- 1School of Basic Medical Sciences, Shanxi Medical University, Taiyuan, China
- 2Key Laboratory of Cellular Physiology at Shanxi Medical University, Ministry of Education, Taiyuan, China
- 3Shanxi Key Laboratory of Big Data for Clinical Decision Research, Taiyuan, China
- 4Department of Rheumatology and Immunology, The Second Hospital of Shanxi Medical University, Taiyuan, China
- 5School of Management, Shanxi Medical University, Taiyuan, China
This study investigated the association between intestinal microbiota abundance and diversity and cluster of differentiation (CD)4+ T cell subpopulations, cytokine levels, and disease activity in rheumatoid arthritis RA. A total of 108 rheumatoid arthritis (RA) patients and 99 healthy control (HC) subjects were recruited. PICRUSt2 was used for functional metagenomic predictions. Absolute counts of peripheral CD4+ T cell subpopulations and cytokine levels were detected by flow cytometry and with a cytokine bead array, respectively. Correlations were analyzed with the Spearman rank correlation test. The results showed that the diversity of intestinal microbiota was decreased in RA patients compared to HCs. At the phylum level, the abundance of Firmicutes, Fusobacteriota, and Bacteroidota was decreased while that of Actinobacteria and Proteobacteria was increased and at the genus level, the abundance of Faecalibacterium, Blautia, and Escherichia-Shigella was increased while that of Bacteroides and Coprococcus was decreased in RA patients compared to HC subjects. The linear discriminant analysis effect size indicated that Bifidobacterium was the most significant genus in RA. The most highly enriched Kyoto Encyclopedia of Genes and Genomes pathway in RA patients was amino acid metabolism. The relative abundance of Megamonas, Monoglobus, and Prevotella was positively correlated with CD4+ T cell counts and cytokine levels; and the relative numbers of regulatory T cells (Tregs) and T helper (Th17)/Treg ratio were negatively correlated with disease activity in RA. These results suggest that dysbiosis of certain bacterial lineages and alterations in gut microbiota metabolism lead to changes in the host immune profile that contribute to RA pathogenesis.
Introduction
Rheumatoid arthritis (RA) is a chronic autoimmune disease characterized by irreversible peripheral joint damage (England et al., 2018). The etiopathogenic mechanism of RA involves abnormal immune activation with various autoantibodies, an imbalance of lymphocyte subpopulations, and cytokine dysregulation.
Alterations in the gut microbiome profile are associated with immune dysfunction in several rheumatic diseases (Li and Wang, 2021). The abundance of intestinal microbiota is known to differ between RA patients and healthy subjects (Vaahtovuo et al., 2008; Scher et al., 2013; Taneja, 2014). Moreover, gut dysbiosis may negatively affect immune function in RA (Li and Wang, 2021). Interaction between gut microbiota and the host is important for maintaining immune homeostasis (Chu and Mazmanian, 2013). Gut bacteria have been shown to influence the polarization of lymphocyte subpopulations and their cytokines and thereby regulate immune functions (Abdulla et al., 2021).
Dysbiosis in the gut microbiome is associated with various autoimmune diseases, and the detailed mechanisms of how changes in the abundance and diversity of these microorganisms contribute to disease pathogenesis in the host is unknown (Li et al., 2017). To address this question, in this study we investigated the associations between gut microbiota community composition and metabolic pathways, cluster of differentiation (CD)4+ T cell subpopulations, cytokine levels, and disease activity in RA.
Materials and Methods
Study Population
A total of 108 RA patients (73 females, 35 males) were recruited at the Department of Rheumatology, Second Hospital of Shanxi Medical University (Zhao et al., 2019) between December 2018 and August 2019. No medication was provided to patients, and the RA group specimens were collected on the day of hospitalization. The median age was 52.7 years and the age range was 21–70 years. We also recruited 99 healthy controls (HCs) (68 females, 31 males) from our health examination center (Li Y. et al., 2021). The mean age of healthy volunteers was 56.56 years. There were no statistically significant differences (t-test) in the composition of the 2 groups with regard to age, and sex. All patients met the 2010 American College of Rheumatology/European League Against Rheumatism classification criteria for RA (Lee and Kim, 2017). Exclusion criteria were subjects who had another autoimmune-related or gastrointestinal tract or inflammatory bowel disease, take any probiotics or antibiotics within 1 month, serious infection, or a malignant tumor within the previous 6 months (Zhang et al., 2021). Each participant signed the consent form. This study was approved by the institutional ethics committee of the Second Affiliated Hospital of Shanxi Medical University (approval no. 2019-YX-107).
We recorded all clinical symptoms and laboratory measures of disease activity including C-reactive protein, erythrocyte sedimentation rate, swollen joint count, and tender joint count; these were used to calculate the disease activity score (DAS28) (Li et al., 2020).
Fecal Sample Collection and 16S rRNA Gene Sequencing
Fresh fecal samples were collected and immediately stored at −80°C in a sterile box for microbial DNA extraction (Li Y. et al., 2021). After obtaining consent, peripheral blood samples were obtained from 106 patients and 109 patients were tested for cytokine levels. Microbial DNA was extracted from about 250 mg of fecal sample using the QIAamp PowerFecal DNA Kit (Qiagen, Valencia, CA, United States) according to the manufacturer’s instructions. It was then quantified by agarose gel electrophoresis on a NanoDrop One spectrophotometer (Thermo Fisher Scientific, Waltham, MA, United States) (Ahmad et al., 2019; Li Y. et al., 2021). The primers 341F (CCTACGGGNGGCWGCAG) and 805 R (GACTACHVGGGTATCTAATCC) were used to PCR amplify the V3–V4 region of the 16S rRNA gene (Liang et al., 2017) using KAPA HiFi HotStart Ready Mix (Roche, Indianapolis, IN, United States). The resultant amplicons were sequenced on a MiSeq platform (Illumina, San Diego, CA, United States).
QIIME2 was used to process representative sequence clusters with a similarity cutoff of 100% (Ahmad et al., 2019). Operational taxonomic units (OTUs) were partitioned into taxonomic lineages in the SILVA 16S rDNA database (Frisbee et al., 2019). Based on the rarefied OTUs, α and β diversity were calculated using the R package “PhyloSeq.” Shannon (also known as Shannon-Weaver index) (Ott et al., 2014; Gabriel et al., 2019), simpson, and invSimpson index (richness and evenness) and richness (observed OTUs, chao1, ACE) (Ramadan et al., 2021) was used to measure alpha-diversity. Biomarker species were identified based on linear discriminant analysis effect size (LefSe).
Metagenome Functional Predictions
Microbial functions were predicted using PICRUSt2 (Chen et al., 2020), which contains reference genomes and gene families and provides interoperability with OTU picking and a denoising algorithm that incorporates the Benjamini–Hochberg correction (Muraoka et al., 2020), allowing assignment of OTUs to reference genomes and linking of taxonomic information to Kyoto Encyclopedia of Genes and Genomes (KEGG) annotations (Douglas et al., 2020). PICRUSt2 was used to assess the potential metabolic functions of the gut microbiome in RA (Suárez-Moo et al., 2020).
Flow Cytometry
CD4+ T cells in whole blood collected in a 3-ml anticoagulant tube were detected using a FACSCalibur flow cytometer (BD Biosciences, San Jose, CA, United States) (Kojima et al., 1976; Niu et al., 2020). Four multicolor monoclonal antibodies were used for immunofluorescence labeling of circulating CD4+ T cell subpopulations. An 80-μl volume of blood was combined with 10 μl ionomycin, 10 μl phorbol 12-myristate 13-acetate, and 1 μl GolgiStop and incubated at 37°C for 5 h (Niu et al., 2020). Fluorescein isothiocyanate-conjugated anti-CD4 antibody was added to the tube, followed by incubation for 30 min in the dark at room temperature (20–25°C). The cells were immobilized using Cytofix/Cytoperm reagent and incubated at 4°C for 30 min; they were then labeled with allophycocyanin (APC)-conjugated anti-interferon (IFN)-γ, phycoerythrin (PE)-conjugated anti-interleukin (IL)-17A, and PE-conjugated anti-IL-4 antibodies to detect Th1, Th17, and Th2 cells, respectively, followed by incubation for 30 min in the dark at room temperature (20–25°C). To detect regulatory T cells (Tregs), 80 μl of anticoagulant-treated blood was labeled with APC-conjugated anti-CD25 and PE-conjugated anti-forkhead box (Fox) P3 antibodies. After washing, the samples were analyzed by flow cytometry. The percentages and absolute counts of CD4+ T cell subpopulations were determined using BD Multitest software (BD Biosciences) (Niu et al., 2021).
A flow cytometric bead array (Jiangsu, China) was used to analyze the levels of IL-2, IL-4, IL-6, IL-10, IL-17, tumor necrosis factor (TNF)-α, and IFN-γ, followed by flow cytometry analysis (Chen et al., 2012). The standard curve of each cytokine was in the range of 1–5,000 pg/ml.
Statistical Analysis
Alpha diversity was compared between groups with Welch’s t-test. Correlations were evaluated with the Spearman rank correlation test (Anuradha et al., 2017). Differences with P < 0.05 were considered statistically significant.
Results
Clinical Characteristics of the Study Population
A total of 207 stool samples were collected. The detailed clinical characteristics of the study population are shown in Table 1.
Disease Duration Is Associated With Decreased Microbial Diversity
We obtained 13,147 high-quality OTUs including 12 phyla, 19 classes, 42 orders, 72 families, and 189 genera. The Observed, ACE, Chao1 (richness) and Shannon (richness and evenness) indices showed difference in RA patients compared to HCs (P < 0.05) (Figure 1), although there was no difference in Simpson index between the 2 groups (Figure 1). Above all, gut microbiota was markedly less diverse in composition in RA patients than in the HC group. These indexs lay a foundation for species analyzed. The weighted-UniFrac distance principal coordinate analysis based on OTUs showed that the gut microbial community structure between RA and HC groups differed (Figure 2). Helper T cell (Th)2 (Figure 3A) and Th17 (Figure 3B) counts were negatively correlated with α diversity in RA patients (P < 0.05 and < 0.01, respectively).
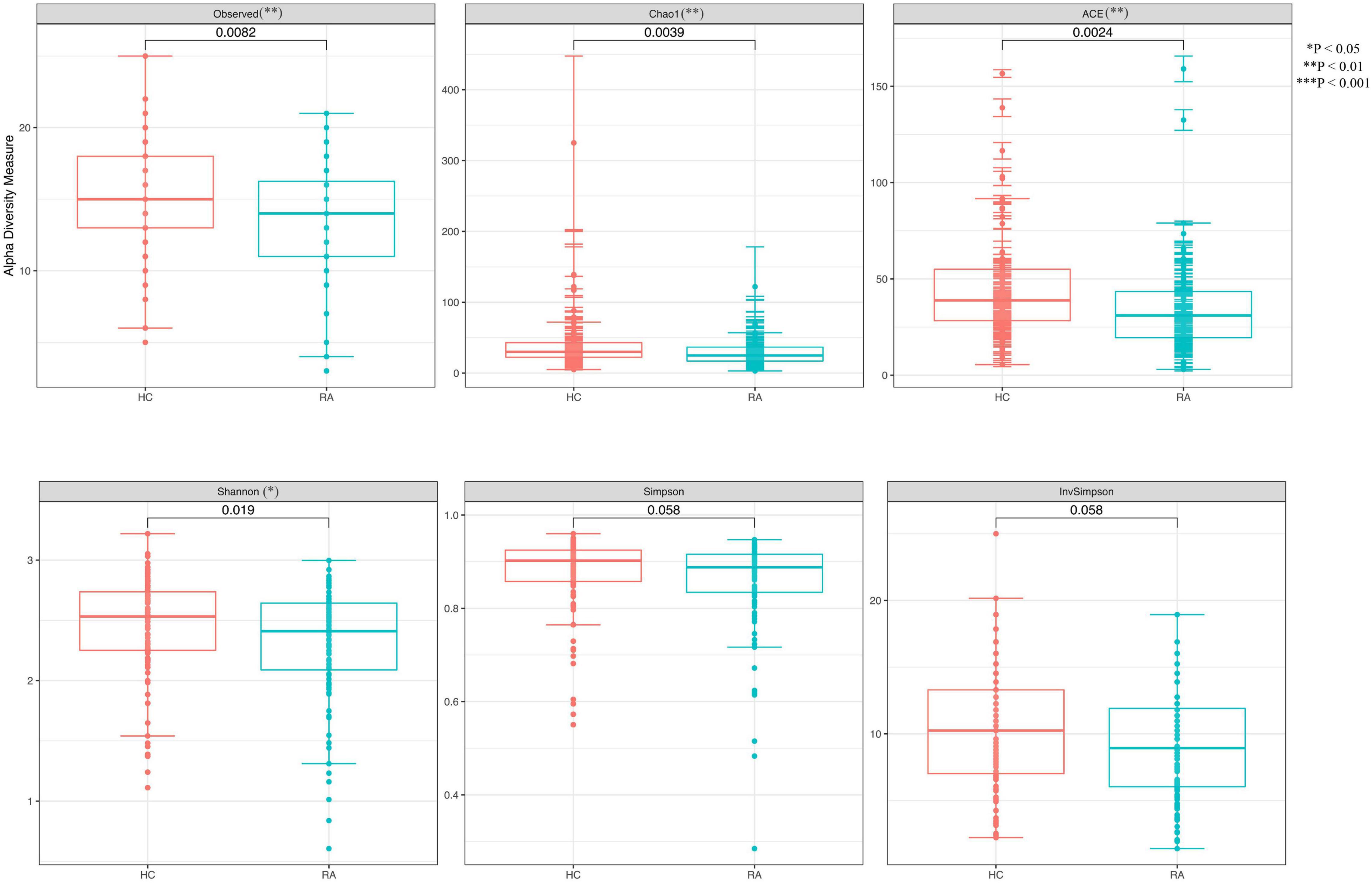
Figure 1. Differences in α diversity of the gut microbiome between RA patients and HCs. **P < 0.01, ***P < 0.001.
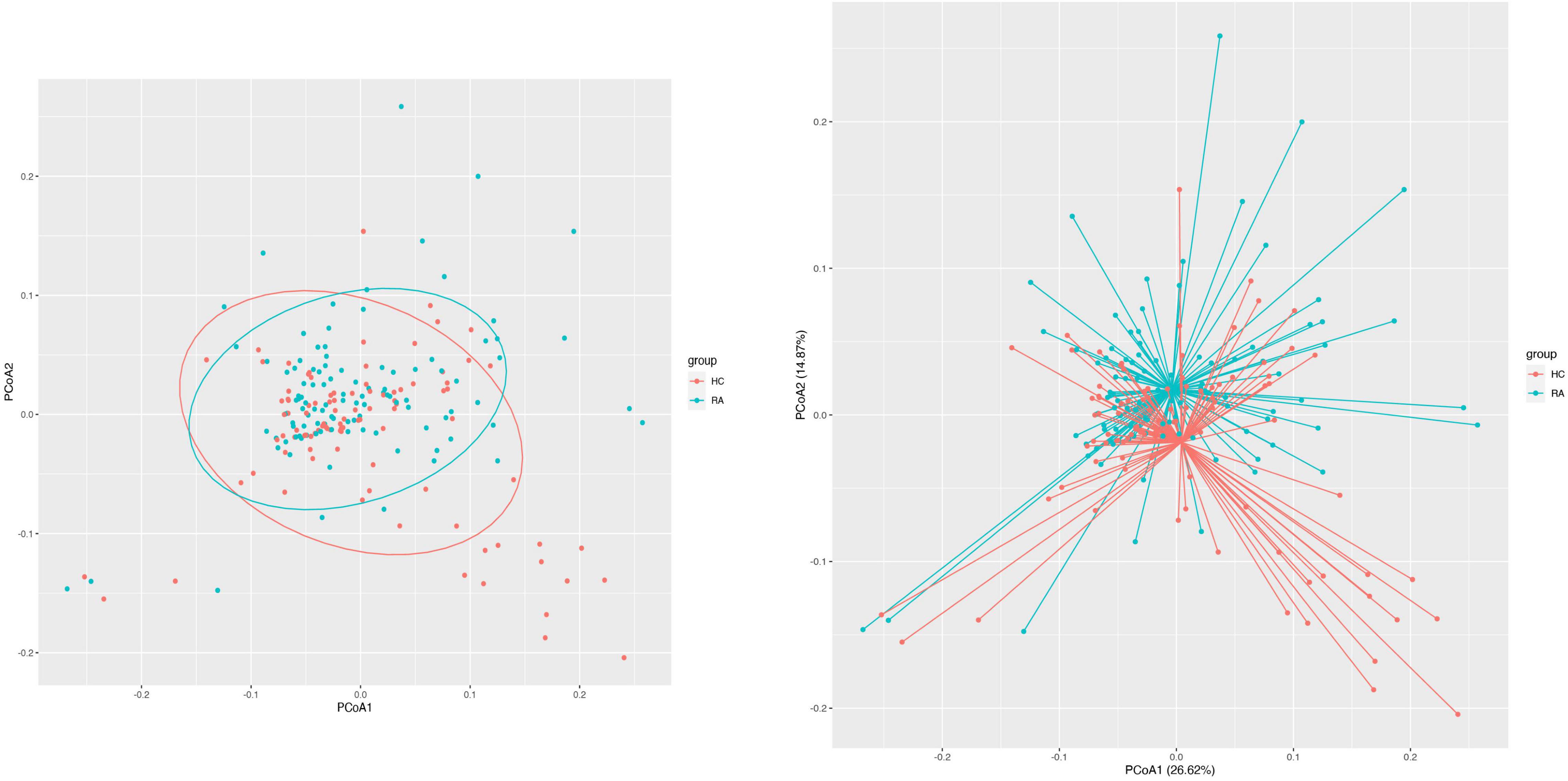
Figure 2. β Diversity of the gut microbiome in RA patients and HCs. Principal coordinate analysis plot generated from the weighted UniFrac analyze. The x and y axes represent the first and second coordinates, respectively; and values in parentheses show the percentage of the community variation explained by each coordinate. Blue and red symbols represent bacteria in the RA and control groups, respectively.
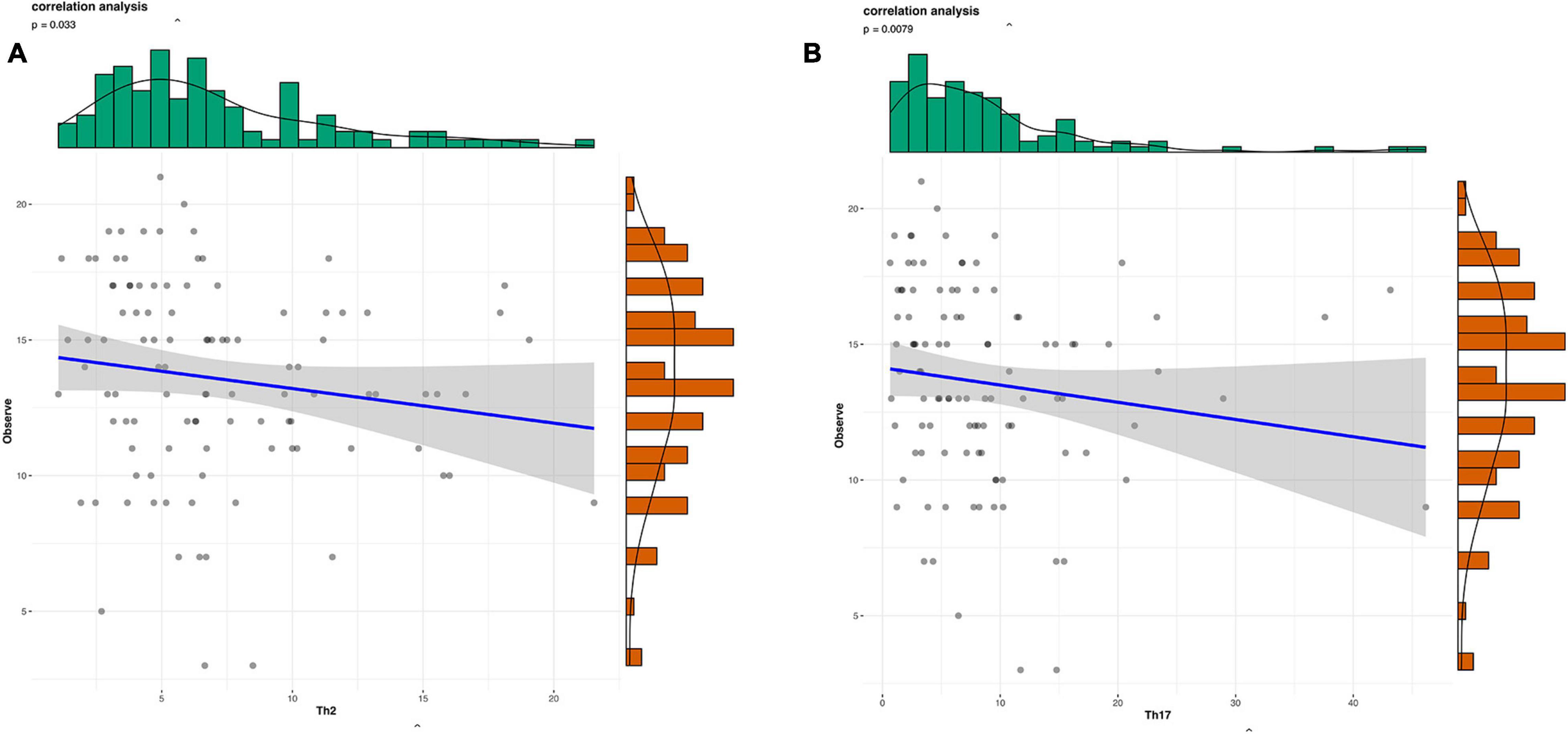
Figure 3. Scatterplots of the correlation between cytokine levels and α diversity. (A,B) Correlation between Th1 (A) and Th17 (B) and Observed value (richness).
Gut microbial community structure differed between RA and HC groups, as revealed by phylum and genus Bray–Curtis distances (Figures 4A, 5A). At the phylum level, the relative abundance of Firmicutes, Fusobacteriota, and Bacteroidota was decreased (P < 0.05) whereas that of Actinobacteria and Proteobacteria was increased (P < 0.05) in RA patients compared to HCs (Figures 4B,C). At the genus level, the abundance of Faecalibacterium, Blautia, Terrisporobacter, Escherichia-Shigella, and Fusicatenibacter was increased while that of Bacteroides, Coprococcus, and Parabacteroides was decreased (P < 0.05) in RA patients compared to HCs (Figures 5B, 6).
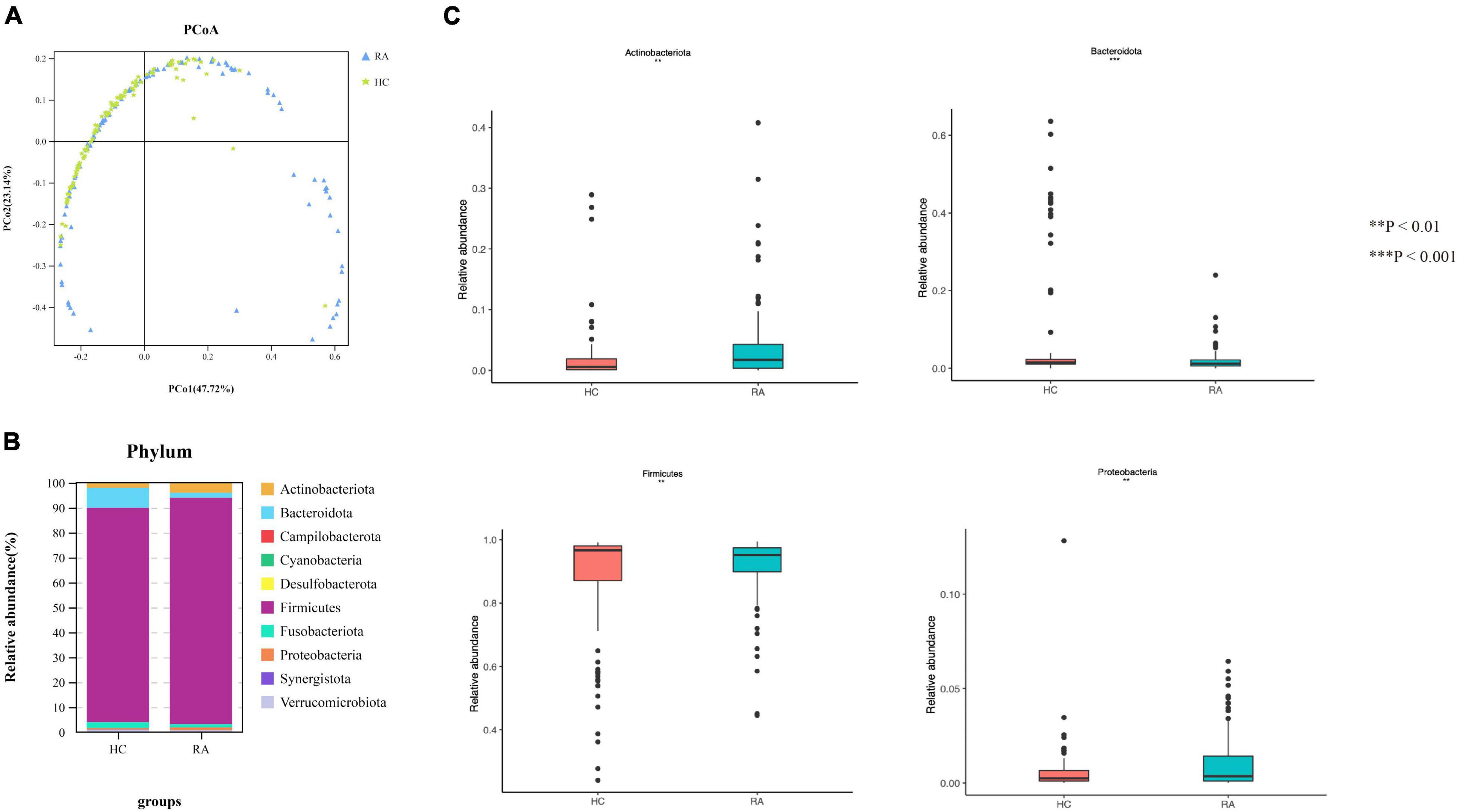
Figure 4. Microbiota community composition of RA patients at the phylum level. (A) Principal coordinate analysis plots at the phylum level for RA patients and HCs. The x and y axes represent the first and second coordinates, respectively, and values in parentheses show the percentages of the community variation explained by each coordinate. Blue and green symbols represent bacterial phyla in the RA and control groups, respectively. (B) Relative abundance of phyla in all subjects and in each group. (C) Phylum-level comparison of the relative abundance of gut microbiota between RA patients and HCs. *P < 0.05, **P < 0.01, ****P < 0.001.
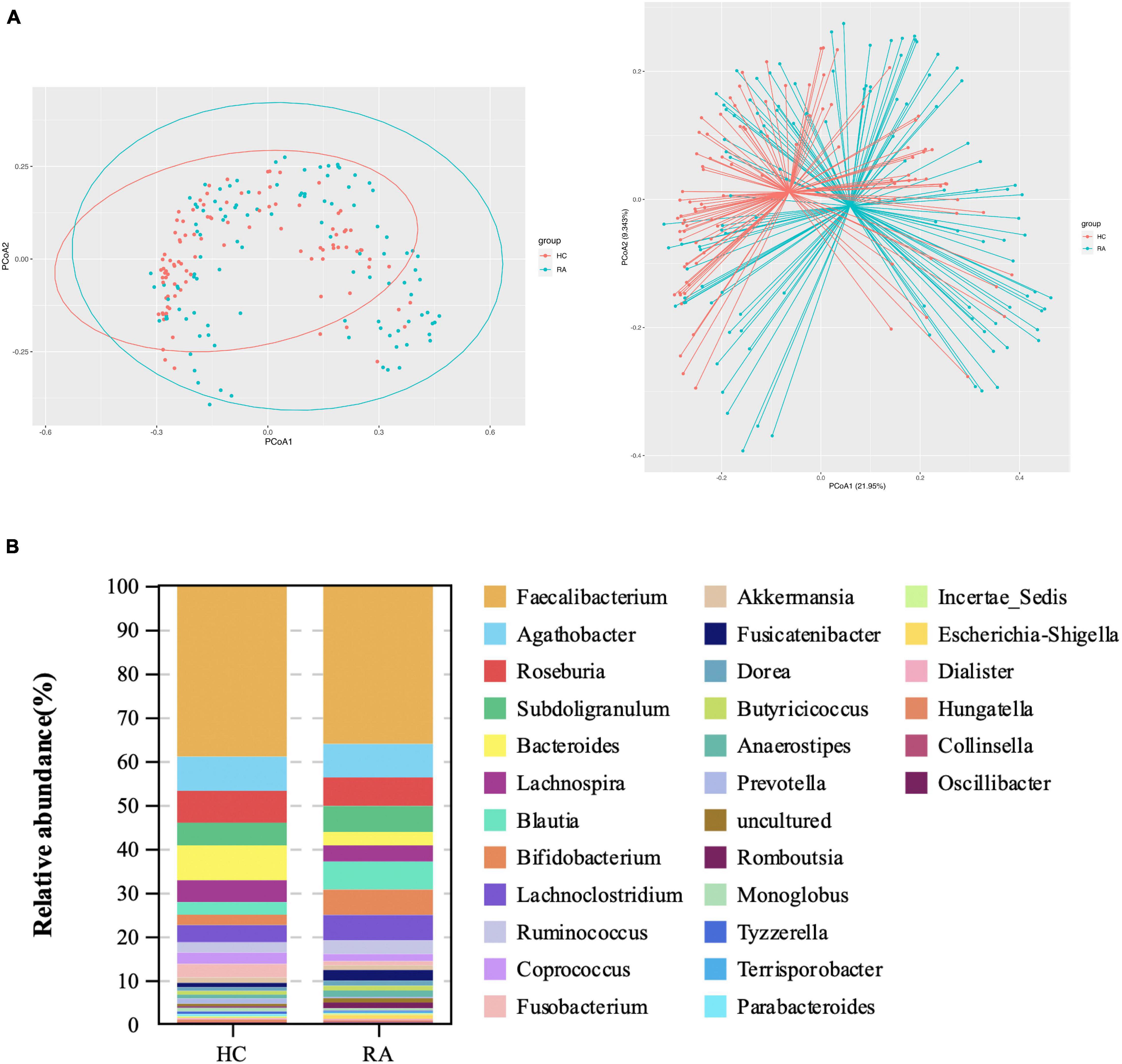
Figure 5. Microbiota community composition of RA patients at the genus level. (A) Principal coordinate analysis plots generated from the Bray–Curtis analysis. The x and y axes represent the first and second coordinates, respectively, and values in parentheses show the percentages of the community variation explained by each coordinate. Blue and red symbols represent bacterial genera in the RA and control groups, respectively. (B) Relative abundance of genera in all subjects and in each group.
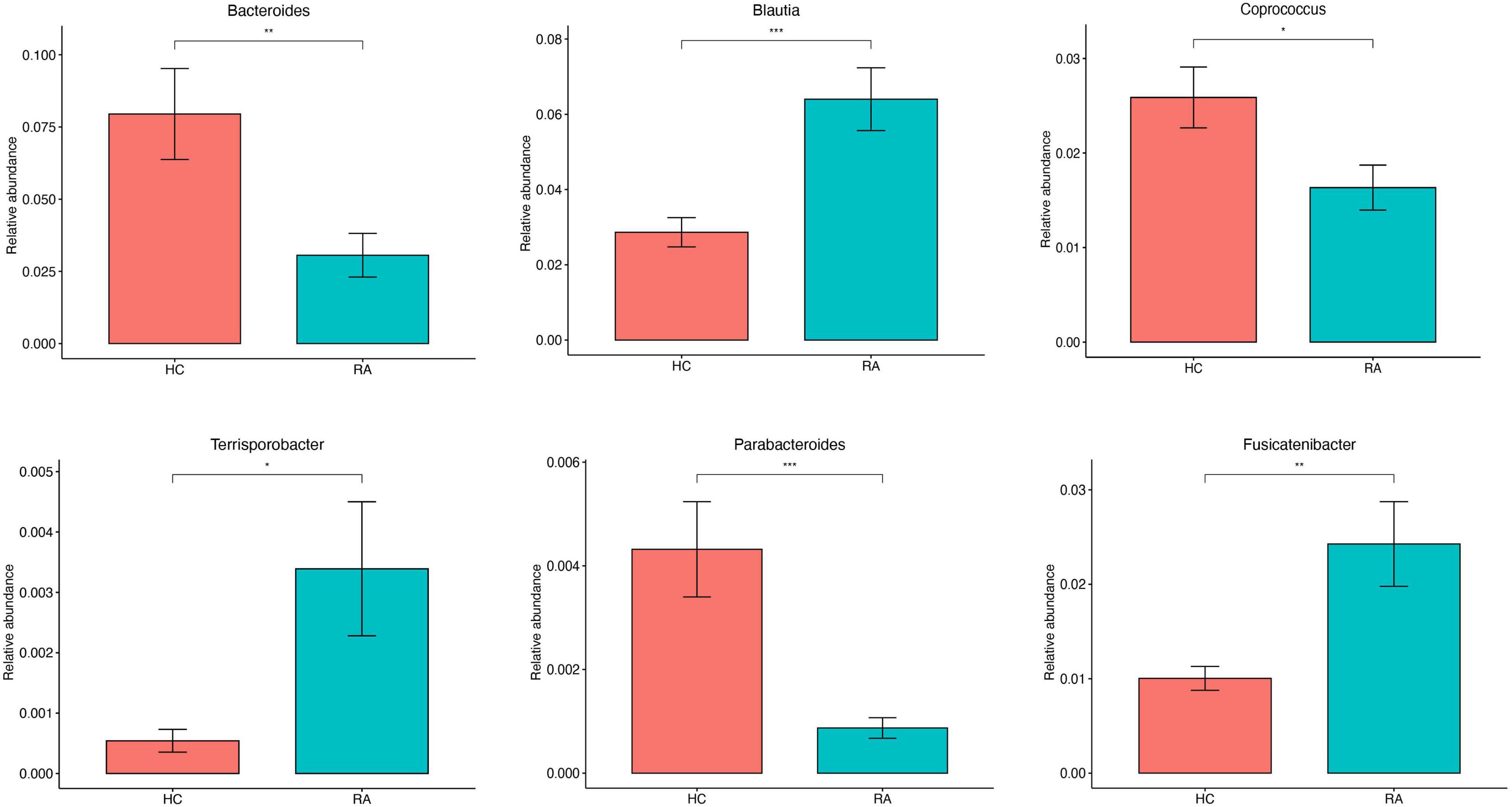
Figure 6. Genus-level comparison of the relative abundance of gut microbiota between RA patients and HCs. *P < 0.05, **P < 0.01, ***P < 0.001.
Gut Flora Is Altered in Rheumatoid Arthritis
A total of 28 differentially abundant taxa were observed by LEfSe analysis (Mollazadeh et al., 2019). The abundance of phylum Actinobacteria, including the genus Bifidobacterium, was increased in RA patients compared to HCs. The genus Dialister was most closely associated with RA. The Bacteroidaceae and Marinifilaceae families in phylum Bacteroidota were expanded in the HC group; and Sutterella and Escherichia-Shigella in phylum Proteobacteria, which formed separate clusters, were expanded in the RA and HC groups, respectively (Figures 7A,B).
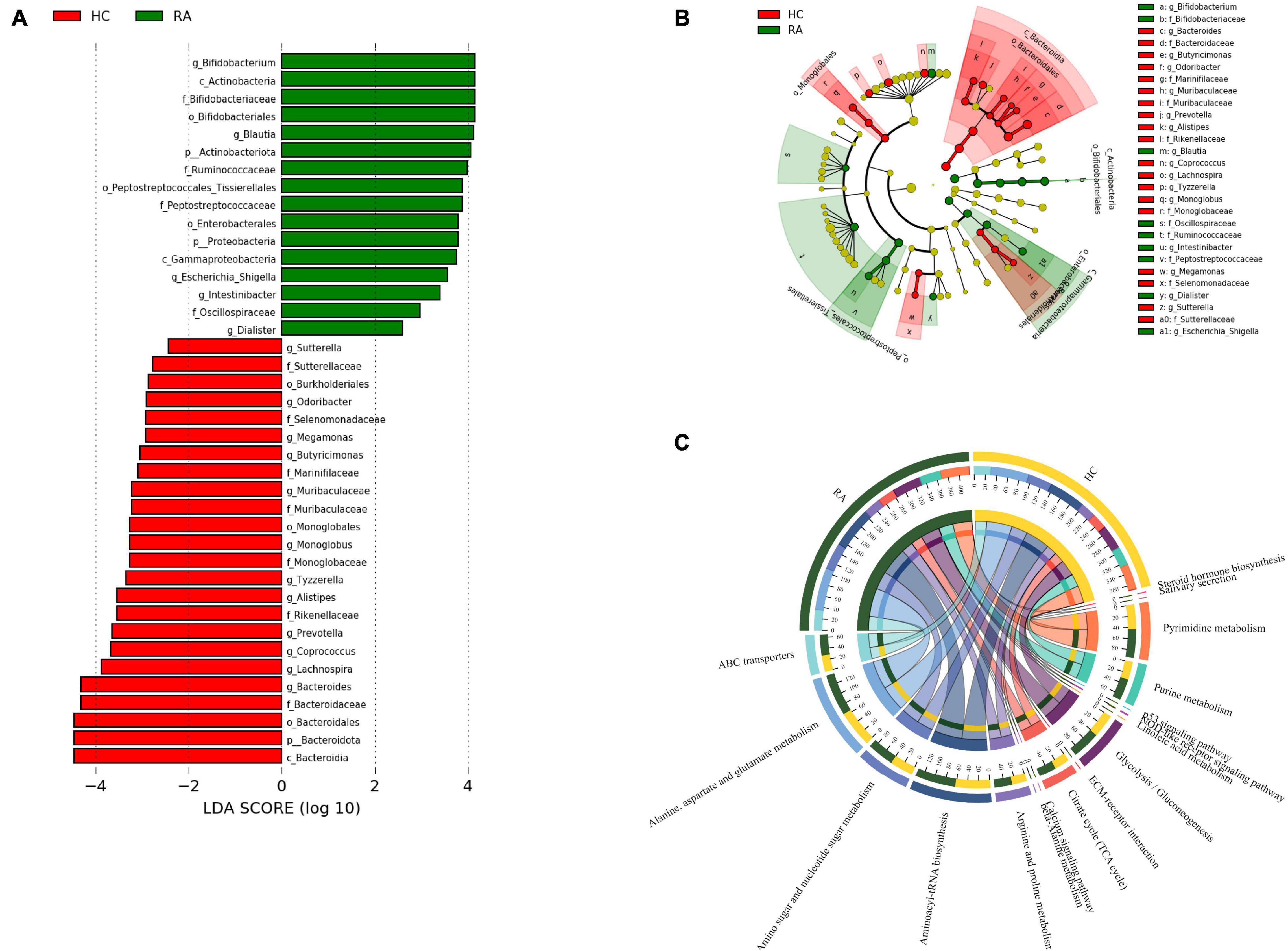
Figure 7. Patients with RA characterized by expansion of rare microbial lineages. (A,B) LEfSe analysis was performed to identify differentially abundant taxa, which are highlighted on the phylogenetic tree in cladogram format (A) and for which the linear discriminant analysis scores are shown (B). Red and green colors indicate an increase or decrease in taxa, respectively, in RA patients compared to HCs. (C) KEGG pathways significantly enriched in RA patients compared to HCs.
Functional Analysis of Gut Microbes in Rheumatoid Arthritis
PICRUSt2 was used to infer the functions of the significantly different taxa between RA patients and HCs based on 16S rRNA gene sequences. KEGG pathways that were significantly enriched in RA patients included amino acid metabolism (e.g., alanine, aspartate, and glutamate), amino sugar and nucleotide sugar metabolism, β-alanine metabolism, ATP-binding cassette (ABC) transporters, glycolysis/gluconeogenesis, NOD-like receptor signaling pathway, and p53 signaling pathway (Figure 7C). The metabolic pathway of ABC transporters was negatively correlated with the absolute numbers of Tregs (P < 0.01), while IL-6 level and NOD-like receptor signaling pathway showed positive correlations with the absolute number of Th1 cells (P < 0.05) (Figures 8A,B).
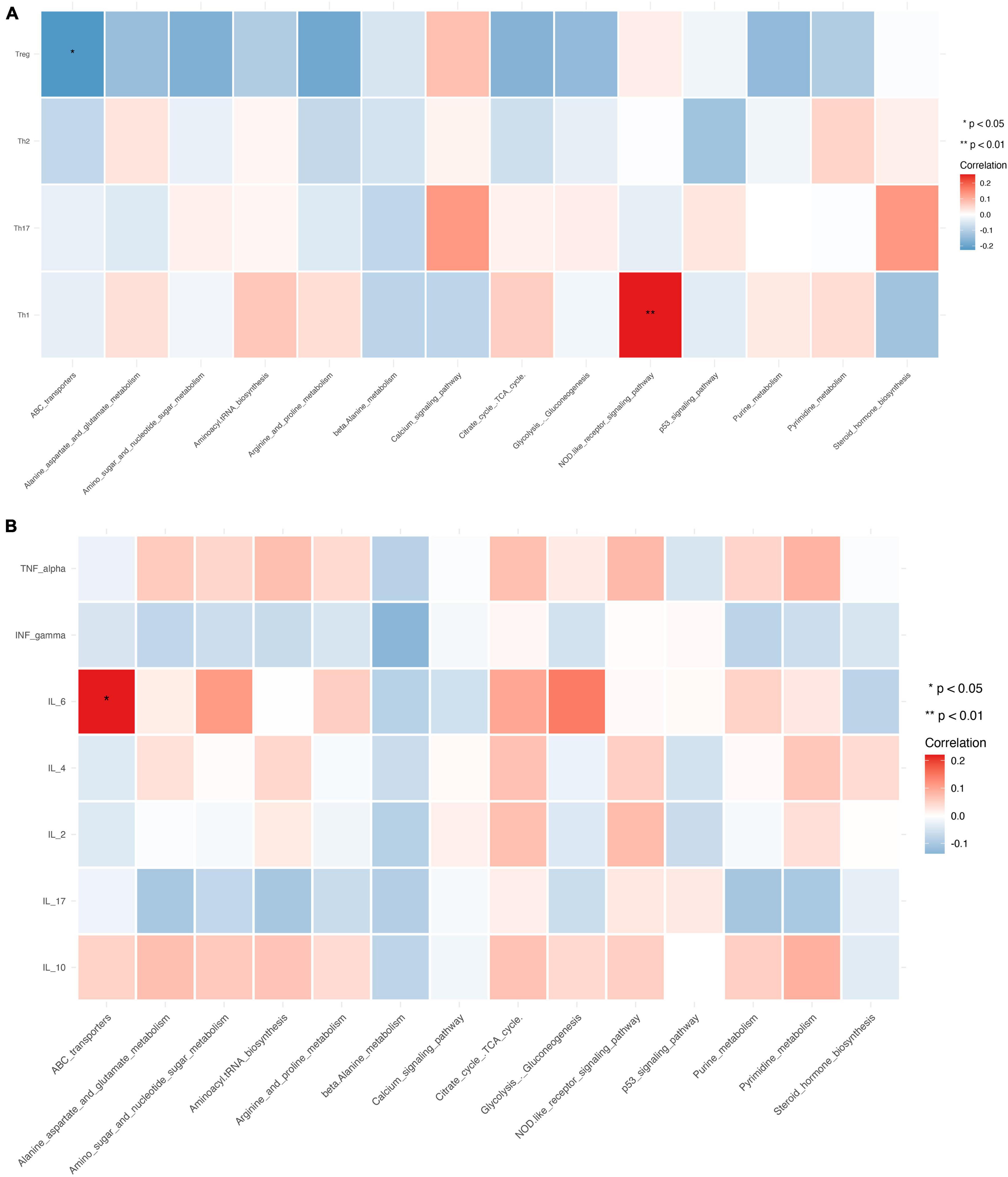
Figure 8. Relationship between CD4+ T cell subpopulations, cytokines, and metabolic pathways. (A,B) Heat map of the correlation between different metabolic pathways and CD4+ T cell subpopulations (A) and cytokines. Colors indicate the Spearman rank correlation, with lighter and darker colors corresponding to weaker and stronger correlations, respectively. *P < 0.05, **P < 0.01.
Relationship Between Gut Microbiota and CD4+ T Cell Subpopulations and Cytokine Levels
The relative abundance of Megamonas and Monoglobus was positively correlated with the absolute numbers of Th1 and Th2 cells, which are CD4+ T cell subsets (P < 0.01). Verrucomicrobiota was positively correlated with the absolute number of Tregs (P < 0.01), while Firmicutes was negatively correlated with the absolute number of Th17 cells (P < 0.05) (Figure 9A).
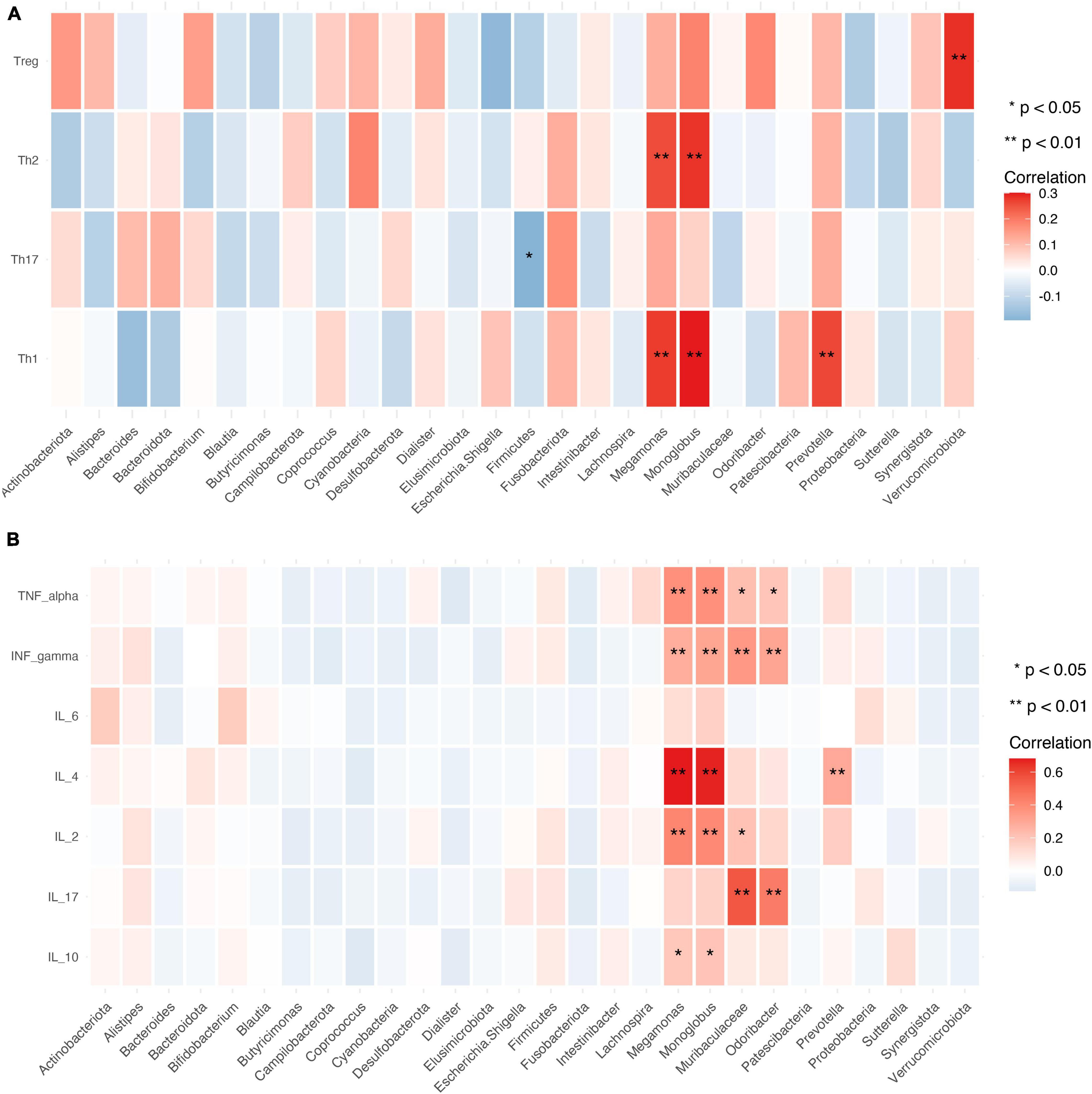
Figure 9. Relationship between gut microbiota, CD4+ T cell subpopulations, and cytokines. (A,B) Heat map of the correlation between different gut microbial species and CD4+ T cell subpopulations (A) and cytokines (B). Colors indicate the Spearman rank correlation, with lighter and darker colors corresponding to weaker and stronger correlations, respectively. *P < 0.05, **P < 0.01.
Gut microbiota abundance was closely associated with cytokine levels. The relative abundance of Megamonas and Monoglobus showed significant positive correlations with the levels of IL-10, IL-2, IL-4, TNF-α, and IFN-γ (P < 0.01 or < 0.05). Muribaculaceae and Odoribacter were positively correlated with IL-17, and TNF-α, and IFN-γ levels (P < 0.01 or < 0.05) and Prevotella was positively correlated with IL-4 level (Figure 9B).
Disease Activity in Rheumatoid Arthritis Is Closely Linked to Gut Microbiota, Lymphocyte Subpopulations, and Cytokine Levels
RA patients were classified according to disease activity into low (DAS28 ≥ 2.6 and ≤ 3.2), moderate (DAS28 > 3.2 and ≤ 5.1), and high (DAS28 > 5.1) activity groups. The relative abundance of Bifidobacterium and Lachnospira increased with disease activity (Figure 10A). The relative abundance of Blautia was higher in patients with high disease activity compared to those with moderate and low disease activity (P < 0.01; Figure 10A). Muribaculaceae abundance was positively correlated with disease activity (P = 0.0084; Figure 10B).
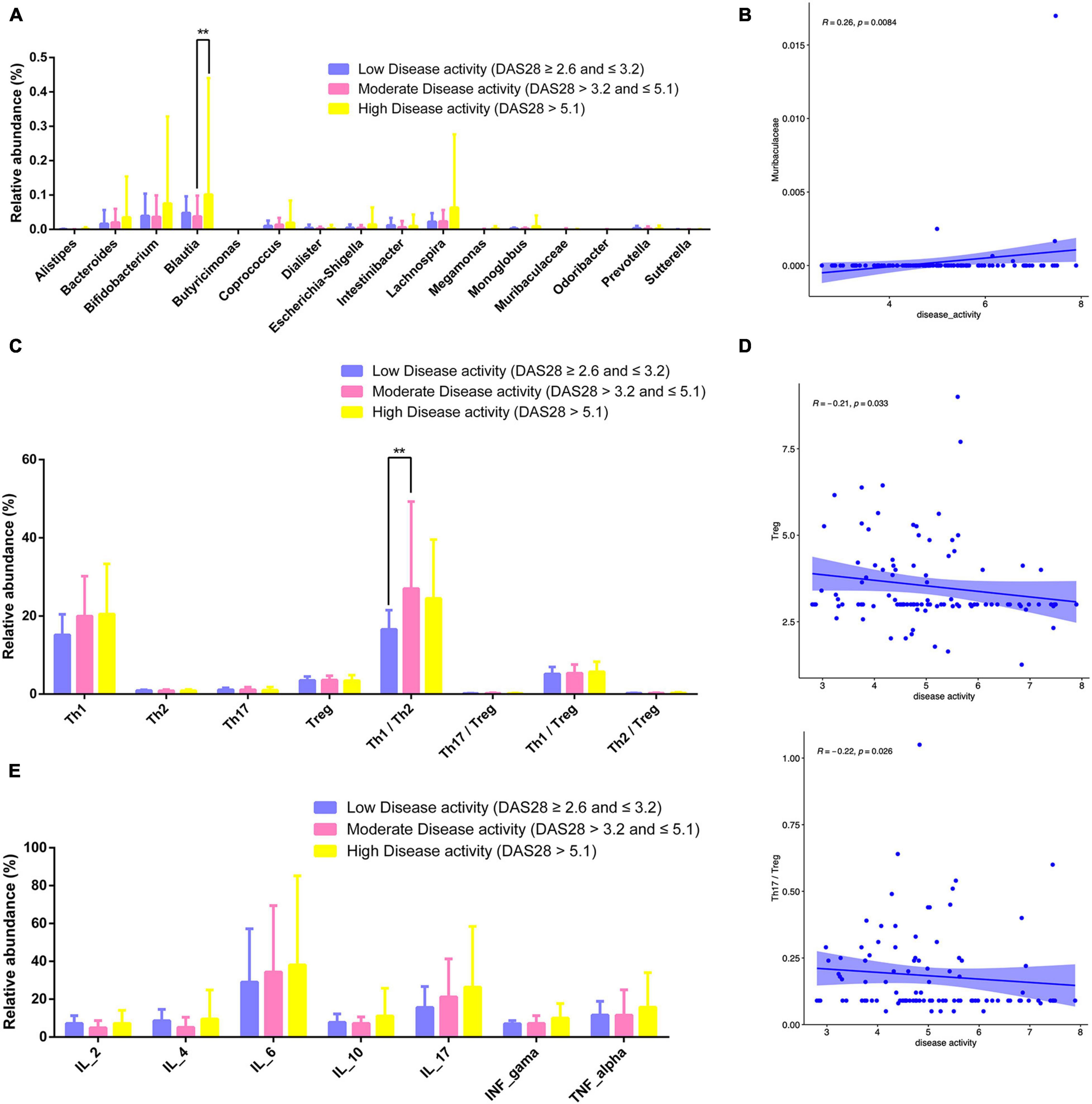
Figure 10. Gut microbiota, CD4+ T cell subpopulations, and cytokines in different RA disease activity groups. (A) Relative abundance of gut microbiota for different disease activity levels. (B) Scatter plot of the correlation between disease activity and Muribaculaceae. (C) Relative abundance of lymphocyte subpopulations for different disease activity levels. (D) Scatterplot of the correlation between disease activity and Treg counts Th17/Treg ratio. (E) Relative abundance of cytokines for different disease activity levels. **P < 0.01.
The number of Tregs and Th17/Tregs was inversely correlated with disease activity (P = 0.033 and 0.026; Figure 10D). The relative abundance of Th1/Th2 cells was higher patients with moderate disease activity than in those with low or high disease activity (P < 0.01; Figure 10C). Relative abundance of cytokines for different disease activity levels has been shown in Figure 10E.
Discussion
The results of this study demonstrate that gut flora composition and α and β diversity were altered in RA patients, which is consistent with previous studies (Maeda and Takeda, 2019; Sun et al., 2019). RA patients significantly decreased α-diversity in the gut microbiome (Observed, Chao1, ACE, and Shannon), indicating that the richness and evenness of bacteria in RA groups were lower. Perturbation in the community was also found via the analysis of β-diversity (PCoA). As to beta diversity, PCoA results indicated that the composition of gut microbiota was significantly different in these two groups. At the phylum level, the profile of gut microbiota appeared dominated by Firmicutes and Bacteroidetes and the abundance of Firmicutes, Fusobacteriota, and Bacteroidota was shown to be decreased in RA patients (Kasselman et al., 2018) whereas that of Actinobacteria was higher (Chen et al., 2016). Firmicutes, a butyrate-producing bacterium, regulates the differentiation of Tregs that inhibit inflammation; thus, a reduction in Firmicutes abundance and consequent decrease in butyrate levels can lead to inflammation in RA patients. Actinobacteria was reported increased in the rheumatoid arthritis, some of which were consistent with our findings (Chen et al., 2016). Actinobacteria can predict the RA status (Chen et al., 2016), However, another study is in contrast to our findings (Jeong et al., 2019). The role of Actinobacteria expression and function in RA needed to investigate further.
At the genus level, the profile of gut microbiota appeared dominated by the abundance of Faecalibacterium, Agathobacter, Roseburia, Subdoligranulum, and Bacteroidetes. The relactive ahundnce of Faecalibacterium (phylum Firmicutes) showed reduced abundance in RA patients compared to HCs. Faecalibacterium produces butyrate, which maintains the integrity of the intestinal epithelial cell layer (Khan et al., 2012). Collinsella was also increased in RA patients. A recent study found that decreased Faecalibacterium and increased Collinsella abundance may enhance epithelial permeability, resulting in the entry of microbial products into the lamina propria and subepithelial space (Chen et al., 2016). Changes in the composition of gut microbiota are tightly linked with host immune status and local inflammation (Faber and Bäumler, 2014; Lee and Ko, 2016; Katayama et al., 2019). Bacteroides, which was decreased in RA patients compared to HCs, has been implicated in chronic inflammation and may cause osteomyelitis in RA (Sun et al., 2019). On the other hand, Blautia and Lachnoclostridium were increased in RA patients; Lachnoclostridium may contribute to the progression of inflammatory arthritis. Dialister has been linked to antidepressants drug along with Coprococcus and butyrate-producing Faecalibacterium. Whether there is reciprocal interaction in preventing autoimmune diseases requires further study. Above all, a correlation may exist between the abundance of these bacteria and RA patients.
The functions of gut microbiota showing altered abundance in RA patients included ABC transporters, glycolysis/gluconeogenesis, and NOD-like receptor signaling. ABC transporters enable the passage of endogenous and xenobiotic compounds through the cell membrane, which may be related to the development of rheumatic disease resistance and disease activity (Atisha-Fregoso et al., 2016). ABC transporters were shown to induce C-X-C chemokine receptor (CXCR) 4 overexpression in B cells and the production of various inflammatory cytokines such as TNF and IL-6 (Tsujimura et al., 2018). Glycolysis/gluconeogenesis plays an important role in maintaining adequate sugar sources (Cheng et al., 2017). The activation of NOD-like receptor signaling modulates the innate immune response and inflammation (Franca et al., 2016; Cheng et al., 2017; Root-Bernstein, 2020). On the other hand, steroid hormone biosynthesis (Cutolo et al., 2004) and p53 signaling were decreased in RA. Steroid hormone-related gene polymorphisms have been linked to bone erosion in rheumatoid arthritis (Sánchez-Maldonado et al., 2019). The p53 signaling pathway mediates the cellular response to stress, cell cycle, DNA repair, senescence, and apoptosis (Hou et al., 2016; Zhang et al., 2020). Bacteroides abundance was reduced in RA patients compared to HCs, suggesting that Bacteroides contributes to disease pathogenesis through the release of bioactive molecules such as butanoate, dicarboxylate, and glyoxylate (Wang et al., 2021). Notably, there was a close correlation between metabolic pathways and some genera (Blautia, Escherichia-Shigella, Proteobacteria, Coprococcus, and Verrucomicrobiota).
Intestinal microbiota are closely related to the immune profile of RA (Hu et al., 2017; Zhang et al., 2018; Li H. et al., 2021). Lactobacillus casei has been shown to alleviate arthritis by altering Treg/Th17 balance and modulating gut microbiota abundance and the plasma metabolome (Jhun et al., 2020; Fan et al., 2021). Verrucomicrobia (Akkermansia muciniphila) plays an anti-inflammatory role by regulating Treg differentiation and enhancing the production of short-chain fatty acids (Zhai et al., 2019). The study about Verrucomicrobia in RA is scarce, our finding may provide a new clue for the function of Verrucomicrobia in disease causation. It was reported that Firmicutes-rich suppresses inflammation and Th17 pathways (Natividad et al., 2015), suggesting that it has therapeutic potential in ulcerative colitis. Bacteroides fragilis and Faecalibacterium prausnitzii induce the secretion of IL-10 by CD4+ T cells (Mazmanian et al., 2005; Sokol et al., 2008). Gut dysbiosis caused by segmented filamentous bacteria may reduce the number of anti-inflammatory Tregs and increase the risk of autoimmunity (Scher et al., 2013). IL-10 is an anti-inflammatory cytokine secreted by various lymphocytes including Th1, Th2, and Th17 cells and Tregs (Mollazadeh et al., 2019). The dysregulation of IL-10 has been reported in various inflammatory diseases such as lupus, arthritis, and psoriasis. In our study, IL-10 level and Th1 and Th2 counts were positively correlated with Megamonas and Monoglobus abundance; meanwhile, the relative abundance of Prevotella and Monoglobus was positively correlated with the absolute number of Th1 and Th2 cells and Il-4, IL-2, IL-10, TNF-α, and IFN-γ levels. Improving the balance of gut microbiota with Blautia along with Bifidobacterium and Ruminococcus was shown to alleviate inflammation by suppressing Tregs (Picchianti-Diamanti et al., 2018). Our results speculate that gut microorganisms influence RA by modulating the immune system. This requires further studying.
We found that the abundance of Escherichia-Shigella, Monoglobus, and Lachnospira increased while that of Intestinibacter and Prevotella decreased with disease activity in RA. An increase in RA disease activity was shown to be associated with elevated levels of TNF-α and IFN-γ, a higher Th17 count, and altered Treg balance (i.e., Th17/Treg and Th2/Treg ratios) (Edavalath et al., 2016). IL-6, IL-17, and IL-10 levels were higher in RA patients than in HCs and IL-17 and IL-10 were positively correlated with DAS28 score, suggesting that these cytokines can serve as markers for disease outcome and inflammatory response (Ge et al., 2015; Marwa et al., 2017; Dhaouadi et al., 2018). In our study, IL-6 level was significantly higher in RA patients with a high DAS28 score compared to those with lower scores. Meanwhile, high IL-6 level was shown to be an independent risk factor for high disease activity in RA, as reflected by the DAS28 score (Park et al., 2016), and alters the Treg/Th17 balance by downregulating IL-6 (Samson et al., 2012). These results suggest that regulating cytokine levels and lymphocyte subpopulations may be an effective strategy in controlling disease activity in RA.
Conclusion
The results of our study demonstrate that gut microbiota play an important role in the pathogenesis of RA, as evidenced by the observed correlations between gut microbiota and CD4+ T cell counts, cytokine levels, and disease activity in RA patients compared to HCs. We found that gut microbiota composition was altered in RA, which was primarily associated with changes in metabolic signaling that could contribute to imbalances in CD4+ T cell subpopulations and cytokines and affect disease activity. These findings provide novel insight into the possible pathogenic mechanisms of RA and suggest that the disease can potentially be regulated by affecting CD4+ T cell subpopulations and cytokines through regulation of the gut microbiome profile.
Data Availability Statement
The original contributions presented in the study are included in the article/supplementary material, further inquiries can be directed to the corresponding author/s.
Ethics Statement
The studies involving human participants were reviewed and approved by The Second Affiliated Hospital of Shanxi Medical University. The patients/participants provided their written informed consent to participate in this study.
Author Contributions
QW designed the study and wrote the manuscript. S-XZ, M-JC, and JQ performed the experiments. QW and JQ analyzed the data. C-HW, X-FL, QY, and P-FH contributed to manuscript revision, read, and approved the submitted version. All authors contributed to the article and approved the submitted version.
Funding
This work was supported by the National Natural Science Foundation of China (Grant No. 82001740), the National Social Science Fund of China (Grant No. 21BTQ050), and Key R&D Program of Shanxi Province (201903D311011).
Conflict of Interest
The authors declare that the research was conducted in the absence of any commercial or financial relationships that could be construed as a potential conflict of interest.
Publisher’s Note
All claims expressed in this article are solely those of the authors and do not necessarily represent those of their affiliated organizations, or those of the publisher, the editors and the reviewers. Any product that may be evaluated in this article, or claim that may be made by its manufacturer, is not guaranteed or endorsed by the publisher.
Acknowledgments
We thank the institutional ethics committee of The Second Affiliated Hospital of Shanxi Medical University for providing samples.
References
Abdulla, O. A., Neamah, W., Sultan, M., Alghetaa, H. K., Singh, N., Busbee, P. B., et al. (2021). The Ability of AhR Ligands to Attenuate Delayed Type Hypersensitivity Reaction Is Associated With Alterations in the Gut Microbiota. Front. Immunol. 12:684727. doi: 10.3389/fimmu.2021.684727
Ahmad, A., Yang, W., Chen, G., Shafiq, M., Javed, S., Ali Zaidi, S. S., et al. (2019). Analysis of gut microbiota of obese individuals with type 2 diabetes and healthy individuals. PLoS One 14:e0226372. doi: 10.1371/journal.pone.0226372
Anuradha, R., Munisankar, S., Bhootra, Y., Dolla, C., Kumaran, P., Nutman, T. B., et al. (2017). Modulation of Mycobacterium tuberculosis-specific humoral immune responses is associated with Strongyloides stercoralis co-infection. PLoS Negl. Trop. Dis. 11:e0005569. doi: 10.1371/journal.pntd.0005569
Atisha-Fregoso, Y., Lima, G., Pascual-Ramos, V., Baños-Peláez, M., Fragoso-Loyo, H., Jakez-Ocampo, J., et al. (2016). Rheumatoid Arthritis Disease Activity Is Determinant for ABCB1 and ABCG2 Drug-Efflux Transporters Function. PLoS One 11:e0159556. doi: 10.1371/journal.pone.0159556
Chen, J., Li, J., Gao, H., Wang, C., Luo, J., Lv, Z., et al. (2012). Comprehensive evaluation of different T-helper cell subsets differentiation and function in rheumatoid arthritis. J. Biomed. Biotechnol. 2012:535361. doi: 10.1155/2012/535361
Chen, J., Wright, K., Davis, J. M., Jeraldo, P., Marietta, E. V., Murray, J., et al. (2016). An expansion of rare lineage intestinal microbes characterizes rheumatoid arthritis. Genome Med. 8:43. doi: 10.1186/s13073-016-0299-7
Chen, Z., Wong, P. Y., Ng, C. W. K., Lan, L., Fung, S., Li, J. W., et al. (2020). The Intersection between Oral Microbiota, Host Gene Methylation and Patient Outcomes in Head and Neck Squamous Cell Carcinoma. Cancers 12:3425. doi: 10.3390/cancers12113425
Cheng, B., Zheng, H., Wu, F., Wu, J., Liu, X., Tang, C., et al. (2017). Metabolomics analysis of Danggui Sini decoction on treatment of collagen-induced arthritis in rats. J. Chromatogr. B Analyt. Technol. Biomed. Life Sci. 1061-1062, 282–291. doi: 10.1016/j.jchromb.2017.07.043
Chu, H., and Mazmanian, S. K. (2013). Innate immune recognition of the microbiota promotes host-microbial symbiosis. Nat. Immunol. 14, 668–675. doi: 10.1038/ni.2635
Cutolo, M., Villaggio, B., Seriolo, B., Montagna, P., Capellino, S., Straub, R. H., et al. (2004). Synovial fluid estrogens in rheumatoid arthritis. Autoimmun. Rev. 3, 193–198. doi: 10.1016/j.autrev.2003.08.003
Dhaouadi, T., Chahbi, M., Haouami, Y., Sfar, I., Abdelmoula, L., Ben Abdallah, T., et al. (2018). IL-17A, IL-17RC polymorphisms and IL17 plasma levels in Tunisian patients with rheumatoid arthritis. PLoS One 13:e0194883. doi: 10.1371/journal.pone.0194883
Douglas, G. M., Maffei, V. J., Zaneveld, J. R., Yurgel, S. N., Brown, J. R., Taylor, C. M., et al. (2020). PICRUSt2 for prediction of metagenome functions. Nat. Biotechnol. 38, 685–688. doi: 10.1038/s41587-020-0548-6
Edavalath, S., Singh, A., Soni, N., Mohindra, N., Kumar, S., and Misra, R. (2016). Peripheral blood T helper type 17 frequency shows an inverse correlation with disease activity and magnetic resonance imaging-based osteitis and erosions in disease-modifying anti-rheumatic drug- and steroid-naive established rheumatoid arthritis. Clin. Exp. Immunol. 186, 313–320. doi: 10.1111/cei.12860
England, B. R., Thiele, G. M., Anderson, D. R., and Mikuls, T. R. (2018). Increased cardiovascular risk in rheumatoid arthritis: mechanisms and implications. BMJ 361:k1036. doi: 10.1136/bmj.k1036
Faber, F., and Bäumler, A. J. (2014). The impact of intestinal inflammation on the nutritional environment of the gut microbiota. Immunol. Lett. 162, 48–53. doi: 10.1016/j.imlet.2014.04.014
Fan, Z., Ross, R. P., Stanton, C., Hou, B., Zhao, J., Zhang, H., et al. (2021). Lactobacillus casei CCFM1074 Alleviates Collagen-Induced Arthritis in Rats via Balancing Treg/Th17 and Modulating the Metabolites and Gut Microbiota. Front. Immunol. 12:680073. doi: 10.3389/fimmu.2021.680073
Franca, R., Vieira, S. M., Talbot, J., Peres, R. S., Pinto, L. G., Zamboni, D. S., et al. (2016). Expression and activity of NOD1 and NOD2/RIPK2 signalling in mononuclear cells from patients with rheumatoid arthritis. Scand. J. Rheumatol. 45, 8–12. doi: 10.3109/03009742.2015.1047403
Frisbee, A. L., Saleh, M. M., Young, M. K., Leslie, J. L., Simpson, M. E., Abhyankar, M. M., et al. (2019). IL-33 drives group 2 innate lymphoid cell-mediated protection during Clostridium difficile infection. Nat. Commun. 10:2712. doi: 10.1038/s41467-019-10733-9
Gabriel, B., Medin, C., Alves, J., Nduati, R., Bosire, R. K., Wamalwa, D., et al. (2019). Analysis of the TCR Repertoire in HIV-Exposed but Uninfected Infants. Sci. Rep. 9:11954. doi: 10.1038/s41598-019-48434-4
Ge, L., Huang, Y., Zhang, H., Liu, R., and Xu, N. (2015). Association between polymorphisms of interleukin 10 with inflammatory biomarkers in East Chinese Han patients with rheumatoid arthritis. Joint Bone Spine 82, 182–186. doi: 10.1016/j.jbspin.2014.11.007
Hou, J., Ouyang, Y., Deng, H., Chen, Z., Song, B., Xie, Z., et al. (2016). Whole-Genome Expression Analysis and Signal Pathway Screening of Synovium-Derived Mesenchymal Stromal Cells in Rheumatoid Arthritis. Stem Cells Int. 2016:1375031. doi: 10.1155/2016/1375031
Hu, L., Geng, S., Li, Y., Cheng, S., Fu, X., Yue, X., et al. (2017). Exogenous Fecal Microbiota Transplantation from Local Adult Pigs to Crossbred Newborn Piglets. Front. Microbiol. 8:2663. doi: 10.3389/fmicb.2017.02663
Jeong, Y., Kim, J. W., You, H. J., Park, S. J., Lee, J., Ju, J. H., et al. (2019). Gut Microbial Composition and Function Are Altered in Patients with Early Rheumatoid Arthritis. J. Clin. Med. 8:693. doi: 10.3390/jcm8050693
Jhun, J., Min, H. K., Ryu, J., Lee, S. Y., Ryu, J. G., Choi, J. W., et al. (2020). Lactobacillus sakei suppresses collagen-induced arthritis and modulates the differentiation of T helper 17 cells and regulatory B cells. J. Transl. Med. 18:317. doi: 10.1186/s12967-020-02477-8
Kasselman, L. J., Vernice, N. A., DeLeon, J., and Reiss, A. B. (2018). The gut microbiome and elevated cardiovascular risk in obesity and autoimmunity. Atherosclerosis 271, 203–213. doi: 10.1016/j.atherosclerosis.2018.02.036
Katayama, Y., Yamada, T., Tanimura, K., Yoshimura, A., Takeda, T., Chihara, Y., et al. (2019). Impact of bowel movement condition on immune checkpoint inhibitor efficacy in patients with advanced non-small cell lung cancer. Thorac. Cancer 10, 526–532. doi: 10.1111/1759-7714.12969
Khan, M. T., Duncan, S. H., Stams, A. J., van Dijl, J. M., Flint, H. J., and Harmsen, H. J. (2012). The gut anaerobe Faecalibacterium prausnitzii uses an extracellular electron shuttle to grow at oxic-anoxic interphases. ISME J. 6, 1578–1585. doi: 10.1038/ismej.2012.5
Kojima, A., Tanaka-Kojima, Y., Sakakura, T., and Nishizuka, Y. (1976). Prevention of postthymectomy autoimmune thyroiditis in mice. Lab. Invest. 34, 601–605.
Lee, H., and Ko, G. (2016). Antiviral effect of vitamin A on norovirus infection via modulation of the gut microbiome. Sci. Rep. 6:25835. doi: 10.1038/srep25835
Lee, N., and Kim, W. U. (2017). Microbiota in T-cell homeostasis and inflammatory diseases. Exp. Mol. Med. 49:e340. doi: 10.1038/emm.2017.36
Li, B., Guo, Q., Wang, Y., Su, R., Gao, C., Zhao, J., et al. (2020). Increased Serum Interleukin-2 Levels Are Associated with Abnormal Peripheral Blood Natural Killer Cell Levels in Patients with Active Rheumatoid Arthritis. Mediators Inflamm. 2020:6108342. doi: 10.1155/2020/6108342
Li, H., Li, S., Fan, S., Xu, Y., and Tian, X. (2021). Profiling intestinal microbiota of Metaplax longipes and Helice japonica and their co-occurrence relationships with habitat microbes. Sci. Rep. 11:21217. doi: 10.1038/s41598-021-00810-9
Li, Y., Zhang, S. X., Yin, X. F., Zhang, M. X., Qiao, J., Xin, X. H., et al. (2021). The Gut Microbiota and Its Relevance to Peripheral Lymphocyte Subpopulations and Cytokines in Patients with Rheumatoid Arthritis. J. Immunol. Res. 2021:6665563. doi: 10.1155/2021/6665563
Li, M., and Wang, F. (2021). Role of Intestinal Microbiota on Gut Homeostasis and Rheumatoid Arthritis. J. Immunol. Res. 2021:8167283. doi: 10.1155/2021/8167283
Li, S., Wang, Z., Yang, Y., Yang, S., Yao, C., Liu, K., et al. (2017). Lachnospiraceae shift in the microbial community of mice faecal sample effects on water immersion restraint stress. AMB Express 7:82. doi: 10.1186/s13568-017-0383-4
Liang, C., Tseng, H. C., Chen, H. M., Wang, W. C., Chiu, C. M., Chang, J. Y., et al. (2017). Diversity and enterotype in gut bacterial community of adults in Taiwan. BMC Genomics 18:932. doi: 10.1186/s12864-016-3261-6
Maeda, Y., and Takeda, K. (2019). Host-microbiota interactions in rheumatoid arthritis. Exp. Mol. Med. 51, 1–6. doi: 10.1038/s12276-019-0283-6
Marwa, O. S., Kalthoum, T., Wajih, K., and Kamel, H. (2017). Association of IL17A and IL17F genes with rheumatoid arthritis disease and the impact of genetic polymorphisms on response to treatment. Immunol. Lett. 183, 24–36. doi: 10.1016/j.imlet.2017.01.013
Mazmanian, S. K., Liu, C. H., Tzianabos, A. O., and Kasper, D. L. (2005). An immunomodulatory molecule of symbiotic bacteria directs maturation of the host immune system. Cell 122, 107–118. doi: 10.1016/j.cell.2005.05.007
Mollazadeh, H., Cicero, A. F. G., Blesso, C. N., Pirro, M., Majeed, M., and Sahebkar, A. (2019). Immune modulation by curcumin: the role of interleukin-10. Crit. Rev. Food Sci. Nutr. 59, 89–101. doi: 10.1080/10408398.2017.1358139
Muraoka, W. T., Granados, J. C., Gomez, B. I., Nicholson, S. E., Chung, K. K., Shupp, J. W., et al. (2020). Burn resuscitation strategy influences the gut microbiota-liver axis in swine. Sci. Rep. 10:15655. doi: 10.1038/s41598-020-72511-8
Natividad, J. M., Pinto-Sanchez, M. I., Galipeau, H. J., Jury, J., Jordana, M., Reinisch, W., et al. (2015). Ecobiotherapy Rich in Firmicutes Decreases Susceptibility to Colitis in a Humanized Gnotobiotic Mouse Model. Inflamm. Bowel Dis. 21, 1883–1893. doi: 10.1097/mib.0000000000000422
Niu, H. Q., Yuan, C., Yan, C., Li, N., Lei, Y. S., Li, X., et al. (2021). Decreased numbers and sex-based differences of circulating regulatory T cells in patients with seropositive undifferentiated arthritis. Ther. Adv. Chronic Dis. 12:2040622320986721. doi: 10.1177/2040622320986721
Niu, H. Q., Zhao, X. C., Li, W., Xie, J. F., Liu, X. Q., Luo, J., et al. (2020). Characteristics and reference ranges of CD4(+)T cell subpopulations among healthy adult Han Chinese in Shanxi Province, North China. BMC Immunol. 21:44. doi: 10.1186/s12865-020-00374-9
Ott, B. M., Rickards, A., Gehrke, L., and Rio, R. V. (2014). Characterization of shed medicinal leech mucus reveals a diverse microbiota. Front. Microbiol. 5:757. doi: 10.3389/fmicb.2014.00757
Park, Y. J., Yoo, S. A., Kim, G. R., Cho, C. S., and Kim, W. U. (2016). Urinary interleukin-6 as a predictor of radiographic progression in rheumatoid arthritis: A 3-year evaluation. Sci. Rep. 6:35242. doi: 10.1038/srep35242
Picchianti-Diamanti, A., Panebianco, C., Salemi, S., Sorgi, M. L., Di Rosa, R., Tropea, A., et al. (2018). Analysis of Gut Microbiota in Rheumatoid Arthritis Patients: disease-Related Dysbiosis and Modifications Induced by Etanercept. Int. J. Mol. Sci. 19:2938. doi: 10.3390/ijms19102938
Ramadan, M., Hetta, H. F., Saleh, M. M., Ali, M. E., Ahmed, A. A., and Salah, M. (2021). Alterations in skin microbiome mediated by radiotherapy and their potential roles in the prognosis of radiotherapy-induced dermatitis: a pilot study. Sci. Rep. 11:5179. doi: 10.1038/s41598-021-84529-7
Root-Bernstein, R. (2020). Synergistic Activation of Toll-Like and NOD Receptors by Complementary Antigens as Facilitators of Autoimmune Disease: review, Model and Novel Predictions. Int. J. Mol. Sci. 21:4645. doi: 10.3390/ijms21134645
Samson, M., Audia, S., Janikashvili, N., Ciudad, M., Trad, M., Fraszczak, J., et al. (2012). Brief report: inhibition of interleukin-6 function corrects Th17/Treg cell imbalance in patients with rheumatoid arthritis. Arthritis Rheum. 64, 2499–2503. doi: 10.1002/art.34477
Sánchez-Maldonado, J. M., Cáliz, R., Canet, L., Horst, R. T., Bakker, O., den Broeder, A. A., et al. (2019). Steroid hormone-related polymorphisms associate with the development of bone erosions in rheumatoid arthritis and help to predict disease progression: results from the REPAIR consortium. Sci. Rep. 9:14812. doi: 10.1038/s41598-019-51255-0
Scher, J. U., Sczesnak, A., Longman, R. S., Segata, N., Ubeda, C., Bielski, C., et al. (2013). Expansion of intestinal Prevotella copri correlates with enhanced susceptibility to arthritis. Elife 2:e01202. doi: 10.7554/eLife.01202
Sokol, H., Pigneur, B., Watterlot, L., Lakhdari, O., Bermúdez-Humarán, L. G., Gratadoux, J. J., et al. (2008). Faecalibacterium prausnitzii is an anti-inflammatory commensal bacterium identified by gut microbiota analysis of Crohn disease patients. Proc. Natl. Acad. Sci. U. S. A. 105, 16731–16736. doi: 10.1073/pnas.0804812105
Suárez-Moo, P., Cruz-Rosales, M., Ibarra-Laclette, E., Desgarennes, D., Huerta, C., and Lamelas, A. (2020). Diversity and Composition of the Gut Microbiota in the Developmental Stages of the Dung Beetle Copris incertus Say (Coleoptera, Scarabaeidae). Front. Microbiol. 11:1698. doi: 10.3389/fmicb.2020.01698
Sun, Y., Chen, Q., Lin, P., Xu, R., He, D., Ji, W., et al. (2019). Characteristics of Gut Microbiota in Patients With Rheumatoid Arthritis in Shanghai, China. Front. Cell. Infect. Microbiol. 9:369. doi: 10.3389/fcimb.2019.00369
Taneja, V. (2014). Arthritis susceptibility and the gut microbiome. FEBS Lett. 588, 4244–4249. doi: 10.1016/j.febslet.2014.05.034
Tsujimura, S., Adachi, T., Saito, K., Kawabe, A., and Tanaka, Y. (2018). Relevance of P-glycoprotein on CXCR4(+) B cells to organ manifestation in highly active rheumatoid arthritis. Mod. Rheumatol. 28, 276–286. doi: 10.1080/14397595.2017.1341458
Vaahtovuo, J., Munukka, E., Korkeamäki, M., Luukkainen, R., and Toivanen, P. (2008). Fecal microbiota in early rheumatoid arthritis. J. Rheumatol. 35, 1500–1505.
Wang, H., Ong, E., Kao, J. Y., Sun, D., and He, Y. (2021). Reverse Microbiomics: a New Reverse Dysbiosis Analysis Strategy and Its Usage in Prediction of Autoantigens and Virulent Factors in Dysbiotic Gut Microbiomes From Rheumatoid Arthritis Patients. Front. Microbiol. 12:633732. doi: 10.3389/fmicb.2021.633732
Zhai, R., Xue, X., Zhang, L., Yang, X., Zhao, L., and Zhang, C. (2019). Strain-Specific Anti-inflammatory Properties of Two Akkermansia muciniphila Strains on Chronic Colitis in Mice. Front. Cell. Infect. Microbiol. 9:239. doi: 10.3389/fcimb.2019.00239
Zhang, K. X., Ip, C. K., Chung, S. K., Lei, K. K., Zhang, Y. Q., Liu, L., et al. (2020). Drug-resistance in rheumatoid arthritis: the role of p53 gene mutations, ABC family transporters and personal factors. Curr. Opin. Pharmacol. 54, 59–71. doi: 10.1016/j.coph.2020.08.002
Zhang, Q., Li, C., Niu, X., Zhang, Z., Li, F., and Li, F. (2018). An intensive milk replacer feeding program benefits immune response and intestinal microbiota of lambs during weaning. BMC Vet. Res. 14:366. doi: 10.1186/s12917-018-1691-x
Zhang, S. X., Wang, J., Wang, C. H., Jia, R. H., Yan, M., Hu, F. Y., et al. (2021). Low-dose IL-2 therapy limits the reduction in absolute numbers of circulating regulatory T cells in rheumatoid arthritis. Ther. Adv. Musculoskelet. Dis. 13:1759720x211011370. doi: 10.1177/1759720x211011370
Keywords: rheumatoid arthritis, gut microbiota, cytokines, CD4, T cells, immune system diseases, DAS28
Citation: Wang Q, Zhang S-X, Chang M-J, Qiao J, Wang C-H, Li X-F, Yu Q and He P-F (2022) Characteristics of the Gut Microbiome and Its Relationship With Peripheral CD4+ T Cell Subpopulations and Cytokines in Rheumatoid Arthritis. Front. Microbiol. 13:799602. doi: 10.3389/fmicb.2022.799602
Received: 21 October 2021; Accepted: 07 January 2022;
Published: 03 February 2022.
Edited by:
Magdalena Plebanski, RMIT University, AustraliaReviewed by:
Jennifer Carla Boer, RMIT University, AustraliaGislane Lelis Vilela de Oliveira, São Paulo State University, Brazil
Copyright © 2022 Wang, Zhang, Chang, Qiao, Wang, Li, Yu and He. This is an open-access article distributed under the terms of the Creative Commons Attribution License (CC BY). The use, distribution or reproduction in other forums is permitted, provided the original author(s) and the copyright owner(s) are credited and that the original publication in this journal is cited, in accordance with accepted academic practice. No use, distribution or reproduction is permitted which does not comply with these terms.
*Correspondence: Qi Yu, yuqi@sxmu.edu.cn; Pei-Feng He, hepeifeng2006@126.com