- 1Laboratório de Biologia Molecular Aplicada, Instituto Biológico, São Paulo, Brazil
- 2Escola Superior de Agricultura Luiz de Queiroz (ESALQ), Universidade de São Paulo, Piracicaba, Brazil
- 3Centro de Citricultura Sylvio Moreira, Cordeirópolis, Brazil
- 4Embrapa Mandioca e Fruticultura, Cruz das Almas, Brazil
Plant viruses transmitted by mites of the genus Brevipalpus are members of the genera Cilevirus, family Kitaviridae, or Dichorhavirus, family Rhabdoviridae. They produce non-systemic infections that typically display necrotic and/or chlorotic lesions around the inoculation loci. The cilevirus citrus leprosis virus C (CiLV-C) causes citrus leprosis, rated as one of the most destructive diseases affecting this crop in the Americas. CiLV-C is vectored in a persistent manner by the flat mite Brevipalpus yothersi. Upon the ingestion of viral particles with the content of the infected plant cell, virions must pass through the midgut epithelium and the anterior podocephalic gland of the mites. Following the duct from this gland, virions reach the salivary canal before their inoculation into a new plant cell through the stylet canal. It is still unclear whether CiLV-C multiplies in mite cells and what mechanisms contribute to its movement through mite tissues. In this study, based on direct observation of histological sections from viruliferous mites using the transmission electron microscope, we posit the hypothesis of the paracellular movement of CiLV-C in mites which may involve the manipulation of septate junctions. We detail the presence of viral particles aligned in the intercellular spaces between cells and the gastrovascular system of Brevipalpus mites. Accordingly, we propose putative genes that could control either active or passive paracellular circulation of viral particles inside the mites.
Introduction
Plant diseases caused by Brevipalpus-transmitted viruses (BTV) result in non-systemic infections that produce local necrotic and chlorotic lesions on leaves, stems, and fruits (Kitajima et al., 2003, 2010). Early studies based on ultrastructural analyses of BTV-infected tissues revealed two types of viruses which were further recognized as BTV-Cytoplasmic and -Nuclear types (Kitajima et al., 2003). BTV-C and -N have contrasting molecular biology but they still display some common features suggesting a possible convergent evolution (Freitas-Astúa et al., 2018).
The infection by BTV-N induces the formation of electron-lucent viroplasms in the nucleus. Virions are naked, short rod-like particles (40 nm × 100-110 nm) that accumulate both in the nucleus and the cytoplasm of plant and mite cells (Freitas-Astúa et al., 2018; Figure 1A). The genomes of BTV-N comprise two ssRNA molecules (∼ 6 kb each) of negative sense with six open reading frames (Dietzgen et al., 2018). Five BTV-N have been molecularly characterized and are definitive members of the genus Dichorhavirus, family Rhabdoviridae (Dietzgen et al., 2018; Amarasinghe et al., 2019; Kuhn et al., 2020).
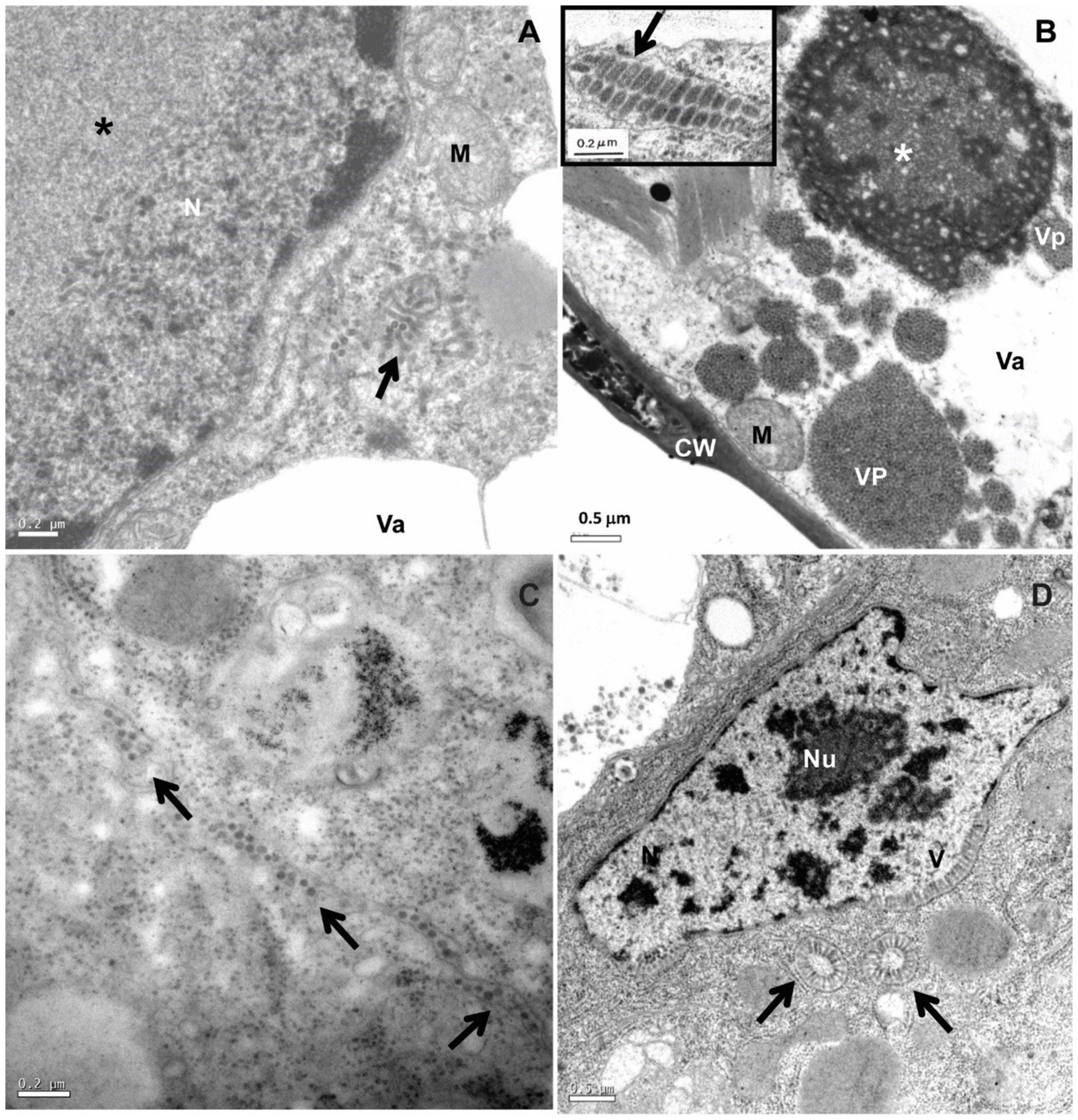
Figure 1. Transmission electron micrographs of BTV-infected leaf lesion cell and mite vector Brevipalpus yothersi tissues. (A) Clerodendrum thomsonae infected by the dichorhavirus clerodendrum chlorotic spot virus (ClCSV), with electron lucent viroplasm (*) and short, rod-like particles (arrow) in the cytoplasm. (B) Ligustrum sinense infected with the cilevirus passion fruit green spot virus (PfGSV) that induces large electron dense and vacuolated viroplasm (*) in the cytoplasm; short bacilliform virions (VP) are present within endoplasmic reticulum cisternae (insert). (C) Sections from adults Brevipalpus yothersi collected from L. sinense infected with PfGSV showing virus particles (arrows) in the extracellular space. (D) Sections from adults Brevipalpus yothersi collected from C. thomsonae, infected by ClCSV showing evidences of viral replication in their tissues, large nuclei of the anterior podocephalic gland cell, with virus-like particles (V) at the nuclear periphery. These particles are also present in the cytoplasm, forming the spoke wheel configuration (arrows). C, chloroplast; CW, cell wall; M, mitochondrion; N, nucleus; Va, vacuole; Nu, nucleolus.
Virions of the BTV-C type are short, enveloped bacilliform particles of 70-80 nm wide and 100-120 nm long. Virus particles are commonly found inside cisternae of the endoplasmic reticulum, and form electron-dense, vacuolated inclusions (viroplasms) in the infected plant cell cytoplasm (Figure 1B). BTV-C genomes consist of two (+) sense single-stranded (ss) RNA molecules of ∼5 and 9 kb. They are assigned to the genus Cilevirus, family Kitaviridae (Freitas-Astúa et al., 2018; Quito-Avila et al., 2021). Citrus leprosis virus C (CiLV-C) is the best-characterized cilevirus at both molecular and epidemiological levels (Chabi-Jesus et al., 2021).
Besides the genus Cilevirus, the family Kitaviridae comprises other two plant-infecting virus genera: Higrevirus and Blunervirus (Quito-Avila et al., 2021). Overall, kitaviruses show a heterogeneous genome organization and share a phylogenetic relationship with arthropod-infecting viruses of the groups negevirus (including nelorpiviruses and sandewaviruses), centivirus, and aphiglyvirus (Kondo et al., 2020; Ramos-González et al., 2020).
Few species of Brevipalpus are known as vectors of cileviruses and dichorhaviruses (de Lillo et al., 2021). Dichorhaviruses are transmitted in a circulative propagative manner, whereas cileviruses are transmitted in a circulative manner, but whether cileviruses replicate in the mites is still unclear (Roy et al., 2015; Tassi et al., 2017). Little is known about the mode of transmission of negeviruses and kitaviruses other than cileviruses. The isolation of Okushiri virus from mosquito larvae suggests the vertical transmission of negeviruses (Vasilakis et al., 2013; Carapeta et al., 2015; O’Brien et al., 2017). The expansion of perinuclear spaces filled with vesicles or microtubules, sometimes in paracrystalline arrays, and the accumulation of cytoplasmic vacuoles similar to those detected during the alphavirus infection, are indicators of the multiplication of the sandewavirus Tanay virus in C6/36 mosquito cells (Vasilakis et al., 2013; Zhao et al., 2019). Brevipalpus mites have been associated with the transmission of hibiscus green spot virus 2, genus Higrevirus, and the cilevirus-like hibiscus yellow blotch virus (Melzer et al., 2013; Olmedo-Velarde et al., 2021).
Brevipalpus Mites as Viral Vectors
The genus Brevipalpus groups almost 300 valid species (Castro et al., 2020) of obligatory phytophagous, mostly polyphagous red-brownish mites, which are distributed across the subtropical and equatorial regions of the world. Brevipalpus mites are flattened, of approximately 0.3 mm long, move slowly, and display five developmental phases, i.e., egg, larvae, protonymph, deutonymph, and adult (Welbourn et al., 2003; Alberti and Kitajima, 2014; Tassi et al., 2017; Dietzgen et al., 2018).
Data on Brevipalpus-dichorhavirus relationships are almost limited to studies derived from the pathosystem orchid fleck virus (OFV)-B. californicus (Kondo et al., 2003). Upon acquisition, OFV transmission has a latent period of three weeks, the inoculation access period is approximately 30 min, and viral retention in mites occurs for at least three weeks (Kondo et al., 2003). Nymphs and adults, but not the larvae, have vector activity, suggesting a persistent propagative type of transmission (Kondo et al., 2003).
Different species of Brevipalpus colonizing dichorhavirus-infected plants exhibit electron-lucent viroplasms in the nucleus, and short rod-like particles in both the nucleus and the cytoplasm of midgut and anterior podocephalic gland cells (Alberti and Kitajima, 2014; Ramos-González et al., 2018). In the nucleus, viral particles may appear dispersed within nucleoplasm or viroplasm and arranged perpendicularly onto the inner membrane of the nuclear envelope (Figure 1). In the cytoplasm, they are commonly seen associated with endoplasmic reticulum membranes, occasionally radially arranged, forming the so-called “spoke wheel” configuration (Figure 1). The accumulation patterns of viral particles in viruliferous mites are essentially similar to those observed in dichorhavirus-infected plant cells, suggesting that dichorhaviruses replicate in the mite (Kitajima et al., 2003). No accumulation of particles is observed between adjacent cells of dichorhavirus-infected mites.
All the active life stages of B. yothersi can transmit the cilevirus CiLV-C, but no transovarial passage occurs (Chiavegato, 1996; Tassi et al., 2017). Using common bean (Phaseolus vulgaris) as indicator plant (Garita et al., 2013), CiLV-C acquisition and inoculation access periods by B. yothersi are 4- and 2 h, respectively, with a latent period of 7 h (Tassi et al., 2017). Once the virus is acquired, B. yothersi remains viruliferous for at least 20 days (Tassi et al., unpublished). Viral particles are consistently found between adjacent cells at the basal part of the caeca and in the anterior podocephalic gland of mites (Figure 1C; Alberti and Kitajima, 2014). The load of viral particles in the intercellular spaces seems to increase proportionally with the number of acquisitions (Tassi et al., 2017). Frequently, lines of viral particles are interrupted by septate junctions (Figures 2B,C). Differently from what is easily seen in plant cells infected by CiLV-C, viroplasms are not observed in CiLV-C viruliferous mites. To improve the viral identification, in situ immunogold labeling using polyclonal antibodies against the P29 protein of CiLV-C (Calegario et al., 2013) was carried out as previously described for plant tissues (Calegario et al., 2013) and Brevipalpus mites (Alberti and Kitajima, 2014). Sets of virion particles aligned up to 10 μm in length were detected in paracellular spaces of Brevipalpus mites that fed on CiLV-C-infected oranges (Figure 2).
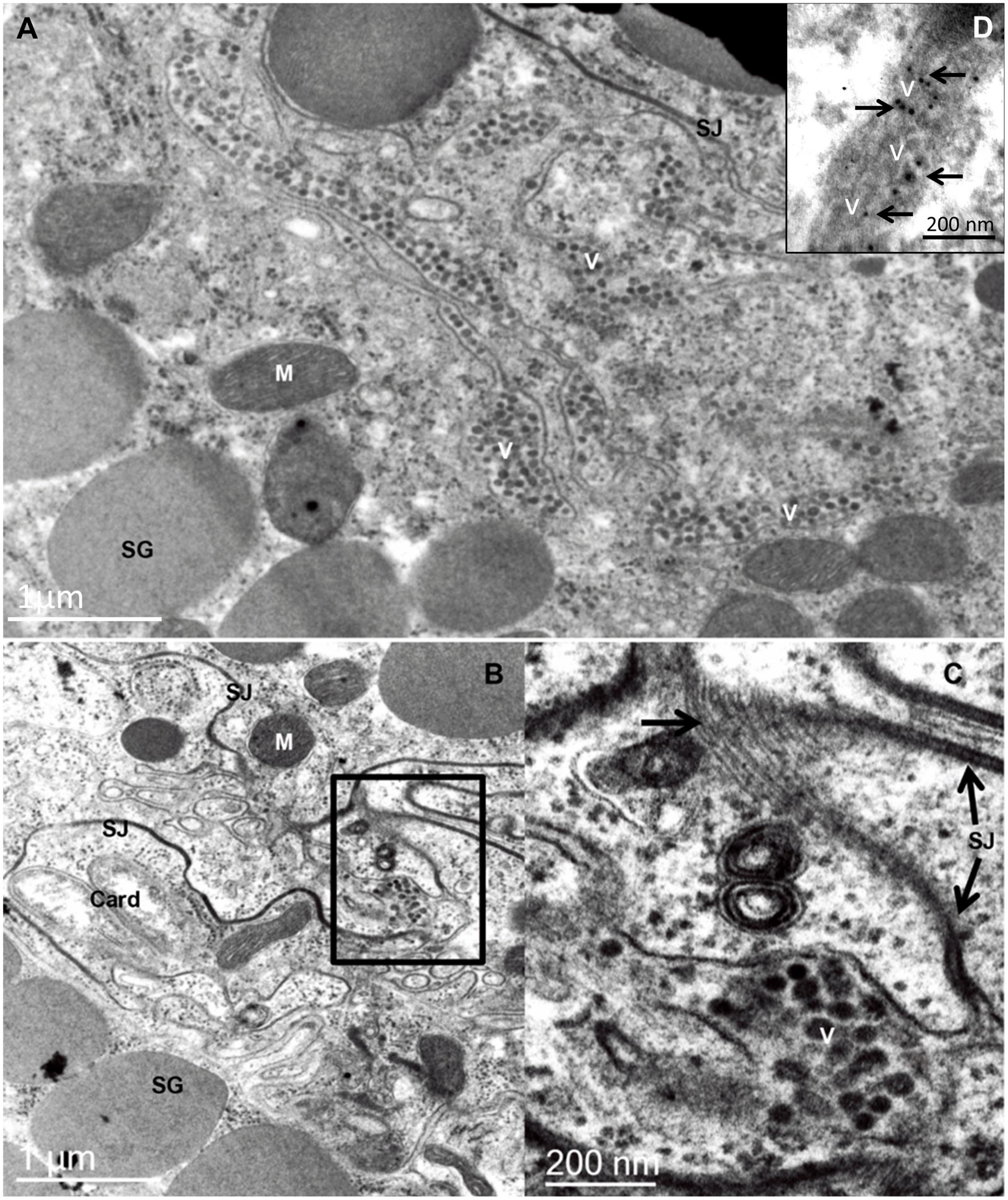
Figure 2. Transmission electron micrographs of sections of the prosomal region of an adult female Brevipalpus yothersi, viruliferous for citrus leprosis virus C (CiLV-C). (A) Basal part of midgut caeca, showing several rows of virions (V), aligned in the extracellular space formed by four layers of cells. It is presumed that once internalized, crossing the midgut epithelial cell barrier, these particles move passively in the direction of the anterior podocephalic gland (= salivary gland) following the celomic flux, where they will reach the stylet channel, after overtaking the gland cell barrier. (B) An area of the branched ceaca, revealing a labyrinth of membranes running between adjacent cells. A small group of virions (V) is present in one of these intercellular spaces. (C) An enlarged region of figure B in which septate junctions (SJ) are well depicted. The large arrow points to a tangential section through a septate junction, revealing the rows of intermembrane proteins. (D) in situ immunolabeling using anti-p29 polyclonal antibody in aldehyde-fixed and LRWhite embedded B. yothersi viruliferous for CiLV-C. Card, bacterial endosymbiont Cardinium; M, mitochondrion; SG, secretion granules.
The detection of anti-genomic (complementary strand) RNA of the cileviruses CiLV-C and CiLV-C2 in viruliferous B. yothersi was considered evidence of cilevirus replication within the vector (Roy et al., 2015). However, anti-genomic RNA molecules of CiLV-C may have arisen in the mite body upon feeding on infected plants or may have been generated as a result of self-primed genomic molecules during the RT-PCR detection. Therefore, further assays, including new controls, a time-course experiment, and the search for putative replication sites in specific mite tissues not yet visualized by transmission electron microscopy are ongoing (Tassi et al., unpublished).
Cileviruses Movement Within Their Mite Vectors: A Critical Evaluation of the Alternatives
Transcytosis in Circulative Non-propagative Viruses
In addition to whether cileviruses replicate in their mite vectors or not, the mechanisms that promote virion internalization, movement, and their release into the stylet canal also remain uncertain. Transcytosis is a cellular mechanism in which extracellular materials, enclosed in vesicles generated by endocytosis, move across the cell and eject the content in the distal section of the cells by exocytosis (Whitfield et al., 2015). Transcytosis has been also described as a form of circulation of plant viruses in their vectors, but the underlying mechanisms are not fully elucidated (Brault et al., 2007; Hogenhout et al., 2008; Blanc et al., 2014; Di Mattia et al., 2020). The internalization in the vector body of several viruses of the families Luteoviridae, Geminiviridae, and Nanoviridae occurs without replication of the viral genome. Virus particles are transported across cells into membrane vesicles, preventing any contact between viruses and the insect cell cytoplasm in the epithelia of the gut and salivary gland (Brault et al., 2007; Hogenhout et al., 2008; Blanc et al., 2014). The vesicles formed during transcytosis seemingly follow the early endosomal pathway before the appearance of non-coated tubular vesicles. Inside these vesicles, virions likely reach the basal membrane and exit the gut cells into the hemolymph (Ali et al., 2018). A transcytosis process is also observed when luteovirids cross the cellular barrier of the accessory salivary gland (Brault et al., 2007). For cileviruses, however, although transcytosis should not be completely disregarded, it seems an unlikely route of circulation in Brevipalpus mites. Viral particles have been observed neither inside cells of the anterior midgut epithelium nor in cells of anterior and dorsal podocephalic glands of mites feeding on cilevirus-infected plants (Alberti and Kitajima, 2014).
It is important to notice that anatomic differences between Brevipalpus mites and insects may account for the nonexistence of transcytosis in these mites. During feeding, Brevipalpus mites use stylets to perforate the epidermal layer of plant organs and reach the underlying parenchymal cell content after punctuating its wall and membrane. Saliva produced by the anterior podocephalic gland is injected to pre-digest the cellular content (Alberti and Kitajima, 2014). The ingested material then follows to the esophagus that crosses the synganglion and ends into the anterior midgut through a small valve, the ventriculus, which consists of a small lumen and the highly branched caeca (Alberti and Kitajima, 2014). The caeca, formed by large epithelial cells, extend both to anterior and posterior parts of the mite body, occupying every space among the organs, producing a complex labyrinth of cell membranes and intercellular spaces, many of which are joined by septate junctions (Figures 2A-C), leaving the hemolymph confined to small and restricted cavities. This complex of cells comprises the so-called gastrovascular system which may directly irrigate several organs with digested nutrients and, probably, virus particles in viruliferous mites (Alberti and Kitajima, 2014). Brevipalpus mites lack a pulsating organ, so the circulation of the hemolymph depends on the movement generated by their muscles and internal organs, diverging from insects that have a circulatory system and, therefore, a more active circulation of nutrients and other fluids (Alberti and Coons, 1999).
Paracellular Route of CiLV-C in Brevipalpus yothersi: A Hypothesis
The persistent circulatory transmission of cileviruses by Brevipalpus mites poses a challenge to explain how the cilevirus movement occurs. A raising question is how virions get access from the midgut lumen to the hemolymph space, and later to the stylet channel, since two epithelial barriers hamper it: the midgut (caeca) and the anterior podocephalic gland. Ultrastructural observations of viruliferous B. yothersi mites feeding on CiLV-C-infected plants reveal the presence of viral particles between cells (Alberti and Kitajima, 2014), which led us to ponder the existence of a paracellular pathway of virion movement within its vector.
In the epithelium of invertebrates, occluding structures named septate junctions (SJ) act as a barrier that separates distinct compartments, limiting the paracellular passage of fluids (Izumi and Furuse, 2014). Unlike tight junction (TJ), the structure sealing the apical part of epithelial cells in the vertebrates, the SJ forms circumferential belts around the apicolateral regions of the cells. Visually, they appear as a ladder-like septum, with 15-20 nm of spacing (Izumi and Furuse, 2014). Studies of the morphophysiology of the SJ in the fly Drosophila melanogaster exposed two types of SJ, i.e., the pleated septate junctions (pSJ), present in ectodermal-derived epithelium, i.e., epidermis, fore- and hindgut, salivary gland, etc., and the smooth septate junctions (sSJ), which occurs in the endodermal-derived epithelium, i.e., midgut, gastric caeca (Izumi and Furuse, 2014; Hall and Ward, 2016). Mutations of the SJ proteins may be lethal or produce functionally deficient junctions, but the exact mechanisms underlying the transient opening of SJ have not been described. To our knowledge, there are no reports of arthropod viruses interacting with tight or septate junctions.
In vertebrates, TJs could act as physical barriers from the innate immune defense system, especially on the respiratory tract. The coordination of TJ opening is mediated by chemical signals and membrane receptors, as happens during the paracellular passage of lymphocytes through the walls of capillary vessels (Yonekawa and Harlan, 2005; Yumine et al., 2019). In humans, the permeability of TJ between cells of the wall of the digestive tract is mediated by zonulin, a pre-haptoglin protein, and by gliadin, a component of gluten, in patients suffering from celiac disease (Fasano, 2012). Similarly, to replicate or transit through epithelia, viruses take advantage of the structural proteins that form the TJ and adherent junctions (AJ) as their receptors (Mateo et al., 2015). Viruses evolved selecting strategies that could counter the antiviral function of TJs, by degradation processes, e.g., it is suggested that mosquito-borne viruses like west nile virus and Japanese encephalitis virus establish infections in vertebrate hosts by degrading TJ molecules to disrupt the epithelial barriers (Medigeshi et al., 2009; Yumine et al., 2019).
The gastrovascular system of Brevipalpus mites, composed of the highly branched caeca that fill all spaces left by other tissues on the hemolymph, may serve as a pathway to the circulation of viruses inside the mites.
Based on our transmission electron microscopy studies following the methodology proposed by Alberti and Kitajima (2014), observations of the accumulation of virus particles between cells and the possible role of SJ limiting or coordinating the circulation of viruses to new tissues, we pose alternative hypotheses to explain virus-gastrovascular system interaction including either passive or active mechanisms. The passive interaction involves the SJs transient openings which allow the transport of nutrients between cells. Following the flow, the virus could reach the spaces between cells, leading to a circulation of virus particles within the hemolymph. Alternatively, virus-encoded proteins could recognize the SJs components and induce transient openings, allowing the passage of viruses in an active process, as seen in some vertebrates-infecting viruses, or even in other physiological or pathological processes, i.e., zonulin and gliadin-like interaction (Figure 3). The viral protein P61, a putative membrane glycoprotein, or P24, a putative viral structural protein, might trigger a transient local opening of the SJ, ensuing the paracellular traffic of cileviruses in Brevipalpus mites.
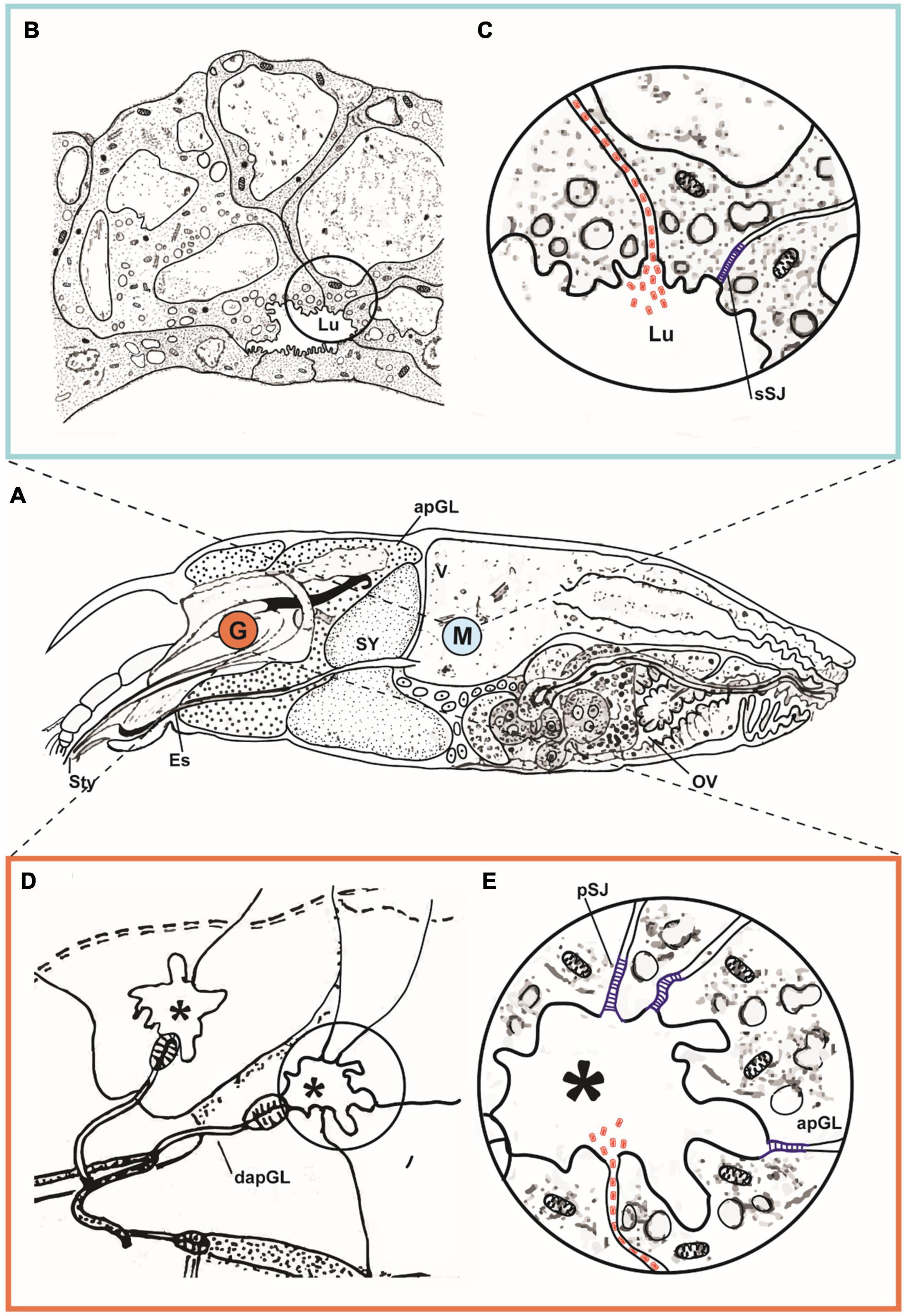
Figure 3. Hypothetical pathway of cileviruses circulating within the mite vector. (A) Schematic representation of sagittal section of an adult female of Brevipalpus yothersi, revealing details of its internal anatomy [stylet complex, prosomal glands, synganglion, digestive tract (ventriculus, caeca), ovary]. Details of ventriculus (V) and anterior podocephalic gland (apGL) are shown indicated in the marked area M and detailed in (B,C). Portion of the anterior podocephalic gland (equivalent to salivary gland) marked by G, is detailed in (D,E). (B) Part of ventriculum (V) exhibiting epithelial cells of caeca with small lumen (Lu). In the detail (C), hypothetical transitional opening of the smooth septate junction (sSJ) at the apical part of the epithelial cell, induced by the presence of viral particles represented in red. Virions gain access to the intercellular space (Lu), being carried out by the celomic flux, to the apical part of the anterior podocephalic gland. (D) Schematic drawing of collecting reservoir (*) of the apGL secretions. (E) Detail depicted in (D). Glandular cells are held together by pleated septate junctions (pSJ) represented in blue, which hypothetically open due to the presence of viral particles (in red) arriving by the intercellular space, releasing them into the reservoir (*), and subsequently to the stylet channel. dapGL, duct of the anterior podocephalic gland (adapted from Alberti and Kitajima, 2014).
Orthologue Proteins of SJ and sSJ Factors in Mites
The involvement of TJs and AJs in cell-to-cell viral movement and their role as receptors have been reported for human viruses (Cifuentes-Munoz et al., 2020), but there is no information on viruses using this route in arthropods. In humans, for instance, after primary infection of the respiratory airway, measles virus, family Paramyxoviridae, spreads laterally into the epithelium via AJs (Mühlebach et al., 2011; Nakatsu et al., 2013). Besides, human TJ components act as viral receptors, e.g., the glycoprotein of hepatitis C virus (HCV) uses occludin and claudin as co-receptors to enter hepatocytes (Reynolds et al., 2008; Timpe et al., 2008; Brimacombe et al., 2011; Yumine et al., 2019), the coxsackieviruses (positive-strand RNA viruses) and adenoviruses (double-stranded DNA viruses), although exploring different strategies, target the integral chimeric antigen receptor (CAR) protein associated with TJ (Bergelson et al., 1997; Bergelson, 2009; Mateo et al., 2015), reoviruses use the TJ protein junctional adhesion molecule A (JAM-A) as receptor (Barton et al., 2001), claudin 1 is involved on dengue virus entry by the interaction of the viral protein prM and TJ component (Che et al., 2013).
Proteins of the family LY6_uPAR, also called three-finger proteins (TFP), are found associated with the membrane by a glycosylphosphatidylinositol anchor and play essential roles in cell adhesion, signaling, and lipid metabolism (Vasilyeva et al., 2017). TFPs display one or several domains consisting of 60-90 amino acids which have an β-structural core stabilized by a system of four invariant disulfide bonds. Proteins with similar structural characteristics have been found in the mite Tetranychus urticae and insects (Grbić et al., 2011; Zhang et al., 2019). In flies, TFP-like proteins are involved in the formation of SJ adhesion structures, suggesting a common ancient role for these proteins in arthropods (Hijazi et al., 2009).
In this study, based on literature review and Blast N search1, we were able to identify putative homologs of proteins that regulate SJ and sSJ in Drosophila encoded by the genomes of T. urticae (the most studied mite in the superfamily Tetranychoidea) and B. yothersi (Table 1). One of the genes identified is the polychaetoid (pyd) gene, which is a recognized homolog of zonulin (Seppa et al., 2008; Choi et al., 2011; Djiane et al., 2011). The study of the transcriptomic profile of these genes in viruliferous mites will probably add hints on SJ and sSJ opening processes in mites and their possible relationship with cileviruses.
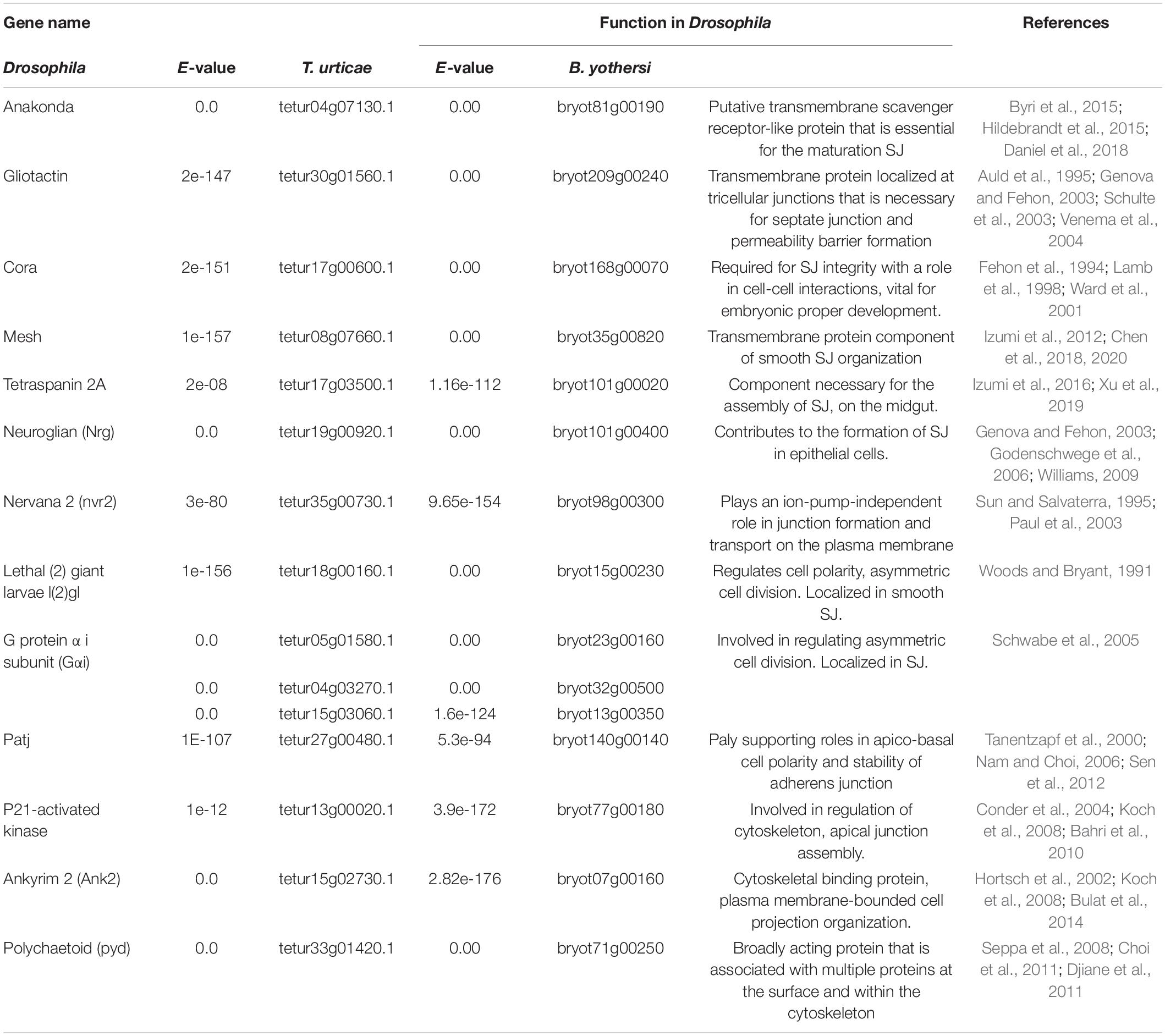
Table 1. Genes of Drosophila melanogaster involved in SJ and sSJ regulation and their respective orthologs in Tetranychus urticae and Brevipalpus yothersi.
Discussion
Virion particles of CiLV-C are routinely observed between the membrane of adjacent cells of B. yothersi (Alberti and Kitajima, 2014). In contrast, neither virions nor structures such as viroplasms, commonly associated with viral multiplication, have been observed inside mite cells. In this context, this study presents elements that guided us to pose the hypothesis of the paracellular movement of CiLV-C inside Brevipalpus mites. This unconventional viral movement has been described in the circulation of several viruses in localized organs by inducing disruptions of TJ in vertebrates. An example is the coronavirus SARS-COV-2, which is favored by the disruption of the airway epithelium. This process facilitates the virus paracellular spread into other tissues besides the translocation of endothelial cells (Tugizov, 2021). A similar mechanism is triggered by the rotavirus VP4 capsid protein. VP4 interacts with zonulin 1, occludin, and claudin, stimulating their redistribution and granting access to the junctional areas, which promotes viral spread in a paracellular way (Nava et al., 2004; Tugizov, 2021).
In phytopathosystems comprising (+)ssRNA viruses, a common virus-vector mode of transmission is circulative non-replicative (Hogenhout et al., 2008; Whitfield et al., 2015; Kondo et al., 2019). In many of these systems, the ingested virions must pass through vector cell barriers to reach the salivary glands, including the gut and hemocoel, involving specific interactions between the virus and vector membrane (Bragard et al., 2013; Blanc et al., 2014).
Negeviruses and other insect-borne viruses are likely vertical transmitted to host offprints (Vasilakis and Tesh, 2015; Kondo et al., 2019). Vertical transmission efficiency of plant-infecting (+)ssRNA viruses is generally low due to biological barriers via RNA silencing mechanisms that protect plant germ cells against viral infection (Foster et al., 2002; Martín-Hernández and Baulcombe, 2008). As consequence, most of these viruses depend on arthropod vectors for horizontal transmission in a non-replicative manner (Hull, 2013; Whitfield et al., 2015; Andika et al., 2016).
It is speculated that during virus evolution, some viruses, whose ancestors were arthropods-infecting viruses, adapted to plant hosts, but maintained an intimate relationship with those species of arthropods that eventually became their vectors. In these cases, virus circulation and replication are observed within the arthropod and plant hosts, as are the cases of the plant-infecting reoviruses rice dwarf virus and Southern rice black-streaked dwarf virus and their hemipteran vectors Nephotettix cincticeps and Sogatella furcifera, respectively, or the bunyavirus tomato spotted wilt virus and its thrips vector Frankliniella dentalis (Whitfield et al., 2015, 2018; Dolja and Koonin, 2018; Chen et al., 2019; Lefeuvre et al., 2019).
Biological, cytopathic, and molecular data on the cilevirus-mite relationship suggest that these viruses circulate in the vectors, but whether they replicate still needs to be addressed. It has been proposed that the cileviruses have evolved from an arthropod virus ancestor that somehow was able to infect plants after acquiring a movement protein from a plant virus (Ramos-González et al., 2020). The identification of some nege-like viruses infecting plants gives further support to the arthropod-plant host transitional process particularly involving kitaviruses and kita-like viruses infecting arthropods (Morozov et al., 2020). On this basis, it is tempting to speculate that during adaptation to plants, the presumed ancestor of kitaviruses lost arthropod fitness as it gradually adapted to plant hosts, but still, some viral factors required for its interaction with the arthropod were retained, for instance, those minimal components allowing for the circulative route using paracellular spaces. The study of Tetranychus urticae kitavirus (Niu et al., 2019), the closest kita-like virus infecting mites known, would likely add new elements to the mechanism underlying the movement of nege-kita-like viruses in their hosts.
If the paracellular route of cilevirus circulation in mites may be controlled in an active form, virion-membrane receptor(s) interaction has to be assumed. Accordingly, the transmissibility or not and efficiency of this process for a given pair of virus- Brevipalpus species must be dictated by the receptor(s) required for the virus entry into different groups of tissues by interactions with SJ. Studies on the molecular interactions between mite vectors and plant viruses are scarce (de Lillo et al., 2021). Global transcriptomic response of wheat streak mosaic virus (WSMV; genus Tritimovirus; family Potyviridae) and Aceria tosichella (Eriophyidae) showed the upregulation of two gene families that participate in the SJ formation (Gupta et al., 2019). Recent sequencing of the B. yothersi genome (Navia et al., 2019) and investigations on the function of CiLV-C-coded proteins (Leastro et al., 2018, 2020; Arena et al., 2020) may provide new insights into the putative participation of orthologues of these genes in Brevipalpus-cilevirus interaction.
In case the paracellular route of CiLV-C movement in Brevipalpus mites is confirmed, it will be the first example of a plant virus using this unconventional route of cell-to-cell movement in its arthropod vector. The unusual type of interaction with its vector, as also happens during the interaction of CiLV-C with their plant hosts, suggest that this virus, and probably other members of the family Kitaviridae, represent unique chimeric genetic systems that are likely reshaping features inherited from their ancestors to adapt to new ecological challenges.
Data Availability Statement
The original contributions presented in the study are included in the article/supplementary material, further inquiries can be directed to the corresponding author/s.
Author Contributions
AT, EK, PR-G, and JF-A: conceptualization and methodology. PR-G, AT, EK, and TS: formal analysis. JF-A and EK: funding acquisition. AT, TS, PR-G, EK, and JF-A: investigation. PR-G, EK, and JF-A: supervision. AT: writing—original draft. AT, PR-G, EK, TS, and JF-A: writing—review and editing. All authors contributed to the article and approved the submitted version.
Conflict of Interest
The authors declare that the research was conducted in the absence of any commercial or financial relationships that could be construed as a potential conflict of interest.
Publisher’s Note
All claims expressed in this article are solely those of the authors and do not necessarily represent those of their affiliated organizations, or those of the publisher, the editors and the reviewers. Any product that may be evaluated in this article, or claim that may be made by its manufacturer, is not guaranteed or endorsed by the publisher.
Acknowledgments
The authors are grateful to CAPES, CNPq, and FAPESP for scholarships, research fellowships, and research grants associated with this work (CAPES PNPD20132154 – 33141010001P4 – PNPD – IBSP, 88882.157041/2017-01; CNPq 312528/2020-5; FAPESP 2017/50222-0, 2017/50334-3, 2018/12252-8, and 2019/25078-9).
Footnotes
References
Alberti, G., and Coons, L. B. (1999). “Microscopic anatomy of invertebrates. Volume 8c. Chelicerate arthropoda,” in Microscopic Anatomy of Invertebrates, eds F. W. Harrison and R. Foelix (Chichester: Wiley & Sons), 267–514.
Alberti, G., and Kitajima, E. W. (2014). Anatomy and fine structure of brevipalpus mites (Tenuipalpidae) – economically important plant-virus vectors. Zoologica 160, 1–192.
Ali, M., Anwar, S., Shuja, M. N., Tripathi, R. K., and Singh, J. (2018). The genus Luteovirus from infection to disease. Eur. J. Plant Pathol. 151, 841–860. doi: 10.1007/s10658-018-1425-8
Amarasinghe, G. K., Ayllón, M. A., Bào, Y., Basler, C. F., Bavari, S., Blasdell, K. R., et al. (2019). Taxonomy of the order Mononegavirales: update 2019. Arch. Virol. 164, 1967–1980. doi: 10.1007/s00705-019-04247-4
Andika, I. B., Kondo, H., and Sun, L. (2016). Interplays between soil-borne plant viruses and RNA silencing-mediated antiviral defense in roots. Front. Microbiol. 7:1458. doi: 10.3389/fmicb.2016.01458
Arena, G. D., Ramos-González, P. L., Falk, B. W., Casteel, C. L., Freitas-Astúa, J., and Machado Marcos, A. (2020). Plant Immune system activation upon citrus leprosis virus c infection is mimicked by the ectopic expression of the p61 viral protein. Front. Plant Sci. 7:1757. doi: 10.3389/fpls.2020.01188
Auld, V. J., Fetter, R. D., Broadie, K., and Goodman, C. S. (1995). Gliotactin, a novel transmembrane protein on peripheral glia, is required to form the blood-nerve barrier in Drosophila. Cell 81, 757–767. doi: 10.1016/0092-8674(95)90537-5
Bahri, S., Wang, S., Conder, R., Choy, J., Vlachos, S., Dong, K., et al. (2010). The leading edge during dorsal closure as a model for epithelial plasticity: pak is required for recruitment of the Scribble complex and septate junction formation. Development 137, 2023–2032. doi: 10.1242/dev.045088
Barton, E. S., Forrest, J. C., Connolly, J. L., Chappell, J. D., Liu, Y., Schnell, F. J., et al. (2001). Junction adhesion molecule is a receptor for reovirus. Cell 104, 441–451. doi: 10.1016/S0092-8674(01)00231-8
Bergelson, J. M. (2009). Intercellular junctional proteins as receptors and barriers to virus infection and spread. Cell Host Microbe 5, 517–521. doi: 10.1016/j.chom.2009.05.009
Bergelson, J. M., Cunningham, J. A., Droguett, G., Kurt-Jones, E. A., Krithivas, A., Hong, J. S., et al. (1997). Isolation of a common receptor for coxsackie B viruses and adenoviruses 2 and 5. Science 275, 1320–1323. doi: 10.1126/science.275.5304.1320
Blanc, S., Drucker, M., and Uzest, M. (2014). Localizing viruses in their insect vectors. Annu. Rev. Phytopathol. 52, 403–425. doi: 10.1146/annurev-phyto-102313-045920
Bragard, C., Caciagli, P., Lemaire, O., Lopez-Moya, J. J., MacFarlane, S., Peters, D., et al. (2013). Status and prospects of plant virus control through interference with vector transmission. Annu. Rev. Phytopathol. 51, 177–201. doi: 10.1146/annurev-phyto-082712-102346
Brault, V., Herrbach, É, and Reinbold, C. (2007). Electron microscopy studies on luteovirid transmission by aphids. Micron 38, 302–312. doi: 10.1016/j.micron.2006.04.005
Brimacombe, C. L., Grove, J., Meredith, L. W., Hu, K., Syder, A. J., Flores, M. V., et al. (2011). Neutralizing antibody-resistant hepatitis c virus cell-to-cell transmission. J. Virol. 85, 596–605. doi: 10.1128/jvi.01592-10
Bulat, V., Rast, M., and Pielage, J. (2014). Presynaptic CK2 promotes synapse organization and stability by targeting Ankyrin2. J. Cell Biol. 204, 77–94. doi: 10.1083/jcb.201305134
Byri, S., Misra, T., Syed, Z. A., Bätz, T., Shah, J., Boril, L., et al. (2015). The triple-repeat protein anakonda controls epithelial tricellular junction formation in Drosophila. Dev. Cell 33, 535–548. doi: 10.1016/j.devcel.2015.03.023
Calegario, R. F., Locali, E. C., Stach-Machado, D. R., Peroni, L. A., Caserta, R., Salaroli, R. B., et al. (2013). Polyclonal antibodies to the putative coat protein of CiLV-C (Citrus leprosis virus C) expressed in Escherichia coli: production and use in immunodiagnosis. Trop. Plant Pathol. 38, 188–197. doi: 10.1590/S1982-56762013005000005
Carapeta, S., do Bem, B., McGuinness, J., Esteves, A., Abecasis, A., Lopes, Â, et al. (2015). Negeviruses found in multiple species of mosquitoes from southern portugal: isolation, genetic diversity, and replication in insect cell culture. Virology 483, 318–328. doi: 10.1016/j.virol.2015.04.021
Castro, E. B., Mesa, N. C., Feres, R. J. F., de Moraes, G. J., Ocha, R., Beard, J. J., et al. (2020). A newly available database of an important family of phytophagous mites: tenuipalpidae database. Zootaxa 4868, 577–583. doi: 10.11646/zootaxa.4868.4.7
Chabi-Jesus, C., Ramos-González, P. L., Postclam-Barro, M., Fontenele, R. S., Harakava, R., Bassanezi, R. B., et al. (2021). Molecular epidemiology of citrus leprosis virus c: a new viral lineage and phylodynamic of the main viral subpopulations in the americas. Front. Microbiol. 12:641252. doi: 10.3389/fmicb.2021.641252
Che, P., Tang, H., and Li, Q. (2013). The interaction between claudin-1 and dengue viral prM/M protein for its entry. Virology 446, 303–313. doi: 10.1016/j.virol.2013.08.009
Chen, H. J., Li, Q., Nirala, N. K., and Ip, Y. T. (2020). The snakeskin-mesh complex of smooth septate junction restricts yorkie to regulate intestinal homeostasis in Drosophila. Stem Cell Rep. 14, 828–844. doi: 10.1016/j.stemcr.2020.03.021
Chen, J., Sayadian, A. C., Lowe, N., Lovegrove, H. E., and St Johnston, D. (2018). An alternative mode of epithelial polarity in the Drosophila midgut. PLoS Biol. 16:e3000041. doi: 10.1371/journal.pbio.3000041
Chen, Y., Dessau, M., Rotenberg, D., Rasmussen, D. A., and Whitfield, A. E. (2019). Entry of bunyaviruses into plants and vectors. Adv. Virus Res. 104, 65–96. doi: 10.1016/BS.AIVIR.2019.07.001
Chiavegato, L. (1996). Aspectos biológicos e transmissão de leprose pelo ácaro Brevipalpus phoenicis (Geijskes, 1939) (Acari: Tenuipalpidae) em citros. Laranja 17, 229–235. doi: 10.1590/S0100-83582012000100011
Choi, W., Jung, K. C., Nelson, K. S., Bhat, M. A., Beitel, G. J., Peifer, M., et al. (2011). The single Drosophila ZO-1 protein polychaetoid regulates embryonic morphogenesis in coordination with canoe/afadin and Enabled. Mol. Biol. Cell 22, 2010–2030. doi: 10.1091/mbc.E10-12-1014
Cifuentes-Munoz, N., El Najjar, F., and Dutch, R. E. (2020). Viral cell-to-cell spread: conventional and non-conventional ways. Adv. Virus Res. 108, 85–125. doi: 10.1016/bs.aivir.2020.09.002
Conder, R., Yu, H., Ricos, M., Hing, H., Chia, W., Lim, L., et al. (2004). DPak is required for integrity of the leading edge cytoskeleton during Drosophila dorsal closure but does not signal through the JNK cascade. Dev. Biol. 276, 378–390. doi: 10.1016/j.ydbio.2004.08.044
Daniel, E., Daudé, M., Kolotuev, I., Charish, K., Auld, V., and Le Borgne, R. (2018). Coordination of septate junctions assembly and completion of cytokinesis in proliferative epithelial tissues. Curr. Biol. 28, 1380–1391. doi: 10.1016/j.cub.2018.03.034
de Lillo, E., Freitas-Astúa, J., Kitajima, E. W., Ramos-González, P. L., Simoni, S., Tassi, A. D., et al. (2021). Phytophagous mites transmitting plant viruses: update and perspectives. Entomol. Gen. 41, 439–462. doi: 10.1127/ENTOMOLOGIA/2021/1283
Di Mattia, J., Vernerey, M.-S., Yvon, M., Pirolles, E., Villegas, M., Gaafar, Y., et al. (2020). Route of a multipartite nanovirus across the body of its aphid vector. J. Virol. 94:e01998–19. doi: 10.1128/jvi.01998-19
Dietzgen, R. G., Freitas-Astúa, J., Chabi-Jesus, C., Ramos-González, P. L., Goodin, M. M., Kondo, H., et al. (2018). Dichorhaviruses in their host plants and mite vectors. Adv. Virus Res. 102, 119–148. doi: 10.1016/bs.aivir.2018.06.001
Djiane, A., Shimizu, H., Wilkin, M., Mazleyrat, S., Jennings, M. D., Avis, J., et al. (2011). Su(dx) E3 ubiquitin ligase-dependent and -independent functions of polychaetoid, the Drosophila ZO-1 homologue. J. Cell Biol. 192, 189–200. doi: 10.1083/jcb.201007023
Dolja, V. V., and Koonin, E. V. (2018). Metagenomics reshapes the concepts of RNA virus evolution by revealing extensive horizontal virus transfer. Virus Res. 244, 36–52. doi: 10.1016/j.virusres.2017.10.020
Fasano, A. (2012). Zonulin, regulation of tight junctions, and autoimmune diseases. Ann. N. Y. Acad. Sci. 1258, 25–33. doi: 10.1111/j.1749-6632.2012.06538.x
Fehon, R. G., Dawson, L. A., and Artavanis-Tsakonas, S. (1994). A Drosophila homologue of membrane-skeleton protein 4.1 is associated with septate junctions and is encoded by the coracle gene. Development 120, 545–557. doi: 10.1242/dev.120.3.545
Foster, T. M., Lough, T. J., Emerson, S. J., Lee, R. H., Bowman, J. L., Forster, R. L. S., et al. (2002). A surveillance system regulates selective entry of rna into the shoot apex. Plant Cell 14, 1497–1508. doi: 10.1105/tpc.001685
Freitas-Astúa, J., Ramos-González, P. L., Arena, G. D., Tassi, A. D., and Kitajima, E. W. (2018). Brevipalpus-transmitted viruses: parallelism beyond a common vector or convergent evolution of distantly related pathogens? Curr. Opin. Virol. 33, 66–73. doi: 10.1016/J.COVIRO.2018.07.010
Garita, L. C., Tassi, A. D., Calegario, R. F., Kitajima, E. W., Carbonell, S. A. M., and Freitas-Astúa, J. (2013). Common bean: experimental indicator plant for citrus leprosis virus C and some other cytoplasmic-type brevipalpus-transmitted viruses. Plant Dis. 97, 1346–1351. doi: 10.1094/PDIS-12-12-1143-RE
Genova, J. L., and Fehon, R. G. (2003). Neuroglian, gliotactin, and the Na+/k+ ATPase are essential for septate junction function in Drosophila. J. Cell Biol. 161, 979–989. doi: 10.1083/jcb.200212054
Godenschwege, T. A., Kristiansen, L. V., Uthaman, S. B., Hortsch, M., and Murphey, R. K. (2006). A conserved role for Drosophila neuroglian and human L1-CAM in central-synapse formation. Curr. Biol. 16, 12–23. doi: 10.1016/j.cub.2005.11.062
Grbić, M., Van Leeuwen, T., Clark, R. M., Rombauts, S., Rouzé, P., Grbić, V., et al. (2011). The genome of Tetranychus urticae reveals herbivorous pest adaptations. Nature 479, 487–492. doi: 10.1038/nature10640
Gupta, A. K., Scully, E. D., Palmer, N. A., Geib, S. M., Sarath, G., Hein, G. L., et al. (2019). Wheat streak mosaic virus alters the transcriptome of its vector, wheat curl mite (Aceria tosichella keifer), to enhance mite development and population expansion. J. Gen. Virol. 100, 889–910. doi: 10.1099/jgv.0.001256
Hall, S., and Ward, R. E. (2016). Septate junction proteins play essential roles in morphogenesis throughout embryonic development in Drosophila. G3 Genes Genomes Genet. 6, 2375–2384. doi: 10.1534/g3.116.031427
Hijazi, A., Masson, W., Augé, B., Waltzer, L., Haenlin, M., and Roch, F. (2009). Boudin is required for septate junction organisation in Drosophila and codes for a diffusible protein of the Ly6 superfamily. Development 136, 2199–2209. doi: 10.1242/dev.033845
Hildebrandt, A., Pflanz, R., Behr, M., Tarp, T., Riedel, D., and Schuh, R. (2015). Bark beetle controls epithelial morphogenesis by septate junction maturation in Drosophila. Dev. Biol. 400, 237–247. doi: 10.1016/j.ydbio.2015.02.008
Hogenhout, S. A., Ammar, E.-D., Whitfield, A. E., and Redinbaugh, M. G. (2008). Insect vector interactions with persistently transmitted viruses. Annu. Rev. Phytopathol. 46, 327–359. doi: 10.1146/annurev.phyto.022508.092135
Hortsch, M., Paisley, K. L., Tian, M. Z., Qian, M., Bouley, M., and Chandler, R. (2002). The axonal localization of large Drosophila ankyrin2 protein isoforms is essential for neuronal functionality. Mol. Cell. Neurosci. 20, 43–55. doi: 10.1006/mcne.2002.1113
Izumi, Y., and Furuse, M. (2014). Molecular organization and function of invertebrate occluding junctions. Semin. Cell Dev. Biol. 36, 186–193. doi: 10.1016/j.semcdb.2014.09.009
Izumi, Y., Motoishi, M., Furuse, K., and Furuse, M. (2016). A tetraspanin regulates septate junction formation in Drosophila midgut. J. Cell Sci. 129, 1155–1164. doi: 10.1242/jcs.180448
Izumi, Y., Yanagihashi, Y., and Furuse, M. (2012). A novel protein complex, Mesh-Ssk, is required for septate junction formation in the Drosophila midgut. J. Cell Sci. 125, 4923–4933. doi: 10.1242/jcs.112243
Kitajima, E. W., Chagas, C. M., and Rodrigues, J. C. V. (2003). Brevipalpus-transmitted plant virus and virus-like diseases: cytopathology and some recent cases. Exp. Appl. Acarol. 30, 135–160. doi: 10.1023/B:APPA.0000006546.55305.e3
Kitajima, E. W., Rodrigues, J. C. V., and Freitas-Astua, J. (2010). An annotated list of ornamentals naturally found infected by Brevipalpus mite-transmitted viruses. Sci. Agric. 67, 348–371. doi: 10.1590/S0103-90162010000300014
Koch, I., Schwarz, H., Beuchle, D., Goellner, B., Langegger, M., and Aberle, H. (2008). Drosophila ankyrin 2 Is required for synaptic stability. Neuron 58, 210–222. doi: 10.1016/j.neuron.2008.03.019
Kondo, H., Chiba, S., Maruyama, K., Andika, I. B., and Suzuki, N. (2019). A novel insect-infecting virga/nege-like virus group and its pervasive endogenization into insect genomes. Virus Res. 262, 37–47. doi: 10.1016/j.virusres.2017.11.020
Kondo, H., Fujita, M., Hisano, H., Hyodo, K., Andika, I. B., and Suzuki, N. (2020). Virome analysis of aphid populations that infest the barley field: the discovery of two novel groups of Nege/Kita-like viruses and other novel RNA Viruses. Front. Microbiol. 11:509. doi: 10.3389/fmicb.2020.00509
Kondo, H., Maeda, T., and Tamada, T. (2003). Orchid fleck virus: Brevipalpus californicus mite transmission, biological properties and genome structure. Exp. Appl. Acarol. 30, 215–223. doi: 10.1023/b:appa.0000006550.88615.10
Kuhn, J. H., Adkins, S., Alioto, D., Alkhovsky, S. V., Amarasinghe, G. K., Anthony, S. J., et al. (2020). 2020 taxonomic update for phylum Negarnaviricota (Riboviria: Orthornavirae), including the large orders Bunyavirales and Mononegavirales. Arch. Virol. 165, 3023–3072. doi: 10.1007/s00705-020-04731-2
Lamb, R. S., Ward, R. E., Schweizer, L., and Fehon, R. G. (1998). Drosophila coracle, a member of the protein 4.1 superfamily, has essential structural functions in the septate junctions and developmental functions in embryonic and adult epithelial cells. Mol. Biol. Cell 9, 3505–3519. doi: 10.1091/mbc.9.12.3505
Leastro, M. O., Kitajima, E. W., Silva, M. S., Resende, R. O., and Freitas-Astúa, J. (2018). Dissecting the subcellular localization, intracellular trafficking, interactions, membrane association, and topology of citrus leprosis Virus C proteins. Front. Plant Sci. 9:1299. doi: 10.3389/fpls.2018.01299
Leastro, M. O., Ortega-Castro, D. Y., Freitas-Astúa, J., Kitajima, E. W., Pallas, V., Sanchéz-Navarro, J. A., et al. (2020). Citrus leprosis virus C encodes three proteins with gene silencing suppression activity. Front. Microbiol. 11:1231. doi: 10.3389/fmicb.2020.01231
Lefeuvre, P., Martin, D. P., Elena, S. F., Shepherd, D. N., Roumagnac, P., and Varsani, A. (2019). Evolution and ecology of plant viruses. Nat. Rev. Microbiol. 17, 632–644. doi: 10.1038/s41579-019-0232-3
Martín-Hernández, A. M., and Baulcombe, D. C. (2008). Tobacco rattle virus 16-kilodalton protein encodes a suppressor of rna silencing that allows transient viral entry in meristems. J. Virol. 82, 4064–4071. doi: 10.1128/JVI.02438-07
Mateo, M., Generous, A., Sinn, P. L., and Cattaneo, R. (2015). Connections matter - How viruses use cell-cell adhesion components. J. Cell Sci. 128, 431–439. doi: 10.1242/jcs.159400
Medigeshi, G. R., Hirsch, A. J., Brien, J. D., Uhrlaub, J. L., Mason, P. W., Wiley, C., et al. (2009). West nile virus capsid degradation of claudin proteins disrupts epithelial barrier function. J. Virol. 83, 6125–6134. doi: 10.1128/JVI.02617-08
Melzer, M. J., Simbajon, N., Carillo, J., Borth, W. B., Freitas-Astúa, J., Kitajima, E. W., et al. (2013). A cilevirus infects ornamental hibiscus in Hawaii. Arch. Virol. 158, 2421–2424. doi: 10.1007/s00705-013-1745-0
Morozov, S. Y., Lazareva, E. A., and Solovyev, A. G. (2020). Sequence relationships of RNA helicases and other proteins encoded by blunervirus RNAs highlight recombinant evolutionary origin of kitaviral genomes. Front. Microbiol. 11:561092. doi: 10.3389/fmicb.2020.561092
Mühlebach, M. D., Mateo, M., Sinn, P. L., Prüfer, S., Uhlig, K. M., Leonard, V. H. J., et al. (2011). Adherens junction protein nectin-4 is the epithelial receptor for measles virus. Nature 480, 530–533. doi: 10.1038/nature10639
Nakatsu, Y., Ma, X., Seki, F., Suzuki, T., Iwasaki, M., Yanagi, Y., et al. (2013). Intracellular transport of the measles virus ribonucleoprotein complex is mediated by rab11A-positive recycling endosomes and drives virus release from the apical membrane of polarized epithelial cells. J. Virol. 87, 4683–4693. doi: 10.1128/jvi.02189-12
Nam, S. C., and Choi, K. W. (2006). Domain-specific early and late function of Dpatj in Drosophila photoreceptor cells. Dev. Dyn. 235, 1501–1507. doi: 10.1002/dvdy.20726
Nava, P., López, S., Arias, C. F., Islas, S., and González-Mariscal, L. (2004). The rotavirus surface protein VP8 modulates the gate and fence function of tight junctions in epithelial cells. J. Cell Sci. 117, 5509–5519. doi: 10.1242/jcs.01425
Navia, D., Novelli, V. M., Rombauts, S., Freitas-Astúa, J., Santos de Mendonça, R., Nunes, M. A., et al. (2019). Draft genome assembly of the false spider mite Brevipalpus yothersi. Microbiol. Resour. Announc. 8:e01563–18. doi: 10.1128/mra.01563-18
Niu, J., Zhang, W., Sun, Q.-Z., and Wang, J.-J. (2019). Three novel RNA viruses in the spider mite Tetranychus urticae and their possible interactions with the host RNA interference response. J. Invertebr. Pathol. 166:107228. doi: 10.1016/j.jip.2019.107228
O’Brien, C. A., McLean, B. J., Colmant, A. M. G., Harrison, J. J., Hall-Mendelin, S., van den Hurk, A. F., et al. (2017). Discovery and characterisation of castlerea virus, a new species of Negevirus isolated in Australia. Evol. Bioinform. Online 13:1176934317691269. doi: 10.1177/1176934317691269
Olmedo-Velarde, A., Hu, J., and Melzer, M. J. (2021). A Virus Infecting Hibiscus rosa-sinensis represents an evolutionary link between Cileviruses and Higreviruses. Front. Microbiol. 12:660237. doi: 10.3389/fmicb.2021.660237
Paul, S. M., Ternet, M., Salvaterra, P. M., and Beitel, G. J. (2003). The Na+/K+ ATPase is required for septate junction function and epithelial tube-size control in the Drosophila tracheal system. Development 130, 4963–4974. doi: 10.1242/dev.00691
Quito-Avila, D. F., Freitas-Astúa, J., and Melzer, M. J. (2021). “Bluner-, cile-, and higreviruses (Kitaviridae),” in Reference Module in Life Sciences, ed. B. D. Roitberg (Elsevier: Amstersam), 247–251. doi: 10.1016/B978-0-12-809633-8.21248-X
Ramos-González, P. L., Chabi-Jesus, C., Banguela-Castillo, A., Tassi, A. D., Rodrigues, M., da, C., et al. (2018). Unveiling the complete genome sequence of clerodendrum chlorotic spot virus, a putative dichorhavirus infecting ornamental plants. Arch. Virol. 163, 2519–2524. doi: 10.1007/s00705-018-3857-z
Ramos-González, P. L., dos Santos, G. F., Chabi-Jesus, C., Harakava, R., Kitajima, E. W., and Freitas-Astúa, J. (2020). Passion fruit green spot virus genome harbors a new orphan ORF and highlights the flexibility of the 5’-end of the RNA2 segment across cileviruses. Front. Microbiol. 11:206. doi: 10.3389/fmicb.2020.00206
Reynolds, G. M., Harris, H. J., Jennings, A., Hu, K., Grove, J., Lalor, P. F., et al. (2008). Hepatitis C virus receptor expression in normal and diseased liver tissue. Hepatology 47, 418–427. doi: 10.1002/hep.22028
Roy, A., Hartung, J. S., Schneider, W. L., Shao, J., Leon, M. G., Melzer, M. J., et al. (2015). Role bending: Complex relationships between viruses, hosts, and vectors related to citrus leprosis, an emerging disease. Phytopathology 105, 872–884. doi: 10.1094/PHYTO-12-14-0375-FI
Schulte, J., Tepass, U., and Auld, V. J. (2003). Gliotactin, a novel marker of tricellular junctions, is necessary for septate junction development in Drosophila. J. Cell Biol. 161, 991–1000. 161. doi: 10.1083/jcb.200303192
Schwabe, T., Bainton, R. J., Fetter, R. D., Heberlein, U., and Gaul, U. (2005). GPCR signaling is required for blood-brain barrier formation in Drosophila. Cell 123, 133–144. doi: 10.1016/j.cell.2005.08.037
Sen, A., Nagy-Zsvér-Vadas, Z., and Krahn, M. P. (2012). Drosophila PATJ supports adherens junction stability by modulating Myosin light chain activity. J. Cell Biol. 199, 685–698. doi: 10.1083/jcb.201206064
Seppa, M. J., Johnson, R. I., Bao, S., and Cagan, R. L. (2008). Polychaetoid controls patterning by modulating adhesion in the Drosophila pupal retina. Dev. Biol. 318, 1–16. doi: 10.1016/j.ydbio.2008.02.022
Sun, B., and Salvaterra, P. M. (1995). Characterization of nervana, a drosophila melanogaster neuron-specific glycoprotein antigen recognized by anti-horseradish peroxidase antibodies. J. Neurochem. 65, 434–443. doi: 10.1046/j.1471-4159.1995.65010434.x
Tanentzapf, G., Smith, C., McGlade, J., and Tepass, U. (2000). Apical, lateral, and basal polarization cues contribute to the development of the follicular epithelium during Drosophila oogenesis. J. Cell Biol. 151, 891–904. doi: 10.1083/jcb.151.4.891
Tassi, A. D., Garita-Salazar, L. C., Amorim, L., Novelli, V. M., Freitas-Astúa, J., Childers, C. C., et al. (2017). Virus-vector relationship in the Citrus leprosis pathosystem. Exp. Appl. Acarol. 71, 227–241. doi: 10.1007/s10493-017-0123-0
Timpe, J. M., Stamataki, Z., Jennings, A., Hu, K., Farquhar, M. J., Harris, H. J., et al. (2008). Hepatitis C virus cell-cell transmission in hepatoma cells in the presence of neutralizing antibodies. Hepatology 47, 17–24. doi: 10.1002/hep.21959
Tugizov, S. (2021). Virus-associated disruption of mucosal epithelial tight junctions and its role in viral transmission and spread. Tissue Barries 9:194374. doi: 10.1080/21688370.2021.1943274
Vasilakis, N., and Tesh, R. B. (2015). Insect-specific viruses and their potential impact on arbovirus transmission. Curr. Opin. Virol. 15, 69–74. doi: 10.1016/J.COVIRO.2015.08.007
Vasilakis, N., Forrester, N. L., Palacios, G., Nasar, F., Savji, N., Rossi, S. L., et al. (2013). Negevirus: a proposed new taxon of insect-specific viruses with wide geographic distribution. J. Virol. 87, 2475–2488. doi: 10.1128/JVI.00776-12
Vasilyeva, N. A., Loktyushov, E. V., Bychkov, M. L., Shenkarev, Z. O., and Lyukmanova, E. N. (2017). Three-finger proteins from the Ly6/uPAR family: functional diversity within one structural motif. Biochemistry 82, 1702–1715. doi: 10.1134/S0006297917130090
Venema, D. R., Zeev-Ben-Mordehai, T., and Auld, V. J. (2004). Transient apical polarization of Gliotactin and Coracle is required for parallel alignment of wing hairs in Drosophila. Dev. Biol. 275, 301–314. doi: 10.1016/j.ydbio.2004.07.040
Ward, R. E. IV, Schweizer, L., Lamb, R. S., and Fehon, R. G. (2001). The protein 4.1, ezrin, radixin, moesin (FERM) domain of drosophila coracle, a cytoplasmic component of the septate junction, provides functions essential for embryonic development and imaginal cell proliferation. Genetics 159, 219–228. doi: 10.1093/genetics/159.1.219
Welbourn, W. C., Ochoa, R., Kane, E. C., and Erbe, E. F. (2003). Morphological observations on Brevipalpus phoenicis (Acari: Tenuipalpidae) including comparisons with B. californicus and B. obovatus. Exp. Appl. Acarol. 30, 107–133. doi: 10.1023/B:APPA.0000006545.40017.a0
Whitfield, A. E., Falk, B. W., and Rotenberg, D. (2015). Insect vector-mediated transmission of plant viruses. Virology 479–480, 278–289. doi: 10.1016/j.virol.2015.03.026
Whitfield, A. E., Huot, O. B., Martin, K. M., Kondo, H., and Dietzgen, R. G. (2018). Plant rhabdoviruses—their origins and vector interactions. Curr. Opin. Virol. 33, 0198–207. doi: 10.1016/j.coviro.2018.11.002
Williams, M. J. (2009). The Drosophila cell adhesion molecule Neuroglian regulates Lissencephaly-1 localisation in circulating immunosurveillance cells. BMC Immunol. 10:17. doi: 10.1186/1471-2172-10-17
Woods, D. F., and Bryant, P. J. (1991). The discs-large tumor suppressor gene of Drosophila encodes a guanylate kinase homolog localized at septate junctions. Cell 66, 451–464. doi: 10.1016/0092-8674(81)90009-X
Xu, C., Tang, H. W., Hung, R. J., Hu, Y., Ni, X., Housden, B. E., et al. (2019). The septate junction protein Tsp2A restricts intestinal stem cell activity via endocytic regulation of aPKC and Hippo signaling. Cell Rep. 26, 670–688. doi: 10.1016/j.celrep.2018.12.079
Yonekawa, K., and Harlan, J. M. (2005). Targeting leukocyte integrins in human diseases. J. Leukoc. Biol. 77, 129–140. doi: 10.1189/jlb.0804460
Yumine, N., Matsumoto, Y., Ohta, K., Fukasawa, M., and Nishio, M. (2019). Claudin-1 inhibits human parainfluenza virus type 2 dissemination. Virology 531, 93–99. doi: 10.1016/j.virol.2019.01.031
Zhang, Y. X., Chen, X., Wang, J. P., Zhang, Z. Q., Wei, H., Yu, H. Y., et al. (2019). Genomic insights into mite phylogeny, fitness, development, and reproduction. BMC Genomics 20:954. doi: 10.1186/s12864-019-6281-1
Keywords: virus vector relationship, Kitaviridae, virus movement, septate junctions, flat mite, citrus leprosis virus C
Citation: Tassi AD, Ramos-González PL, Sinico TE, Kitajima EW and Freitas-Astúa J (2022) Circulative Transmission of Cileviruses in Brevipalpus Mites May Involve the Paracellular Movement of Virions. Front. Microbiol. 13:836743. doi: 10.3389/fmicb.2022.836743
Received: 15 December 2021; Accepted: 15 February 2022;
Published: 06 April 2022.
Edited by:
Elvira Fiallo-Olivé, La Mayora Experimental Station (CSIC), SpainReviewed by:
Miguel A. Aranda, Spanish National Research Council (CSIC), SpainJaqueline Franciosi Della Vechia, São Paulo State University, Brazil
Copyright © 2022 Tassi, Ramos-González, Sinico, Kitajima and Freitas-Astúa. This is an open-access article distributed under the terms of the Creative Commons Attribution License (CC BY). The use, distribution or reproduction in other forums is permitted, provided the original author(s) and the copyright owner(s) are credited and that the original publication in this journal is cited, in accordance with accepted academic practice. No use, distribution or reproduction is permitted which does not comply with these terms.
*Correspondence: Aline Daniele Tassi, YWxpbmV0YXNzaUBnbWFpbC5jb20=