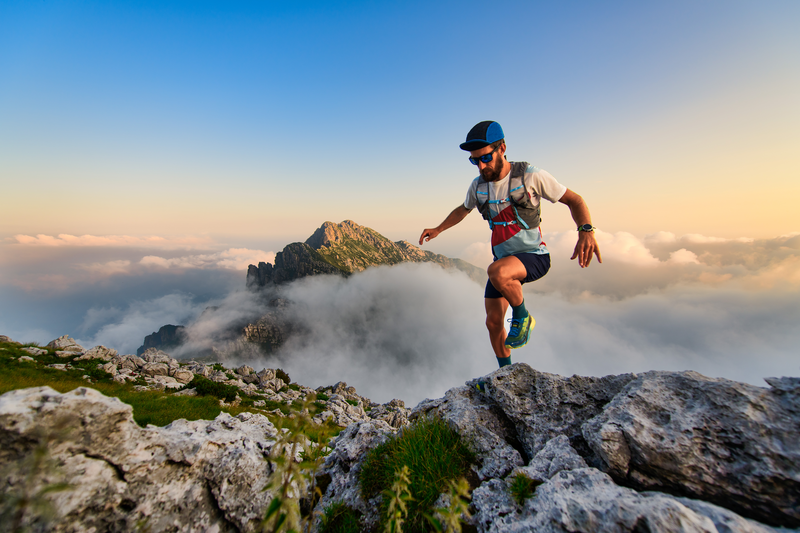
95% of researchers rate our articles as excellent or good
Learn more about the work of our research integrity team to safeguard the quality of each article we publish.
Find out more
ORIGINAL RESEARCH article
Front. Microbiol. , 25 April 2022
Sec. Food Microbiology
Volume 13 - 2022 | https://doi.org/10.3389/fmicb.2022.803688
This article is part of the Research Topic Probiotic bacteria-derived effector molecules and their impact on the host in health and disease View all 16 articles
The metabolites of lactic acid bacteria (LAB) and bifidobacteria (Bb) have recently received a lot of attention due to their ability to protect interactions in blood and tissues, as well as their biodegradability and biocompatibility in human tissue. Exopolysaccharides (EPS) derived from bacteria have a long history of use in therapeutic and other industrial applications with no adverse effects. In this regard, EPSs were isolated and characterized from LAB and Bb culture supernatants to determine their antioxidant, antitumor, and periodontal regeneration properties. The antioxidant capacity of the EPSs varied with concentration (0.625–20 mg/ml). The highest antioxidant activity was found in LAB: Streptococcus thermophiles DSM 24731-EPS1, Lactobacillus delbrueckii ssp. bulgaricus DSM 20081T-EPS5, Limosilactobacillus fermentum DSM 20049-EPS6, and Bb; Bifidobacterium longum ssp. longum DSM 200707-EPS10. Human breast cancer cells (MCF7), human colon cancer cells (CaCo2), human liver cancer cells (HepG2), and human embryonic kidney 293 (HEK 293) cells were used as controls to assess the antitumor properties of the selected EPSs. According to the 3-(4,5-dimethylthiazol-2-yl)-2,5-diphenyltetrazolium-bromide (MTT) assay, EPS5 had the highest cytotoxicity against MCF7, CaCo2, and HepG2, with IC50 values of 7.91, 10.69, and 9.12 mg/ml, respectively. Lactate dehydrogenase (LDH) activity was significantly higher in cell lines treated with EPS5-IC50 values compared to other EPSs-IC50 values (p < 0.05). Real time (RT)-PCR results showed that EPS5 treatment increased Bax, Caspase 8, Caspase 3, and p53 gene expression. The expression of the BCL2, MCL1, and Vimentin genes, on the other hand, was reduced. The MTT test was used to examine the effect of EPS5 on the viability of human periodontal ligament fibroblast cells (hPDLFCs), and it was discovered that EPS5 increased hPDLFC viability. According to high-performance liquid chromatography (HPLC) analysis, galactose made up 12.5% of EPS5. The findings of this study pave the way for the use of EPS, which hold great promise for a variety of therapeutic purposes such as antioxidant, antitumor, and periodontal regeneration.
Graphical Abstract. Therapeutic applications of exopolysaccharides derived from lactic acid bacteria and bifidobacteria.
Bacteria are recognized for their ability to produce a wide variety of polysaccharides. These polysaccharides may either be tightly attached to the cell surface as capsular polysaccharides (CPS) or they can be released as exopolysaccharides (EPSs). Many bacterial taxa, particularly lactic acid bacteria (LAB) and bifidobacteria (Bb), produce a wide range of carbohydrate polymers during fermentation (Sanalibaba and Cakmak, 2016). LAB are generally considered as safe microorganisms (GRAS—generally recognized as safe) and also capable of creating EPSs with many different structures without any health risks (Surayot et al., 2014). LAB refers to Gram-positive bacteria that are often isolated from fermented natural products and are extensively employed in industrial operations. LAB and their metabolic products have been shown to enhance immunity, gastro-intestinal function, resistance to obesity, antioxidant activity, and blood glucose and cholesterol levels (Mathur et al., 2020; Wang et al., 2020). Additionally, they also have potential health benefits such as anticancer activity (Tukenmez et al., 2019), immunostimulatory activity (Adebayo-Tayo et al., 2018), antibiofilm activity (Lakra et al., 2020), and antiviral activity (Biliavska et al., 2019).
Exopolysaccharides have garnered significant interest in recent years owing to their potential pharmacological and biomedical uses (Barcelos et al., 2019). EPSs are classified into two categories: homopolysaccharides (HoPs) are composed entirely of single monosaccharides, heteropolysaccharides (HePs) are composed of a polymer chain of monomer units composed of two or more monosaccharides (Mende et al., 2016). High-molecular-mass polysaccharides exhibit a variety of chemical properties, including molecular weights, charges, side chains, and rigidity (Sanalibaba and Cakmak, 2016; Wang et al., 2020). Simultaneously, the growth conditions used to produce probiotic bacteria alter the chemical structure of EPS (Zannini et al., 2016). Additionally, experiments have demonstrated that the composition and structure of EPSs are strain-dependent (Li C. et al., 2014). Due to their chemical properties, including molecular structures, chain linkage, and molecular mass, EPS exhibit anticancer activity with minimal side effects. As a result, EPS with a diverse chemical structure and property profile may be advantageous in a variety of therapeutic applications (Wang et al., 2014; Hussain et al., 2017; Lin et al., 2018).
Concerns have grown about the safety and toxicity of synthetic antioxidants, but researchers face a difficult task in identifying natural antioxidants that are safe for human health (Adebayo-Tayo and Fashogbon, 2020). It has recently been demonstrated that LAB EPS have antioxidant properties and could be used as potential antioxidants (El-Adawi et al., 2012; Adebayo-Tayo et al., 2018). Lactobacillus helveticus MB2-1 EPS has significant antioxidant and anticancer activity in colon cancer cells (Li W. et al., 2014; Li et al., 2015). Adebayo-Tayo and Fashogbon (2020) confirmed that EPS activity has pharmacological and nutraceutical applications in wild-type and mutant Lactobacillus delbrueckii. Furthermore, Joo Seo et al. (2015) established that purified Lactiplantibacillus plantarum YML009-EPS had high antioxidant activity. Lactobacillus acidophilus, Lactobacillus gasseri, Lactiplantibacillus plantarum, and Lacticaseibacillus rhamnosus were reported to have anticancer and antioxidant properties (Sungur et al., 2017; Adesulu-Dahunsi et al., 2018). The antioxidant activity of EPS extracted from fermented milk of L. delbrueckii ssp. bulgaricus SRFM-1 has been demonstrated to be acceptable (Tang et al., 2017). Additionally, EPS extracted from L. plantarum ZDY2013 was shown to have increased antioxidant activity following sulfonation (Zhang et al., 2016).
Periodontal diseases are noteworthy as a global “hidden” epidemic in terms of disease burden and socioeconomic consequences. Periodontal disease is caused by bacteria that live in the gingiva and on the tooth and is characterized by inflammation and destruction of the oral supporting tissue. If the biofilm is not removed from the periodontal pocket, it may induce an immune response and inflammation in other periodontal tissues, resulting in progressive periodontitis. Periodontitis left untreated may result in further bone loss and, eventually, tooth loss (Nasajpour et al., 2018). While dental scaling and open-flap surgery are effective at removing biofilms and regulating inflammation in order to prevent disease development, they are ineffective at regenerating periodontal tissue (Iviglia et al., 2019). The identification of naturally occurring biomaterials with multiple functions may hold enormous promise for periodontal repair and regeneration, as well as serve as a springboard for future research.
At the moment, researchers are investigating low-risk cancer medications, and one of their areas of interest is the antitumor properties of LAB (Norouzi et al., 2018). Cancer is a term that refers to the uncontrolled proliferation of abnormal cells that have the ability to invade and damage surrounding tissue. According to the Global Cancer Center, cancer has surpassed heart disease as the leading cause of death (Bray et al., 2018). Surgical excision, radiation, and medication therapy are all considered traditional cancer therapies (Qin, 2015). Drug therapy can have a detrimental effect on normal organs and tissues, including the bone marrow, kidneys, and oral mucosa, as well as impair normal metabolism. Additionally, these three approaches may result in inflammation and subsequent lymphedema following therapy (Hu et al., 2017; Adebayo-Tayo and Fashogbon, 2020). As a result of their affordability, availability, and lack of adverse effects, people prefer complementary and alternative medications (Liu et al., 2019). As a result, there is an increasing need for targeted therapies capable of successfully treating cancer while having a negligible effect on normal tissues or at the very least acting as adjuvants to reduce clinical doses and increase the potency of conventional chemotherapy agents (Liu et al., 2021).
Lactobacilli are anticancer agents because they can induce apoptosis in cancerous cells, differentiate them, and bind to genotoxic substances (Wu et al., 2021). Due to the fact that LAB EPS inhibits the growth of a variety of tumor cells, including those from the stomach, liver, and breast, it may be used as a complementary or alternative cancer treatment. These agents have a variety of antitumor effects, including cell cycle arrest and inducing apoptosis, as well as antimutagenic, antioxidative, antiangiogenesis, and anti-inflammatory properties (Nazir et al., 2018; Milner et al., 2021; Wu et al., 2021). Additionally, EPS has a wide range of effects, including decreasing tumor-promoting enzyme activity, increasing immune response, forming host-supporting metabolites and resistant pathogens, and limiting cell proliferation by inhibiting cancer cell spread via apoptosis (Liu et al., 2021). Apoptosis (programmed cell death) occurs at the molecular level via two distinct mechanisms, intrinsic and extrinsic (Śliżewska et al., 2021). The BCl2 family of pro- and anti-apoptotic proteins regulates intrinsic or mitochondrial pathways. Exogenous apoptosis, alternatively referred to as the cytoplasmic route, is mediated by the death receptor Fas, a member of the tumor necrosis factor family (Zhao et al., 2019). Numerous publications have demonstrated that EPSs possess a variety of biologically active properties, including antioxidant, immune-stimulating, and anticancer properties (El-Adawi et al., 2012; El-Deeb et al., 2018; Angelin and Kavitha, 2020). In this regard, EPSs from the marine bacterium L. plantarum 70810 have shown an anti-proliferative effect on a hepatocellular cancer cell line (HepG2) (Yahya et al., 2019). Rajoka et al. (2018) investigated the bioactivity of the cell-free culture supernatant (CFCS) of Lactobacillus strains Lactobacillus casei SR1, L. casei SR2, and Lactobacillus paracasei SR4 obtained from human breast milk. They revealed that the CFCSs exhibited adequate anticancer effects on cervical cancer (HeLa) cells by upregulating the apoptotic genes BAX, BAD, Caspase 3, Caspase 8, and Caspase 9 and downregulating the BCl2 gene expression.
In terms of EPS’s unique properties and potential effects, we hypothesized that it has a profound effect on periodontal repair and regeneration, as well as on cancer cell growth inhibition and apoptosis induction. To address this issue, we assessed the EPS’s cytotoxicity against breast cancer (MCF7), colon cancer (CaCo2), human liver cancer cells (HepG2), and human embryonic kidney 293 (HEK 293) cells (control), as well as its antioxidant capacity. Additionally, using a variety of cancer cell lines, the current research examined their effect on apoptosis-related gene expression. Additionally, the effect of EPS on the survival of human periodontal ligament fibroblast cells (hPDLFCs) in vitro was investigated.
Numerous research were performed recently to characterize and comprehend the biotechnological application of EPS produced from LAB and Bb. However, the tremendous therapeutic potential of EPS, particularly in periodontal healing and regeneration, is still unknown. Due to the wide range of uses for EPS and the rising demand for biopolymers, it is vital to develop new EPS produced from LAB. To our knowledge, the multifunctional therapeutic applications of EPS produced by L. delbrueckii ssp. bulgaricus DSM 20081T have been emphasized for the first time. Under this scope, this work aims to look into the antioxidant, antitumor, and periodontal regeneration properties of EPSs derived using LAB and Bb culture supernatants. As a result, EPSs derived from L. delbrueckii ssp. bulgaricus DSM 20081T may be a promising candidate for a variety of therapeutic applications.
On Dulbecco’s Modified Eagle Medium (DMEM), non-cancerous HEK 293 cells were grown. CaCo2 and HepG2 cells were grown in DMEM; MCF7 cells were grown in Rosewell Park Memorial Institute (RPMI) medium. All cells were cultured in a medium containing 2 mM L-glutamine, 10% fetal bovine serum (FBS), and 1% penicillin–streptomycin combination. The cultures were incubated at 37°C for 24 h in a humidified environment containing 5% CO2 and 95% air.
Lactic acid bacteria (n = 9) including Streptococcus thermophiles DSM 24731, Lactobacillus lactis ssp. cremoris DSM 20069T, Lacticaseibacillus casei DSM 27537, Lactobacillus delbrueckii ssp. bulgaricus DSM 20080, Lactobacillus delbrueckii ssp. bulgaricus DSM 20081T, Limosilactobacillus fermentum DSM 20049, Lactobacillus acidophilus DSM 20079T, Lactobacillus rhamnosus DSM 20021, and Lactobacillus plantarum ssp. plantarum DSM 20174 were kindly obtained from the culture collection of the Faculty of Agriculture Ain Shams University and the Faculty of Science Tanta University (El-Adawi et al., 2012). LAB strains were grown in De Man–Rogosa–Sharpe (MRS, Biokar Diagnostics, Beauvais, France) broth [10.0 g/l peptone, 8.0 g/l meat extract, 4.0 g/l yeast extract, 20.0 g/l D (+)-glucose, 2.0 g/l dipotassium hydrogen phosphate, 5 g/l sodium acetate trihydrate, 2.0 g/l triammonium citrate, 0.2 g/l magnesium sulfate heptahydrate, 0.05 g/l manganous sulfate tetrahydrate, final pH 6.2] at 37°C for 24 h anaerobically. However, Streptococcus thermophilus, was cultured on M17 agar media and incubated at 37°C under aerobic and anaerobic conditions.
Bifidobacterium longum ssp. longum DSM 200707 was provided from Kafr El-Sheik University. Bifidobacterium was grown in MRS medium supplemented with l-cysteine at 0.5 g/l (MRSc) at 37°C in anaerobic environment.
The experimental setup for extracting EPS from a variety of LAB is depicted in Figure 1. Centrifugation at 4°C, 10,000 rpm, for approximately 20 min was used to remove bacteria from overnight cultures (Bajpai et al., 2016). The supernatant was collected and diluted with 14% trichloroacetic acid (1:1), followed by centrifugation (10,000 rpm at 4°C for 20 min) to remove the proteins. The clear supernatant was collected and concentrated using a rotary evaporator. The EPS was precipitated by adding double the amount of absolute cold ethanol and maintaining it at 4°C for 24 h, followed by 20 min of centrifugation at 4°C, 10,000 rpm. After dilution with deionized water, the residue was dialyzed for 48 h. The solution was concentrated and lyophilized to obtain dry powdered crude EPS (mg/l). The total carbohydrate content (%) of crude EPS was determined using the phenol-sulfuric acid method, using glucose (2 mg/ml) as a standard (Dubois et al., 1956). Approximately 0.5 ml of EPS solution (100 mg/ml) was combined with 0.5 ml of phenol 6% (v/v) in a test tube. A mixed solution of concentrated sulfuric acid (2.5 ml) was added to the test tube. After 10 min, the mixture was kept in a water bath (30°C) for 20 min, and the absorbance (Abs) at 490 nm was determined using a spectrophotometer. By substituting Abs into the standard curve, the carbohydrate content was determined. Simultaneously, the protein content of EPS was determined using Bradford method (Bradford, 1976). Protein reagent (2.5 ml, 50 ml containing 2.0 mg/ml Coomassie Brilliant Blue G-250 in 95% ethanol and 100 ml 85% phosphoric acid) was added to the polysaccharide solution (0.5 ml, 2 mg/ml). Abs at 595 nm was determined using a spectrophotometer following agitation. The protein content was determined by substituting Abs into a standard curve.
Figure 1. Experimental setup for extraction and characterization of exopolysaccharides (EPS) by lactic acid bacteria (LAB) and bifidobacteria (Bb) exploring their therapeutic potentials.
The 1,1-diphenyl-2-pyridyl-hydrazine (DPPH) assay was used to investigate the antioxidant activity of EPSs (Kao and Chen, 2006) and the total antioxidant capacity (TAC) (Dilna et al., 2015), as shown in Figure 1.
The ability of EPS to scavenge DPPH radicals was determined using the procedures described by Kao and Chen (2006). Five millimeters of 0.02 methanolic DPPH radical solution was added to 5 ml of various EPS concentrations (0.625–20 mg/ml). For 30 min, the reaction mixture was kept in a darkroom. The Abs of the mixture was determined spectrophotometrically at 517 nm using a Spectrophotometer UV-visible 2401PC (Shimadzu, Japan). Three times for each measurement, the means and standard deviations (SD) were computed. The following equation was used to determine the capacity to scavenge the DPPH radical:
Ac, Absorbance of control (all reagents except the checked substance is replaced by distilled water).
As, Absorbance of the sample/standard.
Ascorbic acid has traditionally been utilized as a free radical scavenger.
The TAC of the EPS samples was determined as described by Dilna et al. (2015). To make the TAC reagent, mix 7.45 ml sulfuric acid (0.6 M), 1.235 g ammonium molybdate (4 mM), and 0.9942 g sodium sulfate (28 mM) in 250 ml distilled water. After 15 min, 0.1 ml of various EPS concentrations (0.625–20 mg/ml) were dissolved in 1 ml of total antioxidant reagent, and the Abs at 695 nm was measured with a spectrophotometer. Ascorbic acid served as a control substance. Additionally, the most antioxidant-potent candidate EPSs (EPS1, EPS5, EPS6, and EPS10) were chosen for future investigation.
The 3-(4,5-dimethylthiazol-2-yl)-2,5-diphenyltetrazolium-bromide (MTT) test was used to assess cytotoxicity (Sigma Aldrich, St. Louis, MO, United States), as previously described by Manivasagan et al. (2013) and Khalil et al. (2022). The cells (100 μl, MCF7, CaCo2, and HepG2) were seeded at a density of 6 × 104 cells/ml in 96-well microplates and treated with EPS1, EPS5, EPS6, and EPS10 at various concentrations (0.625–20 mg/ml). The optical density of the formazan solution was determined using a BIORAD Micro-Plate ELISA Reader at 490 nm. Additionally, to ensure that the extracted EPSs are safe for normal cells, their inhibitory effects on the normal HEK 293 cell line were determined. Because MTT decreases only in metabolically active cells, activity is a proxy for cell viability. The EPS extracts were tested in triplicate on the five cell lines, and the viability of the cells was estimated using the following equation: Viability % = Absorbance of sample/Absorbance of control × 100. According to El-Deeb et al. (2018) and Khalil et al. (2022), the IC50 values for EPS were calculated using the graphpad prism 9 program and indicate a 50% reduction in growth when compared to control cells (untreated cells).
The annexin V test and the amount of lactate dehydrogenase (LDH) leaking out of the cell were used to determine the antitumor activity of EPSs (Gurunathan et al., 2013), as represented in Figure 1.
According to the manufacturer’s instructions, all cell lines exhibited apoptosis as determined by a human Annexin V platinum ELISA (eBioscience BMS252/BMS252TEN; Thermo Fisher Scientific, Waltham, MA, United States). For 24 h in a humidified incubator at 37°C and 5% CO2, cell lines were grown in 96-well flat-bottom culture plates with the IC50 concentrations of EPS1, EPS5, EPS6, and EPS10. This assay is based on the fact that human Annexin V present in the sample or standard will bind to the Microwell Plate coating antibodies. As a result, a biotin-conjugated anti-human Annexin V antibody was added, which specifically recognizes the human Annexin V captured by the first antibody. Following incubation, all unbound biotin-conjugated anti-human Annexin V antibody was removed during the washing step. Streptavidin horseradish peroxidase (HRP) was used, which reacts with an anti-human Annexin V antibody conjugated to biotin. It is possible to create a colored substance that is proportional to the amount of human Annexin V in the sample or standard. After adding acid to terminate the reaction, the Abs at 450 nm is measured using a microplate reader. For the standard curve preparation, seven human Annexin V standard dilutions and seven human Annexin V sample concentrations were calculated.
The amount of LDH leaking out of EPS-treated cell lines was used to determine the plasma membrane integrity (Gurunathan et al., 2013). The cells were exposed to various EPSs at their IC50 concentrations for 24 h. LDH activity was determined in the culture after 3 h of incubation under standard conditions (K726-500) according to the manufacturer’s instructions (Biovision, Mountain View, CA, United States). The quantification of LDH is based on the color change caused by the reduction of NAD to NADH by 450 nm of LDH using a microplate reader. The activity of LDH was determined according to the following equations; LDH Activity level (nmol/ml) = B/(ΔT × V) × sample dilution; where B is the amount of NADH produced from ΔT (T2 - T1), T1 is the time of first reading (A1) (in min), T2 is the time of second reading (A2) (in min), and V is the volume of pretreated sample added into the reaction well (in ml).
Fourier transform infrared (FT-IR) spectroscopy was used to determine the major structural groups of the selected EPS5. The KBr technique was used to determine the EPS spectrum. Polysaccharide samples were crushed into KBr pellets at a sample ratio of 1:100. The infrared Fourier transform spectra were acquired using a Bruker Tensor 27 instrument with a resolution of 4 cm–1 in the range 4,000–400 cm–1 (Fontana et al., 2015). The monosaccharide content of EPS5 was determined using high-performance liquid chromatography (HPLC) as described by Yang et al. (2012). Briefly, the rehydrated EPS1 and EPS5 samples were derivatized with 1-phenyl-3-methyl-5-pyrazolone and analyzed by HPLC using a four-unit pump (Agilent Technologies, Wilmington, DE, United States) and a Shim-Pak VPODS column (4.6 × 150 mm) with Abs monitoring at 245 nm. An integrator was used to compare different sugar standards to the EPS sample’s retention time.
RNA was isolated from all cancer cell lines using a MagJET RNA purification kit, either untreated (negative control) or 24 h after treatment with EPS5 IC50. The quality and quantity of extracted RNAs were determined using 1% agarose gel electrophoresis and Nanodrop (Thermo Fisher Scientific, Inc., Wilmington, DE, United States). Quantitative real-time (qRT)-PCR was performed according to the manufacturer’s protocol using the Sybr Green Supermix RT-PCR kit, forward and reverse primers for each gene (Supplementary Table 1), and SYBR green PCR master mixture. The genes BCL2, MCL1, Bax, Caspase 3, Caspase 8, Vimentin, and p53 were normalized with the housekeeping gene (GAPDH) and expressed about untreated cells. Following amplification, the obtained data were analyzed using the formula for relative gene expression (Winner et al., 1999; Livak and Schmittgen, 2001).
Human periodontal ligament fibroblast cells (hPDLFCs) were extracted and grown according to Arslan and Kantarcioglu (2019). The Clinical Study Ethics Committee of Taif University accepted the research on an ethical basis. To examine the impact of various EPS5 concentrations on viability, hPDLFCs were plated of 1 × 104 cells/well in 96-well plates, and viability was assessed using the MTT assay.
Minitab statistical software (17.1.0.0, Minitab Inc., Chicago, IL, United States) and GraphPad Prism version were used to interpret and process the data (9.0.0). To assess LDH activity and relative gene expression, the means of the groups were compared using an unpaired t-test. However, ANOVA single factor was used to analyze the synthesis of crude EPS by different probiotic bacterial strains and antioxidant activities of EPS. All measurements are presented as means ± SD. The one-way ANOVA test for variance was used to compare two or more groups, and the Tukey test was used for multiple comparisons. A p-value less than 0.05 was considered significant.
Table 1 depicts the production of EPSs by LAB and Bb, as well as their carbohydrate and protein contents. The amount of EPS produced by various bacterial strains varied significantly (p < 0.05). The EPS derived from bacteria ranged from 174.9 to 295.4 mg/l. L. delbrueckii ssp. bulgaricus DSM 20081T produced the most EPS (EPS5, 295.4 ± 9.7), while L. plantarum DSM 20174 produced the least (EPS9, 174.9 ± 5.2). The carbohydrate and protein content of crude EPS varied with strain (p < 0.05). The total carbohydrate content ranged from 85.0 to 96.7%, while the protein content ranged from 3.3 to 9.3%. The average carbohydrate level for EPS5 is significantly higher (96.7 ± 0.3%) than for the other strains (p < 0.05). Furthermore, in the final lyophilized crude EPS samples, S. thermophiles DSM 24731-EPS1 had the lowest concentration of protein contamination.
As shown in Tables 2, 3, the antioxidant capabilities of crude EPSs from 10 bacterial strains were evaluated in vitro by evaluating the activity of radical scavengers (DPPH and TAC, respectively). The DPPH free radical scavenging potential of various EPS concentrations increased significantly concentration-dependently (p < 0.05) (Table 2). At 20 mg/ml, the capacity of EPS to scavenge DPPH radicals ranged from 33.3% to 77.0%. S. thermophiles DSM 24731-EPS1 had the highest scavenging rate of all strains, at 77%, followed by L. delbrueckii ssp. bulgaricus DSM 20081T-EPS5, B. longum DSM 200707-EPS10, and L. fermentum DSM 20049-EPS6. L. acidophilus DSMZ 20079T-EPS7 had the least amount of scavenging activity (Table 2). The ability of a non-enzymatic antioxidant defense mechanism is represented by TAC. Table 3 shows that the antioxidant capacity ranged between 0.75 and 2.5%; the highest rate of inhibition was 2.5% for L. delbrueckii ssp. bulgaricus DSM 20081T-EPS5, followed by S. thermophiles DSM 24731-EPS1, L. fermentum DSM 20049-EPS6, and B. longum ssp. longum DSM 200707-EPS10, all of which had comparable degrading capabilities, and L. acidophilus DSM 20079 T-EPS7 had the lowest rate of inhibition at 23.95%. Because EPS1, EPS5, EPS6, and EPS10 exhibited significantly greater antioxidant activity than other EPSs tested, they were chosen for subsequent assays in this study.
Table 2. Antioxidant activities (%) of EPS derived from LAB and Bb using DPPH radical scavenging assay.
Table 3. Antioxidant activities (%) of EPS derived from LAB and Bb using total antioxidant capacity (TAC) scavenging assay.
As illustrated in Figure 2, MCF7, CaCo2, and HepG2 cells all responded differently to the MTT cytotoxicity experiment. All of the EPSs inhibited cells in a concentration-dependent manner, with varying effects on various cell lines. After 24 h of treatment with the highest doses (20 mg/ml) of EPS1, EPS5, EPS6, and EPS10, the viability percentages for HEK 293 cells were 93.07, 96.3, 93.05, and 90.07 mg/ml, respectively (Figure 2A); MCF7 cells were 55.9, 52.1, 85.52, and 75.01 mg/ml, respectively (Figure 2B); CaCo2 cells were 68.69, 67.0, and 87.59 mg/ml, respectively (Figure 2C) and HepG2 cells were 62.0, 59.5, 83.86, and 72.66 mg/ml, respectively (Figure 2D). As indicated in Figure 2A, the impact of the chosen EPSs on viability (%) of HEK 293 cells showed that at 0.625 mg/ml (98.9%), the viability rate of HEK 293 cells was not significantly different (p = 0.1340). When the growth rate of HEK 293 cells was compared to that of cells treated with EPS at doses of 0.125 mg/ml (p = 0.0027), 2.5 mg/ml (p = 0.0021), 5 mg/ml (p = 0.0090), 10 mg/ml (p = 0.0037), and 20 mg/ml (p = 0.0012), a significant difference was observed. At 20 mg/ml, MCF7 viability decreased by more than 30% as compared to control viability, a significant difference of p = 0.0066. On the other hand, at EPSs concentrations of 0.625 (p = 0.1340), the MCF7 viability rate was not significantly different (Figure 2B). A significant difference was detected when the CaCo2 viability% of the control was compared to cells treated with EPSs concentrations of 5 mg/ml (p = 0.0022), 10 mg/ml (p = 0.0019), and 20 mg/ml (p = 0.0038) (Figure 2C). When the HepG2 viability% of the control was compared to cells treated with EPSs doses of 2.5 mg/ml (p = 0.0007) and 5 mg/ml (p = 0.0010), a very significant difference was seen (Figure 2D). Generally, according to the obtained data for EPS1 and EPS5, the extract’s potency was in the order MCF7 > HepG2 > CaCo2 at various concentrations; however, EPS6 and EPS10 had a strong inhibitory rate in the order HepG2 > MCF7 > CaCo2. Furthermore, EPSs were found to have a minimal cytotoxic effect on HEK 293 cells. According to the findings, EPS greatly reduced the viability of different cancer cells while having no impact on normal cells.
Figure 2. Cytotoxicity assessments of exopolysaccharides on different cancer cell lines using MTT assay. (A) On HEK293 cell line, (B) on MCF7 cell line, (C) on CaCo2 cell line, and (D) on HepG2 cell line. EPS1, EPS derived from S. thermophilus DSM 24731; EPS5, EPS derived from L. delbrueckii ssp. bulgaricus DSMZ 20081T; EPS6, EPS extracted from L. fermentum DSMZ 20049, and EPS10, EPS derived from B. longum ssp. longum DSMZ 200707.
As shown in Figure 3, the IC50 values of EPS1, EPS5, EPS6, and EPS10, for MCF7 cells were 8.06, 7.91, 22.97, and 12.36 mg/ml, respectively; for CaCo2, they were 10.69, 11.22, 25.85, and 15.23 mg/ml, respectively; and for HepG2 cells, they were 9.12, 9.37, 20.40, and 12.54 mg/ml, respectively. EPS5 had the most active components and exhibited the highest cytotoxicity with the lowest IC50 values on MCF7, CaCo2, and HepG2 (7.91, 10.69, and 9.12 mg/ml, respectively). EPS6, on the other hand, had the highest IC50 values against MCF7, CaCo2, and HepG2 at 12.36, 15.23, and 12.54 mg/ml, respectively. It is worth noting that untreated control cells retained a high viability. While EPS decreased the viability of normal HEK 293, their IC50 values of 29.26–85.7 mg/ml were significantly greater than those of cancerous cells (Figure 3). These results demonstrated that different EPSs may have a significant influence on EPS biological activities and that the inhibitory feature differed with human cell strains and produced severe cytotoxicity at large doses.
Figure 3. Cytotoxicity of EPSs against HEK293, MCF7, CaCo2, and HepG2 using MTT assay. EPS1, EPS derived from S. thermophilus DSM 24731; EPS5, EPS derived from L. delbrueckii ssp. bulgaricus DSMZ 20081T; EPS6, EPS extracted from L. fermentum DSMZ 20049, and EPS10, EPS derived from B. longum ssp. longum DSMZ 200707.
Annexin V has been shown to attach to apoptotic phosphatidylserine-releasing cells and decrease their pro-coagulant and pro-inflammatory activity. The obtained results showed that cellular apoptosis was triggered in MCF7, CaCo2, and HepG2-treated cells following the IC50 value treatment of the selected crude EPSs, as shown in Figure 4. In MCF7, CaCo2, and HepG2 cells treated with EPS5-IC50 values, the greatest Annexin V quantities (18.4, 10.28, and 16.30 ng/ml, respectively) were observed in comparison to other EPS treatments. On the other hand, apoptosis assays after EPS6 treatment revealed that total endogenous Annexin V levels were low in a variety of cancer cells, suggesting that EPS6 is an ineffective anticancer agent. Generally, the total endogenous Annexin V levels in cells treated with various EPSs indicated that the concentrations of Annexin V were not significantly different (p = 0.2002, 0.1077, 0.7084, and 0.5011) in cells treated with EPS1, EPS5, EPS6, and EPS10 compared to control cells (untreated) (Figure 4).
Figure 4. Apoptosis assessment using annexin V ELISA kit on cancer cell lines. EPS1, EPS derived from S. thermophilus DSM 24731; EPS5, EPS derived from L. delbrueckii ssp. bulgaricus DSMZ 20081T; EPS6, EPS extracted from L. fermentum DSMZ 20049, and EPS10, EPS derived from B. longum ssp. longum DSMZ 200707.
The quantity of LDH released, a soluble cytoplasmic enzyme, was utilized to determine the degree of degradation and leakage in cell membranes impacted by the IC50 of EPS1, EPS5, EPS6, and EPS10 (Figure 5). Regarding MCF7, LDH activity in EPS1 and EPS5-treated cells significantly increased by 2.36 and 2.58 times, respectively, related to the control (untreated) MCF7 cells (p < 0.0001) as illustrated in Figure 5. Furthermore, in the case of CaCo2, LDH activity increased by 2.85- and 3.02-fold, respectively, in the cells treated with IC50 values of each EPS1 and EPS5 relative to the untreated CaCo2 cells. LDH activity was highest (670 nmol/ml) in HepG2 cells treated with EPS5. As illustrated in Figure 5, EPS5 had a considerably higher LDH activity than EPS1 (p = 0.0001), followed by EPS10 and EPS6. In comparison to the control cell, LDH leakage concentrations in MCF7 cells treated with various EPSs varied significantly (p = 0.0202). In general, EPS5 demonstrated significant antitumor activity in the three types of cell lines analyzed in our research, based on the data obtained on the antitumor abilities of the tested EPSs. As a result, EPS5 will be further characterized and used in the following biological applications.
Figure 5. Lactate dehydrogenase (LDH) activity in MCF7, CaCo2, and HepG2 treated with different EPSs. EPS1, EPS derived from S. thermophilus DSM 24731; EPS5, EPS derived from L. delbrueckii ssp. bulgaricus DSMZ 20081T; EPS6, EPS extracted from L. fermentum DSMZ 20049, and EPS10, EPS derived from B. longum ssp. longum DSMZ 200707.
Fourier transform infrared analysis showed a variation in the functional groups formed in EPS5, as shown in Figure 6. There were eight absorbance bands in the FT-IR spectrum of EPS5; 3410, 2931, 2502, 1650, 1381, 1136, 872, 620, and 530 cm–1. Table 4 displays the retention period, area under the curve, and monosaccharide composition of EPS5 samples as measured by HPLC. The EPS samples’ sugar content varied substantially (p < 0.05). EPS5 included a high concentration of sugars such as rhamnose, mannose, ribose, glucose, and galactose. The sugar concentrations in EPS5 varied from 1.7938 to 38.8584 mg/100 g, with galactose being the greatest concentration. The ratio of ribose, rhamnose, glucose, mannose, and galactose in EPS5 was 1:2.7:7.4:5.1:12.5. Consequently, the EPS5 samples were heteropolysaccharides based on their sugar content.
Table 4. Determination of monosaccharide composition of L. delbrueckii ssp. bulgaricus DSM 20081T-EPS5 using HPLC.
For 24 h, we used quantitative real-time PCR to determine the mRNA levels of apoptotic and cell cycle-related genes in response to the IC50-EPS5 value (Figure 7). The data of gene expression revealed that EPS5 with IC50 value was significantly upregulated Bax gene expression in CaCo2 cells (p = 0.0065) compared with that in control (Figure 7A). However, a marked reduction in expression of the BCL2 and MCL1 (p = 0.0001) genes were observed in treated CaCo2 cells (Figure 7A). Additionally, the results indicated that EPS5 administration increased the expression of apoptotic genes Caspase 3 and Caspase 8. The most significant upregulation of the p53 gene was detected in CaCo2 cells treated with IC50-EPS5 (p = 0.0001). In comparison, the least significant upregulation of the Bax gene was found in MCF7 cells treated with the same concentration of EPS5 (p = 0.0002) (Figure 7B). Additionally, in the treated MCF7 cells, BCl2, MCL1, and Vimentin genes observed a downregulation at their expression level (p < 0.0001). However, the Caspase 3, Caspase 8, and Bax genes recorded upregulation of expression (Figure 7B). As demonstrated in Figure 7C, BCL2 expression was strongly downregulated in treated HepG2 cells, as has been the case with MCL1 and Vimentin expression, dramatically lowering the levels of the studied genes relative to control (p = 0.0001). EPS5, on the other hand, resulted in a significant increase in the expression of p53 (12.04) as compared to the control (p = 0.0001) but had no influence on Bax expression.
Figure 7. Effect of L. delbrueckii subsp. bulgaricus DSM 20081T-EPS5 on the relative expression of genes linked to apoptosis in different cell lines. (A) MCF7, (B) CaCo2, (C) HepG2.
Cultured hPDLFCs were successfully extracted from healthy molar teeth (Figure 8). After 24 h of co-incubation with various concentrations of crude EPS5, cell viability was determined using the MTT assay. As illustrated in Figure 9, the viability of hPDLFCs was significantly increased following exposure to crude EPS. When hPDLFCs were treated with EPSs at a concentration of 0.625 mg/ml, the viability rate was not significantly different from that of untreated cells (p = 0.1583). When the viability percent of untreated hPDLFCs was compared to cells treated with 2.5 mg/ml (p = 0.0003) and 5 mg/ml (p = 0.0003) EPSs, a significant difference (p = 0.0001) was observed (Figure 9).
Figure 8. Isolation steps of human periodontal ligament fibroblast cells (hPDLF). (A) Healthy molar tooth, (B) scraping periodontal layers, (C,D) mincing of the periodontal membrane.
Figure 9. The effect of crude EPS5 derived from L. delbrueckii ssp. bulgaricus DSM 20081T on cell viability in hPDLF cells using MTT assay.
In this study, LAB (n = 9) and Bb (n = 1) were used to extract EPS, and their EPS yield was determined. The EPS obtained demonstrated a high degree of concentration- and strain-dependent variation (p < 0.05). When compared to other tested strains, L. delbrueckii ssp. bulgaricus DSM 20081T produced the most EPS (295.49 ± 0.7). Tukenmez et al. (2019) reported that L. delbrueckii ssp. bulgaricus B3 produced the highest amount of EPS (449.0 ± 0.4 mg/l). According to Sungur et al. (2017), two strains of L. gasseri produced 242 and 255 mg/l of EPS. Bifidobacterium bifidum WBIN03 produced EPS at a concentration of 241.20 mg/l, whereas L. plantarum R315 produced EPS at a concentration of 290.17 mg/l (Li S. et al., 2014).
Reactive oxygen species (ROS) and oxygen-centered free radicals are byproducts of a number of metabolic and physiological processes in the body (Thibessard et al., 2004). Increased levels of ROS degrade proteins, DNA, and lipids, causing additional tissue damage and organ dysfunction. This causes serious side effects in humans, such as carcinoma, arteriosclerosis, osteoarthritis, and neurodegeneration (Li C. et al., 2014). Antioxidants are substances that prevent or postpone oxidation. Tumor cells produce ROS as a result of increased metabolic activity and a variety of other tumorigenic processes, which promotes tumor cell proliferation and survival, mesenchymal migration and penetration, genetic mutations, and angiogenesis. Antioxidant-based therapy that reduces ROS generation may be used to delay or even prevent tumor development (Liou and Storz, 2010; Gurunathan et al., 2013). Numerous research have been conducted on EPS derived from probiotic bacteria, which may possess antioxidant activities (Adebayo-Tayo and Fashogbon, 2020; Wu et al., 2021). According to our findings, the radical scavenging capacity of EPS1 (77%) and EPS5 (75.2%) significantly contributes to antioxidant activity. Adebayo-Tayo and Fashogbon (2020) evaluate the antioxidant activity of EPS produced by wild type EPSWLD L. delbrueckii ssp. bulgaricus and mutant EPSMLD L. delbrueckii ssp. bulgaricus using the DPPH system and other biological tests. The antioxidant potential of EPSWLD and EPSMLD was found to be between 1.21 and 1.80% and between 0.41 and 1.42% for their DPPH-scavenging capacities (37.5–73.4% vs. 37.5–65.6%, respectively). El-Adawi et al. (2012) reported that extracellular extracts of B. longum and L. plantarum had a DPPH scavenging capacity of 89.8 and 89.8%, respectively. The entire culture of L. delbrueckii ssp. bulgaricus DSM 20081T possessed the highest DPPH radical scavenging activity. According to Li S. et al. (2014), B. bifidum WBIN03 (B-EPS) and L. plantarum R315 (L-EPS) both had a high DPPH scavenging potential that increased with EPS concentration. Clearly, 70 mg/ml EPSs had a high DPPH scavenging potential when they compared the DPPH scavenging behavior of different concentrations of B-EPS (30 mg/ml, 94.4%) and L-EPS (70 mg/ml, 94.49%). Recent research has revealed that the antioxidant ability of EPS is affected by a number of factors, including molecular weight, monosaccharide composition, monosaccharide molar ratio, and glycoside bonding (Wu et al., 2021). As a result, the significant antioxidant activity of EPS could be attributed to its chemical groups, which include the hydroxyl group, carbon-free radicals, and the sulfated group (Wang et al., 2015).
Cancer is a disease that causes uncontrollable cell proliferation, resulting in organ damage and, eventually, death. The death toll from various types of cancer is steadily rising year after year. Current chemo and radiation therapy for cancer patients harms both tumor and normal cells (El Ghany et al., 2015; Zhang et al., 2017). The current research aims to produce antitumor agents that have fewer side effects than currently available synthetic pharmaceuticals. As a result, the antitumor properties of EPS are being investigated as a potential cancer treatment in this study. The cytotoxicity of EPSs against human breast, colon, and liver cancer cells was determined, as well as their role in apoptotic gene expression. The majority of anti-cancer medications have been shown to be cytotoxic to healthy cells, highlighting the critical need for safe alternatives in biomedicine (Zhang et al., 2017). Mohanta et al. (2017) demonstrated that the MTT test is one of the most advanced methods for determining the cytotoxic effects of cancerous cells. It is based on the reduction of MTT into formazan by mitochondrial succinate dehydrogenase in the mitochondria of metabolically active cells. The results of the cytotoxicity tests in this experiment revealed that treatment with EPSs elicited different cellular responses depending on the cause of cell death and the sensitivity of the treatment. Specifically, the IC50 values for EPSs on normal mammalian cells (HEK 293) ranged from 85.7 to 29.68 mg/ml. Nami et al. (2015) found that Enterococcus lactis IW5 secretions had no negative effects on normal cells, with 95% of the cells developing normally. Furthermore, a dosage of 2–5 mg/ml of new purified EPS L. acidophilus 20079 in healthy mammalian cells was shown to have a selectivity index of 1.96–51.3 for destroying cancerous cells (El-Deeb et al., 2018).
Exopolysaccharides from L. plantarum has not been found to be harmful in normal fibroblast cells L929 until a concentration of 50 mg/ml is reached (Ismail and Nampoothiri, 2013). The viability percentage of L929 is greater than 60 after treatment with 40 mg/ml EPS from L. paracasei and Lactobacillus brevis on L929 and HT29. On the other hand, HT29 has a survival rate of less than 20% (Mojibi et al., 2019). Based on the multiple cancerous cell death pathways and related sensitivity to treatment, we can assume that cytotoxicity experiments revealed the presence of non-identical cellular responses to EPS treatment. EPS5 had the most active components and exhibited the lowest IC50 values on MCF7, CaCo2, and HepG2 (7.91, 10.69, and 9.12 mg/ml, respectively, p < 0.05). Vidhyalakshmi and Vallinachiyar (2013) demonstrated that EPS derived from Bacillus sp. has a high potential for cytotoxicity against MCF7 cells at low concentrations while having no effect on normal cells. Additionally, Liu et al. (2011) found that L. casei 01 EPS inhibited HT29 growth at doses ranging from 5 to 100 μg/ml. L. fermentum extracts inhibited colon cancer cell growth while enhancing non-cancerous colon cell growth (Kahouli et al., 2015). Their findings on cancer cells suggest that the presence of propionate and butyrate may contribute to the probiotic bacteria’s selectivity, as histone deacetylase inhibitors (HDACs) stimulate proliferation in healthy colon cells but kill cancer cells (Kahouli et al., 2015). Nami et al. (2015) reported that the E. lactis IW5 byproducts reduced the viability of several types of cancer cells, including HeLa, MCF7, AGS, and HT-29, and that the primary mechanism underlying this effect was the activation of apoptosis in cancer cells.
Annexin V analysis revealed a decrease in the percentage of viable cells in all investigated cell lines with increased EPSs concentrations. A rise in the concentration of Annexin V in treated cells indicates that EPS induces apoptosis. The investigation revealed that treatment with the IC50 of EPS5 resulted in a significantly increased number of apoptotic cells (18.4, 10.28, and 16.3 ng/ml) on MCF7, CaCo2, and HepG2, respectively, followed by treatment with EPS1, whereas treatment with the IC50 of EPS6 resulted in a significantly decreased number of apoptotic cells. Apoptosis is primarily defined by changes in the morphology and biochemistry of the cells. EPS of L. paracasei and L. brevis inhibited the proliferation of HT29 cancer cells (Mojibi et al., 2019). When anti-proliferative activity and cell growth are compared 24 h after treatment with L. paracasei or L. brevis EPS at a concentration of 40 mg/ml, anti-proliferative activity and cell death increase from 36 to 80% and from 40 to 90%, respectively. S. thermophilus CH9-EPS-3a demonstrated greater anticancer activity in vitro against human liver cancer HepG2 cells, and the antitumor efficacy of EPS’s-3a was associated with cell apoptosis in HepG2 cells (Liu et al., 2019). Normal cells possess significantly fewer anti-proliferative and inhibitory properties than cancerous cells. Increased LDH leakage into the culture supernatant is also indicative of EPS-induced membrane damage (Deepak et al., 2016). LDH activity was found to be significantly greater in cancer cells treated with EPS5 than in cancer cells treated with EPS1 (p = 0.0001), followed by EPS10 and EPS6, confirming disruption of the plasma membrane and subsequent LDH dispersion into extracellular space (Fotakis and Timbrell, 2006).
It has been demonstrated that EPSs perform a variety of bioactive functions, and as a result, these functions are typically influenced positively by the EPS’s physicochemical properties (Wang et al., 2014; Li et al., 2016). This indicates a distinction between the functional groups defined in EPS5 when discussing FT-IR results. The strongest bands in the 3,410 cm–1 region are indicative of the presence of the EPS5 hydroxyl-stretching vibration (Abdhul et al., 2014). The bands at 2,931 cm–1 are produced by CH2 stretching vibrations, whereas the bands at 1,650 cm–1 are produced by –OH bending vibrations. Absorption at 1,381 cm–1 may be due to symmetric CH3 bending. At 1,136 cm–1, vibrations stretching the glycosidic bond (C–O–C) were responsible for the strong absorption (Adebayo-Tayo and Fashogbon, 2020). Additionally, strong infrared absorptions at 872 cm–1 indicated that EPS5 was β-anomeric (Pan and Mei, 2010). The monosaccharide of EPS5 was investigated using HPLC. The EPS5 is primarily composed of various sugars with molecular ratios 1:2.7:7.4:5.1:12.5, reflecting ribose:rhamnose:glucose:mannose:galactose, respectively.
The most critical biochemical process that occurs during early apoptosis is phosphatidylserine translocation. Several biochemical pathways converge during apoptosis, resulting in the activation of a family of cysteine-dependent aspartate-directed proteases (caspases). Although caspase-dependent or caspase-independent mechanisms can regulate apoptosis, the latter is more prevalent because the majority of cells initiate apoptosis via caspase activation (Gamal-Eldeen et al., 2009). We investigated the effect of L. delbrueckii ssp. bulgaricus DSMZ 20081T-EPS5 on apoptotic marker gene expression in MCF7, CaCo2, and HepG2 cells as a result of the antitumor data obtained. The relative expression of the apoptotic genes Bax, BCL2, Caspase 3, Caspase 8, and MCL1 was determined in this experiment. Board proteins, such as BCL2, regulate both cell damage and proliferation. BCL2 family proteins are required for mitochondria-mediated apoptosis, as they maintain the mitochondrial membrane’s integrity (Aouacheria et al., 2017).
The mechanism by which polysaccharides induce apoptosis in cancerous cells was investigated using BCL2 family proteins such as BCL2, which inhibits apoptosis, and Bax, which induces apoptosis. The results of the gene expression analysis indicated that EPS5 increased Bax gene expression in the cell lines examined. However, cells treated with EPS5 showed a significant decrease in BCL2 gene expression. Numerous studies have shown that tumor cells treated with LAB EPS expressed significantly less BCL2. Tukenmez et al. (2019) found that L. delbrueckii ssp. bulgaricus B3-EPS inhibited time-dependent proliferation and induced apoptosis by increasing Bax, Caspase 3, and Caspase 9 expression and decreasing BCL2 and Survivin expression. Cell-bound exopolysaccharides (cb-EPS) derived from L. acidophilus 606 inhibited the growth of colon cancer cells HT-29 by activating the Bax gene (Yang et al., 2007). Additionally, the MCL1 gene, a member of the BCL2 family of anti-apoptotic proteins that promotes apoptosis activation via mitochondrial pathways in C32 melanoma cells, inhibits apoptosis in a variety of cancers Bak and Bax pro-apoptotic proteins (Beberok et al., 2020). Caspases are involved in the regulation of the majority of molecules involved in cell death. Caspases involved in apoptosis can be classified as initiators (Caspases 2, 8, 9, and 10) or effectors (Caspases 3, 6, and 7) (Wang et al., 2016).
Given the critical role of Caspase 3 in apoptosis and the fact that many well-characterized breast cancer cell lines have altered Caspase 3 expression, RT-PCR was performed using Caspase 3-specific primers in treated MCF7 cells. Caspase 3 was significantly overexpressed in treated MCF7 cells compared to other cancer cells tested, according to our findings. Caspase 3 activity was detected in response to a variety of apoptotic factors, including chemotherapeutic agents, radiotherapy, and cytokines. Selective Caspase 3 inhibition, on the other hand, is associated with cell death inhibition. It has been postulated that a lack of Caspase 3 expression allows apoptosis-resistant breast cancer cells to respond to apoptotic stimuli like chemotherapy and radiotherapy. Several findings have significant clinical implications for using Caspase 3 as a disease marker for breast cancer as well as a potential therapeutic target (Devarajan et al., 2002). Northern blot analysis was used to confirm the absence of Caspase 3 expression in breast cancer cells. Caspase 3 mRNA levels in breast cancer cells were 10- to 50-fold lower than in normal breast tissue. In drug-sensitive MCF7 cells, Caspase 3 mRNA was truncated by a 125-bp transcript deletion. Additionally, it was established that drug-resistant (MCF7/DOX) MCF7 cells derived from continuous MCF7 cell culture had a maximum Caspase 3 transcript duration in the presence of doxorubicin (Devarajan et al., 2002). The significant increases in Caspase 8 and Caspase 3 genes in treated cells in our results are consistent with Chang et al. (2013). Caspase 3 was expected to be an adequate death protease catalyzing protein breakdown. Caspase 8 is found at the apex of an apoptotic cascade, where it causes proteolytic activation of members of the downstream caspase family, which contributes to apoptosis (Chang et al., 2013). Two pathways triggered the initiator: the first is regulated by Caspase 8. It includes the insertion of cell death ligands, which then activates Caspase 8 and Caspase 3, and the later involves apoptosis mediated by Caspase 9 mitochondria (Karpel-Massler et al., 2017).
The p53 tumor suppressor can also regulate pro-apoptotic genes related to both intrinsic and extrinsic pathways (Chipuk et al., 2003). El-Deeb et al. (2018) observed that after treating CaCo2 cells with L. acidophilus LA-EPS-20079 EPS, p53 gene expressions increased. EPS5 increased the level of p53 transcript in the tested CaCo2 cell line, implying that a p53-dependent pathway is active in the apoptotic mechanism of CaCo2 cells. To maintain cellular integrity and tolerance to stress, normal mesenchymal cells produce Vimentin, a protein belonging to the intermediate filament (IF) family (Chipuk et al., 2003). Vimentin aberrant overexpression has been linked to a higher level of aggressiveness in cancer cells, including melanoma, breast cancer, gastrointestinal cancers, and nervous system tumors. Vimentin overexpression in cancer is linked to increased tumor development, invasion, and a bad prognosis; nevertheless, the function of Vimentin in the development of cancer is still unknown. To our knowledge, no research on the effect of EPS on Vimentin gene expression regulation in MCF7 has been conducted. McInroy and Määttä (2007) demonstrated that silencing Vimentin expression inhibits the migration and invasion of colon and breast cancer cell lines. They demonstrated that downregulation of Vimentin expression impairs migration in both scratch wound experiments and invasion studies using collagen-coated cell culture inserts.
Recent research has established the efficacy of polysaccharides in inducing apoptosis in cancer cells by focusing on signaling molecules (Alwarsamy et al., 2016). As illustrated in Figure 10, polysaccharides exert their antitumor activity via two distinct mechanisms: the BCL2-regulated (also known as intrinsic, mitochondrial, or stress) pathway, which is activated by cytokine deprivation, ER stress, or DNA damage, and the death receptor (also known as extrinsic) pathway, which is activated by ligation of members of the tumor necrosis factor receptor (TNFR) family bearing an intracellular death domain (Green, 2005; Czabotar et al., 2014; Zhang et al., 2017). Overexpression of the pro-apoptotic BH3-only members (BIM, PUMA, BID, BMF, BAD, BIK, NOXA, and HRK) of the BCL2 protein family, which are members of the pro-apoptotic BH3-only subfamily, promotes cell death through the BCL2-regulated apoptotic pathway. The BH3-only proteins interact with and block pro-survival BCL2 proteins (BCL2, BCLXL, MCL1, BCLW, and A1/BFL1), hence activating the cell death effectors BAX and BAK (the pro-apoptotic multi-BH domain members of the BCL2 family that may also contain BOK) (Ke et al., 2015; Llambi et al., 2016). Certain BH3-only proteins have been shown to directly activate BAX/BAK (Green, 2005; Youle and Strasser, 2008). When BAX/BAK is activated, the mitochondrial outer membrane (MOMP) is permeabilized, triggering the activation of a cascade of aspartate-specific cysteine proteases (Zhang et al., 2007) and its activator APAF-1 (Cecconi et al., 1998) that dismantle cells (Figure 10). In comparison, the downregulation system induces apoptosis by binding and activating Procaspase-8 at ligated death receptors in the plasma membrane via the adaptors FADD and, in rare cases, TRADD. In so-called type 1 cells, activation of Caspase 8 followed by activation of effector caspases (Caspases 3 and 7) is sufficient to successfully induce apoptosis (e.g., thymocytes). On the other hand, effective cell death in so-called type 2 cells (e.g., hepatocytes) requires caspase cascade amplification via crossover activation of the BCL2-regulated apoptotic pathway, which is accomplished through Caspase 8-mediated proteolytic activation of the normally inert BH3-only protein BID (Figure 10; Zhang et al., 2007, 2017; Fan et al., 2014).
Human periodontal ligament fibroblast cells (hPDLFCs) are one of several cell types involved in periodontal tissue regeneration (Son et al., 2019). In addition, few studies have been published on the effect of EPSs on the viability of hPDLFCs. In conclusion, EPS5 derived from L. delbrueckii ssp. bulgaricus DSM 20081T exhibits a range of bioactivities including antioxidant, antitumor, and proliferative properties, thereby establishing it as a possible natural agent for the development of novel periodontal healing and regeneration strategies. Furthermore, because of its extraordinary cytotoxic effect at the IC50 value in cancer cells, EPS5 could be used in medical research as a potential antitumor agent. As a result, the potential for EPS to replace chemotherapeutic medications in the future must be considered alongside their safety and public health issues. Overall, the LAB EPS has significant therapeutic potential, most notably in the antioxidant, antitumor, and periodontal regeneration fields. However, additional research is necessary to fully understand EPS5’s mechanism of action in periodontal healing and tissue restoration.
The original contributions presented in the study are included in the article/Supplementary Material, further inquiries can be directed to the corresponding author/s.
The studies involving human participants were reviewed and approved by College of Dentistry, Taif University, Taif, Saudi Arabia, Dentistry Clinics Hospitals Ethical Committee Centers. The patients/participants provided their written informed consent to participate in this study.
MK: conceptualization, methodology, formal analysis, data curation, and writing—review and editing. FS: conceptualization, validation, and visualization. LA-M and AA: methodology and investigation. TA: methodology and writing—review and editing. SA: statistical analysis, formal analysis, data curation, and writing—review and editing. All authors contributed to the article and approved the submitted version.
This study was supported by Taif University Researchers Supporting Project Number (TURSP-2020/95), Taif, Saudi Arabia. This investigation was also funded by Research Project Funds from Tanta University (Tu-03-13-04).
The authors declare that the research was conducted in the absence of any commercial or financial relationships that could be construed as a potential conflict of interest.
All claims expressed in this article are solely those of the authors and do not necessarily represent those of their affiliated organizations, or those of the publisher, the editors and the reviewers. Any product that may be evaluated in this article, or claim that may be made by its manufacturer, is not guaranteed or endorsed by the publisher.
The Supplementary Material for this article can be found online at: https://www.frontiersin.org/articles/10.3389/fmicb.2022.803688/full#supplementary-material
Abdhul, K., Ganesh, J., Lee, M., Shanmughapriya, S., Kanagavel, M., Anbarasu, K., et al. (2014). Antioxidant activity of exopolysaccharide from probiotic strain Enterococcus faecum (BDU7) from Ngari. Int. J. Biol. Macromol. 70, 450–454. doi: 10.1016/j.ijbiomac.2014.07.026
Adebayo-Tayo, B., and Fashogbon, R. (2020). In vitro antioxidant, antibacterial, in vivo immunomodulatory, antitumor and hematological potential of exopolysaccharide produced by wild type and mutant Lactobacillus delbureckii subsp. bulgaricus. Heliyon 6:e03268. doi: 10.1016/j.heliyon.2020.e03268
Adebayo-Tayo, B., Ishola, R., and Oyewunmi, T. (2018). Characterization, antioxidant and immunomodulatory potential on exopolysaccharide produced by wild type and mutant Weissella confusa strains. Biotechnol. Rep. 19:e00271 doi: 10.1016/j.btre.2018.e00271
Adesulu-Dahunsi, A. T., Sanni, A. I., and Jeyaram, K. (2018). Production, characterization and in vitro antioxidant activities of exopolysaccharide from Weissella cibaria GA44. LWT 87, 432–442. doi: 10.1016/j.lwt.2017.09.013
Alwarsamy, M., Gooneratne, R., and Ravichandran, R. (2016). Effect of fucoidanfrom Turbinaria conoides on human lung adenocarcinoma epithelial (A549) cells. Carbohydr. Polym. 152, 207–213. doi: 10.1016/j.carbpol.2016.06.112
Angelin, J., and Kavitha, M. (2020). Exopolysaccharides from probiotic bacteria and their health potential. Int. J. Biol. Macromol. 162, 853–865. doi: 10.1016/j.ijbiomac.2020.06.190
Aouacheria, A., Baghdiguian, S., Lamb, H. M., Huska, J. D., Pineda, F. J., and Hardwick, J. M. (2017). Connecting mitochondrial dynamics and life-or-death events via Bcl-2 family proteins. Neurochem. Int. 109, 141–161. doi: 10.1016/j.neuint.2017.04.009
Arslan, Y. E., and Kantarcioglu, I. (2019). Salvadora persica extract-laden jellyfish collagen hybrid constructs for periodontal tissue regeneration. J. Turk. Chem. Soc. Sec. A Chem. 6, 51–62 doi: 10.18596/jotcsa.484936
Bajpai, V. K., Majumder, V., Rather, I. A., and Kim, K. (2016). Extraction, isolation and purification of exopolysaccharide from lactic acid bacteria using ethanol precipitation method. Bangladesh J. Pharmacol. 11, 573–576. doi: 10.3329/bjp.v11i3.27170
Barcelos, M. C. S., Vespermann, K. A. C., Pelissari, F. M., and Molına, G. (2019). Current status of biotechnological production and applications of microbial exopolysaccharides. Crit. Rev. Food Sci. Nutr. 60, 1475–1495. doi: 10.1080/10408398.2019.1575791
Beberok, A., Rok, J., Rzepka, Z., Marciniec, K., Boryczka, S., and Wrześniok, D. (2020). The role of MITF and Mcl-1 proteins in the antiproliferative and proapoptotic effect of ciprofloxacin in amelanotic melanoma cells: in silico and in vitro study. Toxicol. In Vitro 66:104884 doi: 10.1016/j.tiv.2020.104884
Biliavska, L., Pankivskay, P., Povnitsa, O., and Zagorodnya, S. (2019). Antiviral activity of exopolysaccharides produced by lactic acid bacteria of the genera Pediococcus, Leuconostoc and Lactobacillus against human adenovirus type 5. Medicina 55, 519–531. doi: 10.3390/medicina55090519
Bradford, M. M. (1976). A rapid and sensitive method for the quantitation of microgram quantities of protein utilizing the principle of proteindye binding. Anal. Biochem. 72, 248–254.
Bray, F., Ferlay, J., Soerjomataram, I., Siegel, R. L., Torre, L. A., and Jemal, A. (2018). Global cancer statistics 2018: GLOBOCAN estimates of incidence and mortality worldwide for 36 cancers in 185 countries. CA Cancer J. Clin. 68, 394–424. doi: 10.3322/caac.21492
Cecconi, F., Alvarez-Bolado, G., Meyer, B. I., Roth, K. A., and Gruss, P. (1998). Apaf-1 (CED-4 homologue) regulates programmed cell death in mammalian development. Cell 94, 727–737. doi: 10.1016/s0092-8674(00)81732-8
Chang, C. J., Lin, J. F., Chang, H. H., Lee, G. A., and Hung, C. F. (2013). Lutein protects against methotrexate-induced and reactive oxygen species-mediated apoptotic cell injury of IEC-6 cells. PLoS One 8:e72553. doi: 10.1371/journal.pone.0072553
Chipuk, J. E., Maurer, U., Green, D. R., and Schuler, M. (2003). Pharmacologic activation of p53 elicits Bax-dependent apoptosis in the absence of transcription. Cancer Cell 4, 371–381. doi: 10.1016/s1535-6108(03)00272-1
Czabotar, P. E., Lessene, G., Strasser, A., and Adams, J. M. (2014). Control of apoptosis by the BCL-2 protein family: implications for physiology and therapy. Nat. Rev. Mol. Cell Biol. 15, 49–63. doi: 10.1038/nrm3722
Deepak, V., Ramachandran, S., Balahmar, R. M., Pandian, S. R. K., Sivasubramaniam, S. D., Nellaiah, H., et al. (2016). In vitro evaluation of anticancer properties of exopolysaccharides from Lactobacillus acidophilus in colon cancer cell lines. In Vitro Cell Dev. Biol. Anim. 52, 163–173. doi: 10.1007/s11626-015-9970-3
Devarajan, E., Sahin, A. A., Chen, J. S., Krishnamurthy, R. R., Aggarwal, N., Brun, A. M., et al. (2002). Down-regulation of caspase 3 in breast cancer: a possible mechanism for chemoresistance. Oncogene 21, 8843–8851. doi: 10.1038/sj.onc.1206044
Dilna, S. V., Surya, H., Aswathy, R. G., Varsha, K. K., Sakthikumar, D. N., Pandey, A., et al. (2015). Characterization of an exopolysaccharide with potential health-benefit properties from a probiotic Lactobacillus plantarum RJF4. LWT Food Sci. Technol. 64, 1179–1186 doi: 10.1016/j.lwt.2015.07.040
Dubois, M., Gilles, K. A., Hamilton, J. K., Pebers, P. A., and Smith, F. (1956). Colorimetric method for determination of sugar and related substances. Anal. Chem. 28, 350–356. doi: 10.1021/ac60111a017
El-Adawi, H. I., Khalil, M. A., El-Sheekh, M. M., EL-Deeb, N. M., and Hussein, M. Z. (2012). Cytotoxicity assay and antioxidant activities of the lactic acid bacterial strains. Afr. J. Microb. Res. 6, 1700–1712. doi: 10.4014/jmb.1301.01032
El-Deeb, N. M., Yassin, A. M., Al-Madboly, L. A., and El-Hawiet, A. (2018). A novel purified Lactobacillus acidophilus 20079 exopolysaccharide, LA-EPS-20079, molecularly regulates both apoptotic and NF-κB inflammatory pathways in human colon cancer. Microb. Cell Fact. 17:29. doi: 10.1186/s12934-018-0877-z
El Ghany, K. A., Hamouda, R., Elhafez, E. A., Mahrous, H., Salem-Bekhit, M., and Hamza, H. A. (2015). A potential role of Lactobacillus acidophilus LA1 and its exopolysaccharides on cancer cells in male albino mice. Biotechnol. Biotechnol. Equip. 29, 977–983. doi: 10.1080/13102818.2015.1050455
Fan, Y., Lin, M., Luo, A., Chun, Z., and Luo, A. (2014). Characterization and antitumor activity of a polysaccharide from Sarcodia ceylonensis. Molecules 19, 10863–10876. doi: 10.3390/molecules190810863
Fontana, C., Li, S., and Yang, Z. (2015). Structural studies of the exopolysaccharide from Lactobacillus plantarum C88 using NMR spectroscopy and the program CASPER. Carbohydr. Res. 402, 7–94. doi: 10.1016/j.carres.2014.09.003
Fotakis, G., and Timbrell, A. (2006). In vitro cytotoxicity assays: comparison of LDH, neutral red, MTT and protein assay in hepatoma cell lines following exposure to cadmium chloride. Toxicol. Lett. 5, 171–177. doi: 10.1016/j.toxlet.2005.07.001
Gamal-Eldeen, A. M., Ahmed, E. F., and Abo-Zeid, M. A. (2009). In vitro cancer chemopreventive properties of polysaccharide extract from the brown alga, Sargassum latifolium. Food Chem. Toxicol. 47, 1378–1384 doi: 10.1016/j.fct.2009.03.016
Green, D. R. (2005). Apoptotic pathways: ten minutes to dead. Cell 121, 671–674. doi: 10.1016/j.cell.2005.05.019
Gurunathan, S., Han, J. W., Eppakayala, V., Jeyaraj, M., and Kim, J. H. (2013). Cytotoxicity of biologically synthesized silver nanoparticles in MDA-MB-231 human breast cancer cells. Biomed. Res. Int. 2013:535796. doi: 10.1155/2013/535796
Hu, Y. Q., Zhang, S., Xu, Z., Lv, Z. S., Liu, M. L., and Feng, L. S. (2017). 4-Quinolone hybrids and their antibacterial activities. Eur. J. Med. Chem. 141, 335–345. doi: 10.1016/j.ejmech.2017.09.05
Hussain, A., Zia, K. M., Tabasum, S., Noreen, A., Ali, M., Iqbal, R., et al. (2017). Blends and composites of exopolysaccharides; properties and applications: a review. Int. J. Biol. Macromol. 94, 10–27. doi: 10.1016/j.ijbiomac.2016.09.104
Ismail, B., and Nampoothiri, K. M. (2013). Exposition of antitumour activity of a chemically characterized exopolysaccharide from a probiotic Lactobacillus plantarum MTCC 9510. Biologia 68, 1041–1047. doi: 10.2478/s11756-013-0275-2
Iviglia, G., Kargozar, S., and Baino, F. (2019). Biomaterials, current strategies, and novel nanotechnological approaches for periodontal regeneration. J. Funct. Biomater. 10, 1–3. doi: 10.3390/jfb10010003
Joo Seo, B., Bajpai, V., Rather, I., and Park, Y. (2015). Partially purified exopolysaccharide from Lactobacillus plantarum YML009 with total phenolic content, antioxidant and free radical scavenging efficacy. Ind. J. Pharm. Educ. Res. 49, 282–292. doi: 10.5530/ijper.49.4.6
Kahouli, I., Malhotra, M., Alaoui-Jamaliand, M., and Prakash S. (2015). In-vitro characterization of the anticancer activity of the probiotic bacterium Lactobacillus fermentum NCIMB 5221 and potential against colorectal Cancer. Cancer Sci. Ther. 7, 224–235.
Kao, T. H., and Chen, B. H. (2006). Functional components in soybean cake and their effects on antioxidant activity. J. Agric. Food Chem. 54, 7544–7555. doi: 10.1021/jf061586x
Karpel-Massler, G., Ishida, C. T., Bianchetti, E., Shu, C., Perez-Lorenzo, R., Horst, B., et al. (2017). Inhibition of mitochondrial matrix chaperones and anti-apoptotic Bcl-2 family proteins empower antitumor therapeutic responses. Cancer Res. 77, 3513–3526. doi: 10.1158/0008-5472.CAN-16-3424
Ke, F., Grabow, S., Kelly, G. L., Lin, A., O’Reilly, L. A., and Strasser, A. (2015). Impact of the combined loss of BOK, BAX and BAK on the hematopoietic system is slightly more severe than compound loss of BAX and BAK. Cell Death Dis. 6:e1938. doi: 10.1038/cddis.2015.304
Khalil, M. A., El-Shanshoury, A.-E. R. R., Alghamdi, M. A., Alsalmi, F. A., Mohamed, S. F., Sun, J., et al. (2022). Biosynthesis of silver nanoparticles by Marine actinobacterium Nocardiopsis dassonvillei and exploring their therapeutic potentials. Front. Microbiol. 12:705673. doi: 10.3389/fmicb.2021.705673
Lakra, A. K., Domdi, L., Tilwani, Y. M., and Arul, V. (2020). Physicochemical and functional characterization of mannan exopolysaccharide from Weissella confusa MD1 with bioactivities. Int. J. Biol. Macromol. 143, 797–805. doi: 10.1016/j.ijbiomac.2019.09.139
Li, C., Li, W., Chen, X., Feng, M., Rui, X., Jiang, M., et al. (2014). Microbiological, physicochemical and rheological properties of fermented soymilk produced with exopolysaccharide (EPS) producing lactic acid bacteria strains. LWT Food Sci. Technol. 57, 477–485.
Li, C., Li, Z., Wang, Y., and Liu, H. (2016). Gold nanoparticles promote proliferation of human periodontal ligament stem cells and have limited effects on cells differentiation. J. Nanomater. 2016:1431836. doi: 10.1155/2016/1431836
Li, S., Huang, R., Shah, N. P., Tao, X., Xiong, Y., and Wei, H. (2014). Antioxidant and antibacterial activities of exopolysaccharides from Bifidobacterium bifidum WBIN03 and Lactobacillus plantarum R315. J. Dairy Sci. 97, 7334–7343 doi: 10.3168/jds.2014-7912
Li, W., Ji, J., Chen, X., Jiang, M., Rui, X., and Dong, M. (2014). Structural elucidation and antioxidant activities of exopolysaccharides from Lactobacillus helveticus MB2-1. Carbohydr. Polym. 102, 351–359. doi: 10.1016/j.carbpol.2013.11.053
Li, W., Tang, W., Ji, J., Xia, X., Rui, X., Chen, X., et al. (2015). Characterization of a novel polysaccharide with anti-colon cancer activity from Lactobacillus helveticus MB2-1. Carbohydr. Res. 411, 6–14. doi: 10.1016/j.carres.2014.12.014
Lin, X., Xia, Y., Wang, G., Yang, Y., Xiong, Z., Lv, F., et al. (2018). Lactic acid bacteria with antioxidant activities alleviating oxidized oil induced hepatic injury in mice. Front. Microbiol. 9:2684. doi: 10.3389/fmicb.2018.02684
Liou, G. Y., and Storz, P. (2010). Reactive oxygen species in cancer. Free Radic. Res. 44, 479–496. doi: 10.3109/10715761003667554
Liu, C., Zheng, J., Ou, X., and Han, Y. (2021). Anti-cancer substances and safety of lactic acid bacteria in clinical treatment. Front. Microbiol. 12:722052. doi: 10.3389/fmicb.2021.722052
Liu, C.-T., Chu, F.-J., Chou, C.-C., and Yu, R.-C. (2011). Antiproliferative and anticytotoxic effects of cell fractions and exopolysaccharides from Lactobacillus casei 01. Mutat. Res. 721, 157–162. doi: 10.1016/j.mrgentox.2011.01.005
Liu, J., Ren, Z., Fan, L., Wei, J., Tang, X., Xu, X., et al. (2019). Design, synthesis, biological evaluation, structure-activity relationship, and toxicity of clinafloxacin-azole conjugates as novel antitubercular agents. Bioorganic Med. Chem. 27, 175–187. doi: 10.1016/j.bmc.2018.11.035
Livak, K., and Schmittgen, D. (2001). Analysis of relative gene expression data using real time quantitative PCR and the 2 method. Methods 25, 402–408. doi: 10.1006/meth.2001.1262
Llambi, F., Wang, Y. M., Victor, B., Yang, M., Schneider, D. M., Gingras, S., et al. (2016). BOK is a noncanonical BCL-2 family effector of apoptosis regulated by ER-associated degradation. Cell 165, 421–433. doi: 10.1016/j.cell.2016.02.026
Manivasagan, P, Venkatesan, J, Senthikumar, K, Sivakumar, K, and Kim, S. K. (2013). Biosynthesis, antimicrobial and cytotoxic effect of silver nanoparticles using a novel Nocardiopsis sp. MBRC-1. Biomed. Res. Int. 2013:287638. doi: 10.1155/2013/287638
Mathur, H., Beresford, T. P., and Cotter, P. D. (2020). Health benefits of lactic acid bacteria (LAB) fermentates. Nutrients 12:1679. doi: 10.3390/nu12061679
McInroy, L., and Määttä, A. (2007). Down-regulation of vimentin expression inhibits carcinoma cell migration and adhesion. Biochem. Biophys. Res. Commun. 360, 109–114. doi: 10.1016/j.bbrc.2007.06.036
Mende, S., Rohm, H., and Jaros, D. (2016). Influence of exopolysaccharides on the structure, texture, stability and sensory properties of yoghurt and related products. Int. Dairy J. 52, 57–71. doi: 10.1016/j.idairyj.2015.08.002
Milner, E., Stevens, B., An, M., Lam, V., Ainsworth, M., Dihle, P., et al. (2021). Utilizing probiotics for the prevention and treatment of gastrointestinal diseases. Front. Microbiol. 12:689958. doi: 10.3389/fmicb.2021.689958
Mohanta, Y. K., Panda, S. K., Bastia, A. K., and Mohanta, T. K. (2017). Biosynthesis of silver nanoparticles from Protium serratum and investigation of their potential impacts on food safety and control. Front. Microbiol. 8:626. doi: 10.3389/fmicb.2017.00626
Mojibi, P., Tafvizi, F., and Bikhof Torbati, M. (2019). Cell-bound exopolysaccharide extract from indigenous probiotic bacteria induce apoptosis in HT–29 cell-line. Iran. J. Pathol. 14, 41–51. doi: 10.30699/ijp.14.1.41
Nami, Y., Haghshenas, B., Haghshenas, M., Abdullah, N., and Khosroushahi, A. (2015). The prophylactic effect of probiotic Enterococcus lactis IW5 against different human cancer cells front. Microbiology 6:1317. doi: 10.3389/fmicb.2015.01317
Nasajpour, A., Ansari, S., Rinoldi, C., Rad, A. S. Aghaloo, T., Shin, S. R., et al. (2018). A multifunctional polymeric periodontal membrane with osteogenic and antibacterial characteristics. Adv. Funct. Mater. 28, 1703437–1703445. doi: 10.1002/adfm.201703437
Nazir, Y., Hussain, S. A., Abdul Hamid, A., and Song, Y. (2018). Probiotics and their potential preventive and therapeutic role for cancer, high serum cholesterol, and allergic and HIV diseases. Biomed. Res. Int. 2018, 1–17. doi: 10.1155/2018/3428437
Norouzi, Z., Salimi, A., Halabian, R., and Fahimi, H. (2018). Nisin, a potent bacteriocin and anti-bacterial peptide, attenuates expression of metastatic genes in colorectal cancer cell lines. Microb. Pathog. 123, 183–189. doi: 10.1016/j.micpath.2018.07.006
Pan, D., and Mei, X. (2010). Antioxidant activity of an exopolysaccharide purified from Lactococcus lactis subsp. lactis 12. Carbohydr. Polym. 80, 908–914 doi: 10.1016/j.carbpol.2010.01.005
Qin, J.-Y. (2015). Current situation and prospect of human cancer prevention and control. Sci. Technol. Rev. 33:125
Rajoka, M. R. S., Zhao, H., Lu, Y., Lian, Z., Li, N., Hussain, N., et al. (2018). Anticancer potential against cervix cancer (HeLa) cell line of probiotic Lactobacillus casei and Lactobacillus paracasei strains isolated from human breast milk. Food Funct. 9, 2705–2715. doi: 10.1039/c8fo00547h
Sanalibaba, P., and Cakmak, G. A. (2016). Exopolysaccharides production by lactic acid bacteria. Appl. Micro. Open Access 2:1000115. doi: 10.4172/2471-9315.1000115
Śliżewska, K., Markowiak-Kopeæ, P., and Śliżewska, W. (2021). The role of probiotics in cancer prevention. Cancers 13:20. doi: 10.3390/cancers13010020
Son, H., Jeon, M., Choi, H. J., Lee, H. S., Kim, I. H., Kang, C. M., et al. (2019). Decellularized human periodontal ligament for periodontium regeneration. PLoS One 14:e0221236 doi: 10.1371/journal.pone.0221236
Sungur, T., Aslim, B., Karaaslan, C., and Aktas, B. (2017). Anaerobe impact of exopolysaccharides (EPSs) of Lactobacillus gasseri strains isolated from human vagina on cervical tumor cells (HeLa) Anaerobe 47, 137–144. doi: 10.1016/j.anaerobe.2017.05.013
Surayot, U., Wang, J., Seesuriyachan, P., Kuntiya, A., Tabarsa, M., Lee, Y., et al. (2014). Exopolysaccharides from lactic acid bacteria: structural analysis, molecular weight effect on immunomodulation. Int. J. Biol. Macromol. 68, 233–240. doi: 10.1016/j.ijbiomac.2014.05.005
Tang, W., Dong, M., Wang, W., Han, S., Rui, X., Chen, X., et al. (2017). Structural characterization and antioxidant property of released exopolysaccharides from Lactobacillus delbrueckii ssp. bulgaricus SRFM-1. Carbohydr. Polym. 173, 654–664. doi: 10.1016/j.carbpol.2017.06.039
Thibessard, A., Borges, F., Fernandez, A., Gintz, B., Decaris, B., and LeblondBourget, N. (2004). Identification of Streptococcus thermophilus CNRZ368 genes involved in defense against superoxide stress. Appl. Environ. Microbiol. 70, 2220–2229. doi: 10.1128/aem.70.4.2220-2229.2004
Tukenmez, U., Aktas, B, Aslim, B., and Yavuz, S. (2019). The relationship between the structural characteristics of lactobacilli-EPS and its ability to induce apoptosis in colon cancer cells in vitro. Sci. Rep. 9:8268. doi: 10.1038/s41598-019-44753-8
Vidhyalakshmi, R., and Vallinachiyar, C. (2013). Apoptosis of human breast cancer cells (MCF-7) induced by polysaccharides produced by bacteria. J. Cancer Sci. Ther. 5, 31–34.
Wang, G., Si, Q., Yang, S., Jiao, T., Zhu, H., Tian, P., et al. (2020). Lactic acid bacteria reduce diabetes symptoms in mice by alleviating gut microbiota dysbiosis and inflammation in different manners. Food Funct. 11, 5898–5914. doi: 10.1039/C9FO02761K
Wang, J., Hu, S., Nie, S, Yu, Q., and Xie, M. (2016). Reviews on mechanisms of in vitro antioxidant activity of polysaccharides. Oxid. Med. Cell Longev. 2016, 1–13. doi: 10.1155/2016/5692852
Wang, J., Zhao, X., Yang, Y., Zhao, A., and Yang, Z. (2015). Characterization and bioactivities of an exopolysaccharide produced by Lactobacillus plantarum YW32. Int. J. Biolog. Macromole 74, 119–126. doi: 10.1016/j.ijbiomac.2014.12.006
Wang, K., Li, W., Rui, X., Chen, X., Jiang, M., and Dong, M. (2014). Characterization of a novel exopolysaccharide with antitumor activity from Lactobacillus plantarum 70810. Int. J. Biol. Macromol. 63, 133–139. doi: 10.1016/j.ijbiomac.2013.10.036
Winner, J., Jung, C. K., Shackel, I., and Williams, P. M. (1999). Development and validation of real-time quantitative reverse transcriptase-polymerase chain reaction for monitoring gene expression in cardiac myocytes in vitro. Anal Biochem. 270, 41–49. doi: 10.1006/abio.1999.4085
Wu, J., Zhang, Y., Ye, L., and Wang, C. (2021). The anti-cancer effects and mechanisms of lactic acid bacteria exopolysaccharides in vitro: a review. Carbohydr. Polym. 253:117308. doi: 10.1016/j.carbpol.2020.117308
Yahya, S. M. M., Abdelnasser, S. M., Hamed, A. R., El Sayed, O. H., and Asker, M. S. (2019). Newly isolated marine bacterial exopolysaccharides enhance antitumor activity in HepG2 cells via affecting key apoptotic factors and activating toll like receptors. Mol. Biol. Rep. 46, 6231–6241. doi: 10.1007/s11033-019-05061-6
Yang, C. M., Cao, G. T., Ferket, P. R., Liu, T. T., Zhou, L., Zhang, L., et al. (2012). Effects of probiotic, Clostridium butyricum, on growth performance, immune function, and cecal microflora in broiler chickens. Poult. Sci. 91, 2121–2129. doi: 10.3382/ps.2011-02131
Yang, P., Peairs, J. J., Tano, R., Zhang, N., Tyrell, N., and Jaffe, G. J. (2007). Caspase-8–mediated apoptosis in human RPE cells. IVOS 48, 3341–3349. doi: 10.1167/iovs.06-1340
Youle, R. J., and Strasser, A. (2008). The BCL-2 protein family: opposing activities that mediate cell death. Nat. Rev. Mol. Cell Biol. 9, 47–59. doi: 10.1038/nrm2308
Zannini, E., Waters, D. M., Coffey, A., and Arendt, E. K. (2016). Production, properties, and industrial food application of lactic acid bacteria-derived exopolysaccharides. Appl. Microbiol. Biotechnol. 100, 1121–1135. doi: 10.1007/s00253-015-7172-2
Zhang, F., Shi, J.-J., Thakur, K., Hu, F., Zhang, J.-G., and Wei, Z.-J. (2017). Anti-cancerous potential of polysaccharide fractions extracted from peony seed dreg on various human cancer cell lines via cell cycle arrest and apoptosis. Front. Pharmacol. 8:102. doi: 10.3389/fphar.2017.00102
Zhang, M., Cui, S. W., Cheung, P. C. K., and Wang, Q. (2007). Antitumor polysaccharides from mushrooms: a review on their isolation process, structural characteristics and antitumor activity. Trends Food Sci. Technol. 18, 4–19. doi: 10.1016/j.tifs.2006.07.013
Zhang, Z., Liu, Z., Tao, X., and Wei, H. (2016). Characterization and sulfated modification of an exopolysaccharide from Lactobacillus plantarum ZDY2013 and its biological activities. Carbohydr. Polym. 153, 25–33. doi: 10.1016/j.carbpol.2016.07.084
Keywords: lactic acid bacteria, bifidobacteria, exopolysaccharide, RT-PCR, MCF7, CaCo2, HepG2, therapeutic agent
Citation: Khalil MA, Sonbol FI, Al-Madboly LA, Aboshady TA, Alqurashi AS and Ali SS (2022) Exploring the Therapeutic Potentials of Exopolysaccharides Derived From Lactic Acid Bacteria and Bifidobacteria: Antioxidant, Antitumor, and Periodontal Regeneration. Front. Microbiol. 13:803688. doi: 10.3389/fmicb.2022.803688
Received: 28 October 2021; Accepted: 16 February 2022;
Published: 25 April 2022.
Edited by:
Sabina Górska, Hirszfeld Institute of Immunology and Experimental Therapy (PAS), PolandReviewed by:
Junling Shi, Northwestern Polytechnical University, ChinaCopyright © 2022 Khalil, Sonbol, Al-Madboly, Aboshady, Alqurashi and Ali. This is an open-access article distributed under the terms of the Creative Commons Attribution License (CC BY). The use, distribution or reproduction in other forums is permitted, provided the original author(s) and the copyright owner(s) are credited and that the original publication in this journal is cited, in accordance with accepted academic practice. No use, distribution or reproduction is permitted which does not comply with these terms.
*Correspondence: Maha A. Khalil, bWFoYS5ha0B0dS5lZHUuc2E=, bWFoYS5raGFsaWxAc2NpZW5jZS50YW50YS5lZHUuZWc=; Sameh S. Ali, c2FtaEB1anMuZWR1LmNu, c2FtaF9zYW1pckBzY2llbmNlLnRhbnRhLmVkdS5lZw==
Disclaimer: All claims expressed in this article are solely those of the authors and do not necessarily represent those of their affiliated organizations, or those of the publisher, the editors and the reviewers. Any product that may be evaluated in this article or claim that may be made by its manufacturer is not guaranteed or endorsed by the publisher.
Research integrity at Frontiers
Learn more about the work of our research integrity team to safeguard the quality of each article we publish.