- 1Key Laboratory of Forage Cultivation, Processing and High Efficient Utilization of Ministry of Agriculture and Rural Affairs, Inner Mongolia Agricultural University, Hohhot, China
- 2Key Laboratory of Grassland Resources of Ministry of Education, Inner Mongolia Agricultural University, Hohhot, China
- 3National Engineering Laboratory of Biological Feed Safety and Pollution Prevention and Control, Key Laboratory of Animal Nutrition and Feed Science of Zhejiang Province, Institute of Feed Science, Zhejiang University, Hangzhou, China
- 4Inner Mongolia Academy of Agricultural & Animal Husbandry Sciences, Hohhot, China
- 5College of Animal Science, Guizhou University, Guiyang, China
Ensiling native grass is an effective method to protect the nutritional quality of forage and alleviate feed shortages in the cold winter of the Inner Mongolian Plateau. To improve the usability of native grass resources as feed in China, the effects of lactic acid bacteria and molasses additions on the microbial population, fermentation quality, and nutritional quality of native grass during silage were investigated. Treatments were a control treatment with no additive (CK), lactic acid bacteria (L), molasses (M), and lactic acid bacteria in combination with molasses (L+M), all of which were stored at ambient temperature (17–28°C) for 7, 14, 30, and 60 days. The results showed that all additives improved nutritional value and fermentation quality with low pH and ammonia nitrogen (NH3–N) and high crude protein (CP) and water soluble carbohydrate (WSC) than control silage over the ensiling period. Compared with L or M silage, the L+M silage combination improved fermentability, as evidenced by higher LA content and a faster pH drop during the first 7 days of ensiling. With prolonged ensiling time, the combined addition of L and M could increase the count of desirable Lactobacillus, decrease microbial diversity, and inhibit the growth of undesirable microorganism, such as Clostridia, Escherichia, and Enterobacter abundance compared with silage treated with CK, L. or M. Application of L together with M could further improve the silage quality of native grass by altering bacterial community structure. In summary, the addition of lactic acid bacteria and molasses increased the relative abundance of Lactobacillus of native grass silage and improved fermentation quality.
Introduction
Native grass is an herb that grows on native grasslands, which are widely scattered across the Mongolian Plateau; large needlegrass (Stipa grandis P. Smirn) and Chinese leymus [Leymus chinensis (Trin.) Tzvel] dominate typical steppe communities (Zhang et al., 2016), are mostly utilized for grazing and haymaking, and are the main source of forage in pastoral areas (Du S. et al., 2020). Seasonal changes affect the quality and productivity of native grass (Kang et al., 2007). From June to September, native grass grows well, and animals usually get enough nutrition and gain weight during this time (Long et al., 1999). However, feed deficiency during the long cold season (November to June) is one of the major issues confronting the Mongolian Plateau’s traditional animal grazing system (Zhou et al., 2017; Ding et al., 2020), which is due to both the limited quantity and quality of native grass during this period. Farmers usually begin storing grass in mid-August. During harvest and storage, there is a significant loss of dry matter (DM) and crude protein (CP) (Scarbrough et al., 2002). Therefore, efficient native grass preservation is regarded as a crucial farming strategy for alleviating feed shortages in the Mongolian Plateau’s traditional year-round grazing system.
Ensiling has been considered as a traditional method to preserve forage nutrients in the harvesting season because of various advantages, such as easy to operate, prolonging supply time, and suitability for a wide range of feedstock (Ding et al., 2020), and can effectively address animal feed shortages during the winter months on the Mongolian Plateau (Zhang et al., 2016). Ensiling mainly depends on lactic acid bacteria to transfer soluble carbohydrates to lactic acid, resulting in a low pH environment and inhibiting the growth of aerobic bacteria, making the feed preserved (Lee et al., 2018) and improving palatability (Driehuis, 2013). Ensiling has been shown in previous research to improve fermentation quality while also preserving the nutrients of natural grass, resulting in a high feeding value (Khota et al., 2016; Du Z. M. et al., 2020). However, due to the low water content, low water soluble carbohydrate, low lactic acid bacteria attachment, and high buffer energy of natural forage, direct silage is difficult to succeed (Zhang et al., 2016; Hou et al., 2017). To solve this problem, Cai et al. (2020) evaluated the effects of additives on native grass silage and found that the use of L and cellulase can improve the fermentation quality of native grass silage. L is the most common type of silage additive, capable of ensuring extensive fermentation and efficient substrate utilization in ensiled materials. It has been reported that adding L to rice straw silage improved its quality (Zhao et al., 2019). M is a sugar industry byproduct that is high in soluble carbohydrate and serves as a fermentable substrate. M not only improved fermentation quality but also significantly altered the microbial community of cassava foliage silage (Li et al., 2021). However, there is limited information related to the microbial community and fermentation products during the ensiling process of native grass with additive treatments. Therefore, this study was conducted to increase understanding to inform the regulation of fermentation of native grass on the Mongolian Plateau. The purpose of this study was to assess the effects of adding L, M, and their combination on the fermentation quality and microbial community dynamics of native grass silage.
Materials and Methods
Study Sites and Silage Preparation
Native grass was harvested at the heading stage from a typical grassland (45°58′E, 118°57′N) on the Inner Mongolian Plateau, People’s Republic of China, on July 15, 2020. The dominant species harvested were large needlegrass (Stipa grandis P. Smirn), Chinese Leymus [Leymus chinensis (Trin.) Tzvel.], and Dahurian Bushclover [Lespedeza davurica (Laxm.) Schindl.]. Harvested native grass was chopped to lengths of 2–3 cm. Lactic acid bacteria (L) inoculant and molasses (M) were used as additives for ensiling the native grass. L (Lactobacillus plantarum, Snow Brand Seed Co. Ltd., Sapporo, Japan) was provided by the Institute of Animal Husbandry and Grassland of Japan. M was obtained from Baihui Biological Technology Co. Ltd. (Chifeng, Inner Mongolia, China). The L inoculant was applied at a level of 106 colony-forming units (cfu) per gram of fresh material (FM), and molasses was applied at 30 g/kg of FM. The chopped native grass was mixed and divided into equal portions for four treatments: no additive control (CK), L treatment (L), M treatment (M), and L + M treatment (L + M). All the additives were mixed homogenously with native grass. In detail, each treated batch was divided into four replicates (one for backup) of 200 g each, which were packed into polyethylene bags and vacuum sealed. A total of 64 bags (4 treatments × 4 ensiling days × 4 repeats) were prepared and kept at ambient temperature (17–28°C). After 7, 14, 30, and 60 days of ensiling, fermentation characteristics, chemical compositions, microorganism counts, and the microbial community were analyzed. Initial fresh forage samples were taken before ensiling.
Analysis of Chemical Composition, Organic Acid, and Microbial Population
For chemical analysis, each sample set had three parallel determinations. Dry matter (DM) contents were determined by oven drying at 65°C for 48 h (Zhang et al., 2016). The crude protein (CP) content was computed by multiplying TN content by 6.25 (Sun et al., 2021). The neutral detergent fiber (NDF) and acid detergent fiber (ADF) contents of fresh material were determined by the methods of Van Soest et al. (1991). The WSC was determined using the method of Thomas (1977).
Each polyethylene bag was opened on a clean bench. About 10 g of fresh forage or silage was blended with 90 ml of sterilized water, and the extract was serially diluted to quantify the bacterial group in a sterile solution. The numbers of LAB, aerobic bacteria, coliform bacteria, yeast, and molds were measured through the method of Sun et al. (2021).
Last, the liquid extract was filtered through four layers of cheesecloth and filtered paper. The prepared filtrate was used to analyze the pH values, organic acids, and ammonia nitrogen (NH3–N) of the sample. The lactic acid (LA), acetic acid (AA), propionic acid (PA), and butyric acid (BA) content of silage was analyzed by high-performance liquid chromatography according to the method of Cheng et al. (2021). The nitrogen (NH3–N) content was determined according to the method of Broderick and Kang (1980).
DNA Extraction, Polymerase Chain Reaction Amplification, and Sequencing
The E.Z.N.A.® sample DNA kit was used to isolate bacterial community genomic DNA from native grass and silage samples. The reagent, which was developed to extract DNA from trace amounts of sample, has been demonstrated to be effective for preparing DNA from most bacteria. The blank was made of non-nuclear water. Total DNA was eluted in 50 L of elution buffer and stored at −80°C until it was measured in the PCR (LC-Bio Technology Co. Ltd., Hangzhou, Zhejiang Province, China).
Subsequently, extracted DNA samples were subjected to PCR amplification on bacteria 16S rDNA (V3 and V4 regions). For bacterial amplification, the following primers were used: 341F and 805R. The PCR reactions were conducted using the following program: 3 min of denaturation at 95°C, 27 cycles of 30 s at 95°C, 30 s for annealing at 55°C, and 45 s for elongation at 72°C, and a final extension at 72°C for 10 min. PCR reactions were performed in triplicate 20-μl mixture containing 4 μl of 5× FastPfu buffer, 2 μl of 2.5 mM dNTPs, 0.8 μl of each primer (5 μM), 0.4 μl of FastPfu Polymerase, and 10 ng of template DNA. Agarose gel electrophoresis [2% (w/w)] was used to confirm the PCR products. To eliminate the possibility of false-positive PCR results as a negative control, ultrapure water was used throughout the DNA extraction process instead of a sample solution. AMPure XT beads (Beckman Coulter Genomics, Danvers, MA, United States) were used to purify the PCR products, and Qubit was used to quantify them (Invitrogen, Carlsbad, CA, United States).
The PCR products were sequenced on an Illumina platform (Guangzhou Gene Denovo Co. Ltd., Guangzhou, China) after purification and quantification. Fqtrim was used to perform quality filtering on the raw reads under specific filtering conditions in order to obtain high-quality clean tags (v0.94). Vsearch software (v2.3.4) was used to filter chimeric sequences. QIIME2 was used to calculate alpha and beta diversity, with the same number of sequences extracted randomly by reducing the number of sequences to the minimum of some samples, and relative abundance (X bacteria count/total count) was used in bacteria taxonomy. The QIIME2 process was used to examine alpha and beta diversity. Blast was used to perform species annotation sequence alignment, and the alignment databases were SILVA and NT-16S.
Statistical Analyses
The data was subjected using two-way analysis of variance with the fixed effects of additives, ensiling time, and additives × ensiling time using the general linear model (GLM) procedure of SAS 9.3 software (SAS Institute, Inc., Cary, NC, United States). The effect was considered significant when p < 0.05. The data of high-throughput sequencing were analyzed on the free online platform www.omicstudio.cn.
Results
Chemical Parameters and Microbial Compositions of Silage Materials
The chemical parameters and microbial compositions by native grass before ensiling are presented in Table 1. The DM, WSC, CP, NDF, and ADF were 37.72, 4.31, 10.83, 62.98, and 36.58%, respectively. Microbial compositions in the native grass for L, aerobic bacteria, coliform bacteria, and yeasts were 4.26, 8.75, 5.82, and 6.35 log10 cfu/g FM, respectively. Fresh native grass molds were below the detectable level.
Effect of Additives and Ensiling Days on Chemical Parameters of Native Grass Silage
Effects of additives and ensiling days on chemical parameters of native grass silages are shown in Table 2. The additive treatments significantly altered the contents of DM, CP, and WSC, but had no effects on NDF and ADF contents. L + M silage had higher WSC and CP contents and lower ADF and DNF contents compared with L and M silage after 60 days of silage. Ensiling days had significant (p < 0.05) effects on the chemical composition (except DM) of silage. All additives, except for the CK treatment, significantly decreased (p < 0.05) NDF content of native grass silage. In addition, the L + M silage had significantly (p < 0.05) higher CP contents than other silage at the end of ensiling. The WSC contents in M and M + L silages were significantly (p < 0.05) lower than the CK silage. The NDF and ADF content exhibited a continuous downtrend during the whole ensiling process. The interaction of additive treatments and ensiling days had a significant impact on silage WSC (p < 0.05), but did not affect the silage DM, CP, NDF, or ADF contents (p > 0.05).
Effect of Additives and Ensiling Days on Fermentation Quality of Native Grass Silage
Table 3 illustrates the dynamics of the fermentation quality of native grass silage during ensiling. These parameters were significantly affected by additive treatments, ensiling days, and their interaction (P < 0.05). Compared with the control, all additives significantly (p < 0.05) increased lactic acid (LA) concentration, and decreased acetic acid (AA), propionic acid (PA), and NH3–N concentration in silage. Compared with the other treatments, L + M addition further increased LA concentration and decreased the contents of AA, PA, and NH3–N; butyric acid (BA) was not detected in the native grass silage. The highest LA concentration was recorded in L + M silage, which had a value of 29.94% on the 60th day of ensiling (p < 0.05). The pH values of the additive silages were significantly decreased (p < 0.05) compared with the control during 14 days of ensiling, and L + M silage always maintained a lower pH value during ensiling. Moreover, NH3–N content exhibited a continuous increasing trend during the whole ensiling process, and the content of NH3–N in the L + M treatment group remained low after 14 days of ensiling.
Effect of Additives and Ensiling Days on Microorganism Counts of Native Grass Silage
Table 4 shows the effects of additives and ensiling days on microbial counts of native grass silages. Overall, additive treatments, ensiling days, and their interaction had a significant impact on microbial counts (p < 0.05). Additive treatment silages had lower (p < 0.05) coliform bacteria content and higher L and yeast numbers compared with the control silage after 60 days of ensiling. Compared with other treatments, the L and yeast contents in L + M-treated silage were significantly (p < 0.05) higher, while the coliform bacteria contents were lower than that of other silages at the end of ensiling. The microbial count of silages exhibited a continuous decreasing trend during the whole ensiling process. Mold counts were not detected in any treatment after 14 days of ensiling.
Microbial Community of Native Grass Silage
The bacterial diversity of native grass silage was discovered using high-throughput analyses targeting variable regions 3 and 4 of 16S rDNA. The valid sequences of all 51 triplicate samples added up to 1,294,499 after unqualified sequences were removed. The average Good’s coverage for all of the samples was greater than 99%, indicating that the sequencing depth was sufficient for reliable bacterial community analysis (Table 5). The interaction of additive treatments and ensiling days had a significant impact on Simpson and Shannon (p < 0.05), but did not affect Reads, OUT, and Chao1 (p > 0.05). According to the OTU and Chao 1 indexes, the bacterial community’s richness decreased with increased ensiling time in the CK silage. The Shannon and Simpson indexes were remarkably lower in the M and L + M-treated silages than in the CK silage at days 7 and 14 of ensiling (p < 0.05). In addition, the Chao 1 index and Simpson index showed trends similar to the trend in OTUs. Compared with other treatment groups, L + M addition resulted in the decrease in the richness of the bacterial community without dose effect in silage at day 60 of ensiling (p > 0.05). The lowest Shannon index of bacterial diversity was observed in the L + M-treated silage (i.e., 3.01).
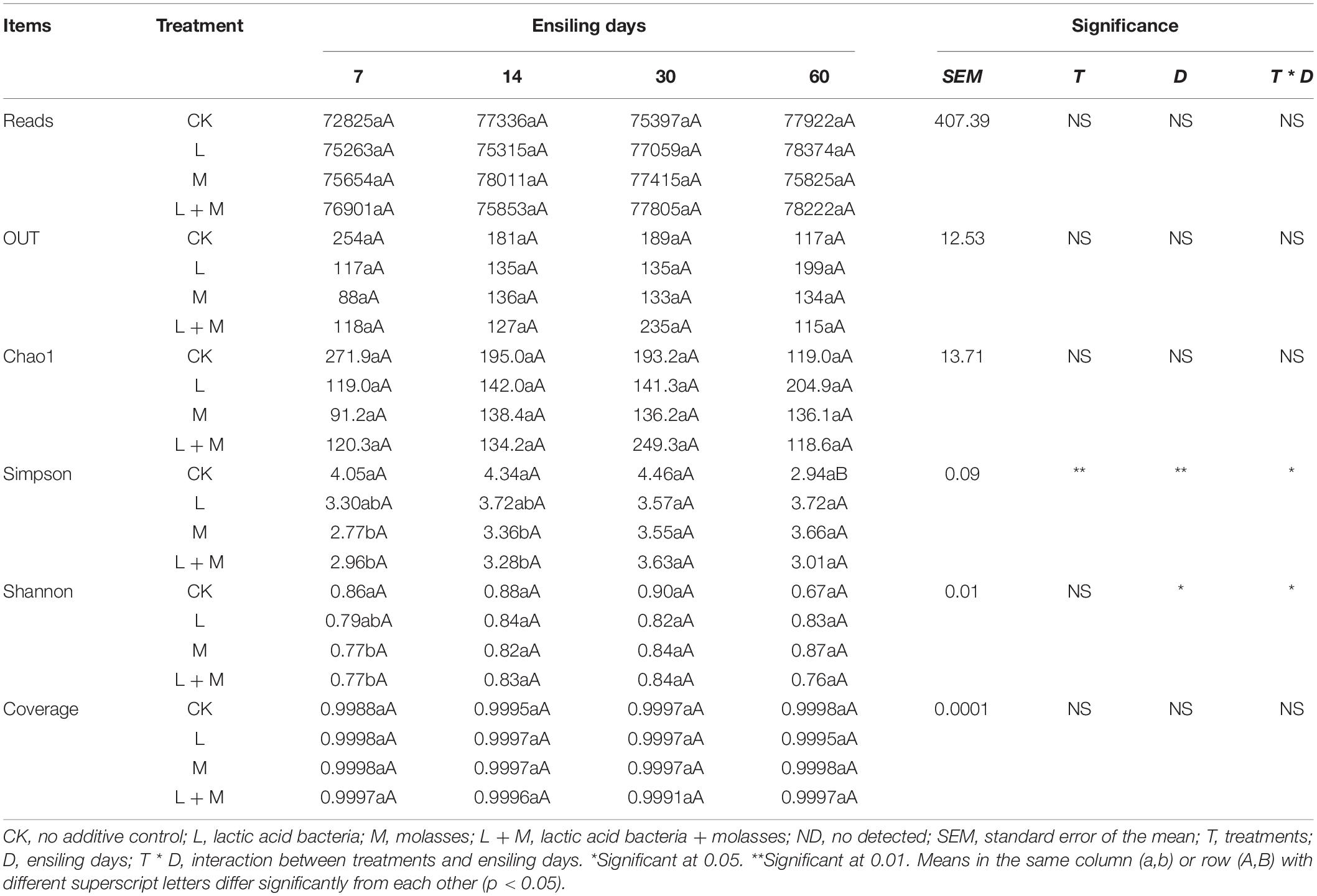
Table 5. Effect of additives and ensiling days on bacterial alpha diversity of native grass silages.
The PCoA analysis (Figure 1) showed the differences in bacterial communities between fresh samples and different additive treatments. The bacterial communities of fresh samples and different additive treatments were significantly separated, indicating that the microbial communities of fresh samples and different additive treatments changed during silage. A clear difference in the additive treatments in contrast with the control indicates that the additive treatments and control silage bacterial communities were different on day 60 of ensiling.
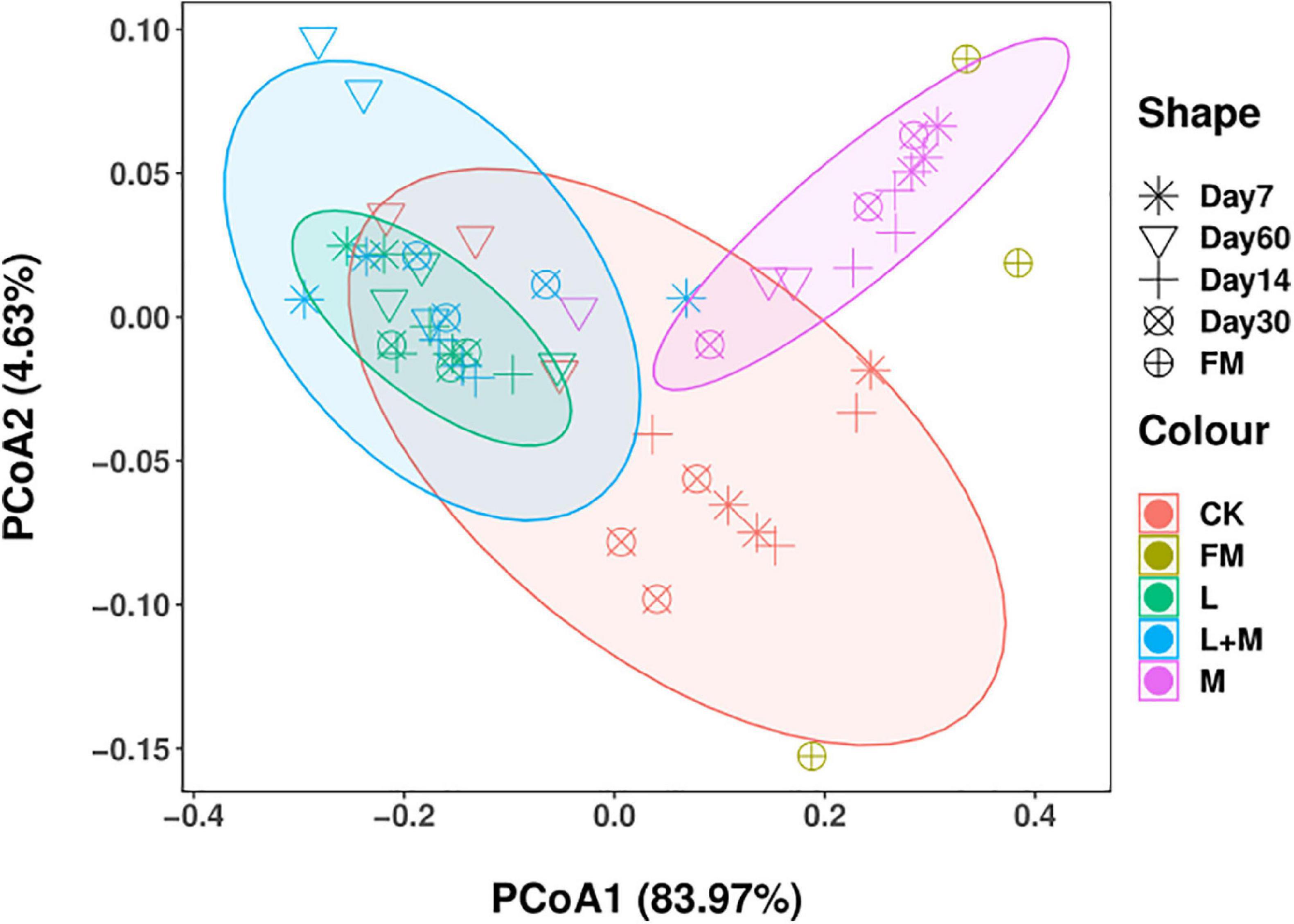
Figure 1. The community dissimilarities in different additives treatments and fermentation time, calculated via weighted UniFrac distances, with coordinates calculated using principal coordinates analysis (PCoA). FM, fresh material; CK, no additive control; L, lactic acid bacteria; M, molasses; L + M, lactic acid bacteria + molasses.
The dynamics of bacterial communities in native grass silage at the phylum level are shown in Figure 2A. Proteobacteria (89.82%) and Firmicutes (9.87%) dominated the majority of the total phylum sequence in the fresh sample. During the process of ensiling, Firmicutes and Proteobacteria dominated in all groups, but the community composition was affected by the ensiling treatments. Compared with the CK treatment, Firmicutes increased and Proteobacteria decreased in the L and L + M-treated groups. However, the relative abundance of Proteobacteria in M treatments was higher than that of the other treatments. In addition, the increase in Firmicutes abundance was greater with added L + M, and the relative abundance of Firmicutes (at 60 days) was higher.
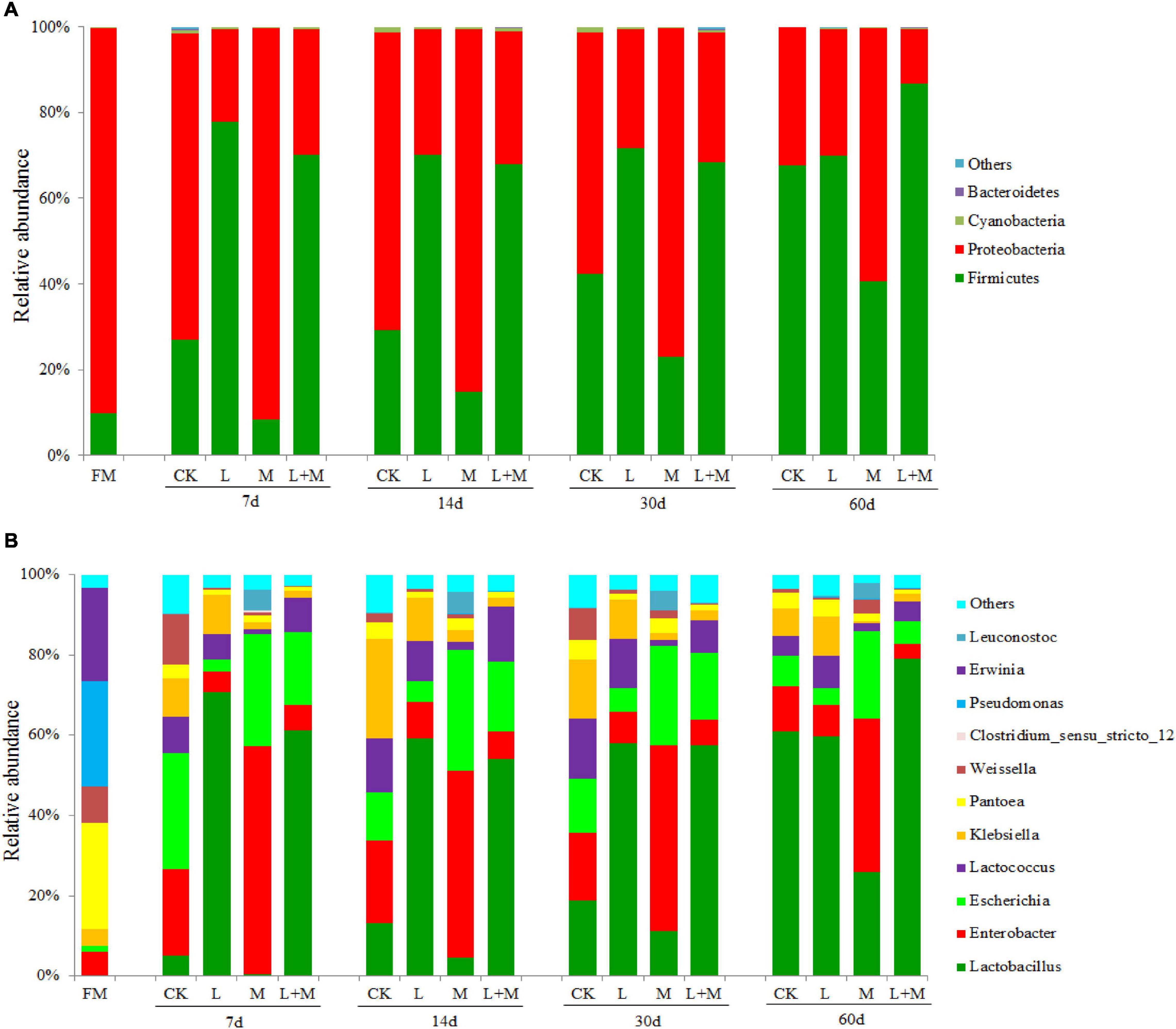
Figure 2. The bacterial abundance at phylum and genus level in native grass silage. FM, fresh material; CK, no additive control; L, lactic acid bacteria; M, molasses; L + M, lactic acid bacteria + molasses. The bacterial communities are shown at the phylum level (A) and the genus level (B).
Figure 2B illustrates the dynamics of bacterial populations in native grass silage at the genus level. The main epiphytic genera of fresh native grass were Pantoea and Pseudomonas, followed by Erwinia. Their abundance decreased greatly during the ensiling process, especially in the treated silage. Lactobacillus was the most abundant genus in silage with L. plantarum added (L and L + M; >54%) after 30 days of ensiling. The addition of L and L + M decreased the abundance of Enterobacter and Escherichia compared with silage with added M alone. In the absence of additives, after 60 days of ensiling, the dominant species were Pantoea and Lactobacillus. Under the influence of the fermentation promoter, there was a slight difference from the CK treatment. The main microbes were Lactobacillus (60.96%) and Enterobacter (11.26%) in the control group, whereas the main genera were Lactobacillus (58.29%), Enterobacter (7.72%), Escherichia (4.00%), and Pantoea (4.17%) in the L group. In the M group, the main genera were Lactobacillus (23.86%), Enterobacter (35.31%), Escherichia (20.12%), and Pantoea (1.74%), and the main genera were Lactobacillus (67.44%), Escherichia (4.85%), and Enterobacter (3.29%) in the L + M combination group.
To further reveal the effect of additives on the bacterial community in natural forage silage, one-way analysis of variance bar plots of the genus level among native grass silage groups after 60 days of ensiling are shown in Figure 3. In this study, Pantoea was the dominant genus in the fresh native grass (FM) (26.48%). Furthermore, Clostridium_sensu_stricto_12 was the subdominant family in FM, following Pantoea. The additive treatments significantly changed the relative abundance of Lactobacillus, Enterobacter, Escherichia, Pantoea, Pseudomonas, Clostridium_sensu_stricto_12, Erwinia, Klebsiella, and Lactococcus after 60 days of native grass silage. Lactobacillus was the most abundant genus in the L + M-treated silage (L + M; >65%). Molasses enhanced the growth of Enterobacter and Escherichia.
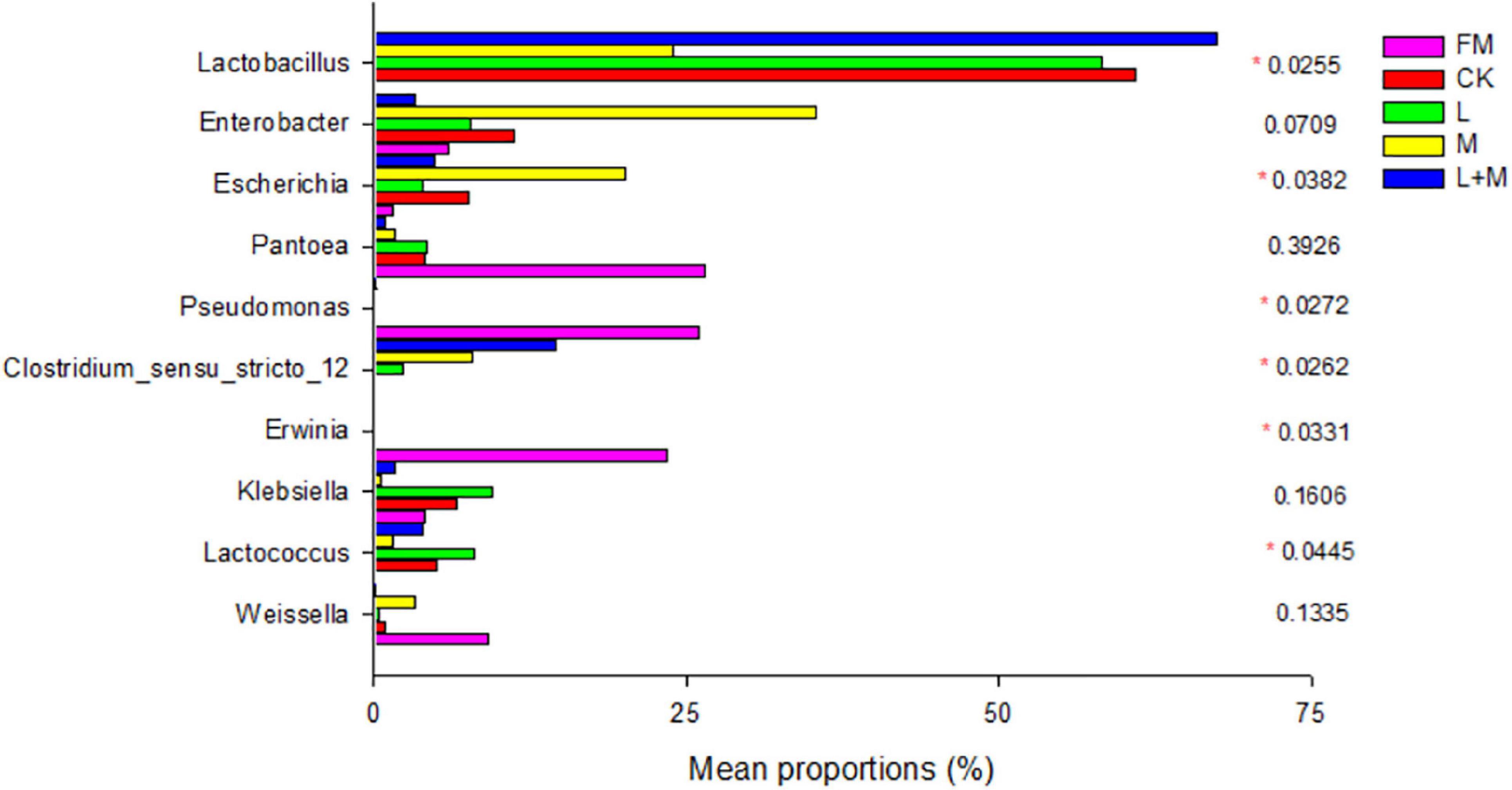
Figure 3. One-way analysis of variance bar plots of the genus level (10 most abundant genera) among native grass silage groups after 60 days of silage. *p < 0.05. FM, fresh material; CK, no additive control; L, lactic acid bacteria; M, molasses; L + M, lactic acid bacteria + molasses.
The effects of metabolic pathway changes in bacterial communities were quantified by predicting the functional characteristics of bacterial communities. Therefore, Figure 4 showed the metabolic pathways of silage treated with different additives. In the present study, higher relative abundance of carbohydrate metabolism was observed in the L-inoculated silage during the early phase of ensiling (7 days). With increased fermentation time, the relative abundance of carbohydrate metabolism gradually increased. Amino acid metabolism was lowest in the M-inoculated silage from 7 days until the end of ensiling. Metabolism of other amino acids showed the same pattern. The relative abundance of energy metabolism in L and L + M silages were higher than that of the CK and M treatments during the entire ensiling period, and nucleotide metabolism showed the same trend. After 60 days of fermentation of silage treated with additives, the metabolism of cofactors and vitamins were increased.
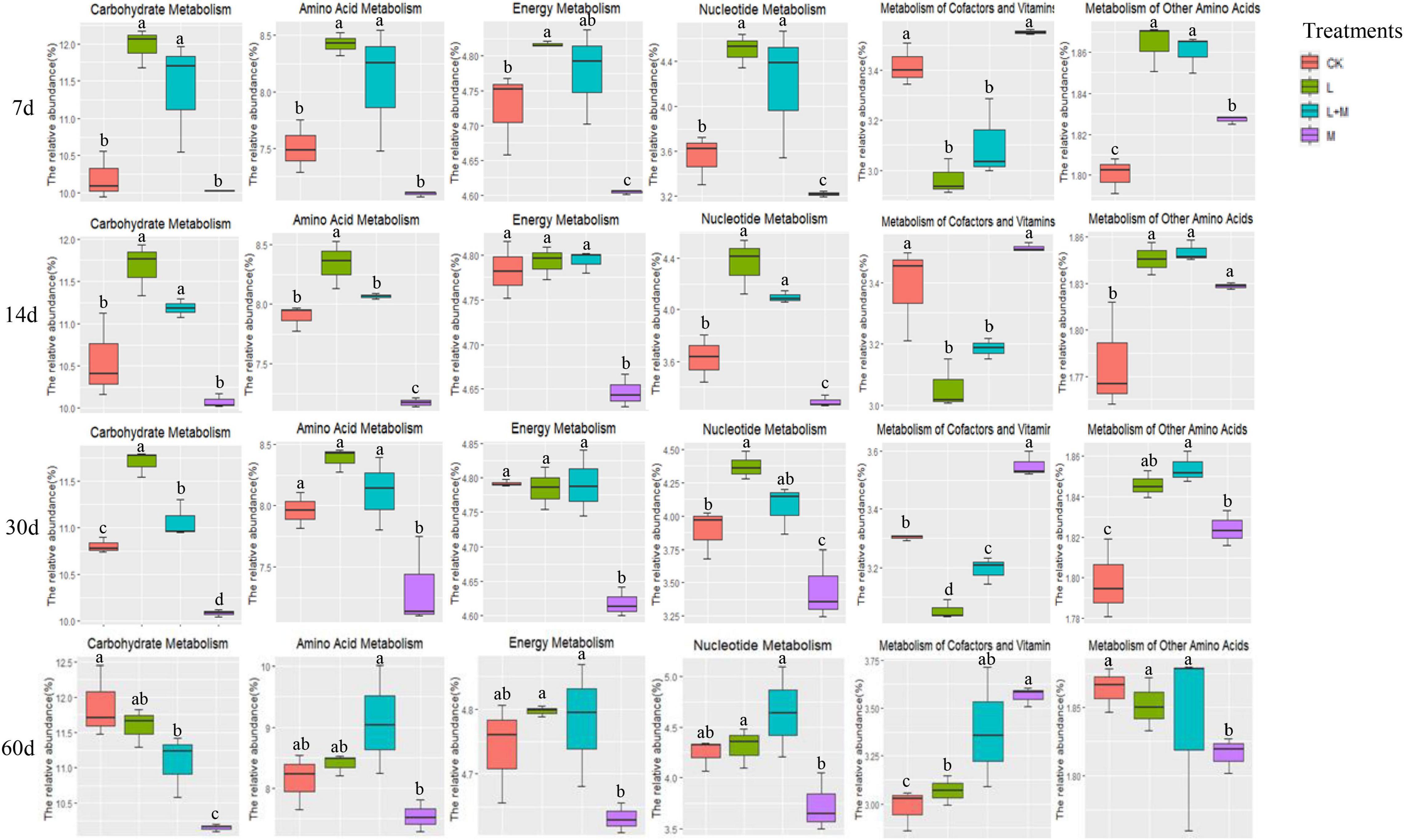
Figure 4. Statistics of additives and ensiling days on the abundance of native grass silage microorganism KEGG pathway. Summary of significant functional shifts predicted using Phylogenetic Investigation of Communities by Reconstruction of Unobserved States (PICRUSt). For each KEGG pathway, the second level of the predicted functional shift is shown with respect to the fermentation processes and additive treatments. a–d indicates significant differences between different additive treatments in the same silage period at p < 0.05. CK, no additive control; L, lactic acid bacteria; M, molasses; L + M, lactic acid bacteria + molasses.
Discussion
The amount of epiphytic L to raw materials is the key factor for the success of silage (Cai et al., 1999). The amount of epiphytic L is more than 105 cfu g–1 of FM; it is easier for silage to be successful and preserved (Cai et al., 1999). In addition, WSC is a significant factor for silage fermentation; the content of WSC in raw material is more than 5% DM, which can ensure the success of silage fermentation (Moselhy et al., 2015; Ni et al., 2017). The content of WSC and the number of epiphytic L influence the rate of increase in LA and drop in pH during the early stages of ensiling, which are critical for successful silage (Wang S. et al., 2019). In our study, the counts of L on native grass were <105 cfu g–1 of FM (Table 1), and the WSC content of FM was 4.31, which indicated difficulty in good preservation of native grass through ensiling without any additives. Thus, it was necessary to add exogenous L and/or M to enhance lactic acid fermentation during ensiling.
Silage treated with L + M had higher content of DM than that of other silages and could be attributed to molasses providing additional substrates, promoting sufficient lactic acid production, and resulting in pH reduction and DM loss prevention (Muck et al., 2018); this finding is similar to that of Chen et al. (2017). The addition treatment silages had lower NDF and ADF content than the control silages, which might be due to the additives encouraging microorganism proliferation and enhancing microbial respiration and fermentation of the fiber component. This finding is in line with those of Baytok et al. (2014) and Li et al. (2021). During the ensiling process of native grass, the content of CP significantly decreased. This was mostly due to the fact that some microorganisms involved in silage fermentation degrade protein (Du Z. M. et al., 2020). Sufficient content of WSC could provide more substance for lactic acid fermentation. The study of Ge et al. (2018) found that inoculating with L would ensure efficient conversion and utilization of WSC during ensiling, followed by a considerable drop in pH and reduction of nutrient loss, because L transformed the WSC into lactic acid during the early stage of fermentation. Therefore, the addition of M and L prior to ensiling seems to be necessary for high-quality native grass silage.
Ensiling is a complex bacterial fermentation process that causes a buildup of organic acid and a drop in pH (Ding et al., 2020). Silage pH is a key indication for determining fermentation quality, with a pH of 4.2 or below indicating well-fermented silage (Mcdonald et al., 1991). All of the treatments in our research had lower pH values than the CK treatment for the whole ensiling time, and a combination of L and M had the highest reduction in pH among the treatments. In this paper, it was found that the decrease in pH value occurred mainly in the first 7 or 14 days of silage, and then decreased with the increase in silage time, which was following the report of Ni et al. (2017). After 60 days of ensiling, the pH values of the L + M treatments declined to below 4.00, which could be due to the direct increase in fermentable substrate that promoted sufficient LA production and preserved silage due to the low pH, and which may also explain the decreased concentration of PA. In contrast, the pH value of the CK treatment remained above 4.20. The above results further showed that the addition of L and M prior to ensiling could stimulate the decrease in pH. In addition, L addition showed lower pH values than with M addition alone, which may be because the WSC were decomposed to a certain extent by L in the early ensiling stage, which, in turn, might accelerate the domination of L (Wang et al., 2021). Our present study showed that additive treatments decreased pH, AA, PA, and NH3–N contents, as a result of the increased LA contents in silage. The L and L + M silages had higher LA contents than other silage treatments. This may be because both additives contained L, which could initiate an accumulation of LA, thereby resulting in a decrease in the final pH of silage (Martinez-Fernandez et al., 2010). This is in line with studies on typical woody forage (Wang et al., 2021), fodder ramie (Ning et al., 2012), and soybean (Ni et al., 2017). NH3–N production is related to CP degradation in all silages and reveals the extent of proteolysis in silage (Wilkinson, 2005). Compared with the CK silage, adding L and M alone or in combination significantly decreased NH3–N content in silage, which might be because the addition of additives reduces the pH value, and the acidic environment reduced undesirable fermentation and proteolysis. After 60 days of ensiling, the decline in NH3–N content in L and L + M might be because some LAB can induce nitrification, which transformed ammonia nitrogen to nitrate nitrogen (Si et al., 2019). Similarly, Hou et al. (2017) found that additive treatments improved the fermentation quality of native grass silage. The abovementioned results indicated that L and M addition in the ensiling process could promote fermentation quality, and that the combination treatment enhanced the fermentation more efficiently.
Before ensiling, L, aerobic bacteria, coliform bacteria, molds, and yeasts are often found in Inner Mongolian native grasses. Aerobic bacteria are the dominating microorganisms during the initial stage of ensiling, resulting in some fermentation loss (Ge et al., 2018). In the present study, L counts of all silages during ensiling were >105 cfu g–1 of FM. Compared with the control group, the content of lactic acid bacteria in the additive treatment group increased by 12.4–17.9%, and the growth of non-important bacteria was inhibited. The physiological growth of L and an adequate supply of M as a substrate could explain an increase in L count (So et al., 2020). Control silage had no satisfactory microorganisms (e.g., aerobic bacteria and yeasts), while the addition treatments dramatically reduced the counts of aerobic bacteria and molds. This was most likely due to the additive treatments’ ability to create enough lactic acid to lower pH and limit the growth of other hazardous bacteria during silage fermentation (Henderson, 1993). Ni et al. (2017) discovered that a combination of L and 2% M might prevent the growth of clostridia and enterobacter in soybean silage. Overall, these findings demonstrated that additives can lower the number of unwanted microorganisms in native grass silage, with the L + M treatment having the greatest impact.
Bacteria in fresh native grass and silage samples were sequenced using amplicon sequencing. The recovered reads retrieved from each sample ranged from 69,317 to 78,375. All of the samples had coverage values of approximately 0.99. This demonstrates that the sequencing breadth was rather broad, and the microbial high-throughput data were sufficient to define the bacterial microbial community’s features (Yang et al., 2019). It is generally recognized that these specific OTUs may have contributed to differences in silage quality (Mu et al., 2021). The OTUs, Shannon index, and Chao1 value in L + M were lower after 60 days of ensiling than in CK, L, and M. Presumably, the combined addition of L and M was more likely to the native grass fermentation, thereby inhibiting the growth of other microorganisms, because the additive treatments decreased the pH, inhibited harmful microorganisms, and promoted the growth of L species. As a consequence, the silage of the combined addition of L and M in combination had the lowest microbial diversity and richness.
The quality of silage is determined by the outcome of the competition between L and spoilage microorganisms, as well as the competition and collaboration between L (Bai et al., 2021). The PCoA plot revealed a distinct separation of bacteria in FM and additive-treated silages, indicating that ensiling rebuilds the microbial community. These results are in line with the work of Zeng et al. (2020), who also found that the bacterial communities in FM and silages were distinct. In the present study, in the early fermentation stage (7 days), the bacterial communities in the L and L + M silages were clearly separated from the other groups. This might be due to L quickly initiating lactic acid fermentation, lowering the pH, and as such impacting bacterial community succession. In addition, compared with the control treatment, the PCoA of additive treated silages was also separate, which showed that additives significantly influenced the microbial community. The variation in bacterial community might explain the difference in silage quality (Ni et al., 2017; Dong et al., 2019; Li et al., 2021). Therefore, based on the results of α diversity and beta diversity, L and M treatments could affect the microbial diversity and community structure of native grass silage.
This study found that Proteobacteria was the most abundant phylum in FM, accounting for more than 85%, which is consistent with previous studies that Proteobacteria was the dominant phylum in fresh native grass (You et al., 2021). In the present study, Firmicutes and Proteobacteria were prevalent in all treatments in our research, and the community composition altered with ensiling time. Compared with the CK group, Firmicutes increased, while Proteobacteria declined in the L and L + M groups. For maize stover and red clover silage, Xu et al. (2017) and Dong et al. (2019) found comparable findings, which might be explained by the higher microbial populations of L (You et al., 2021). Proteobacteria have a major role in polysaccharide utilization, organic matter degradation, and carbon cycling (Ma et al., 2018). The higher relative abundance of Proteobacteria in M compared with other treatments (Figure 2A) may have been due to increased fermentation substrates, which can be rapidly hydrolyzed and utilized of M by Proteobacteria, as was also observed by Mu et al. (2021).
In the present study, Pantoea was found to be the most common facultative aerobic genus in FM. Pantoea has been observed in fresh native grass (You et al., 2021), alfalfa (Sa et al., 2021), and soybean (Ni et al., 2017). Pantoea abundance decreased after 30 days of fermentation, which might be attributable to their great sensitivity to pH decline (Ogunade et al., 2018). Lactobacillus is a well-known microbe that decreases pH during the ensilaging process by producing lactic acid, which impacts the quality of the fermentation (Li et al., 2021). Lactobacillus, Weissella, and Leuconostoc are the genera with the most bacteria involved in lactic acid fermentation in silage (Yang et al., 2019), Similarly, Lactobacillus was the predominant genus in native grass silage treated with L + M. Ni et al. (2017) found a similar outcome when they ensiled soybean infected with L + M, and Lactobacillus became the dominating genus after silage fermentation was completed. Enterobacteria are facultative anaerobes (i.e., can live in anaerobic and acidic environments) and can metabolize WSCs and LA to produce AA, PA, and other fermentation products (Li et al., 2019; Wang Y. et al., 2019). Combined addition of M and L also contributed to the growth of Lactobacillus, and Lactobacillus abundance reached nearly 70% of the total population. It is known that lactic acid-producing cocci (Weissella, Leuconostocs, and Lactococcus) initiate lactic fermentation in the early ensiling process, whereas lactic acid rod (Lactobacillus) play a key role in pH reduction at the later stage (Cai et al., 1998). The high abundance of Lactobacillus in the L + M combined addition treatment could explain their relatively good fermentation quality compared with the other treatments (Ni et al., 2017; Li et al., 2021). Interestingly, in this study, the abundance of Enterobacter and Escherichia increased in M silage compared with other silages. This may be attributed to the separate addition of molasses providing a rich availability of WSC, which directly improves the competitiveness of Enterobacter and Escherichia in the silage, and thus, L could not quickly become a dominant flora. A similar trend was also observed by Mu et al. (2021). Combined addition of M and L treatments could increase the abundance of Lactobacillus, decrease the abundance of the Pseudomonas, and improve fermentation quality of native grass silage. Furthermore, their combination had a positive synergistic effect on silage fermentation and the microbial community.
Silage fermentation is a very complex biological process involving a variety of microorganisms that produces a variety of metabolites during ensiling by degrading substrates or transforming metabolites via sophisticated metabolic pathways (Guan et al., 2018). Carbohydrate, amino acid, energy and cofactor metabolism, and vitamin metabolism were found to be metabolic pathways linked to silage fermentation in previous investigations. As a result, we chose these metabolic pathways for statistical analysis, including nucleotide metabolism (Bai et al., 2021; Xu et al., 2021). In L and L + M-treated silage, carbohydrate metabolism was higher during the whole ensiling process. This might be because the addition of L accelerated the decomposition of WSC in the silage. Similarly, Bai et al. (2021) also discovered that the expression of the carbohydrate metabolism pathway was connected to the relative abundance of total L in the bacterial community. The abundance of amino acid metabolism, nucleotide metabolism, and cofactor and vitamin metabolism gradually increased with fermentation, which contradicted the findings of Sa et al. (2021) who observed that amino acid metabolism, nucleotide metabolism, and metabolism of cofactors and vitamins were decreased in the inoculated silages during the fermentation stage. This might be because the raw materials attached to L and WSC content were low, while the exogenous additives contributed to the increase in this metabolism.
Conclusion
The present study illustrated that additives could improve the silage quality of native grass to different degrees, and that native grass silage treated with combined addition of L and M had better fermentation quality than other treatments. The use of additives prior to ensiling could reduce undesirable microorganisms and improve the nutritional value of forage native grass silage, with L + M having the best effects. In summary, the results confirmed that lactic acid bacteria and molasses exerted a beneficial synergistic effect on silage fermentation, which effectively improved silage quality, enhanced the relative abundance of Lactobacillus, and inhibited the growth of undesirable microorganisms on native grass.
Data Availability Statement
The datasets presented in this study can be found in online repositories. The names of the repository/repositories and accession number(s) can be found below: https://www.ncbi.nlm.nih.gov/, PRJNA783886.
Author Contributions
YL methodology, visualization, validation, data curation, and wrote the original draft. SD and LS interpreted the data and edited the language. QC wrote, reviewed, and edited the manuscript. GG and ZW conceptualization, acquisition of funding, and writing—reviewing and editing of the manuscript. QL and JH software. YJ conceptualization and funding acquisition. All authors have read and agreed to the published version of the manuscript.
Funding
This work was supported by the Key Laboratory of Forage Cultivation, Processing and High Efficient Utilization of Ministry of Agriculture and Rural Affairs, the Key Laboratory of Grassland Resources of Ministry of Education, the program for the National Natural Science Foundation of China (31760710), National Key Research and Development Program (2017YFD0502103), and Technology Project of Inner Mongolia (2020GG0032), China.
Conflict of Interest
The authors declare that the research was conducted in the absence of any commercial or financial relationships that could be construed as a potential conflict of interest.
Publisher’s Note
All claims expressed in this article are solely those of the authors and do not necessarily represent those of their affiliated organizations, or those of the publisher, the editors and the reviewers. Any product that may be evaluated in this article, or claim that may be made by its manufacturer, is not guaranteed or endorsed by the publisher.
References
Bai, J., Ding, Z. T., Ke, W. C., Xu, D. M., Wang, M. S., Huang, W. K., et al. (2021). Different lactic acid bacteria and their combinations regulated the fermentation process of ensiled alfalfa: ensiling characteristics, dynamics of bacterial community and their functional shifts. Microb. Biotechnol. 14, 1171–1182. doi: 10.1111/1751-7915.13785
Baytok, E., Aksu, T., Karsli, M. A., and Muruz, H. (2014). The effects of formic acid, molasses and inoculant as silage additives on corn silage composition and ruminal fermentation characteristics in sheep. Turkish J. Vet. Anim. Sci. 29, 469–474. doi: 10.1021/am506877b
Broderick, G. A., and Kang, J. H. (1980). Automated simultaneous determination of ammonia and total amino acids in ruminal fluid and in vitro media. J. Dairy Sci. 63, 64–75. doi: 10.3168/jds.S0022-0302(80)82888-8
Cai, Y. M., Benno, Y., Ogawa, M., and Kumai, S. (1999). Effect of applying lactic acid bacteria isolated from forage crops on fermentation characteristics and aerobic deterioration of silage. J. Dairy Sci. 82, 520–526. doi: 10.3168/jds.S0022-0302(99)75263-X
Cai, Y. M., Benno, Y., Ogawa, M., Ohmomo, S., and Nakase, T. (1998). Influence of Lactobacillus spp. from an Inoculant and of Weissella and Leuconostoc spp. from forage crops on silage fermentation. Appl. Environ. Microbiol. 64, 2982–2987. doi: 10.1128/aem.64.8.2982-2987.1998
Cai, Y. M., Du, Z. M., Yamasaki, S., Nguluve, D., Tinga, B., Macome, F., et al. (2020). Influence of microbial additive on microbial populations, ensiling characteristics, and spoilage loss of delayed sealing silage of Napier grass. Asian Australas J. Anim. Sci. 33, 1103–1112. doi: 10.5713/ajas.19.0471
Chen, X., Li, W., Gao, C., Zhang, X., Weng, B., and Cai, Y. M. (2017). Silage preparation and fermentation quality of kudzu, sugarcane top and their mixture treated with lactic acid bacteria, molasses and cellulase. Anim. Sci. J. 88, 1715–1721. doi: 10.1111/asj.12840
Cheng, Q. M., Li, P., Xiao, B., Yang, F., Li, D., Ge, G. T., et al. (2021). Effects of LAB inoculant and cellulase on the fermentation quality and chemical composition of forage soybean silage prepared with corn stover. Grassl. Sci. 67, 83–90. doi: 10.1111/grs.12289
Ding, Z., Bai, J., Xu, D., Li, F., Zhang, Y., and Guo, X. (2020). Microbial community dynamics and natural fermentation profiles of ensiled alpine grass Elymus nutans prepared from different regions of the qinghai-tibetan plateau. Front. Microbiol. 11:855. doi: 10.3389/fmicb.2020.00855
Dong, Z., Li, J., Chen, L., Wang, S., and Shao, T. (2019). Effects of freeze–thaw event on microbial community dynamics during red clover ensiling. Front. Microbiol. 10:1559. doi: 10.3389/fmicb.2019.01559
Driehuis, F. (2013). Silage and the safety and quality of dairy foods: a review. Agric. Food Sci. 22, 16–34. doi: 10.23986/afsci.6699
Du, S., You, S. H., Bao, J., Ge, G. T., and Cai, Y. M. (2020). Growth performance, carcass characteristics, and meat quality of Mongolian lambs fed native grass or hay with or without concentrate on the Inner Mongolian Plateau. Can. J. Anim. Sci. 100, 470–478. doi: 10.1139/cjas-2019-0126
Du, Z. M., Risu, N., Ge, G. T., Jia, Y. S., and Cai, Y. M. (2020). Dynamic changes and characterization of the protein and carbohydrate fractions of native grass grown in Inner Mongolia during ensiling and the aerobic stage. Asian Australas J. Anim. Sci. 33, 556–567. doi: 10.5713/ajas.19.0212
Ge, G. T., Hou, M. L., Liu, T. Y., Jia, Y. S., and Cai, Y. M. (2018). Microbial population, chemical composition and silage fermentation of native grasses growing on the Inner Mongolian Plateau. Grassl. Sci. 64, 226–233. doi: 10.1111/grs.12207
Guan, H., Yan, Y. H., Li, X. L., Li, X. M., Shuai, Y., Feng, G. Y., et al. (2018). Microbial communities and natural fermentation of corn silages prepared with farm bunker-silo in Southwest China. Bioresour. Technol. 265, 282–290. doi: 10.1016/j.biortech.2018.06.018
Henderson, N. (1993). Silage additives. Anim. Feed Sci. Technol. 45, 35–56. doi: 10.1016/0377-8401(93)90070-Z
Hou, M., Ge, G. T., Liu, T. Y., Jia, Y. S., and Cai, Y. M. (2017). Silage preparation and fermentation quality of natural grasses treated with lactic acid bacteria and cellulase in meadow steppe and typical steppe. Asian Australas J. Anim. Sci. 30, 788–796. doi: 10.5713/ajas.16.0578
Kang, L., Han, X., Zhang, Z., and Sun, O. J. (2007). Grassland ecosystems in China: review of current knowledge and research advancement. Philos. Trans. R. Soc. Lond. 362, 997–1008. doi: 10.1098/rstb.2007.2029
Khota, W., Pholsen, S., Higgs, D., and Cai, Y. M. (2016). Natural lactic acid bacteria population of tropical grasses and their fermentation factor analysis of silage prepared with cellulase and inoculant. J. Dairy Sci. 99, 9768–9781. doi: 10.3168/jds.2016-11180
Lee, I. S., Lee, S. Y., Choi, M. K., Kang, C. H., and Kim, J. M. (2018). Evaluation of the fermentation ability of lactic acid bacteria to manufacture highest quality rice straw silage. Korean J. Crop Sci. 63, 106–111. doi: 10.7740/kjcs.2018.63.2.106
Li, D. X., Ni, K. K., Zhang, Y. C., Lin, Y. L., and Yang, F. Y. (2019). Fermentation characteristics, chemical composition and microbial community of tropical forage silage under different temperatures. Asian Australas J. Anim. Sci. 32, 665–674. doi: 10.5713/ajas.18.0085
Li, M., Zi, X., Zhou, H., Lv, R., Tang, J., and Cai, Y. (2021). Effect of lactic acid bacteria, molasses, and their combination on the fermentation quality and bacterial community of cassava foliage silage. Anim. Sci. J. 92, 1–10. doi: 10.1111/asj.13635
Long, R. J., Zhang, D. G., Wang, X., Hu, Z. Z., and Dong, S. K. (1999). Effect of strategic feed supplementation on productive and reproductive performance in yak cows. Prev. Vet. Med. 38, 195–206. doi: 10.1016/S0167-5877(98)00125-1
Ma, S. S., Fang, C., Sun, X. X., Han, L. J., He, X. Q., and Huang, G. Q. (2018). Bacterial community succession during pig manure and wheat straw aerobic composting covered with a semi-permeable membrane under slight positive pressure. Bioresour. Technol. 259, 221–227. doi: 10.1016/j.biortech.2018.03.054
Martinez-Fernandez, A., Soldado, A., Vicente, F., Martinez, A., and de la Roza-Delgado, B. (2010). Wilting and inoculation of Lactobacillus buchneri on intercropped triticale-fava silage: effects on nutritive, fermentative and aerobic stability characteristics. Agric. Food Sci. 19, 302–312. doi: 10.2137/145960610794197597
Mcdonald, P., Henderson, A. R., and Heron, S. (1991). The Biochemistry Of Silage, 2nd Edn. Marlow: Chalcombe Publications.
Moselhy, M. A., Borba, J. P., and Borba, A. (2015). Improving the nutritive value, in vitro digestibility and aerobic stability of Hedychium gardnerianum silage through application of additives at ensiling time. Anim. Feed Sci. Technol. 206, 8–18. doi: 10.1016/j.anifeedsci.2015.05.001
Mu, L., Xie, Z., Hu, L., Chen, G., and Zhang, Z. (2021). Lactobacillus plantarum and molasses alter dynamic chemical composition, microbial community and aerobic stability of mixed (amaranth and rice straw) silage. J. Sci. Food Agric. 101, 5225–5235. doi: 10.1002/jsfa.11171
Muck, R. E., Nadeau, E., Mcallister, T. A., Contreras-Govea, F. E., and Kung, L. J. (2018). Silage review: recent advances and future uses of silage additives. J. Dairy Sci. 101, 3980–4000. doi: 10.3168/jds.2017-13839
Ni, K., Wang, F., Zhu, B., Yang, J., Zhou, G., Pan, Y., et al. (2017). Effects of lactic acid bacteria and molasses additives on the microbial community and fermentation quality of soybean silage. Bioresour. Technol. 238, 706–715. doi: 10.1016/j.biortech.2017.04.055
Ning, T. T., Xu, C. C., Wang, H. L., Hao, W., and Lei, H. (2012). Effects of ensiling fodder ramie (Boehmeria nivea L.) with lactic acid bacteria and molasses on fermentation quality. Adv. Mater. Res. 524-527, 2167–2171.
Ogunade, I. M., Jiang, Y., Cervantes, A. A. P., Kim, D. H., Oliveira, A. S., Vyas, D., et al. (2018). Bacterial diversity and composition of alfalfa silage as analyzed by Illumina MiSeq sequencing: effects of Escherichia coli O157:H7 and silage additives. J. Dairy Sci. 101, 2048–2059. doi: 10.3168/jds.2017-12876
Sa, D. W., Lu, Q., Wang, Z., Ge, G. T., Sun, L., and Jia, Y. S. (2021). The potential and effects of saline-alkali alfalfa microbiota under salt stress on the fermentation quality and microbial. BMC Microbiol. 21:149. doi: 10.1186/s12866-021-02213-2
Scarbrough, D. A., Coblentz, W. K., Coffey, K. P., Turner, J. E., Davis, G. V., Kellogg, D. W., et al. (2002). Effects of summer management and fall harvest date on ruminal in situ degradation of crude protein in stockpiled bermudagrass. Anim. Feed Sci. Technol. 96, 119–133. doi: 10.1016/S0377-8401(01)00349-2
Si, H. Z., Liu, H. L., Li, Z. P., Nan, W. X., Jin, C. N., Sui, Y. T., et al. (2019). Effect of Lactobacillus plantarum and Lactobacillus buchneri addition on fermentation, bacterial community and aerobic stability in lucerne silage. Anim. Prod. Sci. 59, 1528–1536.
So, S., Cherdthong, A., and Wanapat, M. (2020). Improving sugarcane bagasse quality as ruminant feed with Lactobacillus, cellulase, and molasses. J. Anim. Sci. Technol. 62, 648–658. doi: 10.5187/jast.2020.62.5.648
Sun, L., Na, N., Li, X., Li, Z., Wang, C., Wu, X., et al. (2021). Impact of packing density on the bacterial community, fermentation, and in vitro digestibility of whole-crop barley silage. Agriculture 11:672. doi: 10.3390/agriculture11070672
Thomas, T. A. (1977). An automated procedure for the determination of soluble carbohydrates in herbage. J. Sci. Food Agric. 28, 639–642. doi: 10.1002/jsfa.2740280711
Van Soest, P. J., Robertson, J. B., and Lewis, B. A. (1991). Methods for dietary fiber, neutral detergent fiber, and nonstarch polysaccharides in relation to animal nutrition. J. Dairy Sci. 74, 3583–3597. doi: 10.3168/jds.S0022-0302(91)78551-2
Wang, S., Guo, G., Li, J., Chen, L., Dong, Z., and Shao, T. (2019). Improvement of fermentation profile and structural carbohydrate compositions in mixed silages ensiled with fibrolytic enzymes, molasses and Lactobacillus plantarum MTD-1. Ital. J. Anim. Sci. 18, 328–335. doi: 10.1080/1828051X.2018.1528899
Wang, X., Liu, H., Xie, Y., Zhang, Y., Lin, Y., Zheng, Y., et al. (2021). Effect of sucrose and lactic acid bacteria additives on fermentation quality, chemical composition and protein fractions of two typical woody forage silages. Agriculture 11:256. doi: 10.3390/agriculture11030256
Wang, Y., He, L. W., Xing, Y. Q., Zhou, W., Pian, R. Q., Yang, F. Y., et al. (2019). Bacterial diversity and fermentation quality of Moringa oleifera leaves silage prepared with lactic acid bacteria inoculants and stored at different temperatures. Bioresour. Technol. 284, 349–358. doi: 10.1016/j.biortech.2019.03.139
Xu, D. M., Wang, N., Rinne, M., Ke, W. C., Weinberg, Z. G., Da, M., et al. (2021). The bacterial community and metabolome dynamics and their interactions modulate fermentation process of whole crop corn silage prepared with or without inoculants. Microb. Biotechnol. 14, 561–576. doi: 10.1111/1751-7915.13623
Xu, Z. S., He, H. Y., Zhang, S. S., and Kong, J. (2017). Effects of inoculants Lactobacillus brevis and Lactobacillus parafarraginis on the fermentation characteristics and microbial communities of corn stover silage. Sci. Rep. 7:13614. doi: 10.1038/s41598-017-14052-1
Yang, L., Yuan, X., Li, J., Dong, Z., and Shao, T. (2019). Dynamics of microbial community and fermentation quality during ensiling of sterile and nonsterile alfalfa with or without Lactobacillus plantarum inoculant. Bioresour. Technol. 275, 280–287. doi: 10.1016/j.biortech.2018.12.067
You, S. H., Du, S., Ge, G. T., Wan, T., and Jia, Y. S. (2021). Microbial community and fermentation characteristics of native grass prepared without or with isolated lactic acid bacteria on the mongolian plateau. Front. Microbiol. 12:731770. doi: 10.3389/fmicb.2021.731770
Zeng, T. R., Li, X. L., Guan, H., Yang, W. Y., Liu, W. G., Liu, J., et al. (2020). Dynamic microbial diversity and fermentation quality of the mixed silage of corn and soybean grown in strip intercropping system. Bioresour. Technol. 313:123655. doi: 10.1016/j.biortech.2020.123655
Zhang, Q., Wu, B. Y. L., Nishino, N., Wang, X. G., and Yu, Z. (2016). Fermentation and microbial population dynamics during the ensiling of native grass and subsequent exposure to air. Anim. Sci. J. 87, 389–397. doi: 10.1111/asj.12427
Zhao, J., Dong, Z., Li, J., Chen, L., Bai, Y., Jia, Y., et al. (2019). Effects of lactic acid bacteria and molasses on fermentation dynamics, structural and nonstructural carbohydrate composition and in vitro ruminal fermentation of rice straw silage. Asian Australas J. Anim. Sci. 32, 783–791. doi: 10.5713/ajas.18.0543
Zhou, J., Mi, J., Degen, A., Ding, L., Guo, X., Shang, Z., et al. (2017). Urinary purine derivatives excretion, rumen microbial nitrogen synthesis and the efficiency of utilization of recycled urea in Tibetan and fine-wool sheep. Anim. Feed Sci. Technol. 227, 24–31. doi: 10.1016/j.anifeedsci.2017.03.005
Keywords: native grass, lactic acid bacteria, molasses, microorganisms, silage
Citation: Li Y, Du S, Sun L, Cheng Q, Hao J, Lu Q, Ge G, Wang Z and Jia Y (2022) Effects of Lactic Acid Bacteria and Molasses Additives on Dynamic Fermentation Quality and Microbial Community of Native Grass Silage. Front. Microbiol. 13:830121. doi: 10.3389/fmicb.2022.830121
Received: 06 December 2021; Accepted: 26 January 2022;
Published: 16 March 2022.
Edited by:
Niranjan Koirala, Gandaki Province Academy of Science and Technology, Pokhara, NepalReviewed by:
Xianjun Yuan, Nanjing Agricultural University, ChinaRobin Anderson, Agricultural Research Service (USDA), United States
Copyright © 2022 Li, Du, Sun, Cheng, Hao, Lu, Ge, Wang and Jia. This is an open-access article distributed under the terms of the Creative Commons Attribution License (CC BY). The use, distribution or reproduction in other forums is permitted, provided the original author(s) and the copyright owner(s) are credited and that the original publication in this journal is cited, in accordance with accepted academic practice. No use, distribution or reproduction is permitted which does not comply with these terms.
*Correspondence: Yushan Jia, jys_nm@sina.com