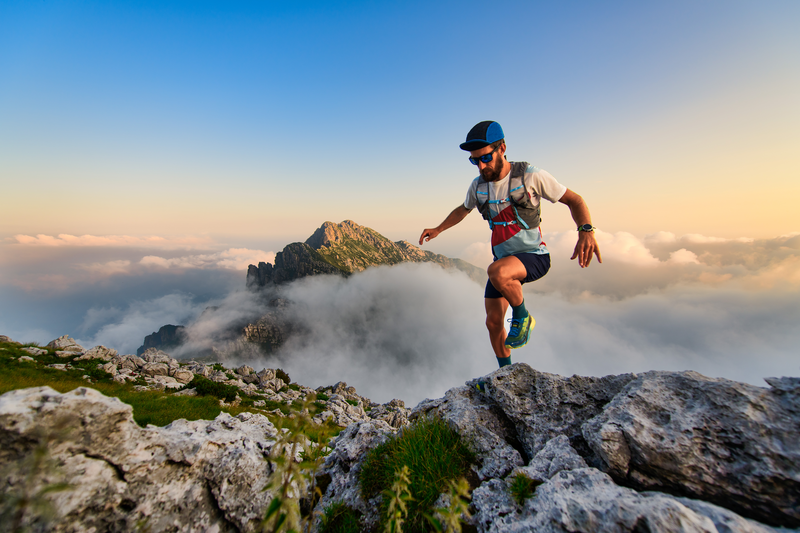
95% of researchers rate our articles as excellent or good
Learn more about the work of our research integrity team to safeguard the quality of each article we publish.
Find out more
ORIGINAL RESEARCH article
Front. Microbiol. , 07 March 2022
Sec. Virology
Volume 13 - 2022 | https://doi.org/10.3389/fmicb.2022.835443
This article is part of the Research Topic HIV-1 Genetic Diversity, Volume II View all 21 articles
The HIV-1 epidemic in the Amazonas state, as in most of Brazil, is dominated by subtype B. The state, nonetheless, is singular for its significant co-circulation of the variants BCAR, which can mostly be found in the Caribbean region, and BPAN, a clade that emerged in the United States and aggregates almost the totality of subtype B infections world-wide. The Amazonian HIV-1 epidemic provides a unique scenario to compare the epidemic potential of BPAN and BCAR clades spreading in the same population. To reconstruct the spatiotemporal dynamic and demographic history of both subtype B lineages circulating in Amazonas, we analyzed 1,272 HIV-1 pol sequences sampled in that state between 2009 and 2018. Our phylogeographic analyses revealed that while most BCAR infections resulted from a single successful founder event that took place in the Amazonas state around the late 1970s, most BPAN infections resulted from the expansion of multiple clusters seeded in the state since the late 1980s. Our data support the existence of at least four large clusters of the pandemic form in Amazonas, two of them nested in Brazil’s largest known subtype B cluster (BBR–I), and two others resulting from new introductions detected here. The reconstruction of the demographic history of the most prevalent BPAN (n = 4) and BCAR (n = 1) clades identified in Amazonas revealed that all clades displayed a continuous expansion [effective reproductive number (Re) > 1] until most recent times. During the period of co-circulation from the late 1990s onward, the Re of Amazonian BPAN and BCAR clusters behaved quite alike, fluctuating between 2.0 and 3.0. These findings support that the BCAR and BPAN variants circulating in the Brazilian state of Amazonas displayed different evolutionary histories, but similar epidemic trajectories and transmissibility over the last two decades, which is consistent with the notion that both subtype B variants display comparable epidemic potential. Our findings also revealed that despite significant advances in the treatment of HIV infections in the Amazonas state, BCAR and BPAN variants continue to expand and show no signs of the epidemic stabilization observed in other parts of the country.
The HIV-1 subtype B pandemic started when the ancestral virus arrived and first established itself in the Caribbean region during the mid-1960s (Gilbert et al., 2007). The subsequent subtype B spread generated a set of local clades, designated as BCAR, that remained mostly confined to the Caribbean region (Gilbert et al., 2007; Cabello et al., 2014). One of those viruses, however, migrated from the Caribbean to the United States around the late 1960s and established a pandemic clade, called BPAN, that was then disseminated worldwide (Worobey et al., 2016). The remarkable dissemination of the BPAN clade was probably shaped by ecological, rather than virological factors (Gilbert et al., 2007; Arantes et al., 2020); but there are no studies comparing the epidemic potential of BCAR and BPAN clades co-circulating outside the Caribbean region.
The HIV-1 subtype B epidemic in Brazil is mainly driven by the BPAN clade, with a few notable exceptions, like the Northern state of Amazonas, which is characterized by the co-circulation of the BPAN (75%) and BCAR (25%) variants at a high prevalence (Divino et al., 2016; Crispim et al., 2019; Gräf et al., 2021). Among regional HIV-1 epidemics in Brazil, the one in the state of Amazonas stands out as the second largest AIDS detection rate (34.8 cases per 100,000 inhabitants) in the country, well above the mean national rate (17.8 cases per 100,000 inhabitants) (Ministério da Saúde, 2020). Furthermore, despite important advances in HIV diagnosis and treatment, the Amazonas HIV/AIDS epidemic is not stabilized and has displayed a rising AIDS incidence over the last 10 years (Ministério da Saúde, 2020).
The Amazonian HIV-1 epidemic thus provides a great opportunity to compare the epidemic dynamics of the BPAN and BCAR clades spreading in the same population outside the Caribbean region. Previous studies identified four major BPAN (BPAN–BR–I to BPAN–BR–IV) and four major BCAR (BCAR–BR–I to BCAR–BR–IV) clusters circulating in Brazil (Mir et al., 2015; Divino et al., 2016). The lineage BCAR–BR–I aggregates the majority (51%) of non-pandemic subtype B sequences from Brazil (Divino et al., 2016) and its most recent common ancestor (MRCA) could be traced back to Amazonas in the late 1970s, from a viral migration probably originated in the French Guiana (Divino et al., 2016; Arantes et al., 2019). The cluster composition and the evolutionary history of the BPAN clade in the Amazonas state are currently unknown.
This work aims to characterize the spatiotemporal dynamics of the major BPAN clusters circulating in the state of Amazonas and to compare the evolutionary and demographic history of major pandemic and non-pandemic subtype B lineages that drive the expanding HIV-1 subtype B epidemic in this Northern Brazilian state.
In this study, we used a total of 1,272 HIV-1 subtype B pol sequences (nucleotides 2,253–3,275 of reference strain HXB2) from Amazonas state sampled between 2009 and 2018 that were either available at the Los Alamos HIV Database1 by March 2021 or were recently published (Chaves et al., 2021; Gräf et al., 2021) and made available in GenBank2. Only one sequence per subject was selected. Sequences were aligned using the ClustalW program (Larkin et al., 2007) and all sites associated with major antiretroviral drug resistance in protease and reverse transcriptase were excluded.
Amazonian HIV-1 subtype B sequences were initially classified as BCAR or BPAN and next within major BCAR or BPAN Brazilian clusters by using an evolutionary placement algorithm (EPA) available in RAxML v.8.0.0 (Stamatakis, 2014) for the rapid assignment of query sequences to edges of a reference phylogenetic tree under a maximum-likelihood (ML) model. This analysis allowed us to classify sequences within 10 different clades: BCAR–BR–I to BCAR–BR–IV, BPAN–BR–I to BPAN–BR–IV, other BCAR lineages, and other BPAN lineages.
After the initial cluster assignment, the HIV-1 subtype B pol Amazonian sequences were aligned with different sub-sets of non-Amazonian subtype B pol reference sequences (covering nucleotides 2,253–3,260 relative to HXB2 genome). Amazonian subtype B sequences classified within major BCAR (BCAR–BR–I to BCAR–BR–IV) or BPAN (BPAN–BR–I to BPAN–BR–IV) Brazilian clusters were aligned with Brazilian sequences from other states that were previously classified within those major lineages (Mir et al., 2015; Divino et al., 2016). Amazonian subtype B sequences classified as “others BCAR lineages” were aligned with sequences representative of the BCAR diversity in the Caribbean region (n = 228) that were also described previously (Cabello et al., 2014; Mendoza et al., 2014). Finally, Amazonian subtype B sequences classified as “others BPAN lineages” were aligned with: (i) one subset of closely related BPAN sequences from Brazil (n = 687) selected from a large dataset of Brazilian sequences (n = 88,441) described previously (Gräf et al., 2021) and (ii) one subset of closely related BPAN sequences from different countries (n = 1,700) selected from a large dataset of worldwide sampled sequences (n = 71,160) recovered from Los Alamos HIV Database. We used the basic local alignment search tool (BLAST)3 to select the 10 subtype B reference sequences (Brazilian and worldwide) with the highest similarity score (>95%) to each Amazonian subtype B sequence.
Amazonian and non-Amazonian subtype B sequences were subject to ML phylogeographic analyses to identify the BCAR and BPAN sub-clusters that probably originated in Amazonas. ML phylogenetic trees were inferred with the PhyML program (Guindon et al., 2010) using an online web server (Guindon et al., 2005) under the GTR + I + Γ4 nucleotide substitution model, as selected by the jModelTest program (Posada, 2008), and the SPR branch-swapping algorithm of heuristic tree search. The reliability of the obtained tree topology was estimated with the approximate likelihood-ratio test (aLRT) (Anisimova and Gascuel, 2006) based on the Shimodaira-Hasegawa-like procedure. Trees were rooted using subtype D sequences and visualized using the FigTree v1.4.0 software (Rambaut, 2009). The ML trees were employed for the ancestral character state reconstruction (ACR) of epidemic locations with PastML (Ishikawa et al., 2019), using the maximum likelihood Joint (Pupko et al., 2000) and marginal posterior probabilities approximation (MPPA) methods with an F81-like model. Beyond Amazonas, remaining Brazilian states were aggregated in five discrete locations according to their geographic regions and sequences from other countries were aggregated in a single “non-Brazilian” location. Amazonian BCAR and BPAN clades were defined as those monophyletic clusters with high support (aLRT ≥ 0.85) that were mostly composed of sequences from Amazonas (>85%) and displayed Amazonas as the most probable (P ≥ 0.85) state location of its MRCA. Amazonian clades were further subdivided according to size into large (n > 30), medium (n = 10–30), and small (n = 2–9) clades.
The study of epidemiological and evolutionary parameters of major BCAR and BPANDEMIC clusters from Amazonas was done by Bayesian inference using coalescent and birth-death tree priors implemented, respectively, in BEAST v1.10 (Drummond et al., 2002; Suchard et al., 2018) with BEAGLE (Suchard and Rambaut, 2009) to improve run-time, and in BEAST 2.6 (Bouckaert et al., 2019) software packages. Dated phylogenies were inferred with the flexible Bayesian skyline coalescent model (Drummond, 2005). Changes across time in their effective sample size (Ne) were estimated using the coalescent Bayesian Skygrid (BSKG) model (Gill et al., 2012), and in their effective reproductive number (Re), using the Birth-death Skyline (BDSKY) model (Stadler et al., 2012). For BDSKY, the sampling rate (δ) was set to zero for the period before the oldest sample and estimated afterward. The Re was modeled in a piecewise manner in equidistant intervals from the most recent sample up to the root of the tree with a lognormal prior (mean = 0; standard deviation = 1), and the becoming non-infectious rate with a lognormal prior (mean = 0.25; standard deviation = 0.5). All Bayesian MCMC analyses were performed using the GTR + I + Γ4 nucleotide substitution model, and a relaxed uncorrelated lognormal molecular clock model (Drummond et al., 2006) with a uniform prior distribution on the substitution rate that encompasses mean values previously estimated for the subtype B pol gene (2.0–3.0 × 10–3 subst./site/year) (Hue et al., 2005; Zehender et al., 2010; Chen et al., 2011; Mendoza et al., 2014; Bello et al., 2018). MCMC chains were run for 50–100 × 106 generations and convergence and uncertainty of parameter estimates were assessed by calculating the effective sample size (ESS) and 95% highest probability density (HPD) values, respectively, after excluding the initial 10% of each run with Tracer v1.7.1 (Rambaut et al., 2018). The convergence of parameters was considered when ESS ≥ 200.
Demographic information of age group and gender of individuals with samples included in the present study were compared using Pearson’s chi-squared test as implemented in R version 3.6.3 (R Core Team, 2018), with 10,000 replicates. The false discovery rate (FDR) method was used to correct for multiple hypothesis testing and to reduce false positives. Statistical significance was defined as p-values <0.05.
The 1,272 HIV-1 subtype B pol sequences from Amazonas were assigned to either BCAR (23%) or BPAN (77%) lineages (Table 1). The sub-lineage assignment reveals that most BCAR Amazonian sequences belong to the major Brazilian clade BCAR–BR–I (89%), while the remaining sequences were classified within clades BCAR–BR–II (1%), BCAR–BR–III (1%), or branched outside known Brazilian clades (8%) (Table 1). Although a high proportion of BPAN Amazonian sequences also branched within major Brazilian BPAN clades (45%), particularly the BPAN–BR–I (37%), most pandemic sequences (55%) branched outside known countrywide Brazilian clades (Table 1). To identify the major subtype clades circulating in Amazonas, we next conducted independent ML phylogeographic analyses for: (i) sequences that branched within major Brazilian BCAR or BPAN clades, by combining Brazilian sequences sampled in Amazonas and other states, and (ii) sequences that branched outside major Brazilian clades, by combining Amazonian sequences with either BCAR sequences of Caribbean origin or BPAN sequences sampled in Brazil and worldwide. The ML phylogeographic analyses confirm multiple introductions of BCAR (n = 18) and BPAN (n = 291) variants into the Amazonas state (Supplementary Table 1). The major BCAR founder event resulted in the BCAR–BR–I clade (Figure 1) while the remaining BCAR sequences were distributed among a few local clusters of small size (2–9 sequences, 7%) or singletons (4%) (Supplementary Figures 1A–C). A few BPAN introductions (n = 4) originated highly supported (aLRT > 0.85) Amazonian BPAN clades of large size that were mostly composed by sequences from Amazonas (>85%) and most probably arose in Amazonas (P > 0.90). Two major clusters, BPAN–BR–I–AM–I (n = 39) and BPAN–BR–I–AM–II (n = 35), were nested within the large Brazilian clade BPAN–BR–I (Figure 2). The other two clusters, BPAN–AM–I (n = 86) and BPAN–AM–II (n = 60), branched outside the major BPAN Brazilian clades (Figure 3). Because the phylogenetic placement of some Amazonian basal sequences in clusters BPAN–AM–I and BPAN–AM–II changed according to the reference (Brazilian or worldwide) dataset, we define the final size of those clusters by the monophyletic groups supported by both analyses. The four major Amazonian BPAN clades, together, comprise 23% of all BPAN Amazonian sequences analyzed and the remaining sequences branched within Amazonian clades of medium (24%) or small (35%) size or appeared as singletons that branched with non-Amazonian sequences (18%) (Figures 2, 3 and Supplementary Figures 1D–F).
Figure 1. ML phylogeographic analysis of major cluster BCAR–BR–I disseminated in Brazil. A total of 296 BCAR–BR–I Brazilian sequences sampled in Amazonas (n = 267) and other states (n = 29) were analyzed. The number in parenthesis indicates the inferred marginal probability that the clade ancestor was located in the Amazonas state. The location of taxonomic units at internal nodes across the ML tree was reconstructed and represented according to the color scheme shown in the map. Outside Amazonas state, other Brazilian units were aggregated according to the country region. AM, Amazonas; N, North; SE, Southeast. The tree was rooted using HIV-1 subtype D reference sequences (not shown). The branch lengths are drawn to scale with the bar at the bottom indicating nucleotide substitutions per site. Major migrations of viral clade BCAR–BR–I are represented in the map.
Figure 2. ML phylogeographic analysis of major BPAN–BR–I cluster disseminated in Brazil. A total of 844 BPAN–BR–I Brazilian sequences sampled in Amazonas (n = 360) and other states from the Northern and Southeastern regions (n = 484) were analyzed together. We selected sequences from Northern and Southeastern states because were the regions more strongly connected with the Amazonas state. Major Amazonian clades BPAN–BR–I–AM–I and BPAN–BR–I–AM–II are indicated by colored shaded boxes along with cluster aLRT support (number at basal branch) and the inferred marginal probability that cluster ancestor was located in Amazonas state (in parentheses). The location of taxonomic units at internal nodes across the ML tree was reconstructed and represented according to the color scheme shown in the map. Sequences were grouped in three discrete locations: Amazonas state (AM), Northern region (N), and Southeastern region (SE). The tree was rooted using HIV-1 subtype D reference sequences (not shown). The branch lengths are drawn to scale with the bar at the bottom indicating nucleotide substitutions per site. Major migrations of viral clades BPAN–BR–I–AM–I and BPAN–BR–I–AM–II are represented in the map.
Figure 3. ML phylogeographic analysis of HIV-1 BPAN Amazonian sequences that branched outside major Brazilian clades. A total of 530 BPAN sequences sampled in Amazonas were aligned with: (A) Closely related BPAN sequences sampled in other Brazilian states (n = 687) plus reference BPAN sequences from the United States and France (n = 498), and (B) closely related BPAN sequences sampled worldwide (n = 1,683). Major Amazonian clades BPAN–AM–I and BPAN–AM–II are indicated by colored shaded boxes along with cluster aLRT support (number at basal branch) and the inferred marginal probability that cluster ancestor was located in Amazonas state (in parenthesis). The location of taxonomic units at internal nodes across the ML tree was reconstructed and represented according to the color scheme shown in the map. Sequences were grouped in seven (A) and three (B) discrete locations: Amazonas state (AM), Central-Western region (CW), Northern region (N); Northeastern region (NE), Southeastern region (SE), Southern region (S), United States/France (US/FR), other Brazilian states (BR), and non-Brazilian (Non-BR). Tree was rooted using HIV-1 subtype D reference sequences (not shown). The branch lengths are drawn to scale with the bar at the bottom indicating nucleotide substitutions per site. Major migrations of viral clades (C) BPAN–AM–I and (D) BPAN– AM–II are represented in the maps.
To study in more detail the evolutionary and demographic history of lineages BCAR and BPAN spreading in Amazonas, we selected the five major clades that display both local epidemic importance – as, combined, they comprise 38% of HIV-1 subtype B infections in the state – and adequate sample sizes to give reliable demographic estimates. Time-scaled trees were reconstructed using a Bayesian coalescent model with an informative clock rate prior due to the weak temporal structure of Amazonian subtype B pol datasets (Supplementary Figure 2). Posterior estimates, that were, as expected, largely influenced by the selected clock rate prior, traced the median TMRCA of major Amazonian clades to the late 1970s for BCAR–BR–I, the late 1980s for BPAN–AM–I, the mid-1990s for BPAN–AM–II, and the late 1990s for BPAN–BR–I–AM–I and BPAN–BR–I–AM–II (Table 2). These findings support that the major BCAR clade was successfully spreading in Amazonas for about 10 years before the emergence of the major BPAN clades, which may explain the singular high prevalence of non-pandemic subtype B variants in Amazonas with respect to most other Brazilian states. The BSKG model supports that the Ne of lineages BCAR–BR–I and BPAN–BR–I–AM–I steadily increased until recent years, while the Ne of lineages BPAN–AM–I, BPAN–AM–II, and BPAN–BR–I–AM–II increased until the late-2000s, but then stabilized in more recent years (Figures 4A–E). The temporal trajectories of the Re estimated using the Bayesian BDSKY model, however, support that all major Amazonian HIV-1 subtype B clades continuously expanded (median Re > 1) over all the studied period, with some temporal fluctuations in the rate of expansion (Figures 4A–E). The clade BCAR–BR–I reached the highest median Re (2.5–2.6) between the late 1970s and the early 1990s, while the BPAN clades reached the highest median Re (2.9–3.4) between the mid-1990s and the mid-2000s (Table 2). Despite those differences in the early phase of spread, all major Amazonian subtype B clades converge to the roughly similar median growth rate (Re = 1.6–2.3) at the most recent time period analyzed (2010–2018), with no evidence of recent epidemic stabilization (Re > 1) (Figure 4F).
Table 2. Bayesian estimates of evolutionary and demographic parameters of major HIV-1 subtype B clades originated in the Amazonas state.
Figure 4. Demographic history of subtype B cluster in Brazil’s Amazonas state. Each plot (A–E) details the demographic history of one subtype B large cluster (n ≥ 30) in Amazonas from the pandemic (BPAN) and non-pandemic (BCAR) forms. The graphs exhibit their effective number of HIV-1 infections under the Bayesian Skygrid (BSKG) model in blue (Ne, y-left-axis), and their effective reproductive number under the Birth-death Skyline (BDSKY) model in orange (Ne, y-right-axis). For both parameters are indicated their median (solid lines) and 95% HPD intervals (pale areas) estimates. A dashed vertical line indicates the TMRCA of the clades, accompanied by its median value. The last graph (F) compares the Re obtained for the five clusters in the last period of analysis (2010–2018). For each cluster, its median Re (solid line) and 95% HPD interval (pale area) inferred values are represented.
Previous studies demonstrate that the expanding HIV-1 subtype B epidemic in the Northern Brazilian state of Amazonas was driven by both pandemic (BPAN) and non-pandemic (BCAR) viral variants (Cabello et al., 2015; Divino et al., 2016; Arantes et al., 2019; Crispim et al., 2019; Chaves et al., 2021; Gräf et al., 2021), thus creating a great opportunity to compare the epidemic dynamics of both subtype B forms spreading in the same population. This study revealed that the BPAN and BCAR epidemics in Amazonas have been shaped by different evolutionary histories, but displayed very similar transmissibility and expansion dynamics at most recent times.
Our analysis confirmed that variants BCAR and BPAN were introduced multiple times in the Amazonas state, although the estimated number of BPAN introductions was 16 times higher than that of BCAR. The founder event that originated the clade BCAR–BR–I occurred in the late 1970s (Divino et al., 2016; Arantes et al., 2019) and gave rise to 89% of BCAR and 19% of total subtype B infections in Amazonas. In sharp contrast, most BPAN sequences from Amazonas branched into multiple state-specific clusters of medium/small size (57%) or appeared as unclustered infections (19%). The four largest BPAN Amazonian clades identified probably arose between the late 1980s and late 1990s and, together, comprise 23% of the BPAN and 17% of all subtype B infections in the state. These findings support that the early introduction (late 1970s) of the BCAR–BR–I ancestor in Amazonas from neighboring Caribbean countries probably drove its successful establishment and wide dissemination in the state. Although the BPAN strains arrived in Amazonas later, they reached a high prevalence because they were introduced at much higher numbers and spread through more transmission networks than BCAR strains.
The AIDS detection rate increased ∼10% in the Amazonas state between 2009 and 2019 (Ministério da Saúde, 2020). This finding is consistent with our BDSKY analyses that support a continuous expansion (Re > 1) of major BCAR and BPAN Amazonian clades over all the studied period. The BSKG model indicates a recent stabilization of some Amazonian BPAN clades since the late 2000s, and a previous study conducted by our group also indicated a recent epidemic stabilization of the clade BCAR–BR–I since the late 2000s (Arantes et al., 2019). Although the median estimated Re of the BCAR and BPAN Amazonian clades was somewhat lower between 2010 and 2018 (1.6–2.3) than during the previous decades (2.5–3.4), we found no solid evidence of epidemic stabilization or reduction in the BDSKY analyses. A previous study pointed out that the BSKG model requires strongly informative data to prevent erroneous estimates of Ne stabilization (Volz and Didelot, 2018). Thus, we hypothesize that the much larger number of recent (2009–2018) BCAR–BR–I sequences used in the present study (n = 267) compared to the previous one (n = 45) allowed us to obtain a more accurate demographic reconstruction of the epidemic pattern in the last two decades.
It is interesting to note that the BCAR (2.5–2.6) and BPAN (2.9–3.4) Amazonian clades reached similar highest median Re values. Furthermore, the highest median Re estimated here using a birth-death approach was comparable to the previous ones estimated for the BCAR–BR–I clade (3.8) using a coalescent-based approach (Arantes et al., 2019), but lower than those estimated for major BPAN Brazilian lineages spreading in the Southeastern region (5.0–7.9) (Mir et al., 2015). These findings support that differences in the spreading dynamics of subtype B lineages may reflect discrepancies in the connectivity of underlying transmission networks across different Brazilian states/regions, rather than intrinsic differences in viral transmissibility. A preliminary analysis of the available demographic data (sex and age) of HIV-infected subjects from Amazonas revealed no significant differences between major BCAR and BPAN clades (Supplementary Table 2), supporting that both viral lineages are possibly spreading through networks with similar epidemiological properties. This observation is also consistent with a previous study that revealed comparable epidemic growth rates of BCAR and BPAN lineages circulating in the French Guiana (Bello et al., 2018).
Our study has some limitations. First, inferences about potential sources, total number of viral introductions, and local clade size in Amazonas were limited by both the incomplete sampling of local population and the limited number of non-Amazonian reference sequences included in each ML phylogenetic analysis as revealed by the variable phylogenetic and phylogeographic placement of some Amazonian sequences that branched basal to each local clade. The bulk of BCAR and BPAN sequences that compose each major Amazonian clade, however, remained constant across analyses, and our major phylogeographic conclusions were robust to sampling bias. Second, time-scale reconstructions were largely influenced by the selected clock rate prior due to the weak temporal structure of Amazonian HIV-1 subtype B pol datasets. Despite this, the TMRCA here obtained were fully consistent with the overall time-scale of dissemination of the HIV-1 BCAR and BPAN lineages in the Americas and Brazil described in previous studies (Gilbert et al., 2007; Cabello et al., 2014; Mir et al., 2015; Worobey et al., 2016; Arantes et al., 2019; Bello et al., 2019). Finally, the lack of epidemiological data regarding the mode of transmission of individuals analyzed reduced the power of our study to confirm any association between the inferred rate of viral spread and the ecological characteristics of local transmission networks in Amazonas.
In summary, this study highlights that the HIV-1 epidemic in the Amazonas state mostly results from the local expansion of one BCAR strain (BCAR–BR–I) introduced around the late 1970s and of multiple BPAN viral strains introduced since the late 1980s. Albeit the earlier introduction of the BCAR–BR–I clade granted a much prolonged period of local spread than that of the BPAN strains, this was compensated by a much higher number of independent introductions and the concurrent establishment of multiple BPAN local transmission networks. Despite significant differences in the pattern of early establishment, major BCAR and BPAN clades circulating in Amazonas have been spreading at a quite similar rate over the last two decades, arguing against the hypothesis of significant differences in their intrinsic transmissibility. Our analyses also demonstrate that major Amazonian BCAR and BPAN clades continued to spread and showed no clear signs of recent epidemic stabilization, supporting the relevance of designing more effective strategies to prevent HIV transmission in the region.
Publicly available datasets were analyzed in this study. This data can be found here: GenBank accession numbers: KEXV01000001 to KEXV01046877; HQ127524 to HQ127607; KU762066 to KU762066; MH673055 to MH673280; and MW545333 to MW545424; Los Alamos HIV Sequence Database (http://www.hiv.lanl.gov).
Ethical review and approval were not required for the study on human participants in accordance with the Local Legislation and Institutional Requirements. Written informed consent for participation was not required for this study in accordance with the National Legislation and the Institutional Requirements.
GB conceived and designed the study and supervised the experiments. IA conducted the experiments and analyzed the data. YO, TG, and MG provided HIV-1 sequence data and intellectual input. IA and GB wrote the first draft of the manuscript. All authors assisted with the writing and approved the final manuscript.
GB was supported by fundings from CNPq (Grant No. 304883/2020-4) and Fundação de Amparo à Pesquisa do Estado do Rio de Janeiro (FAPERJ, Grant No. E-26/202.896/2018). IA was funded by a fellowship from FAPERJ and Fundação Oswaldo Cruz (FIOCRUZ).
The authors declare that the research was conducted in the absence of any commercial or financial relationships that could be construed as a potential conflict of interest.
All claims expressed in this article are solely those of the authors and do not necessarily represent those of their affiliated organizations, or those of the publisher, the editors and the reviewers. Any product that may be evaluated in this article, or claim that may be made by its manufacturer, is not guaranteed or endorsed by the publisher.
This study was carried out with public data provided by the Department of Diseases of Chronic Condition and Sexually Transmitted Infections of the Secretariat for Health Surveillance of the Ministry of Health. We would like to thank the reviewers for their valuable and insightful comments toward improving our manuscript.
The Supplementary Material for this article can be found online at: https://www.frontiersin.org/articles/10.3389/fmicb.2022.835443/full#supplementary-material
Anisimova, M., and Gascuel, O. (2006). Approximate likelihood-ratio test for branches: a fast, accurate, and powerful alternative. Syst. Biol. 55, 539–552. doi: 10.1080/10635150600755453
Arantes, I., Esashika Crispim, M. A., Nogueira da Guarda Reis, M., Martins Araújo Stefani, M., and Bello, G. (2019). Reconstructing the dissemination dynamics of the major HIV-1 subtype B non-pandemic lineage circulating in brazil. Viruses 11:909. doi: 10.3390/v11100909
Arantes, I., Ribeiro-Alves, M., de Azevedo, S. S. D., Delatorre, E., and Bello, G. (2020). Few amino acid signatures distinguish HIV-1 subtype B pandemic and non-pandemic strains. PLoS One 15:e0238995. doi: 10.1371/journal.pone.0238995
Bello, G., Arantes, I., Lacoste, V., Ouka, M., Boncy, J., Césaire, R., et al. (2019). Phylogeographic Analyses Reveal the Early Expansion and Frequent Bidirectional Cross-Border Transmissions of Non-pandemic HIV-1 Subtype B Strains in Hispaniola. Front. Microbiol. 10:1340. doi: 10.3389/fmicb.2019.01340
Bello, G., Nacher, M., Divino, F., Darcissac, E., Mir, D., and Lacoste, V. (2018). The HIV-1 subtype B epidemic in french guiana and suriname is driven by ongoing transmissions of pandemic and non-pandemic lineages. Front. Microbiol. 9:1738. doi: 10.3389/fmicb.2018.01738
Bouckaert, R., Vaughan, T. G., Barido-Sottani, J., Duchêne, S., Fourment, M., Gavryushkina, A., et al. (2019). BEAST 2.5: an advanced software platform for Bayesian evolutionary analysis. PLoS Comput. Biol. 15:e1006650. doi: 10.1371/journal.pcbi.1006650
Cabello, M., Junqueira, D. M., and Bello, G. (2015). Dissemination of nonpandemic Caribbean HIV-1 subtype B clades in Latin America. AIDS 29, 483–492. doi: 10.1097/qad.0000000000000552
Cabello, M., Mendoza, Y., and Bello, G. (2014). Spatiotemporal dynamics of dissemination of non-pandemic HIV-1 subtype B clades in the caribbean region. PLoS One 9:e106045. doi: 10.1371/journal.pone.0106045
Chaves, Y. O., Pereira, F. R., de Souza Pinheiro, R., Batista, D. R. L., da Silva Balieiro, A. A., de Lacerda, M. V. G., et al. (2021). High detection rate of HIV drug resistance mutations among patients who fail combined antiretroviral therapy in manaus, brazil. BioMed. Res. Int. 2021:5567332. doi: 10.1155/2021/5567332
Chen, J. H. K., Wong, K. H., Chan, K. C. W., To, S. W. C., Chen, Z., and Yam, W. C. (2011). Phylodynamics of HIV-1 subtype B among the men-having-sex-with-men (MSM) population in Hong Kong. PLoS One 6:e25286. doi: 10.1371/journal.pone.0025286
Crispim, M. A. E., Reis, M. N. D. G., Abrahim, C., Kiesslich, D., Fraiji, N., Bello, G., et al. (2019). Homogenous HIV-1 subtype B from the Brazilian Amazon with infrequent diverse BF1 recombinants, subtypes F1 and C among blood donors. PLoS One 14:e0221151. doi: 10.1371/journal.pone.0221151
Divino, F., de Lima Guerra Corado, A., Gomes Naveca, F., Stefani, M. M. A., and Bello, G. (2016). High prevalence and onward transmission of non-pandemic HIV-1 subtype B clades in northern and northeastern brazilian regions. PLoS One 11:e0162112. doi: 10.1371/journal.pone.0162112
Drummond, A. J. (2005). Bayesian coalescent inference of past population dynamics from molecular sequences. Mol. Biol. Evol. 22, 1185–1192. doi: 10.1093/molbev/msi103
Drummond, A. J., Ho, S. Y. W., Phillips, M. J., and Rambaut, A. (2006). Relaxed phylogenetics and dating with confidence. PLoS Biol. 4:e88. doi: 10.1371/journal.pbio.0040088
Drummond, A. J., Nicholls, G. K., Rodrigo, A. G., and Solomon, W. (2002). Estimating mutation parameters, population history and genealogy simultaneously from temporally spaced sequence data. Genetics 161, 1307–1320. doi: 10.1093/genetics/161.3.1307
Gilbert, M. T. P., Rambaut, A., Wlasiuk, G., Spira, T. J., Pitchenik, A. E., and Worobey, M. (2007). The emergence of HIV/AIDS in the Americas and beyond. Proc. Natl. Acad. Sci. 104, 18566–18570. doi: 10.1073/pnas.0705329104
Gill, M. S., Lemey, P., Faria, N. R., Rambaut, A., Shapiro, B., and Suchard, M. A. (2012). Improving bayesian population dynamics inference: a coalescent-based model for multiple loci. Mol. Biol. Evol. 30, 713–724. doi: 10.1093/molbev/mss265
Gräf, T., Bello, G., Andrade, P., Arantes, I., Pereira, J. M., da Silva, A. B. P., et al. (2021). HIV-1 molecular diversity in Brazil unveiled by 10 years of sampling by the national genotyping network. Sci. Rep. 11:15842. doi: 10.1038/s41598-021-94542-5
Guindon, S., Dufayard, J. F., Lefort, V., Anisimova, M., Hordijk, W., and Gascuel, O. (2010). New algorithms and methods to estimate maximum-likelihood phylogenies: assessing the performance of phyml 3.0. Syst. Biol. 59, 307–321. doi: 10.1093/sysbio/syq010
Guindon, S., Lethiec, F., Duroux, P., and Gascuel, O. (2005). PHYML Online–a web server for fast maximum likelihood-based phylogenetic inference. Nucleic Acids Res. 33, W557–W559. doi: 10.1093/nar/gki352
Hue, S., Pillay, D., Clewley, J. P., and Pybus, O. G. (2005). Genetic analysis reveals the complex structure of HIV-1 transmission within defined risk groups. Proc. Natl. Acad. Sci. 102, 4425–4429. doi: 10.1073/pnas.0407534102
Ishikawa, S. A., Zhukova, A., Iwasaki, W., andGascuel, O. (2019). A Fast Likelihood Method to Reconstruct and Visualize Ancestral Scenarios. Mol. Biol. Evol. 36, 2069–2085. doi: 10.1093/molbev/msz131
Larkin, M. A., Blackshields, G., Brown, N. P., Chenna, R., McGettigan, P. A., McWilliam, H., et al. (2007). Clustal W and Clustal X version 2.0. Bioinformatics 23, 2947–2948. doi: 10.1093/bioinformatics/btm404
Mendoza, Y., Martínez, A. A., Castillo Mewa, J., González, C., García-Morales, C., Avila-Ríos, S., et al. (2014). Human immunodeficiency virus type 1 (HIV-1) subtype B epidemic in Panama is mainly driven by dissemination of country-specific clades. PLoS One 9:e95360. doi: 10.1371/journal.pone.0095360
Ministério da Saúde (2020). Boletim Epidemiológico HIV/AIDS 2020. Available online at: http://www.aids.gov.br/system/tdf/pub/2016/67456/boletim_hiv_aids_2020_com_marcas_2.pdf?file=1&type=node&id=67456&force=1 (accessed December 14, 2021).
Mir, D., Cabello, M., Romero, H., and Bello, G. (2015). Phylodynamics of major HIV-1 subtype B pandemic clades circulating in Latin America. AIDS 29, 1863–1869. doi: 10.1097/qad.0000000000000770
Posada, D. (2008). jModelTest: phylogenetic model averaging. Mol. Biol. Evol. 25, 1253–1256. doi: 10.1093/molbev/msn083
Pupko, T., Pe, I., Shamir, R., and Graur, D. (2000). A fast algorithm for joint reconstruction of ancestral amino acid sequences. Mol. Biol. Evol. 17, 890–896. doi: 10.1093/oxfordjournals.molbev.a026369
R Core Team (2018). R: A Language and Environment for Statistical Computing. Vienna: R Foundation for Statistical Computing. Available online at: https://www.R-project.org
Rambaut, A. (2009). FigTree v1.4: Tree Figure Drawing Tool. Available online at: http://tree.bio.ed.ac.uk/software/figtree/ (accessed on 25 Nov, 2018)
Rambaut, A., Drummond, A. J., Xie, D., Baele, G., and Suchard, M. A. (2018). Posterior summarization in bayesian phylogenetics using tracer 1.7. Syst. Biol. 67, 901–904. doi: 10.1093/sysbio/syy032
Stadler, T., Kuhnert, D., Bonhoeffer, S., and Drummond, A. J. (2012). Birth-death skyline plot reveals temporal changes of epidemic spread in HIV and hepatitis C virus (HCV). Proc. Natl. Acad. Sci. 110, 228–233. doi: 10.1073/pnas.1207965110
Stamatakis, A. (2014). RAxML version 8: a tool for phylogenetic analysis and post-analysis of large phylogenies. Bioinformatics 30, 1312–1313. doi: 10.1093/bioinformatics/btu033
Suchard, M. A., Lemey, P., Baele, G., Ayres, D. L., Drummond, A. J., and Rambaut, A. (2018). Bayesian phylogenetic and phylodynamic data integration using BEAST 1.10. Vir. Evol. 4:vey016. doi: 10.1093/ve/vey016
Suchard, M. A., and Rambaut, A. (2009). Many-core algorithms for statistical phylogenetics. Bioinformatics 25, 1370–1376. doi: 10.1093/bioinformatics/btp244
Volz, E. M., and Didelot, X. (2018). Modeling the growth and decline of pathogen effective population size provides insight into epidemic dynamics and drivers of antimicrobial resistance. Syst. Biol. 67, 719–728. doi: 10.1093/sysbio/syy007
Worobey, M., Watts, T. D., McKay, R. A., Suchard, M. A., Granade, T., Teuwen, D. E., et al. (2016). 1970s and ‘Patient 0’ HIV-1 genomes illuminate early HIV/AIDS history in North America. Nature 539, 98–101. doi: 10.1038/nature19827
Keywords: HIV-1, subtype B, Brazil, Amazonas, phylodynamics
Citation: Arantes I, Gräf T, Andrade P, Oliveira Chaves Y, Guimarães ML and Bello G (2022) Dissemination Dynamics of HIV-1 Subtype B Pandemic and Non-pandemic Lineages Circulating in Amazonas, Brazil. Front. Microbiol. 13:835443. doi: 10.3389/fmicb.2022.835443
Received: 14 December 2021; Accepted: 14 February 2022;
Published: 07 March 2022.
Edited by:
Michael M. Thomson, Instituto de Salud Carlos III (ISCIII), SpainReviewed by:
Juan Angel Patino Galindo, Columbia University, United StatesCopyright © 2022 Arantes, Gräf, Andrade, Oliveira Chaves, Guimarães and Bello. This is an open-access article distributed under the terms of the Creative Commons Attribution License (CC BY). The use, distribution or reproduction in other forums is permitted, provided the original author(s) and the copyright owner(s) are credited and that the original publication in this journal is cited, in accordance with accepted academic practice. No use, distribution or reproduction is permitted which does not comply with these terms.
*Correspondence: Gonzalo Bello, Z2JlbGxvQGlvYy5maW9jcnV6LmJy
Disclaimer: All claims expressed in this article are solely those of the authors and do not necessarily represent those of their affiliated organizations, or those of the publisher, the editors and the reviewers. Any product that may be evaluated in this article or claim that may be made by its manufacturer is not guaranteed or endorsed by the publisher.
Research integrity at Frontiers
Learn more about the work of our research integrity team to safeguard the quality of each article we publish.