
Gazal et al. (2021) sampled broiler farms and found that the main sources of ESBL dissemination were poultry litter and Alphitobius sp. samples, which were detected from day one in farms sampled. Our study did not isolate MDR and ESBL-producing E. coli from all farms in the first period from poultry litter. One of the broiler farms analyzed reported having performed efficient poultry litter management, demonstrated by the low resistance profile of the strains isolated. In this and in other poultry farms it was also possible to observe a reduction or elimination of A. diaperinus in the first periods, possibly due to the poultry litter treatment process carried out between batches. Siller et al. (2020) reported that even when poultry litter undergoes a fermentation process, other events can interfere with the number of microorganisms present, regardless of how many times the litter has already been used. Therefore, it is essential to point out that poultry litter is indeed a sample that presents a potential risk factor inside poultry houses.
In our study, when we subjected E. coli strains harboring fosA3 and blaCTX–M–55 to conjugation experiments with a strain sensitive to fosfomycin and CTX, we observed that they were able to transmit both resistance genes. With the spread of these resistant strains, an increase in therapeutic failures in treating ESBL-producing microorganisms is expected. The use of fosfomycin risks the selection of ESBL coproducers since CTX-M, and fosA3 genes have already been confirmed to colocalize on plasmids (Sarker et al., 2019). Another important point to highlight about conjugation is that the recipient strain belongs to phylogenetic group D, and the donor strain belongs to phylogenetic Group B1, which demonstrates an exchange of genetic material between commensal microorganisms and microorganisms with potential pathogenicity (Clermont et al., 2013).
Regarding the E. coli isolated from water and poultry feed, these strains were not identified as MDR microorganisms or ESBL producers. This finding characterizes the samples as not critical points for the spread of antimicrobial resistance in broiler production (Miles et al., 2006; Rossato et al., 2019; Gazal et al., 2021).
In the present work, 4.33% (25/507) of the strains isolated had more than one gene from the blaCTX–M group, which should be highlighted regardless of the low percentage due to the potential for the development of other enzyme recombinants by these strains in the future (Yin et al., 2020; Leão et al., 2021).
Colistin is an antimicrobial indicated as a last resort to treat infections in humans. The first report of the mcr-1 gene, one of the genes that confers resistance to this antimicrobial, was in China by Liu et al. (2016), and mcr-1 has been correlated with the emergence of pandrug-resistant microorganisms (McGann et al., 2016). The mcr-1 gene is more frequently detected in farm animals than in humans (Elbediwi et al., 2019) but can cause serious infectious diseases such as pyelonephritis in humans (Birgy et al., 2018). Ahmed et al. (2020) found in Bangladesh that 25% (300/1200) of E. coli strains isolated from broiler chicken farms were positive for the mcr-1 gene. Out of 507 E. coli isolates, we detected only one positive mcr-1 gene in our work. This is possibly due to the local prohibition of using this antimicrobial as a growth promoter. This strain is a fourth-generation cephalosporin-resistant, producer of ESBL (blaCTX–M–2 group) and harbors the fosA3 gene.
We used culture media supplemented with antimicrobials to select isolates in the present work. This technique can favor the selection of strains with a higher resistance profile and more significant gene variability (Ceccarelli et al., 2019). We used preselection, CTX, an indicator of the CTX-M group (Paterson and Bonomo, 2005), and CIP antimicrobial with coselection to the ESBL enzyme (Jiang et al., 2008; Hassan et al., 2012; Seo and Lee, 2019). In this way, we were able to detect strains with a high resistance profile, avoiding underreporting of the antimicrobial-resistant profile.
Hence, in this work, a high frequency of E. coli harboring the fosA3/CTX-M-55 strains was detected in poultry farming, from day-old chickens to preslaughter chickens, and in different samples (cloacal swab + meconium, chicken litter, and beetles), with these genes transferable by conjugation. Therefore, considering the importance of fosfomycin to human medicine and the fact that Brazil is one of the largest exporters of chicken meat in the world (ABPA, 2021) and one of the few countries that use this antimicrobial in poultry production, we reinforce the need to ban its use in the poultry sector.
Conclusion
In conclusion, this study demonstrated that the spread of fosA3-mediated fosfomycin resistance was correlated with the presence of the CTX-M-1 group, especially the blaCTX–M–55 gene, in broiler chickens. This profile was observed mainly in day-old chickens, with a high profile of E. coli strains multidrug resistant to antimicrobials. The findings emphasize the importance of conducting longitudinal monitoring to detect the main risk points in poultry production and thus intervene, prevent drug resistance, and promote the rational use of antimicrobials.
Data Availability Statement
The datasets presented in this study can be found in online repositories. The names of the repository/repositories and accession number(s) can be found below: NCBI GenBank – OM326827–OM326869.
Ethics Statement
The animal study was reviewed and approved by Animal Ethics Committee of State University of Londrina (CEUA/UEL). Processing number: 13142.2019.51.
Author Contributions
MM-C contributed to developing experimental research, data analysis, and writing the manuscript. AB, LG, LJ, MdS, MS, BQ, VC, JB, GA, and BG contributed to the development of experimental research. EN contributed to the statistical analysis. RK, GN, AB, EV, LG, and GA contributed to and assisted in the design of the work and preparation of the article and critically reviewed the manuscript. All authors have participated in this study and commented on the manuscript.
Funding
Postgraduate Program supported this study in Microbiology of Universidade Estadual de Londrina and in part by the National Council for Scientific and Technological Development – CNPq (433656/2018-2 Chamada MCTIC/CNPqNo 28/2018 and 313305/2019-6 to RK) and (315435/2018-6 to GN). Maísa Fabiana Menck Costa was funded by a Ph.D. scholarship from Coordination for the Improvement of Higher Education Personnel (CAPES) – Finance Code 001. Additional support was provided by the Bill and Melinda Gates Foundation’s Grand Challenges Explorations Brazil – New Approaches to Characterize the Global Burden of Antimicrobial Resistance (number OPP1193112).
Conflict of Interest
The authors declare that the research was conducted in the absence of any commercial or financial relationships that could be construed as a potential conflict of interest.
Publisher’s Note
All claims expressed in this article are solely those of the authors and do not necessarily represent those of their affiliated organizations, or those of the publisher, the editors and the reviewers. Any product that may be evaluated in this article, or claim that may be made by its manufacturer, is not guaranteed or endorsed by the publisher.
Acknowledgments
We would also like to thank Bill and Melinda Gates Foundation’s (OPP1193112) for the great help in publishing the newspaper.
Supplementary Material
The Supplementary Material for this article can be found online at: https://www.frontiersin.org/articles/10.3389/fmicb.2022.846116/full#supplementary-material
References
Aarestrup, F. M., Bonten, M., and Koopmans, M. (2021). Pandemics–One Health preparedness for the next. Lancet. Reg. Health. Eur. 9:100210. doi: 10.1016/j.lanepe.2021.100210
Abbott, I. J., van Gorp, E., Wijma, R. A., Meletiadis, J., Mouton, J. W., and Peleg, A. Y. (2020). Evaluation of pooled human urine and synthetic alternatives in a dynamic bladder infection in vitro model simulating oral fosfomycin therapy. J. Microbiol. Methods 171:105861. doi: 10.1016/j.mimet.2020.105861
Abelenda-Alonso, G., Padullés, A., Rombauts, A., Gudiol, C., Pujol, M., Alvarez-Pouso, C., et al. (2020). Antibiotic prescription during the COVID-19 pandemic: a biphasic pattern. Infect. Control Hosp. Epidemiol. 41, 1371–1372. doi: 10.1017/ice.2020.381
Ahmed, S., Das, T., Islam, M. Z., Herrero-Fresno, A., Biswas, P. K., and Olsen, J. E. (2020). High prevalence of mcr-1-encoded colistin resistance in commensal Escherichia coli from broiler chicken in Bangladesh. Scientific Rep. 10:18637. doi: 10.1038/s41598-020-75608-2
Apostolakos, I., Feudi, C., Eichhorn, I., Palmieri, N., Fasolato, L., Schwarz, S., et al. (2020). High-resolution characterisation of ESBL/pAmpC-producing Escherichia coli isolated from the broiler production pyramid. Sci. Rep. 10:11123. doi: 10.1038/s41598-020-68036-9
Apostolakos, I., Mughini-Gras, L., Fasolato, L., and Piccirillo, A. (2019). Assessing the occurrence and transfer dynamics of ESBL/pAmpC-producing Escherichia coli across the broiler production pyramid. PLoS One 14:e0217174. doi: 10.1371/journal.pone.0217174
Aquino, C. (2021). Poultry and Products Annual Brazil. Available online at: https://apps.fas.usda.gov/newgainapi/api/Report/DownloadReportByFileName?fileName=Poultry%20and%20Products%20Annual_Brasilia_Brazil_09-01-2021.pdf (accessed March 17, 2022).
Arikan, M. S., Akin, A. C., Akcay, A., Aral, Y., Sariozkan, S., Cevrimli, M. B., et al. (2017). Effects of transportation distance, slaughter age, and seasonal factors on total losses in broiler chickens. Brazilian. J. Poult. Sci. 19, 421–428. doi: 10.1590/1806-9061-2016-0429
Aslam, B., Khurshid, M., Arshad, M. I., Muzammil, S., Rasool, M., Yasmeen, N., et al. (2021). Antibiotic resistance: one health one world outlook. Front. Cell. Infect. Microbiol. 11:1153. doi: 10.3389/fcimb.2021.771510
Aslam, B., Wang, W., Arshad, M. I., Khurshid, M., Muzammil, S., Rasool, M. H., et al. (2018). Antibiotic resistance: a rundown of a global crisis. Infect. Drug Res. 11, 1645–1658. doi: 10.2147/IDR.S173867
Avershina, E., Shapovalova, V., and Shipulin, G. (2021a). Fighting antibiotic resistance in hospital-acquired infections: current state and emerging technologies in disease prevention, diagnostics and therapy. Front. Microbiol. 12:2044. doi: 10.3389/fmicb.2021.707330
Avershina, E., Sharma, P., Taxt, A. M., Singh, H., Frye, S. A., Paul, K., et al. (2021b). AMR-Diag: neural network based genotype-to-phenotype prediction of resistance towards β-lactams in Escherichia coli and Klebsiella pneumoniae. Comput. Struct. Biotechnol. J. 19, 1896–1906. doi: 10.1016/j.csbj.2021.03.027
Awogbemi, J., Moses, A., and Akinkunmi, E. O. (2018). A Survey of antimicrobial agents usage in poultry farms and antibiotic resistance in Escherichia coli and Staphylococci Isolates from the poultry in ile-ife, nigeria. J. Infect.Diseases Epidemiol. 4, 1–8. doi: 10.23937/2474-3658/1510047
Birgy, A., Madhi, F., Hogan, J., Doit, C., Gaschignard, J., Caseris, M., et al. (2018). CTX-M- 55-, MCR- 1-, and FosA-Producing multidrug-resistant Escherichia coli infection in a child in France. Antimicrob. Agents Chemother. 62(4), e00127–e118. doi: 10.1128/AAC.00127-18
Brasil (2013). Fundação Nacional de Saúde. Manual prático de análise de água / Fundação Nacional de Saúde – 4. ed. – Brasília: Funasa.Bengaluru
Brasil (2018). Ministério da Saúde. Secretaria de Vigilância em Saúde. Departamento de Vigilância das Doenças Transmissíveis. Plano de ação nacional de prevenção e controle da resistência aos antimicrobianos no âmbito da saúde única 2018-2022 (PAN-BR) / Ministério da Saúde, Secretaria de Vigilância em Saúde, Departamento de Vigilância das Doenças Transmissíveis. – Brasília: Ministério da Saúde.
Brasil (2020). Instrução Normativa no 1, de 13 de janeiro de 2020—DOU – Imprensa Nacional. South America:AG, Brasil
Büdel, T., Kuenzli, E., Campos-Madueno, E. I., Mohammed, A. H., Hassan, N. K., Zinsstag, J., et al. (2020). On the island of zanzibar people in the community are frequently colonized with the same MDR Enterobacterales found in poultry and retailed chicken meat. J. Antimicrob. Chemother. 75(9), 2432–2441. doi: 10.1093/jac/dkaa198
Campos, A. C., da, C., Andrade, N. L., Couto, N., Mutters, N. T., de Vos, M., Rosa, A. C., de, P., et al. (2020). Characterization of fosfomycin heteroresistance among multidrug-resistant Escherichia coli isolates from hospitalized patients in Rio de Janeiro. Brazil. J. Glob. Antimicrob. Resist. 22, 584–593. doi: 10.1016/j.jgar.2020.04.026
Cantón, R., Gonzalez-Alba, J. M., and Galán, J. C. (2012). CTX-M Enzymes: Origin and Diffusion. Front. Microbiol. 3:110. doi: 10.3389/fmicb.2012.00110
Cao, X.L., Shen, H., Xu, Y.Y., Xu, X.J., Zhang, Z.F., Cheng, L., et al. (2017). High prevalence of fosfomycin resistance gene fosA3 in blaCTX-M-harbouring Escherichia coli from urine in a Chinese tertiary hospital during 2010–2014. Epidemiol. Infect. 145(4), 818–824. doi: 10.1017/S0950268816002879
Cassini, A., Högberg, L. D., Plachouras, D., Quattrocchi, A., Hoxha, A., Simonsen, G. S., Colomb-Cotinat, M., et al. (2019). Attributable deaths and disability-adjusted life-years caused by infections with antibiotic-resistant bacteria in the EU and the European Economic area in 2015: a population-level modelling analysis. Lancet Infect. Dis. 19(1), 56–66. doi: 10.1016/S1473-3099(18)30605-4
Cazares, A., Moore, M. P., Hall, J. P. J., Wright, L. L., Grimes, M., Emond-Rhéault, J.-G., et al. (2020). A megaplasmid family driving dissemination of multidrug resistance in Pseudomonas. Nature Commun. 11(1):1370. doi: 10.1038/s41467-020-15081-7
Ceccarelli, D., Kant, A., van Essen-Zandbergen, A., Dierikx, C., Hordijk, J., Wit, B., et al. (2019). Diversity of plasmids and genes encoding resistance to extended spectrum cephalosporins in commensal Escherichia coli from dutch livestock in 2007–2017. Front. Microbiol. 10:76. doi: 10.3389/fmicb.2019.00076
Clermont, O., Christenson, J. K., Denamur, E., and Gordon, D. M. (2013). The Clermont Escherichia coli phylo-typing method revisited: improvement of specificity and detection of new phylo-groups. Env.Microbiol.Rep. 5(1), 58–65. doi: 10.1111/1758-2229.12019
CLSI (2008). Performance Standards for Antimicrobial Disk and Dilution Susceptibility Tests for Bacteria Isolated From Animals. 5th ed. CLSI standard VET01 (ISBN 978-1-68440-008-9 [Print]; ISBN 978-1-68440- 009-6 [Electronic]). Clinical and Laboratory Standards Institute, Pennsylvania US. 2018.
CLSI (2018). Performance Standards for Antimicrobial Susceptibility Testing. Twenty-Fifth Informational Supplement CLSI Document M100. Wayne, PA: Clinical and Laboratory Standards Institute.
CLSI (2019). Suggested Grouping of US-FDA Approved Antimicrobial Agents That Should Be Considered for Routine Testing and Reporting on Nonfastidious Organisms by Clinical Laboratories. 29ed. CLSI guideline M100-S29. Wayne, PA: Clinical and Laboratory Institute.
Cottell, J. L., and Webber, M. A. (2017). Prevalence, mechanisms and comparison of detection methods of fosfomycin resistance in E. coli from urinary tract infections. BioRxiv 234–435. doi: 10.1101/234435
Cunha, M. P. V., Lincopan, N., Cerdeira, L., Esposito, F., Dropa, M., Franco, L. S., et al. (2017). Coexistence of CTX-M-2, CTX-M-55, CMY-2, FosA3, and QnrB19 in Extraintestinal Pathogenic Escherichia coli from Poultry in Brazil. Antimicrob. Agents Chemother. 61(4), e02474–e2416. doi: 10.1128/AAC.02474-16
Cuong, N. V., Phu, D. H., Van, N. T. B., Dinh Truong, B., Kiet, B. T., Hien, B. V., et al. (2019). High-Resolution monitoring of antimicrobial consumption in vietnamese small-scale chicken farms highlights discrepancies between study metrics. Front. Vet. Sci. 6:174. doi: 10.3389/fvets.2019.00174
Cyoia, P. S., Koga, V. L., Nishio, E. K., Houle, S., Dozois, C. M., de Brito, K. C. T., et al. (2019). Distribution of ExPEC Virulence Factors, blaCTX-M, fosA3, and mcr-1 in Escherichia coli isolated from commercialized chicken carcasses. Front. Microbiol. 9:3254. doi: 10.3389/fmicb.2018.03254
Daga, A. P., Koga, V. L., Soncini, J. G. M., de Matos, C. M., Perugini, M. R. E., Pelisson, M., et al. (2019). Escherichia coli bloodstream infections in patients at a university hospital: virulence factors and clinical characteristics. Front. Cell. Infect. Microbiol. 9:191. doi: 10.3389/fcimb.2019.00191
Dame-Korevaar, A., Fischer, E. A. J., van der Goot, J., Stegeman, A., and Mevius, D. (2019). Transmission routes of ESBL/pAmpC producing bacteria in the broiler production pyramid, a literature review. Prev. Vet. Med. 162, 136–150. doi: 10.1016/j.prevetmed.2018.12.002
Dame-Korevaar, A., Kers, J. G., van der Goot, J., Velkers, F. C., Ceccarelli, D., Mevius, D. J., et al. (2020). Competitive exclusion prevents colonization and compartmentalization reduces Transmission of ESBL-Producing Escherichia coli in Broilers. Front. Microbiol. 11:2853. doi: 10.3389/fmicb.2020.566619
Davis, G. S., Waits, K., Nordstrom, L., Grande, H., Weaver, B., Papp, K., et al. (2018). Antibiotic-resistant Escherichia coli from retail poultry meat with different antibiotic use claims. BMC Microbiol. 18(1):174. doi: 10.1186/s12866-018-1322-5
De Oliveira Sidinei, M. E. A., Marcato, S. M., Perez, H. L., and Bánkuti, F. I. (2021). Biosecurity, environmental sustainability, and typological characteristics of broiler farms in Paraná State, Brazil. Prev. Vet. Med. 194:105426. doi: 10.1016/j.prevetmed.2021.105426
De Oliveira, D. M. P., Forde, B. M., Kidd, T. J., Harris, P. N. A., Schembri, M. A., Beatson, S. A., et al. (2020). Antimicrobial Resistance in ESKAPE pathogens. Clin. Microbiol. Rev. 33(3), e00181–e119. doi: 10.1128/CMR.00181-19
Derington, C. G., Benavides, N., Delate, T., and Fish, D. N. (2020). Multiple-dose oral fosfomycin for treatment of complicated urinary tract infections in the outpatient setting. Open For. Infect. Dis. 7:ofaa034. doi: 10.1093/ofid/ofaa034
Dierikx, C. M., Goot, J. A., van der Smith, H. E., Kant, A., and Mevius, D. J. (2013). Presence of ESBL/AmpC -Producing Escherichia coli in the broiler production pyramid: a descriptive study. PLoS One, 8(11):e79005. doi: 10.1371/journal.pone.0079005
Eckner, K. F. (1998). Comparison of membrane filtration and multiple-tube fermentation by the colilert and enterolert methods for detection of waterborne coliform bacteria, Escherichia coli, and enterococci used in drinking and bathing water quality monitoring in southern sweden. Appl. Env. Microbiol. 64(8), 3079–3083. doi: 10.1128/AEM.64.8.3079-3083.1998
Elbediwi, M., Li, Y., Paudyal, N., Pan, H., Li, X., Xie, S., et al. (2019). Global burden of colistin-resistant bacteria: mobilized colistin resistance genes study (1980–2018). Microorganisms 7:461 doi: 10.3390/microorganisms7100461
European Commission (2018). A European One Health Action Plan Against Antimicrobial Resistance (AMR); 2017/C 212/01. Available online at https://ec.europa.eu/health/antimicrobial-resistance/eu-action-on-antimicrobial-resistance_en [accessed November 11, 2021]
Fei Tian, S., Zhuo Chu, Y., yi Chen, B., Nian, H., and Shang, H. (2011). ISEcp1 element in association with blaCTX-M genes of E. coli that produce extended-spectrum β-lactamase among the elderly in community settings. Enferm. Infecc. Microbiol. Clín. 29, 731–734. doi: 10.1016/j.eimc.2011.07.011
Fischer, J., Rodríguez, I., Baumann, B., Guiral, E., Beutin, L., Schroeter, A., et al. (2014). Blactx-M-15-carrying Escherichia coli and Salmonella isolates from livestock and food in Germany. J. Antimicrob. Chemother. 69(11), 2951–2958. doi: 10.1093/jac/dku270
Frost, A. R., Parsons, D. J., Stacey, K. F., Robertson, A. P., Welch, S. K., Filmer, D., Fothergill, A., et al. (2003). Progress towards the development of an integrated management system for broiler chicken production. Comput. Electron. Agric. 39(3), 227–240. doi: 10.1016/S0168-1699(03)00082-6
Gazal, L. E., de, S., Medeiros, L. P., Dibo, M., Nishio, E. K., Koga, V. L., Gonçalves, B. C., et al. (2021). Detection of ESBL/AmpC-Producing and Fosfomycin-Resistant Escherichia coli from different sources in poultry production in southern brazil. Front. Microbiol. 11:604544. doi: 10.3389/fmicb.2020.604544
Gonçalves, B. C., Dibo, M., Gazal, L. E., de, S., Medeiros, L. P., Costa, M. F. M., Kobayashi, R. K. T., et al. (2021). “Obtaining transconjugant Escherichia coli costs isolated from chicken litter and chicks” “obtenção de cepas transconjugantes de Escherichia coli isoladas de cama de frango e pintainhos”. Braz. J. Dev. 7(2), 18518–18525. doi: 10.34117/bjdv7n2-474
Gurmessa, B., Ashworth, A. J., Yang, Y., Savin, M., Moore, P. A., Ricke, S. C., et al. (2021). Variations in bacterial community structure and antimicrobial resistance gene abundance in cattle manure and poultry litter. Env. Res. 197:111011. doi: 10.1016/j.envres.2021.111011
Hassan, W., Hashim, A., and Domany, R. (2012). Plasmid mediated quinolone resistance determinants qnr, aac(6’)-Ib-cr, and qep in ESBL-producing Escherichia coli clinical isolates from Egypt. Indian J. Med. Microbiol. 30(4), 442–447. doi: 10.4103/0255-0857.103766
Hedman, H. D., Vasco, K. A., and Zhang, L. (2020). A Review of antimicrobial resistance in poultry farming within low-resource settings. Animals (Basel) 10:1264. doi: 10.3390/ani10081264
Heras, J., Domínguez, C., Mata, E., Pascual, V., Lozano, C., Torres, C., et al. (2015). GelJ – a tool for analyzing DNA fingerprint gel images. BMC Bioinformatics, 16(1), 270. doi: 10.1186/s12859-015-0703-0
Huttner, A., Kowalczyk, A., Turjeman, A., Babich, T., Brossier, C., Eliakim-Raz, N., et al. (2018). Effect of 5-Day Nitrofurantoin vs single-dose fosfomycin on clinical resolution of uncomplicated lower urinary tract infection in women: a randomized clinical trial. JAMA 319(17), 1781–1789. doi: 10.1001/jama.2018.3627
Huttner, B. D., Catho, G., Pano-Pardo, J. R., Pulcini, C., and Schouten, J. (2020). COVID-19: Don’t neglect antimicrobial stewardship principles!. Clin. Microbiol. Infect. 26(7), 808–810. doi: 10.1016/j.cmi.2020.04.024
Jarlier, V., Nicolas, M.-H., Fournier, G., and Philippon, A. (1988). Extended broad-spectrum β-lactamases conferring transferable resistance to newer β-lactam agents in Enterobacteriaceae: hospital prevalence and susceptibility patterns. Rev. Infect. Dis. 10(4), 867–878. doi: 10.1093/clinids/10.4.867
Jean, S.-S., Coombs, G., Ling, T., Balaji, V., Rodrigues, C., Mikamo, H., Kim, M.-J., et al. (2016). Epidemiology and antimicrobial susceptibility profiles of pathogens causing urinary tract infections in the Asia-Pacific region: results from the study for monitoring antimicrobial resistance trends (SMART), 2010–2013. Int. J.Antimicrob. Agents. 47(4), 328–334. doi: 10.1016/j.ijantimicag.2016.01.008
Jiang, Y., Zhou, Z., Qian, Y., Wei, Z., Yu, Y., Hu, S., Li, L., et al. (2008). Plasmid-mediated quinolone resistance determinants qnr and aac(6’)-Ib-cr in extended-spectrum β-lactamase-producing Escherichia coli and Klebsiella pneumoniae in China. J. Antimicrob. Chemother. 61(5), 1003–1006. doi: 10.1093/jac/dkn063
Koga, V. L., Rodrigues, G. R., Scandorieiro, S., Vespero, E. C., Oba, A., et al. (2015). Evaluation of the Antibiotic Resistance and Virulence of Escherichia coli Strains Isolated from Chicken Carcasses in 2007 and 2013 from Paraná. Brazil Foodborne Pathogens Dis. 12(6), 479–485. doi: 10.1089/fpd.2014.1888
Kwan, A. C. F., and Beahm, N. P. (2020). Fosfomycin for bacterial prostatitis: a review. Int. J.Antimicrob. Agents 56(4):106106. doi: 10.1016/j.ijantimicag.2020.106106
Laube, H., Friese, A., von Salviati, C., Guerra, B., Käsbohrer, A., Kreienbrock, L., et al. (2013). Longitudinal monitoring of extended-spectrum-beta-lactamase/ampc-producing Escherichia coli at german broiler chicken fattening farms. Appl. Env. Microbiol. 79(16), 4815–4820. doi: 10.1128/AEM.00856-13
Lay, K. K., Jeamsripong, S., Sunn, K. P., Angkititrakul, S., Prathan, R., Srisanga, S., and Chuanchuen, R. (2021). Colistin Resistance and ESBL Production in Salmonella and Escherichia coli from pigs and pork in the thailand, cambodia, lao pdr, and myanmar border area. Antibiotics 10(6):657. doi: 10.3390/antibiotics10060657
Leão, C., Clemente, L., Guerra, V., Botelho, A., and Amaro, A. (2021). Occurrence of a rare multidrug resistant Escherichia coli coharboring blaCTX-M-32 and blaCTX-M-2 Genes in a bovine. Micro. Drug Res. 27(8), 1155–1157. doi: 10.1089/mdr.2020.0520
Liu, Y.-Y., Wang, Y., Walsh, T. R., Yi, L.-X., Zhang, R., Spencer, J., Doi, Y., et al. (2016). Emergence of plasmid-mediated colistin resistance mechanism MCR-1 in animals and human beings in China: a microbiological and molecular biological study. Lancet. Infect. Dis. 16(2), 161–168. doi: 10.1016/S1473-3099(15)00424-7
Lobie, T. A., Roba, A. A., Booth, J. A., Kristiansen, K. I., Aseffa, A., Skarstad, K., et al. (2021). Antimicrobial resistance: a challenge awaiting the post-COVID-19 era. Int. J. Infect. Dis. 111, 322–325. doi: 10.1016/j.ijid.2021.09.003
Loras, C., Mendes, A. C., Peixe, L., Novais, Â., and Alós, J.-I. (2020). Escherichia coli resistant to fosfomycin from urinary tract infections: detection of the fosA3 gene in Spain. J. Glob. Antimicrob. Res. 21, 414–416. doi: 10.1016/j.jgar.2020.01.023
Lu, J., Sanchez, S., Hofacre, C., Maurer, J. J., Harmon, B. G., and Lee, M. D. (2003). Evaluation of broiler litter with reference to the microbial composition as assessed by using 16S rRNA and functional gene markers. Appl. Env.Microbiol. 69(2), 901–908. doi: 10.1128/aem.69.2.901-908.2003
Lukman, D., Sudarwanto, M., Purnawarman, T., Latif, H., Pisestyani, H., Sukmawinata, E., Akineden, Ö., et al. (2017). CTX-M-1 and CTX-M-55 Producing Escherichia coli isolated from broiler feces in poultry slaughterhouse, bogor, west java province. Glob. Adv.Res.J. Med. Med. Sci. 5, 287–291.
Lupo, A., Saras, E., Madec, J.-Y., and Haenni, M. (2018). Emergence of blaCTX-M-55 associated with fosA, rmtB and mcr gene variants in Escherichia coli from various animal species in France. J. Antimicrob. Chem. 73(4), 867–872. doi: 10.1093/jac/dkx489
Magnusson, U. (2020). Prudent and effective antimicrobial use in a diverse livestock and consumer’s world. J. Animal Sci. 98, S4–S8. doi: 10.1093/jas/skaa148
Manges, A. R., Geum, H. M., Guo, A., Edens, T. J., Fibke, C. D., and Pitout, J. D. (2019). Global Extraintestinal Pathogenic Escherichia coli (ExPEC) Lineages. Clin. Microbiol. Rev. 32(3), e135–e118. doi: 10.1128/CMR.00135-18
Martirosov, D. M., and Lodise, T. P. (2016). Emerging trends in epidemiology and management of infections caused by carbapenem-resistant Enterobacteriaceae. Diagn. Microbiol. Infect. Dis. 85(2), 266–275. doi: 10.1016/j.diagmicrobio.2015.10.008
McGann, P., Snesrud, E., Maybank, R., Corey, B., Ong, A. C., Clifford, R., et al. (2016). Escherichia coli Harboring mcr-1 and blaCTX-M on a Novel IncF Plasmid: First report of mcr-1 in the united states. Antimicrob. Agents Chem. 60(7), 4420–4421. doi: 10.1128/AAC.01103-16
Miles, T. D., McLaughlin, W., and Brown, P. D. (2006). Antimicrobial resistance of Escherichia coli isolates from broiler chickens and humans. BMC Vet. Res. 2:7. doi: 10.1186/1746-6148-2-7
Moeinizadeh, H., and Shaheli, M. (2021). Frequency of hlyA, hlyB, hlyC and hlyD genes in uropathogenic Escherichia coli isolated from UTI patients in shiraz. GMS Hygiene Infect. Control 16:Doc25. doi: 10.3205/dgkh000396
Moffo, F., Mouiche, M. M. M., Djomgang, H. K., Tombe, P., Wade, A., Kochivi, F. L., et al. (2021). Poultry litter contamination by Escherichia coli resistant to critically important antimicrobials for human and animal use and risk for public health in cameroon. Antibiotics 10(4):402. doi: 10.3390/antibiotics10040402
Neuner, E. A., Sekeres, J., Hall, G. S., and van Duin, D. (2012). Experience with fosfomycin for treatment of urinary tract infections due to multidrug-resistant organisms. Antimicrob. Agents Chemother. 56(11), 5744–5748. doi: 10.1128/AAC.00402-12
Nieuwlaat, R., Mbuagbaw, L., Mertz, D., Burrows, L. L., Bowdish, D. M. E., Moja, L., et al. (2021). Coronavirus Disease 2019 and antimicrobial resistance: parallel and interacting health emergencies. Clin. Infect. Dis. 72(9), 1657–1659. doi: 10.1093/cid/ciaa773
O’Neill, J. (2014). The Review on Antimicrobial Resistance. Antimicrobial Resistance: Tackling a Crisis for the Health and Wealth of Nations. Available online at https://amr-review.org/sites/default/files/AMR%20Review%20Paper%20-%20Tackling%20a%20crisis%20for%20the%20health%20and%20wealth%20of%20nations_1.pdf (accessed November 20, 2021).
Osman, K. M., Kappell, A. D., Elhadidy, M., ElMougy, F., El-Ghany, W. A. A., Orabi, A., et al. (2018). Poultry hatcheries as potential reservoirs for antimicrobial-resistant Escherichia coli: a risk to public health and food safety. Sci. Rep. 8(1):5859. doi: 10.1038/s41598-018-23962-7
Park, H., Kim, J., Ryu, S., and Jeon, B. (2019). Predominance of blaCTX-M-65 and blaCTX-M-55 in extended-spectrum β-lactamase-producing Escherichia coli from raw retail chicken in South Korea. J. Glob. Antimicrob. Res. 17, 216–220. doi: 10.1016/j.jgar.2019.01.005
Paterson, D. L., and Bonomo, R. A. (2005). Extended-Spectrum β-Lactamases: a clinical update. Clin. Microbiol. Rev. 18(4), 657–686. doi: 10.1128/CMR.18.4.657-686.2005
Pauwels, I., Versporten, A., Drapier, N., Vlieghe, E., Goossens, H., and Saleem, Z. (2021). Hospital antibiotic prescribing patterns in adult patients according to the WHO access, watch and reserve classification (AWaRe): Results from a worldwide point prevalence survey in 69 countries. J. Antimicrob. Chemother. 76, 1614–1624. doi: 10.1093/jac/dkab050
Poirel, L., Madec, J.-Y., Lupo, A., Schink, A.-K., Kieffer, N., Nordmann, P., and Schwarz, S. (2018). Antimicrobial resistance in Escherichia coli. Microbiol. Spectr. 6, 289–316. doi: 10.1128/microbiolspec.ARBA-0026-2017
Poulsen, L. L., Thøfner, I., Bisgaard, M., Christensen, J. P., Olsen, R. H., and Christensen, H. (2017). Longitudinal study of transmission of Escherichia coli from broiler breeders to broilers. Vet. Microbiol. 207, 13–18. doi: 10.1016/j.vetmic.2017.05.029
Rossato, J. M., Brito, B. G., Kobayashi, R. K. T., Koga, V. L., Sarmiento, J. J. P., Nakazato, G., et al. (2019). Antimicrobial resistance, diarrheagenic and avian pathogenic virulence genes in Escherichia coli from poultry feed and the ingredients. Arq. Bras. Med. Vet. Zootec. 71, 1968–1976. doi: 10.1590/1678-4162-10355
Roth, N., Kasbohrer, A., Mayrhofer, S., Zitz, U., Hofacre, C., and Domig, K. J. (2019). The application of antibiotics in broiler production and the resulting antibiotic resistance in Escherichia coli: a global overview. Poul. Sci. 98, 1791–1804. doi: 10.3382/ps/pey539
Saharan, V. V., Verma, P., and Singh, A. P. (2020). Escherichia coli, Salmonella spp., and Staphylococcus aureus susceptibility to antimicrobials of human and veterinary importance in poultry sector of India. J. Food Safety 40(1):e12742. doi: 10.1111/jfs.12742
Sarker, Md., Mannan, MS., Ali, MY., Bayzid, M., Ahad, A., Bupasha, ZB., et al. (2019). Antibiotic resistance of Escherichia coli isolated from broilers sold at live bird markets in Chattogram, Bangladesh. J Adv Vet Anim Res. 6, 272–277. doi: 10.5455/javar.2019.f344
Sato, N., Kawamura, K., Nakane, K., Wachino, J.-I., and Arakawa, Y. (2013). First detection of fosfomycin resistance gene fosA3 in CTX-M-producing Escherichia coli isolates from healthy individuals in Japan. Micro. Drug Res. 19(6), 477–482. doi: 10.1089/mdr.2013.0061
Schnall, J., Rajkhowa, A., Ikuta, K., Rao, P., and Moore, C. E. (2019). Surveillance and monitoring of antimicrobial resistance: Limitations and lessons from the GRAM project. BMC Med. 17(1);176. doi: 10.1186/s12916-019-1412-8
Seo, K. W., and Lee, Y. J. (2019). Characterization of plasmid mediated quinolone resistance determinants in ciprofloxacin resistant-Escherichia coli from chicken meat produced by integrated broiler operations in Korea. Int. J. Food Microbiol. 307:108274. doi: 10.1016/j.ijfoodmicro.2019.108274
Seok, H., Choi, J. Y., Wi, Y. M., Park, D. W., Peck, K. R., and Ko, K. S. (2020). Fosfomycin resistance in escherichia coli isolates from south korea and in vitro activity of fosfomycin alone and in combination with other antibiotics. Antibiotics 9(3):112. doi: 10.3390/antibiotics9030112
Siller, P., Dähre, K., Thiel, N., Nübel, U., and Rösler, U. (2020). Impact of short-term storage on the quantity of extended-spectrum beta-lactamase–producing Escherichia coli in broiler litter under practical conditions. Poul. Sci. 99. 2125–2135. doi: 10.1016/j.psj.2019.11.043
Song, J., Oh, S.-S., Kim, J., Park, S., and Shin, J. (2020). Clinically Relevant Extended-Spectrum β-Lactamase–Producing Escherichia coli isolates from food animals in South Korea. Front. Microbiol. 11:604. doi: 10.3389/fmicb.2020.00604
Thanner, S., Drissner, D., and Walsh, F. (2016). Antimicrobial resistance in agriculture. MBio 7(2), e2227–e2215. doi: 10.1128/mBio.02227-15
Thomrongsuwannakij, T., Narinthorn, R., Mahawan, T., and Blackall, P. J. (2021). Molecular and phenotypic characterization of avian pathogenic Escherichia coli isolated from commercial broilers and native chickens. Poul. Sci. 101:101527. doi: 10.1016/j.psj.2021.101527
Versalovic, J., Koeuth, T., and Lupski, R. (1991). Distribution of repetitive DNA sequences in eubacteria and application to finerpriting of bacterial enomes. Nucleic Acids Res. 19, 6823–6831. doi: 10.1093/nar/19.24.6823
Vieira M. de, F. A., Tinoco I. de, F. F., Santos, B. M., dos Inoue, K. R. A., Mendes, M. A., et al. (2015). Sanitary quality of broiler litter reused. Eng. Agrícola 35(5), 800–807. doi: 10.1590/1809-4430-Eng.Agric.v35n5p800-807/2015
Wachino, J., Yamane, K., Suzuki, S., Kimura, K., and Arakawa, Y. (2010). Prevalence of fosfomycin resistance among ctx-m-producing Escherichia coli clinical isolates in japan and identification of novel plasmid-mediated Fosfomycin-Modifying Enzymes. Antimicrob. Agents Chemother. 54(7), 3061–3064. doi: 10.1128/AAC.01834-09
Waziri, M. I., and Kaltungo, B. Y. (2017). Poultry litter selection, management and utilization in the tropics. Poul. Sci. 6, 44–55. doi: 10.5772/65036
Wei, S., Morrison, M., and Yu, Z. (2013). Bacterial census of poultry intestinal microbiome. Poul. sci. 92, 671–683. doi: 10.3382/ps.2012-02822
WHO (2018). WHO Report On Surveillance Of Antibiotic Consumption: 2016-2018 Early Implementation. Geneva: World Health Organization.
WHO. (2019). The 2019 WHO AWaRe Classification Of Antibiotics For Evaluation And Monitoring Of Use. Available online at https://apps.who.int/iris/handle/10665/327957 [Accessed January 11, 2021]
Woodford, N., Fagan, E. J., and Ellington, M. J. (2006). Multiplex PCR for rapid detection of genes encoding CTX-M extended-spectrum β-lactamases. J. Antimicrob. Chemother. 57(1), 154–155. doi: 10.1093/jac/dki412
Yévenes, K., Pokrant, E., Trincado, L., Lapierre, L., Galarce, N., Martín, B. S., et al. (2021). Detection of antimicrobial residues in poultry litter: monitoring a risk through a selective and sensitive HPLC–MS/MS method. Animals 11(5), 1399. doi: 10.3390/ani11051399
Yin, M., Hu, G., Shen, Z., Fang, C., Zhang, X., Li, D., et al. (2020). In vivo evolution of ctx-m-215, a novel narrow-spectrum β-lactamase in an Escherichia coli clinical isolate conferring resistance to mecillinam. Antimicrob. Agents Chemother. 64(11), e00562–e520. doi: 10.1128/AAC.00562-20
Zalewska, M., Błażejewska, A., Czapko, A., and Popowska, M. (2021). Antibiotics and antibiotic resistance genes in animal manure – consequences of its application in agriculture. Front. Microbiol. 12:640. doi: 10.3389/fmicb.2021.610656
Zhao, S., Wang, C.L., Chang, S.K., Tsai, Y.L., and Chou, C.H. (2019). Characterization of Escherichia coli Isolated from day-old chicken fluff in taiwanese hatcheries. Avian Dis. 63(1), 9–16. doi: 10.1637/11935-072318-Reg.1
Zurfluh, K., Wang, J., Klumpp, J., Nüesch-Inderbinen, M., Fanning, S., and Stephan, R. (2014). Vertical transmission of highly similar blaCTX-M-1-harboring IncI1 plasmids in Escherichia coli with different MLST types in the poultry production pyramid. Front. Microbiol. 5:519.
Keywords: blaCTX–M–55 gene, fosA3 gene, broiler chicken farms, multidrug resistance, fosfomycin, longitudinal monitoring, ESBL (extended spectrum beta lactamase)
Citation: Menck-Costa MF, Baptista AAS, Gazal LES, Justino L, Sanches MS, de Souza M, Nishio EK, Queiroz dos Santos B, Cruz VD, Berbert JVM, Gonçalves BC, Andrade G, Vespero EC, Nakazato G and Kobayashi RKT (2022) High-Frequency Detection of fosA3 and blaCTX–M–55 Genes in Escherichia coli From Longitudinal Monitoring in Broiler Chicken Farms. Front. Microbiol. 13:846116. doi: 10.3389/fmicb.2022.846116
Received: 30 December 2021; Accepted: 11 April 2022;
Published: 18 May 2022.
Edited by:
Ziad Daoud, Central Michigan University, United StatesReviewed by:
Dandan He, Henan Agricultural University, ChinaStephanie Lo, Wellcome Sanger Institute (WT), United Kingdom
Copyright © 2022 Menck-Costa, Baptista, Gazal, Justino, Sanches, de Souza, Nishio, Queiroz dos Santos, Cruz, Berbert, Gonçalves, Andrade, Vespero, Nakazato and Kobayashi. This is an open-access article distributed under the terms of the Creative Commons Attribution License (CC BY). The use, distribution or reproduction in other forums is permitted, provided the original author(s) and the copyright owner(s) are credited and that the original publication in this journal is cited, in accordance with accepted academic practice. No use, distribution or reproduction is permitted which does not comply with these terms.
*Correspondence: Renata Katsuko Takayama Kobayashi, kobayashirkt@uel.br