- 1College of Animal Husbandry and Veterinary Medicine, Shenyang Agricultural University, Shenyang, China
- 2Yichun Tequ Feed Company, Yichun, China
- 3Fujian Syno Biotech Co., Ltd., Fuzhou, China
- 4School of Environmental and Rural Science, University of New England, Armidale, NSW, Australia
Abnormally low or high levels of trace elements in poultry diets may elicit health problems associated with deficiency and toxicity, and impact poultry growth. The optimal supplement pattern of trace mineral also impacts the digestion and absorption in the body. For ducks, the limited knowledge of trace element requirements puzzled duck production. Thus, the objective of this study was to investigate the influence of dietary inclusions of coated and uncoated trace minerals on duck growth performance, tissue mineral deposition, serum antioxidant status, and intestinal microbiota profile. A total of 1,080 14-day-old Cherry Valley male ducks were randomly divided into six dietary treatment groups in a 2 (uncoated or coated trace minerals) × 3 (300, 500, or 1,000 mg/kg supplementation levels) factorial design. Each treatment was replicated 12 times (15 birds per replicate). Coated trace minerals significantly improved average daily gain (p < 0.05), increased Zn, Se, and Fe content of serum, liver, and muscle, increased serum antioxidant enzyme (p < 0.05) and decreased the excreta Fe, Zn, and Cu concentrations. Inclusions of 500 mg/kg of coated trace minerals had a similar effect on serum trace minerals and tissue metal ion deposition as the 1,000 mg/kg inorganic trace minerals. Higher concentrations of Lactobacillus, Sphaerochatea, Butyricimonas, and Enterococcus were found in birds fed with coated trace minerals. In conclusion, diets supplemented with coated trace minerals could reduce the risk of environmental contamination from excreted minerals without affecting performance. Furthermore, coated trace minerals may improve the bioavailability of metal ions and the colonization of probiotic microbiota to protect microbial barriers and maintain gut health.
Introduction
The capacity of dietary metal ions to increase the productivity of poultry is gaining recognition. Essential trace minerals, such as zinc (Zn), iron (Fe), copper (Cu), manganese (Mn), and selenium (Se), play critical roles in the nutrition and physiology of livestock, and are provided through the diet. Abnormally low or high levels of trace elements in the body may elicit health problems associated with deficiency and toxicity, and may thereby impact poultry growth. To satisfy the trace mineral requirements of poultry, dietary supplementation of inorganic trace minerals is widely used in commercial production. This supplementation frequently oversupplies trace minerals in an attempt to avoid deficiency; however, this practice increases the excretion of minerals causing environmental contamination (Mézes et al., 2012). Yang et al. (2021) reported that avoidance of under- and overprovision of dietary minerals will achieve optimal growth performance and reduce mineral excretion. There is currently an imbalance between the development of poultry production and research in trace minerals, leading to a limited knowledge of trace mineral requirements (Bao and Choct, 2009). According to National Research Council (1994), the duck’s requirement for trace minerals was estimated based on other poultry requirements. Therefore, it is important to re-evaluate the trace mineral requirement specifically for ducks.
Furthermore, previous studies indicated that using a carbohydrate matrix to encapsulate trace minerals (coated trace minerals) protects the metal ions, reducing mineral excretion, and improving broiler growth performance (Lu et al., 2020; Yin et al., 2021). However, information on the effects of coated trace minerals in ducks is lacking.
Finally, previous reports only explore trace mineral requirements for optimal growth but do not explore the effect of trace minerals on the gut and general health of the duck (Likittrakulwong et al., 2021). Nevertheless, the concentration of minerals in tissues and blood can reflect the mineral requirements of animal (Burrow et al., 2020). Furthermore, gut microorganisms contain thousands of functionally relevant genes and pathways to support intestinal functions, protect intestinal health, and modify environmental conditions (Pajarillo et al., 2021). The competitive relationship between the host and microbiome provides an expanding field of research. Becker and Skaar (2014) reported that intestinal microbiota could inhibit host trace mineral absorption, while the host developed mechanisms to sequester trace metals and prevent bacterial access. Previous studies also showed that several gut bacteria and pathogens have the ability to transport, metabolize, and use trace minerals for survival. As Andrews et al. (2003) and Braun and Hantke (2011) reported, bacteria may bind their own or neighbor’s siderophores to transport iron across bacterial cell walls to extract metal iron concentrations. Understanding the underlying modification of trace minerals on duck intestinal microbiota is therefore important.
Therefore, the objective of this study was to investigate the effects of coated and uncoated trace minerals at three concentrations on productive performance, mineral absorption, tissue deposition, and cecal microbiota in ducks.
Materials and Methods
Experimental Design
The experimental design consisted of a 2 × 3 factorial arrangement with two types of trace minerals (noncoated and coated) and three levels (300, 500, and 1,000 mg/kg). The coated trace minerals were commercially produced and provided by Fujian Syno Biotech Co., Ltd.
A total of 1,080 14-day-old Cherry Valley male ducks were randomly divided into six treatment groups, each with 12 replicates of 15 ducks per replicate cage. Dietary treatments were applied from 14 to 42 days post hatch (From 1 to 13 days, birds were fed with the same commercial diets with 1,000 mg/kg trace minerals. At 14 days, the similar weight ducks were selected). The composition and nutrient levels of the experimental diets are shown in Table 1. The nutrient values met the recommendations of NY/T 2122-2012 (Ministry of Agriculture of the People’s Republic of China, 2012). Trace mineral content of diets are given in Supplementary Table S1. The ducks were reared in cages (2.2 m × 1.2 m × 0.9 m) in a temperature- and humidity-controlled room and allowed ad libitum access to feed and water. Total feed consumption and body weight for each replicate cage of birds were recorded at 14 and 42 days post-hatch. Average daily feed intake (ADFI), average daily weight gain (ADG) in weight, and feed conversion ratio (FCRs) were determined over the experimental period.
Sample Collection
On day 42, six birds per treatment were randomly selected, and blood samples were drawn from the brachial vein. Serum samples were centrifuged at 1,500 × g at 4°C for 20 min and stored at −20°C until further analysis. Then the selected birds were euthanized by intravenous administration of sodium pentobarbital (50 mg/kg of body weight) into the wing vein. One lobe of the liver and breast muscle samples were immediately frozen in liquid nitrogen and stored at −80°C before analysis.
Nutrient Digestibility
For the determination of apparent crude protein, crude fat, calcium, and phosphorus digestibility, excreta from each pen was collected and weighed for a 78-h period from day 39 to day 42 post-hatch and dried for analysis and to determine excreta dry matter. Feed and excreta samples were then ground to pass through a 40-mesh screen and analyzed for crude protein (CP; method 990.03; AOAC, 2000), ether extract (EE; method 920.39; AOAC, 2000) and crude fiber (CF; method 978.10; AOAC, 2000). Calcium and phosphorus determination was carried out according to the method of David et al. (2019).
Mineral Concentration
A quarter of the total feces content collected was dried at 65°C for 48 h, broken up, and sifted to remove feathers. Then the fecal sample was ground to pass through a 1-mm screen after samples achieved constant weight and packed in self-locked plastic bags and stored at −20°C for analysis. Liver and breast muscle samples were freeze dried and finely ground using a 1-mm screen. Serum sample was deproteinated by 10% (w/v) trichloracetic acid. The Fe, Mn, Zn, and Cu concentrations in fecal samples, tissues, and serum were analyzed using an atomic absorption spectrophotometer (Z-8200, Hitachi, Tokyo, Japan). Selenium concentration was analyzed after wet ashing with nitric and perchloric acids and measured by the fluorometric method (method 996.16; AOAC, 2000).
Antioxidant Indices
The activity of Cu/Zn superoxide dismutase (Cu/Zn-SOD), glutathione peroxidase (GSH-Px), malondialdehyde (MDA), and total antioxidant capacity (T-AOC) content in serum were determined using the colorimtric method, TBA method, an r-911 automatic radioactivity meter (7,160, Hitachi, Japan), and light amide method, respectively, following the instructions of the manufacturer (Jiangsu Baolai Biotechnology Co. Ltd.).
Analysis of the Cecal Microbiota Community by 16S rRNA Analysis
The cecal contents from 12 birds were collected, then mixed in pairs, and treated as one sample. Cecal digesta were immediately frozen in liquid nitrogen and stored at −80°C for DNA isolation. The total genome DNA from the cecal samples was extracted using QIAamp DNA stool Mini Kit (Qiagen, Germany) according to the protocol of the manufacturer. DNA was quantified in a NanoDrop ND-1000 spectrophotometer (Thermo Scientific, Waltham, MA, United States) and stored at −80°C until processed for amplification. The construct 16S rRNA sequencing libraries, the V3–V4 regions of the 16S rRNA gene, were amplified from the DNA samples by PCR using the primer set of 338F (5′-ACTCCTACGGGAGGCAGCAG-3′) and 806R (5′-GGACTACHVGGGTWTCTAAT-3′) by the thermocycler PCR system (GeneAmp 9,700, ABI, United States). PCR reactions were performed in triplicate in a 20-μl mixture containing 4 μl of 5× FastPfu Buffer, 2 μl of 2.5 mM dNTPs, 0.8 μl of each primer (5 μM), 0.4 μl of FastPfu Polymerase, and 10 ng of template DNA. The PCR reactions were conducted using the following program, 3 min of denaturation at 95°C, 30 cycles of 30 s at 95°C, 30 s of annealing at 55°C, and 45 s of elongation at 72°C, and a final extension at 72°C for 10 min. The resulting PCR products were extracted from a 2% agarose gel and further purified using the AxyPrep DNA Gel Extraction Kit (Axygen Biosciences, Union City, CA, United States) and quantified using QuantiFluor™-ST (Promega, United States) according to the protocol of the manufacture. Finally, the libraries were sequenced on an Illumina Hiseq 2500 platform, and 250-bp paired-end reads were generated.
Sequence Processing
Raw data FASTQ files were imported into the format, which could be operated by QIIME2 system using QIIME tools import program. Demultiplexed sequences from each sample were quality filtered and trimmed, de-noised, merged, and then the chimeric sequences were identified and removed using the QIIME2 dada2 plugin to obtain the feature table of amplicon sequence variant (ASV; Callahan et al., 2016). The QIIME2 feature-classifier plugin was then used to align ASV sequences to a pre-trained GREENGENES 13_8 99% database to generate the taxonomy (Bokulich et al., 2018). As usual, the ASV with 99% similarity was classified as one operational taxonomic unit (OTU) to obtain the OTU classification information.
Bioinformatics Analysis
The alpha-diversity analysis was calculated, including microbial community richness parameters (Chao1), and community diversity parameters (Shannon diversity index and Simpson indices). The differences among treatments for α-diversity was calculated according to a Kruskal–Wallis multiple comparison followed by Wilcoxon’s pairwise comparison for significantly different groups. Beta-diversity distance measurements were calculated and visualized via principal coordinate analysis (PCoA). Similarity analysis (permutation multivariate analysis, PERMANOVA; Analysis of similarities, ANOSIM) tests were conducted based on beta diversity.
To identify differential microbial species among the different treatments, the linear discriminant analysis and effect size (LEfSe) analysis based on the linear discriminant analysis (LDA) were used, and the significant differences with an LDA score >2.0 as the critical value were assessed. The redundancy analysis method (RDA) and Spearman’s analysis were used to analyze the potential association between the intestinal flora and environmental variables based on relative abundances of microbial species at order and genus levels using the R package “vegan.”
Function Analysis
The potential Kyoto Encyclopedia of Genes and Genomes (KEGG) functional profiles of microbial communities were predicted with PICRUSt2 and annotated by the MetaCyc and ENZYME database. In KEGG level 3 pathway analysis, the enrichment of each pathway was converted to a Z-score.
Statistical Analysis
Data derived from ducks in 12 replicate cages per treatment were analyzed as a 2 × 3 factorial arrangement (two-way analysis of variance) using IBM SPSS Statistics 20.0 (IBM Corporation, Somers, NY, United States). Probability values p ≤ 0.05 were considered significant.
Results
Growth Performance
The influence of dietary treatments on growth performance is shown in Table 2. Trace minerals significantly affected ADG (p < 0.05), wherein the coated trace minerals had a more pronounced effect than uncoated minerals, and ADG increased with greater supplementation of trace minerals. Dietary treatments had no significant influence on ADFI and FCR. There was no significant interaction between dietary supplementation pattern and supplementation level of trace minerals on growth performance.
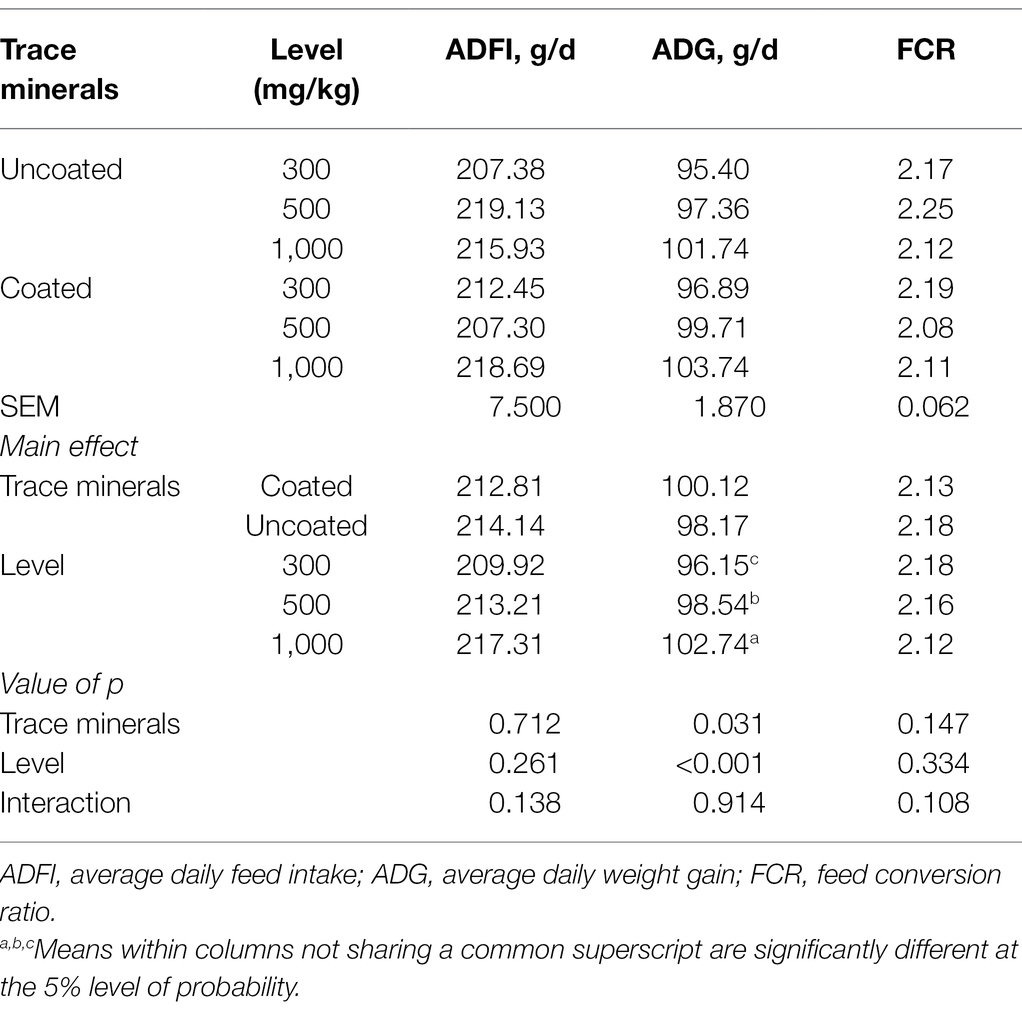
Table 2. Effects of trace mineral type (coated or uncoated) and supplementation level (300, 500, or 1,000 mg/kg) of trace minerals on the growth performance of ducks.
Nutrient Digestibility
The influence of dietary treatments on total tract nutrient digestibility in ducks at 42 days is shown in Table 3. There was a significant main effect where coated trace minerals significantly increased phosphorus digestibility compared with uncoated trace minerals. Additionally, CP digestibility in birds fed with 1,000 mg/kg of trace minerals was higher than those fed with 300 and 500 mg/kg. While not significantly different, there is a tendency that 300 mg/kg of trace minerals had a lower EE (p = 0.051) and CF (p = 0.088) digestibility than 500 and 1,000 mg/kg of trace minerals.
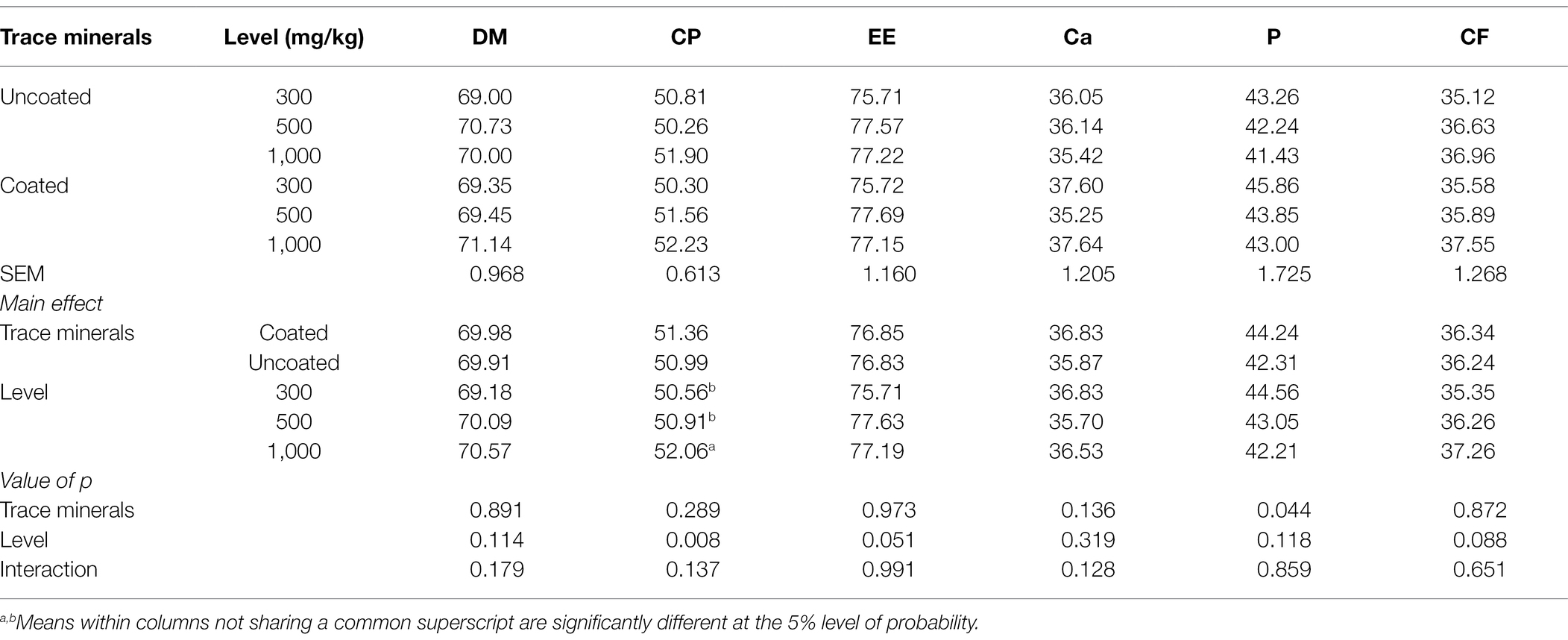
Table 3. Effects of trace mineral type (coated or uncoated) and supplementation level (300, 500, or 1,000 mg/kg) of trace minerals on the total tract nutrient digestibility of ducks.
Tissue Mineral Deposition
Table 4 shows the influence of dietary treatments on liver mineral deposition. There was a significant interaction of liver Se and Mn content. The supplementation of 500 mg/kg of coated trace minerals had the highest liver Se concentration among the dietary treatments. The highest liver Mn concentration was found in 1,000 mg/kg of coated trace minerals. Liver Zn and Cu significantly increased with greater supplementation of trace minerals. Birds fed with 1,000 mg/kg of trace minerals had the lowest liver Fe content. There is a tendency that birds fed with coated trace minerals had an increased liver Zn (p = 0.053) and Fe (p = 0.071) content.
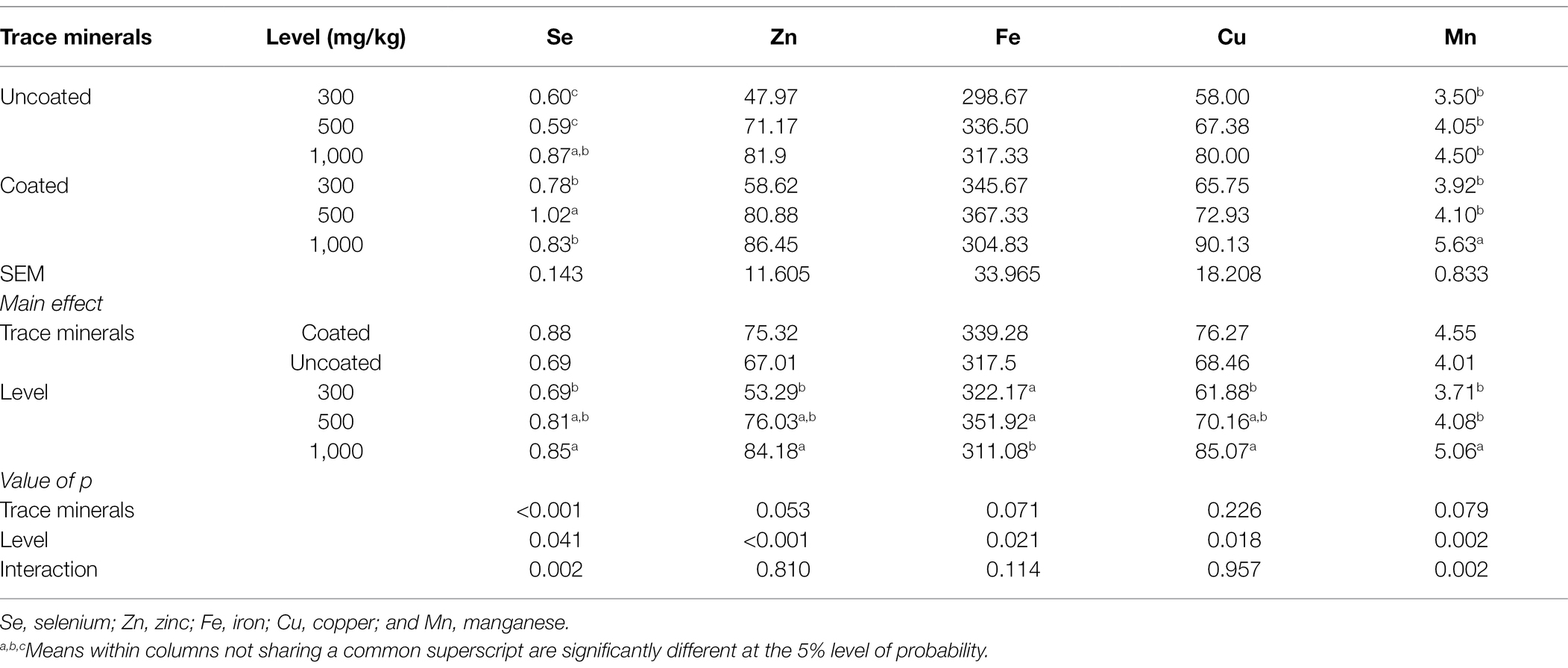
Table 4. Effects of trace mineral type (coated or uncoated) and supplementation level (300, 500, or 1,000 mg/kg) of trace minerals on liver mineral deposition in ducks (mg/kg).
Table 5 shows the influence of dietary treatments on mineral deposition in muscle.
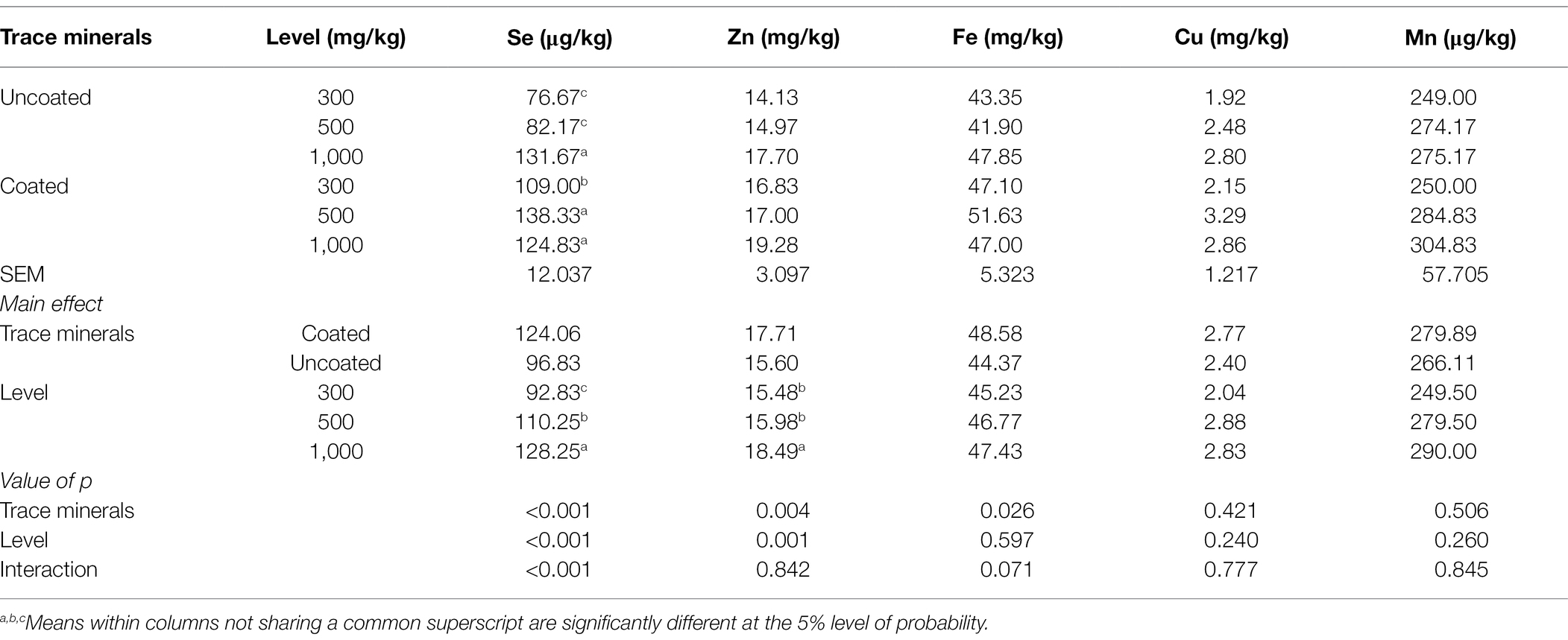
Table 5. Effects of trace minerals type (coated or uncoated) and supplementation level (300, 500, or 1,000mg/kg) of muscle mineral deposition in ducks.
There is a significant interaction for muscle Se content where 500 mg/kg of coated trace minerals generated the highest muscle concentration of Se. Coated trace minerals generated the highest muscle concentrations of Zn and Fe, and 1,000 mg/kg of trace mineral inclusion generated the highest Zn concentration in muscle.
Fecal Mineral Contents
The effects of dietary treatments on fecal mineral excretion are presented in Table 6. There was a significant interaction for Fe, where 1,000 mg/kg of uncoated trace mineral generated the greatest amount of Fe in excreta, whereas 300 mg/kg of uncoated trace mineral generated the lowest. Se, Zn, and Mn concentrations in feces increased with greater supplementation of trace minerals. Uncoated trace minerals generated greater concentration of Zn in excreta, but coated trace minerals generated a greater concentration of Mn within excreta.
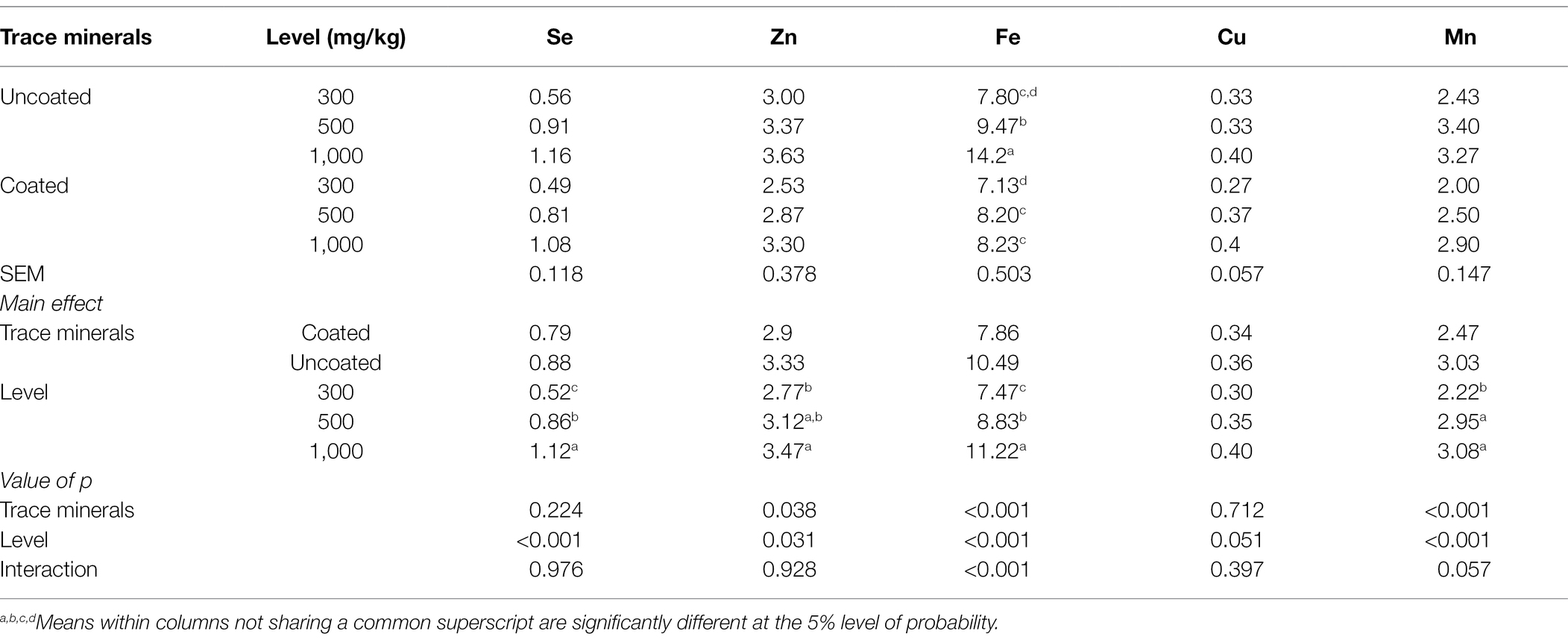
Table 6. Effects of trace mineral type (coated or uncoated) and supplementation level (300, 500, or 1,000 mg/kg) of trace minerals on fecal excretion ion metals contents in ducks (mg/kg).
Serum Mineral Levels
The effects of dietary treatments on serum Se, Zn, Fe, Cu, and Mn concentrations are given in Table 7. There was a significant interaction where 500 mg/kg of coated trace minerals generated the greatest concentration of Se in serum. Zn and Fe serum concentrations were increased with greater trace mineral supplementation. Coated trace minerals increased Zn in serum.
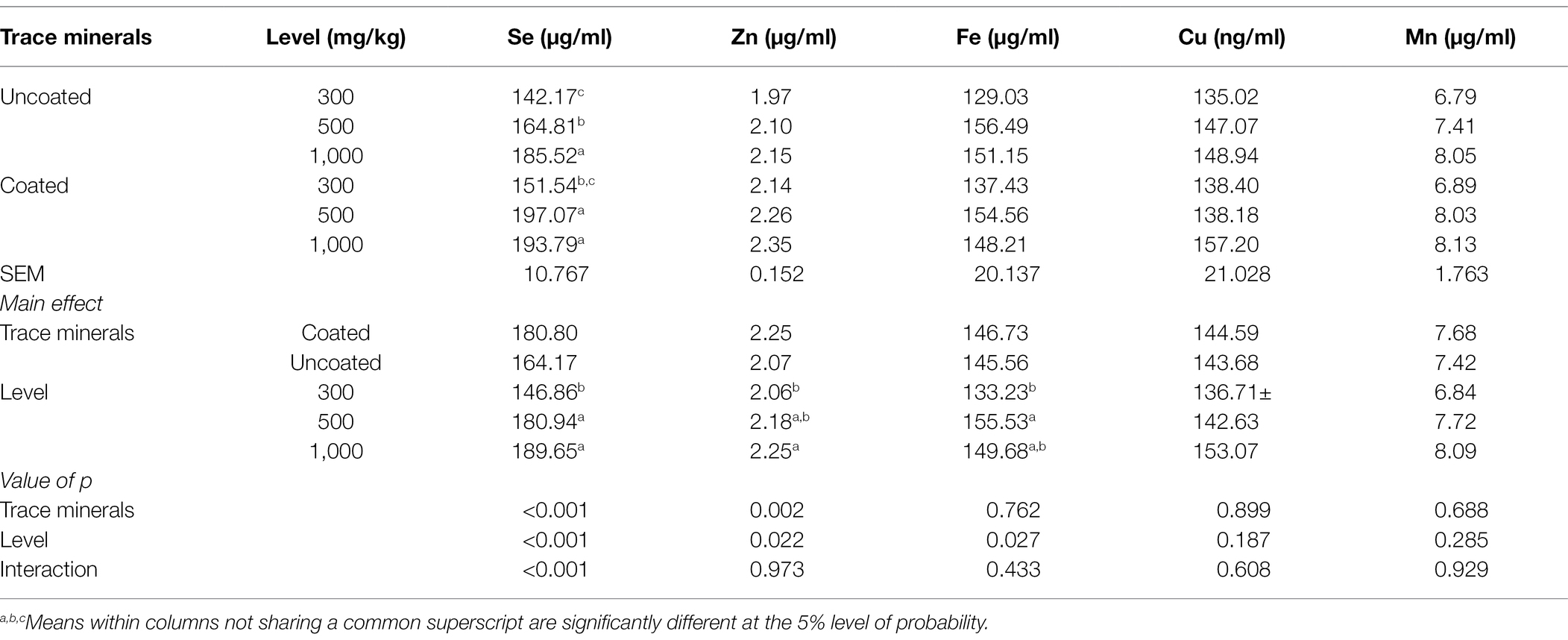
Table 7. Effects of trace mineral type (coated or uncoated) and supplementation level (300, 500, or 1,000 mg/kg) of trace minerals on serum ion metals contents in ducks.
Antioxidant Status
The effects of dietary treatments on serum antioxidant status are shown in Table 8. Significant interactions were found in serum MDA content and GSH-Px activity where ducks offered 500 mg/kg of coated trace minerals resulted in the lowest serum MDA content and highest serum GSH-Px activity. Coated trace minerals and the increasing supplementation of dietary trace minerals significantly improved serum SOD activities. Dietary treatments had no significant influence on serum T-AOC activity.
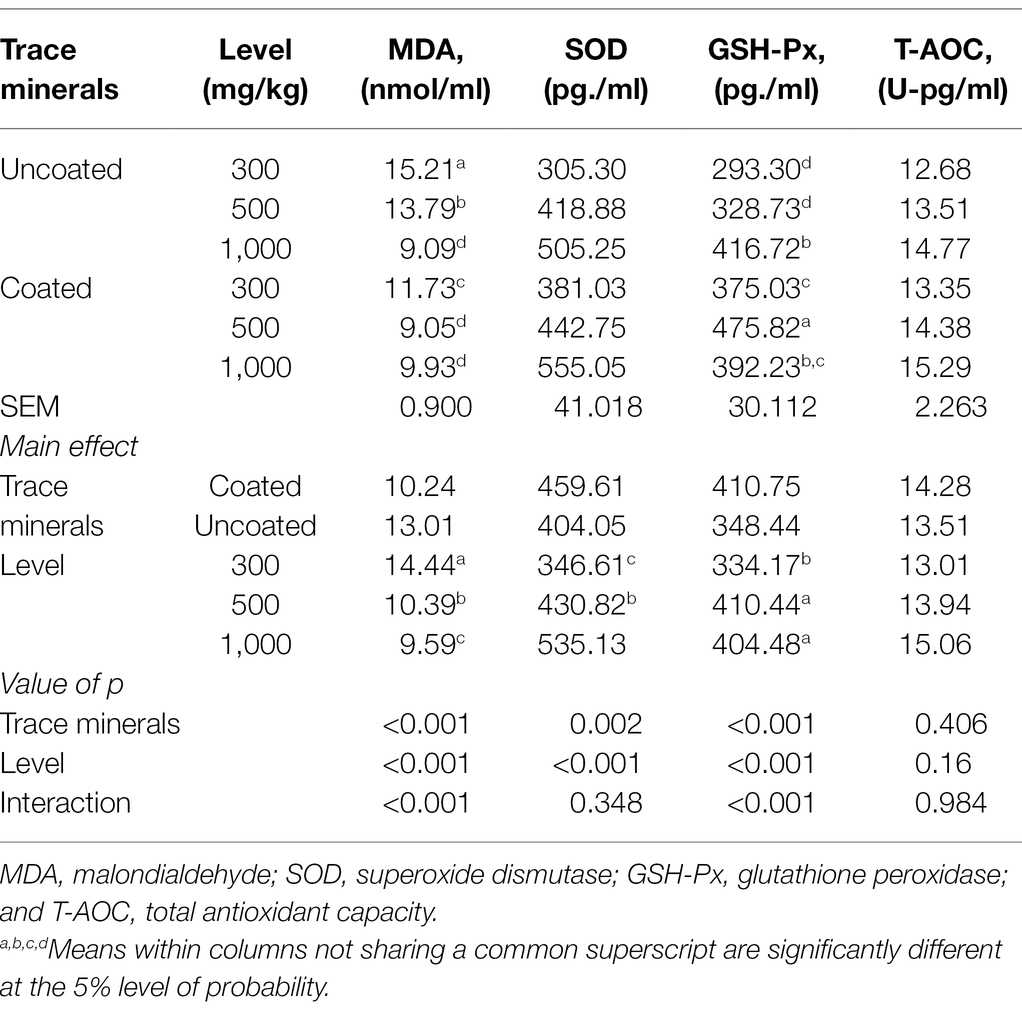
Table 8. Effects of trace mineral type (coated or uncoated) and supplementation level (300, 500, or 1,000 mg/kg) of trace minerals on serum antioxidant status in ducks.
Alpha-Diversity
Dietary treatments had significant interactions for Chao1, Shannon, and Simpson indices (Table 9). Ducks fed with 500 mg/kg of uncoated trace mineral had the highest Chao1, Shannon, and Simpson indices, and the 1,000 mg/kg of uncoated trace mineral treatment resulted in the lowest Chao 1 and Shannon indices. Diets supplemented with 300 mg/kg of uncoated trace minerals significantly decreased Simpson index compared with the other dietary treatments.
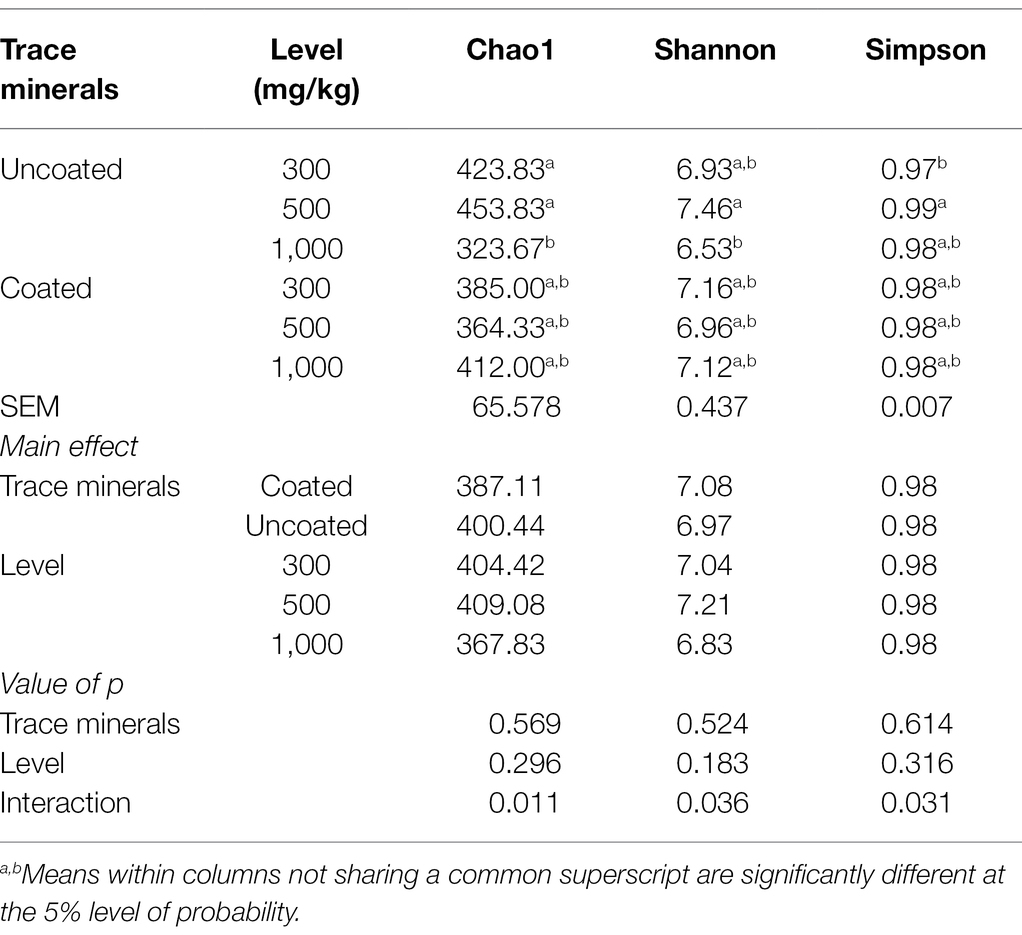
Table 9. Effects of trace mineral type (coated or uncoated) and supplementation level (300, 500, or 1,000 mg/kg) of trace minerals on duck cecal microbiota ⍺-diversity.
Microbial Composition
The taxonomic distribution of the gut microbiota in treatments is shown in Figure 1. At phyla level, Firmicutes, Bacteroidetes, and Proteobacteria were dominant (>1%). Coated trace mineral increased Firmicutes abundance in cecum of ducks. The top five relative abundances at phylum level in each treatment were Firmicutes, Bacteroidetes, Proteobacteria, Actinobacteria, and Cyanobacteria (Figure 1A). Ducks that received coated trace minerals showed a reduction in Tenericutes. As shown in Supplementary Figure S1, the dominant families in the sample were Bacteroidaceae and Ruminococcaceae, followed by Desulfovibrionaceae, Lachnospiraceae, and Bacteroidales in each treatment. At the genus level, the top 20 genera are listed in Figure 1B. Bacteroides was the most abundant genus at 18% of the sequence of all dietary treatments. There was an increase in the abundance of Oscillospira and Enterococcus when birds were fed with coated trace minerals. The Ruminococcus and Phascolarctobacterium were identified in high abundance in lowest trace minerals supplement groups, while Prevotella_1 was identified in high abundance (>0.01%) when ducks were offered the highest level of trace minerals.
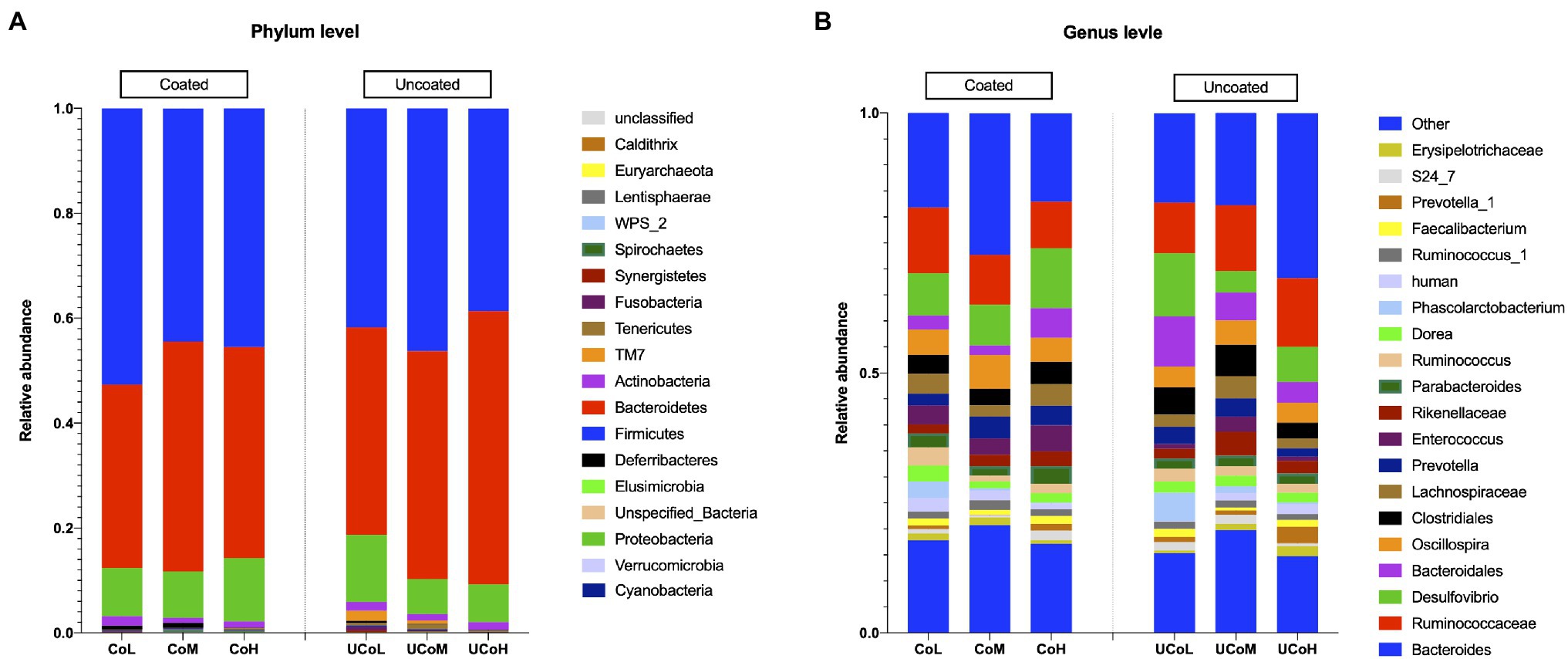
Figure 1. Differentially abundant taxa between diets supplemented with different levels of coated and uncoated trace minerals. (A) Represented phylum level, (B) represented top 20 genera. Data are means of six replicates. CoL, 300 mg/kg of coated trace mineral treatment; CoM, 500 mg/kg of coated trace mineral treatment; CoH, 1,000 mg/kg of coated trace mineral treatment; UCoL, 300 mg/kg of uncoated trace minerals treatment; UCoM, 500 mg/kg of uncoated trace minerals treatment; and UCoH, 1,000 mg/kg of uncoated trace minerals treatment.
Beta Diversity
In the present study, beta diversity was calculated to evaluate the similarity of the microbial community structures. Principal component analysis (PCoA) based on the unweighted UniFrac Distances was employed (Figure 2). The PCoA results showed that the cecal microbiota was barely separated from that in coated and uncoated trace mineral treatments (PERMANOVA, p = 0.001; ANOSIM, R = 0.200, p = 0.001; Figure 2A), while there is also no significant separation between different levels of trace minerals on duck microbiota flora (ANOSIM analysis: L/M: R = 0.207, p = 0.002; L/H: R = 0.207, =0.005; M/H: R = 0.096, p = 0.04; Figure 2B).
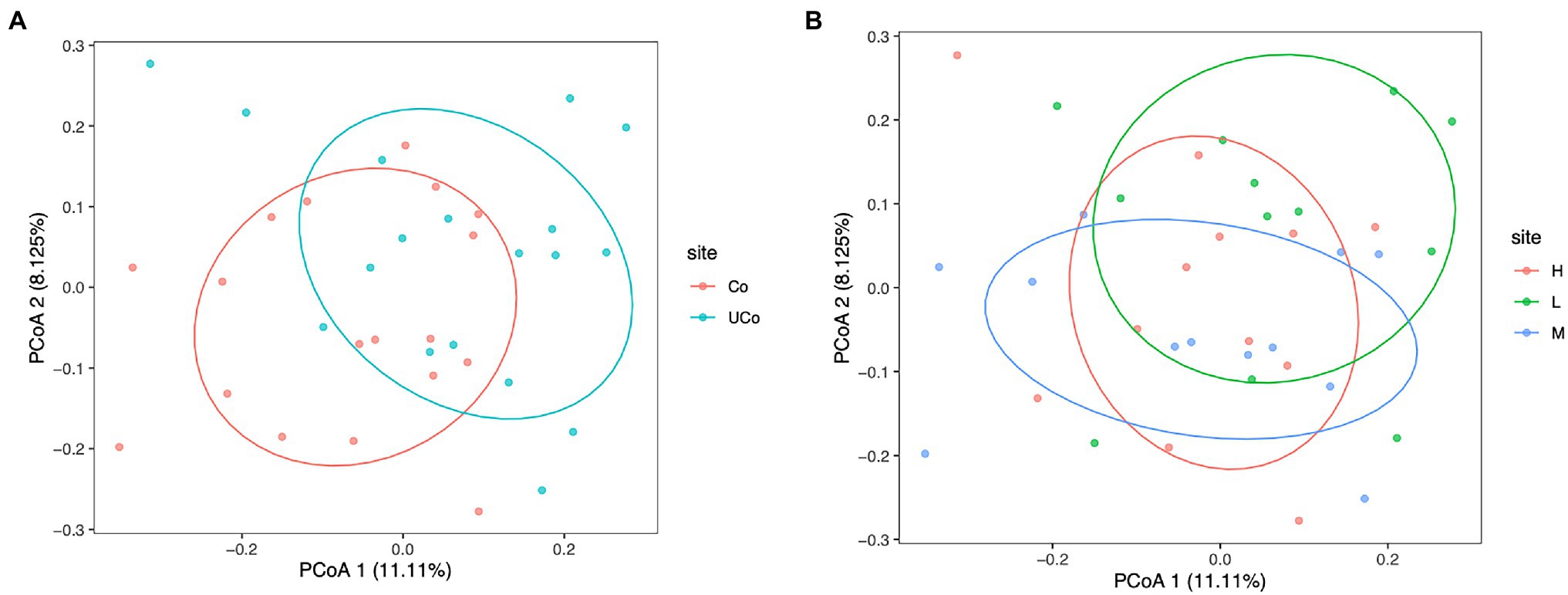
Figure 2. Principal coordinate analysis (PCoA) of cecal samples based on the unweighted UniFrac Distances. (A) The labels of the samples indicate the pattern of trace minerals, Co, coated trace minerals; UCo, uncoated trace minerals. (B) The labels of the samples indicate the supplementation level of trace minerals, L, 300 mg/kg; M, 500 mg/kg; and H, 1,000 mg/kg.
By LEfSe analysis, there were 21 differentially abundant bacterial clades at genus level (LDA score >2.0) between the coated and uncoated trace mineral treatments (Figure 3). Lactobacillus, Sphaerochatea, Butyricimonas, and Enterococcus were the dominant genus in cecum when ducks were fed with coated trace minerals, while the uncoated trace mineral-treated bird cecum were inhabited mostly by Desulfovibronaceae, Clostridiaceae, Elusimicrobiaceae, and Oxalobacter. Alternatively, 13 differentially abundant microbiota were found in three supplementation levels of trace mineral treatments. The 1,000 mg/kg of trace minerals could improve Lactobacillus, Rikenella, and Streptococcus colonization in duck cecum, and Biophila, Blautia, Rikenellaceae, and Desulfovibrionaceae were the dominant genus in 500-mg/kg treatments. When diets were supplemented with 300 mg/kg of trace minerals, Phascolarctobacterium, Turicibacter, Megamonas, and Corynebacterium were enriched in duck cecum.
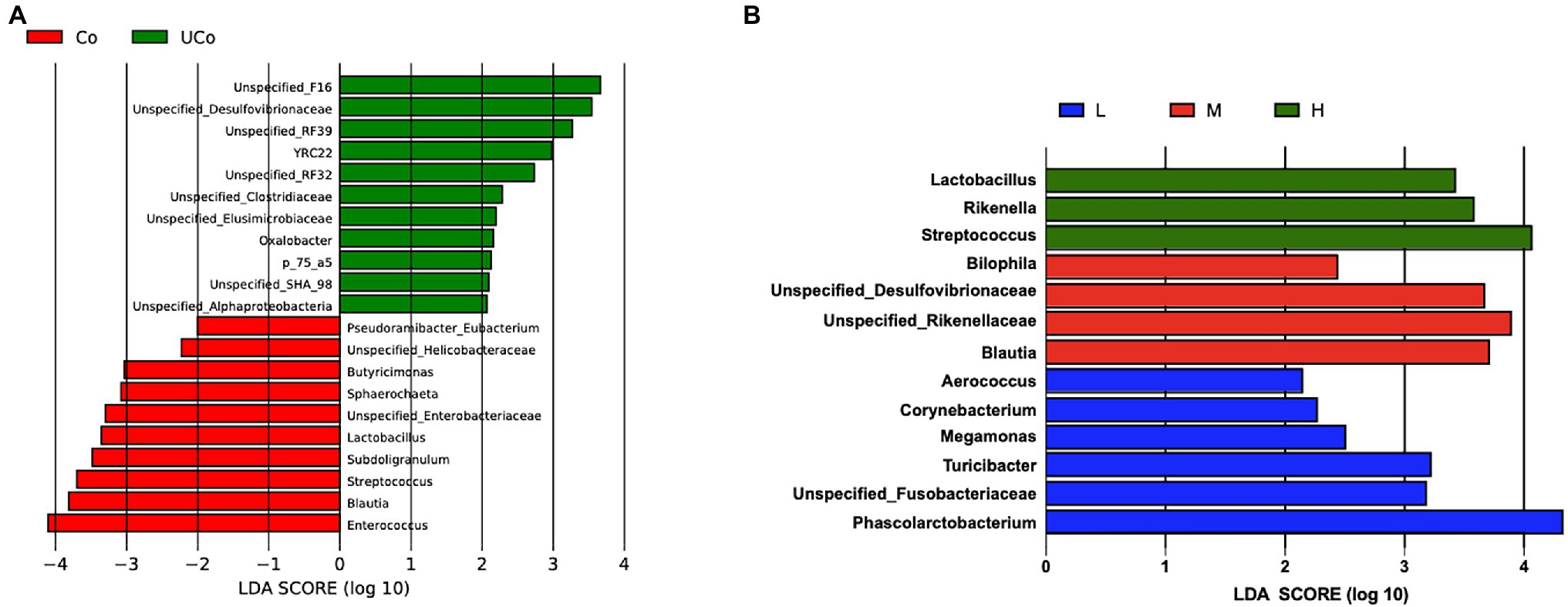
Figure 3. Effect size (LEfSe) analysis based on genus level between treatments. (A) Co, coated trace minerals (red); UCo, uncoated trace minerals (green); (B) L 300 mg/kg (blue), M, 500 mg/kg (red); and H, 1,000 mg/kg (green).
Correlations Between Fecal Metal Concentrations and Gut Microbiota
A multivariate analysis was carried out using the fecal metal concentrations Se, Zn, Fe, and Cu as “environmental variables” and the order groups as “species variable.” RDA showed that a total of 25% of the total variation in the microbiota composition at order level is related to environment variables (fecal metal concentrations). A smaller angle represents a positive relationship. In contrast, an angle more than 90° indicates a negative relationship. The arrow length reflects the importance of the parameter, and the orientation represents the association between the parameter and the axis. Five significant correlated orders are shown in Figure 4A, and among these microbiotas, there is a positive relationship between cecal Bacteroidals and fecal metal concentrations. Fusobacterialse has a negative relationship with fecal Zn concentration. Duck cecal Clostridiales, Turicibacterales, and Bifidobacteriales had negative relationships with fecal trace mineral contents. Additionally, fecal Se concentration had an important influence on excreta metal ion concentrations.
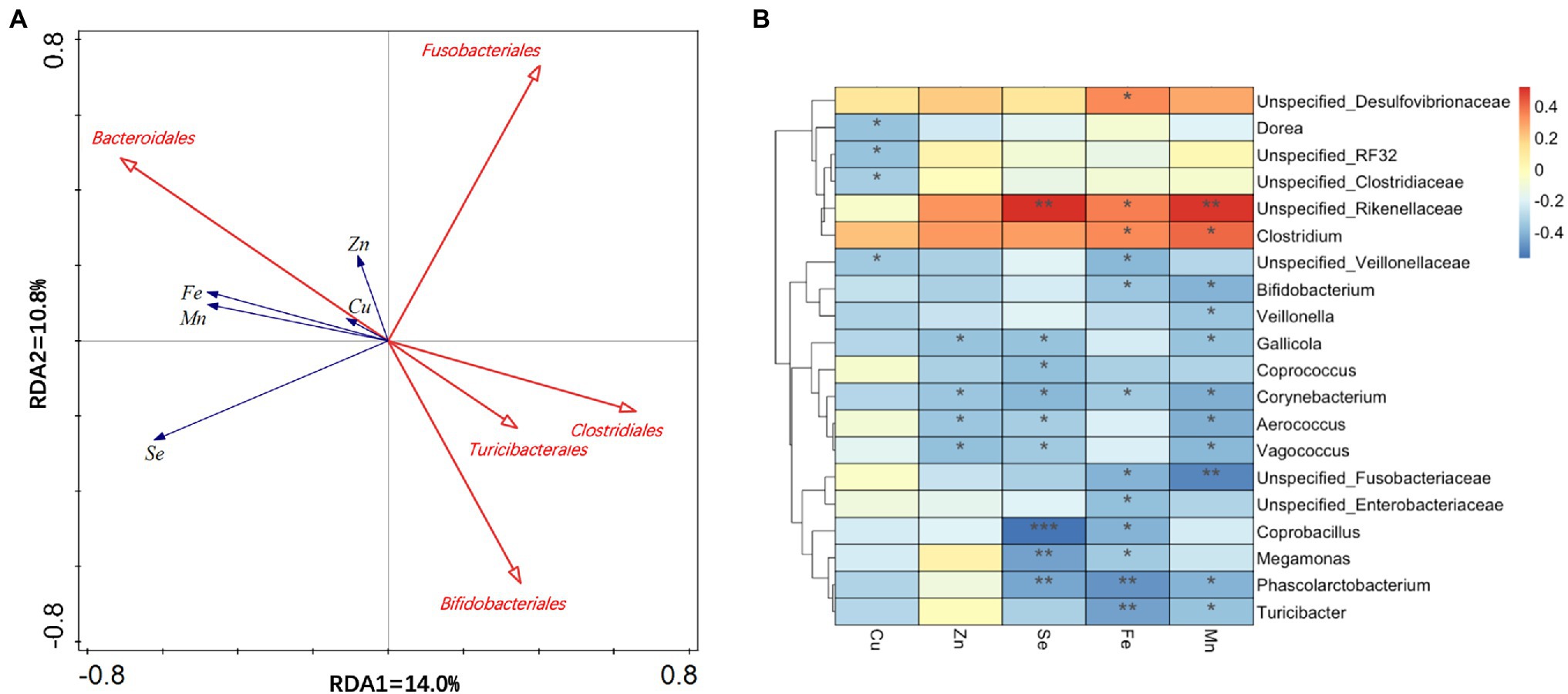
Figure 4. Correlation between fecal metal concentration and gut microbiota at order level. (A) Redundancy analysis (RDA) plot showed significant (p < 0.05) relationships between environmental variables (fecal metal concentration) and cecal bacterial communities. (B) Spearman’s heatmap correlation on order combined with UPGMA cluster analysis between abundance of series of to 25 bacteria order and environmental parameters. Negative correlation and positive correlation were represented by blue color and red color. *0.01 < p ≤ 0.05, **0.001 < p ≤ 0.01, and ***p ≤ 0.001.
Spearman’s correlation analysis of fecal Se, Zn, Fe, Cu, and Mn, and the most abundant 20 bacterial genera among the cecal microbiota was performed. As shown in Figure 4B, positive correlations (correlation coefficient >0) and negative correlations (correlation coefficient <0) were observed between various bacteria and environmental factors. An extreme negative correlation was found between cecal Coprobacillus and fecal Se concentration.
Functional Predictions Based on 16S rRNA Gene Sequence
According to KEGG level 2 analysis (Figure 5), coated trace mineral treatments were significantly enriched in the membrane transport and carbohydrate metabolism, while uncoated trace mineral groups were enriched in metabolism of terpenoids, polyketides, cell growth, and death pathways. Furthermore, supplementation levels of trace minerals had a significant influence on amino acid metabolism, biosynthesis of other secondary metabolites, and folding, sorting, and degradation pathways. At KEGG level 3 (Figure 6), we found 33 pathways that were significantly different between coated and uncoated trace mineral treatments. Among them, coated trace mineral groups significantly upregulated 20 pathways including glycolysis, pentose phosphate pathway, and glycerolipid metabolism. Uncoated trace mineral groups were enriched in 13 pathways, such as TCA cycle, fatty acid degradation, and linoleic acid metabolism.
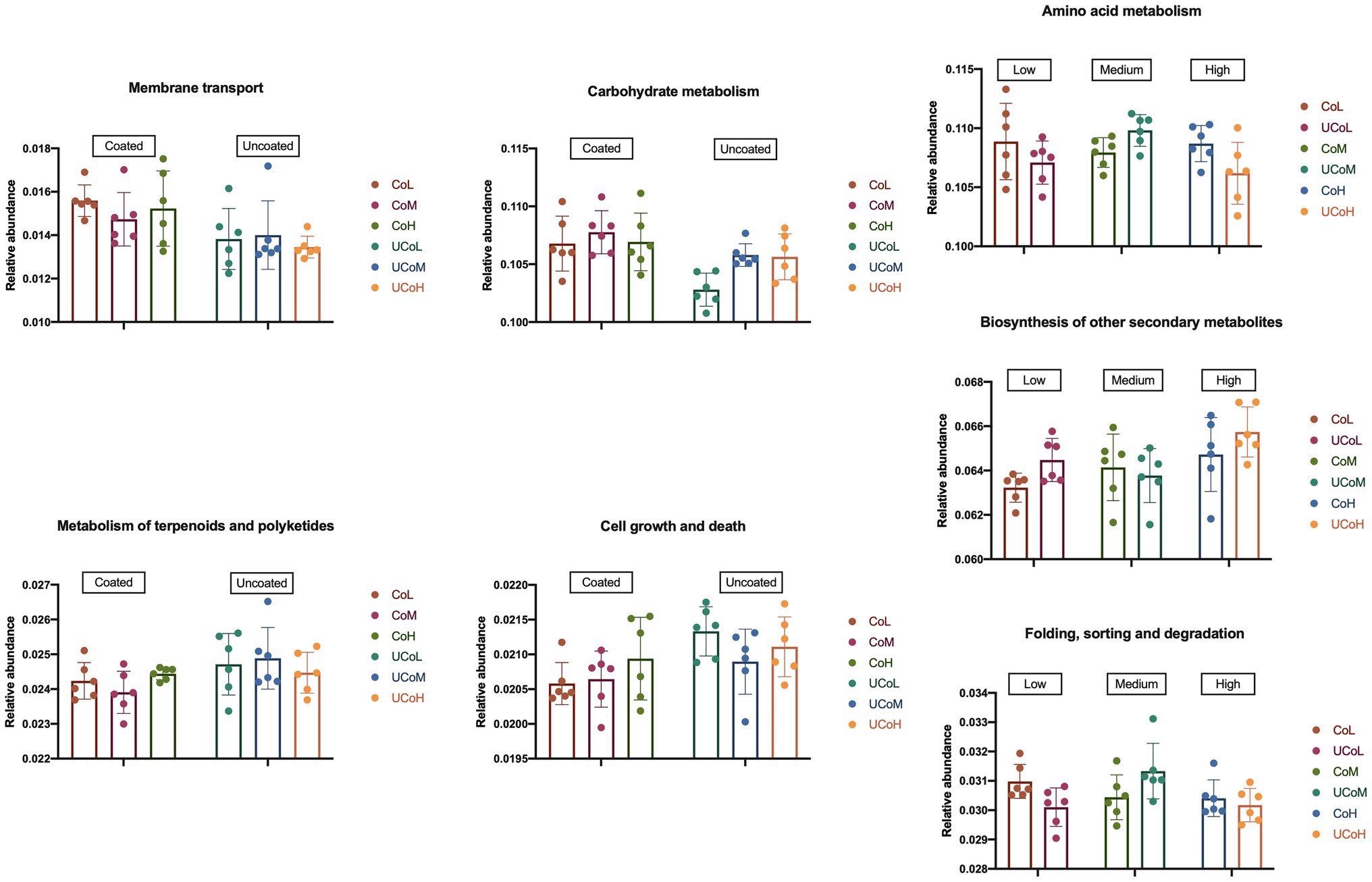
Figure 5. Comparison of microbiota function related to Kyoto Encyclopedia of Genes and Genomes (KEGG) pathway at level 2. CoL, 300 mg/kg of coated trace mineral treatment; CoM, 500 mg/kg of coated trace mineral treatment; CoH, 1,000 mg/kg of coated trace mineral treatment; UCoL, 300 mg/kg of uncoated trace minerals treatment; UCoM, 500 mg/kg of uncoated trace minerals treatment; and UCoH, 1,000 mg/kg of uncoated trace minerals treatment.
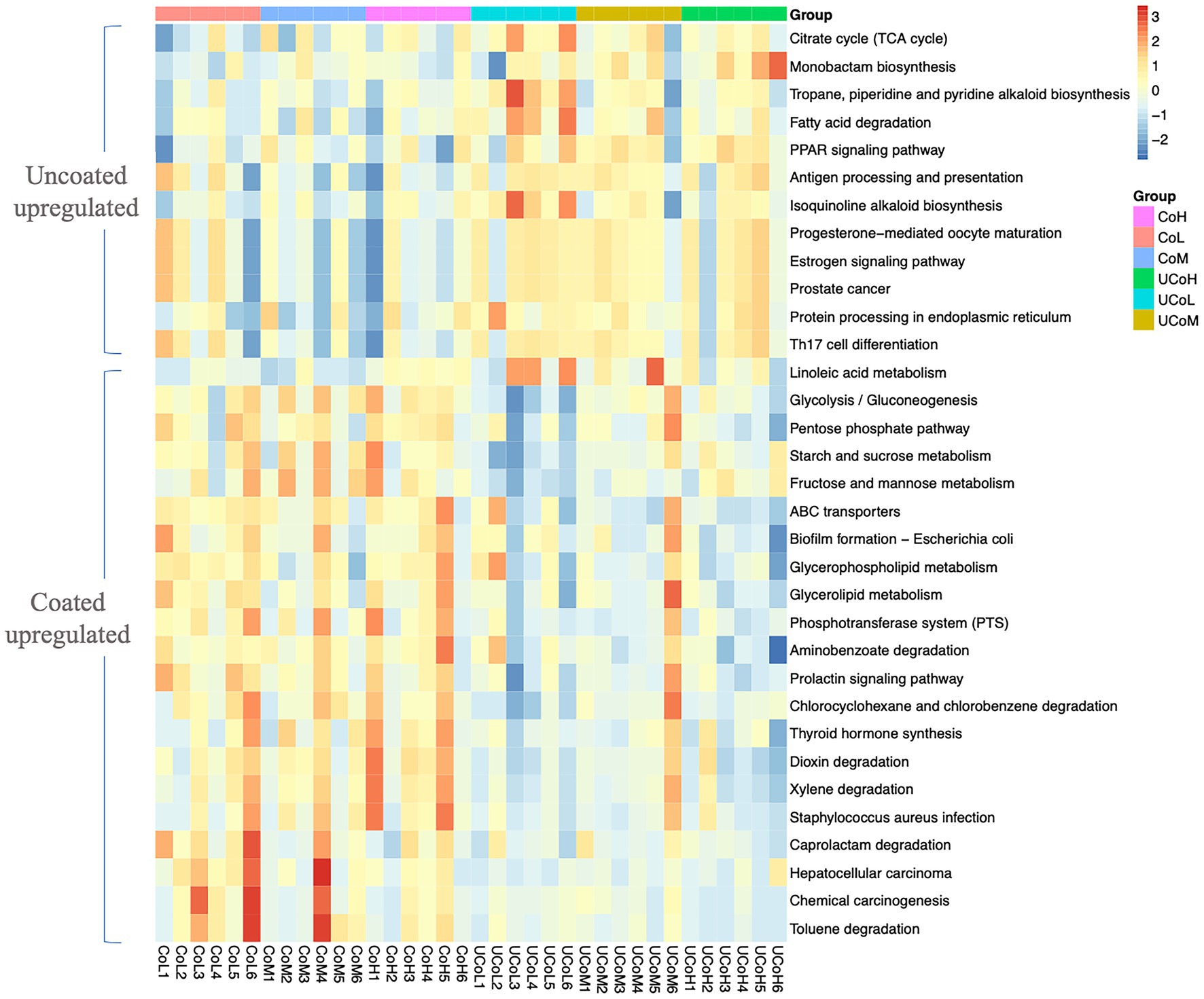
Figure 6. Comparison of microbiota function related to KEGG pathway at level 3. CoL, 300 mg/kg of coated trace mineral treatment; CoM, 500 mg/kg of coated trace mineral treatment; CoH, 1,000 mg/kg of coated trace mineral treatment; UCoL, 300 mg/kg of uncoated trace minerals treatment; UCoM, 500 mg/kg of uncoated trace minerals treatment; and UCoH, 1,000 mg/kg of uncoated trace minerals treatment.
Discussion
Growth Performance and Nutrient Digestibility
The present study aimed to determine the effect of coated and uncoated trace minerals on duck performance, mineral absorption, tissue deposition, and cecal microbiota. It has been clearly established that dietary trace metals can influence duck ADG during the 14- to 42-day feeding period. Diets containing coated trace minerals had superior ADG in weight compared with those containing uncoated trace minerals. Despite the fact that there is little information about the effect of coated trace minerals on duck performance, it is possible that controlling the release of trace metals in the gastrointestinal tract could improve the efficacy of ion metal absorption (Lei and Kim, 2018), and thus duck growth. Villagómez-Estrada et al. (2020) and Shannon and Hill (2019) reported that the primary functions of trace elements are to be part of a large number of coenzymes for various biological processes. Additionally, previous studies have proven that trace minerals play an essential role in digestive enzyme activities in pancreatic tissue and small intestine, such as Zn, Fe, and Mn (Alkhtib et al., 2020). The present study agrees with previous reports in pigs and broilers, where coating technology acted as a performance enhancer owing to higher bioavailability (Lei and Kim, 2018; Khatun et al., 2019; Yin et al., 2021). The cause behind the observed increase in ADG associated with feeding coated trace minerals appears to be an improved mineral utilization. Alternatively, it has been proposed that the positive effect of coated Zn supplementation on broiler performance might be associated with the improvement of appetite, altered growth hormone production, and enhanced capacity for immune response (Alkhtib et al., 2020). The observed higher phosphorus digestibility found in birds fed with coated trace minerals might be associated with high gastric pH values inhibiting the antagonisms between microminerals and phytic acid to improve the absorption and bioavailability (Liu et al., 2014).
Deposition of Minerals
Blood mineral content may reflect the mineral bioavailability (Burrow et al., 2020). The present study found that serum Se and Zn concentrations were improved with coated micromineral supplementation. Dietary inclusion of 1,000 mg/kg of trace minerals had an incremental effect on serum Se and Zn, which is in agreement with previous results (Zhang et al., 2017; Olukosi et al., 2018). Metal ions coated with carbohydrate might alter the ion interaction affecting the antagonism, which might potentially improve the utilization of the minerals or affect the ligand resulting in alteration in their utilization (Wang et al., 2019b). The interaction between trace mineral type and supplementation level in serum Se content suggested that dietary inclusion with 500 mg/kg of coated trace minerals had potential ability to replace 1,000 mg/kg of inorganic trace mineral in duck diets.
Excess intake of dietary metal ions leads to the higher deposition in the liver (Mézes et al., 2012). Thus, it is sensible that in the present study, with the exception of Fe, diets supplemented with the highest dosage of trace minerals resulted in the highest liver ion concentrations. Liver Se was also increased under coated trace mineral treatments. Coated trace minerals might potentially result in unique and more stable structures, which facilitate access to the enterocyte and improve the deposition in tissues (Bai et al., 2017; Wang et al., 2019b). Additionally, Se, Fe, and Zn deposition in muscle were significantly increased in birds fed with coated trace minerals. Qiu et al. (2020) reported that metal ions, such as Fe could be easily stored in tissues, and their absorption rate could be altered by adjusting the amount and form in the feed. The present study suggested that diets supplemented with 500 mg/kg of coated trace minerals were more efficient than the same or higher level of inorganic trace minerals, since coated forms are more conducive to the deposition of Se in the body.
Lower efficiency of utilization occurring with high supplementation of minerals resulted in greater excretion, which can be assumed to produce greater environmental pollution. Creech et al. (2004) indicated that reducing dietary trace mineral level could reduce excretion of minerals in swine waste. The present result found that coated trace minerals significantly reduced fecal Zn, Fe, and Mn contents, which agrees with previous studies. This suggests that the environmental impact of trace mineral supplementation may be mitigated by using coated trace minerals, which are demonstrated to be more bioavailable in the present study.
Antioxidant Status
Damage from reactive oxygen species could be reduced by SOD and GSH-Px (Wang et al., 2019a). These two enzymes are related with Zn, Cu, and Se concentration. MDA is also a marker of lipid peroxidation (Samuel et al., 2017). The present study found that coated trace minerals improved serum antioxidant status, which was similar with a previous report (Yin et al., 2021). The coated form of trace minerals may provide benefits through modulating the antioxidant system owing to resistant antagonisms in the intestine. We also found that ducks offered with 500 mg/kg of coated trace mineral had a similar antioxidant status than those offered with 1,000 mg/kg of inorganic trace minerals, suggesting that the effect of coated trace minerals is greater or equal compared with that of inorganic trace minerals.
Microbiota Profile
The gut microbiota contains thousands of functionally relevant genes and pathways to support essential gut functions and prevent intestinal dysbiosis (Pajarillo et al., 2021). Under a deficiency of trace minerals, gut microbiota would rapidly mature to respond to this stress (Bao et al., 2010; Tako et al., 2011). Reed et al. (2015) reported that the insufficient bioavailable dietary Zn in the lumen might improve gut microbiota colonization. Thus, the higher microbiota α-diversity associated with rapid proliferation in birds fed with inorganic trace minerals might reflect that absorption of inorganic trace mineral supplementation earlier in the intestine may result in a lack of trace minerals in the cecum. The overall microbial structure between coated and uncoated trace mineral treatments showed that trace minerals significantly influenced the microbiota profile in duck cecum. From LEfSe analysis, Lactobacillus, Sphaerochaetea, Butyricimonas, and Enterococcus were predominant genus enriched in birds fed with coated trace minerals. However, Desulfovibronaceae was enriched in inorganic trace mineral treatments. Desulfovibronaceae is a member of sulfate-reducing bacteria family, which potentially produced endotoxin, leading to chronic inflammation (Zhang-Sun et al., 2015). Alternatively, coated trace minerals could improve the probiotic microbiota colonization. Sphaerochaeta is both fermenter and electrogenic bacteria, which might play an important role in substrate transformation and electricity generation (Li et al., 2020), and higher enrichment of Sphaerochaeta is associated with lower ion excreta in coated trace mineral treatments. Latic acid, the main metabolic endproduct of Lactobacillus would improve the bioavailability of trace minerals (Famularo et al., 2005). Nath et al. (2018) reported that Butyricimonas is correlated with lipid metabolism and positively associated with the production of high-density lipoprotein for an individual’s health. Meanwhile, Enterococcus is known to produce enterocins to improve energy and protein utilization associating with higher growth rates, which is in accordance with this studies performance results (Fisher and Phillips, 2009). The predominant microbiota results in this study suggested that dietary supplemented with coated trace minerals could improve the enrichment of beneficial bacterium in duck cecum to improve and maintain gut health.
The contamination of metals in the environment is an important cause of stress and detrimental health effects. Recent studies showed that microorganisms inhabiting the gastrointestinal tract could provide essential services to reduce environmental contamination (Brila et al., 2021). To explore the relationships between the extraction of trace minerals and gut microbiota, RDA analysis was conducted in the present study. Intestinal Fusobacterialse, Clostridiales, Turicibacterales, and Bifidobacteriales had negative relationships with fecal trace mineral contents. Cheng et al. (2020) found that cecal Bifidobacteriales and Clostridiales were altered by copper, which is similar to the findings in the present study. Trace minerals, such as Cu and Zn could be used by Bifidobacteriales to produce probiotic substrates (Ionescu et al., 2009), thus it has a negative relationship with excreted trace minerals. On the other hand, Bifidobacteriales has a crucial role in body health and was seen as a potential beneficial body service provider (Zhang et al., 2016). Spearman’s correlation analysis also showed that fecal ion metals had a significant negative relationship with cecal Coprobacillus, especially Se. Coprobacillus was a Gram-positive bacteria, closely associated with Clostridum ramosum (Kageyama and Benno, 2000), and its colonization could be improved by Enterococcus (Stein et al., 2013). Thus, as coated trace minerals may improve Enterococcus colonization in duck cecum, this might have the ability to improve the growth of Coprobacillus to reduce excretion of trace minerals.
The predicated KEGG analysis results showed that coated trace mineral groups significantly upregulated pathways related to carbohydrate metabolism and stimulated glycerolipid metabolism and membrane transport. The upregulation in carbohydrate metabolism could well support the better growth performance. Yin et al. (2021) reported that coated trace mineral could improve lipid metabolism, which might be associated with the present result. Meanwhile, ion metals, such as Zn and Cu, were related with microbiota function (van Kuijk et al., 2021). Inorganic trace metals would result in lower bioavailability and, thus, greater competition between intestinal microbiota and host (Reed et al., 2015). While coated trace minerals might improve the stability and absorption of minerals via the intestinal brush border, they may also be more effectively associated with the upregulation of microbiota transporter systems. Consequently, dietary supplementation with coated trace minerals could stimulate microbial barrier function.
Conclusion
In conclusion, the present study suggested that coated trace minerals enhanced the growth of meat ducks. Coated trace minerals used in duck diets could reduce the risk of environmental contamination from fecal minerals without affecting performance. Additionally, 500 mg/kg of coated trace mineral had a similar effect on duck serum trace minerals and tissue ion metal deposition relative to 1,000 mg/kg of inorganic trace minerals. Coated trace minerals had a potential to improve bioavailability of ion metals and the colonization of probiotic microbiota, such as Lactobacillus, Butyricimonas, and Enterococcus, in the cecum to protect the intestine microbial barrier and maintain gut health.
Data Availability Statement
The datasets presented in this study can be found in online repositories. The names of the repository/repositories and accession number(s) can be found at: https://www.ncbi.nlm.nih.gov/, PRJNA787257.
Ethics Statement
The animal study was reviewed and approved by Experimental Animal Committee of Shenyang Agricultural University (permit number 202106037).
Author Contributions
DY: data analysis and writing original draft. FZ: data curation and investigation. AM: review and edit draft. YK: investigation. WL, FL, YuZ, and RZ: data curation. YoZ and SZ: supervision. All authors contributed to the article and approved the submitted version.
Funding
This work was supported by the China Postdoctoral Science Foundation (no. 2021M702299).
Conflict of Interest
FZ was employed by the company Yichun Tequ Feed Company and WL and YK were employed by the company Fujian Syno Biotech Co., Ltd.
The remaining authors declare that the research was conducted in the absence of any commercial or financial relationships that could be construed as a potential conflict of interest.
Publisher’s Note
All claims expressed in this article are solely those of the authors and do not necessarily represent those of their affiliated organizations, or those of the publisher, the editors and the reviewers. Any product that may be evaluated in this article, or claim that may be made by its manufacturer, is not guaranteed or endorsed by the publisher.
Acknowledgments
We thank Microeco Tech Co., Ltd., Shenzhen, China, for sequencing service and assistance.
Supplementary Material
The Supplementary Material for this article can be found online at: https://www.frontiersin.org/articles/10.3389/fmicb.2022.831945/full#supplementary-material
References
Alkhtib, A., Scholey, D., Carter, N., Cave, G. W., Hanafy, B. I., Kempster, S. R., et al. (2020). Bioavailability of methionine-coated zinc nanoparticles as a dietary supplement leads to improved performance and bone strength in broiler chicken production. Animals 10:1482. doi: 10.3390/ani10091482
Andrews, S. C., Robinson, A. K., and Rodríguez-Quiñones, F. (2003). Bacterial iron homeostasis. FEMS Microbiol. Rev. 27, 215–237. doi: 10.1016/S0168-6445(03)00055-X
AOAC (2000). Official Methods of Analysis. Vol. 1. Arlington, VA: Association of Official Analytical Chemists.
Bai, S., Gu, J., Delong, L., Ding, X., Wang, J., Zhang, K., et al. (2017). Dietary organic trace minerals level influences eggshell quality and minerals retention in hens. Ann. Anim. Sci. 17, 503–515. doi: 10.1515/aoas-2016-0074
Bao, Y., and Choct, M. (2009). Trace mineral nutrition for broiler chickens and prospects of application of organically complexed trace minerals: a review. Anim. Prod. Sci. 49, 269–282. doi: 10.1071/EA08204
Bao, Y. M., Choct, M., Iji, P., and Bruerton, K. (2010). Trace mineral interactions in broiler chicken diets. Br. Poult. Sci. 51, 109–117. doi: 10.1080/00071660903571904
Becker, K. W., and Skaar, E. P. (2014). Metal limitation and toxicity at the interface between host and pathogen. FEMS Microbiol. Rev. 38, 1235–1249. doi: 10.1111/1574-6976.12087
Bokulich, N. A., Kaehler, B. D., Ram, R. J., Matthew, D., Evan, B., Rob, K., et al. (2018). Optimizing taxonomic classification of marker-gene amplicon sequences with qiime 2’s q2-feature-classifier plugin. Microbiome 6:90. doi: 10.1186/s40168-018-0470-z
Braun, V., and Hantke, K. (2011). Recent insights into iron import by bacteria. Curr. Opin. Chem. Biol. 15, 328–334. doi: 10.1016/j.cbpa.2011.01.005
Brila, I., Lavrinienko, A., Tukalenko, E., Ecke, F., Rodushkin, I., Kallio, E. R., et al. (2021). Low-level environmental metal pollution is associated with altered gut microbiota of a wild rodent, the bank vole (Myodes glareolus). Sci. Total Environ. 790:148224. doi: 10.1016/j.scitotenv.2021.148224
Burrow, K., Young, W., McConnell, M., Carne, A., Barr, D., Reid, M., et al. (2020). The effect of sheep and cow milk supplementation of a low calcium diet on the distribution of macro and trace minerals in the organs of weanling rats. Nutrients 12:594. doi: 10.3390/nu12030594
Callahan, B. J., Mcmurdie, P. J., Rosen, M. J., Han, A. W., Johnson, A. J. A., and Holmes, S. P. (2016). Dada2: high-resolution sample inference from illumina amplicon data. Nat. Methods 13, 581–583. doi: 10.1038/nmeth.3869
Cheng, S., Mao, H., Ruan, Y., Wu, C., Xu, Z., Hu, G., et al. (2020). Copper changes intestinal microbiota of the cecum and rectum in female mice by 16S rRNA gene sequencing. Biol. Trace Elem. Res. 193, 445–455. doi: 10.1007/s12011-019-01718-2
Creech, B., Spears, J., Flowers, W., Hill, G., Lloyd, K., Armstrong, T., et al. (2004). Effect of dietary trace mineral concentration and source (inorganic vs. chelated) on performance, mineral status, and fecal mineral excretion in pigs from weaning through finishing. J. Anim. Sci. 82, 2140–2147. doi: 10.2527/2004.8272140x
David, L., Abdollahi, M., Ravindran, G., Walk, C., and Ravindran, V. (2019). Studies on the measurement of ileal calcium digestibility of calcium sources in broiler chickens. Poult. Sci. 98, 5582–5589. doi: 10.3382/ps/pez314
Famularo, G., De Simone, C., Pandey, V., Sahu, A. R., and Minisola, G. (2005). Probiotic lactobacilli: an innovative tool to correct the malabsorption syndrome of vegetarians? Med. Hypotheses 65, 1132–1135. doi: 10.1016/j.mehy.2004.09.030
Fisher, K., and Phillips, C. (2009). The ecology, epidemiology and virulence of Enterococcus. Microbiology 155, 1749–1757. doi: 10.1099/mic.0.026385-0
Ionescu, A., Angela, C., Boca, E., Mocanu, E., Cojocaru, R., Nicoleta, R., et al. (2009). “The characterization of the Cu, Zn, Co, Cd and Fe content of Lactobacillus and Bifidobacterium strains used for probiotic production.” in Lucrări Științifice-Universitatea de Științe Agronomice Și Medicină Veterinară București. November 19-20, 2009; Seria F, Biotehnologii. 219–222.
Kageyama, A., and Benno, Y. (2000). Catenibacterium mitsuokai gen. nov., sp. nov., a gram-positive anaerobic bacterium isolated from human faeces. Int. J. Syst. Evol. Microbiol. 50, 1595–1599. doi: 10.1099/00207713-50-4-1595
Khatun, A., Chowdhury, S. D., Roy, B. C., Dey, B., Haque, A., and Chandran, B. (2019). Comparative effects of inorganic and three forms of organic trace minerals on growth performance, carcass traits, immunity, and profitability of broilers. J. Adv. Vet. Anim. Res. 6:66. doi: 10.5455/javar.2019.f313
Lei, X. J., and Kim, I. H. (2018). Low dose of coated zinc oxide is as effective as pharmacological zinc oxide in promoting growth performance, reducing fecal scores, and improving nutrient digestibility and intestinal morphology in weaned pigs. Anim. Feed Sci. Technol. 245, 117–125. doi: 10.1016/j.anifeedsci.2018.06.011
Li, W., Quan, X., Chen, L., and Zheng, Y. (2020). Application of slow-release carbon sources embedded in polymer for stable and extended power generation in microbial fuel cells. Chemosphere 244:125515. doi: 10.1016/j.chemosphere.2019.125515
Likittrakulwong, W., Moonsatan, S., and Incharoen, T. (2021). Enhancement of tibia bone and eggshell hardness through the supplementation of bio-calcium derived from fish bone mixed with chelated trace minerals and vitamin D3 in laying duck diet. Vet. Anim. Sci. 14:100204. doi: 10.1016/j.vas.2021.100204
Liu, Y., Ma, Y., Zhao, J., Vazquez-Añón, M., and Stein, H. (2014). Digestibility and retention of zinc, copper, manganese, iron, calcium, and phosphorus in pigs fed diets containing inorganic or organic minerals. J. Anim. Sci. 92, 3407–3415. doi: 10.2527/jas.2013-7080
Lu, W., Kuang, Y., Ma, Z., and Liu, Y. (2020). The effect of feeding broiler with inorganic, organic, and coated trace minerals on performance, economics, and retention of copper and zinc. J. Appl. Poult. Res. 29, 1084–1090. doi: 10.1016/j.japr.2020.10.002
Mézes, M., Erdélyi, M., and Balogh, K. (2012). “Deposition of organic trace metal complexes as feed additives in farm animals,” in 4th International Symposium on Trace Elements in the Food Chain, Friends or Foes ; 15-17 November, 2012; Visegrád, Hungary. 410.
Ministry of Agriculture of the People’s Republic of China (2012). Nutrient Requirments of Meat-Type Duck. The Ministry of Agriculture of the People’s Republic of China.
Nath, A., Molnár, M. A., Csighy, A., Kőszegi, K., Galambos, I., Huszár, K. P., et al. (2018). Biological activities of lactose-based prebiotics and symbiosis with probiotics on controlling osteoporosis, blood-lipid and glucose levels. Medicina 54:98. doi: 10.3390/medicina54060098
National Research Council (1994). National Research Council Nutrient Requirements of Poultry (9th Rev.). Washington, DC: National Academy Press.
Olukosi, O. A., van Kuijk, S., and Han, Y. (2018). Copper and zinc sources and levels of zinc inclusion influence growth performance, tissue trace mineral content, and carcass yield of broiler chickens. Poult. Sci. 97, 3891–3898. doi: 10.3382/ps/pey247
Pajarillo, E. A. B., Lee, E., and Kang, D.-K. (2021). Trace metals and animal health: interplay of the gut microbiota with iron, manganese, zinc, and copper. Anim. Nutr. 7, 750–761. doi: 10.1016/j.aninu.2021.03.005
Qiu, J., Lu, X., Ma, L., Hou, C., He, J., Liu, B., et al. (2020). Low-dose of organic trace minerals reduced fecal mineral excretion without compromising performance of laying hens. Asian Austral. J. Anim. 33, 588–596. doi: 10.5713/ajas.19.0270
Reed, S., Neuman, H., Moscovich, S., Glahn, R. P., Koren, O., and Tako, E. (2015). Chronic zinc deficiency alters chick gut microbiota composition and function. Nutrients 7, 9768–9784. doi: 10.3390/nu7125497
Samuel, K., Wang, J., Yue, H., Wu, S., Zhang, H., Duan, Z., et al. (2017). Effects of dietary gallic acid supplementation on performance, antioxidant status, and jejunum intestinal morphology in broiler chicks. Poult. Sci. 96, 2768–2775. doi: 10.3382/ps/pex091
Shannon, M. C., and Hill, G. M. (2019). Trace mineral supplementation for the intestinal health of young monogastric animals. Front. Vet. Sci. 6:73. doi: 10.3389/fvets.2019.00073
Stein, R. R., Bucci, V., Toussaint, N. C., Buffie, C. G., Rätsch, G., Pamer, E. G., et al. (2013). Ecological modeling from time-series inference: insight into dynamics and stability of intestinal microbiota. PLoS Comput. Biol. 9:e1003388. doi: 10.1371/journal.pcbi.1003388
Tako, E., Blair, M. W., and Glahn, R. P. (2011). Biofortified red mottled beans (Phaseolus vulgaris L.) in a maize and bean diet provide more bioavailable iron than standard red mottled beans: studies in poultry (Gallus gallus) and an in vitro digestion/Caco-2 model. Nutr. J. 10, 1–10. doi: 10.1186/1475-2891-10-113
van Kuijk, S. J., Han, Y., Garcia-Ruiz, A. I., and Rodiles, A. (2021). Hydroxychloride trace minerals have a positive effect on growth performance, carcass quality and impact ileal and cecal microbiota in broiler chickens. J. Anim. Sci. Biotechnol. 12, 1–13. doi: 10.1186/s40104-021-00553-7
Villagómez-Estrada, S., Pérez, J. F., Darwich, L., Vidal, A., van Kuijk, S., Melo-Durán, D., et al. (2020). Effects of copper and zinc sources and inclusion levels of copper on weanling pig performance and intestinal microbiota. J. Anim. Sci. 98:skaa117. doi: 10.1093/jas/skaa117
Wang, G., Liu, L., Tao, W., Xiao, Z., Pei, X., Liu, B., et al. (2019a). Effects of replacing inorganic trace minerals with organic trace minerals on the production performance, blood profiles, and antioxidant status of broiler breeders. Poult. Sci. 98, 2888–2895. doi: 10.3382/ps/pez035
Wang, G., Liu, L., Wang, Z., Pei, X., Tao, W., Xiao, Z., et al. (2019b). Comparison of inorganic and organically bound trace minerals on tissue mineral deposition and fecal excretion in broiler breeders. Biol. Trace Elem. Res. 189, 224–232. doi: 10.1007/s12011-018-1460-5
Yang, K., Hu, S., Mu, R., Qing, Y., Xie, L., Zhou, L., et al. (2021). Effects of different patterns and sources of trace elements on laying performance, tissue mineral deposition, and fecal excretion in laying hens. Animals 11:1164. doi: 10.3390/ani11041164
Yin, D., Tong, T., Moss, A. F., Zhang, R., Kuang, Y., Zhang, Y., et al. (2021). Effects of coated trace minerals and the fat source on growth performance, antioxidant status, and meat quality in broiler chickens. J. Poult. Sci. 59, 56–63. doi: 10.2141/jpsa.0200108
Zhang, G., Gao, B., Adeolu, M., Khadka, B., and Gupta, R. S. (2016). Phylogenomic analyses and comparative studies on genomes of the Bifidobacteriales: identification of molecular signatures specific for the order Bifidobacteriales and its different subclades. Front. Microbiol. 7:978. doi: 10.3389/fmicb.2016.00978
Zhang, Y., Zhang, H., Wang, J., Yue, H., Qi, X., Wu, S., et al. (2017). Effect of dietary supplementation of organic or inorganic zinc on carbonic anhydrase activity in eggshell formation and quality of aged laying hens. Poult. Sci. 96, 2176–2183. doi: 10.3382/ps/pew490
Keywords: duck, gut microbiota, performance, tissue deposition, trace minerals
Citation: Yin D, Zhai F, Lu W, Moss AF, Kuang Y, Li F, Zhu Y, Zhang R, Zhang Y and Zhang S (2022) Comparison of Coated and Uncoated Trace Minerals on Growth Performance, Tissue Mineral Deposition, and Intestinal Microbiota in Ducks. Front. Microbiol. 13:831945. doi: 10.3389/fmicb.2022.831945
Edited by:
Franck Carbonero, Washington State University Health Sciences Spokane, United StatesReviewed by:
Shuangshuang Guo, Wuhan Polytechnic University, ChinaThomas Figueroa, Institut National de la Recherche Agronomique de Toulouse, France
Copyright © 2022 Yin, Zhai, Lu, Moss, Kuang, Li, Zhu, Zhang, Zhang and Zhang. This is an open-access article distributed under the terms of the Creative Commons Attribution License (CC BY). The use, distribution or reproduction in other forums is permitted, provided the original author(s) and the copyright owner(s) are credited and that the original publication in this journal is cited, in accordance with accepted academic practice. No use, distribution or reproduction is permitted which does not comply with these terms.
*Correspondence: Yong Zhang, MTM4ODkxMTM2MjZAMTI2LmNvbQ==; Shuyi Zhang, c3poYW5nQHN5YXUuZWR1LmNu
†These authors have contributed equally to this work