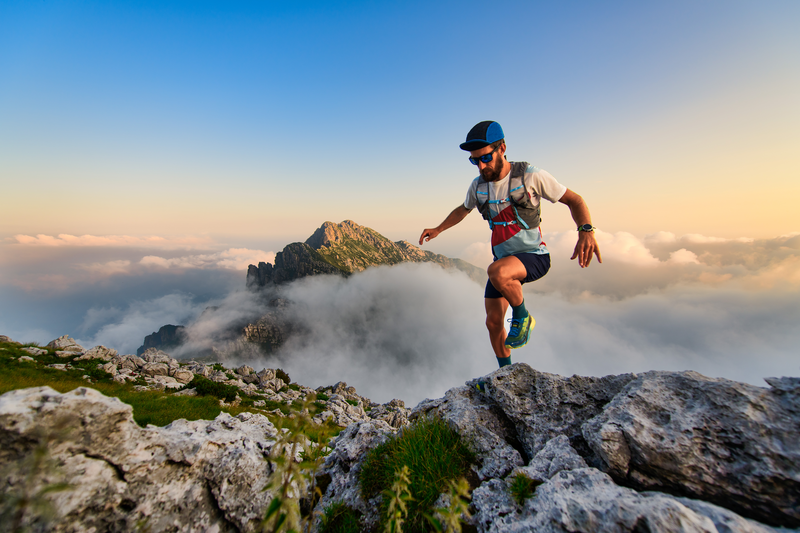
94% of researchers rate our articles as excellent or good
Learn more about the work of our research integrity team to safeguard the quality of each article we publish.
Find out more
ORIGINAL RESEARCH article
Front. Microbiol. , 02 June 2022
Sec. Food Microbiology
Volume 13 - 2022 | https://doi.org/10.3389/fmicb.2022.802625
This article is part of the Research Topic Food Safety and Public Health View all 33 articles
The increasing number of studies reporting the presence of Salmonella in environmental water sources suggests that it is beyond incidental findings originated from sparse fecal contamination events. However, there is no consensus on the occurrence of Salmonella as its relative serovar representation across non-recycled water sources. We conducted a meta-analysis of proportions by fitting a random-effects model using the restricted maximum-likelihood estimator to obtain the weighted average proportion and between-study variance associated with the occurrence of Salmonella in water sources. Moreover, meta-regression and non-parametric supervised machine learning method were performed to predict the effect of moderators on the frequency of Salmonella in non-recycled water sources. Three sequential steps (identification of information sources, screening and eligibility) were performed to obtain a preliminary selection from identified abstracts and article titles. Questions related to the frequency of Salmonella in aquatic environments, as well as putative differences in the relative frequencies of the reported Salmonella serovars and the role of potential variable moderators (sample source, country, and sample volume) were formulated according to the population, intervention, comparison, and outcome method (PICO). The results were reported according to the Preferred Reporting Items for Systematic Review and Meta-Analyzes statement (PRISMA). A total of 26 eligible papers reporting 148 different Salmonella serovars were retrieved. According to our model, the Salmonella frequency in non-recycled water sources was 0.19 [CI: 0.14; 0.25]. The source of water was identified as the most import variable affecting the frequency of Salmonella, estimated as 0.31 and 0.17% for surface and groundwater, respectively. There was a higher frequency of Salmonella in countries with lower human development index (HDI). Small volume samples of surface water resulted in lower detectable Salmonella frequencies both in high and low HDI regions. Relative frequencies of the 148 serovars were significantly affected only by HDI and volume. Considering that serovars representation can also be affected by water sample volume, efforts toward the standardization of water samplings for monitoring purposes should be considered. Further approaches such as metagenomics could provide more comprehensive insights about the microbial ecology of fresh water and its importance for the quality and safety of agricultural products.
Salmonellosis is a cosmopolitan disease caused by Salmonella enterica, a major pathogen causing human foodborne illness worldwide (Majowicz et al., 2010; Hendriksen et al., 2011; European Food Safety Authority [EFSA], 2016; Tack et al., 2019). Salmonella species are estimated to cause 93.8 million cases of gastroenteritis worldwide annually, leading to 59,100 deaths (Majowicz et al., 2010; Roth et al., 2018). In the United States (USA), gastroenteritis caused by non-typhoidal Salmonella only was estimated to affect approximately one million people annually resulting in approximately US$ 3.7 billion medical costs (Majowicz et al., 2010; Batz et al., 2012). Salmonella was associated with 33% of the foodborne illness cases registered in 2018 in ten sites covering 15% of the USA population and has been cited as the second most prevalent foodborne pathogen, preceded only by Campylobacter spp. (Tack et al., 2019).
The microbiological condition of water used in agriculture, regardless of the source, is crucial for the safety of agri-food products. Salmonellosis outbreaks have been associated with the use of contaminated water in agricultural settings (Harris et al., 2003; Walsh et al., 2014; Liu et al., 2018). As the gastrointestinal tract of vertebrates is generally considered the natural habitat of Salmonella enterica, the use of recycled water from animal production systems is usually considered a major risk factor for produce contamination (Abulreesh, 2012). However, Salmonella occurrence in water sources might go beyond short-term accidental findings determined by the transient presence of bacteria as a result of scattered fecal contamination events. Viability mechanisms can enable Salmonella organisms to successfully survive in natural aquatic environments for several months (Domingo et al., 2000; Liu et al., 2018). In laboratory, however, Salmonella has been observed to survive for up to 5 years in phosphate-buffered solution at room temperature (Liao and Shollenberger, 2003).
Although Salmonella can survive in a wide range of pH (4.05–9.5) and temperature (7–48°C) under controlled laboratory conditions (Cox et al., 2014), the natural environment associated with irrigation water sources such as rivers or lakes may impose challenging conditions for the long-term viability of Salmonella. Variations in physicochemical properties (temperature, salts, pH, oxygen), nutrient availability, interaction with other microorganisms, and exposure to UV radiation (Wilkes et al., 2011; Wanjugi and Harwood, 2013) have been shown to reduce Salmonella viability in water over time, generally up to 30 days (Steele and Odumeru, 2004). On the other hand, the production biofilm can facilitate the survival of Salmonella in water and aquatic invertebrates, such as free-living protozoa and vertebrate hosts (Sha et al., 2011; Liu et al., 2018; Chen et al., 2019). Furthermore, re-introduction of Salmonella into irrigation ponds should be also considered, as previously demonstrated for Salmonella Newport (Li B. et al., 2015). Re-introduction events are usually caused by animal waste contamination through sewage discharges, rainfall, or associated surface run-off events. Therefore, natural or non-recycled water sources such as rivers and irrigation canals have been shown to act as reservoirs of viable Salmonella (Baudart et al., 2000; Li et al., 2014; Martínez et al., 2017) and play a critical role as contamination sources of Salmonella and other microbes to fresh produce (Hanning et al., 2009), circulating back to humans and other animals (Li B. et al., 2015).
Despite the increasing number of studies reporting the presence of Salmonella in natural aquatic environments, there is no agreement on its average frequency and relative serovar representation across water sources. Because of this knowledge gap and the great importance of water for the sustainability of food production worldwide, this meta-analysis aimed at determining the weighted average proportion and between-study variance of Salmonella frequency in non-recycled water environments and the role of putative moderators affecting both the frequency and relative representation of serovars.
Three sequential steps were performed by the authors in order to obtain a preliminary selection from identified abstracts and article titles: Identification of information sources, Screening and Eligibility. The selected articles were finally included in the study.
The identification of putative information sources was guided by questions that were formulated according to the population, intervention, comparison, and outcome method (PICO) (Santos et al., 2007). The following questions were asked: What is the occurrence of Salmonella in aquatic environments? Are there differences in the presence of Salmonella between surface and groundwater? Which serovars are most prevalent in surface water? Which serovars are most prevalent in groundwater? Which serovars are present in both surface and groundwater? Are there differences in frequency and diversity of Salmonella serovars among countries? Could differences in the frequency and diversity of Salmonella be attributed to sample volume? Are there differences in presence and abundance related to seasonality?
A literature search was performed using Medical Subject Headings (MeSH) terms on Pubmed, Web of Science, and Embase databases. The search components are described below. The initial screening process was performed from April to November 2020. Further directed searches were carried out by checking the reference lists of relevant articles.
Search component 1 (SC1)—population: Water OR groundwater OR lake OR pond OR river.
Search component 2 (SC2)—intervention: Salmonella spp. OR Salmonella enterica OR Salmonella*.
After retrieving the search components results, the Boolean operator “AND” was used to combine SC1 and SC2.
The research considered only papers in English published between 2015 and 2020 and duplicate articles were excluded. Editorials, letters, and Ph.D. thesis were also excluded. Based on the title and abstract contents, only articles presenting proper identification of the serovars isolated from surface or groundwater sources were selected.
The eligibility assessment was performed after the complete analysis of the entire manuscript. For serotyping characterization, publications using the standard Kauffmann-Le Minor scheme were first selected, but some articles using serotyping through pulsed-field gel electrophoresis (PFGE) were also included. The exclusion of publications using rapid methods of Salmonella detection was justified by two key reasons. Firstly, publication reporting serovar identification provides more information for biological interpretation and therefore fit better the purpose of our study, as these publications can be used to respond all the focus questions. For instance, the assessment of the frequencies of Salmonella serovars could be biased by the inclusion of articles using primers targeting a small group of serovars. Secondly, although some rapid tests could provide higher sensitivity values for Salmonella detection compared to conventional microbiological culture (Cox et al., 2014), the comparative analysis could be biased by the large methodological variation represented by the numerous available tests, including commercial and in-house methods. Therefore, the publications considered in the present study described microbiological isolation methods performed according to standard methodologies such as BAM and AOAC, although minor differences existed, mainly in terms of types of media. Importantly, as the large number of serovars usually requires the use of a combination of culture media (Cox et al., 2014), there is possibility of bias in the comparative analysis of the serovars across the different studies. Finally, the results were reported according to the Preferred Reporting Items for Systematic Review and Meta-Analyses Statement (PRISMA) (Moher et al., 2015).
Possible sources of bias included study inclusion/exclusion criteria and the impact of missing data, missing primary results, the chosen database, date, language, number of articles, and article type selected for this study.
Information regarding the identification of manuscripts (authors, publication year, country), total number of collected samples, number of positive samples, number of Salmonella serovars, sample source (superficial or ground water), and water sample volume were obtained and kept in excel spreadsheets. Frequencies of Salmonella serovars were calculated by dividing the number of positive samples of each serovar by the total number of collected samples. Spreadsheets containing the data used in this meta-analysis are available as Supplementary Material. Because of the existence of proportions outside the range of 0.2–0.8, the frequency values were logit-transformed before analysis. The summary effect size (i.e., the weighted average proportion) was obtained by fitting a random-effects model using the restricted maximum-likelihood estimator (RMLE), assuming there are within- and between-study variances across the studies. The estimates of summary proportions and their confidence intervals were visualized according to forest plot as proposed by Lewis and Clarke (2001). In order to assess the true variation in effect sizes (between-study variance), the study heterogeneity (τ2) was calculated and tested for significance according to Q-test at 95% probability. Heterogeneity was also quantified by I2 statistics as proposed by Higgins et al. (2003). When the effect sizes had high heterogeneity, we conducted a moderator analysis by means of meta-regression in order to investigate potential sources of systematic variation between the studies. Three potential moderators were investigated: (1) Sample source: surface water or groundwater; (2) Water sample volume: small (<999 mL) or large (≥1,000 mL); and (3) Sample origin: samples from countries with low (<0.8) or high (≥ 0.8) human development index (HDI), according to the latest Human Development Index Ranking (United Nations Development Programme [UNDP], 2020). We used China’s HDI for the reports from Taiwan. The analyses were performed using metafor package in R (Viechtbauer, 2010; RStudio Team, 2019; R Core Team, 2020). In addition, a decision tree was built by supervised machine learning using rpart package in R (Therneau et al., 2013), and the Pearson correlation coefficient between observed and estimated frequencies was calculated.
The influence of the moderators on the relative frequency of the reported Salmonella sorovars was verified with canonical correspondence analysis, using the vegan package in R (Oksanen et al., 2011). Diversity indexes Shannon and Pielou were also calculated for richness and evenness estimates, respectively.
The relative frequencies of serovars were submitted to cluster analysis of row and columns, based on binary distance and hierarchical clustering. Hierarchical clustering of the 26 articles condiering the relative frequencies of the serovars was built using average linkage was built from a binary distance matrix in R 4.11. The optimal number of clusters was defined according to the FOM (figure of merit) index using the clValid package in R (Brock et al., 2008). Heatmaps were obtained using the ComplexHeatmap package in R (Gu et al., 2016).
A total of 1,723 articles were identified at PubMed, 1,277 at Web of Science, and 2,194 at Embase, totaling 5,194 papers. Of these, 1,972 duplicates or triplicates were detected and excluded. A total of 3,222 remaining publications were obtained after the exclusion of redundant papers. Most articles (n = 101) were excluded for not informing the proper identification of serovars.
After titles and abstracts were read, 26 papers addressing both Salmonella and non-recycled environmental water were considered adequate and included in the present study (Figure 1). For statistical analysis, these 26 publications resulted in 29 observations as three papers reported Salmonella occurrence for both surface and groundwater.
Figure 1. PRISMA flow diagram showing the sequential steps for articles selection and inclusion in the meta-analysis.
A total of 148 different serovars were reported. Of these, 139 originated from surface water, from which 123 serovars were reported only in this water source. A total of 25 serovars were reported in groundwater, including nine serovars that were exclusively reported in this source. Only 16 serovars were reported in both sources.
The United States was identified as the country with the highest number of papers reporting the identification of Salmonella serovars (13), followed by Canada (3), and Taiwan (2). The remaining articles originated from Burkina Faso, China, Croatia, Ghana, Mexico, Spain, Sri Lanka, and Uganda.
Table 1 presents the relative frequency ranges of Salmonella serovars observed in non-recycled surface water and groundwater. Only serovars that were reported in at least five different studies were included in this table. Supplementary Tables 1, 2 provide detailed information about the occurrence of all reported Salmonella enterica serovars in the 26 publications.
Table 1. Relative representation of Salmonella enterica serovars associated with surface and groundwater sources that have been reported in peer-reviewed scientific publications addressing the occurrence of Salmonella in aquatic environments between the years 2015 and 2020 (Only serovars reported in at least five different studies are considered).
The frequency of Salmonella enterica in water samples varied from 1.14 (Stokdyk et al., 2020) to 100% (Maurer et al., 2015; Kovačić et al., 2017) as observed in Figure 2. The highest number of isolates reported in a single study (n = 247) was associated with surface water samples (Kadykalo et al., 2020), while the highest serovar diversity (35 different serovars) was observed by Jokinen et al. (2015).
Figure 2. Frequency of Salmonella enterica serovars detected in non-recycled surface water (A) and groundwater samples (B) as per reported in 26 peer reviewed scientific publications between the years 2015 and 2020.
Considering groundwater only (Table 1), the relative frequency of Salmonella enterica varied from 3.85 to 100%. The higher number of isolates for this type of water (n = 26) was reported by Dekker et al. (2015). Except for Kovačić et al. (2017), which reported the occurrence of Salmonella Enteritidis in a single sample (100%), the highest frequency of Salmonella and the greatest diversity of serovars were observed in a study conducted in Sri Lanka (Mahagamage et al., 2020). Importantly, although the study from Kovačić et al. (2017) refers to a single sample related to an outbreak investigation, no minimum sample size was predetermined as inclusion criteria for the present meta-analysis and therefore that study has been included in the present investigation.
The Forest plot showing the summary effect size of the Salmonella proportions in water is shown in Figure 3. According to our results, Salmonella frequency in non-recycled water sources was 0.19 [CI: 0.14; 0.25]. Although a significant (P < 0.0001) and high heterogeneity (τ2 = 0.0711; I2 = 99.72%) was observed, only source was identified as a significant mediator (P < 0.10) in the meta regression analysis. The descriptive average frequencies were 31.97 and 20.85% in surface water and groundwater samples, respectively, as shown in Supplementary Tables 1, 2.
Figure 3. Forest plot showing the summary effect size of proportions of Salmonella frequencies in non-recycled water sources using 26 selected articles and 29 observations. This summary effect size was obtained in R 4.11 (package metafor) by fitting a random-effects model using the restricted maximum-likelihood estimator (RMLE). Heterogeneity parameters and statistics are indicated in the model.
The decision tree (Figure 4) obtained by supervised machine learning resulted in a 0.48 Pearson correlation coefficient between observed and estimated frequencies. All three moderators (water source; HDI, and sample volume) were shown to affect Salmonella frequency in water but source was identified as the most relevant one. Estimate frequencies of 0.31 and 0.17% for surface and groundwater were obtained, respectively. Considering surface water only, samples from countries with lower HDI resulted in a higher Salmonella frequency (0.42) compared to developed regions (0.26). Sampling of small water volumes resulted in lower detectable Salmonella frequencies in both high and low HDI regions. The water sampling technique reported by the majority of the studies consisted of transporting determined volume of water to the laboratory for filtering. The use of less than 1 L water samples was reported by seventeen studies (65.38%), while other six studies (23.08%) described the use of 1–4 L water samples. Only two publications (7.69%) reported the use of in situ water filtration (10 L) by means of the modified Moore swab technique (MMS) (Allard et al., 2019; Callahan et al., 2019) and only a single study reported the use of in situ ultrafiltration (728 L) with commercial dialyzers (Stokdyk et al., 2020). Detailed information is shown in Supplementary Tables 1, 2.
Figure 4. Decision tree predicting the frequency of Salmonella in non-recycled water sources in function of the moderator variables source (surface or groundwater), human development index (HDI) of the country from which the samples originated (high or low) and water sample volume (< 1 L or ≥ 1 L). The predictive algorithm has been built in R (package rpart) using meta-analysis data of 26 peer reviewed scientific publications between the years 2015 and 2020.
According to the canonical correspondence analysis, both HDI and sample volume significantly (P < 0.0001) affected the relative frequencies of the 148 Salmonella serovars across the studies. The hierarchical clustering of the 26 publications considering the relative frequencies of the 148 Salmonella serovars is shown in Supplementary Figure 1. Five distinct clusters were observed. The larger cluster (2) is comprised only by studies from countries with high HDI and the majority of these studies reported the use of water samples with less than 1 L. On the other hand, all but one (16) study in the Cluster 1 originated from countries with low HDI. Two manuscripts (17 and 25) were not grouped in any cluster.
The heatmap representing the relative frequencies of Salmonella serovars according to the cluster analysis of rows (y) and columns (x) is shown in Figure 5. Cluster 1 (x) included the 135 less representative serovars, while cluster 5 (x) was comprised the most frequent serovars: S. Newport and S. Typhimurium, which were identified in 19 and 16 studies, respectively. Other frequent serovars were grouped in Clusters 2 (S. Barelly, S. Mbandaka, S. 4,[5],12:i:-, S. Braenderup), 3 (S. Rubislaw, S. Muenchen, S. Give, S. Hartford) and 4 (S. Kentucky, S. Stanleyville). Considering that the relative frequencies of the Salmonella serovars across studies are affected by HDI and volume, individualized heatmaps according to these moderators are shown in Figures 6, 7, respectively. The higher frequencies of some serovars such as S. Rubislaw, S. Muenchen, S. Give, S. Hartford, S. Rissen, S. Saintpaul, and S. Thompson across studies from high HDI countries is shown in Figure 6. According to Figure 7, some serovars were more frequently observed in studies using larger water samples, such as S. Newport, S. Typhimurium, S. Mbandaka, S. Braenderup, and S. Kentucky. On the other hand, S. Agona, S. Derby, and S. Virchow were more frequently observed in some studies using small volume water samples (8, 16, 19) than in studies using greater water volume samples.
Figure 5. Heatmap of the relative frequencies of Salmonella enterica serovars isolated from surface and groundwater sources as per reported in 26 peer-reviewed scientific publications between the years 2015 and 2020. The heatmap was built in R (package ComplexHeatmap).
Figure 6. Heatmap of the relative frequencies of Salmonella enterica serovars isolated from non-recycled water sources according to the human development index (HDI) of countries associated with 26 peer-reviewed scientific publications between the years 2015 and 2020. The heatmap was built in R (package ComplexHeatmap).
Figure 7. Heatmap of the relative frequencies of Salmonella enterica serovars isolated from non-recycled water sources according to the water sample volume used in 26 peer-reviewed scientific publications between the years 2015 and 2020. The heatmap was built in R (package ComplexHeatmap).
There were no differences (P > 0.05) in the diversity of Salmonella serovars across water sources, HDI and volume as measured by Shannon and Pielou indexes for richness and evenness, respectively.
The overall weighted average proportion was 0.19 [CI: 0.14; 0.25] for positive water samples, suggesting that viable Salmonella organisms are frequently found in non-recycled water sources worldwide. The increasing number of human salmonellosis outbreaks associated with the consumption of fresh produce or industrialized plant-based products, including fruits, vegetables, legumes, grains, nuts, and seeds, has posed the question whether environmental water could play a role as potential Salmonella contamination source. The 127 peer-reviewed studies retrieved after the initial screening test of this systematic review confirms the increasing interest of the scientific community on this topic. These publications reported the occurrence of Salmonella from different aquatic environments under a wide range of conditions (Domingo et al., 2000; Bell et al., 2015; Li B. et al., 2015; Liu et al., 2018). Obviously, the capacity of Salmonella to survive and to adapt to challenging environmental conditions is a basic principle for the bacteria to fulfill their biological cycle (fecal-oral route of transmission), suggesting the existence of mechanisms to overcome stressors in aquatic and terrestrial microcosms. Long-term persistence of S. enterica in irrigation ponds has been previously reported (Greene et al., 2008; Luo et al., 2015), indicating that this pathogen is able to adapt to stressors in hydrological niches and highlighting the importance of assessing the quality of irrigation water regularly (Winfield and Groisman, 2003). However, little is known about the real ability of Salmonella to adapt and evolve in natural environments such as surface and groundwater sources.
The amount of true heterogeneity observed in our study, represented by the between-study variance and expressed by the parameter (τ2), (Borenstein et al., 2005) was large and statistically significant, indicating the existence of systematic differences in effects across 26 articles used in the present meta-analysis. Interestingly, the I2 parameter varies from 0 to 100% and allows comparisons of the estimated heterogeneity across different meta-analysis studies. In the case of the present study, the observed I2 value was 99.72%. Therefore, there is evidence that the variance is determined by the existence of importance variable moderators. That said, we highlight the limited number of moderators (n = 3) investigated in the present meta-analysis that could act as sources of heterogeneity, as reflected by the lack of important information across all studies in the meta-analysis, for instance, the proximity of the sampled water of potential contamination sources (agriculture, livestock, sewage), psychochemical properties of water that might play an important role in the survival of Salmonella and competing organisms, the presence of other contaminants, characterization of wildlife in the proximity of the water bodies, among others.
The fact that the source of water was identified as a significant moderator for Salmonella frequency according to the meta regression analysis and was also identified as the most relevant moderator in the regression tree corroborates the hypothesis that viable Salmonella is more frequently recovered from surface water sources than groundwater. This finding was expected, considering the greater exposure of surface water sources to contaminants in general. Indeed, surface water is more easily exposed to discharge of sewage, inadequate agricultural, livestock and industrial run-offs, climatic events and visit of wild animals (Bergholz et al., 2016; Karkey et al., 2016; Toro et al., 2016; Gu et al., 2019; Jechalke et al., 2019). The latter is particularly noteworthy, since a wide range of S. enterica serovars have been extensively reported in wildlife (Maurer et al., 2015; Toro et al., 2016; de Souza et al., 2020).
The lower frequency of Salmonella in groundwater compared with surface water (0.17 vs.0.31, respectively) observed in our study corroborates previous findings (Abulreesh, 2012; Gu et al., 2019). Underground reservoirs have long been considered excellent sources of drinking water to human and animal populations, mainly because it is naturally filtered by the soil underlying rock formations. Although they usually provide superior microbial quality associated with lower microbial loads, the belief that groundwater is pure and no treatment is needed before consumption has been questioned (Li et al., 2018; Liu et al., 2018; Stokdyk et al., 2020) by the increasing number of studies reporting Salmonella contamination in groundwater (Dekker et al., 2015; Li X. et al., 2015; Palamuleni and Akoth, 2015). There are several possibilities of contamination of groundwater, even though some of them are sporadic. Quality can be compromised by insufficient well depth or during construction (Liu et al., 2018) and well pollution may result from events such as improperly functioning sewer systems, contaminated stormwater and agricultural run-off, especially after storms and floods (Gu et al., 2019). Contamination events can be sporadic or one-off, nevertheless, the water sources can become compromised for longer periods (Dekker et al., 2015). Some experimental studies showed that Salmonella can remain viable for periods longer than 100 days in water, and that viability is mainly affected by ambient temperature (Domingo et al., 2000; Ibrahim et al., 2019).
Importantly, there was a considerably higher number of studies on the occurrence of Salmonella in surface water (n = 23) compared with groundwater (n = 6). Possibly, the greater interest in assessing surface water is related to its relevance and economic importance for both rural and urban settings worldwide. In fact, the majority of reports in the present study originated from regions where surface water sources have been commonly used for irrigation purposes in agri-food production systems.
In summary, the occurrence of Salmonella in groundwater should not be neglected. Further studies addressing Salmonella contamination in groundwater are warranted as they could be particularly important in regions where irrigation practices depend on this type of water, such as semiarid settings.
Between 2015 and 2020, S. Newport was the most frequent serovar identified in both surface (464 isolates; 18.33%) and groundwater (20 isolates; 0.78%). Furthermore, Callahan et al. (2019) reported S. Newport as the most isolated serovar throughout the year. S. Newport infection rates have been stable over the decades, with approximately 750 confirmed cases per year in Europe (European Food Safety Authority [EFSA], 2016). Wild birds are considered important reservoirs as recurrent S. Newport outbreaks have been reported due to direct contamination of vegetables such as tomatoes, soil or irrigation water (Bell et al., 2015). The factors causing variations in S. Newport rates in the United States remain unknown (Crim et al., 2018).
Salmonella Typhimurium was the second most frequent serovar contaminating both surface water (9.56%) and groundwater (0.63%). This serovar has been one of the two leading serovars associated with human salmonellosis since 1990 (Herikstad et al., 2002). The persistence of this pathogen in freshwater microcosms has been associated with the expression of the hilA gene, a regulatory system for the expression of invasive Salmonella phenotypes, including the expression of the sspC, invF, and orgA invasion genes (Nutt et al., 2003). Therefore, it is possible that strains circulating in environmental water sources could present increased virulence.
S. Thompson, also a frequent serovar, has been associated with sporadic salmonellosis outbreaks every year in different countries (Friesema et al., 2012; Gaulin et al., 2017; Suijkerbuijk et al., 2017; Eun et al., 2019). Under laboratory conditions, a 3 ppm chlorine water treatment induced the viable but not cultivable state in S. Thompson (Highmore et al., 2018), raising concerns about the efficacy of chlorine-based treatment of water for human consumption. Therefore, S. Thompson may be a potential pathogen of treated water for human consumption.
S. Javiana, S. Kentucky, and S. Rubislaw serovars have been also identified as frequent serovars contaminating non-recycled water sources. These serovars have been shown to play a role in human salmonellosis. The number of cases of S. Javiana has been dramatically increasing in the USA in the last decades (Centers for Disease Control and Prevention [CDC], 2013). It is worth noting that drinking water has been reported as an important source of human infection by S. Javiana (Clarkson et al., 2010; Mukherjee et al., 2019). S. Kentucky is involved in approximately 100 cases of human salmonellosis yearly in the United States (Centers for Disease Control and Prevention [CDC], 2016). Although it is not one of the leading serovars causing human salmonellosis, there is increasing concern with the emergence of multidrug resistance particularly associated with this serovar (Milton et al., 2018; Al-Gallas et al., 2021a,b). On the other hand, S. Rubislaw has been mainly detected in environmental samples (Maurer et al., 2015), and various free-living animals (Potter et al., 2011; Rush et al., 2020; Hernandez et al., 2021).
According to our findings, there are indications that the origin of samples, as determined by the HDI index related to the country of origin, might contribute to both overall isolation frequency and relative distribution of Salmonella serovars. Based on the decision tree (Figure 4), the frequencies of Salmonella-positive samples in surface water were higher in countries with low HDI compared with countries with higher HDI (0.42 vs. 0.26, respectively). This finding could be explained by contamination events that are probably more frequent in developing regions as a result of improper sewage treatment and disposal. However, the opposite was observed for groundwater samples and frequency estimates were 0.069 and 0.26 for low and high HDI, respectively.
Further investigations should be conducted to address the differences in the relative frequencies of serovars between high and low HDI countries. Some serovars such as S. Muenchen, S. Give, S. Hartford, S. Rissen, S. Saintpaul, S. Rubislaw, and S. Thompson were highly frequent across studies from high HDI countries while others (S. Agona, S. Derby, S. Anatum) were more frequently observed in studies from low HDI countries. It is plausible to admit that the relative serovar frequencies across the regions depend on natural, social and economical drivers impacting the epidemiological and evolutionary aspects of Salmonella enterica, and therefore very difficult to be predicted.
Although meta-analysis indicated Salmonella Agona as a frequent serovar present in water samples from the low HDI countries included in our study, it is among the ten leading serovars associated with human salmonellosis in European countries, with 378–582 cases per year (Popa and Popa, 2021). Outbreaks of non-typhoidal salmonellosis associated with this serovar has been linked to fresh food consumption (Estrada-Acosta et al., 2014; Hassan et al., 2019; Ehuwa et al., 2021), such as papaya (Hassan et al., 2019) and tomato (Estrada-Acosta et al., 2014). Moreover, irrigation water is considered a major contamination source in agricultural settings (Estrada-Acosta et al., 2014). However, salmonellosis cases attributed to S. Agona have also been attributed to the consumption of contaminated processed foods such as peanut butter and infant formulae (Ehuwa et al., 2021).
Interestingly, water sample volume was shown to significantly affect the relative frequency of Salmonella serovars across the different studies. According to the decision tree (Figure 4), higher frequency of Salmonella was seen in larger water samples (≥ 1 L) from both high and low HDI countries. In high HDI countries, the frequencies were 0.31 vs. 0.14, while in low HDI countries, a greater difference was observed (0.34 vs. 0.6). Although water sample volume has been referred as critical factor for the recovery of Salmonella enterica from water, there are no previous reports directly assessing the role of water sample volume on Salmonella isolation frequency. This meta-analysis study suggests that water volume might play an important role on the recovery of viable Salmonella serovars in environmental water. Moreover, the relative frequency distribution findings reported in our study and visualized as a heatmap (Figure 7) indicate a higher recovery frequency of public health relevant Salmonella serovars when large water samples are used (≥ 1 L), such as S. Typhimuiurm, S. Newport, and S. Enteritidis.
Important aspects indicate that the occurrence of S. enterica in natural water sources is underestimated. Firstly, a considerable number of the studies in our investigation (27.58%) reported using small-volume samples (<1 L), which may compromise the microbiological recovery. Although there is a consensus toward the use of larger water samples to detect microorganisms present in low densities (Bisha et al., 2011; McEgan et al., 2013; Sbodio et al., 2013), there is a lack of studies comparing the real effect of water volume on the recovery of Salmonella serovars. Furthermore, conventional microbiological isolation is limited in terms of detection of viable but non-culturable bacteria (VBNC), i.e., organisms presenting a very low metabolic rate or state of dormancy (Lin et al., 2016). Problems in VBNC Salmonella cultivation and identification have been well documented (Oliver, 2005; Morishige et al., 2017). This condition might be of particular importance for Salmonella organisms in natural water environments, as bacteria may be subjected to many stressors.
Considering how the number of viable organisms might affect the accuracy of the conventional culture method, alternative techniques have been proposed to overcome cost and logistic problems associated with the transport of large volumes of water to laboratories. Among these, the modified Moore swab (MMS) stands out as a high efficient and low operating cost method alternative for in situ filtration of large sample volumes (usually 10 liters or more) (Sbodio et al., 2013; Sharma et al., 2020; Sikorski and Levine, 2020).
Due to the very limited number of publications with serovar identification covering long periods of time, no statistical analysis was performed to assess the relationship between S. enterica frequency and season or climatic condition. Seventeen of the twenty-six articles reported isolation of S. enterica from all or most of the samples collected during the entire experimental period. There is no substantial variation regarding the frequency of serovars throughout the different seasons of the year (Bell et al., 2015; Dekker et al., 2015; Jokinen et al., 2015; Maurer et al., 2015; Traoré et al., 2015; Afema et al., 2016; Bergholz et al., 2016; Falardeau et al., 2017; Topalcengiz et al., 2017; Harris et al., 2018; Ho et al., 2018; Santiago et al., 2018; Truitt et al., 2018; Callahan et al., 2019; Gu et al., 2019; Díaz-Torres et al., 2020; Stokdyk et al., 2020).
Four articles performed a single sampling per site (Hsu et al., 2015; Li B. et al., 2015; Kovačić et al., 2017; Allard et al., 2019). Despite having made multiple samplings from the same sites over time, one study still analyzed the data as a single set, because it focused on reporting the incidence of antibiotic resistance in the isolated strains and did not assess the variation of isolates over time (Kadykalo et al., 2020).
Two studies carried out in Colorado and Georgia (United States) showed higher isolation rates in different seasons, spring and autumn, respectively (Antaki et al., 2016; Ahlstrom et al., 2018). One study showed higher frequency in the rainy season, between spring and early summer (Song et al., 2018). Interestingly, Mahagamage et al. (2020) reported increased frequencies of S. enterica isolation from surface water in rainy seasons, while the contrary was observed for groundwater. Overall, the relationship between Salmonella isolation frequency and seasons of the year or dry or rainy period seems to depend on several local variables. Factors such as average temperature, predominant type of exploitation in the region (agriculture, livestock or industry), availability of water (scarcity or abundance, regardless of the season), type of source and location of the source (level of preservation or urbanization of the surroundings) seem to have a strong influence on water contamination levels throughout the year.
To better assess these relationships, it is necessary to include further studies on the effects of climatic factors over long periods of time.
In summary, this meta-analysis investigation established the expected frequency of Salmonella recovery from water samples. There is a higher recovery rate from surface water compared with ground water. The serovar representation across those samples can be affected by the investigated region and collected water sample volume, mainly for those serovars that are relevant in public health. Further conclusions about other putative important moderators were not possible because of the lack of information in the accessed studies. In this sense, we encourage longitudinal study designs and thorough serotyping that enable conclusions on seasonal variations or the effects of factors such as physicochemical parameters of water and special-temporal information. Furthermore, high throughput approaches such as metagenomics could provide invaluable information about complex relationships between Salmonella and other biotic factors. Given the importance of water quality for agri-food systems and the public health importance of Salmonella, it is extremely important to better understand this dynamics, so that more effective strategies to control and mitigate salmonellosis can be envisioned and designed.
The raw data supporting the conclusions of this article will be made available by the authors, without undue reservation.
AR: literature search, data analysis, and manuscript writing. RF: conceptualization, literature search, data analysis, and manuscript writing. WP: data analysis and manuscript revision. LL: data analysis and manuscript writing. PG, AM-S, MT, and ES: manuscript writing and revision. JM: conceptualization and manuscript revision. CO: conceptualization, manuscript writing, and manuscript revision. All authors contributed to the article and approved the submitted version.
This study was funded by Conselho Nacional de Pesquisa e Desenvolvimento (CNPq, proc. 140910/2020-4) and Coordenação de Aperfeiçoamento de Pessoal de Nível Superior-Brasil (CAPES), finance code 001. CO holds a Research Productivity Fellowship (Level 1-D) granted by the Brazilian National Council for Scientific and Technological Development (CNPq, proc 313678/2020-0). This study was also supported by the Food and Drug Administration (FDA) of the U.S. Department of Health and Human Services (HHS) as part of an award received from the Joint Institute for Food Safety and Applied Nutrition (JIFSAN), University of Maryland. The contents are those of the authors and do not necessarily represent the official views of, nor an endorsement, by FDA, HHS, or the U.S. Government. For more information, please visit FDA.gov.
The authors declare that the research was conducted in the absence of any commercial or financial relationships that could be construed as a potential conflict of interest.
All claims expressed in this article are solely those of the authors and do not necessarily represent those of their affiliated organizations, or those of the publisher, the editors and the reviewers. Any product that may be evaluated in this article, or claim that may be made by its manufacturer, is not guaranteed or endorsed by the publisher.
We thank Conselho Nacional de Pesquisa e Desenvolvimento (CNPq) for providing scholarships to AR, LL, and CO.
The Supplementary Material for this article can be found online at: https://www.frontiersin.org/articles/10.3389/fmicb.2022.802625/full#supplementary-material
Supplementary Figure 1 | Hierarchical cluster analysis of 29 peer reviewed scientific publications between the years 2015 and 2020 considering the relative frequencies of reported Salmonella serovars. Hierarchical clustering using average linkage was built from a binary distance matrix in R 4.11.
Abulreesh, H. H. (2012). “Salmonellae in the Environment,” in Salmonella - Distribution, Adaptation, Control Measures and Molecular Technologies, eds B. Annous and J. B. Gurtler (London: InTech), 765–785. doi: 10.5772/28201
Afema, J. A., Byarugaba, D. K., Shah, D. H., Atukwase, E., Nambi, M., and Sischo, W. M. (2016). Potential Sources and Transmission of Salmonella and Antimicrobial Resistance in Kampala, Uganda. PLoS One 11:e0152130. doi: 10.1371/journal.pone.0152130
Ahlstrom, C. A., Manuel, C. S., Den Bakker, H. C., Wiedmann, M., and Nightingale, K. K. (2018). Molecular ecology of Listeria spp., Salmonella, Escherichia coli O157:H7 and non-O157 Shiga toxin-producing E. coli in pristine natural environments in Northern Colorado. J. Appl. Microbiol. 124, 511–521.
Al-Gallas, N., Khadraoui, N., Hotzel, H., Tomaso, H., El-Adawy, H., Neubauer, H., et al. (2021a). Quinolone resistance among Salmonella Kentucky and Typhimurium isolates in Tunisia: first report of Salmonella Typhimurium ST34 in Africa and qnrB19 in Tunisia. J. Appl. Microbiol. 130, 807–818. doi: 10.1111/jam.14822
Allard, S. M., Callahan, M. T., Bui, A., Ferelli, A. M. C., Chopyk, J., Chattopadhyay, S., et al. (2019). Creek to Table: tracking fecal indicator bacteria, bacterial pathogens, and total bacterial communities from irrigation water to kale and radish crops. Sci. Total Environ. 666, 461–471. doi: 10.1016/j.scitotenv.2019.02.179
Al-Gallas, N., Belghouthi, K., Barratt, N. A., Ghedira, K., Hotzel, H., Tomaso, H., et al. (2021b). Identification and characterization of multidrug-resistant ESBL-producing Salmonella enterica serovars Kentucky and Typhimurium isolated in Tunisia CTX-M-61/TEM-34, a novel cefotaxime-hydrolysing β−lactamase of Salmonella. J. Appl. Microbiol. 132, 279–289. doi: 10.1111/jam.15211
Antaki, E. M., Vellidis, G., Harris, C., Aminabadi, P., Levy, K., and Jay-Russell, M. T. (2016). Low Concentration of Salmonella enterica and Generic Escherichia coli in Farm Ponds and Irrigation Distribution Systems Used for Mixed Produce Production in Southern Georgia. Foodborne Pathog. Dis. 13, 551–558. doi: 10.1089/fpd.2016.2117
Batz, M. B., Hoffmann, S., and Morris, J. G. (2012). Ranking the disease burden of 14 pathogens in food sources in the united states using attribution data from outbreak investigations and expert elicitation. J. Food Prot. 75, 1278–1291. doi: 10.4315/0362-028X.JFP-11-418
Baudart, J., Grabulos, J., Barusseau, J. -P., and Lebaron, P. (2000). Salmonella spp. and Fecal Coliform Loads in Coastal Waters from a Point vs. Nonpoint Source of Pollution. J. Environ. Qual. 29, 241–250. doi: 10.2134/jeq2000.00472425002900010031x
Bell, R. L., Zheng, J., Burrows, E., Allard, S., Wang, C. Y., Keys, C. E., et al. (2015). Ecological prevalence, genetic diversity, and epidemiological aspects of Salmonella isolated from tomato agricultural regions of the Virginia Eastern Shore. Front. Microbiol. 6:415. doi: 10.3389/fmicb.2015.00415
Bergholz, P. W., Strawn, L. K., Ryan, G. T., Warchocki, S., and Wiedmann, M. (2016). Spatiotemporal Analysis of Microbiological Contamination in New York State Produce Fields following Extensive Flooding from Hurricane Irene, August 2011. J. Food Prot. 79, 384–391. doi: 10.4315/0362-028X.JFP-15-334
Bisha, B., Perez-Mendez, A., Danyluk, M. D., and Goodridge, L. D. (2011). Evaluation of Modified Moore swabs and continuous flow centrifugation for concentration of Salmonella and Escherichia coli O157:H7 from large volumes of water. J. Food Prot. 74, 1934–1937. doi: 10.4315/0362-028X.JFP-11-133
Borenstein, M., Hedges, L., Higgins, J., and Rothstein, H. (2005). Comprehensive Meta-Analysis Version 2. Englewood, NJ: Biostat. Available online at: https://www.meta-analysis.com/downloads/Meta-Analysis-Manual.pdf (accessed March 23, 2022).
Brock, G., Pihur, V., Datta, S., and Datta, S. (2008). clValid?: an R package for cluster validation. J. Stat. Softw. 25, 1–22. doi: 10.18637/jss.v025.i04
Callahan, M. T., Van Kessel, J. A., and Micallef, S. A. (2019). Salmonella enterica recovery from river waters of the Maryland Eastern Shore reveals high serotype diversity and some multidrug resistance. Environ. Res. 168, 7–13. doi: 10.1016/j.envres.2018.09.012
Centers for Disease Control and Prevention [CDC] (2013). An Atlas of Salmonella in the United States, 1968-2011. Atlanta, GA: US Department of Health and Human Services, 1–248.
Centers for Disease Control and Prevention [CDC] (2016). National Salmonella Surveillance Annual Report, 2013. Atlanta, GA: Centers for Disease Control and Prevention, 1–89.
Chen, J., Shi, Y., Cheng, D., Jin, Y., Hutchins, W., and Liu, J. (2019). Survey of pathogenic bacteria of biofilms in a metropolitan drinking water distribution system. FEMS Microbiol. Lett. 366:fnz225. doi: 10.1093/femsle/fnz225
Clarkson, L. S., Tobin-D’Angelo, M., Shuler, C., Hanna, S., Benson, J., and Voetsch, A. C. (2010). Sporadic Salmonella enterica serotype Javiana infections in Georgia and Tennessee: a hypothesis-generating study. Epidemiol. Infect. 138, 340–346. doi: 10.1017/S0950268809990586
Cox, J., Pavic, A., Wang, H., Hammack, T., Dwivedi, H., Devulder, G., et al. (2014). “Salmonella,” in Encyclopedia of Food Microbiology, eds C. A. BATT and M. Lou Tortorello (Amsterdam: Academic Press, Elsevier Ltd), 3248.
Crim, S. M., Chai, S. J., Karp, B. E., Judd, M. C., Reynolds, J., Swanson, K. C., et al. (2018). Salmonella enterica serotype newport infections in the United States, 2004-2013: increased incidence investigated through four surveillance systems. Foodborne Pathog. Dis. 15, 612–620. doi: 10.1089/fpd.2018.2450
de Souza, M. L., Coelho, M. L., da Silva, A. O., da Silva Azuaga, L. B., Macedo Coutinho Netto, C. R., Galhardo, J. A., et al. (2020). Salmonella spp. Infection in psittacidae at a wildlife rehabilitation center in the state of Mato Grosso do Sul, Brazil. J. Wildl. Dis. 56, 288–293. doi: 10.7589/2019-06-171
Dekker, D. M., Krumkamp, R., Sarpong, N., Frickmann, H., Boahen, K. G., Frimpong, M., et al. (2015). Drinking water from dug wells in rural ghana–Salmonella contamination, environmental factors, and genotypes. Int. J. Environ. Res. Public Health 12, 3535–3546. doi: 10.3390/ijerph120403535
Díaz-Torres, O., Lugo-Melchor, O. Y., de Anda, J., Gradilla-Hernández, M. S., Amézquita-López, B. A., and Meza-Rodríguez, D. (2020). Prevalence, distribution, and diversity of Salmonella strains isolated from a subtropical lake. Front. Microbiol. 11:521146. doi: 10.3389/fmicb.2020.521146
Domingo, J. W. S., Harmon, S., and Bennett, J. (2000). Survival of Salmonella species in river water. Curr. Microbiol. 40, 409–417. doi: 10.1007/s002840010079
Ehuwa, O., Jaiswal, A. K., and Jaiswal, S. (2021). Salmonella, Food Safety and Food Handling Practices. Foods 10:907. doi: 10.3390/foods10050907
Estrada-Acosta, M., Jiménez, M., Chaidez, C., León-Félix, J., and Castro-del Campo, N. (2014). Irrigation water quality and the benefits of implementing good agricultural practices during tomato (Lycopersicum esculentum) production. Environ. Monit. Assess. 186, 4323–4330. doi: 10.1007/s10661-014-3701-1
Eun, Y., Jeong, H., Kim, S., Park, W., Ahn, B., Kim, D., et al. (2019). A large outbreak of Salmonella enterica serovar Thompson infections associated with chocolate cake in Busan, Korea. Epidemiol. Health 41:e2019002. doi: 10.4178/epih.e2019002
European Food Safety Authority [EFSA] (2016). The European Union summary report on trends and sources of zoonoses, zoonotic agents and food-borne outbreaks in 2015. EFSA J. 14:4634. doi: 10.2903/j.efsa.2016.4634
Falardeau, J., Johnson, R. P., Pagotto, F., and Wang, S. (2017). Occurrence, characterization, and potential predictors of verotoxigenic Escherichia coli, Listeria monocytogenes, and Salmonella in surface water used for produce irrigation in the Lower Mainland of British Columbia, Canada. PLoS One 12:e0185437. doi: 10.1371/journal.pone.0185437
Friesema, I. H., de Jong, A. E., Fitz James, I. A., Heck, M. E., van den Kerkhof, J. H., Notermans, D. W., et al. (2012). Outbreak of Salmonella Thompson in the Netherlands since July 2012. Euro Surveill. 17:20303. doi: 10.2807/ese.17.43.20303-en
Gaulin, C., Fiset, M., Duchesne, C., Ramsay, D., Savard, N., Urbanek, A., et al. (2017). Salmonella Thompson outbreak associated with consumption of chicken shawarma and the usefulness of genome sequencing in the investigation. Can. Commun. Dis. Rep. 43, 186–192. doi: 10.14745/ccdr.v43i09a05
Greene, S. K., Daly, E. R., Talbot, E. A., Demma, L. J., Holzbauer, S., Patel, N. J., et al. (2008). Recurrent multistate outbreak of Salmonella Newport associated with tomatoes from contaminated fields, 2005. Epidemiol. Infect. 136, 157–165. doi: 10.1017/S095026880700859X
Gu, G., Strawn, L. K., Zheng, J., Reed, E. A., and Rideout, S. L. (2019). Diversity and dynamics of Salmonella enterica in water sources, poultry litters, and field soils amended with poultry litter in a major agricultural area of Virginia. Front. Microbiol. 10:2868. doi: 10.3389/fmicb.2019.02868
Gu, Z., Eils, R., and Schlesner, M. (2016). Complex heatmaps reveal patterns and correlations in multidimensional genomic data. Bioinformatics 32, 2847–2849. doi: 10.1093/bioinformatics/btw313
Hanning, I. B., Nutt, J. D., and Ricke, S. C. (2009). Salmonellosis outbreaks in the united states due to fresh produce: sources and potential intervention measures. Foodborne Pathog. Dis. 6, 635–648. doi: 10.1089/fpd.2008.0232
Harris, C. S., Tertuliano, M., Rajeev, S., Vellidis, G., and Levy, K. (2018). Impact of storm runoff on Salmonella and Escherichia coli prevalence in irrigation ponds of fresh produce farms in southern Georgia. J. Appl. Microbiol. 124, 910–921. doi: 10.1111/jam.13689
Harris, L. J., Farber, J. N., Beuchat, L. R., Parish, M. E., Suslow, T. V., Garrett, E. H., et al. (2003). Outbreaks associated with fresh produce: incidence, growth, and survival of pathogens in fresh and fresh-cut produce. Compr. Rev. Food Sci. Food Saf. 2, 78–141. doi: 10.1111/j.1541-4337.2003.tb00031.x
Hassan, R., Whitney, B., Williams, D. L., Holloman, K., Grady, D., Thomas, D., et al. (2019). Multistate outbreaks of Salmonella infections linked to imported Maradol papayas - United States, December 2016-September 2017. Epidemiol. Infect. 147:e265. doi: 10.1017/S0950268819001547
Hendriksen, R. S., Vieira, A. R., Karlsmose, S., Lo Fo Wong, D. M. A., Jensen, A. B., Wegener, H. C., et al. (2011). Global Monitoring of Salmonella serovar distribution from the World Health Organization global foodborne infections network country data bank: results of quality assured laboratories from 2001 to 2007. Foodborne Pathog. Dis. 8, 887–900. doi: 10.1089/fpd.2010.0787
Herikstad, H., Motarjemi, Y., and Tauxe, R. V. (2002). Salmonella surveillance: a global survey of public health serotyping. Epidemiol. Infect. 129, 1–8. doi: 10.1017/s0950268802006842
Hernandez, S. M., Maurer, J. J., Yabsley, M. J., Peters, V. E., Presotto, A., Murray, M. H., et al. (2021). Free-living aquatic turtles as sentinels of Salmonella spp. for water bodies. Front. Vet. Sci. 8:674973. doi: 10.3389/fvets.2021.674973
Higgins, J. P. T., Thompson, S.G., Deeks, J. J., and Altman, D. G. (2003). Measuring inconsistency in meta-analyses. BMJ 327, 557–560. doi: 10.1136/bmj.327.7414.557
Highmore, C. J., Warner, J. C., Rothwell, S. D., Wilks, S. A., and Keevil, C. W. (2018). Viable-but-Nonculturable Listeria monocytogenes and Salmonella enterica Serovar Thompson Induced by Chlorine Stress Remain Infectious. mBio 9, 1–12. doi: 10.1128/mBio.00540-18
Ho, Y.-N., Tsai, H.-C., Hsu, B.-M., and Chiou, C.-S. (2018). The association of Salmonella enterica from aquatic environmental and clinical samples in Taiwan. Sci. Total Environ. 624, 106–113. doi: 10.1016/j.scitotenv.2017.12.122
Hsu, C.-Y., Hsu, B.-M., Ji, W.-T., Chen, J.-S., Hsu, T.-K., Ji, D.-D., et al. (2015). Antibiotic resistance pattern and gene expression of non-typhoid Salmonella in riversheds. Environ. Sci. Pollut. Res. Int. 22, 7843–7850. doi: 10.1007/s11356-014-4033-y
Ibrahim, E. M. M. E. M., El-Liethy, M. A., Abia, A. L. K., Hemdan, B. A., and Shaheen, M. N. (2019). Survival of E. coli O157:H7, Salmonella Typhimurium, HAdV2 and MNV-1 in river water under dark conditions and varying storage temperatures. Sci. Total Environ. 648, 1297–1304. doi: 10.1016/j.scitotenv.2018.08.275
Jechalke, S., Schierstaedt, J., Becker, M., Flemer, B., Grosch, R., Smalla, K., et al. (2019). Salmonella establishment in agricultural soil and colonization of crop plants depend on soil type and plant species. Front. Microbiol. 10:967. doi: 10.3389/fmicb.2019.00967
Jokinen, C. C., Koot, J., Cole, L., Desruisseau, A., Edge, T. A., Khan, I. U. H. H., et al. (2015). The distribution of Salmonella enterica serovars and subtypes insurface water from five agricultural regions across Canada. Water Res. 76, 120–131. doi: 10.1016/j.watres.2015.02.038
Kadykalo, S., Thomas, J., Parmley, E. J., Pintar, K., and Fleury, M. (2020). Antimicrobial resistance of Salmonella and generic Escherichia coli isolated from surface water samples used for recreation and a source of drinking water in southwestern Ontario, Canada. Zoonoses Public Health 67, 566–575. doi: 10.1111/zph.12720
Karkey, A., Jombart, T., Walker, A. W., Thompson, C. N., Torres, A., Dongol, S., et al. (2016). The ecological dynamics of fecal contamination and Salmonella Typhi and Salmonella Paratyphi A in Municipal Kathmandu Drinking Water. PLoS Negl. Trop. Dis. 10:e0004346. doi: 10.1371/journal.pntd.0004346
Kovačić, A., and Huljev, Ž, and Sušić, E. (2017). Ground water as the source of an outbreak of Salmonella Enteritidis. J. Epidemiol. Glob. Health 7, 181–184. doi: 10.1016/j.jegh.2017.05.001
Lewis, S., and Clarke, M. (2001). Forest plots: trying to see the wood and the trees. BMJ 322, 1479–1480. doi: 10.1136/bmj.322.7300.1479
Li, B., Jackson, S. A., Gangiredla, J., Wang, W., Liu, H., Tall, B. D., et al. (2015). Genomic evidence reveals numerous Salmonella enterica serovar Newport reintroduction events in Suwannee watershed irrigation ponds. Appl. Environ. Microbiol. 81, 8243–8253. doi: 10.1128/AEM.02179-15
Li, X., Atwill, E. R., Antaki, E., Applegate, O., Bergamaschi, B., Bond, R. F., et al. (2015). Fecal Indicator and Pathogenic Bacteria and Their Antibiotic Resistance in Alluvial Groundwater of an Irrigated Agricultural Region with Dairies. J. Environ. Qual. 44, 1435–1447. doi: 10.2134/jeq2015.03.0139
Li, B., Vellidis, G., Liu, H., Jay-Russell, M., Zhao, S., Hu, Z., et al. (2014). Diversity and antimicrobial resistance of Salmonella enterica isolates from surface water in southeastern United States. Appl. Environ. Microbiol. 80, 6355–6365. doi: 10.1128/AEM.02063-14
Li, J., Ding, M., Han, Z., and Ma, J. (2018). Persistence of Salmonella Typhimurium in Well Waters from a Rural Area of Changchun City, China. Int. J. Environ. Res. Public Health 15:1090. doi: 10.3390/ijerph15061090
Liao, C. H., and Shollenberger, L. M. (2003). Survivability and long-term preservation of bacteria in water and in phosphate-buffered saline. Lett. Appl. Microbiol. 37, 45–50. doi: 10.1046/j.1472-765X.2003.01345.x
Lin, Y. W., Li, D., Gu, A. Z., Zeng, S. Y., and He, M. (2016). Bacterial regrowth in water reclamation and distribution systems revealed by viable bacterial detection assays. Chemosphere 144, 2165–2174. doi: 10.1016/j.chemosphere.2015.10.071
Liu, H., Whitehouse, C. A., and Li, B. (2018). Presence and persistence of Salmonella in water: the impact on microbial quality of water and food safety. Front. Public Health 6:159. doi: 10.3389/fpubh.2018.00159
Luo, Z., Gu, G., Ginn, A., Giurcanu, M. C., Adams, P., Vellidis, G., et al. (2015). Distribution and Characterization of Salmonella enterica Isolates from Irrigation Ponds in the Southeastern United States. Appl. Environ. Microbiol. 81, 4376–4387. doi: 10.1128/AEM.04086-14
Mahagamage, M. G. Y. L., Pathirage, M. V. S. C., and Manage, P. M. (2020). Contamination Status of Salmonella spp., Shigella spp. and Campylobacter spp. in Surface and Groundwater of the Kelani River Basin, Sri Lanka. Water 12:2187. doi: 10.3390/w12082187
Majowicz, S. E., Musto, J., Scallan, E., Angulo, F. J., Kirk, M., O’Brien, S. J., et al. (2010). The global burden of nontyphoidal Salmonella gastroenteritis. Clin. Infect. Dis. 50, 882–889. doi: 10.1086/650733
Martínez, M. C., Retamal, P., Rojas-Aedo, J. F., Fernández, J., Fernández, A., and Lapierre, L. (2017). Multidrug-Resistant Outbreak-Associated Salmonella Strains in Irrigation Water from the Metropolitan Region, Chile. Zoonoses Public Health 64, 299–304. doi: 10.1111/zph.12311
Maurer, J. J., Martin, G., Hernandez, S., Cheng, Y., Gerner-Smidt, P., Hise, K. B., et al. (2015). Diversity and Persistence of Salmonella enterica Strains in Rural Landscapes in the Southeastern United States. PLoS One 10:e0128937. doi: 10.1371/journal.pone.0128937
McEgan, R., Rodrigues, C. A. P., Sbodio, A., Suslow, T. V., Goodridge, L. D., and Danyluk, M. D. (2013). Detection of Salmonella spp. from large volumes of water by modified Moore swabs and tangential flow filtration. Lett. Appl. Microbiol. 56, 88–94. doi: 10.1111/lam.12016
Milton, A. A. P., Agarwal, R. K., Priya, G. B., Athira, C. K., Saminathan, M., Reddy, A., et al. (2018). Occurrence, antimicrobial susceptibility patterns and genotypic relatedness of Salmonella spp. isolates from captive wildlife, their caretakers, feed and water in India. Epidemiol. Infect. 146, 1543–1549. doi: 10.1017/S0950268818001553
Moher, D., Shamseer, L., Clarke, M., Ghersi, D., Liberati, A., Petticrew, M., et al. (2015). Preferred reporting items for systematic review and meta-analysis protocols (PRISMA-P) 2015 statement. Syst. Rev. 4:1. doi: 10.1186/2046-4053-4-1
Morishige, Y., Koike, A., Tamura-Ueyama, A., and Amano, F. (2017). Induction of Viable but Nonculturable Salmonella in Exponentially Grown Cells by Exposure to a Low-Humidity Environment and Their Resuscitation by Catalase. J. Food Prot. 80, 288–294. doi: 10.4315/0362-028X.JFP-16-183
Mukherjee, N., Nolan, V. G., Dunn, J. R., and Banerjee, P. (2019). Sources of human infection by Salmonella enterica serotype Javiana: a systematic review. PLoS One 14:e0222108. doi: 10.1371/journal.pone.0222108
Nutt, J. D., Pillai, S. D., Woodward, C. L., Sternes, K. L., Zabala-Díaz, I. B., Kwon, Y. M., et al. (2003). Use of a Salmonella typhimurium hilA fusion strain to assess effects of environmental fresh water sources on virulence gene expression. Water Res. 37, 3319–3326. doi: 10.1016/S0043-1354(03)00244-6
Oksanen, J., Blanchet, F. G., Kindt, R., Legendre, P., Minchin, P., Simpson, G., et al. (2011). Reference Manual for Package “vegan”. Vienna: R Foundation for Statistical Computing.
Palamuleni, L., and Akoth, M. (2015). Physico-chemical and microbial analysis of selected borehole water in Mahikeng, South Africa. Int. J. Environ. Res. Public Health 12, 8619–8630. doi: 10.3390/ijerph120808619
Popa, G. L., and Popa, M. I. (2021). Salmonella spp. infection – a continuous threat worldwide. Germs 11, 88–96. doi: 10.18683/germs.2021.1244
Potter, A. S., Reid, S. A., and Fenwick, S. G. (2011). Prevalence of Salmonella in fecal samples of western grey kangaroos (Macropus fuliginosus). J. Wildl. Dis. 47, 880–887. doi: 10.7589/0090-3558-47.4.880
R Core Team (2020). R: A Language and Environment for Statistical Computing. Vienna: R Foundation for Statistical Computing.
Roth, G. A., Abate, D., Abate, K. H., Abay, S. M., Abbafati, C., Abbasi, N., et al. (2018). Global, regional, and national age-sex-specific mortality for 282 causes of death in 195 countries and territories, 1980–2017: a systematic analysis for the Global Burden of Disease Study 2017. Lancet 392, 1736–1788. doi: 10.1016/S0140-6736(18)32203-7
Rush, E. M., Amadi, V. A., Johnson, R., Lonce, N., and Hariharan, H. (2020). Salmonella serovars associated with Grenadian tree boa (Corallus grenadensis) and their antimicrobial susceptibility. Vet. Med. Sci. 6, 565–569. doi: 10.1002/vms3.234
Santiago, P., Jiménez-Belenguer, A., García-Hernández, J., Estellés, R. M., Hernández Pérez, M., Castillo López, M. A., et al. (2018). High prevalence of Salmonella spp. in wastewater reused for irrigation assessed by molecular methods. Int. J. Hyg. Environ. Health 221, 95–101. doi: 10.1016/j.ijheh.2017.10.007
Santos, C. M., da, C., Pimenta, C. A., de, M., and Nobre, M. R. C. (2007). The PICO strategy for the research question construction and evidence search. Rev. Lat. Am. Enfermagem 15, 508–511. doi: 10.1590/s0104-11692007000300023
Sbodio, A., Maeda, S., Lopez-Velasco, G., and Suslow, T. V. (2013). Modified Moore swab optimization and validation in capturing E. Coli O157: H7 and Salmonella enterica in large volume field samples of irrigation water. Food Res. Int. 51, 654–662. doi: 10.1016/j.foodres.2013.01.011
Sha, Q., Gunathilake, A., Forstner, M. R. J., and Hahn, D. (2011). Temporal analyses of the distribution and diversity of Salmonella in natural biofilms. Syst. Appl. Microbiol. 34, 353–359. doi: 10.1016/j.syapm.2011.01.005
Sharma, M., Handy, E. T., East, C. L., Kim, S., Jiang, C., Callahan, M. T., et al. (2020). Prevalence of Salmonella and Listeria monocytogenes in non-traditional irrigation waters in the Mid-Atlantic United States is affected by water type, season, and recovery method. PLoS One 15:e0229365. doi: 10.1371/journal.pone.0229365
Sikorski, M. J., and Levine, M. M. (2020). Reviving the “Moore Swab”: a classic environmental surveillance tool involving filtration of flowing surface water and sewage water to recover typhoidal Salmonella bacteria. Appl. Environ. Microbiol. 86, e00060–20. doi: 10.1128/AEM.00060-20
Song, Q., Zhang, D., Gao, H., and Wu, J. (2018). Salmonella Species’ Persistence and Their High Level of Antimicrobial Resistance in Flooded Man-Made Rivers in China. Microb. Drug Resist. 24, 1404–1411. doi: 10.1089/mdr.2017.0316
Steele, M., and Odumeru, J. (2004). Irrigation water as source of foodborne pathogens on fruit and vegetables. J. Food Prot. 67, 2839–2849. doi: 10.4315/0362-028X-67.12.2839
Stokdyk, J. P., Firnstahl, A. D., Walsh, J. F., Spencer, S. K., de Lambert, J. R., Anderson, A. C., et al. (2020). Viral, bacterial, and protozoan pathogens and fecal markers in wells supplying groundwater to public water systems in Minnesota, USA. Water Res. 178:115814. doi: 10.1016/j.watres.2020.115814
Suijkerbuijk, A. W. M., Bouwknegt, M., Mangen, M.-J. J., de Wit, G. A., van Pelt, W., Bijkerk, P., et al. (2017). The economic burden of a Salmonella Thompson outbreak caused by smoked salmon in the Netherlands, 2012-2013. Eur. J. Public Health 27, 325–330. doi: 10.1093/eurpub/ckw205
Tack, D. M., Marder, E. P., Griffin, P. M., Cieslak, P. R., Dunn, J., Hurd, S., et al. (2019). Preliminary incidence and trends of infections with pathogens transmitted commonly through food — Foodborne Diseases Active Surveillance Network, 10 U.S. sites, 2015–2018. Am. J. Transplant. 19, 1859–1863. doi: 10.1111/ajt.15412
Therneau, T., Atkinson, B., and Ripley, B. (2013). Rpart: Recursive Partitioning. R Package Version 4.1-3. Available online at: http://cran.r-project.org/package=rpart (accessed March 23, 2022).
Topalcengiz, Z., Strawn, L. K., and Danyluk, M. D. (2017). Microbial quality of agricultural water in Central Florida. PLoS One 12:e0174889. doi: 10.1371/journal.pone.0174889
Toro, M., Retamal, P., Ayers, S., Barreto, M., Allard, M., Brown, E. W., et al. (2016). Whole-Genome Sequencing Analysis of Salmonella enterica Serovar Enteritidis Isolates in Chile Provides Insights into Possible Transmission between Gulls, Poultry, and Humans. Appl. Environ. Microbiol. 82, 6223–6232. doi: 10.1128/AEM.01760-16
Traoré, O., Nyholm, O., Siitonen, A., Bonkoungou, I. J. O., Traoré, A. S., Barro, N., et al. (2015). Prevalence and diversity of Salmonella enterica in water, fish and lettuce in Ouagadougou, Burkina Faso. BMC Microbiol. 15:151. doi: 10.1186/s12866-015-0484-7
Truitt, L. N., Vazquez, K. M., Pfuntner, R. C., Rideout, S. L., Havelaar, A. H., and Strawn, L. K. (2018). Microbial quality of agricultural water used in produce preharvest production on the eastern shore of Virginia. J. Food Prot. 81, 1661–1672. doi: 10.4315/0362-028X.JFP-18-185
United Nations Development Programme [UNDP] (2020). The Next Frontier: Human Development and the Anthropocene. Available online at: http://hdr.undp.org/en/2020-report (accessed March 23, 2022).
Viechtbauer, W. (2010). Conducting Meta-Analyses in R with the metafor Package. J. Stat. Softw. 36, 1–48. doi: 10.18637/jss.v036.i03
Walsh, K. A., Bennett, S. D., Mahovic, M., and Hannah Gould, L. (2014). Outbreaks associated with cantaloupe, watermelon, and honeydew in the United States, 1973-2011. Foodborne Pathog. Dis. 11, 945–952. doi: 10.1089/fpd.2014.1812
Wanjugi, P., and Harwood, V. J. (2013). The influence of predation and competition on the survival of commensal and pathogenic fecal bacteria in aquatic habitats. Environ. Microbiol. 15, 517–526. doi: 10.1111/j.1462-2920.2012.02877.x
Wilkes, G., Edge, T. A., Gannon, V. P. J., Jokinen, C., Lyautey, E., Neumann, N. F., et al. (2011). Associations among pathogenic bacteria, parasites, and environmental and land use factors in multiple mixed-use watersheds. Water Res. 45, 5807–5825. doi: 10.1016/j.watres.2011.06.021
Keywords: agriculture, epidemiology, foodborne pathogens, meta-analysis, one health, salmonellosis, systematic review
Citation: Rocha ADL, Ferrari RG, Pereira WE, Lima LA, Givisiez PEN, Moreno-Switt AI, Toro M, Delgado-Suárez EJ, Meng J and Oliveira CJB (2022) Revisiting the Biological Behavior of Salmonella enterica in Hydric Resources: A Meta-Analysis Study Addressing the Critical Role of Environmental Water on Food Safety and Public Health. Front. Microbiol. 13:802625. doi: 10.3389/fmicb.2022.802625
Received: 26 October 2021; Accepted: 29 April 2022;
Published: 02 June 2022.
Edited by:
Mostafa Ghanem, University of Maryland, College Park, United StatesReviewed by:
Ben Davies Tall, United States Food and Drug Administration, United StatesCopyright © 2022 Rocha, Ferrari, Pereira, Lima, Givisiez, Moreno-Switt, Toro, Delgado-Suárez, Meng and Oliveira. This is an open-access article distributed under the terms of the Creative Commons Attribution License (CC BY). The use, distribution or reproduction in other forums is permitted, provided the original author(s) and the copyright owner(s) are credited and that the original publication in this journal is cited, in accordance with accepted academic practice. No use, distribution or reproduction is permitted which does not comply with these terms.
*Correspondence: Celso José Bruno de Oliveira, Y2Vsc28ub2xpdmVpcmFAYWNhZGVtaWNvLnVmcGIuYnI=
Disclaimer: All claims expressed in this article are solely those of the authors and do not necessarily represent those of their affiliated organizations, or those of the publisher, the editors and the reviewers. Any product that may be evaluated in this article or claim that may be made by its manufacturer is not guaranteed or endorsed by the publisher.
Research integrity at Frontiers
Learn more about the work of our research integrity team to safeguard the quality of each article we publish.