- 1Department of Plant Science, School of Life Sciences, University of Hyderabad, Hyderabad, India
- 2Department of Biosensors and Nanotechnology, CSIR-Institute of Microbial Technology, Chandigarh, India
- 3Dairy Microbiology Division, ICAR-National Dairy Research Institute, Karnal, India
- 4Department of Dairy Microbiology, College of Dairy Science and Technology, Guru Angad Dev Veterinary and Animal Sciences University, Ludhiana, India
- 5Systems Microbiology and Natural Product Discovery Laboratory, Department of Animal Science, University of California, Davis, Davis, CA, United States
There has been growing interest on probiotics to enhance weight gain and disease resistance in young calves and to improve the milk yield in lactating animals by reducing the negative energy balance during the peak lactation period. While it has been well established that probiotics modulate the microbial community composition in the gastrointestinal tract, and a probiotic-mediated homeostasis in the rumen could improve feed conversation competence, volatile fatty acid production and nitrogen flow that enhances the milk composition as well as milk production, detailed changes on the molecular and metabolic level prompted by probiotic feed additives are still not understood. Moreover, as living biotherapeutic agents, probiotics have the potential to directly change the gene expression profile of animals by activating the signalling cascade in the host cells. Various direct and indirect components of probiotic approaches to improve the productivity of dairy animals are discussed in this review.
Introduction
Livestock production is a dynamic sector, critical to satisfying the growing demand for animal-sourced products and dairy products specifically have become a significant source of income for farmers contributing to the growth of developing economies (Thornton, 2010; Tona, 2021). The increasing pressure of the global population, limited arable land, and climate change has led to the urgent need of advanced approaches to enhance cow health and productivity and to make dairy production sustainable in a rapidly changing environment (Britt et al., 2018). Despite the increasing demand, the dairy sector in low- and middle-income countries is still struggling with the challenge of low animal productivity. The use of natural and inexpensive probiotics-based supplements as alternatives to antibiotics to promote animal growth and health has increased in recent years in the livestock industry, especially since the use of antibiotics as growth promoters has been strictly regulated in many countries to limit the evolution and distribution of antibiotic resistance through the food system (Sharma et al., 2018a).
Probiotics are living non-pathogenic microbes and in many cases are also naturally present to some extent in the gastrointestinal tract. Over the years numerous bacteria and fungi have been identified as probiotics (Table 1) (Choct, 2009; Puniya et al., 2015; Markowiak and Ślizewska, 2018; Zommiti and Ferchichi, 2021). Supplementation with probiotics aids in maintaining gut microflora homeostasis, which improves feed conversion efficiency ultimately resulting in increased milk and meat production (Jinturkar et al., 2009; Maake et al., 2021; Mani et al., 2021). Furthermore, probiotics have also been reported to reduce levels of stress-related markers, such as cortisol (Zhang et al., 2016). Here we have outlined major effects of commonly used probiotics on dairy animals’ health, nutrition, and productivity.
Impact of Probiotic Microorganisms on Animals’ Welfare
Dairy production is considered to be among the top industrial sectors and global milk production reached nearly 906 million tonnes in 2020 (FAO, 2021). Thereby, any undesirable shortcoming in milk production caused by disease or malnutrition in lactating animals could cause substantial economic losses and a heavy disease burden in the livestock chain could also lead to public health threats, such as the emergence of antibiotic resistance (Sharma et al., 2018a). Application of probiotics for maintaining the general health, immunity and nutritional requirement of dairy animals and other livestock could provide a sustainable solution to mitigate some of these problems.
Probiotics are live beneficial bacteria that, when administered in adequate amounts, confer a health benefit on the host (Hill et al., 2014), often by colonizing the gastrointestinal tract and supporting the native microflora that is already established in the animal’s digestive system. Indirectly, probiotics can also support mucosal immunity by promoting the beneficial mucosal microflora and preventing colonization of the mucosa by pathogens (Uyeno et al., 2015). In addition, the general health benefits of probiotics in the digestive system of ruminants also includes the control of acidosis, digestive comfort, reducing methanogenesis, promoting the growth of rumen and intestinal epithelium, increased nutrient uptake, and an increased feed conversion ratio (Abd El-Tawab et al., 2016; Retta, 2016). Reduced inflammation markers in the host are also linked to the establishment of probiotic mediated intestinal homeostasis that strengthens the animal’s natural defense mechanism and overall health (Figure 1). The different routes through which probiotics can benefit the general health of animals are summarized in the following sections.
Gut and Rumen Microflora Homeostasis
The microbiome of the gastrointestinal tract plays a critical role in the well-being of the host animal due to its ability to closely regulate the components of both innate and adaptive immunity. A healthy microflora keeps animal healthy by maintaining immunological homeostasis and removing pathogenic microorganisms (Wu and Wu, 2012; Butel, 2014). The rumen microbiome has also been proven to significantly impact the development of native and systemic immune components (Wu and Wu, 2012). The gut microbial ecosystem is made up of a diverse mix of bacteria, anaerobic fungi, and ciliated protozoa (Chaucheyras-Durand and Durand, 2010). Therefore, probiotics are primarily used to establish homeostasis conditions within the gut and rumen microflora by supporting the population of beneficial microbial species (Xu et al., 2017). Probiotics specifically enhance the relative abundance of beneficial genera that help to prevent pathogenic invasion into the gastrointestinal tract. For instance, Fernández-Ciganda et al. (2021) reported that supplementing two probiotic strains, Lactobacillus johnsonii TP1.6 and Lactobacillus reuteri TP1.3B, to young calves increased the abundance of beneficial taxa including Bifidobacterium and Akkermansia in the intestine. Similarly, Bacillus amyloliquefaciens fsznc-06 and Bacillus pumilus fsznc-09 enhanced the relative richness of potentially beneficial bacteria in the rumen and intestine of weanling goats (Zhang et al., 2020). This beneficial shift was also correlated with enhanced ruminal papilla and small intestinal villus growth of the studied animals. In another study, the effect of a multi-strain probiotic product containing Bifidobacterium animalis, Lactobacillus casei, Streptococcus faecalis, and Bacillus cerevisiae on general health and the fecal bacterial composition of Holstein’s calves were investigated and it was found that although the probiotic supplements did not affect the overall fecal microflora at the phylum level, the relative abundance of beneficial Prevotella increased while abundance of the opportunistic pathogens Dorea decreased (Guo et al., 2022), suggesting that this probiotic supplements supported an enhanced defence mechanism of the rumen and the intestine, potentially reducing the risk of colonization by pathogens.
Digestion Efficiency
Lignocellulosic plant fibers, which are significant components of the ruminant diet, require the symbiotic activity of diverse microorganisms to hydrolyze and extract nutrients from it. The rumen microbial community is a diverse blend of taxa from prokaryotic bacteria and archaea as well as eukaryotic fungi and protozoa. During the preweaning stage of life, the relative number of beneficial genera of Bacteroidetes, Firmicutes, Proteobacteria remains dominant, followed by Elusimicrobia, Fibrobacteres, Bifidobacterium, Tenericutes and Verrucomicrobia (Meale et al., 2016). However, at the later stage of life, change in feed shifts the microbial composition by significantly increasing the population of Proteobacteria and decreasing the number of Bacteroidetes, Elusimicrobia, Fibrobacteres, Tenericutes, and Verrucomicrobia in dairy calves (Meale et al., 2017). The alteration in the composition of the commensal microbial population could significantly influence the fermentation, digestion and nutrient uptake from the diet. Likewise, Zhang et al. (2016) suggested that the ratio of Bacteroidetes, Firmicutes, and Proteobacteria could improve the feed conversion efficiency and reduce enteric methane production from rumen fermentation process. The role of probiotics to improve the digestive efficiency and feed conversion ratio in dairy animals has also been observed by the researchers. A preliminary in vitro study suggested that probiotics could improve the rumen organic matter digestibility as well as reduce total gas and methane production (Ellis et al., 2016). Kondrashova et al. (2020) studied the possible effect of the combination of Bifidobacterium longum, Bifidobacterium adolescentis and Lactobacillus acidophilus with vanillin in rumen fluid on the metabolic state of rumen microflora. In vitro evaluation suggested that the combination of vanillin with these three probiotic strains increase the digestibility of the dry feed. Besides, probiotics could simultaneously affect the rumen fermentation and rumen microbial community structure to improve the digestive metabolism. For instance, the activity of Bacillus subtilis natto reportedly improved the concentrations of ammonia nitrogen, microbial protein and volatile fatty acids (VFA) in rumen fluid connected to the change in 18 genera of rumen fluid (Chang et al., 2021).
Probiotic-mediated mechanisms to improve the digestive efficiency also includes the control of diet-induced acidosis and detoxification of harmful metabolites. Lactating animals are usually kept on concentrated grain-rich diets that increase the risk of rumen acidosis due to access accumulation of organic acid and VFAs exceeding the buffering capacity of rumen. Rumen acidosis is one of the most prevalent digestive disorders seen in cattle, with ranging from sub-acute to acute severity (Nagaraja and Titgemeyer, 2007). The negative impact of rumen acidosis can also lead to additional severe health problems to the other organs, such as laminitis and liver swelling (Enemark, 2008). Earlier studies in this line have shown that live yeast can help to stabilize rumen pH and reduce susceptibility to acidosis in dairy animals (Chaucheyras-Durand et al., 2008; Marden et al., 2008; Maamouri and Ben Salem, 2021).
Besides concentrated grain, many dairy animals are often kept on a diet that is naturally high in nitrate to reduce enteric methane production. Microbial conversion of nitrate to ammonium also yields nitrite as toxic intermediate and accumulation of nitrite can cause severe methemoglobinemia and acute toxicosis in ruminants. Effects of nitrite toxicosis may also be subacute or chronic and can include delayed growth, decreased milk production, vitamin A deficiency, minor transitory goitrogenic effects, abortion, fetotoxicity, and increased susceptibility to infection. Other sources of disproportionally high nitrate concentration are run-off water or shallow wells in regions where large amounts of fertilizers are applied to the surface (Hall, 2018). Latham et al. (2019) noted that administration of the nitrite-metabolizing bacterium Paenibacillus fortis to the rumen could promote nitrite metabolism and thus remove the toxic nitrite produced from the rumen. Hence, the use of probiotic-supplement could enhance the digestion efficiency and reduce the accumulation of toxic metabolites in the animals’ digestive tract.
Intestinal Antimicrobial Activity
A major cause of reduced milk productivity is animal sickness during the lactation period (Carvalho et al., 2019). Different studies with in vitro models have proven that probiotic microorganisms which naturally colonize the digestive tract of the host animal show strong antimicrobial activity against enteric pathogens (Adeniyi et al., 2015; Lin et al., 2020). The antimicrobial activity of probiotics against the enteric pathogens suggests their utility as an effective biotherapeutic to prevent related gastrointestinal infections (Prabhurajeshwar and Chandrakanth, 2019). Specifically these native probiotics support the intestinal epithelial barrier by enhancing the expression of barrier function components (Rokana et al., 2016; Bron et al., 2017) and prevent the enteric infections in the host (Lucey et al., 2021). Many of the known Lactobacillus probiotic strains produce metabolites, such as diacetyl, acetoin, hydrogen peroxide, and bacteriocins, which prevent the growth of pathogens and assist in defence mechanisms that are involved keeping infections at bay (Osuntoki and Korie, 2010; Pyar and Peh, 2014). For example, Pediococcus pentosaceus strains LJR1, LJR5, and LJR9 isolated from the rumen fluid of a healthy goat exhibited antibacterial activity against a broad spectrum of foodborne pathogens such as Listeria monocytogenes, Escherichia coli and Streptococcus pyrogenes (Ladha and Jeevaratnam, 2018). Probiotics could also prevent infection by competing with pathogens for attachment to the intestinal epithelium (Rokana et al., 2017; Figure 2).
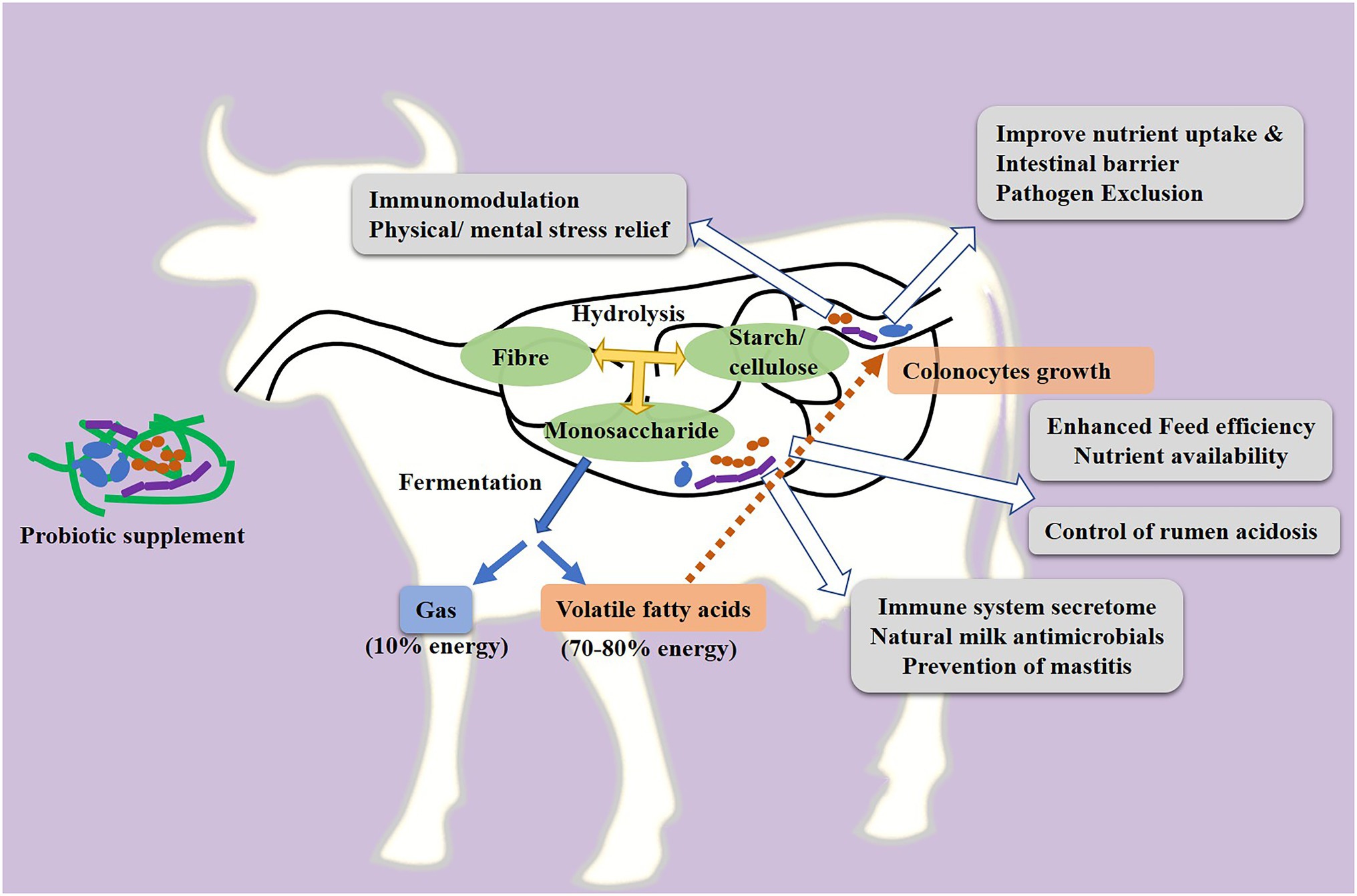
Figure 2. Probiotic activity to enhance the growth and general health of dairy animals. Yellow arrows in the figure indicate enzymatic digestion of feed in the gut; blue arrows indicate microbial fermentation of feed-derived monosaccharides; white arrows indicate the different beneficial mechanisms of probiotics, and the dotted red arrow indicates the VFA-mediated growth promotion of colonocytes.
The preventive mode of probiotic action against different types of infections is also currently being investigated. For instance, parasite-borne diseases, in addition to bacterial infections, are prevalent in dairy animals. The beneficial effects of probiotics on the recovery from parasite infections, such as helminths (e.g., Trichuris, Ascaris), Eimeria and Cryptosporidium, have been proven in several studies using various animal models (Travers et al., 2011). Furthermore, numerous farm-level case studies in poultry sector have shown that the use of probiotics (including Pediococcus, Lactobacillus, Enterococcus, Bifidobacterium, and Bacillus species) improved the host resistance to Eimeria-borne coccidiosis (Dalloul et al., 2003; Lee et al., 2007; Giannenas et al., 2012; Abdelrahman et al., 2014). Similarly, researchers have reviewed the possible approach of manipulation of ruminant gut microbiome to control the parasite infection in dairy animals (Cortés et al., 2020). Ramírez et al. (2021) have attepted to identify the compositional change in intestinal microflora of Fasciola hepatica infected Holstein cows. The study revealed reduced diversity in prokaryotic and eukaryotic microorganisms and a specific decrease in the relative abundance of Bacteroidetes and fungi Ascomycota in Fasciola infected cows. Earlier studies on probiotic-mediated gut microbiome manipulation suggest that probiotics could be used as an alternative strategy to ameliorate the helminth infections in dairy animals. However, more clinical research focusing on the probiotic mechanism in the presence of parasite infection is needed to determine their efficacy in this area.
The antiviral efficacy of probiotics against viruses that cause intestinal, urogenital and respiratory infections have also been successfully demonstrated (Arena et al., 2018). Probiotics may directly interact with viruses, inhibit them using antiviral metabolites or they may indirectly influence the host immune system against viral pathogenesis (Al Kassaa et al., 2014; Drider et al., 2016). The live cells of probiotics use adsorptive, trapping mechanisms or produce hydrogen peroxide, lactic acid and antimicrobial peptides to inactivate target viruses (Al Kassaa et al., 2014). Villena et al. (2018) have reviewed that these beneficial microbes could also stimulate the antiviral innate immune response by activating the expression of antiviral pattern recognition receptors in host cells that subsequently induce the secretion of nuclear factor κB pathway-related proinflammatory cytokines and chemokines. The activation of the nuclear factor κB dependent pathway help to reduce the severity of viral infection in the animal host (Villena et al., 2018). Even though these studies are limited to in vitro cell line models or small animals, the results are promising and comparable effects are anticipated to occur in extended in situ experiments. In addition to enteric, parasite and viral infections, mastitis is a highly prevalent disease in lactating dairy animals that causes a substantial economic loss to milk producers (Bhakat et al., 2020). Several probiotics administered orally or locally have shown their potential to reduce mastitis infection by modulating the intestinal microbial composition, immunological responses and by enhancing the epithelial barrier function (Figures 2 and 3; Tanbayeva et al., 2016; Armas et al., 2017; Rainard and Foucras, 2018; Pellegrino et al., 2019; Steinberg et al., 2021). Different types of preventive mechanisms have been proposed with multiple types of probiotics. For instance, Saccharomyces cerevisiae and Lactococcus species reduced the mastitis-related inflammation by lowering serum concentration of tumor necrosis factor-α (TNF-α), Interleukin-6 (IL-6), and IL-1β in dairy cows (Gao et al., 2020). Both probiotics also inhibited the neutrophil infiltration indicator, i.e., milk myeloperoxidase and a mastitis indicator, i.e., N-Acetyl-β-d-Glucosaminidase activity in milk suggesting a decline in mastitis related damage to mammary cells. Likewise, an in vitro study demonstrated the immunomodulatory potential of probiotic L. casei BL23 on bovine mammary epithelial cells infected with mastitis causing Staphylococcus aureus (Souza et al., 2018). The work indicated the indirect mechanism of probiotic action against mastitis pathogens. L. casei BL23 decreased the expression of several pro-inflammatory cytokines, including interleukins IL-6, IL-8, IL-1α and IL-1β and TNF-α in S. aureus-stimulated mammary epithelial cells. Interestingly, probiotics also slightly improved the expression of antimicrobial peptides and defensin β in host cells. Investigation of the entire mechanism showed that probiotic L. casei BL23 has helped to recover from mastitis infection by reducing inflammation and strengthening the cellular protection mechanism of bovine mammary cells. A study on heat-inactivated Lactobacillus gasseri LA806 to counteract S. aureus and E. coli infection in an in vitro model of bovine mastitis suggested that even inactivated probiotic cells could carry immunomodulatory properties (Blanchet et al., 2021). Observations recorded from these studies suggest that probiotics could modulate the host cell defense mechanism using the cell receptor-mediated channel.
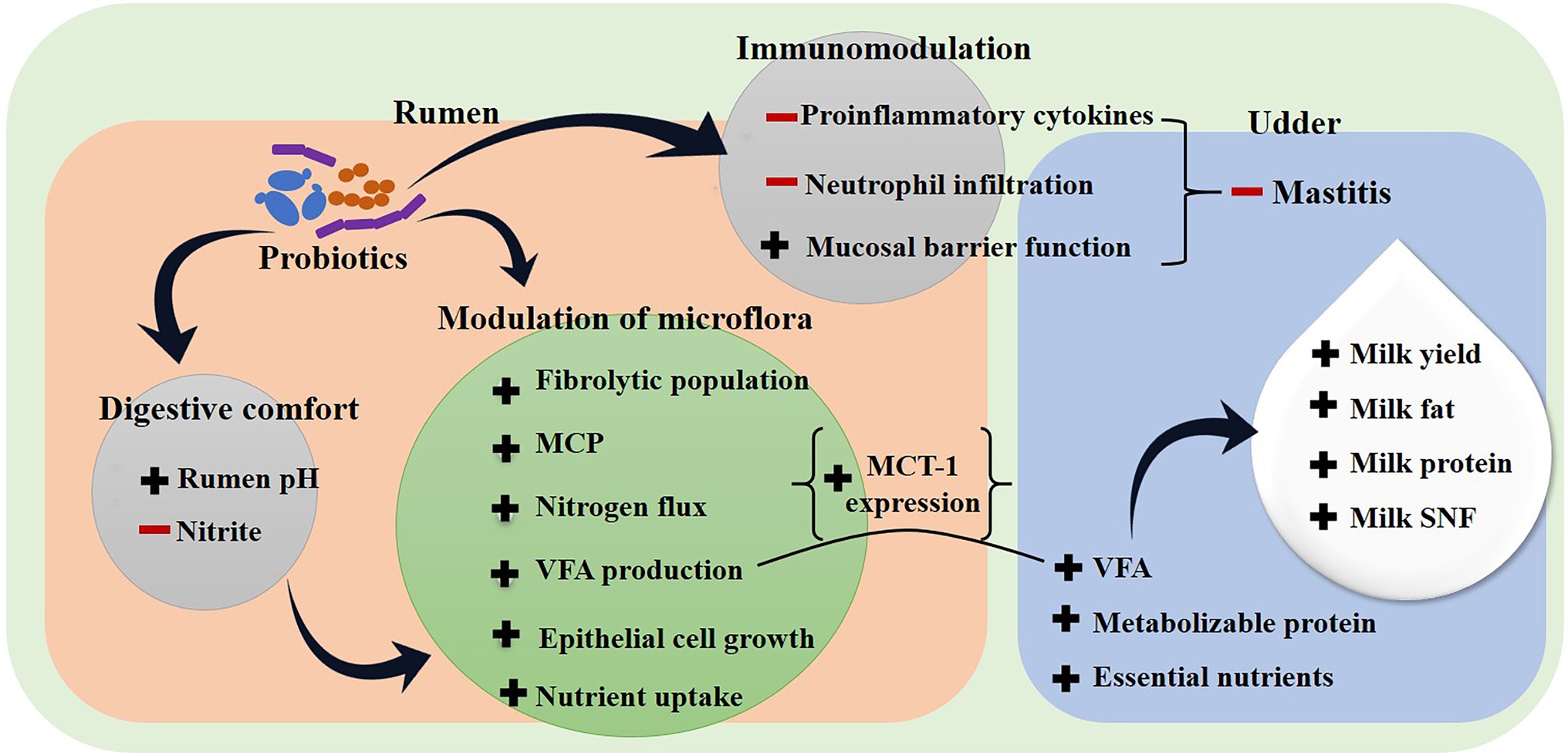
Figure 3. Effect of probiotics to improve milk productivity in dairy animals. (−) Sign indicates inhibition, (+) sign indicates enhancement, MCP: microbial crude protein, SNF: slid not fat, VFA: volatile fatty acid.
Stress Management via Modulation of the Gut-Brain Axis
The connection between intestinal microflora and the general health of the host has been well established and growing evidence suggests that the gut microbiome also impacts brain operation and cognitive behavior (Desbonnet et al., 2015) (Figure 2). Some important neurotransmitters related to mood regulation, i.e., serotonin, dopamine and aminobutyric acid, are produced in both gut and brain (Cheng et al., 2019). In addition, studies have supported the gut-brain axis theory by identifying the connection between the vagus nerve of the parasympathetic nervous system and gut microbiome (Bonaz et al., 2018). The functions of the vagus nerve include communication between the brain and intestinal epithelium that coordinates the individual’s adaptive response to environmental or physiological stress (Breit et al., 2018). The stress signals through the vagus nerve activate the pituitary–adrenal axis and a neural and hormonal communication that influence the activities of intestinal epithelial and immune cells (Breit et al., 2018). Studies have shown that the gut microbiome could influence the vagus nerve mediated effects using endocrine, immune or neural mechanisms (Fülling et al., 2019). The variety of the gut microbiome has been proven to regulate cognitive behavior by stimulating the synthesis of essential neurotrans-modulators (i.e., tryptophan and neuropeptides) in rodents (Desbonnet et al., 2015). The ability of probiotics to restore gut homeostasis has also been linked to reduced anxiety alleviating stress level in individuals (Scott et al., 2013; Zhang et al., 2019). A small number of investigations, involving farm animals, have revealed that the gut microbiome can modulate stress level in dairy animals in a similar way. Kelsey and Colpoys (2018) discovered that feeding probiotics can reduce stress-related behavior in weaned and developing calves. Based on these findings, probiotics may be used to help dairy animals to cope with stress behavior, but additional studies will be required to define the extent of how probiotics might facilitate improved stress response.
Probiotics to Promote the Growth of Dairy Animals
Emergence and manifestation of antibiotic resistance in the food chain has prompted a search for alternatives to antibiotics that have growth-promoting effects on livestock. Since the diversity of the rumen microbiome is closely related to the animal’s ability to acquire and assimilate nutrients, ideal growth promoters would have only a negligible impact on the animal’s natural microbiome, while enhancing the animal’s growth, well-being and reproduction (Breves et al., 2000; Mostafa et al., 2014). Many animal probiotics have been shown to improve feed efficiency (Moreira et al., 2019), growth performance (Tripathi and Karim, 2010; Didarkhah and Bashtani, 2018), nitrogen retention (Schofield et al., 2018) and also reduced the risk of intestinal infections (Aldana et al., 2009; Tripathi and Karim, 2010; Signorini et al., 2012; Didarkhah and Bashtani, 2018).
Primarily, probiotics could improve the rumen and intestinal epithelial cells growth that enhance nutrition uptake capacity. Probiotics implement these beneficial effects by improving the production of VFAs that act as growth promoters for epithelial cells (Figure 2). An investigation on reweaning calves by Stefańska et al. (2021) explains the possible mechanism behind it. The calves received a multi strain probiotics feed additive containing Lactobacillus casei PKM B/00103, Lactobacillus salivarius PKM B/00102 and Lactobacillus sakei PKM B/00101 that improved ruminal fermentation and increased the concentration of total VFA, propionate, butyrate and the ratio of butyrate to valerate. The change in VFA profile further enhanced the total dry matter intake and growth of reweaning calves. Another interesting study on growth delayed calves by Du et al. (2018) has also presented similar observations. Supplementation of probiotics B. amyloliquefaciens and B. subtilis improved feed intake, energy and short-chain fatty acid production by increasing the number of intestinal fiber degrading bacteria, including Proteobacteria, Rhodospirillaceae, Campylobacterales, and Butyricimonas. Additionally, probiotic supplement improved the growth hormone/insulin-like grows factor-I ratio that enhanced body weights of growth delayed calves. Comparable observations are also recorded in sheep and lambs by Devyatkin et al. (2021). They found that supplementation of a commercial probiotics preparation Enzimsporin (consisting of Bacillus subtilis B-2998D, B-3057D, and Bacillus licheniformis B-2999D) increased body weight gain of sheep and lambs. The growth-promoting effect was related to the increasing intestinal Lactobacillus and Bifidobacterium population and decreasing E. coli, Enterococcus, and yeast in the fecal contents.
The growth promotion of dairy animals is also related to the nutrition extraction and retention from the provided feed. Schofield et al. (2018) found that probiotic Bacillus amyloliquefaciens H57 could benefit the ruminant host by increasing nitrogen retention, feed intake, weight gain and reducing the methane emission in ruminants (Table 2). The probiotic indirectly induced these effects by increasing the proliferation of plant fiber digesting Prevotella species and Roseburia faecis in the rumen. Likewise, Chen et al. (2021) observed the enhanced average daily gain in ruminant receiving feed supplemented with a combination of three probiotics, Bacillus licheniformis, Bacillus subtilis and Lactobacillus plantarum blended with a Chinese medicine polysaccharide. The probiotic effect was mediated by increasing the relative abundance of Fibrobacteria that promoted rumen protein fermentation. Probiotics could also target fibrolytic eukaryotic commensal microflora to improve nitrogen utilization from the roughage. Phesatcha et al. (2022) have determined the influence of adding Saccharomyces cerevisiae to the diet in cattle. The outcomes of the study revealed that yeast supplementation increased the nutritional digestibility and efficiency of microbial protein nitrogen production and nitrogen absorption while increasing fibrolytic fungi population and lowering the protozoa population. These results suggest that probiotics can improve the nutrition availability and physical growth that support the productivity of dairy animals.
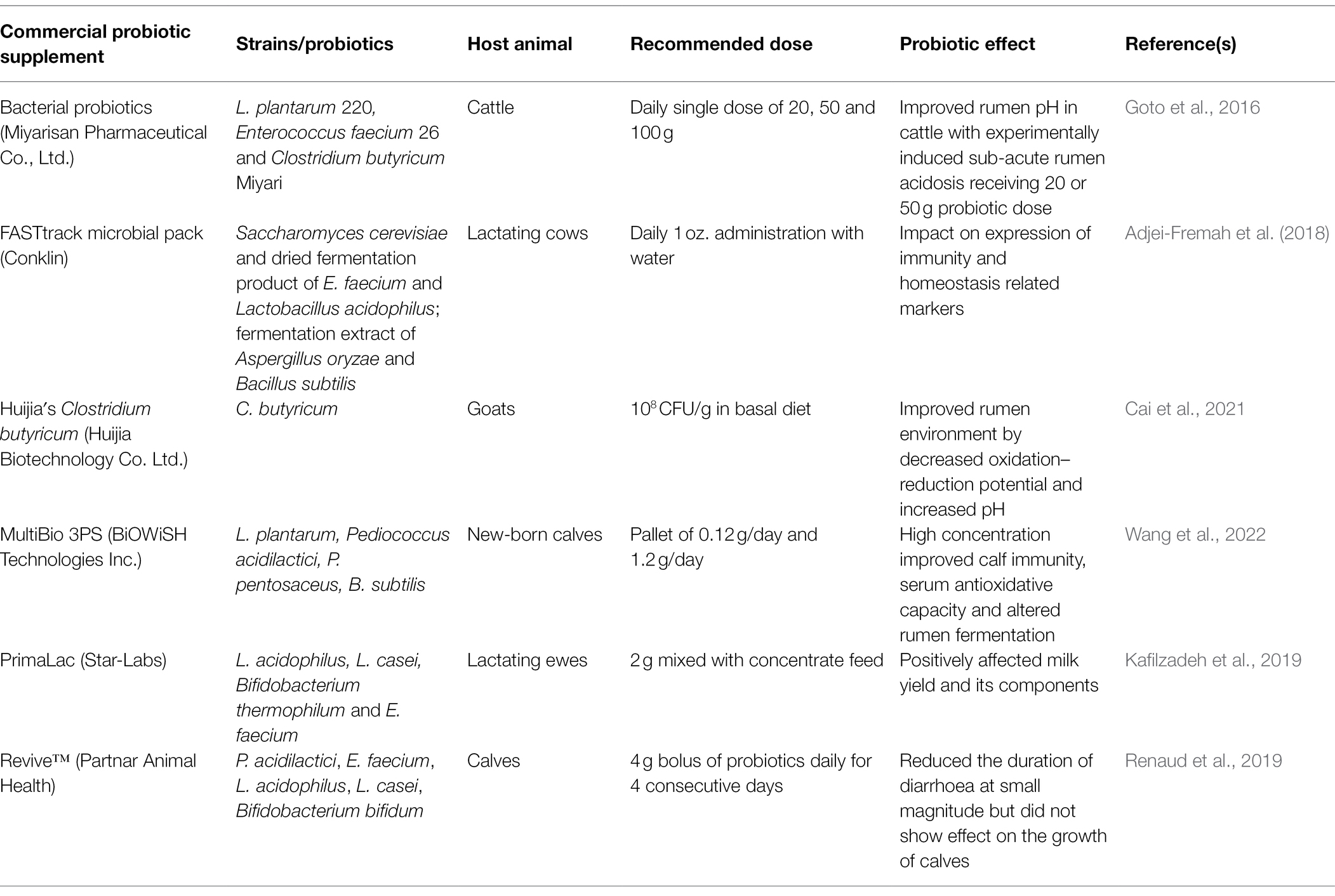
Table 2. Commercial probiotic oral supplements that demonstrated specific health benefits in dairy animals.
Probiotics to Improve the Productivity of Dairy Animals
In previous sections, we have discussed that the benefits of probiotic-based feed supplements on growth improvement are mediated by the change in the rumen microbiome composition that improved digestion and fermentation processes and ultimately increased nutrient bioavailability to the animal. Dairy nutritionists and researchers in this field also attempted to explore whether the similar impact of probiotics is extended to the milk productivity of the lactating animal. Studies have demonstrated that probiotic supplementation also improve the quality and quantity of milk production (Figure 3; Desnoyers et al., 2009; Xu et al., 2017). The role of probiotics in enhancing the milk production in dairy animals could be divided in two different modes.
The first is an indirect mechanism, where probiotics improve milk production by modulating the digestive metabolism, nutrient availability and uptake in the intestine. For example, So et al. (2021) found that L. casei TH14 had a positive effect on milk yield as well as other physiological factors such as digestibility of dry matter and fiber, gross energy, metabolic energy intake, blood glucose, and total VFAs, while suppressing somatic cell count in milk. The similar finding is corroborated in several studies where supplementing dairy animals with probiotics improved milk production without affecting the basic nutritional makeup of the milk (Moallem et al., 2009; Peng et al., 2012).
In addition, researchers have also recorded the qualitative improvement in milk production using probiotic interventions. A study conducted on lactating Holstein cows by Suntara et al. (2021b) presented an interesting fact that administration of animal feed prepared with Crabtree-negative yeast (P. kudriavzevii KKU20 and C. tropicalis KKU20) increased the milk protein content. The reason was connected to the increased beneficial microbial population in the rumen, which enhanced the amount of microbial crude protein. Similar observations on ruminal microbial crude protein mediated increased milk and milk protein yield were reported by Xie et al. (2019). The authors also comprehended the mechanism and explained that microbial crude protein is synthesized by microorganisms of the gut that significantly impact the quantity and quality of metabolizable protein to be absorbed in the intestine. The metabolizable protein subsequently converts to milk protein and influences milk yield. Ma et al. (2020) also noted the effects of Saccharomyces cerevisiae, Bacillus subtilis and Enterococcus faecalis on milk production and milk composition in lactating goats by increasing milk fat, protein and total solid percentage in milk. Also, Sun et al. (2021) assessed the effects of Saccharomyces cerevisiae on milk quality of dairy cows and reported that cows receiving probiotic supplementation produced more milk with higher fat content. Findings also included improved rumen pH, acetic and propionic ratio, indicating the influence of probiotics on increased milk fat content. Because after absorption, VFAs serve as precursors for milk synthesis (Figure 3).
The second mode is probiotic-mediated modulation of the gene expression profile of lactating animal. A direct mechanism of probiotic mediated modulation of gene expression in the dairy animal has been observed by Izuddin et al. (2019). The study was conducted on newly-weaned lambs receiving spent culture supernatant (i.e., postbiotic) of probiotic L. plantarum RG14. In addition to increasing the population of fiber degrading bacteria and decreasing the relative population of methanogens and protozoa in the rumen, postbiotics increased the gene expression of hepatic Insulin-like growth factor-1 and ruminal monocarboxylate transporter-1 (MCT-1). Growth factor-1 is a mediator of growth promoter hormone, whereas MCT-1 is associated with the rumen epithelium membrane transport system. The study does not present the evident correlation between milk productivity and probiotic mediated gene expression modulation. Yet, presumably, the increased expression of MCT-1 in lactating animals could induce higher uptake of VFA from rumen epithelium, resulting in improved milk production. Further identification of the active cellular components of probiotics that impact the gene expression profile related to milk production could open novel ways concerning the dairy industry.
Probiotics implement a beneficial impact on dairy animals’ growth and milk production efficiency. The underlying mechanism is chiefly connected with the management of the rumen and intestinal microbial community. The probiotic mediated microbial homeostasis favors the positive nutritional balance and strengthens the general health of the lactating animal. These beneficial microorganisms propose a natural, consequence free approach to boost the dairy production.
Outlook
Besides general health advantages, probiotics could also serve as a potential biological source to improve the productivity of dairy animals. The natural mechanisms of probiotics include reducing disease burden, modulation of rumen metabolism and modulation of host gene expression. Collectively, it can be stated that the addition of probiotics in feed may enhance the yield of milk and improve the compositional quality of milk in lactating animals. However, the effects of the probiotics are influenced heavily by the strain variation, diet and host physiological state. Hence, developing new intervention strategies using probiotics or active molecular components from probiotics will be worth exploring.
Author Contributions
KN, NKM, and NR did literature search and prepared the draft. HD participated in preparation of manuscript. SK, MH, and AKP reviewed and edited the manuscript. All the authors contributed to the article and approved the submission.
Conflict of Interest
The authors declare that the research was conducted in the absence of any commercial or financial relationships that could be construed as a potential conflict of interest.
Publisher’s Note
All claims expressed in this article are solely those of the authors and do not necessarily represent those of their affiliated organizations, or those of the publisher, the editors and the reviewers. Any product that may be evaluated in this article, or claim that may be made by its manufacturer, is not guaranteed or endorsed by the publisher.
References
Abd El-Tawab, M. M., Youssef, I. M., Bakr, H. A., Fthenakis, G. C., and Giadinis, N. D. (2016). Role of probiotics in nutrition and health of small ruminants. Pol. J. Vet. Sci. 19, 893–906. doi: 10.1515/pjvs-2016-0114
Abdelrahman, W., Mohnl, M., Teichmann, K., Doupovec, B., Schatzmayr, G., Lumpkins, B., et al. (2014). Comparative evaluation of probiotic and salinomycin effects on performance and coccidiosis control in broiler chickens. Poult. Sci. 93, 3002–3008. doi: 10.3382/ps.2014-04212
Adeniyi, B. A., Adetoye, A., and Ayeni, F. A. (2015). Antibacterial activities of lactic acid bacteria isolated from cow faeces against potential enteric pathogens. Afr. Health Sci. 15, 888–895. doi: 10.4314/ahs.v15i3.24
Adjei-Fremah, S., Ekwemalor, K., Asiamah, E. K., Ismail, H., Ibrahim, S., and Worku, M. (2018). Effect of probiotic supplementation on growth and global gene expression in dairy cows. J. Appl. Anim. Res. 46, 257–263. doi: 10.1080/09712119.2017.1292913
Al Kassaa, I., Hober, D., Hamze, M., Chihib, N. E., and Drider, D. (2014). Antiviral potential of lactic acid bacteria and their Bacteriocins. Probiotics Antimicrob. Pr. 6, 177–185. doi: 10.1007/s12602-014-9162-6
Aldana, C., Cabra, S., Ospina, C. A., Carvajal, F., and Rodríguez, F. (2009). Effect of a probiotic compound in rumen development, diarrhea incidence and weight gain in young Holstein calves. World Acad. Sci. Eng. Technol. 57, 378–382. doi: 10.5281/zenodo.1080562
Angulo, M., Reyes-Becerril, M., Cepeda-Palacios, R., Tovar-Ramírez, D., Esteban, M. Á., and Angulo, C. (2019). Probiotic effects of marine Debaryomyces hansenii CBS 8339 on innate immune and antioxidant parameters in newborn goats. Appl. Microbiol. Biotechnol. 103, 2339–2352. doi: 10.1007/s00253-019-09621-5
Apas, A. L., González, S. N., and Arena, M. E. (2014). Potential of goat probiotic to bind mutagens. Anaerobe 28, 8–12. doi: 10.1016/j.anaerobe.2014.04.004
Aphale, D., Natu, A., Laldas, S., and Kulkarni, A. (2019). Administration of Streptococcus bovis isolated from sheep rumen digesta on rumen function and physiology as evaluated in a rumen simulation technique system. Vet. World. 12, 1362–1371. doi: 10.14202/vetworld.2019.1362-1371
Arena, M. P., Capozzi, V., Russo, P., Drider, D., Spano, G., and Fiocco, D. (2018). Immunobiosis and probiosis: antimicrobial activity of lactic acid bacteria with a focus on their antiviral and antifungal properties. Appl. Microbiol. Biotechnol. 102, 9949–9958. doi: 10.1007/s00253-018-9403-9
Armas, F., Camperio, C., and Marianelli, C. (2017). In vitro assessment of the probiotic potential of Lactococcus lactis LMG 7930 against ruminant mastitis-causing pathogens. PLoS One 12:e0169543. doi: 10.1371/journal.pone.0169543
Ayala, D. I., Chen, J. C., Bugarel, M., Loneragan, G. H., den Bakker, H. C., Kottapalli, K. R., et al. (2018). Molecular detection and quantification of viable probiotic strains in animal feedstuffs using the commercial direct fed microbial lactobacillus animalis NP51 as a model. J.Microbiol. Methods. 149, 36–43. doi: 10.1016/j.mimet.2018.04.012
Ayala-Monter, M. A., Hernández-Sánchez, D., González-Muñoz, S., Pinto-Ruiz, R., Martínez-Aispuro, J. A., Torres-Salado, N., et al. (2019). Growth performance and health of nursing lambs supplemented with inulin and lactobacillus casei. Asian-Australasian J. Animal Sci. 32, 1137–1144. doi: 10.5713/ajas.18.0630
Bhakat, C., Mohammad, A., Mandal, D. K., Mandal, A., Rai, S., Chatterjee, A., et al. (2020). Readily usable strategies to control mastitis for production augmentation in dairy cattle: a review. Vet. WORLD 13:2364. doi: 10.14202/vetworld.2020.2364-2370
Blanchet, F., Rault, L., Peton, V., Le Loir, Y., Blondeau, C., Lenoir, L., et al. (2021). Heat inactivation partially preserved barrier and immunomodulatory effects of lactobacillus gasseri LA806 in an in vitro model of bovine mastitis. Benef. Microbes. 12, 95–106. doi: 10.3920/BM2020.0146
Bonaz, B., Bazin, T., and Pellissier, S. (2018). The Vagus nerve at the Interface of the microbiota-gut-brain Axis. Front. Neurosci. 12:49. doi: 10.3389/fnins.2018.00049
Breit, S., Kupferberg, A., Rogler, G., and Hasler, G. (2018). Vagus nerve as modulator of the brain-gut Axis in psychiatric and inflammatory disorders. Front. Psych. 9:44. doi: 10.3389/fpsyt.2018.00044
Breves, G., Walter, C., Burmester, M., and Schröder, B. (2000). In vitro studies on the effects of saccharomyces boulardii and Bacillus cereus var. toyoi on nutrient transport in pig jejunum. J. Anim. Physiol. Anim. Nutr. 84, 9–20. doi: 10.1046/j.1439-0396.2000.00277.x
Britt, J. H., Cushman, R. A., Dechow, C. D., Dobson, H., Humblot, P., Hutjens, M. F., et al. (2018). Invited review: learning from the future:a vision for dairy farms and cows in 2067. J. Dairy Sci. 101, 3722–3741. doi: 10.3168/jds.2017-14025
Bron, P. A., Kleerebezem, M., Brummer, R. J., Cani, P. D., Mercenier, A., MacDonald, T. T., et al. (2017). Can probiotics modulate human disease by impacting intestinal barrier function? Br. J. Nutr. 117, 93–107. doi: 10.1017/S0007114516004037
Bunesova, V., Domig, K. J., Killer, J., Vlková, E., Kopečný, J., Mrázek, J., et al. (2012). Characterization of bifidobacteria suitable for probiotic use in calves. Anaerobe 18, 166–168. doi: 10.1016/j.anaerobe.2011.09.008
Butel, M. J. (2014). Probiotics, gut microbiota and health. In. Med. Mal. Infect. 44, 1–8. doi: 10.1016/j.medmal.2013.10.002
Cai, L., Hartanto, R., Zhang, J., and Qi, D. (2021). Clostridium butyricum improves rumen fermentation and growth performance of heat-stressed goats in vitro and in vivo. Animals 11:3261. doi: 10.3390/ani11113261
Carey, M. A., Medlock, G. L., Alam, M., Kabir, M., Uddin, M. J., Nayak, U., et al. (2021). Megasphaera in the stool microbiota is negatively associated with diarrheal cryptosporidiosis. Clin. Infect. Dis. 73, e1242–e1251. doi: 10.1093/cid/ciab207
Carvalho, M. R., Peñagaricano, F., Santos, J. E. P., DeVries, T. J., McBride, B. W., and Ribeiro, E. S. (2019). Long-term effects of postpartum clinical disease on milk production, reproduction, and culling of dairy cows. J. Dairy Sci. 102, 11701–11717. doi: 10.3168/jds.2019-17025
Chang, M., Ma, F., Wei, J., Liu, J., Nan, X., and Sun, P. (2021). Live Bacillus subtilis natto promotes rumen fermentation by modulating rumen microbiota in vitro. Animals 11:1519. doi: 10.3390/ani11061519
Chaucheyras-Durand, F., and Durand, H. (2010). Probiotics in animal nutrition and health. Benef. Microbes 1, 3–9. doi: 10.3920/BM2008.1002
Chaucheyras-Durand, F., Walker, N. D., and Bach, A. (2008). Effects of active dry yeasts on the rumen microbial ecosystem: past, present and future. Anim. Feed Sci. Technol. 145, 5–26. doi: 10.1016/j.anifeedsci.2007.04.019
Chen, H., Guo, B., Yang, M., Luo, J., Hu, Y., Qu, M., et al. (2021). Response of growth performance, blood biochemistry indices, and rumen bacterial diversity in lambs to diets containing supplemental probiotics and Chinese medicine polysaccharides. Front. Vet. Sci. 8:681389. doi: 10.3389/fvets.2021.681389
Cheng, H.-W., Jiang, S., and Hu, J. (2019). “Gut-brain axis: probiotic, Bacillus subtilis prevents aggression via the modification of the central serotonergic system” in Oral Health by Using Probiotic Products (London: IntechOpen).
Chiquette, J., Allison, M. J., and Rasmussen, M. (2012). Use of Prevotella bryantii 25A and a commercial probiotic during subacute acidosis challenge in midlactation dairy cows. Int. J. Dairy Sci. 95, 5985–5995. doi: 10.3168/jds.2012
Choct, M. (2009). Managing gut health through nutrition. British. Poult. Sci. 50, 9–15. doi: 10.1080/00071660802538632
Cortés, A., Rooney, J., Bartley, D. J., Nisbet, A. J., and Cantacessi, C. (2020). Helminths, hosts, and their microbiota: new avenues for managing gastrointestinal helminthiases in ruminants. Expert Rev. Anti-Infect. Ther. 18, 977–985. doi: 10.1080/14787210.2020.1782188
Dalloul, R. A., Lillehoj, H. S., Shellem, T. A., and Doerr, J. A. (2003). Enhanced mucosal immunity against Eimeria acervulina in broilers fed a lactobacillus-based probiotic. Poult. Sci. 82, 62–66. doi: 10.1093/ps/82.1.62
Desbonnet, L., Clarke, G., Traplin, A., O’Sullivan, O., Crispie, F., Moloney, R. D., et al. (2015). Gut microbiota depletion from early adolescence in mice: implications for brain and behaviour. Brain Behav. Immun. 48, 165–173. doi: 10.1016/j.bbi.2015.04.004
Desnoyers, M., Giger-Reverdin, S., Bertin, G., Duvaux-Ponter, C., and Sauvant, D. (2009). Meta-analysis of the influence of Saccharomyces cerevisiae supplementation on ruminal parameters and milk production of ruminants. Int. J. Dairy Sci. 92, 1620–1632. doi: 10.3168/jds.2008-1414
Devyatkin, V., Mishurov, A., and Kolodina, E. (2021). Probiotic effect of Bacillus subtilis B-2998D, B-3057D, and bacillus licheniformis B-2999D complex on sheep and lambs. J. Adv. Vet. Anim. Res. 8, 146–157. doi: 10.5455/javar.2021.h497
Didarkhah, M., and Bashtani, M. (2018). Effects of probiotic and peribiotic supplementation in milk on performance and nutrition digestibility in Holstein calves. Res. Ani. Prod. 9, 70–78. doi: 10.29252/rap.9.20.70
Drider, D., Bendali, F., Naghmouchi, K., and Chikindas, M. L. (2016). Bacteriocins: not only antibacterial agents. Probiotics Antimicrob. Pr. 8, 177–182. doi: 10.1007/s12602-016-9223-0
Du, R., Jiao, S., Dai, Y., An, J., Lv, J., Yan, X., et al. (2018). Probiotic bacillus amyloliquefaciens C-1 improves growth performance, stimulates GH/IGF-1, and regulates the gut microbiota of growth-retarded beef calves. Front. Microbiol. 9:2006. doi: 10.3389/fmicb.2018.02006
Ellis, J. L., Bannink, A., Hindrichsen, I. K., Kinley, R. D., Pellikaan, W. F., Milora, N., et al. (2016). The effect of lactic acid bacteria included as a probiotic or silage inoculant on in vitro rumen digestibility, total gas and methane production. Anim. Feed Sci. Technol. 211, 61–74. doi: 10.1016/j.anifeedsci.2015.10.016
Enemark, J. M. D. (2008). The monitoring, prevention and treatment of sub-acute ruminal acidosis (SARA): a review. Vet. J. 176, 32–43. doi: 10.1016/j.tvjl.2007.12.021
FAO. (2021). Dairy market review. Overview of global dairy market developments in 2020. Available at: https://www.fao.org/3/cb4230en/cb4230en.pdf (Accessed February 07, 2022).
Fernandes, T., Carvalho, B. F., Mantovani, H. C., Schwan, R. F., and Ávila, C. L. S. (2019). Identification and characterization of yeasts from bovine rumen for potential use as probiotics. J. Appl. Microbiol. 127, 845–855. doi: 10.1111/jam.14350
Fernandez, S., Fraga, M., Silveyra, E., Trombert, A. N., Rabaza, A., Pla, M., et al. (2018). Probiotic properties of native Lactobacillus spp. strains for dairy calves. Ben. Microbes. 9, 613–624. doi: 10.3920/BM2017.0131
Fernández-Ciganda, S., Fraga, M., and Zunino, P. (2021). Probiotic lactobacilli administration induces changes in the fecal microbiota of preweaned dairy calves. Probiotics Antimicrob. Pr., 1–12. doi: 10.1007/s12602-021-09834-z [Epub ahead of print]
Fukuda, S., Suzuki, Y., Murai, M., Asanuma, N., and Hino, T. (2006). Isolation of a novel strain of Butyrivibrio fibrisolvens that isomerizes linoleic acid to conjugated linoleic acid without hydrogenation, and its utilization as a probiotic for animals. J. Appl. Microbiol. 100, 787–794. doi: 10.1111/j.1365-2672.2006.02864.x
Fülling, C., Dinan, T. G., and Cryan, J. F. (2019). Gut microbe to brain signaling: what happens in vagus…. Neuron 101, 998–1002. doi: 10.1016/j.neuron.2019.02.008
Gao, J., Liu, Y. C., Wang, Y., Li, H., Wang, X. M., Wu, Y., et al. (2020). Impact of yeast and lactic acid bacteria on mastitis and milk microbiota composition of dairy cows. AMB Express 10, 22–12. doi: 10.1186/s13568-020-0953-8
Giannenas, I., Papadopoulos, E., Tsalie, E., Triantafillou, E., Henikl, S., Teichmann, K., et al. (2012). Assessment of dietary supplementation with probiotics on performance, intestinal morphology and microflora of chickens infected with Eimeria tenella. Vet. Parasitol. 188, 31–40. doi: 10.1016/j.vetpar.2012.02.017
Goto, H., Qadis, A. Q., Kim, Y. H., Ikuta, K., Ichijo, T., and Sato, S. (2016). Effects of a bacterial probiotic on ruminal pH and volatile fatty acids during subacute ruminal acidosis (SARA) in cattle. J. Vet. Med. Sci. 78, 1595–1600. doi: 10.1292/jvms.16-0211
Guo, Y., Li, Z., Deng, M., Li, Y., Liu, G., Liu, D., et al. (2022). Effects of a multi-strain probiotic on growth, health, and fecal bacterial flora of neonatal dairy calves. Animal Biosci. 35, 204–216. doi: 10.5713/ab.21.0084
Hall, J. O. (2018). “Nitrate-and nitrite-accumulating plants,” in Veterinary Toxicology. (Academic Press), 941–946.
Hill, C., Guarner, F., Reid, G., Gibson, G. R., Merenstein, D. J., Pot, B., et al. (2014). Expert consensus document. The international scientific Association for Probiotics and Prebiotics consensus statement on the scope and appropriate use of the term probiotic. Nat. Rev. Gastroenterol. Hepatol. 11, 506–514. doi: 10.1038/nrgastro.2014.66
Izuddin, W. I., Loh, T. C., Samsudin, A. A., Foo, H. L., Humam, A. M., and Shazali, N. (2019). Effects of postbiotic supplementation on growth performance, ruminal fermentation and microbial profile, blood metabolite and GHR, IGF-1 and MCT-1 gene expression in post-weaning lambs. BMC Vet. Res. 15, 315–310. doi: 10.1186/s12917-019-2064-9
Jinturkar, A. S., Gujar, B. V., Chauhan, D. S., and Patil, R. A. (2009). Effect of feeding probiotics on the growth performance and feed conversion efficiency in goat. Indian J. Anim. Res. 43, 49–52.
Kafilzadeh, F., Payandeh, S., Gómez-Cortés, P., Ghadimi, D., Schiavone, A., and Martínez Marín, A. L. (2019). Effects of probiotic supplementation on milk production, blood metabolite profile and enzyme activities of ewes during lactation. Ital. J. Anim. Sci. 18, 134–139. doi: 10.1080/1828051X.2018.1496040
Kelsey, A. J., and Colpoys, J. D. (2018). Effects of dietary probiotics on beef cattle performance and stress. J. Vet. Behav. 27, 8–14. doi: 10.1016/j.jveb.2018.05.010
Kondrashova, K., Duskaev, G., and Kvan, O. (2020). Evaluation of effects of rumen fluid in combination with probiotic preparations and vanillin on the luminescence of a recombinant strain E. coli. E3S web Conf. EDP Sciences. 143:02034. doi: 10.1051/e3sconf/202014302034
Ladha, G., and Jeevaratnam, K. (2018). Probiotic potential of Pediococcus pentosaceus LJR1, a bacteriocinogenic strain isolated from rumen liquor of goat (Capra aegagrus hircus). Food Biotechnol. 32, 60–77. doi: 10.1080/08905436.2017.1414700
Latham, E. A., Pinchak, W. E., Trachsel, J., Allen, H. K., Callaway, T. R., Nisbet, D. J., et al. (2018). Isolation, characterization and strain selection of a Paenibacillus species for use as a probiotic to aid in ruminal methane mitigation, nitrate/nitrite detoxification and food safety. Bioresour. Technol. 263, 358–364. doi: 10.1016/j.biortech.2018.04.116
Latham, E. A., Pinchak, W. E., Trachsel, J., Allen, H. K., Callaway, T. R., Nisbet, D. J., et al. (2019). Paenibacillus 79R4, a potential rumen probiotic to enhance nitrite detoxification and methane mitigation in nitrate-treated ruminants. Sci. Total Environ. 671, 324–328. doi: 10.1016/j.scitotenv.2019.03.390
Lee, S. H., Lillehoj, H. S., Dalloul, R. A., Park, D. W., Hong, Y. H., and Lin, J. J. (2007). Influence of Pediococcus-based probiotic on coccidiosis in broiler chickens. Poult. Sci. 86, 63–66. doi: 10.1093/ps/86.1.63
Lin, W. C., Ptak, C. P., Chang, C. Y., Ian, M. K., Chia, M. Y., Chen, T. H., et al. (2020). Autochthonous lactic acid bacteria isolated From dairy cow feces exhibiting promising probiotic properties and in vitro antibacterial activity against foodborne pathogens in cattle. Front. Vet. Sci. 7:239. doi: 10.3389/fvets.2020.00239
Lucey, P. M., Lean, I. J., Aly, S. S., Golder, H. M., Block, E., Thompson, J. S., et al. (2021). Effects of mannan-oligosaccharide and Bacillus subtilis supplementation to preweaning Holstein dairy heifers on body weight gain, diarrhea, and shedding of fecal pathogens. Int. J. Dairy Sci. 104, 4290–4302. doi: 10.3168/jds.2020-19425
Ma, Z. Z., Cheng, Y. Y., Wang, S. Q., Ge, J. Z., Shi, H. P., and Kou, J. C. (2020). Positive effects of dietary supplementation of three probiotics on milk yield, milk composition and intestinal flora in Sannan dairy goats varied in kind of probiotics. J. Anim. Physiol. Anim. Nutr. 104, 44–55. doi: 10.1111/jpn.13226
Maake, T. W., Adeleke, M., and Aiyegoro, O. A. (2021). Effect of lactic acid bacteria administered as feed supplement on the weight gain and ruminal pH in two south African goat breeds. Trans. R. Soc. South Africa 76, 35–40. doi: 10.1080/0035919X.2020.1870018
Maamouri, O., and Ben Salem, M. (2021). The effect of live yeast Saccharomyces cerevisiae as probiotic supply on growth performance, feed intake, ruminal pH and fermentation in fattening calves. Vet. Med. Sci. 8, 398–404. doi: 10.1002/vms3.631
Maldonado, N. C., de Ruiz, C. S., Otero, M. C., Sesma, F., and Nader-Macías, M. E. (2012). Lactic acid bacteria isolated from young calves--characterization and potential as probiotics. Res.Vet. Sci. 92, 342–349. doi: 10.1016/j.rvsc.2011.03.017
Mani, S., Aiyegoro, O. A., and Adeleke, M. A. (2021). Characterization of rumen microbiota of two sheep breeds supplemented with direct-fed lactic acid bacteria. Front. Vet. Sci. 7:1199. doi: 10.3389/fvets.2020.570074
Marcinakova, M., Simonová, M., and Lauková, A. (2004). Probiotic properties of enterococcus faecium EF9296 strain isolated from silage. Acta Vet. Brno 73, 513–519. doi: 10.2754/avb200473040513
Marden, J. P., Julien, C., Monteils, V., Auclair, E., Moncoulon, R., and Bayourthe, C. (2008). How does live yeast differ from sodium bicarbonate to stabilize ruminal pH in high-yielding dairy cows? Int. J. Dairy Sci. 91, 3528–3535. doi: 10.3168/jds.2007-0889
Markowiak, P., and Ślizewska, K. (2018). The role of probiotics, prebiotics and synbiotics in animal nutrition. Gut Pathogens. 10:1. doi: 10.1186/s13099-018-0250-0
Meale, S. J., Chaucheyras-Durand, F., Berends, H., and Steele, M. A. (2017). From pre-to postweaning: transformation of the young calf's gastrointestinal tract. Int. J. Dairy Sci. 100, 5984–5995. doi: 10.3168/jds.2016-12474
Meale, S. J., Li, S., Azevedo, P., Derakhshani, H., Plaizier, J. C., Khafipour, E., et al. (2016). Development of ruminal and fecal microbiomes are affected by weaning but not weaning strategy in dairy calves. Frontiers Microbiol. 7:582. doi: 10.3389/fmicb.2016.00582
Moallem, U., Lehrer, H., Livshitz, L., Zachut, M., and Yakoby, S. (2009). The effects of live yeast supplementation to dairy cows during the hot season on production, feed efficiency, and digestibility. Int. J. Dairy Sci. 92, 343–351. doi: 10.3168/jds.2007-0839
Moreira, G. M., Meneses, J. A. M., Ribeiro, C. V., de Melo Faria, A., Arantes, H. G., da Luz, M. H., et al. (2019). Performance and feed efficiency of beef cattle fed high energy diet with probiotic consortium technology. Rev. Bras. Saude Prod. Anim. 20, 1–13. doi: 10.1590/S1519-9940200182019
Mostafa, T. H., Elsayed, F. A., Ahmed, M. A., and Elkholany, M. A. (2014). Effect of using some feed additives (tw-probiotics) in dairy cow rations on production and reproductive performance. Egypt. J. Anim. Prod. 51, 1–11. doi: 10.21608/ejap.2014.93661
Nagaraja, T. G., and Titgemeyer, E. C. (2007). Ruminal acidosis in beef cattle: The current microbiological and nutritional outlook. Int. J. Dairy Sci. 90, E17–E38. doi: 10.3168/jds.2006-478
Osuntoki, A., and Korie, I. (2010). Antioxidant activity of whey from milk fermented with lactobacillus species isolated from Nigerian fermented foods. Food Technol. Biotechnol. 48.
Pellegrino, M. S., Frola, I. D., Natanael, B., Gobelli, D., Nader-Macias, M. E. F., and Bogni, C. I. (2019). In vitro characterization of lactic acid bacteria isolated from bovine milk as potential probiotic strains to prevent bovine mastitis. Probiotics Antimicrob. Pr. 11, 74–84. doi: 10.1007/s12602-017-9383-6
Peng, H., Wang, J. Q., Kang, H. Y., Dong, S. H., Sun, P., Bu, D. P., et al. (2012). Effect of feeding Bacillus subtilis natto fermentation product on milk production and composition, blood metabolites and rumen fermentation in early lactation dairy cows. J. Anim. Physiol. Anim. Nutr. 96, 506–512. doi: 10.1111/j.1439-0396.2011.01173.x
Phesatcha, K., Phesatcha, B., Wanapat, M., and Cherdthong, A. (2022). The effect of yeast and roughage concentrate ratio on ruminal pH and protozoal population in Thai native beef cattle. Animals 12:53. doi: 10.3390/ani12010053
Prabhurajeshwar, C., and Chandrakanth, K. (2019). Evaluation of antimicrobial properties and their substances against pathogenic bacteria in-vitro by probiotic lactobacilli strains isolated from commercial yoghurt. Clin. Nutr. Exp. 23, 97–115. doi: 10.1016/j.yclnex.2018.10.001
Puniya, A. K., Salem, A. Z. M., Kumar, S., Dagar, S. S., Griffith, G. W., Puniya, M., et al. (2015). Role of live microbial feed supplements with reference to anaerobic fungi in ruminant productivity: a review. J. Integr. Agric. 14, 550–560. doi: 10.1016/S2095-3119(14)60837-6
Pyar, H., and Peh, K. K. (2014). Characterization and identification of lactobacillus acidophilus using biolog rapid identification system. Int. J. Pharm. Pharm. Sci. 6, 189–193. doi: 10.3168/jds.2016-12474
Rainard, P., and Foucras, G. (2018). A critical appraisal of probiotics for mastitis control. In Front. Vet. Sci. 5:251. doi: 10.3389/fvets.2018.00251
Ramírez, A. L., Herrera, G., Muñoz, M., Vega, L., Cruz-Saavedra, L., García-Corredor, D., et al. (2021). Describing the intestinal microbiota of Holstein Fasciola-positive and-negative cattle from a hyperendemic area of fascioliasis in Central Colombia. PLoS Negl. Trop. Dis. 15:e0009658. doi: 10.1371/journal.pntd.0009658
Reddy, P. V. M., Reddy, K. K., Kumar, M. S., Harikrishna, C., and Raghunandan, T. (2011). Effect of feeding Pediococcus acidilactici and Saccharomyces boulardii as probiotics in lambs. Indian J. Small Rumin. 17, 53–58.
Renaud, D. L., Kelton, D. F., Weese, J. S., Noble, C., and Duffield, T. F. (2019). Evaluation of a multispecies probiotic as a supportive treatment for diarrhea in dairy calves: a randomized clinical trial. J. Dairy Sci. 102, 4498–4505. doi: 10.3168/jds.2018-15793
Retta, K. S. (2016). Role of probiotics in rumen fermentation and animal performance: a review. Int. J. Livest. Prod. 7, 24–32. doi: 10.5897/IJLP2016.0285
Rokana, N., Mallappa, R. H., Batish, V. K., and Grover, S. (2017). Interaction between putative probiotic lactobacillus strains of Indian gut origin and salmonella: impact on intestinal barrier function. LWT Food Sci. Technol. 84, 851–860. doi: 10.1016/j.lwt.2016.08.021
Rokana, N., Singh, R., Mallappa, R. H., Batish, V. K., and Grover, S. (2016). Modulation of intestinal barrier function to ameliorate salmonella infection in mice by oral administration of fermented milks produced with lactobacillus plantarum MTCC 5690 - a probiotic strain of Indian gut origin. J. Med. Microbiol. 65, 1482–1493. doi: 10.1099/jmm.0.000366
Royan, M., Seighalani, R., Mortezaei, F., and Pourebrahim, M. (2021). In vitro assessment of safety and functional probiotic properties of Lactobacillus mucosae strains isolated from Iranian native ruminants intestine. Ital. J. Anim. Sci. 20, 1187–1200. doi: 10.1080/1828051X.2021.1947908
Santos, F. D. S., Maubrigades, L. R., Gonçalves, V. S., Ferreira, M. R. A., Brasil, C. L., Cunha, R. C., et al. (2021). Immunomodulatory effect of short-term supplementation with Bacillus toyonensis BCT-7112T and saccharomyces boulardii CNCM I-745 in sheep vaccinated with clostridium chauvoei. Vet. Immunol. Immunopathol. 237:110272. doi: 10.1016/j.vetimm.2021.110272
Sasazaki, N., Obi, T., Aridome, C., Fujimoto, Y., Furumoto, M., Toda, K., et al. (2020). Effects of dietary feed supplementation of heat-treated Lactobacillus sakei HS-1 on the health status, blood parameters, and fecal microbes of Japanese black calves. J. Vet. Med. Sci. 82, 1428–1435. doi: 10.1292/jvms.20-0181
Schofield, B. J., Lachner, N., Le, O. T., McNeill, D. M., Dart, P., Ouwerkerk, D., et al. (2018). Beneficial changes in rumen bacterial community profile in sheep and dairy calves as a result of feeding the probiotic Bacillus amyloliquefaciens H57. J. Appl. Microbiol. 124, 855–866. doi: 10.1111/jam.13688
Scott, L. V., Clarke, G., and Dinan, T. G. (2013). The brain-gut axis: a target for treating stress-related disorders. Inflamm. Psychiatry. 28, 90–99. doi: 10.1159/000343971
Shakira, G., Qubtia, M., Ahmed, I., Hasan, F., Anjum, M. I., and Imran, M. (2018). Effect of indigenously isolated Saccharomyces cerevisiae probiotics on milk production, nutrient digestibility, blood chemistry and fecal microbiota in lactating dairy cows. J. Anim. Plant. Sci. 28, 407–420.
Sharma, A. N., Kumar, S., and Tyagi, A. K. (2018b). Effects of mannan-oligosaccharides and Lactobacillus acidophilus supplementation on growth performance, nutrient utilization and faecal characteristics in Murrah buffalo calves. J. Anim. Physiol. Anim. Nutr. 102, 679–689. doi: 10.1111/jpn.12878
Sharma, C., Rokana, N., Chandra, M., Singh, B. P., Gulhane, R. D., Gill, J. P. S., et al. (2018a). Antimicrobial resistance: its surveillance, impact, and alternative management strategies in dairy animals. Front. Vet. Sci. 4:237. doi: 10.3389/fvets.2017.00237
Shreedhar, J. N., Patil, M., and Kumar, P. (2016). Effect of probiotics supplementation on milk yield and its composition in lactating Holstein Fresien and Deoni cross bred cows. J. Med. Bioeng. 5, 19–23. doi: 10.12720/jomb.5.1.19-23
Signorini, M. L., Soto, L. P., Zbrun, M. V., Sequeira, G. J., Rosmini, M. R., and Frizzo, L. S. (2012). Impact of probiotic administration on the health and fecal microbiota of young calves: a meta-analysis of randomized controlled trials of lactic acid bacteria. Res. Vet. Sci. 93, 250–258. doi: 10.1016/j.rvsc.2011.05.001
So, S., Wanapat, M., and Cherdthong, A. (2021). Effect of sugarcane bagasse as industrial by-products treated with Lactobacillus casei TH14, cellulase and molasses on feed utilization, ruminal ecology and milk production of mid-lactating Holstein Friesian cows. J. Sci. Food Agric. 101, 4481–4489. doi: 10.1002/jsfa.11087
Souza, R. F. S., Rault, L., Seyffert, N., Azevedo, V., Le Loir, Y., and Even, S. (2018). Lactobacillus casei BL23 modulates the innate immune response in Staphylococcus aureus-stimulated bovine mammary epithelial cells. Benef. Microbes. 9, 985–995. doi: 10.3920/BM2018.0010
Stefańska, B., Sroka, J., Katzer, F., Goliński, P., and Nowak, W. (2021). The effect of probiotics, phytobiotics and their combination as feed additives in the diet of dairy calves on performance, rumen fermentation and blood metabolites during the preweaning period. Anim. Feed Sci. Technol. 272:114738. doi: 10.1016/j.anifeedsci.2020.114738
Steinberg, R. S., Silva, L. C. S. e., de Souza, M. R., Reis, R. B., Bicalho, A. F., Nunes, J. P. S., et al. (2021). Prospecting of potentially probiotic lactic acid bacteria from bovine mammary ecosystem: imminent partners from bacteriotherapy against bovine mastitis. Int. Microbiol. 25, 189–206. doi: 10.1007/s10123-021-00209-6
Sucu, E., Moore, C., VanBaale, M. J., Jensen, H., Sanz-Fernandez, M. V., and Baumgard, L. H. (2018). Effects of feeding Aspergillus oryzae fermentation product to transition Holstein cows on performance and health. Can. J. Anim. Sci. 99, 237–243. doi: 10.1139/cjas-2018-0037
Sun, X., Wang, Y., Wang, E., Zhang, S., Wang, Q., Zhang, Y., et al. (2021). Effects of Saccharomyces cerevisiae culture on ruminal fermentation, blood metabolism, and performance of high-yield dairy cows. Animals 11:2401. doi: 10.3390/ani11082401
Suntara, C., Cherdthong, A., Uriyapongson, S., Wanapat, M., and Chanjula, P. (2021b). Novel Crabtree negative yeast from rumen fluids can improve rumen fermentation and milk quality. Sci. Rep. 11, 6236–6213. doi: 10.1038/s41598-021-85643-2
Suntara, C., Cherdthong, A., Wanapat, M., Uriyapongson, S., Leelavatcharamas, V., Sawaengkaew, J., et al. (2021a). Isolation and characterization of yeasts from rumen fluids for potential use as additives in ruminant feeding. Vet. Sci. 8:52. doi: 10.3390/vetsci8030052
Tanbayeva, G., Myrzabekov, Z., Tagayev, O., Ratnikova, I., Gavrilova, N., Barakhov, B., et al. (2016). The results of the application of a probiotic as a therapeutic and prophylactic agent in the early form of mastitis in dairy cows. Biosci. Biotechnol. Res. Asia 13, 1579–1584. doi: 10.13005/bbra/2302
Thornton, P. K. (2010). Livestock production: recent trends, future prospects. Philos. Trans. R. Soc. Lond. B, Biol. Sci. 365, 2853–2867. doi: 10.1098/rstb.2010.0134
Tkalcic, S., Zhao, T., Harmon, B. G., Doyle, M. P., Brown, C. A., and Zhao, P. (2003). Fecal shedding of enterohemorrhagic Escherichia coli in weaned calves following treatment with probiotic Escherichia coli. J. Food Prot. 66, 1184–1189. doi: 10.4315/0362-028x-66.7.1184
Tona, G. O. (2021). “Impact of beef and Milk sourced from cattle production on global food security” in Bovine Science—Challenges and Advances (London: IntechOpen).
Travers, M. A., Florent, I., Kohl, L., and Grellier, P. (2011). Probiotics for the control of parasites: an overview. J. Parasitol. Res. 2011, 1–11. doi: 10.1155/2011/610769
Tripathi, M. K., and Karim, S. A. (2010). Effect of individual and mixed live yeast culture feeding on growth performance, nutrient utilization and microbial crude protein synthesis in lambs. Anim. Feed Sci. Technol. 155, 163–171. doi: 10.1016/j.anifeedsci.2009.11.007
Uyeno, Y., Shigemori, S., and Shimosato, T. (2015). Effect of probiotics/prebiotics on cattle health and productivity. Microbes Environ. 30, 126–132. doi: 10.1264/jsme2.ME14176
Varada, V. V., Tyagi, A. K., Banakar, P. S., Das, A., Tyagi, N., Mallapa, R. H., et al. (2022). Autochthonous Limosilactobacillus reuteri BFE7 and Ligilactobacillus salivarius BF17 probiotics consortium supplementation improves performance, immunity, and selected gut health indices in Murrah buffalo calves. Vet. Res. Commun. doi: 10.1007/s11259-022-09896-6 [Epub ahead of print]
Vasconcelos, J. T., Elam, N. A., Brashears, M. M., and Galyean, M. L. (2008). Effects of increasing dose of live cultures of Lactobacillus acidophilus (strain NP 51) combined with a single dose of Propionibacterium freudenreichii (strain NP 24) on performance and carcass characteristics of finishing beef steers. J. Anim. Sci. 86, 756–762. doi: 10.2527/jas.2007-0526
Villena, J., Aso, H., Rutten, V., Takahashi, H., van Eden, W., and Kitazawa, H. (2018). Immunobiotics for the bovine host: their interaction with intestinal epithelial cells and their effect on antiviral immunity. Fronti. Immunol. 9:326. doi: 10.3389/fimmu.2018.00326
Vlkova, E., Grmanová, M., Rada, V., Homutová, I., and Dubná, S. (2009). Selection of probiotic bifidobacteria for lambs. Czech J. Anim. Sci. 54, 552–565. doi: 10.17221/151/2009-CJAS
Von Buenau, R., Jaekel, L., Schubotz, E., Schwarz, S., Stroff, T., and Krueger, M. (2005). Escherichia coli strain Nissle 1917: significant reduction of neonatal calf diarrhea. J. Dairy Sci. 88, 317–323. doi: 10.3168/jds.S0022-0302(05)72690-4
Wang, H., Yu, Z., Gao, Z., Li, Q., Qiu, X., Wu, F., et al. (2022). Effects of compound probiotics on growth performance, rumen fermentation, blood parameters, and health status of neonatal Holstein calves. J. Dairy Sci. 105, 2190–2200. doi: 10.3168/jds.2021-20721
Wu, H. J., and Wu, E. (2012). The role of gut microbiota in immune homeostasis and autoimmunity. Gut Microbes 3, 4–14. doi: 10.4161/gmic.19320
Xie, Y., Wu, Z., Wang, D., and Liu, J. (2019). Nitrogen partitioning and microbial protein synthesis in lactating dairy cows with different phenotypic residual feed intake. J. Anim. Sci. Biotechnol. 10, 1–8. doi: 10.1186/s40104-019-0356-3
Xu, H., Huang, W., Hou, Q., Kwok, L. y., Sun, Z., Ma, H., et al. (2017). The effects of probiotics administration on the milk production, milk components and fecal bacteria microbiota of dairy cows. Sci. Bull. 62, 767–774. doi: 10.1016/j.scib.2017.04.019
Zhang, N., Liao, X., Zhang, Y., Li, M., Wang, W., and Zhai, S. (2019). Probiotic supplements for relieving stress in healthy participants: a protocol for systematic review and meta-analysis of randomized controlled trials. Medicine (United States) 98:e15416. doi: 10.1097/MD.0000000000015416
Zhang, N., Wang, L., and Wei, Y. (2020). Effects of bacillus amyloliquefaciens and Bacillus pumilus on rumen and intestine morphology and microbiota in weanling Jintang black goat. Animals 10:1604. doi: 10.3390/ani10091604
Zhang, R., Zhou, M., Tu, Y., Zhang, N. F., Deng, K. D., Ma, T., et al. (2016). Effect of oral administration of probiotics on growth performance, apparent nutrient digestibility and stress-related indicators in Holstein calves. J. Anim. Physiol. Anim. Nutr. 100, 33–38. doi: 10.1111/jpn.12338
Keywords: probiotics, feed supplements, biotherapeutics, dairy production, gut microbiome
Citation: Nalla K, Manda NK, Dhillon HS, Kanade SR, Rokana N, Hess M and Puniya AK (2022) Impact of Probiotics on Dairy Production Efficiency. Front. Microbiol. 13:805963. doi: 10.3389/fmicb.2022.805963
Edited by:
George Tsiamis, University of Patras, GreeceReviewed by:
Martha Olivera, University of Antioquia, ColombiaMohamed Zommiti, Université de Rouen, France
Copyright © 2022 Nalla, Manda, Dhillon, Kanade, Rokana, Hess and Puniya. This is an open-access article distributed under the terms of the Creative Commons Attribution License (CC BY). The use, distribution or reproduction in other forums is permitted, provided the original author(s) and the copyright owner(s) are credited and that the original publication in this journal is cited, in accordance with accepted academic practice. No use, distribution or reproduction is permitted which does not comply with these terms.
*Correspondence: Namita Rokana, drnamita.rokana@gmail.com; namitarokana@gadvasu.in; Anil Kumar Puniya, akpuniya@gmail.com; anil.puniya@icar.gov.in
†ORCID: Matthias Hess, orcid.org/0000-0003-0321-0380
‡These authors have contributed equally to this work