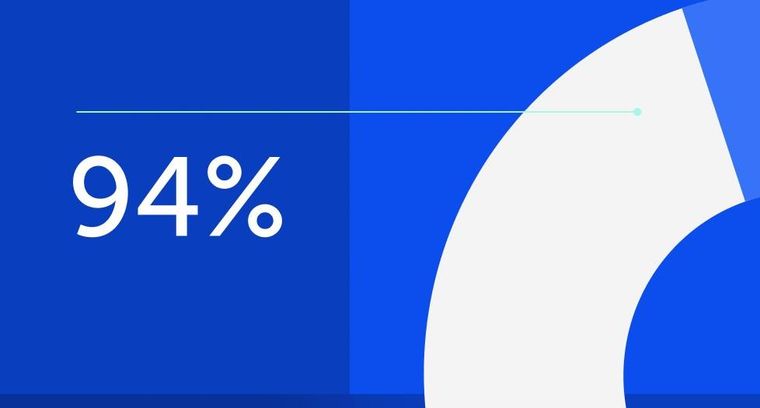
94% of researchers rate our articles as excellent or good
Learn more about the work of our research integrity team to safeguard the quality of each article we publish.
Find out more
ORIGINAL RESEARCH article
Front. Microbiol., 24 February 2022
Sec. Evolutionary and Genomic Microbiology
Volume 13 - 2022 | https://doi.org/10.3389/fmicb.2022.767912
This article is part of the Research TopicEvolution and Genomics of the Mycobacterium tuberculosis complexView all 6 articles
A correction has been applied to this article in:
Corrigendum: In-depth Analysis of IS6110 Genomic Variability in the Mycobacterium tuberculosis Complex
Corrigendum: In-depth Analysis of IS6110 Genomic Variability in the Mycobacterium tuberculosis Complex
The insertion sequence (IS) 6110 is a repetitive mobile element specific for the Mycobacterium tuberculosis complex (MTBC) used for years to diagnose and genotype this pathogen. It contains the overlapping reading frames orfA and orfB that encode a transposase. Its genetic variability is difficult to study because multiple copies are present in the genome. IS6110 is randomly located, nevertheless some preferential locations have been reported, which could be related to the behaviour of the strains. The aim of this work was to determine the intra- and inter-strain genetic conservation of this element in the MTBC. For this purpose, we analysed 158 sequences of IS6110 copies from 55 strains. Eighty-four copies were from 17 strains for which we knew all the locations in their genome. In addition, we studied 74 IS6110 copies in 38 different MTBC strains in which the location was characteristic of different families including Haarlem, LAM, S, and L6 strains. We observed mutation in 13.3% of the copies studied and we found 10 IS6110 variants in 21 copies belonging to 16 strains. The high copy number strains showed 6.2% of their IS6110 copies mutated, in contrast with the 31.1% in the low-copy-number strains. The apparently more ancient copy localised in the DR region was that with more variant copies, probably because this was the most studied location. Notably, all Haarlem and X family strains studied have an IS6110 in Rv0403c, suggesting a common origin for both families. Nevertheless, we detected a variant specific for the X family that would have occurred in this location after the phylogenetic separation. This variant does not prevent transposition although it may occur at a lower frequency, as X strains remain with low copy number (LCN) of IS6110.
The insertion sequence (IS) 6110 has been described as a repetitive mobile element specific for the Mycobacterium tuberculosis complex (MTBC; Thierry et al., 1990). It has been used for years to diagnose and to characterise at the molecular level M. tuberculosis strains by the IS6110-restriction fragment length polymorphism (RFLP) technique (Thierry et al., 1990; Brisson-Noel et al., 1991; Otal et al., 1991). It is 1,355 bp and is flanked by inverted repeats (IR) of 28 bp (Thierry et al., 1990). When transposition occurs, it creates a duplication of 2–4 bp in the insertion site (Mendiola et al., 1992; Dale, 1995). IS6110 contains two overlapping reading frames called orfA and orfB. While the product orfAB is a transposase, the individual products orfA and orfB inhibit transposition, so the proportion of each product is important for the autoregulation of transposition (Sekine et al., 1997). Transcription of this element is regulated by a-1 frameshift due to a ribosomal slippery sequence and by a pseudoknot in the mRNA; these processes lead to a transposition based on a copy-out-paste-in mechanism (Gonzalo-Asensio et al., 2018).
The global M. tuberculosis population can be divided into several phylogeographic lineages (Ls) and families. The genetic diversity found in circulating strains plays an important role in disease outcome. The number of IS6110 copies in the genome is variable and seems to be related to the evolution of the strains. Modern Ls have more IS6110 copies than ancient Ls, and they are considered to be better adapted to high-density populations (Gonzalo-Asensio et al., 2018). It seems that if the IS6110 is inserted in a transcriptionally active region of the genome, the number of copies increases, so the variable number of copies present in different strains could be due to the genomic region in which they are inserted, although a large number of IS6110 copies may be deleterious for the strain because they can remove parts of the genome (Wall et al., 1999).
While IS6110 supposedly transposes randomly, there are some preferential location sites such as the CRISPR region, also known as the DR region in M. tuberculosis; it was probably the first IS copy because it is present in almost all MTBC isolates (Hermans et al., 1991). Other hot spots described are: the plcD gene (Vera-Cabrera et al., 2001), members of the PPE family genes (Sampson et al., 1999; Beggs et al., 2000), the intergenic region dnaA:dnaN (Supply et al., 2006), and other ISs (Fang and Forbes, 1997; Fang et al., 1999). In addition, characteristic locations of IS6110 in different Ls have been observed (Roychowdhury et al., 2015). The function of IS6110 remains unknown although some general effects have been described. The first is the disruption of the gene in which it has been inserted, with a possible deleterious effect (Sampson et al., 1999; Beggs et al., 2000; Warren et al., 2000; Yesilkaya et al., 2005). The second is the recombination and the deletion or inversion of the DNA in between, a phenomenon that can occur when there are two IS relatively close to each other (Sampson et al., 2003). A third effect is that IS6110 can act as a promoter of the downstream gene (Beggs et al., 2000; Safi et al., 2004; Soto et al., 2004).
For the MTBC, the rate of point mutations has been determined to be 1 × 10−9 events per site per generation. However, it has been demonstrated that the rate of point mutations for the IS6110 is about 7.9 × 10−5, higher compared with the rest of the genome, indicating that IS6110 is under selective selection (Tanaka, 2004). There are few studies focused on the sequence genetic variability of this element. Dale et al. (1997) amplified and sequenced IS6110 of four M. tuberculosis strains, each carrying one copy, and one of the copies carried by the Beijing W strain, which has 17 copies. They compared the sequences with the Bacillus Calmette and Guérin (BCG) copy published and found they are identical. Nevertheless, they only checked one copy of each strain. Conversely, Thabet et al. (2015) found several different haplotypes when they randomly cloned and sequenced only one of the IS6110 copies of some characteristic strains from their region.
In this work, we aimed to determine whether IS6110 is a conserved gene, as Dale et al. (1997) suggested or, on the contrary, it is relatively common to find this IS mutated, as Thabet et al. (2015) suggested. In addition to the clinical relevance, since it is widely used for TB diagnosis, we thought mutations of the IS6110 could affect its transposition ability. Therefore, we planned to find out the genetic variability of the sequence. For this purpose, we applied two different approaches. The first was the study of all the IS6110 copies present in the same strain to determine whether they had an identical sequence. For the second approach, a sample collection was used to study IS6110 copies in different MTBC families for which the location had been previously published (Reyes et al., 2012; Comín et al., 2021) to elucidate how conserved IS6110 is among families for the same location.
We selected the available MTBC complete genomes from the NBCI database. First, we checked the entire sequence of the 16 IS6110 copies of H37Rv (NC_000962.3) using the Tuberculist BLAST tool.1 Second, we looked for the IS6110 copies of CDC1551 (AE000516.2), Mycobacterium africanum (CP010334.1 and FR878060.1), Mycobacterium bovis (LT708304.1), and M. bovis BCG (CP033311.1, CP014566.1, and AM408590.1) loaded in the NCBI database. We checked the insertion points using Tuberculist. All the points detailed in the results refer to the H37Rv genome, unless otherwise indicated (Table 1).
Table 1. Summary of IS6110 copies analysed in the strains for which the location of all the IS copies within their genomes is known, detailing the IS copies mutated by comparison with the reference IS in Mycobacterium tuberculosis H37Rv.
All the DNAs used in this work were extracted from clinical M. tuberculosis isolates previously genotyped by IS6110-RFLP and spoligotyping in our laboratory, and stored at −20°C. Strains previously characterised and with all their IS6110 copies located were selected to amplify all the copies within the genome. These strains were: M. tuberculosis Zaragoza (MtZ, L4; Isabel Millan-Lou et al., 2013), GC1237 (L2; Alonso et al., 2011), and seven low copy number (LCN) of IS6110 strains belonging to different lineages. These LCN strains were HMS 2407 and HMS 2382 (L6), HCU 3445 (L4), HMS 2484, HMS 2445, HCU 3717 (Comín et al., 2021; L4 strains belonging to X family), and M. bovis B (hypervirulent strain; Sagasti et al., 2016; Table 1). For the extension of the study (Table 2), we used 32 L4 strains distributed in different families: 10 LAM strains (HMS 18005, HSJ 241, HMS 18045, HMS 18017, HMS 18025, HMS 18010, HMS 18047, HMS 18048, HMS 18018, and ara217, a LAM9 strain studied for producing an outbreak in our region; Comin et al., 2020), 12 Haarlem strains (HSJ 238, HMS 18009, HMS 18021, HMS 18037, HCU 3729, HMS 18031, HMS 18022, HMS 18007, HMS 18046, HSJ 234, HMS 18001, and HMS 18002), one S strain (HMS 18019), and seven T strains (HCU 3718, HMS 18041, HMS 18035, HMS 18042, HMS 18014, HMS 18040, and HMS 18044). In addition, we also included one L3 strain belonging to the CAS1_DELHI family (HMS 18038) and seven M. africanum L6 strains (HCU 2828, HCU 3775, HMS 14017, HMS 2000, HMS 1942, HSJ 66, and HMS 1693; Table 2).
Table 2. Summary of the IS6110 sequences localised in preferential sites published for determined Mycobacterium tuberculosis families (Reyes et al., 2012; Comín et al., 2021).
For the PCR, MyTaq DNA polymerase (Lot No: MT-7171128, Bioline) and 5x MyTaq Reaction Buffer (Lot No: MTB-T17212A, Bioline) were used. We performed an initial denaturation at 95°C for 1 min. Then we performed 35 cycles: denaturation at 95°C for 15 s, annealing at the primer temperature for 15 s, and extension at 72°C for 30 s. The specific primers used for each point of insertion are shown in Supplementary Tables S1–S4. We used a commercial kit (GFX PCR DNA and Gel Band Purification Kit, GE Healthcare, Lot: 16834032) to clean the samples before sequencing. We used the same kit when we needed to extract the DNA from a gel band. The samples were sequenced using capillary electrophoresis with the specific primers flanking the different IS copies and the internal IS primers: IS7 (TTCGGACCACCAGCACCTAACC), IS9 (GCTTTGCCGCGGGTGG), and IScom (ATGTCAGGTGGTTCATCGAGGAGG) in a 3500XL Genetic Analyser (Applied Biosystem).
The sequences obtained were studied using the BioEdit program (Informer Technologies, Inc.), a biological sequence alignment editor. We also used Tuberculist1 and Bovilist,2 where the annotation of M. tuberculosis H37Rv and M. bovis AF2122/97 can be found. We also used the BLAST tool from the NCBI3 to study CDC1551, two M. africanum strains, and three M. bovis BCG strains. Integrative Genomics Viewer (IGV; Robinson et al., 2011) software, which allows the study of the whole genome in an interactive way, was also used.
We aimed to determine the genetic variability of the IS6110 sequence. With this purpose, a large number of copies were analysed. The first approach of the study focused on the comparison of all the IS copies present in a strain to determine whether different copies coexist in the same genome. Initially, we analysed the strains whose genomes were available in the databases. Furthermore, we studied several strains whose IS6110 locations were known as result of our previous studies. For the second approach, we studied IS6110 in specific locations defined for different families, including Haarlem, LAM, S, and L6 strains (Reyes et al., 2012; Comín et al., 2021) to determine its inter- and intra-strain variability using a sample collection. In addition, CAS_DELHI and T strains were included to study the IS copy located in the DR region. As a result, 158 IS6110 sequences from 55 different strains were analysed (Figure 1). Twenty-one of the copies, belonging to 16 strains, had a mutation (13.3%). Among the high copy number strains, seven out of the 113 copies studied (6.2%) were mutated while 14 out of the 45 copies (31.1%) showed a mutation in the LCN strains.
To investigate the IS6110 sequence variability, we checked MTBC strains whose genomes are available in the NCBI and Tuberculist databases: M. tuberculosis H37Rv and CDC1551, M. africanum 25 and GM041182, M. bovis AF2122/97, and three different BCG strains. The results are summarised in Table 1.
Initially, the analysis of the 16 IS6110 copies registered in Tuberculist belonging to the H37Rv strain showed that all the sequences are identical to each other. Therefore, we chose the first copy in its genome, from points 889,020 to 890,375 (Rv0795 and Rv0796), as the reference wild-type IS for the successive comparisons.
Searching the IS6110 copies in the different genomes available in the NCBI database showed several IS copy variants. The CDC1551 strain has four IS6110 copies, located in Rv403c [point 483,296, reverse direction (−)], cut1 (1,989,058, −), DR region (3,120,523:3121879, −), and ppe46 genes [3,377,327, forward direction (+)]. Only the copy in the DR region is wild type, while the other three IS6110 copies share a mutation in the first base of codon 215, within orfB of the IS sequence (G/A, Gly/Ser).
We performed the same procedure for M. africanum 25. We found five copies of IS6110 in its genome, inserted in lipX:mshB (1,300,194, −), Rv1765c (1,998,416, +), DR region (3,120,523:3,121,879, −), moaX (3,709,622, −), and Rv3750c genes (4,198,431, +). Only the copy in Rv3750c showed a gap in nucleotide 110 of orfB. Mycobacterium africanum GM041182 has seven IS6110 copies. Five of them are at the same location and direction as those in strain 25. Nevertheless, the one located in the DR region has a synonymous mutation in the last base of codon 33 (A/G), within orfA of the IS sequence. The previous gap found in strain 25 was not detected. The sixth and seventh copies are inserted in pks8 (1,882,012, +) and Rv3128c:Rv3129 genes (3,494,393, −), both presenting the wild-type sequence.
The single IS6110 copy of M. bovis AF2122/97, inserted in the DR region (3,120,523–3,121,879, −), is wild type. We chose three BCG strains, CP033311.1, CP014566.1, and AM408590.1. All of them have the copy of the DR region inserted at the same point and direction as M. bovis AF2122/97. A second copy of CP014566.1 is upstream the phoP gene (851,590, −). All the copies are wild type.
Using the information obtained in previous studies by our group, we were able to successfully amplify and sequence 47 IS6110 copies from the genomes of three outbreak strains previously characterised (MtZ, GC1237, and M. bovis B) and six LCN strains (HMS 2382, HMS 2407, HCU 3445, HMS 2485, HMS 2445, and HMS 3717). The specific characteristics of these strains can be found in Table 1. The 12 IS6110 copies of the MtZ strain (locations can be found in Supplementary Table S1), the 18 copies of the GC1237 strain (Supplementary Table S2), and the two copies of M. bovis B present the wild-type sequence. Among the analysed sequences of the LCN strains, we found two different mutations. Mycobacterium africanum HMS 2382 has a change in the overlapping region of orfA and orfB in its IS6110 copy within the DR region (−). This mutation (A/G) affects the second amino acid (Asp/Gly) after the ribosomal slippery. We also found a mutation in the IS6110 copies inserted at Rv0403c (−) in HMS 2485, HMS 2445, and HCU 3717 strains, all belonging to X family. This Gly/Ser (G/A) mutation coincides with that found in CDC1551 IS copies (which also belongs to X family). This mutation was also found in three other IS copies of HCU 3717, located in MT1802:cut1 and the DR region (two copies). We were not successful in the amplification of its fifth copy, located in ppe46, but if it was also mutated, HCU 3717 would be the unique strain with all its IS copies mutated. All the other copies from the LCN strains analysed are wild type. The exact point of the mutations found is detailed in the sequence of IS6110 in Figure 2.
Figure 2. Sequence of IS6110, highlighting in different coloured squares the points, the nucleotide changes, and the different strains where the 10 mutations were found. orfA is written in yellow, orfB is in red, and the overlapping region is in green. The black letters at the ends represent the inverted repeats.
To extend the study to other MTBC families and to more strains, we relied on the specific IS6110 locations for Haarlem, LAM, S, and L6 strains detailed in other publications (Reyes et al., 2012; Comín et al., 2021). For the hot spot DR location, we included additional T and CAS_DELHI isolates. The results are shown in Table 2.
According to Reyes et al. (2012), 96% of Haarlem strains have an IS inserted in Rv0403c (483,296, −), Rv2336 (2,610,861, +), and Rv1754c genes (1,986,622, +), and 89% have a copy in Rv0963c (1,075,948, −). We succeeded in the amplification of 10 IS6110 copies in Rv0403c in different strains, five copies in Rv2336 and seven copies in Rv1754c. All of them are wild type. However, we found two different synonymous mutations for the IS copy in Rv0963c in two of the 10 strains analysed. HMS 18031 has a mutation in the last base of codon 174 and HSJ 234 has a mutation in the last base of codon 103, both in orfB. The rest of the copies are identical to the reference IS sequence.
Regarding the LAM family, it has been reported (Reyes et al., 2012) that more than 96% strains have an IS inserted in Rv1754c (1,986,623, −), more than 95% have it inserted in lpqQ:Rv0836c (932,202, −), and more than 90% have it inserted in Rv3113 (3,480,371, +). Based on these findings, we analysed four sequences from the IS inserted in Rv1754c, four sequences from the IS inserted in lpqQ:Rv0836c, and three sequences from the IS inserted in Rv3113. All the sequences are wild type, except the one in lpqQ:Rv0836c of HMS 18025, which has a mutation in the first base of codon 123 after the ribosomal slippery sequence (orfB, Gly/Arg). Furthermore, we studied one more location in MT3426:MT3430 in the context of a LAM strain outbreak investigation (ara217; Comin et al., 2020), successfully amplified for this strain. We also studied this location for the other LAM strains but it was only successfully amplified for one of them. Both present the wild-type genotype.
According to Reyes et al. (2012), more than 85% of S strains have an IS6110 copy in the pks9 gene (1,889,066, −). We studied the IS6110 copy inserted in this location in one S family strain (HMS 18019). This copy is identical to the reference IS sequence.
A recent work (Comín et al., 2021) determined that 100% of the L6 strains have a copy of IS6110 in the moaX gene (3,709,622, −). We analysed five copies for this position, all presenting the wild-type genotype. In the same work, the authors reported that it is common to find a copy between the lipX and mshB genes (1,300,194, −) for L6. We successfully amplified four copies for this location, each showing the wild-type orfA and orfB genes.
Almost all MTBC strains have an IS6110 inserted in the DR region. We amplified the IS of this region for 30 strains. We found three different mutations in five strains (Figure 2). HMS 2382 (L6), HMS 18009, and HMS 18046 (both belonging to the Haarlem family) share a mutation in the second base of codon 2 after the ribosomal slippery (overlapping region, Asp2Gly). HMS 18014 (T5_MAD2 family) has a mutation in the second base of codon 67 (orfA, Thr67Iso). As we have commented above, HCU 3717 (X family) has its two IS copies inserted in the DR region mutated (orfB, Gly215Ser). The rest of the strains studied have the wild-type IS.
Among all the copies studied, we found two different mutations for three strains in the IR of the IS6110 (Figure 2): a SNP in base 6 of the IR flanking orfA, shared by HMS 1693 and HMS 14017 strains (L6) in the copy inserted in lipX:mshB; and a different SNP in base 9 of the IR flanking the orfB, in the copy inserted in Rv0963c of the HMS 18037 Haarlem strain.
PPE regions are hot spots for the IS6110 insertion (Sampson et al., 1999; Beggs et al., 2000), generating a high variability in the genome. In addition, these genes are difficult to amplify because the high number of repetitive regions and a high GC content. Nevertheless, we were successful in the amplification of two IS copies located in ppe38 and ppe71 for the MtZ strain (−) and one copy located between ppe38/ppe71 (+) in Beijing GC1237 (Figure 3). This IS in the Beijing GC1237 strain produced a deletion of esx genes and the truncation of both ppe genes. We were also successful in the amplification of an IS6110 copy located downstream of the ppe46 gene, between the pe27a and esxR genes. In addition, we amplified another IS6110 inserted within ppe34. We observed a different organisation of this gene compared with the H37Rv strain, with short repeated fragments of ppe34 after the IS, a feature that may be typical of the Beijing family (H37Rv does not have these repetitions), as using the BLAST tool of the NCBI; Beijing genomes were the full matches. As we stated before, these copies do not have mutations.
Figure 3. (A) Configuration of the ppe38 locus in the MtZ strain. (B) Configuration of the ppe38 locus in the Beijing GC1237 strain. The red lines indicate a truncation of the gene.
This work deepens the knowledge of the IS6110 sequence in the MTBC. IS6110 has been used for a long time for diagnosis and genotyping, but very little is known about its genetic variability, mainly due to the difficulties in determining its complete sequence for each copy location in the genome. The limited data that have been published regarding this issue have shown dissimilar results (Dale et al., 1997; Thabet et al., 2015).
We have analysed the sequences of both orfA and orfB genes that constitute the transposase in several strains for which we knew its exact location in the genome using specific primers for the amplification of each copy. So far, the only data reported on the sequence of IS6110 were obtained using internal primers that interchangeably amplify all the IS copies of the genome (Thabet et al., 2015), or the number of analysed copies was really low (Dale et al., 1997). In addition, as only one copy of each strain was analysed, the intra-strain variability was not studied. Therefore, the novelty of this work, as far as we know, is that for the first time the IS6110 sequence corresponding to each location has been identified and sequenced, and likewise, the sequences of the different copies in the same strain or among families have been compared. Furthermore, IS6110 cannot be studied directly using the most frequent whole genome sequencing (WGS) technologies, as is the case of Illumina, due to repetitive sequences are not correctly aligned; thus, this study complements this handicap.
We studied 158 IS6110 sequences from 55 different strains belonging to L2 (Beijing), L3 (CAS_DELHI), L4 (Haarlem, LAM, T, S, and X), L6, and the animal branch, including strains with a high and a LCN of IS6110. There were variations of the wild-type sequence in 21 (13.3%) of the copies belonging to 16 strains (29%). We observed a higher percentage of mutated copies among the LCN strains (31.1 vs. 6.2% in high copy number), which could be related with a less transposition ability. To support this idea, a higher number of copies with a widely strain spectrum should be studied.
We found 10 different mutations (Figure 2). Five of them are located in the orfB of the transposase vs. two in the orfA, findings that seem to indicate that orfB is more susceptible to mutation. A possible explanation could be that the orfB is three times longer than orfA. One mutation is in the overlapping region affecting both ORFs. In addition, two other mutations are in the IR, which we do not expect to be involved in the transposase function as they are outside the coding region. Thabet et al. (2015) also found more polymorphic sites for orfB than for orfA. They suggested that non-synonymous mutations tend to be eliminated because the ancestral IS6110 copy inherited by the MTBC would be functionally optimal. However, we found five out of eight mutations in the coding orfA and orfB that are non-synonymous. The fact that some of them are conserved in different strains supports the idea that those non-synonymous mutations are neutral in these cases, as Thabet et al. (2015) suggested. They described different haplotypes for orfA and orfB, reporting the wild type as the most frequent, similarly to our findings. On the other hand, our results differed from Thabet et al. (2015) in other aspects, including the description of some haplotypes with 1–3 SNPs. This caught our attention because all the variant copies we found have just one SNP. The high variability found by these authors could be partially caused by the different procedures applied to obtain the IS6110 DNA sequence. We used specific primers for each location, what allowed us to sequence directly, for each copy, the DNA obtained from PCR. In contrast, other authors used IS internal primers to amplify, clone and sequence one of the copies of each strain without knowing what copy had been amplified or if the sequence obtained was the sum of several. One of the mutations they found (haplotype 20 of orfB) is Gly215Ser (orfB), the same we found in the X family. Since their collection did not include any X strains, we conclude that this mutation is not restricted to the X family. None of the other mutations obtained matched their results. Our results completely differed from Dale et al. (1997), who did not find any mutation when comparing five IS copies of different M. tuberculosis strains, so they affirmed that the IS has been totally conserved among the MTBC. Surely, the low number of copies they studied caused these differences.
As far as we know, this is the first time that all the IS copies of a strain have been studied. In some cases, we observed that it exists variability among the IS6110 copies of the same strain, finding wild-type and mutated copies at the same time. In addition, we did not find copies with different mutations in the same strain.
Our expertise in genotyping M. tuberculosis by IS6110-RFLP supports the fact, previously described (Gonzalo-Asensio et al., 2018), that modern lineages (L2, L3, and L4) have accumulated more copies of IS6110 in their genomes than ancient lineages (L1, L5, and L6). Modern lineages are better adapted to high-density populations, so it is possible that the number of ISs is related to this success (Gonzalo-Asensio et al., 2018). This eventuality agrees with the obtained results because the two most successful strains in our population, used in this work, are high copy number strains (MtZ and GC1237) and, curiously, all their copies (12 and 18, respectively) are wild type. Remarkably, all the strains of the X family (L4) studied, such as CDC1551; share a characteristic variant in at least one of their IS6110 copies. According to these results, it is possible that this mutation could affect the transposition ability of IS6110, reducing its transposition frequency. Thereby, there would be a greater probability that the wild-type copies transpose, leading to a higher copy number of IS6110 in the genome. This phenomenon could partially explain why the X family strains, belonging to L4, are LCN strains despite being a successful clinical family. Nevertheless, the mutation found in the X family strains did not prevent the transposition, as there are more copies with the same SNP in the same strain. Dale et al. (1997) suggested that the differences observed in the copy number are not related to structural changes in the transposase, but rather to the genomic region in which it is inserted. In view of our results, focusing especially on the X strains, we think that both facts are not mutually exclusive and more than one factor must be affecting its transposition.
It is notable that the Haarlem and X family strains studied have an IS6110 inserted in Rv0403c at the same point and direction (483,296, −), in addition to an identical 3 bp created duplication. This finding suggests a common origin for both families, supporting all the phylogenetic trees in which the X and Haarlem families are closely related (Stucki et al., 2016). All the X strains studied in this work have a mutated copy located in Rv0403c, unlike the Haarlem strains, which lack a mutation. The evolutionary process that is inferred by these results is that the common ancestor of these two families had the IS6110 copies located in the DR region and in the Rv0403c gene. Subsequently, the mutation in the copy located in Rv0403c of the X strains occurred after the phylogenetic separation between the Haarlem and X families, and this mutated copy was the one that transposed and led to the rest of the copies in this family, because all IS6110 copies have the same mutation, except the one located in the DR region. The exception is HCU 3717, which has its two DR copies also mutated, suggesting a different evolutionary process.
Researchers demonstrated that the synthesis of the transposase is regulated post-transcriptionally by the ribosome (Gonzalo-Asensio et al., 2018). orfA finishes on Leu92 (UUA), but the ribosome can make a −1 frameshift and starts orfB with Lys1 (AAA). Considering this, we conclude that the mutation in the IS sequences of the X family strains has changed glycine 215 for serine (orfB). Glycine is the smallest amino acid and the only one that is not chiral. Furthermore, it could provide more flexibility to the protein region. On the contrary, serine is a polar amino acid, uncharged, and is bigger than glycine, a factor that diminishes the flexibility of the protein chain. We believe it is possible that this amino acid change may affect the protein structure but, according to our results, it does not prevent the transposition ability.
Regarding M. africanum, in both NCBI strains one of its IS6110 copies is mutated. While the mutation found in the GM041182 strain is synonymous, strain 25 has a gap in the sequence, which completely alters the reading frame and probably disrupts the transposase. Among the LCN strains we sequenced, isolated in our region, M. africanum HMS 2382 has a non-synonymous mutation in one of its three IS6110 copies. It changes aspartic acid for glycine, an important change because aspartic acid has negative charge and is larger than glycine. Although the Haarlem family is a completely different lineage (L4) than M. africanum (L6), we found that two Haarlem strains (HMS 18009 and HMS 18046) share the same mutation as HMS 2382, in the same nucleotide and in the same IS copy (DR region). In addition, IS6110 has been inserted at exactly the same point in the three strains. As both L6 and L4 are separate phylogenetic branches, it is not likely that they acquired the mutation from a common ancestor; therefore, the mutation could be random. There is one mutation in the IR of the IS6110 for HMS 1693 and HMS 14017 (L6). These two strains seem to be from the same cluster according to their RFLP patterns, a finding that would be supported by this shared mutation. As it is out of the coding region, we do not know if it affects the transposase somehow.
Among the findings in the other MTBC families studied is the mutation in the IS6110 copy of the DR region in HMS 18014 (T family), which changes threonine to isoleucine. Both amino acids have a similar size, but they are in different classification groups. Threonine has a polar lateral chain without a charge, whereas isoleucine has a hydrophobic lateral chain, so the interactions will be different. Notably, the three Haarlem strains studies have a different mutation in the copy located in Rv0963c, one with a mutation in the IR of the IS (HMS 18037) and two with a synonymous mutation in the coding region of the transposase (HSJ 234 and HMS 18031). These mutations seem to be random as they are not the same or conserved among strains. The chance of three different mutations in the same location could be because a high number of these copies were sequenced. HMS 18025 (LAM) has a mutation in the copy inserted in lpqQ:Rv0836c, with a change from glycine to arginine. This is an important structural change because arginine is larger than glycine and has a positive charge. More studies are needed to determine how these mutations affect the transposition ability.
In total, we studied 38 IS copies located in the DR region. We successfully amplified this copy in 30 strains of our collection and examined eight more from the NCBI strains. Among them, we have found three different variants in five strains, described above. This is the copy in which we have found the most mutations, a phenomenon that can be explained because this is the region for which more IS copies have been studied, even though the percentage of mutation is constant with regard to the total mutations found. As almost all MTBC strains have an IS copy in this region, it would be reasonable to think that this copy could have been the first IS6110 that started to transpose and thus has increased its number within the genome (McEvoy et al., 2007). If this is true, we have to assume that mutations in this copy occurred after the transposition, as the other studied copies do not have any mutations. Again, the exception is HCU 3717 (X family), in which all its IS6110 copies, including the two of the DR region, seems to be mutated (in the absence of knowing the genotype of the copy located in ppe46).
Ates et al. (2018a) showed that modern Beijing families have different deletions involving the ppe38 locus, suggesting that these changes may contribute to a higher growth rate in vivo and lung inflammation due to the block of PE_PGRS secretion, rendering some Beijing strains hypervirulent. The most virulent deletion described in their work was the one that disrupts the ppe38 and ppe71 genes with the loss of the esx genes between ppe38 and ppe71. This is the deletion we found in the Beijing GC1237 strain (Figure 3). We found that this IS copy has no direct repeats, which suggests that there has been a recombination event between two IS6110 copies with the removal of the genes between them (Brosch et al., 1999; Sampson et al., 2003). Regarding the MtZ strain, there is an IS6110 within the ppe38 gene and another one in the ppe71 gene. We do not know how the insertion affects both genes. Ates et al. (2018b) demonstrated that a single copy of these ppe genes is enough to support PE_PGRS secretion; therefore, MtZ could have this secretion affected. Several experiments are ongoing to determine whether the secretion of PE_PGRS in the MtZ strain is altered. McEvoy et al. (2009) found IS6110 insertion events in the ppe38 locus in other MTBC families. As we also found this in the MtZ strain, we conclude that it is not something specific to the Beijing family. As we have stated previously, none of the IS6110 copies amplified that are inserted in ppe genes have a mutation.
In summary, we have studied the genetic variability of IS6110. We have analysed the DNA sequence of the different IS6110 copies in the same strain of M. tuberculosis as well as in different MTBC strains. We have observed mutation in 13.3% of the copies studied. Gly215Ser (orfB) mutation seems to be characteristic of the X family. In general, the high copy number strains analysed carry wild-type copies whereas several LCN strains, such as the X family and L6, have one or more of their copies mutated. Some strains share the location as well as mutations in these copies, allowing us in these cases to establish the transposition events that have occurred over time and indicating a close relationship in the evolution of these strains. The detailed study of each of the copies has also allowed us to provide information regarding the evolution of some MTBC families. Many publications have studied the variability among strains of the MTBC caused by changes in the number and location of IS6110. To these, we add the changes produced over time in the sequence itself as one more factor that will probably affect its evolution.
The datasets presented in this study can be found in online repositories. The names of the repository/repositories and accession number(s) can be found at: https://www.ncbi.nlm.nih.gov/; MZ574181–MZ574188.
SS and IO: conceptualised the work. JC: carried out the laboratory experiments and curated the data. JC, IO, and SS: wrote the manuscript. All authors contributed to the article and approved the submitted version.
The author(s) declare financial support was received for the research, authorship, and/or publication of this article. This work was supported by the Carlos III Health Institute (ISCIII), AES2018 program, in the context of a Grant (FIS18/0336), co-financed by European Regional Development Funds of the European Commission: “A way of making Europe.” JC was awarded a scholarship by the Government of Aragon/European Social Fund, “Building Europe from Aragon.”
The authors declare that the research was conducted in the absence of any commercial or financial relationships that could be construed as a potential conflict of interest.
All claims expressed in this article are solely those of the authors and do not necessarily represent those of their affiliated organizations, or those of the publisher, the editors and the reviewers. Any product that may be evaluated in this article, or claim that may be made by its manufacturer, is not guaranteed or endorsed by the publisher.
The Supplementary Material for this article can be found online at: https://www.frontiersin.org/articles/10.3389/fmicb.2022.767912/full#supplementary-material
The authors would like to acknowledge the use of Servicio General de Apoyo a la Investigación-SAI, Universidad de Zaragoza (Servicio de Análisis Microbiológico), and Servicios Científico Técnicos, IACS (Servicio de Secuenciación y Genómica Funcional and Servicio de Biocomputación). We would like to thank the EPIMOLA group for supplying the genotyped bacterial DNA used in this work.
Alonso, H., Aguilo, J. I., Samper, S., Caminero, J. A., Campos-Herrero, M. I., Gicquel, B., et al. (2011). Deciphering the role of IS6110 in a highly transmissible Mycobacterium tuberculosis Beijing strain, GC1237. Tuberculosis 91, 117–126. doi: 10.1016/j.tube.2010.12.007
Ates, L. S., Dippenaar, A., Ummels, R., Piersma, S. R., Van Der Woude, A. D., Van Der Kuij, K., et al. (2018a). Mutations in ppe38 block PE-PGRS secretion and increase virulence of Mycobacterium tuberculosis. Nat. Microbiol. 3, 181–188. doi: 10.1038/s41564-017-0090-6
Ates, L. S., Sayes, F., Frigui, W., Ummels, R., MPM, D., Bottai, D., et al. (2018b). RD5-mediated lack of PE_PGRS and PPE-MPTR export in BCG vaccine strains results in strong reduction of antigenic repertoire but little impact on protection. PLoS Pathog. 14:e1007139. doi: 10.1371/journal.ppat.1007139
Beggs, M. L., Eisenach, K. D., and Cave, M. D. (2000). Mapping of IS6110 insertion sites in two epidemic strains of Mycobacterium tuberculosis. J. Clin. Microbiol. 38, 2923–2928. doi: 10.1128/JCM.38.8.2923-2928.2000
Brisson-Noel, A., Aznar, C., Chureau, C., Nguyen, S., Pierre, C., Bartoli, M., et al. (1991). Diagnosis of tuberculosis by DNA amplification in clinical practice evaluation. Lancet 338, 364–366. doi: 10.1016/0140-6736(91)90492-8
Brosch, R., Philipp, W. J., Stavropoulos, E., Colston, M. J., Cole, S. T., and Gordon, S. V. (1999). Genomic analysis reveals variation between Mycobacterium tuberculosis H37Rv and the attenuated M. tuberculosis H37Ra strain. Infect. Immun. 67, 5768–5774. doi: 10.1128/IAI.67.11.5768-5774.1999
Comín, J., Cebollada, A., Ibarz, D., Viñuelas, J., Vitoria, M. A., Iglesias, M. J., et al. (2021). A whole-genome sequencing study of an X-family tuberculosis outbreak focus on transmission chain along 25 years. Tuberculosis 126:102022. doi: 10.1016/j.tube.2020.102022
Comin, J., Chaure, A., Cebollada, A., Ibarz, D., Viñuelas, J., Vitoria, M. A., et al. (2020). Investigation of a rapidly spreading tuberculosis outbreak using whole-genome sequencing. Infect. Genet. Evol. 81:104184. doi: 10.1016/j.meegid.2020.104184
Comín, J., Monforte, M. L., Samper, S., and Otal, I. (2021). Analysis of Mycobacterium africanum in the last 17 years in Aragon identifies a specific location of IS6110 in lineage 6. Sci. Rep. 11:10359. doi: 10.1038/s41598-021-89511-x
Dale, J. W., Tang, T. H., Wall, S., Zainuddin, Z. F., and Plikaytis, B. (1997). Conservation of IS6110 sequence in strains of Mycobacterium tuberculosis with single and multiple copies. Tuber. Lung Dis. 78, 225–227. doi: 10.1016/S0962-8479(97)90002-2
Fang, Z., Doig, C., Morrison, N., Watt, B., and Forbes, K. J. (1999). Characterization of IS1547, a new member of the IS900 family in the Mycobacterium tuberculosis complex, and its association with IS6110. J. Bacteriol. 181, 1021–1024. doi: 10.1128/JB.181.3.1021-1024.1999
Fang, Z., and Forbes, K. J. (1997). A Mycobacterium tuberculosis IS6110 preferential locus (ipl) for insertion into the genome. J. Clin. Microbiol. 35, 479–481. doi: 10.1128/jcm.35.2.479-481.1997
Gonzalo-Asensio, J., Pérez, I., Aguiló, N., Uranga, S., Picó, A., Lampreave, C., et al. (2018). New insights into the transposition mechanisms of IS6110 and its dynamic distribution between Mycobacterium tuberculosis complex lineages. PLoS Genet. 14:e1007282. doi: 10.1371/journal.pgen.1007282
Hermans, P. W. M., Van Soolingen, D., Bik, E. M., De Haas, P. E. W., Dale, J. W., and Van Embden, J. D. A. (1991). Insertion element IS987 from Mycobacterium bovis BCG IS located in a hot-spot integration region for insertion elements in Mycobacterium tuberculosis complex strains. Infect. Immun. 59, 2695–2705. doi: 10.1128/iai.59.8.2695-2705.1991
Isabel Millan-Lou, M., Isabel López-Calleja, A., Colmenarejo, C., Antonia Lezcano, M., Asunción Vitoria, M., Del Portillo, P., et al. (2013). Global study of is6110 in a successful Mycobacterium tuberculosis strain: clues for deciphering its behavior and for its rapid detection. J. Clin. Microbiol. 51, 3631–3637. doi: 10.1128/JCM.00970-13
McEvoy, C. R. E., Falmer, A. A., van Pittius, N. C. G., Victor, T. C., van Helden, P. D., and Warren, R. M. (2007). The role of IS6110 in the evolution of Mycobacterium tuberculosis. Tuberculosis 87, 393–404. doi: 10.1016/j.tube.2007.05.010
McEvoy, C. R., Van Helden, P. D., Warren, R. M., and Van Pittius, N. C. G. (2009). Evidence for a rapid rate of molecular evolution at the hypervariable and immunogenic Mycobacterium tuberculosis PPE38 gene region. BMC Evol. Biol. 9, 237–221. doi: 10.1186/1471-2148-9-237
Mendiola, M. V., Martin, C., Otal, I., and Gicquel, B. (1992). Analysis of the regions responsible for IS6110 RFLP in a single Mycobacterium tuberculosis strain. Res. Microbiol. 143, 767–772. doi: 10.1016/0923-2508(92)90104-V
Otal, I., Martin, C., Vincent-Levy-Frebault, V., Thierry, D., and Gicquel, B. (1991). Restriction fragment length polymorphism analysis using IS6110 as an epidemiological marker in tuberculosis. J. Clin. Microbiol. 29, 1252–1254. doi: 10.1128/jcm.29.6.1252-1254.1991
Reyes, A., Sandoval, A., Cubillos-Ruiz, A., Varley, K. E., Hernández-Neuta, I., Samper, S., et al. (2012). IS-seq: a novel high throughput survey of in vivo IS6110 transposition in multiple Mycobacterium tuberculosis genomes. BMC Genomics 13:249. doi: 10.1186/1471-2164-13-249
Robinson, J. T., Thorvaldsdóttir, H., Winckler, W., Guttman, M., Lander, E. S., Getz, G., et al. (2011). Integrative genome viewer. Nat. Biotechnol. 29, 24–26. doi: 10.1038/nbt.1754
Roychowdhury, T., Mandal, S., and Bhattacharya, A. (2015). Analysis of IS6110 insertion sites provide a glimpse into genome evolution of Mycobacterium tuberculosis. Sci. Rep. 5, 1–10. doi: 10.1038/srep12567
Safi, H., Barnes, P. F., Lakey, D. L., Shams, H., Samten, B., Vankayalapati, R., et al. (2004). IS6110 functions as a mobile, monocyte-activated promoter in Mycobacterium tuberculosis. Mol. Microbiol. 52, 999–1012. doi: 10.1111/j.1365-2958.2004.04037.x
Sagasti, S., Millán-Lou, M. I., Soledad Jiménez, M., Martín, C., and Samper, S. (2016). In-depth analysis of the genome sequence of a clinical, extensively drug-resistant Mycobacterium bovis strain. Tuberculosis 100, 46–52. doi: 10.1016/j.tube.2016.06.005
Sampson, S. L., Warren, R. M., Richardson, M., van der Spuy, G. D., and van Helden, P. D. (1999). Disruption of coding regions by IS6110 insertion in Mycobacterium tuberculosis. Tuber. Lung Dis. 79, 349–359. doi: 10.1054/tuld.1999.0218
Sampson, S. L., Warren, R. M., Richardson, M., Victor, T. C., Jordaan, A. M., Van der Spuy, G. D., et al. (2003). IS6110-mediated deletion polymorphism in the direct repeat region of clinical isolates of Mycobacterium tuberculosis. J. Bacteriol. 185, 2856–2866. doi: 10.1128/JB.185.9.2856-2866.2003
Sekine, Y., Izumi, K. I., Mizuno, T., Ohtsubo, E., and Ishihama, A. (1997). Inhibition of transpositional recombination by OrfA and OrfB proteins encoded by insertion sequence IS3. Genes Cells 2, 547–557. doi: 10.1046/j.1365-2443.1997.1440342.x
Soto, C. Y., Menéndez, M. C., Pérez, E., Samper, S., Gómez, A. B., García, M. J., et al. (2004). IS6110 mediates increased transcription of the phoP virulence gene in a multidrug-resistant clinical isolate responsible for tuberculosis outbreaks. J. Clin. Microbiol. 42, 212–219. doi: 10.1128/JCM.42.1.212-219.2004
Stucki, D., Brites, D., Jeljeli, L., Coscolla, M., Liu, Q., Trauner, A., et al. (2016). Mycobacterium tuberculosis lineage 4 comprises globally distributed and geographically restricted sublineages. Nat. Genet. 48, 1535–1543. doi: 10.1038/ng.3704
Supply, P., Allix, C., Lesjean, S., Cardoso-Oelemann, M., Rüsch-Gerdes, S., Willery, E., et al. (2006). Proposal for standardization of optimized mycobacterial interspersed repetitive unit-variable-number tandem repeat typing of Mycobacterium tuberculosis. J. Clin. Microbiol. 44, 4498–4510. doi: 10.1128/JCM.01392-06
Tanaka, M. M. (2004). Evidence for positive selection on Mycobacterium tuberculosis within patients. BMC Evol. Biol. 4, 31–38. doi: 10.1186/1471-2148-4-31
Thabet, S., Namouchi, A., and Mardassi, H. (2015). Evolutionary trends of the transposase-encoding open reading frames A and B (orfA and orfB) of the mycobacterial IS6110 insertion sequence. PLoS One 10:e0130161. doi: 10.1371/journal.pone.0130161
Thierry, D., Brisson-Noel, A., Vincent-Levy-Frebault, V., Nguyen, S., Guesdon, J. L., and Gicquel, B. (1990). Characterization of a Mycobacterium tuberculosis insertion sequence, IS6110, and its application in diagnosis. J. Clin. Microbiol. 28, 2668–2673. doi: 10.1128/jcm.28.12.2668-2673.1990
Vera-Cabrera, L., Hernández-Vera, M. A., Welsh, O., Johnson, W. M., and Castro-Garza, J. (2001). Phospholipase region of Mycobacterium tuberculosis is a preferential locus for IS6110 transposition. J. Clin. Microbiol. 39, 3499–3504. doi: 10.1128/JCM.39.10.3499-3504.2001
Wall, S., Ghanekar, K., McFadden, J., and Dale, J. W. (1999). Context-sensitive transposition of IS6110 in mycobacteria. Microbiology 145, 3169–3176. doi: 10.1099/00221287-145-11-3169
Warren, R. M., Sampson, S. L., Richardson, M., Van Der Spuy, G. D., Lombard, C. J., Victor, T. C., et al. (2000). Mapping of IS6110 flanking regions in clinical isolates of Mycobacterium tuberculosis demonstrates genome plasticity. Mol. Microbiol. 37, 1405–1416. doi: 10.1046/j.1365-2958.2000.02090.x
Keywords: tuberculosis, IS6110, Mycobacterium tuberculosis complex, IS6110 genomic variability, tuberculosis evolution
Citation: Comín J, Otal I and Samper S (2022) In-depth Analysis of IS6110 Genomic Variability in the Mycobacterium tuberculosis Complex. Front. Microbiol. 13:767912. doi: 10.3389/fmicb.2022.767912
Received: 31 August 2021; Accepted: 04 February 2022;
Published: 24 February 2022.
Edited by:
Daniel Yero, Universidad Autónoma de Barcelona, SpainReviewed by:
Brosch Roland, Université Louis-Pasteur, FranceCopyright © 2022 Comín, Otal and Samper. This is an open-access article distributed under the terms of the Creative Commons Attribution License (CC BY). The use, distribution or reproduction in other forums is permitted, provided the original author(s) and the copyright owner(s) are credited and that the original publication in this journal is cited, in accordance with accepted academic practice. No use, distribution or reproduction is permitted which does not comply with these terms.
*Correspondence: Jessica Comín, amNvbWluLmlhY3NAYXJhZ29uLmVz
†These authors have contributed equally to this work and share last authorship
Disclaimer: All claims expressed in this article are solely those of the authors and do not necessarily represent those of their affiliated organizations, or those of the publisher, the editors and the reviewers. Any product that may be evaluated in this article or claim that may be made by its manufacturer is not guaranteed or endorsed by the publisher.
Research integrity at Frontiers
Learn more about the work of our research integrity team to safeguard the quality of each article we publish.