- 1Centro Nacional de Acuicultura e Investigaciones Marinas (CENAIM), Escuela Superior Politécnica del Litoral (ESPOL), Guayaquil, Ecuador
- 2Facultad de Ciencias de la Vida (FCV), Escuela Superior Politécnica del Litoral (ESPOL), Guayaquil, Ecuador
- 3Facultad de Ciencias Agropecuarias, Universidad Técnica de Machala, Machala, Ecuador
- 4Industrial Biotechnology Research Group, Center for Nanoscience and Nanotechnology (CENCINAT), Universidad de las Fuerzas Armadas (ESPE), Sangolquí, Ecuador
- 5Facultad de Ingeniería Marítima y Ciencias del Mar (FIMCM), Escuela Superior Politécnica del Litoral (ESPOL), Guayaquil, Ecuador
Acute hepatopancreatic necrosis disease (AHPND) is an emerging bacterial disease of cultured shrimp caused mainly by Vibrio parahaemolyticus, which harbors the lethal PirAB toxin genes. Although Penaeus vannamei (P. vannamei) postlarvae are susceptible to AHPND, the changes in the bacterial communities through the larval stages affected by the disease are unknown. We characterized, through high-throughput sequencing, the microbiome of P. vannamei larvae infected with AHPND-causing bacteria through the larval stages and compared the microbiome of larvae collected from high- and low-survival tanks. A total of 64 tanks from a commercial hatchery were sampled at mysis 3, postlarvae 4, postlarvae 7, and postlarvae 10 stages. PirAB toxin genes were detected by PCR and confirmed by histopathology analysis in 58 tanks. Seven from the 58 AHPND-positive tanks exhibited a survival rate higher than 60% at harvest, despite the AHPND affectation, being selected for further analysis, whereas 51 tanks exhibited survival rates lower than 60%. A random sample of 7 out of these 51 AHPND-positive tanks was also selected. Samples collected from the selected tanks were processed for the microbiome analysis. The V3–V4 hypervariable regions of the 16S ribosomal RNA (rRNA) gene of the samples collected from both the groups were sequenced. The Shannon diversity index was significantly lower at the low-survival tanks. The microbiomes were significantly different between high- and low-survival tanks at M3, PL4, PL7, but not at PL10. Differential abundance analysis determined that biomarkers associated with high and low survival in shrimp hatchery tanks affected with AHPND. The genera Bacillus, Vibrio, Yangia, Roseobacter, Tenacibaculum, Bdellovibrio, Mameliella, and Cognatishimia, among others, were enriched in the high-survival tanks. On the other hand, Gilvibacter, Marinibacterium, Spongiimonas, Catenococcus, and Sneathiella, among others, were enriched in the low-survival tanks. The results can be used to develop applications to prevent losses in shrimp hatchery tanks affected by AHPND.
Introduction
Diseases that affect the early larval stages of farmed shrimp are one of the main limitations for the sustainability of the shrimp industry. Thus, it is essential to implement mitigation tools, such as administering probiotics for bacterial infections. Some genera and species such as Vibrio harveyi (V. harveyi) (Haldar et al., 2011; Mirbakhsh et al., 2014), Vibrio alginolyticus (V. alginolyticus) (Karunasagar et al., 1998; Hasan et al., 2017), and Vibrio campbellii (V. campbellii) (Hameed, 1995; Soto-Rodríguez et al., 2006; Haldar et al., 2011) have been reported as recurrent pathogens in commercial shrimp hatcheries from many producing countries. Acute hepatopancreatic necrosis disease (AHPND), caused mainly by Vibrio parahaemolyticus (Tran et al., 2013), produces massive mortalities (Kongrueng et al., 2015; Sirikharin et al., 2015) and affects cultured Penaeus vannamei (P. vannamei) and Penaeus monodon (P. monodon) shrimps (de la Peña et al., 2015). AHPND-causing strains contain plasmids of approximately 70 kbp and harbor genes encoding for two toxins related to the PirA and PirB insecticidal toxin (Han et al., 2015). The PirAB toxins are released in the digestive system of the shrimp, causing severe desquamation of the hepatopancreas cells and the consequent death of the infected shrimp.
The diversity of species conforming to the intestinal microbiome performs critical functions in a host, such as aiding nutrient absorption, antagonizing against pathogenic bacteria, and improving the immune system (Dai et al., 2020). Therefore, the studies on the intestinal microbiome are helpful to identify biomarkers that could predict the onset of diseases through comparative analysis. For example, in a study of the shrimp microbiome throughout early stages, the genus Cupriavidus was always present and significantly abundant in healthy larvae (Zheng et al., 2017). Similarly, Meridianimaribacter has been identified in healthy shrimp water (Xue et al., 2015). Recently, the function and assembly of the bacterial community and biomarkers associated with each developmental stage for shrimp P. vannamei have been reported (Wang H. et al., 2020). Most investigations have characterized the P. vannamei microbiome in juvenile stages (Chen et al., 2017; Cornejo-Granados et al., 2017; Xiong et al., 2018; Dong et al., 2021; Restrepo et al., 2021). Thus, the microbiome of juvenile P. vannamei shrimp affected by AHPND is significantly different than in healthy shrimp (Cornejo-Granados et al., 2017). However, little is known about the bacterial community in larval stages under AHPND affectation.
This study aimed to characterize, through high-throughput sequencing (HTS), the microbiome of P. vannamei naturally infected with AHPND-causing bacteria across the larval stages and to obtain biomarkers associated with high and low survival in shrimp hatchery tanks affected with AHPND. The results can be used for further development of probiotics to mitigate AHPND.
Materials and Methods
Sample Collection and Processing
Penaeus vannamei larvae were collected from January to March 2021, during the whole production cycle, from 64 tanks (35 tons) from a commercial hatchery in South America (Figure 1). Sampling was performed in each tank at four stages, for a total of 233 samples of larvae: mysis 3 (M3) (n = 64), postlarvae 4 (PL4) (n = 64), postlarvae 7 (PL7) (n = 56), and postlarvae 10 (PL10) (n = 49). Only 56 and 49 samples were collected at PL7 and PL10 as the population of 8 and 7 tanks died at PL6 and PL9 stages, respectively. All the tanks received the same management protocol: feeding, water exchange, and supplementation of commercial probiotics (Supplementary Table 1). Water quality parameters during the production cycles were: water temperature = 32.5–33.0°C, dissolved oxygen concentration = 5.0 mg/L, pH = 7.9–8.1, and salinity = 35.0 ± 0.0 g/L. Observations of external signs of disease were carried out during each sampling (swimming behavior, empty digestive tract, and larval activity). After a maximum of 1 h from sampling to processing at the laboratory, 20 larvae of each sample collected at PL7 and PL10 stages were preserved in Davidson’s AFA fixative solution for histopathologic examination. Approximately, 1 g of each sample (M3, PL4, PL7, and PL10) was rinsed with a 2% NaCl sterile solution to remove impurities and external microorganisms. The last group of samples was macerated for homogenization of the bacterial load. A first aliquot (500 μl) was processed to detect subsequent PirAB toxin genes. A second aliquot (500 μl) was distributed in 2 ml cryovial tubes to submerge them for 30 min in liquid nitrogen (−196°C) and immediately stored at −80°C for further microbiome analysis. Samples of the four commercial probiotics and a pool of the four commercial feeds administered during the production cycle (Supplementary Table 1) were also collected and preserved, as mentioned above, for further microbiome analysis.
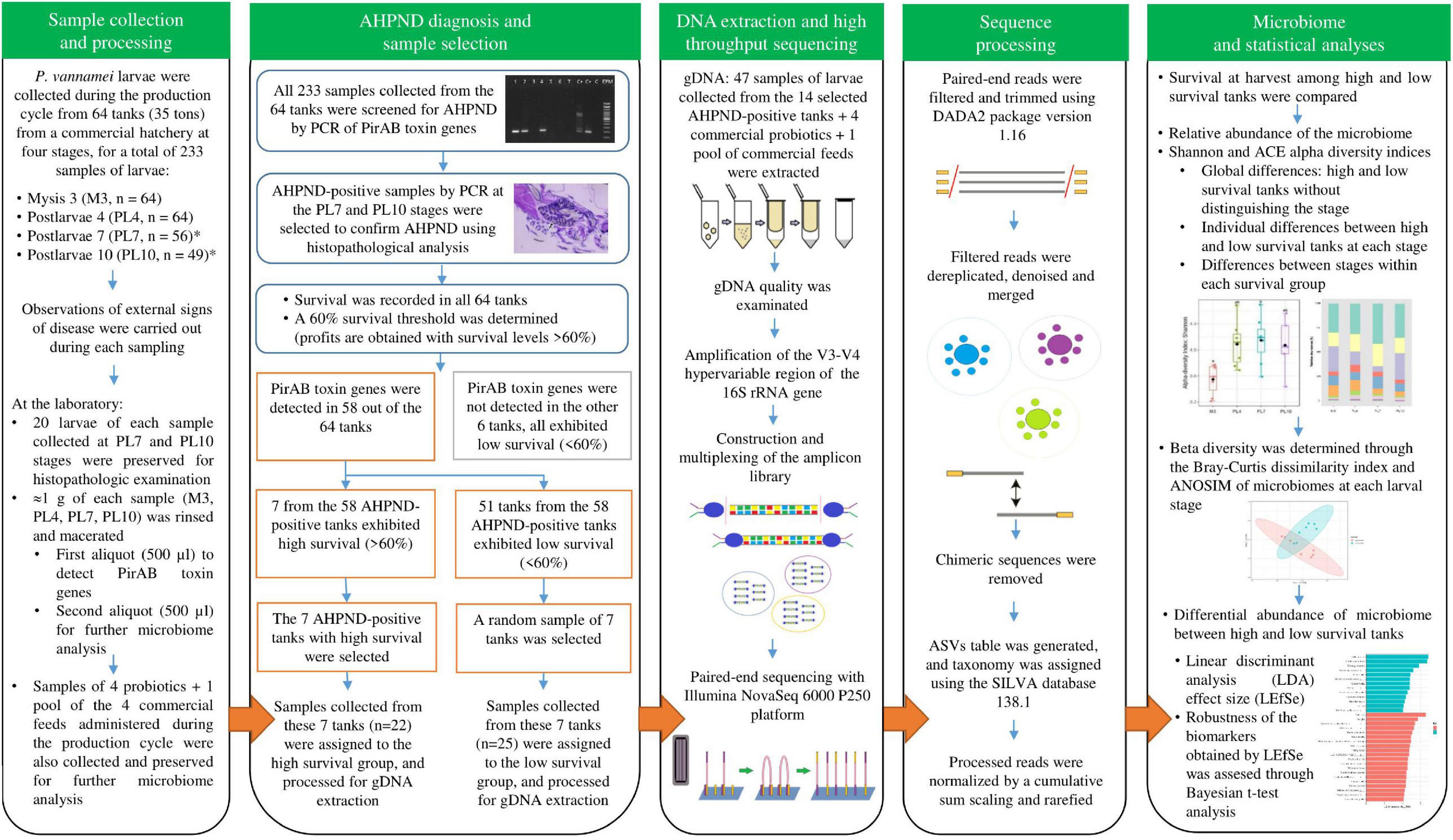
Figure 1. Experimental design for the microbiome characterization of Penaeus vannamei (P. vannamei) larvae affected with acute hepatopancreatic necrosis disease (AHPND) and identification of potential biomarkers associated with high and low survival in shrimp hatchery tanks through high-throughput sequencing. *Only 56 and 49 samples were collected at PL7 and PL10 as the population of 8 and 7 tanks died at PL6 and PL9 stages, respectively.
Acute Hepatopancreatic Necrosis Disease Diagnosis and Sample Selection
All the 233 samples collected from the 64 tanks were screened for AHPND by PCR of PirAB toxin genes (Figure 1). Genomic DNA (gDNA) was extracted from each sample using the phenol standard protocol (Kerkhof and Ward, 1993). PirAB toxin genes were amplified with the primers AP4 F1/R1–AP4 F2/R2, VpPirA-284F/R, and VpPirB-392F/R (Dangtip et al., 2015; Han et al., 2015). AHPND-positive samples by PCR at the PL7 and PL10 stages were selected to confirm AHPND affectation using histopathological analysis. The paraffin-embedded tissue sections were cut at 4 μm, stained with Mayer–Bennet H&E (Bell and Lightner, 1988), and examined for histopathological changes. Survival was recorded in all the 64 tanks: at harvest in 49 tanks (PL11) and reported as 0% for the other 15 tanks, as their populations died at PL6 (8 tanks) and PL9 (7 tanks). A 60% survival threshold was determined according to the historical data of the shrimp hatchery, where profits were obtained with survival levels higher than 60% (Figure 1). PirAB toxin genes were detected in 58 out of the 64 tanks and not detected in the other 6 tanks (Figure 1). These six tanks exhibited low survival (<60%). Seven from the 58 AHPND-positive tanks exhibited high survival (>60%), whereas 51 tanks exhibited low survival (<60%) (Figure 1). The seven AHPND-positive tanks with high survival were selected for further analysis (Figure 1). As the number of AHPND-positive tanks with low survival was much higher than the high ones, a random sample of seven tanks was selected for further analysis to obtain an equal number of tanks for each survival condition (Figure 1). Samples of larvae collected from these 14 AHPND-positive tanks were assigned to the two groups of survival conditions: high (survival >60%, n = 22 samples of larvae) and low (survival <60%, n = 25 samples of larvae) and processed for gDNA extraction (Figures 1, 2).
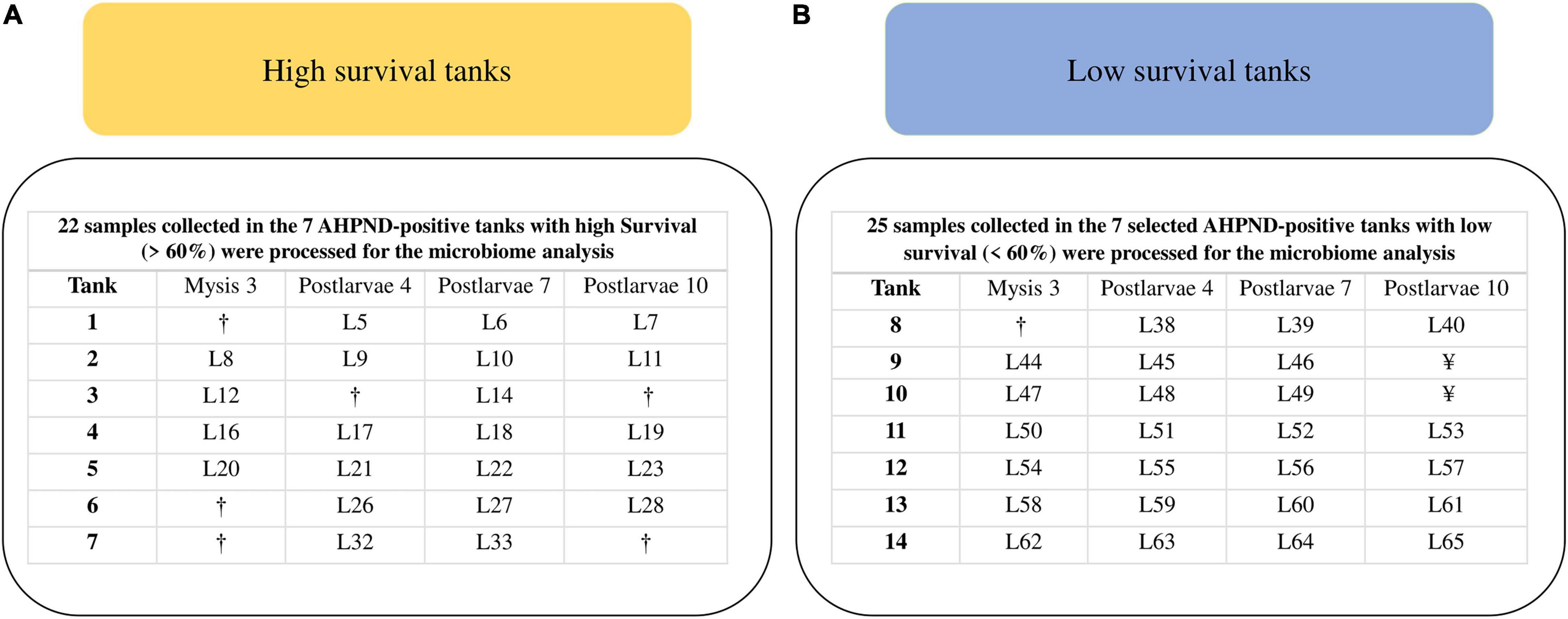
Figure 2. Samples selected for the microbiome characterization of P. vannamei larvae affected by AHPND. (A) High-survival tanks. (B) Low-survival tanks. †Sample did not pass the DNA quality control. ¥Sample not collected as the tank population died at PL9.
Deoxyribonucleic Acid Extraction and High-Throughput Sequencing
The gDNA of the 47 AHPND-positive samples of larvae from the 14 selected tanks (high and low survival), four commercial probiotics, and the pool of commercial feeds were extracted (Figure 1) using the ZymoBIOMICS DNA Microprep Isolation Kit (Zymo Research, United States), following the manufacturer’s instructions. An aliquot of 20 μl of gDNA was diluted in DNase-free ultrapure water. DNA quality (1.8–2.0 A260/280) was examined through a NanoDrop One Microvolume UV-Vis Scanning Spectral Spectrophotometer (Thermo Fisher Scientific, United States). The amplification of the V3–V4 hypervariable region (470 bp) of the 16S ribosomal RNA (rRNA) gene was performed with the 341F/806R primers (Takahashi et al., 2014). DNA was submitted to Novogene Incorporation (Beijing, China) for construction and multiplexing of the amplicon library. Paired-end sequencing was performed with Illumina NovaSeq 6000 P250 platform. The sequences were deposited in the National Center for Biotechnology Information (NCBI) Sequence Read Archive (SRA) under accession number PRJNA800805.
Sequence Processing
Paired-end reads were processed using DADA2 package version 1.16 (Callahan et al., 2016) in the R software version 3.6.3 (Figure 1). Without adapters and primers, paired-ends were filtered and trimmed using the filterAndTrim function [sequences removed with more than two expected errors—maxEE = c(2,2) and trimming of last 10 nt of the forward reads and last 20 nt of the reverse reads—truncLen = c(240,230)]. The filtered reads were dereplicated, denoised, and merged (forward and reverse amplicon sequences) to get a merging sequence. Then, the chimeric sequences were removed using the “consensus” method from the removeBimeraDenovo function. The table of amplicon sequence variants (ASVs) was generated with the makeSequenceTable function and the taxonomy was assigned with the assignTaxonomy function using the public SILVA database version 138.1. A total number of 7 million clean reads was obtained (Supplementary Table 2), with an average of 149,047 clean reads per sample (range: 130,040–168,784). The error rate averaged 0.03% for all the reads. On average, 88% of the reads exhibited a Phred quality (Q > 30) and a GC content of 54%. A reading length of 250 bp was averaged for the alignment. A total of 8,626 ASVs was obtained, with an average of 393 ASVs per sample. The processed reads were normalized by a cumulative sum scaling and rarefied to 77,220 sequences per sample, which was sufficient to capture the alpha-diversity of the microbial communities through all the larval stages. Good’s coverage was higher than 99.99%, indicating an optimal sequencing depth.
Microbiome and Statistical Analyses
The survivals at harvest among the high- and low-survival tanks were compared by the t-test (Figure 1). Previously, the variance homogeneity and assumption of normality of both the treatments were examined through the F and Shapiro–Wilk normality tests. The null hypothesis, equal survival at both the tank groups, was rejected with a p < 0.05.
The microbiome analyses were performed at the ASVs level. The variability of the alpha-diversity indices and relative abundance of the microbiome were studied for the four stages from the high- and low-survival tanks (Figure 1). The alpha-diversity of the larval microbiome was estimated through the Shannon (Shannon and Weaver, 1949) and abundance-based coverage estimator—ACE (Hughes et al., 2001) indices. Three statistical analyses were carried out to evaluate the hypotheses of each alpha-diversity index. The first statistical analysis evaluated the global differences between the high- and low-survival tanks without distinguishing the stage. The second analysis evaluated individual differences between the high- and low-survival tanks at each stage. Both the analyses were performed with the t-tests. Previously, the variance homogeneity and assumption of normality of treatments were examined through the F and Shapiro–Wilk tests. The third statistical analysis evaluated differences between stages within each survival condition group using the one-way ANOVA. The variance homogeneity and assumption of normality of treatments were previously examined through the Bartlett and Shapiro–Wilk tests. When significant results were found in the ANOVA test, the pairwise Tukey’s honestly significant difference test for multiple comparisons was used to evaluate statistical differences of the correspondent alpha-diversity index between stages. The null hypothesis, equal alpha-diversity indices between the groups, was rejected with a p < 0.05.
The beta-diversity was determined through the multivariate analysis of the Bray–Curtis dissimilarity index and visualized through a principal coordinate analysis (PCoA) for each stage (Figure 1). The null hypothesis of equal microbiomes between the high- and low-survival tanks was evaluated by the analysis of similarities (ANOSIM) at each larval stage.
Differential abundance analysis of the larval microbiome between the high- and low-survival tanks (Figure 1) was performed using a linear discriminant analysis (LDA) effect size (LEfSe) (Segata et al., 2011). A p-value correction for the multiple hypotheses, simultaneously tested at the LEfSe analysis, was performed to control the false discovery rate (FDR) (proportion of type I error) and increase the power to detect true positive biomarkers (Nearing et al., 2021; Wallen, 2021). ASVs with LDA cutoff of 2.0 and significant differences (p < 0.05) were considered as potential biomarkers associated with high and low survival in shrimp hatchery tanks affected with AHPND. To assess the robustness of the biomarkers obtained with the LEfSe analysis, a Bayesian t-test analysis was also performed for each biomarker associated either to the high- or low-survival tanks. The predictive performances of the null hypothesis H0 (equal ASVs abundance between the high- and low-survival tanks) and alternative hypothesis H1 (different ASVs abundance between the high- and low-survival tanks) were compared. The hypothesis testing was performed through the Bayes factor BF10, where BF10 indicates the relative performance in favor of H1 over H0 provided by the data and calculated as the ratio of the probability of H1 over the probability of H0 based on the data. Bayes factors higher than 1 showed evidence in favor of H1, with ranges of 1–3, 3–10, and >10 interpreted as weak, moderate, and strong evidence in favor of H1 (van Doorn et al., 2021). Microbiome and statistical analyses were conducted with the MicrobiomeAnalyst (Dhariwal et al., 2017), microbiomeMarker (Cao, 2020), Stats (R Core Team, 2013), and BayesFactor (Morey and Rouder, 2011) packages implemented in the R software version 3.6.3.
Results
Acute Hepatopancreatic Necrosis Disease Diagnosis and Sample Selection
PirAB toxin genes were detected in 58 out of 64 sampled tanks, whereas 6 tanks were negative. Larvae from the positive tanks presented symptoms of diseases (erratic swimming and pale hepatopancreas), especially at PL4 and PL7. The histopathologic analysis of these larvae samples at PL7 and PL10 revealed AHPND lesions at the hepatopancreas. Seven tanks from the 58 AHPND-positive tanks exhibited survival rates higher than 60% at harvest (mean ± SEM = 67.8 ± 3.10%), despite the AHPND affectation. The 22 samples of larvae collected from these 7 AHPND-positive tanks were assigned to the group of high-survival tanks and processed for gDNA extraction (Figure 2). The other 51 AHPND-positive tanks presented survival rates (15.2 ± 1.7%) lower than 60%. The random sample of 7 tanks selected from these 51 tanks exhibited an average survival of 17.9 ± 4.6% at harvest. The 25 samples of larvae collected from these 7 AHPND-positive tanks were assigned to the group of low-survival tanks and processed for gDNA extraction (Figure 2). A significant (p < 0.001) survival difference of 49.9% was observed between the high- and the selected low-survival tanks at harvest. The prevalences of AHPND lesions (mean ± SEM) in the larvae were 40.4 ± 13.6% in the high-survival tanks and 61.3 ± 13.2% in the low-survival tanks.
Microbiome and Statistical Analyses
In the first statistical analysis, significant differences (p = 0.004) in the Shannon index were found between the high- and low-survival tanks without distinguishing the stage (Supplementary Figure 1A); however, the ACE index was non-significantly different (p = 0.273) between both the groups (Supplementary Figure 1B). In the second statistical analysis, significantly lower Shannon and ACE indices (p = 0.002 and p = 0.030, respectively) were observed at M3 between the high- and low-survival tanks (Supplementary Figures 2A,B). Non-significant differences (p > 0.055) in the Shannon and ACE diversity indices were found when the samples from the high- and low-survival tanks were compared at the other three stages (Supplementary Figures 2A,B). In the third analysis, non-significant differences (p = 0.933 and p = 0.538, respectively) of the Shannon and ACE diversity indices were identified within the high-survival tanks (Figures 3A,B). However, the Shannon index was significantly lower (p = 0.038) at M3 than PL7 at the low-survival tanks (Figure 3C). The ACE index was significantly lower (p < 0.026) at M3 compared with the other three stages (Figure 3D), but non-significantly different (p > 0.998) between the other three stages at low-survival tanks (Figure 3D).
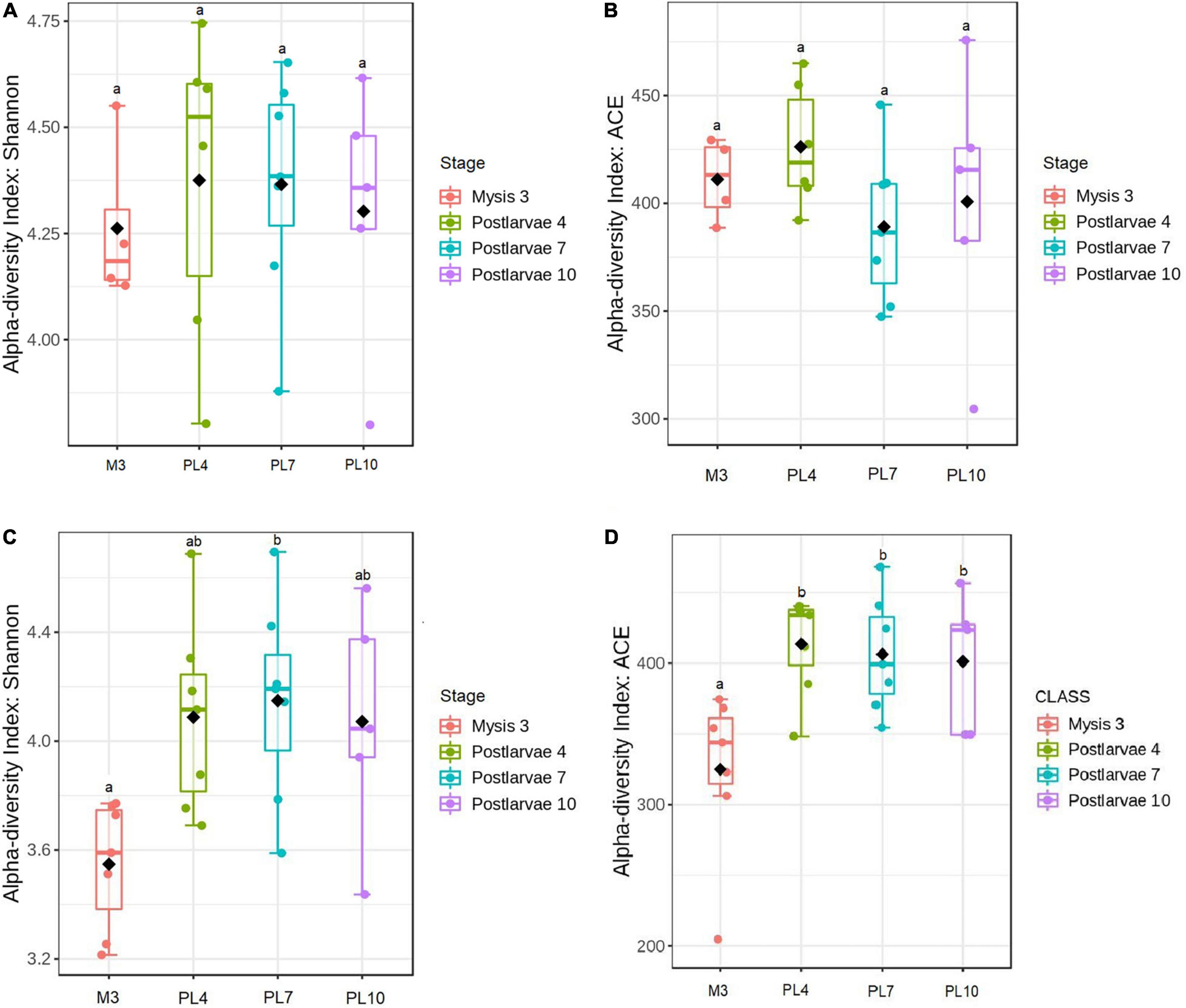
Figure 3. Alpha-diversity of the larval microbiome at amplicon sequence variant (ASV) level in the high- and low-survival tanks affected by AHPND. (A) Shannon index for the high-survival tanks. (B) ACE index for the high-survival tanks. (C) Shannon index for the low-survival tanks. (D) ACE index for the low-survival tanks. At each survival condition, pair of larval stages with different letters indicates alpha-diversity indices significantly different at p < 0.05, based on the Tukey’s honestly significant difference test.
Eighteen phyla, 29 classes, 74 orders, 107 families, 180 genera, and 58 species were identified in all the larva samples.
At the phylum level, Pseudomonadota exhibited the highest, and similar, abundances at both the high (74.2%) and low (73.0%) survival tanks, with 73.6% across the four stages at both the survival conditions (Figure 4A and Supplementary Table 3). Bacteroidota and Bacillota followed global average abundances of 13.6 and 5.2%, respectively (Figure 4A and Supplementary Table 3).
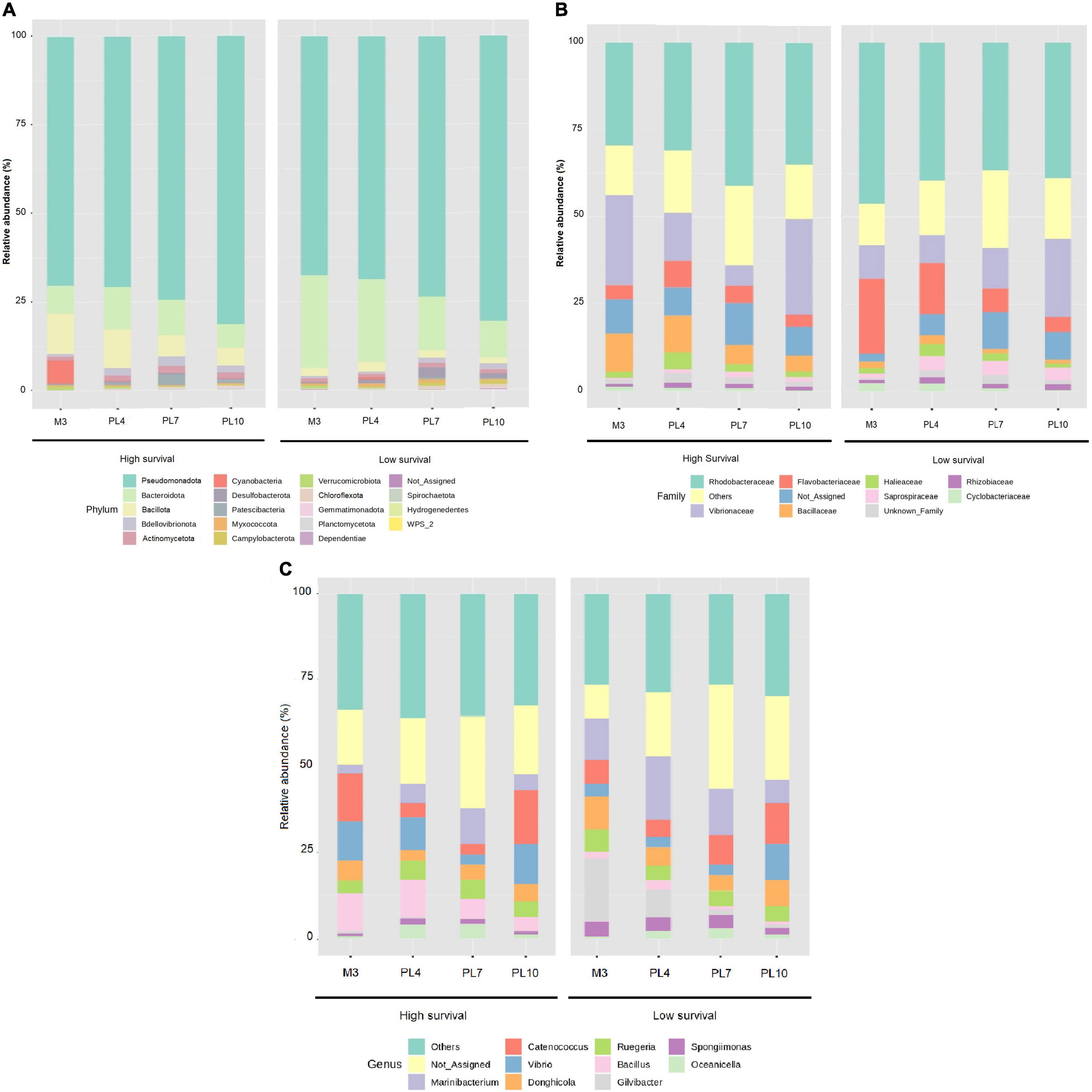
Figure 4. Relative abundance of the larval microbiome in the high- and low-survival tanks affected with AHPND. (A) Phylum level. (B) Family level. (C) Genus level.
The Rhodobacteraceae family exhibited the highest dominance compared to other families at both the high- and low-survival tanks (Figure 4B and Supplementary Table 3). The Vibrionaceae was the second most abundant family, especially at PL10 (41%), and at M3 (25%), in the high-survival tanks, contrasted with the same stages at the low-survival tanks (23 and 11%, respectively, Figure 4B and Supplementary Table 3). In contrast, Flavobacteriaceae was more abundant, especially at M3 and PL4, in the low-survival tanks, six and twofold higher than the abundances reported at the same stages in the high-survival tanks (Figure 4B and Supplementary Table 3). Bacillaceae showed the highest dominance in the high-survival tanks, five and twofold higher at M3 and PL4 than the low-survival tanks (Figure 4B and Supplementary Table 3).
The genus Vibrio was observed to be increased at M3 and PL4 stages in the high-survival tanks (Figure 4C and Supplementary Table 3), while Bacillus was more abundant in the high compared with the low-survival tanks (Figure 4C and Supplementary Table 3). The abundance of the Marinibacterium and Gilvibacter genus across stages was higher in the low-survival tanks compared with the high one (Figure 4C and Supplementary Table 3).
The dominant phyla in the probiotics samples were Bacillota (87%) and Pseudomonadota (11%) (Supplementary Figure 3A). At the genus level, the commercial probiotics 1, 2, and 3 samples were dominated by the genus Lentilactobacillus, Bacillus, and Clostridium (Supplementary Figure 3B). The probiotic four sample was dominated by the genus Weissella and Bacillus (Supplementary Figure 3B).
The phyla Bacillota (58%), Pseudomonadota (33%), and Cyanobacteria (10%) dominated the commercial feed sample (Supplementary Figure 3A). At the genus level, Lactobacillus and Bacillus were the most dominant in the commercial feed sample (Supplementary Figure 3B).
The ANOSIM analysis showed that the microbiomes were significantly different (p = 0.001) between the high- and low-survival tanks, with significant differences (p < 0.012) at M3, PL4, and PL7 (Figure 5). Such difference was more evident at M3 (Figure 5). Non-significant differences (p = 0.112) were found in the microbiomes at PL10 comparing both the survival conditions (Figure 5).
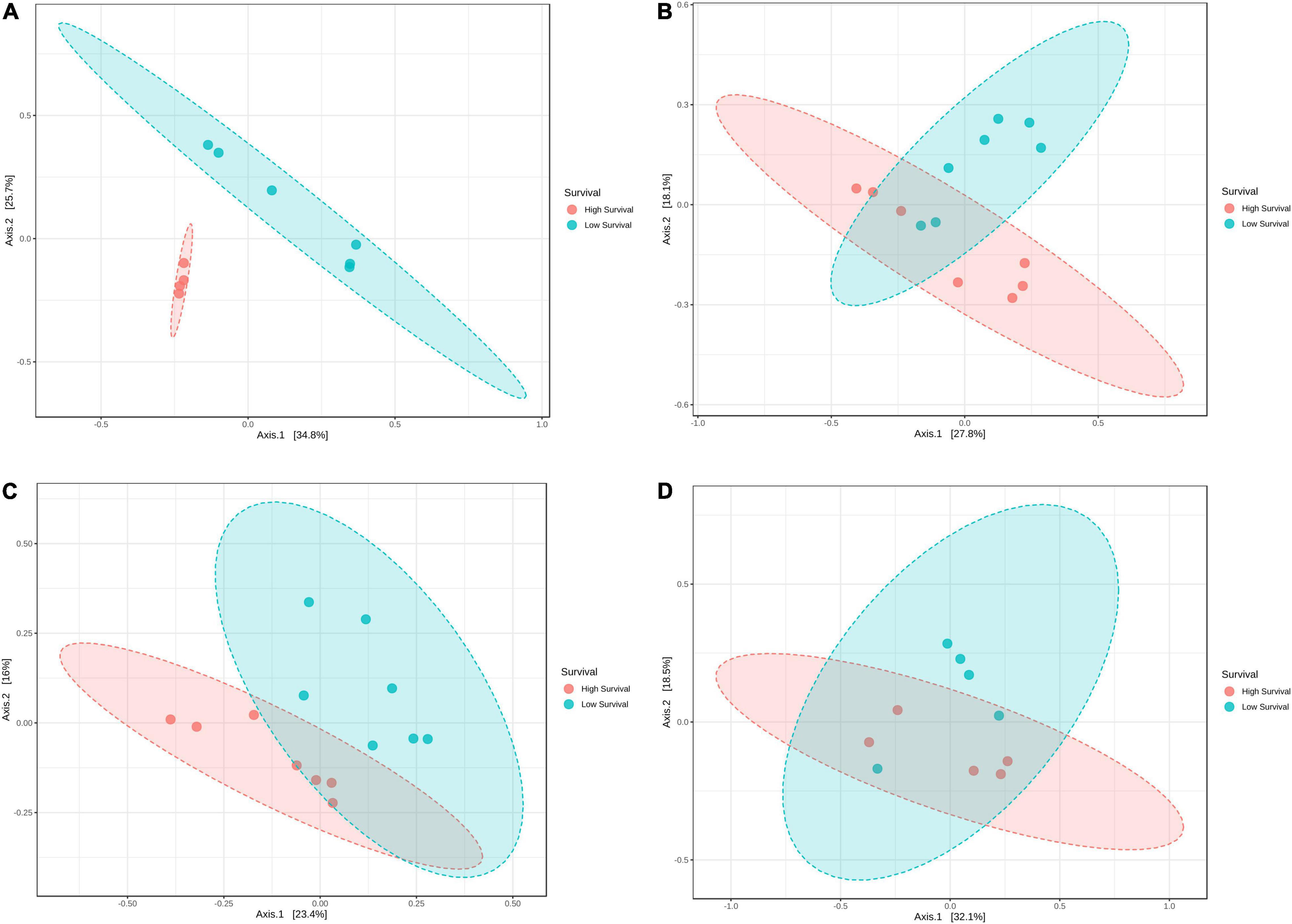
Figure 5. Principal coordinate analysis (PCoA) of the Bray–Curtis dissimilarity index from larvae collected at the high- and low-survival tanks affected with AHPND. (A) Mysis 3. (B) Postlarvae 4. (C) Postlarvae 7. (D) Postlarvae 10.
The LEfSe analysis showed 35 significant ASVs, 25 of which were differentially abundant for the high-survival tanks and 10 for the low-survival tanks (Supplementary Table 4 and Supplementary Figure 4). Consistently, the Bayesian analysis showed evidences (strong and moderate) for difference in the ASVs abundances between the high- and low-survival tanks for 33 ASVs (Supplementary Table 5). Only for two AVSs (ASV_58 and ASV_152), a weak evidence in favor of differences in the ASVS abundance between both the groups was reported (Supplementary Table 5). The genera Bacillus, Vibrio, Yangia, Roseobacter, Tenacibaculum, Bdellovibrio, Mameliella, Cognatishimia, and Pelagibaca, among others, were significantly enriched at the high-survival tanks, with Bacillus being the most abundant (Figures 6, 7). On the other hand, the genera Gilvibacter, Marinibacterium, Spongiimonas, Catenococcus, Sneathiella, and Escherichia, among others, were significantly enriched at the low-survival tanks (Figures 6, 7). Gilvibacter and Marinibacterium showed the largest effect size (LDA >2.0) at the low-survival tanks (Figure 6). Four ASVs differentially abundant at the high-survival tanks were also found in the probiotic samples (ASV-8, ASV-9, ASV-29, and ASV-52). These four ASVs were taxonomically identified as Bacillus (Figure 6 and Supplementary Figure 4). One ASV (ASV-73), differentially abundant at the high-survival tanks and identified as Bacillus, was not present in the probiotic samples (Figure 6 and Supplementary Figure 4). In addition, only one commercial probiotic sample (probiotic 1) did not report ASVs with significant abundance at the high-survival tanks.
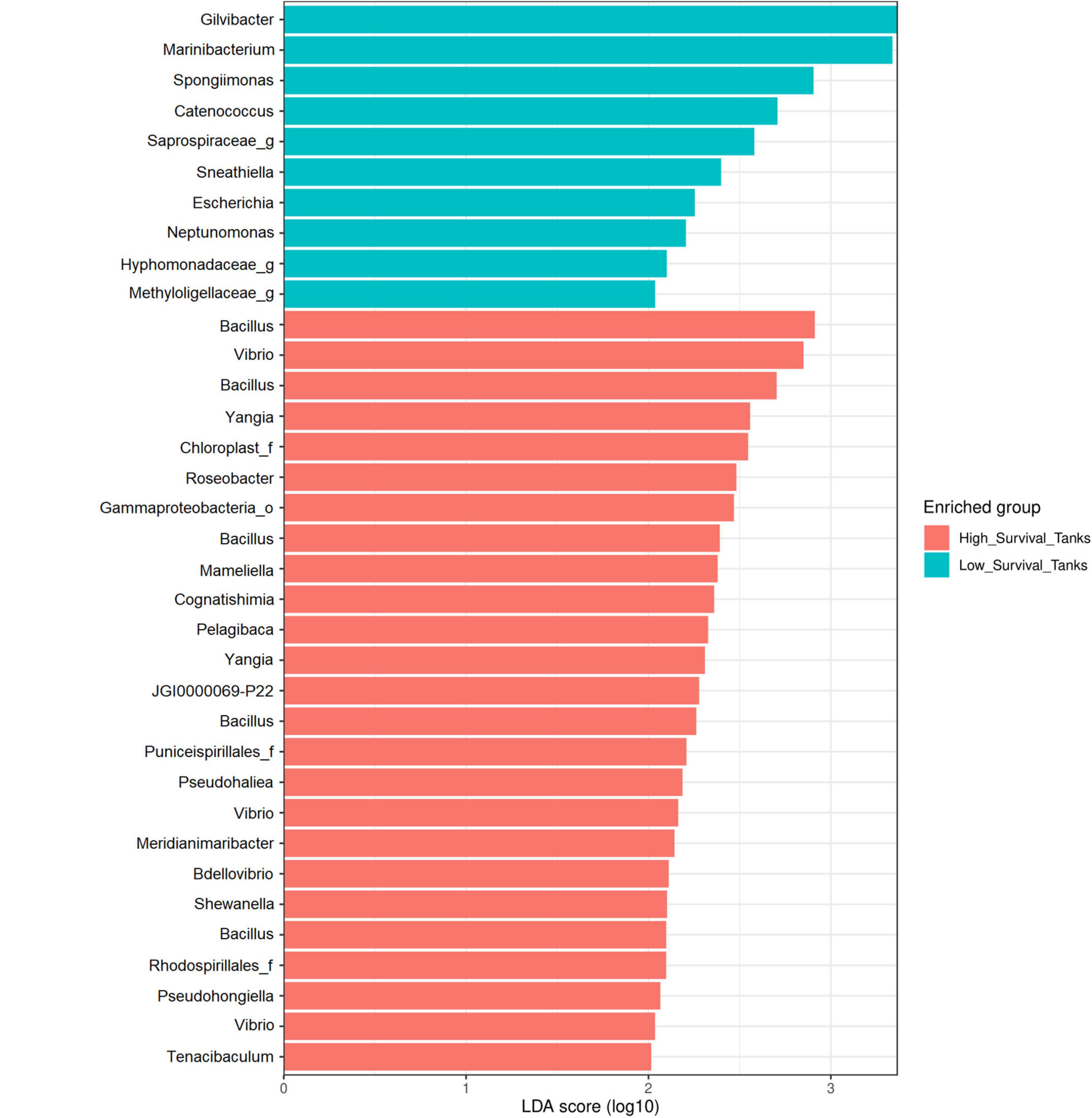
Figure 6. Results of the differential abundance analysis using a linear discriminant analysis (LDA) effect size (LEfSe) of larvae collected from the high- and low-survival tanks affected with AHPND. The length of the bar represents the effect size (LDA cutoff = 2) of all the bacterial lineages at the genus level.
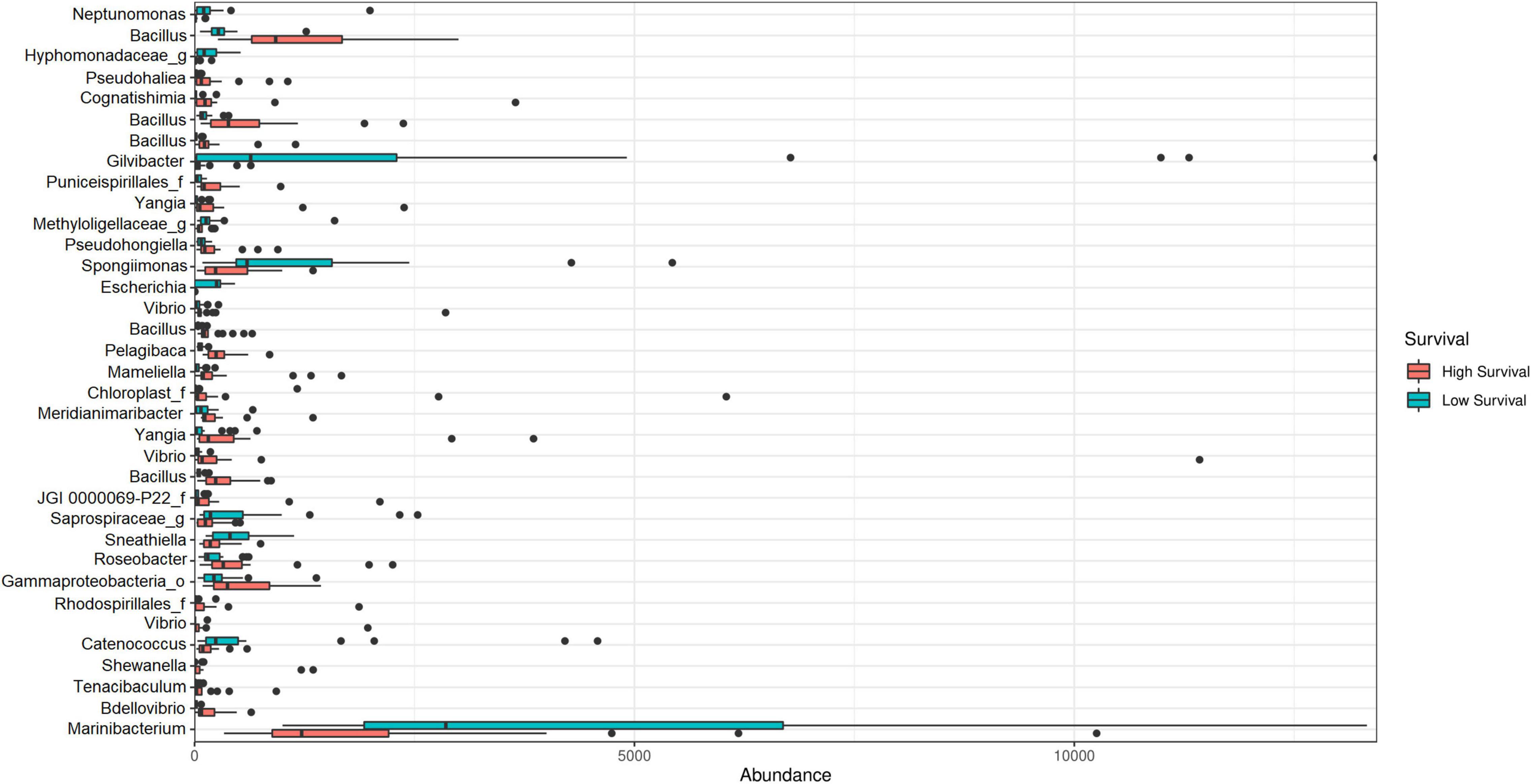
Figure 7. Absolute abundance of ASV biomarkers at the genus level at the high- and low-survival tanks affected with AHPND.
Discussion
Most studies on the P. vannamei microbiome have characterized the bacterial communities by comparing shrimp organs, diets, larval stages, culture systems, culture water and sediments, ecosystems, weight ranges, and stress conditions (Tzuc et al., 2014; Zhang M. et al., 2014; Zeng et al., 2017; Gainza et al., 2018; Huang et al., 2018; Zoqratt et al., 2018; Fan et al., 2019; Liu et al., 2019). Little study has been done on the shrimp microbiome under a sanitary approach, focusing primarily on juvenile stages (Chen et al., 2017; Cornejo-Granados et al., 2017). This study is part of a larger project that intends to discover effective probiotics for the control of AHPND in shrimp hatcheries. In this context, the main objectives of this study were to characterize the microbiome of P. vannamei larvae affected by AHPND in a commercial hatchery and to study the effect of the survival condition (high vs. low) on the microbiomes of larvae affected with AHPND to find biomarkers with the potential of being probiotics for a future AHPND control. For this reason, we did not use the control group (non-infected samples of larvae), as it would not indicate whether their microbiome would provide protection specifically for AHPND infection. The prevalence of AHPND was high and most tanks showed low survival at harvest. However, although a few AHPND-positive tanks with high survival at harvest were reported, a significant survival difference between the high- and low-survival tanks allowed the comparison of the microbiome between both the groups of samples.
We performed a description of the microbiome variability at four stages and compared two survival conditions. The Shannon diversity index was significantly lower at the low-survival tanks. ACE index at the M3 stage was significantly lower than the other stages at the low-survival tanks and lower than the same stage at the high-survival tanks. On the contrary, alpha-diversity indices were not different among stages at the high-survival tanks. Similar findings have been found by Xiong et al. (2018) and Wang Y. et al. (2020), who concluded that the success of a larval culture depends on the high bacterial diversity from the beginning of the host’s life. Also, the comparative analysis of the beta-diversity showed a significant difference in the microbiomes between both the survival conditions at M3, PL4, and PL7, but not at PL10. A plausible explanation for the difference in the microbiome structures at M3, PL4, and PL7 could be that the outbreak events occurred at the earlier stages, as larvae with symptoms of diseases were observed especially at PL4 and PL7. Samples collected at PL10, when the mortality stopped, could have been the survivors with a similar microbiome structure. Three top genera (Vibrio, Marinibacterium, and Gilvibacter) presented similar relative abundance in the high- and low-survival tanks at the end of the production cycle (PL10). However, Bacillus was less predominant in the low-survival tanks at PL10. In fact, Bacillus decreased over the time in the high-survival tanks. Another explanation could be that a different bacterial diversity is adhered to the dermis in the early larval stages, but through larval development, the digestive system is forming, the larvae are acquiring the necessary communities to their benefit and much of the dermal microbiome is lost, thus acquiring a more stable gut microbiome (Angthong et al., 2020; Wang H. et al., 2020; Wang Y. et al., 2020).
Pseudomonadota is the most abundant phylum reported for P. vannamei larvae and cultured water (Wang H. et al., 2020; Xue et al., 2020) for healthy and diseased larvae (Zheng et al., 2016). Pseudomonadota is also reported as abundant in P. vannamei juvenile shrimp (Xiong et al., 2015; Zeng et al., 2017) and wild and domesticated adult P. monodon (Rungrassamee et al., 2014). Bacteroidota is the second most abundant phylum reported for healthy larvae (Zheng et al., 2016) and juvenile P. vannamei (Wang et al., 2019) and water samples from healthy cultures (Xue et al., 2020). Bacillota is also reported but with a lower abundance in the P. vannamei larvae microbiome (Wang Y. et al., 2020) and healthy juvenile shrimp (Zeng et al., 2017; Wang et al., 2019; Restrepo et al., 2021). Our results are consistent with these observations of the highest dominance of Pseudomonadota at both the survival conditions, followed by a lower abundance of Bacteroidota and Bacillota. The Bacillota/Bacteroidota ratios of relative abundance were different between both the groups, with more than sevenfold higher ratio in the high-survival tanks (0.92) compared to the low ones (0.13) (Supplementary Table 3). The Bacillota/Bacteroidota ratio is an important index for several processes where the microbiota plays an important role in their host. Lower Bacillota/Bacteroidota ratios have been reported in the gut microbiome of P. vannamei with a low growth rate (0.06) compared to a normal (0.93) growth rate (Fan and Li, 2019). The latter ratio, which was similar to the value reported in this study for the high-survival tanks, has been explained by a better nutrient uptake in gut shrimp (Fan and Li, 2019). Bacillota could contribute to the energy storage of food by playing a role in the breakdown of polysaccharides. They have been reported in human and shrimp microbiota (Ley et al., 2006; Turnbaugh et al., 2006; Su et al., 2021). Bacillota and Bacteroidota also might contribute to the metabolism regulation to conserve energy during temperature changes, as higher ratios increase during temperature fluctuation. Bacillota is also one of the important bacterial groups for health and immunity in crustaceans (Duan et al., 2018; Foysal et al., 2019). Interestingly, we observed that the ratio Bacillota/Bacteroidota at the M3 stage was higher and exhibited more differences between the high (1.52) and low (0.09) survival tanks, which meant increase of 17-fold of the ratio Bacillota/Bacteroidota at the M3 stage at the high-survival tanks than the same stage at the low-survival tanks. Considering such a consistent pattern of decrease of the Bacillota/Bacteroidota ratio in the low-survival tanks, which was more intense at the M3 stage, it will be important to perform additional investigations to clarify the relation of both the taxa on shrimp health.
Rhodobacteraceae family is dominant and persistent in the digestive tract of juvenile and adult shrimp and adults. Therefore, it is considered vital in the search for probiotics (Xiong et al., 2018). We observed that Rhodobacteraceae was the dominant and ubiquitous family at all the larval stages. Specifically, the genera Yangia, Mameliella, Roseobacter, and Pelagibaca, which belong to this family, were significantly most enriched at the high-survival tanks. Mameliella is a genus involved in toxin degradation and production of metabolites, such as poly-β-hydroxybutyrate (PHB) that has beneficial effects on the immune response of P. vannamei (Duan et al., 2019, 2020). Roseobacter has been described as a suppressor of the growth of pathogenic vibrios in fish and its antibacterial effect against pathogens is enhanced by the presence of phytoplankton (Hjelm et al., 2004; Sharifah and Eguchi, 2011).
Some Vibrio species, such as V. harveyi, V. alginolyticus, and V. campbellii, are pathogenic for shrimp larvae (Karunasagar et al., 1994, 1998; Hameed, 1995; Robertson et al., 1998; Vandenberghe et al., 1998, 1999; Soto-Rodríguez et al., 2006; Haldar et al., 2011; Mirbakhsh et al., 2014; Hasan et al., 2017). Unexpectedly, the Vibrionaceae family was less abundant in larvae collected from the low-survival tanks. These results coincide with observations in diseased larvae (Zhang D. et al., 2014; Zheng et al., 2017) and AHPND-affected juvenile shrimp (Cornejo-Granados et al., 2017). Vibrio is the most important genus of the Vibrionaceae family in shrimp culture. It is ubiquitous in the marine environmental microbiome and, therefore, part of the shrimp culture. Moreover, Vibrio can be effective as a shrimp probiotic (Restrepo et al., 2021). Vibrio is abundant in nauplius and it is not considered a disease biomarker (Wang H. et al., 2020; Wang Y. et al., 2020). Consistently, three ASVs were enriched in the high-survival tanks (ASV-35, ASV-58, and ASV-164) and none in the low-survival tanks. In this study, we did not perform a reverse transcriptase quantitative PCR (RT-qPCR) analysis and, therefore, we could not quantify the expression of PirAB toxin genes. However, given the relevance of the AHPND control, it will important to investigate the relationship between expression of AHPND-causing bacteria and abundance and structure of other members of the Vibrionaceae family in the high- and low-survival tanks.
The Flavobacteriaceae family has been reported to be dominant in the microbiome of shrimp in metamorphosis (pre- and postlarvae) stages (Yan et al., 2020) and prevalent in culture water from zoea to early postlarvae stages (Xue et al., 2020). In this study, Flavobacteriaceae were more abundant at the earlier stages of M3 and PL4, especially at the low-survival tanks. After that, the abundances decreased reaching PL10. We found genera from this family at both the survival tanks meaning that the biomarker condition depends on the bacteria genus.
Bdellovibrio has been described to improve the growth and health of Scophthalmus maximus fish and P. monodon shrimp (Li et al., 2014; Cao et al., 2015). Also, it has the potential to reduce the biofilm formation of Vibrio (Guo et al., 2016), depredate pathogenic Vibrio of P. vannamei (Cao et al., 2020), and has been proposed as probiotics for P. vannamei shrimp (Kongrueng et al., 2017). Furthermore, in this study, an ASV assigned to the Bdellovibrio genus (ASV-112) was significantly more enriched in the high-survival tanks. Therefore, it is a potential candidate for shrimp probiotics, being necessary to resolve the taxonomic identification to a species level.
The Bacillus genus is widely described as an effective probiotic (Balcázar and Rojas-Luna, 2007; Tseng et al., 2009). Some Bacillus strains have significant inhibitory effects on pathogens and improve the resistance of P. vannamei shrimp in the presence of AHPND-causing bacteria (Kewcharoen and Srisapoome, 2019). Four ASVs found in the probiotic samples, taxonomically assigned as Bacillus, were also significantly enriched in the larva microbiota from the high-survival tanks. These ASVs were reported in three probiotic samples. The fourth probiotic did not show any ASVs significantly enriched in the high-survival tanks. The fact that the four Bacillus biomarkers from the probiotics were only detected in the high-survival tanks, despite the administration of probiotics at the same supplementation protocol in all the tanks, may suggest some synergic requirement of a bacteria consortium to obtain a successful performance.
The LEfSe analysis showed some genera and species that can be considered as biomarkers for improved survival after AHPND infection in shrimp hatcheries. However, biomarkers found in this study cannot be considered as healthy biomarkers as we did not perform a comparison with non-infected controls. Only 6 out of 64 tanks were AHPND negative and survival of these 6 tanks was low (32.2 ± 18.4%), indicating that they were not suitable as a control for non-infected successful tanks. Yangia pacifica, Mameliella alba, and Pelagibaca bermudensis, together with other two ASVs (ASV-20 and ASV-80), corresponding to the genera Roseobacter and Cognatishimia, belonging to the Rhodobacteraceae family, were significantly enriched in the high-survival tanks. On the other hand, the genus Gilvibacter has been reported as a biomarker associated at early stages (zoea 2) in P. vannamei larvae (Wang H. et al., 2020). In this study, this genus was significantly enriched in the low-survival tanks. To the best of our knowledge, there are no reports of the association of Gilvibacter with AHPND or any disease affecting P. vannamei. Therefore, it could be recommended to study this genus more closely.
The following evidence sufficiently supported the biomarkers associated with high and low survival in shrimp hatchery tanks: (a) larvae samples used for the microbiota comparison were collected from the two groups of tanks with a marked and significant difference in survival at harvest despite both the groups were AHPND positive, (b) alpha- and beta-diversity indices were significantly different between the high- and low-survival tanks, especially at M3 stage, and (c) Bacillota/Bacteroidota ratios were different between the high- and low-survival tanks. The survival levels reported at both the groups of tanks could depend on the presence or absence of the consortium of biomarkers shown by the LEfSe analysis. It suggests that the biomarkers found in the high-survival tanks could improve the performance of the production cycles. However, due to the high prevalence of AHPND in most tanks, the biomarkers identified for low survival also deserve special attention. This study constitutes the first characterization of the microbiome of the P. vannamei larval stages affected by AHPND in a commercial hatchery. The results provide new insights into the microbiome-host relations and contribute to the development of effective probiotics to prevent significant losses in AHPND-affected larvae. Most probiotics in shrimp culture are identified through in vitro antagonism screening, which overlooks many bacterial strains. Therefore, this analysis contributes with knowledge directed to different approaches of probiotic discovery based on the in vivo identification of prospective probiotics that include modes of action other than just antagonism. This is based on detecting the composition of the natural bacterial consortium present at high survival and the identification of their synergic interactions requirement to obtain a successful performance.
Data Availability Statement
The sequences from this study are available in the National Center for Biotechnology Information (NCBI) Sequence Read Archive (SRA) under accession number PRJNA800805.
Author Contributions
GR and BB contributed with conceptualization, methodology, validation, formal analysis, data curation, visualization, writing, and original draft preparation of this study. GR contributed with software. BB contributed with supervision and project administration. GR, BB, RR, BA, FP, IB, LS, and LT contributed with investigation. BB, LS, and LT contributed with resources and funding acquisition. All authors contributed with writing, reviewing, and editing.
Funding
This study was funded by the Corporación Ecuatoriana para el Desarrollo de la Investigación y la Academia (CEDIA) through its CEPRA program, project CEPRA XV-2021-05 BIOMARCADORES Descubrimiento de biomarcadores de potenciales probióticos para la industria camaronera ecuatoriana. GR conducted the study as part of his master’s thesis for the joint graduate program Red VLIR Ecuador and was supported by a grant from Secretaría Nacional de Educación Superior, Ciencia, Tecnología e Innovación (SENESCYT).
Conflict of Interest
The authors declare that the research was conducted in the absence of any commercial or financial relationships that could be construed as a potential conflict of interest.
Publisher’s Note
All claims expressed in this article are solely those of the authors and do not necessarily represent those of their affiliated organizations, or those of the publisher, the editors and the reviewers. Any product that may be evaluated in this article, or claim that may be made by its manufacturer, is not guaranteed or endorsed by the publisher.
Supplementary Material
The Supplementary Material for this article can be found online at: https://www.frontiersin.org/articles/10.3389/fmicb.2022.838640/full#supplementary-material
References
Angthong, P., Uengwetwanit, T., Arayamethakorn, S., Chaitongsakul, P., Karoonuthaisiri, N., and Rungrassamee, W. (2020). Bacterial analysis in the early developmental stages of the black tiger shrimp (Penaeus monodon). Sci. Rep. 10, 1–12. doi: 10.1038/s41598-020-61559-1
Balcázar, J. L., and Rojas-Luna, T. (2007). Inhibitory activity of probiotic Bacillus subtilis UTM 126 against Vibrio species confers protection against vibriosis in juvenile shrimp (Litopenaeus vannamei). Curr. Microbiol. 55, 409–412. doi: 10.1007/s00284-007-9000-0
Bell, T., and Lightner, D. (1988). A Handbook of Normal Penaeid Shrimp Histology. Baton Rouge, LA: The World Aquaculture Society.
Callahan, B. J., McMurdie, P. J., Rosen, M. J., Han, A. W., Johnson, A. J. A., and Holmes, S. P. (2016). DADA2: high-resolution sample inference from Illumina amplicon data. Nat. Methods 13, 581–583. doi: 10.1038/nmeth.3869
Cao, H., An, J., Zheng, W., and He, S. (2015). Vibrio cholerae pathogen from the freshwater-cultured whiteleg shrimp Penaeus vannamei and control with Bdellovibrio bacteriovorus. J. Invertebr. Pathol. 130, 13–20. doi: 10.1016/j.jip.2015.06.002
Cao, Q., Najnine, F., Han, H., Wu, B., and Cai, J. (2020). BALOs improved gut microbiota health in postlarval shrimp (Litopenaeus vannamei) after being subjected to salinity reduction treatment. Front. Microbiol. 0:1296. doi: 10.3389/FMICB.2020.01296
Cao, Y. (2020). microbiomeMarker: Microbiome Biomarker Analysis Toolkit. R package version 0.99.0. Available at: https://github.com/yiluheihei/microbiomeMarker (accessed July 5, 2021).
Chen, W. Y., Ng, T. H., Wu, J. H., Chen, J. W., and Wang, H. C. (2017). Microbiome dynamics in a shrimp grow-out pond with possible outbreak of acute hepatopancreatic necrosis disease. Sci. Rep. 7, 1–12. doi: 10.1038/s41598-017-09923-6
Cornejo-Granados, F., Lopez-Zavala, A. A., Gallardo-Becerra, L., Mendoza-Vargas, A., Sánchez, F., Vichido, R., et al. (2017). Microbiome of Pacific whiteleg shrimp reveals differential bacterial community composition between Wild, Aquacultured and AHPND/EMS outbreak conditions. Sci. Rep. 7, 1–15. doi: 10.1038/s41598-017-11805-w
Dai, W., Sheng, Z., Chen, J., and Xiong, J. (2020). Shrimp disease progression increases the gut bacterial network complexity and abundances of keystone taxa. Aquaculture 517:734802. doi: 10.1016/j.aquaculture.2019.734802
Dangtip, S., Sirikharin, R., Sanguanrut, P., Thitamadee, S., Sritunyalucksana, K., Taengchaiyaphum, S., et al. (2015). AP4 method for two-tube nested PCR detection of AHPND isolates of Vibrio parahaemolyticus. Aquac. Rep. 2, 158–162. doi: 10.1016/j.aqrep.2015.10.002
de la Peña, L., Cabillon, N., Catedral, D., Amar, E., Usero, R., Monotilla, W., et al. (2015). Acute hepatopancreatic necrosis disease (AHPND) outbreaks in Penaeus vannamei and P. monodon cultured in the Philippines. Dis. Aquat. Organ. 116, 251–254. doi: 10.3354/dao02919
Dhariwal, A., Chong, J., Habib, S., King, I. L., Agellon, L. B., and Xia, J. (2017). MicrobiomeAnalyst?: a web-based tool for comprehensive statistical, visual and meta-analysis of microbiome data. Nucleic Acids Res. 45, 180–188. doi: 10.1093/nar/gkx295
Dong, P., Guo, H., Wang, Y., Wang, R., Chen, H., Zhao, Y., et al. (2021). Gastrointestinal microbiota imbalance is triggered by the enrichment of Vibrio in subadult Litopenaeus vannamei with acute hepatopancreatic necrosis disease. Aquaculture 533:736199. doi: 10.1016/J.AQUACULTURE.2020.736199
Duan, Y., Liu, Q., Wang, Y., Zhang, J., and Xiong, D. (2018). Impairment of the intestine barrier function in Litopenaeus vannamei exposed to ammonia and nitrite stress. Fish Shellfish Immunol. 78, 279–288. doi: 10.1016/j.fsi.2018.04.050
Duan, Y., Wang, Y., Ding, X., Xiong, D., and Zhang, J. (2020). Response of intestine microbiota, digestion, and immunity in Pacific white shrimp Litopenaeus vannamei to dietary succinate. Aquaculture 517:734762. doi: 10.1016/J.AQUACULTURE.2019.734762
Duan, Y., Wang, Y., Liu, Q., Dong, H., Li, H., Xiong, D., et al. (2019). Changes in the intestine microbial, digestion and immunity of Litopenaeus vannamei in response to dietary resistant starch. Sci. Rep. 9, 1–10. doi: 10.1038/s41598-019-42939-8
Fan, J., Chen, L., Mai, G., Zhang, H., Yang, J., Deng, D., et al. (2019). Dynamics of the gut microbiota in developmental stages of Litopenaeus vannamei reveal its association with body weight. Sci. Rep. 9, 1–10. doi: 10.1038/s41598-018-37042-3
Fan, L., and Li, Q. X. (2019). Characteristics of intestinal microbiota in the Pacific white shrimp Litopenaeus vannamei differing growth performances in the marine cultured environment. Aquaculture 505, 450–461. doi: 10.1016/j.aquaculture.2019.02.075
Foysal, M. J., Fotedar, R., Tay, C.-Y., and Gupta, S. K. (2019). Dietary supplementation of black soldier fly (Hermetica illucens) meal modulates gut microbiota, innate immune response and health status of marron (Cherax cainii, Austin 2002) fed poultry-by product and fishmeal based diets. PeerJ 7:e6891. doi: 10.7717/peerj.68
Gainza, O., Ramírez, C., Ramos, A. S., and Romero, J. (2018). Intestinal microbiota of white shrimp Penaeus vannamei under intensive cultivation conditions in Ecuador. Microb. Ecol. 75, 562–568. doi: 10.1007/s00248-017-1066-z
Guo, Y., Yan, L., and Cai, J. (2016). Effects of Bdellovibrio and like organisms on survival and growth performance of juvenile turbot, Scophthalmus maximus. J. World Aquac. Soc. 47, 633–645. doi: 10.1111/jwas.12304
Haldar, S., Chatterjee, S., Sugimoto, N., Das, S., Chowdhury, N., Hinenoya, A., et al. (2011). Identification of Vibrio campbellii isolated from diseased farm-shrimps from south India and establishment of its pathogenic potential in an Artemia model. Microbiology 157, 179–188. doi: 10.1099/mic.0.041475-0
Hameed, A. S. S. (1995). Susceptibility of three penaeus species to a Vibrio campbellii-like Bacterium. J. World Aquac. Soc. 26, 315–319. doi: 10.1111/j.1749-7345.1995.tb00260.x
Han, J., Tang, K., Tran, L., and Lightner, D. (2015). Photorhabdus insect-related (Pir) toxin-like genes in a plasmid of Vibrio parahaemolyticus, the causative agent of acute hepatopancreatic necrosis disease (AHPND) of shrimp. Dis. Aquat. Organ. 113, 33–40. doi: 10.3354/dao02830
Hasan, M. A. R., Siddique, M. A., Hasan, M., Hossain, M. A., and Rahman, M. S. (2017). 16S rRNA gene sequence based identification of Vibrio spp. in shrimp and tilapia hatcheries of Bangladesh. Dhaka Univ. J. Biol. Sci. 26, 45–58. doi: 10.3329/dujbs.v26i1.46349
Hjelm, M., Bergh, Ø, Riaza, A., Nielsen, J., Melchiorsen, J., Jensen, S., et al. (2004). Selection and identification of autochthonous potential probiotic bacteria from turbot larvae (Scophthalmus maximus) rearing units. Syst. Appl. Microbiol. 27, 360–371. doi: 10.1078/0723-2020-00256
Huang, F., Pan, L., Song, M., Tian, C., and Gao, S. (2018). Microbiota assemblages of water, sediment, and intestine and their associations with environmental factors and shrimp physiological health. Appl. Microbiol. Biotechnol. 102, 8585–8598. doi: 10.1007/s00253-018-9229-5
Hughes, J. B., Hellmann, J. J., Ricketts, T. H., and Bohannan, J. M. (2001). Counting the uncountable: statistical approaches to estimating microbial diversity. Appl. Environ. Microbiol. 67, 4399–4406. doi: 10.1128/AEM.67.10.4399-4406.2001
Karunasagar, I., Otta, S. K., and Karunasagar, I. (1998). Disease problems affecting cultured penaeid shrimp in India. Fish Pathol. 33, 413–419. doi: 10.3147/jsfp.33.413
Karunasagar, I., Pai, R., Malathi, G. R., and Karunasagar, I. (1994). Mass mortality of Penaeus monodon larvae due to antibiotic-resistant Vibrio harveyi infection. Aquaculture 128, 203–209. doi: 10.1016/0044-8486(94)90309-3
Kerkhof, L., and Ward, B. B. (1993). Comparison of nucleic acid hybridization and fluorometry for measurement of the relationship between RNA/DNA ratio and growth rate in a marine bacterium. Appl. Environ. Microbiol. 59, 1303–1309. doi: 10.1128/aem.59.5.1303-1309.1993
Kewcharoen, W., and Srisapoome, P. (2019). Probiotic effects of Bacillus spp. from Pacific white shrimp (Litopenaeus vannamei) on water quality and shrimp growth, immune responses, and resistance to Vibrio parahaemolyticus (AHPND strains). Fish Shellfish Immunol. 94, 175–189. doi: 10.1016/j.fsi.2019.09.013
Kongrueng, J., Mitraparp-Arthorn, P., Bangpanwimon, K., Robins, W., Vuddhakul, V., and Mekalanos, J. (2017). Isolation of Bdellovibrio and like organisms and potential to reduce acute hepatopancreatic necrosis disease caused by Vibrio parahaemolyticus. Dis. Aquat. Organ. 124, 223–232. doi: 10.3354/DAO03120
Kongrueng, J., Tansila, N., Mitraparp-Arthorn, P., Nishibuchi, M., Vora, G. J., and Vuddhakul, V. (2015). LAMP assay to detect Vibrio parahaemolyticus causing acute hepatopancreatic necrosis disease in shrimp. Aquac. Int. 23, 1179–1188. doi: 10.1007/s10499-014-9874-3
Ley, R. E., Turnbaugh, P. J., Klein, S., and Gordon, J. I. (2006). Human gut microbes associated with obesity. Nature 444, 1022–1023. doi: 10.1038/4441022a
Li, H., Chen, C., Sun, Q., Liu, R., and Cai, J. (2014). Bdellovibrio and like organisms enhanced growth and survival of Penaeus monodon and altered bacterial community structures in its rearing water. Appl. Environ. Microbiol. 80, 6346–6354. doi: 10.1128/AEM.01737-14
Liu, J., Wang, K., Wang, Y., Chen, W., Jin, Z., Yao, Z., et al. (2019). Strain-specific changes in the gut microbiota profiles of the white shrimp Litopenaeus vannamei in response to cold stress. Aquaculture 503, 357–366. doi: 10.1016/j.aquaculture.2019.01.026
Mirbakhsh, M., Afsharnasab, M., Khanafari, A., and Razavi, M. R. (2014). Molecular identification of Vibrio harveyi from larval stage of Pacific white shrimp (Litopenaeus vannamei) Boone (Crustacea: Decapoda) by polymerase chain reaction and 16S rDNA sequencing. Iranian J. Fish. Sci. 13, 384–393.
Morey, R. D., and Rouder, J. N. (2011). Bayes factor approaches for testing interval null hypotheses. Psychol. Methods 16:406. doi: 10.1037/a0024377
Nearing, J. T., Douglas, G. M., Hayes, M., MacDonald, J., Desai, D., Allward, N., et al. (2021). Microbiome differential abundance methods produce disturbingly different results across 38 datasets. BioRxiv [Preprint]. doi: 10.1101/2021.05.10.443486
R Core Team (2013). R: A Language and Environment for Statistical Computing. Vienna: R Foundation for Statistical Computing.
Restrepo, L., Domínguez-Borbor, C., Bajaña, L., Betancourt, I., Rodríguez, J., Bayot, B., et al. (2021). Microbial community characterization of shrimp survivors to AHPND challenge test treated with an effective shrimp probiotic (Vibrio diabolicus). Microbiome 9, 1–20. doi: 10.1186/S40168-021-01043-8
Robertson, P. A. W., Calderon, J., Carrera, L., Stark, J. R., Zherdmant, M., and Austin, B. (1998). Experimental Vibrio harveyi infections in Penaeus vannamei larvae. Dis. Aquat. Organ. 32, 151–155. doi: 10.3354/dao032151
Rungrassamee, W., Klanchui, A., Maibunkaew, S., Chaiyapechara, S., Jiravanichpaisal, P., and Karoonuthaisiri, N. (2014). Characterization of intestinal bacteria in wild and domesticated adult black tiger shrimp (Penaeus monodon). PLoS One 9:e91853. doi: 10.1371/journal.pone.0091853
Segata, N., Izard, J., Waldron, L., Gevers, D., Miropolsky, L., Garrett, W. S., et al. (2011). Metagenomic biomarker discovery and explanation. Genome Biol. 12:R60. doi: 10.1186/gb-2011-12-6-r60
Shannon, C. E., and Weaver, W. (1949). The Mathematical Theory of Communication. Urbana, IL: University of Illinois Press.
Sharifah, E. N., and Eguchi, M. (2011). The phytoplankton Nannochloropsis oculata enhances the ability of Roseobacter clade bacteria to inhibit the growth of fish pathogen Vibrio anguillarum. PLoS One 6:e26756. doi: 10.1371/journal.pone.0026756
Sirikharin, R., Taengchaiyaphum, S., Sanguanrut, P., Chi, T. D., Mavichak, R., Proespraiwong, P., et al. (2015). Characterization and PCR detection of binary, pir-like toxins from vibrio parahaemolyticus isolates that cause acute hepatopancreatic necrosis disease (AHPND) in shrimp. PLoS One 10:e0126987. doi: 10.1371/journal.pone.0126987
Soto-Rodríguez, S. A., Simoes, N., Roque, A., and Gómez Gil, B. (2006). Pathogenicity and colonization of Litopenaeus vannamei larvae by luminescent vibrios. Aquaculture 258, 109–115. doi: 10.1016/j.aquaculture.2006.04.035
Su, C., Liu, X., Li, J., Zhang, M., Pan, L., Lu, Y., et al. (2021). Effects of bile acids on the growth performance, lipid metabolism, non-specific immunity and intestinal microbiota of Pacific white shrimp (Litopenaeus vannamei). Aquac. Nutr. [Epub ahead of print]. doi: 10.1111/anu.13338
Takahashi, S., Tomita, J., Nishioka, K., Hisada, T., and Nishijima, M. (2014). Development of a prokaryotic universal primer for simultaneous analysis of bacteria and archaea using next-generation sequencing. PLoS One 9:105592. doi: 10.1371/JOURNAL.PONE.0105592
Tran, L., Nunan, L., Redman, R., Mohney, L., Pantoja, C., Fitzsimmons, K., et al. (2013). Determination of the infectious nature of the agent of acute hepatopancreatic necrosis syndrome affecting penaeid shrimp. Dis. Aquat. Organ. 105, 45–55. doi: 10.3354/dao02621
Tseng, D. Y., Ho, P. L., Huang, S. Y., Cheng, S. C., Shiu, Y. L., Chiu, C. S., et al. (2009). Enhancement of immunity and disease resistance in the white shrimp, Litopenaeus vannamei, by the probiotic, Bacillus subtilis E20. Fish Shellfish Immunol. 26, 339–344. doi: 10.1016/j.fsi.2008.12.003
Turnbaugh, P. J., Ley, R. E., Mahowald, M. A., Magrini, V., Mardis, E. R., and Gordon, J. I. (2006). An obesity-associated gut microbiome with increased capacity for energy harvest. Nature 444, 1027–1031. doi: 10.1038/nature05414
Tzuc, J. T., Escalante, D. R., Rojas Herrera, R., Gaxiola Cortes, G., and Ortiz, M. L. A. (2014). Microbiota from Litopenaeus vannamei: digestive tract microbial community of Pacific white shrimp (Litopenaeus vannamei). SpringerPlus 3, 1–10. doi: 10.1186/2193-1801-3-280
van Doorn, J., van den Bergh, D., Böhm, U., Dablander, F., Derks, K., Draws, T., et al. (2021). The JASP guidelines for conducting and reporting a Bayesian analysis. Psychon. Bull. Rev. 28, 813–826. doi: 10.3758/s13423-020-01798-5
Vandenberghe, J., Li, Y., Verdonck, L., Li, J., Sorgeloos, P., Xu, H. S., et al. (1998). Vibrios associated with Penaeus chinensis (Crustacea: Decapoda) larvae in Chinese shrimp hatcheries. Aquaculture 169, 121–132. doi: 10.1016/S0044-8486(98)00319-6
Vandenberghe, J., Verdonck, L., Robles-Arozarena, R., Rivera, G., Bolland, A., Balladares, M., et al. (1999). Vibrios associated with Litopenaeus vannamei larvae, postlarvae, broodstock, and hatchery probionts. J. Appl. Environ. Microbiol. 65, 2592–2597. doi: 10.1128/AEM.65.6.2592-2597.1999
Wallen, Z. D. (2021). Comparison study of differential abundance testing methods using two large Parkinson disease gut microbiome datasets derived from 16S amplicon sequencing. BMC Bioinformatics 22:265.
Wang, H., Huang, J., Wang, P., and Li, T. (2020). Insights into the microbiota of larval and postlarval Pacific white shrimp (Penaeus vannamei) along early developmental stages: a case in pond level. Mol. Genet. Genomics 295, 1517–1528. doi: 10.1007/s00438-020-01717-2
Wang, J., Huang, Y., Xu, K., Zhang, X., Sun, H., Fan, L., et al. (2019). White spot syndrome virus (WSSV) infection impacts intestinal microbiota composition and function in Litopenaeus vannamei. Fish Shellfish Immunol. 84, 130–137. doi: 10.1016/j.fsi.2018.09.076
Wang, Y., Wang, K., Huang, L., Dong, P., Wang, S., Chen, H., et al. (2020). Fine-scale succession patterns and assembly mechanisms of bacterial community of Litopenaeus vannamei larvae across the developmental cycle. Microbiome 8, 1–16. doi: 10.1186/s40168-020-00879-w
Xiong, J., Dai, W., Qiu, Q., Zhu, J., Yang, W., and Li, C. (2018). Response of host–bacterial colonization in shrimp to developmental stage, environment and disease. Mol. Ecol. 27, 3686–3699. doi: 10.1111/mec.14822
Xiong, J., Wang, K., Wu, J., Qiuqian, L., Yang, K., Qian, Y., et al. (2015). Changes in intestinal bacterial communities are closely associated with shrimp disease severity. Appl. Microbiol. Biotechnol. 99, 6911–6919.
Xue, M., He, Y., Chen, D., Wang, L., Liang, H., Liu, J., et al. (2020). Temporal dynamics of aquatic microbiota and their correlation with environmental factors during larviculture of the shrimp Litopenaeus vannamei. Aquaculture 529:735605. doi: 10.1016/j.aquaculture.2020.735605
Xue, M., Liang, H., He, Y., and Wen, C. (2015). Characterization and in-vivo evaluation of potential probiotics of the bacterial flora within the water column of a healthy shrimp larviculture system. Chinese J. Oceanol. Limnol. 34, 484–491. doi: 10.1007/S00343-016-5024-2
Yan, M., Zhang, X., Hu, L., Huang, X., Zhou, Q., Zeng, G., et al. (2020). Bacterial community dynamics during nursery rearing of Pacific white shrimp (Litopenaeus vannamei) revealed via high-throughput sequencing. Indian J. Microbiol. 60, 214–221. doi: 10.1007/s12088-019-00853-7
Zeng, S., Huang, Z., Hou, D., Liu, J., Weng, S., and He, J. (2017). Composition, diversity and function of intestinal microbiota in pacific white shrimp (Litopenaeus vannamei) at different culture stages. PeerJ 5:e3986.
Zhang, D., Wang, X., Xiong, J., Zhu, J., Wang, Y., Zhao, Q., et al. (2014). Bacterioplankton assemblages as biological indicators of shrimp health status. Ecol. Indic. 38, 218–224. doi: 10.1016/j.ecolind.2013.11.002
Zhang, M., Sun, Y., Chen, K., Yu, N., Zhou, Z., Chen, L., et al. (2014). Characterization of the intestinal microbiota in Pacific white shrimp, Litopenaeus vannamei, fed diets with different lipid sources. Aquaculture 434, 449–455. doi: 10.1016/j.aquaculture.2014.09.008
Zheng, Y., Yu, M., Liu, J., Qiao, Y., Wang, L., Li, Z., et al. (2017). Bacterial community associated with healthy and diseased Pacific white shrimp (Litopenaeus vannamei) larvae and rearing water across different growth stages. Front. Microbiol. 8:1362. doi: 10.3389/fmicb.2017.01362
Zheng, Y., Yu, M., Liu, Y., Su, Y., Xu, T., Yu, M., et al. (2016). Comparison of cultivable bacterial communities associated with Pacific white shrimp (Litopenaeus vannamei) larvae at different health statuses and growth stages. Aquaculture 451, 163–169. doi: 10.1016/j.aquaculture.2015.09.020
Keywords: AHPND, biomarkers, differential abundance, microbiome, Penaeus vannamei larvae, 16S rRNA gene
Citation: Reyes G, Betancourt I, Andrade B, Panchana F, Román R, Sorroza L, Trujillo LE and Bayot B (2022) Microbiome of Penaeus vannamei Larvae and Potential Biomarkers Associated With High and Low Survival in Shrimp Hatchery Tanks Affected by Acute Hepatopancreatic Necrosis Disease. Front. Microbiol. 13:838640. doi: 10.3389/fmicb.2022.838640
Received: 18 December 2021; Accepted: 21 March 2022;
Published: 09 May 2022.
Edited by:
Catarina Magalhães, University of Porto, PortugalReviewed by:
Alejandra Prieto-Davó, Universidad Nacional Autónoma de México, MexicoZheng Sun, Brigham and Women’s Hospital and Harvard Medical School, United States
Copyright © 2022 Reyes, Betancourt, Andrade, Panchana, Román, Sorroza, Trujillo and Bayot. This is an open-access article distributed under the terms of the Creative Commons Attribution License (CC BY). The use, distribution or reproduction in other forums is permitted, provided the original author(s) and the copyright owner(s) are credited and that the original publication in this journal is cited, in accordance with accepted academic practice. No use, distribution or reproduction is permitted which does not comply with these terms.
*Correspondence: Guillermo Reyes, guianrey@espol.edu.ec; Bonny Bayot, bbayot@espol.edu.ec