- 1Pharmacologie Cellulaire et Moléculaire, Louvain Drug Research Institute, Université catholique de Louvain, Brussels, Belgium
- 2Institute of Hygiene, University Hospital Münster, Münster, Germany
- 3Institute of Medical Microbiology, University Hospital Münster, Münster, Germany
Achromobacter genus (including Achromobacter xylosoxidans, the most prevalent Achromobacter species in patients with cystic fibrosis) is poorly susceptible to most conventional antibiotics. Contribution of efflux by AxyABM, AxyXY-OprZ, and AxyEF-OprN and of target mutations were studied in clinical isolates of A. xylosoxidans and Achromobacter insuavis. Forty-one isolates longitudinally collected from 21 patients with CF were studied by whole-genome sequencing (WGS)-typing, determination of minimum inhibitory concentrations (MICs) of β-lactams, aminoglycosides, colistin, azithromycin, ciprofloxacin, chloramphenicol, and doxycycline, and expression (quantitative RT-PCR) and function (measure of the uptake of a fluorescent substrate) of efflux pumps. WGS-based typing resulted in 10 clusters comprising 2 or 3 isolates and 20 singletons. The efflux activity was high in strains with elevated MICs for amikacin or azithromycin. This work sheds a new light on the impact of efflux and target mutations in resistance of Achromobacter to several drugs.
Introduction
In adults with cystic fibrosis (CF), Pseudomonas aeruginosa is one of the main respiratory pathogens, but in recent years, other non-fermenting Gram-negative bacterial species, such as Stenotrophomonas, Burkholderia, or Achromobacter have been increasingly isolated (Cystic Fibrosis Foundation, 2020). This could be due, collectively, to a better eradication of P. aeruginosa by aggressive therapies, a lengthening of patients’ life expectancy, and the development of new techniques for bacterial identification. Among these bacteria, Achromobacter spp. are ubiquitous environmental microorganisms, also part of the microbiota of the ear and the gastrointestinal tract (Steinberg and Del Rio, 2005). They may become opportunistic pathogens capable of causing a large variety of infections, including endophthalmitis, keratoconjunctivitis, catheter-associated bloodstream infection, endocarditis, pneumonia, meningitis, and peritonitis (Spilker et al., 2012). They are also isolated in patients with CF and cause serious respiratory tract infections (Swenson and Sadikot, 2015; Hoyle et al., 2018). Achromobacter can be found in up to 10% of the sputum samples collected from patients with CF, with A. xylosoxidans being the most prevalent Achromobacter species, identified in 35–80% of the cases (Raidt et al., 2015; Amoureux et al., 2016; Gade et al., 2017; Isler et al., 2020). Its pathogenic role in CF remains unclear, but chronic colonization is associated with a decline in respiratory function (Edwards et al., 2017; Tetart et al., 2019; Marsac et al., 2021) and a higher risk of death or lung transplantation (Somayaji et al., 2017), suggesting the need for an active treatment. At this stage, however, there is no standard treatment protocols for Achromobacter infections in CF, and treatment options need to be selected on a case-by-case basis (Isler et al., 2020).
In a clinical perspective, ceftazidime, meropenem, ciprofloxacin, and colistin are representative of the classes for which EUCAST has published MIC distributions against Achromobacter.1 Extended-spectrum β-lactams also often represent a first option for infections by Achromobacter in patients with CF (Swenson and Sadikot, 2015). Inhaled antibiotics (colistin, or tobramycin) proved useful complements to intravenous drugs (Wang et al., 2013). Tetracyclines and chloramphenicol are among the most active agents in vitro (Saiman et al., 2001). In addition, temocillin is indicated against Burkholderia (Zeiser et al., 2019), and azithromycin is widely used for its anti-virulence and immunomodulatory properties (Cramer et al., 2017), so that patients are also possibly exposed to these drugs even though they have no meaningful activity on Achromobacter.
Antibiotic selection remains a real challenge because Achromobacter displays not only innate but also frequent acquired multidrug resistance to a wide range of antibiotics commonly used for the management of infections by Gram-negative microorganisms (Traglia et al., 2012; Bador et al., 2013; Isler et al., 2020). Unfortunately, the knowledge of drug resistance mechanisms in this genus is limited. Genes located on mobile genetic elements, which encode β-lactamases or aminoglycoside-modifying enzymes or confer fluoroquinolone resistance, have been reported thus far (Hu et al., 2015; Isler et al., 2020) and contribute to acquired resistance. β-Lactamases can be highly diverse, including extended-spectrum (CTX-M, VEB-1) or AmpC-type (CMY-2, AmpC) β-lactamases hydrolyzing all beta-lactams except carbapenems, and plasmidic (IMP and VIM) carbapenemases (Isler et al., 2020). In addition, five predicted β-lactamase genes have been identified in the chromosome, encoding one class D (blaOXA–114), one class C, two class B, and one class A enzymes (Doi et al., 2008).
Another potential resistance mechanism consists in active efflux through AxyABM, AxyXY-OprZ, or AxyEF-OprN pumps, which seem orthologs of MexAB-OprM, MexXY-OprA, and MexEF-OprN in P. aeruginosa, respectively (Bador et al., 2011, 2013; Nielsen et al., 2019; Magallon et al., 2021). The substrate specificity of these pumps and their impact on antibiotic activity is, however, different between these two species.
This study investigated resistance mechanisms in clinical isolates of A. xylosoxidans and the closely related species Achromobacter insuavis. To this effect, a collection of 41 isolates was assembled longitudinally from patients with CF, which allowed us to consider microevolution in specific genes.
Materials and Methods
Isolates, Identification, Whole-Genome Sequencing, and Relatedness
Forty-one successive isolates of Achromobacter spp. from sputum samples of 21 patients with CF and cultures remaining positive over prolonged time periods (0.3–11 years interval between the 2 successive samples; mean value, 4.4 years; Table 1) were collected at the CF centers of the University Hospital and Clemenshospital, Münster, Germany (2006–2017). A. xylosoxidans ATCC 27061 (Yabuuchi and Oyama, 1971) was used as a reference throughout this work, as being one of the few fully sequenced clinical isolates of A. xylosoxidans and for which the three efflux pumps of interest have been functionally characterized among the 6 identified (Hu et al., 2015). In addition, all sequences were compared to those of A. insuavis AXX-A, which shows a wild-type phenotype, in particular regarding its susceptibility to ciprofloxacin (Bador et al., 2011). P. aeruginosa ATCC 27853 (Fang et al., 2012) was also used as internal control for antimicrobial susceptibility testing. Two A. insuavis [Ai: parental clinical isolate, Ai ΔB/ΔY: Ai with deletions in axyB (Bador et al., 2011) or axyY (Bador et al., 2013)] were provided by Dr. Julien Bador, Department of Bacteriology, University Hospital of Dijon, Dijon, France. AX-08 and its deletion mutant in axyE were provided by Niels Norskov-Lauritsen, Aarhus University, Aahrus, Denmark (Nielsen et al., 2019).
Strain relatedness was analyzed and represented by a minimum spanning tree, after whole genome sequencing (WGS). Isolates were compared via WGS-based typing using the Illumina MiSeq platform (Illumina Inc., San Diego, CA) (Dekker and Frank, 2016). After quality trimming, coding core genome regions were compared in a gene-by-gene approach (core genome multilocus sequence typing, cgMLST) using the SeqSphere + software version 6.0.2 (Ridom GmbH, Münster, Germany) and A. xylosoxidans ATCC 27061 within an ad hoc scheme as a reference sequence (GenBank accession number LN831029.1). SeqSphere + software was used to display the clonal relationship in a minimum-spanning tree. Threshold defining close genetic relation was set after plotting allelic changes over time of strains derived from each and the same patient. Species identification was performed using the nrdA gene data (Spilker et al., 2012). These were first extracted with the help of SeqSphere + from the WGS data in silico and thereafter subjected to nrdA_765 typing available by PubMLST. On the basis of all nrdA genes, a phylogenetic analysis was performed using software MEGA 11 (Tamura et al., 2021) in which the evolutionary history was inferred from a Maximum Likelihood method and General Time Reversible model (Nei and Kumar, 2000) implying 1,000 bootstrap replications. The sequences of rrl encoding 23S rRNA, of rpl4 and rpl22 ribosomal genes and of gyrA, gyrB, parE, and parC were compared to the reference strains AXX-A and ATCC 27061 by pairwise sequence alignment.
Antimicrobial Susceptibility Testing
MICs were determined by broth microdilution according to CLSI guidelines (Clinical and Laboratory Standards Institute, 2020) for the following antibiotics (potency and origin): amikacin (Amukin 500 mg/2 ml for injection, S.A. Bristol-Myers Squibb, Belgium), azithromycin (100%, SMB, Brussels, Belgium), ceftazidime (2 g for IV injection, 72.5%, PAN Pharma, Luitré, France), chloramphenicol (98%, Sigma-Aldrich), ciprofloxacin (98%, Fluka, Sigma-Aldrich), colistin sulfate salt (79.6%, Sigma-Aldrich), doxycycline hyclate (86.6%, Sigma-Aldrich), meropenem (500 mg powder for solution for injection or infusion, 92%, Hospira UK Ltd., Hurley, United Kingdom), piperacillin (94.2%, Sigma-Aldrich, Maryland Heights, MO), temocillin (84%, Eumedica Pharmaceuticals, Manage, Belgium), ticarcillin disodium salt (85.2%, Sigma-Aldrich), and tobramycin (100%, Teva, Wilrijk, Belgium). Tazobactam sodium salt (92.4%, Cubist Pharmaceuticals, Lexington, MA) and avibactam (99.6%, AstraZeneca Pharmaceuticals, Waltham, MA) were used as inhibitors for β-lactamases. Berberine (chloride hydrate, 82.1%; Sigma-Aldrich), known to attenuate the MexXY-OprM/OprA-mediated aminoglycoside resistance in P. aeruginosa (Morita et al., 2016), was used to reduce AxyXY-OprZ activity.
Uptake of N-Phenyl-1-Naphthylamine
The uptake of the lipophilic probe N-phenyl-1-naphthylamine (NPN) was measured following the general methodology described previously (Lomovskaya et al., 2001). In brief, 10 ml of bacterial culture in exponential growth phase (OD620nm 0.6) was harvested by centrifugation (3,000 g; 10 min) and resuspended in buffer (NaCl, 110 mM; KCl, 7 mM; NH4Cl, 40 mM; NA2HPO4, 0.4 mM; Tris base, 52 mM; glucose, 0.2%; pH 7.5 adjusted with HCl). NPN was added at a final concentration of 10 μM, and cells were incubated 10 min at 37°C; then, 200-μl aliquots were dispensed in standard 96-well plates. The fluorescence signal (excitation/emission wavelengths, 340/410 nm) was measured on a Spectramax® multiplate reader (Molecular Devices, Sunnyvale, CA). The protonophore carbonyl cyanide m-chlorophenyl hydrazine (CCCP, 97%, Sigma-Aldrich; final concentration, 100 μM) was used as a positive control; buffer solutions containing 10 μM NPN without cells or cells without NPN were used as blanks.
Phenotypic Screening of β-Lactamases
Cephalosporinase and carbapenemase activity was detected using the ESBL NDP/carba NP test (Nordmann et al., 2012a,b), adapted by using cefotaxime or imipenem (3 mg/ml) as substrates. A. xylosoxidans ATCC 27061 and P. aeruginosa ATCC 27853 were included as negative controls and clinical isolates of P. aeruginosa expressing IMP-13 or VIM-2 metallo-β-lactamases or Klebsiella pneumoniae expressing OXA-48, as positive controls.
Quantification of Efflux Gene Expression
The expression levels of axyB, axyY, and axyF (encoding the inner membrane protein of AxyABM, AxyXY-OprZ, or AxyEF-OprN pumps, respectively) were quantified by real-time PCR, relative to those measured for the reference strain ATCC 27061. RNA was extracted (Invitrap Spin Cell RNA Mini Kit, 1061100300, STRATEC, Birkenfeld, Germany) from log-phase cultures (OD620 nm, around 0.7) and treated by DNase (TURBO DNA-free™ Kit, AM1907, Thermo Fisher Scientific, MA). The absence of DNA contamination was checked by performing a PCR on purified RNA, which did not amplify any residual material. cDNA was synthesized (Transcriptor First Strand cDNA Synthesis Kit, 04379012001, Roche, LifeSciences, Penzberg, Germany). A real-time PCR was performed on a CFX-96 machine (BIORAD, Hercules, CA) using SsoAdvanced™ Universal SYBR® Green Supermix, #1725271, the primers described in Supplementary Table 1, and 16S rRNA as housekeeping gene. Triplicates measurements were repeated in two independent experiments.
Statistical Analyses
Descriptive statistics and graphs were produced using GraphPad Prism version 8.4.3 (GraphPad Software, San Diego, CA) or JMP Pro v.14 (partition tree analysis; SAS Institute Inc., Cary, NC).
Results
Genetic Relation of Strains
The clonal relationship of the 41 isolates (from 21 patients, including 18 pairs/triplets of early/late isolates) was analyzed by WGS typing. Species identification was confirmed via extracted nrdA gene data, resulting in A. xylosoxidans (isolates from patients 1, 2, 4, 5, 6, 7, 8, 9, 11, 12, 13, 14, 17, 18, 19, and 26) or A. insuavis (isolates from patients 3, 10, 15, 16, and 27) according to the nrdA_765 typing scheme (Supplementary Figure 1A). As the isolates of patient 18 (18.1 and 18.3) are distantly related to all known species according to the phylogenetic analysis, these isolates may represent a new species within the genus Achromobacter.
After plotting allele changes in patient isolate pairs per year (range from 0.5 in pairs from patient 12 to 215.5 in pair from 11), the cutoff value for a very close genetic relation resulted in ≤ 20 alleles and for a close genetic relation in ≤ 57 alleles change per year. Results of WGS typing of the 41 Achromobacter isolates were displayed in two minimum spanning trees (MST) separated by species. Considering the allelic differences over time, MST of A. xylosoxidans strains resulted in 12 clusters (Supplementary Figure 1B-A, black borders) comprising 2, 3, or 5 very closely related or closely related isolates and 3 singletons via cgMLST algorithm of 5,778 target genes. Ten clusters harbored strains isolated from one patient and two clusters strains derived from two patients, illuminating the possibility of a patient-to-patient cross-transmission (patients 9 and 19; patients 7 and 26) of A. xylosoxidans. MST of A. insuavis strains resulted in four clusters (Supplementary Figure 1B-B, black borders) comprising two or three very closely related isolates. Three of these clusters harbored strains isolated from one patient, while one cluster included strains from two patients (patients 3 and 27), also giving hint for a patient-to-patient cross-transmission. Both MST reference genomes (GenBank accession numbers LN831029.1 and GCA_003096315.1) were not genetically related to any genotype from patient isolates of this study.
Minimum Inhibitory Concentrations, Resistance Profile
MICs were measured for antibiotics commonly used in CF and belonging to classes described as substrates for efflux in other Gram-negative bacteria (Table 1 for individual values and Table 2 for a summary).
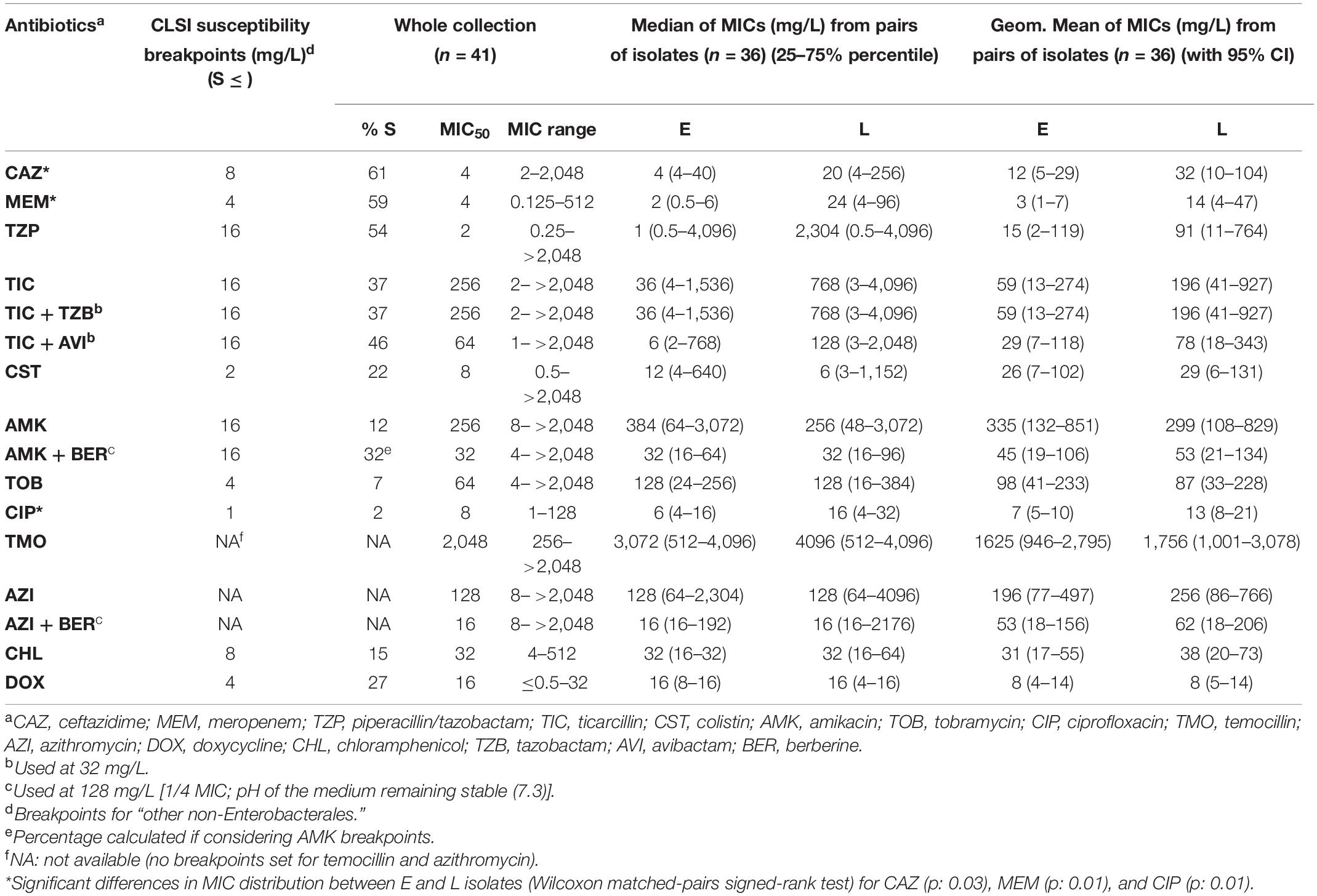
Table 2. Antimicrobial susceptibility to different antibiotics among the 41 clinical isolates [including 18 pairs of successive isolates with 1–11 year’s interval between the early (E) and late (L) sample] of Achromobacter spp.
Susceptibility was higher for β-lactams (37–61%) than for doxycycline (27%), colistin (22%), chloramphenicol (15%), aminoglycosides (7–12%), and ciprofloxacin (2%). High MIC50 were observed for temocillin and azithromycin (no susceptibility breakpoint set). Broad ranges of MIC values were observed for all drugs, and MIC50 were higher than the concentrations reachable in the serum of treated patients, except for ceftazidime, meropenem, and piperacillin/tazobactam. No systematic difference in MICs could be evidenced between strains identified as A. xylosoxidans or A. insuavis, but the number of strains in each species was too small to draw meaningful conclusions in this respect. When comparing longitudinally the MIC distributions in the 18 pairs/triplets of isolates, a significant increase was observed for ceftazidime, meropenem, and ciprofloxacin between early and late isolates (Wilcoxon matched-pairs signed-rank test: p: 0.03, 0.01, p: 0.01, respectively; see the changes in the median and geometric mean values for MICs of all drugs in early and late isolates in Table 2 and the detailed analysis for drugs showing a significant loss in susceptibility between early and late isolates in Figure 1).
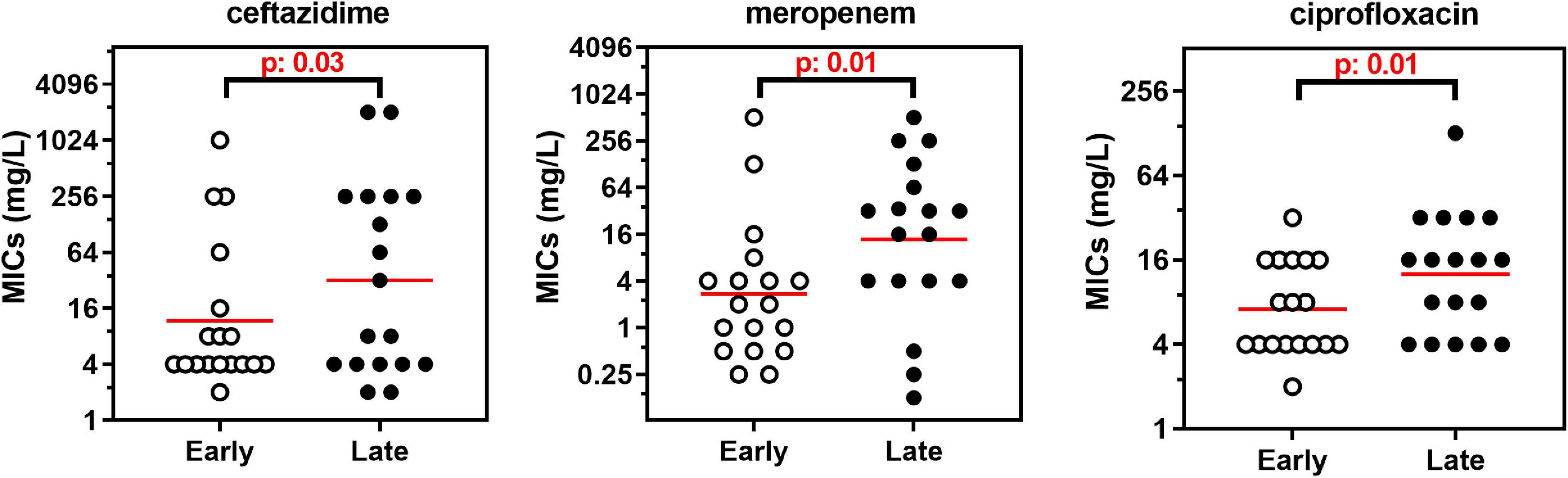
Figure 1. MICs of 3 antibiotics in the 18 pairs of isolates collected successively in the same patients with an interval time ranging from 1 to 11 years. The graphs show the individual MIC for each isolate, with the geometric mean rep-resented by the red horizontal line. Only antibiotics for which a significant difference between the successive isolates (Early vs. late) was observed are illustrated (see Table 1). Statistical analysis: Wilcoxon matched-pairs signed-rank test.
Phenotypic Screening of β-Lactamase Activity
The percentage of susceptibility to ticarcillin (37%; 15/41) was not modified in the presence of tazobactam, but increased to 46% (19/41) in the presence of avibactam (Table 2). Among the 16 isolates resistant to ceftazidime (MICs, 16–2,048 mg/L), MICs were decreased by 2.2 twofold dilutions on average by avibactam, with only one isolate (8.1; MIC, 16 mg/L) regaining susceptibility to ceftazidime (MIC with avibactam, 8 mg/L; Table 1). All isolates were also screened for β-lactamase activity using the NDP/carba NP phenotypic test. Fourteen isolates were displaying cephalosporinase activity (degradation of cefotaxime), among which 12 were resistant to ticarcillin, 7 to piperacillin-tazobactam, to ceftazidime, and 7 to meropenem, respectively (Table 1 for an identification of these isolates). No carbapenemase activity was detected in the whole collection.
Efflux Pumps and Influence on Antibiotic Activity
We first showed a minor impact of deletion of each efflux pumps in reference strains on the MIC of the whole panel of antibiotics (Table 1). We therefore rather examined whether the expression levels of axyB, axyY, and axyF encoding the inner membrane protein of each of the three main RND efflux pumps were variable among isolates. The expression levels of these genes in early and late isolates are compared in Supplementary Figure 2. Supplementary Figure 3 shows that there is a significant correlation between the level of expression of axyB and the MICs of amikacin, azithromycin, and meropenem, between the expression level of axyY and the MICs od amikacin (± berberine), tobramycin, azithromycin, and colistin, and between the expression level of axyF and the MICs of chloramphenicol (see Supplementary Table 2 for statistical analyses).
The MIC of amikacin and azithromycin was then measured in the whole collection in the presence of the MexXY efflux attenuator berberine (Morita et al., 2016). At 1/4 MIC, berberine decreased these MICs, causing a reduction of the MIC50 of 2 and 3 doubling dilutions, respectively (Tables 1, 2). Yet, no changes in the MIC were observed for a few strains with low or high MICs. Thus, amikacin activity remained unaffected by berberine in 3/9 isolates with MIC > 2,048 mg/L (isolates 8.7, 11.1, 11.10) and 1/1 and 1/4 isolates with MIC of 8 and 16 mg/L (isolates 19.5 and 6.14, respectively), respectively (Supplementary Figure 4A). Azithromycin activity was not improved by berberine in 8/12 isolates with MIC > 2,048 mg/L and 6/6 isolates with MIC ≤ 32 mg/L (Supplementary Figure 4B). In four pairs of isolates, the MICs of amikacin and azithromycin changed in parallel between the early and late isolates (late isolate more resistant for patients 9 and 10 or more susceptible for patients 6 and 19; see Table 1), giving us the opportunity to examine the relationship between this change in MIC and the expression levels of axyB or axyY. No systematic correlation was observed between MICs and the expression level of axyB (not shown) but well between MIC values and the expression levels of axyY (Figure 2A). We also evaluated the efflux activity in individual strains by measuring the fluorescence signal associated with the incorporation in bacterial membranes of N-phenyl-1-naphthylamine (NPN), a well-established substrate for efflux (Ocaktan et al., 1997). To validate this approach, we first showed that NPN accumulation was markedly increased in mutants of an A. insuavis reference strain deleted in axyB or axyY and in an A. xylosoxidans reference strain incubated with CCCP as compared to their wild-type counterparts (Figure 2B). NPN fluorescence was then measured in clinical isolates, with data stratified according to antibiotic MICs (Figures 2C–E). Isolates with low MICs for aminoglycosides or azithromycin showed significantly higher NPN accumulation (MIC threshold set at 256, 128, and 64 mg/L for amikacin, tobramycin, and azithromycin, respectively, by partition analysis).
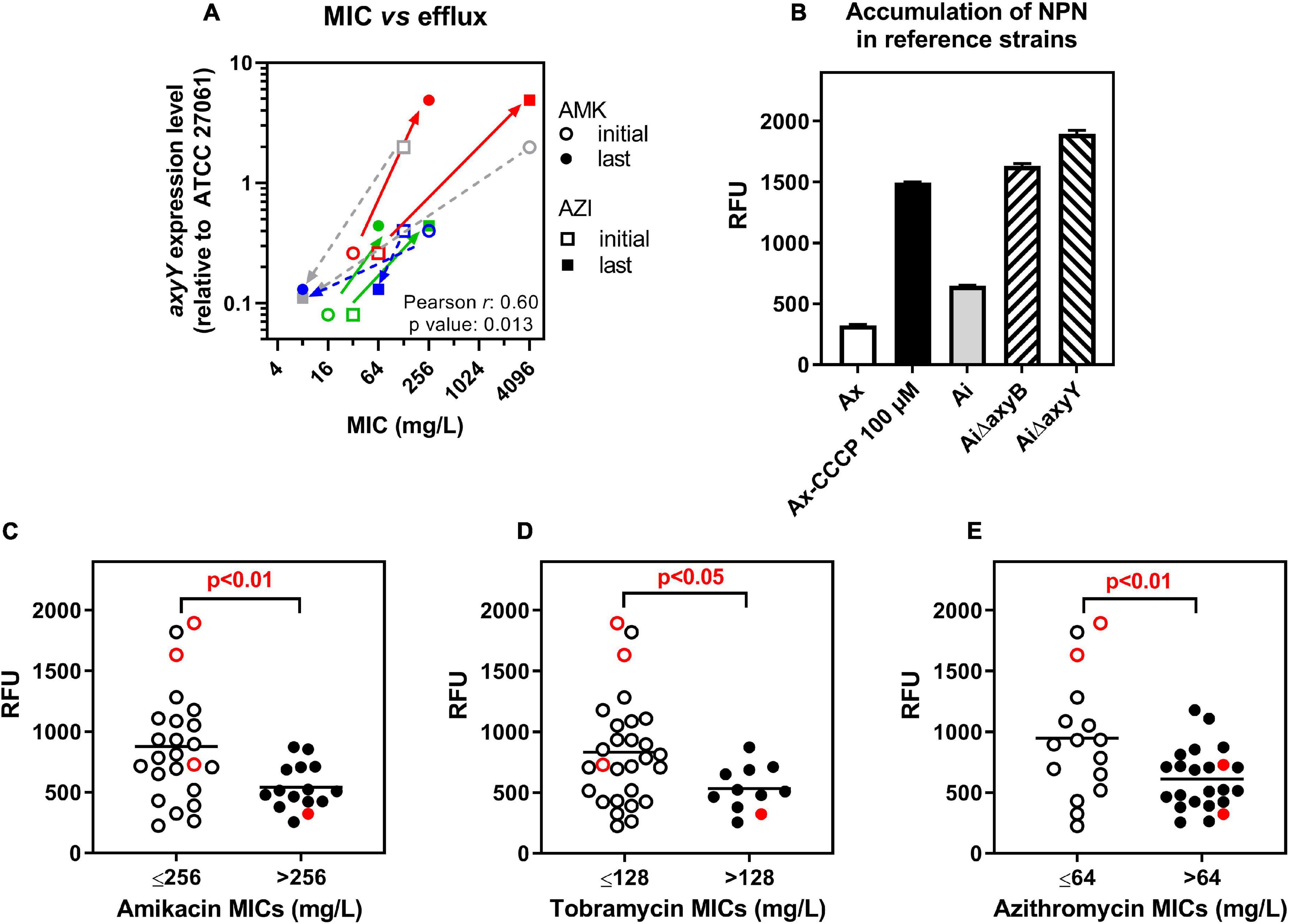
Figure 2. Influence of active efflux on amikacin, tobramycin and azithromycin activity. (A) Correlation between the MIC of amikacin (AMK) or azithromycin (AZI) and the level of expression of axyY (relative to that in ATCC 27061) in 4 pairs of isolates, represented by different colors. Plain arrows joint data points of pairs for which the late isolate was more resistant [from patient 9 (red) and 10 (green)]; dotted arrows, those for which the late isolate was more susceptible [from patient 6 (gray) and 19 (dark blue)]. Pearson coefficient and p-values of the correlation are given. (B) Accumulation of the fluorophore NPN in reference strains (Ax: A. xylosoxidans ATCC 27061, Ai: A. insuavis), mutants (AiΔB: A. insuavis lacking axyB gene, AiΔY: A. insuavis lacking axyY gene), or in the presence of CCCP (100 μM) used as a positive control in Ax to reduce the activity of efflux systems, after 10 min of incubation at 37°C. Data are means ± SD of three independent determinations and are expressed in arbitrary fluorescence units (standardized initial inoculum for all strains). (C–E) Accumulation of NPN in the same conditions in reference and clinical isolates stratified as a function of their MICs for amikacin, tobramycin, or azithromycin. Red open-closed symbols: reference strains; black open-closed symbols: clinical isolates. Statistical analysis: partition tree to determine the MIC value splitting the distribution in 2 with the highest Logworth (-log p-value) value (p-value indicated on the graphs). The horizontal line corresponds to the mean value.
Ribosomal Mutations and Decreased Azithromycin Activity
Fourteen isolates in this collection showed azithromycin MICs > 256 mg/L (2 isolates with a MIC of 512 mg/L and 12 isolates with a MIC > 2,048 mg/L, respectively; see Table 1). Ribosomal mutations in rpl4, rpl22, and rrl genes were therefore searched in these isolates and in 17 representative isolates with lower MICs (8–256 mg/L). The sequences of the isolates were first compared to those of A. xylosoxidans ATCC 27061. However, this reference strain does not show a wild-type phenotype (elevated MICs to some antibiotics; see Table 1). Since there is no fully sequenced wild-type A. xylosoxidans, we rather decided to compare all sequences to that of A. insuavis AXX-A which is more susceptible to meropenem, ticarcillin, aminoglycosides, ciprofloxacin, chloramphenicol, and doxycycline; Table 1). All the data are compiled in Supplementary Table 3 together with azithromycin MICs (± berberine) and axyB or axyX gene expression levels. In this Supplementary Table 3, we specifically identify in blue color the mutations that distinguish A. xysoloxidans ATCC 27061 from A. insuavis AXX-A, and isolates are ordered to show close from one another those which share the same mutations, classified according to their MIC. Three silent mutations (T96C, T123C, and T411C) were commonly observed in rpl4, including in A. xysoloxidans ATCC 27061, while a few others were only seen in clinical isolates (C219G, C330T, C444T, and C414T). Two mutations leading to the replacement of an uncharged amino acid by a charged amino acid (Q65R and G69R) were detected in isolates with an MIC of 512 mg/L (isolates 5.1 and 9.6). In rpl22, silent point mutations (T78C, T189C) were seen in A. xysoloxidans ATCC 27061 and in several clinical isolates. A missense conservative rpl22 T134C (V45A) mutation was observed in the reference ATCC 27061 and in many isolates. It is most likely not related to resistance, as it was also found in isolates with an MIC of azithromycin of ≤ 64 mg/L (viz., isolates 27.1, 27.3, or 10.1 and ATCC 27061). A series of mutations were commonly observed in rrl, independent of their azithromycin MIC in the presence of berberine (16 to > 2,048 mg/L), most of them being identified when comparing A. xysoloxidans ATCC 27061 and A. insuavis AXX-A. Among the 12 isolates with azithromycin MICs > 2,048 mg/L, 11 showed specific mutations in the rrl gene (A1284G, T1325C, A2043T, A2043G, A2044G, or C2596T). No specific mutation was identified in isolate 17.1. axyY expression was globally low in isolates that were more susceptible to azithromycin than the reference strain or in isolates presenting mutations in rpl4 or rpl22. Conversely, axyY expression was variable among isolates harboring mutations in rrl, but at least one of the two efflux-associated genes was overexpressed in all isolates with MICs > 2,048 mg/L, except for isolates 17.1 and 11.1.
Mutations in Fluoroquinolone Targets and Resistance to Ciprofloxacin
Ciprofloxacin displayed marginal activity against this collection, with MIC ranging from 1 to 32 mg/L. As A. xylosoxidans shows an elevated MIC to ciprofloxacin (4 mg/L), its sequence was first compared with that of A. insuavis AXX-A. Several missense mutations (shown in green) in the sequence of gyrA, gyrB, parC, and parE were detected and an insertion of 3 amino acids at the end of the gyrA sequence, which were also found in other isolates with an MIC of 4 mg/L (Supplementary Table 4). Of note, the isolate 10.1, with an MIC of 4 mg/L and a basal expression of axyF, shows the same sequence for the four genes as A. insuavis AXX-A, which may suggest that the mutations seen in A. xylosoxidans ATCC 27061 are not necessarily explaining its elevated MIC. The isolate 3.1 (susceptible; MIC = 1 mg/L) showed only a few differences with the sequence of A. insuavis AXX-A, some of them being also found in gyrB or parC of A. xylosoxidans ATCC 27061. Other mutations were specifically identified in clinical isolates with ciprofloxacin MIC ≥ 4 mg/L, namely, T527S and M706V in gyrA (isolates from patients 18 and 8), N11T, G12S, N592S or N592G, S609A, A631T, A633S, and A634T in gyrB (isolates from patients 18, 15, and 5); V417L, E441Q, S482T, and K764R in parC (isolates from patients 9, 19, and 18); and V41I, A150T, and T593S in parE (isolate from patient 18). Lastly, other missense mutations, located exclusively in gyrA (Q83L, D87N, L454M, T881M; patients 4, 8, 16, 17) and gyrB (I683V; patient 5), were detected only in isolates with MICs ≥ 16 mg/L. Among all these mutations, only Q83L and D87N were located in the QRDR for gyrA. axyF expression was low in most of the isolates, except 10.3, 16.1, and 19.2 and associated with specific target mutations in 16.1 and 19.2. Of note, isolates 9.1 and 19.2 showed the same mutations in parC but different MICs depending on the level of expression of axyF. Noteworthy, the only isolate in the collection with a MIC of 128 mg/L (10.3, patient 10) did not show any specific QRDR mutation but rather a particularly high level of expression of axyF as compared to the previous isolate from the same patient (10.1). No other mutations were found in the isolates that are not shown in Supplementary Table 4.
Discussion
This study highlights a major, and sometimes unexpected, role of efflux and target mutations in the resistance of Achromobacter isolated from patients with CF to specific antibiotic classes, thanks to the exploitation of a collection containing in majority longitudinal pairs of isolates from the same patients.
Whereas WGS-based typing has been successfully set up, a public cgMLST scheme for interpretation of genetic relatedness is lacking. Hence, an ad hoc scheme was established here [≤ 20 allele difference (de Been et al., 2015)]. Based on this strict criterion, only about half of the isolates kept a very high degree of relatedness over time, possibly indicating that genetic adaptations have taken place under selective pressure during antibiotic treatment. Although not powered as an epidemiological survey, our study shows that, among the tested drugs, the most active ones belong to the class of β-lactams, in accordance with previous reports from France (Amoureux et al., 2013; Dupont et al., 2017), Italy (Raso et al., 2008), United Kingdom (Okoliegbe et al., 2020), or United States (Duggan et al., 1996; Swenson and Sadikot, 2015). Nevertheless, remarkable changes in specific pairs allowed us to delineate important findings in terms of mechanisms of resistance, which was the main purpose of this work.
We first document a major role of efflux in resistance to several drugs, by evidencing quantitative correlations between the expression levels of the genes encoding efflux pumps and the increase in MICs of drug substrates, an aspect that was not examined in previous works (Bador et al., 2011, 2013; Magallon et al., 2021).
Regarding resistance to β-lactams, we did not observe any correlation between the expression of axyB and the MIC of ceftazidime, described as a substrate for this pump (Magallon et al., 2022), possibly because the concomitant contribution of cephalosporinase activity in resistance levels masks a potential contribution of efflux to this loss of susceptibility. This mechanism was phenotypically detected in one-third of the collection, but was not characterized at the molecular level, as it was out of the scope of this study. It is known for example that most A. xylosoxidans express a narrow-spectrum class D β-lactamase (Doi et al., 2008), but its role is considered marginal in resistance to cephalosporins or carbapenems (Doi et al., 2008; Amoureux et al., 2013; Papalia et al., 2020). Regarding meropenem specifically, no carbapenemase activity was detected in the collection, but we rather observed a correlation between MICs and axyB expression level. AxyABM has been previously shown to confer resistance to several cephalosporins and aztreonam (Bador et al., 2011) and, only very recently, to carbapenems as well (Magallon et al., 2022). In fact, previous work on carbapenems did not evidence this transport (Bador et al., 2011), probably because these authors used a strain with low meropenem MIC (0.094 mg/L), thus probably expressing the pump at a low level. This indicates the interest of also quantifying efflux pump expression level in order to better characterize their effects on susceptibility to drugs. The involvement of efflux in meropenem resistance seems to be a trait for CF isolates. We previously described that MexAB-OprM (homologous to AxyABM) plays a crucial role in meropenem resistance for P. aeruginosa isolates from CF (Chalhoub et al., 2016).
Aminoglycosides are considered as innately inactive against A. xylosoxidans (Bador et al., 2016) due to the constitutive expression of AxyXY-OprZ [expressed only in Achromobacter species resistant to aminoglycosides [A. xylosoxidans, A. ruhlandii, A. dolens, A. insuavis, A. denitrificans, A. insolitus, and A. aegrifaciens (Bador et al., 2016)]. We confirm the role of this efflux transporter in aminoglycoside resistance by demonstrating (a) the capacity of berberine to decrease aminoglycoside MIC and (b) a correlation between the expression level of axyY and the MICs of amikacin or tobramycin. Noteworthy, we found a few isolates that were susceptible to aminoglycosides, which could be ascribed to a particularly low level of expression of the pump. This impact of efflux on susceptibility to aminoglycosides is best seen for pairs 9, 10, 6, and 19 for which a commensurate change in MIC and in gene expression was noticed between early and late isolates. Likewise, we observed a correlation between axyY expression and colistin MICs, suggesting that it could be a substrate for AxyXY-OprZ. Of note, polymyxins susceptibility has been linked to MexXY-OprM/OprA expression in P. aeruginosa (Poole et al., 2015).
Macrolides act by inhibiting bacterial protein synthesis. A well-established resistance mechanism (in Gram-positive organisms) consists in mutations in 23S rRNA-encoding gene and in the ribosomal proteins L4 and L22 (Fyfe et al., 2016). We previously showed that both efflux and ribosomal mutations act in concert to confer high levels of resistance to macrolides in P. aeruginosa CF isolates (Mustafa et al., 2017). Here, we found a correlation between azithromycin MICs and the expression level of axyB and axyY, which is in line with the previously demonstrated role of efflux in macrolide resistance in P. aeruginosa (Morita et al., 2016). Surprisingly, however, the MexXY efflux pump attenuator berberine was able to reduce azithromycin MICs only in a limited fraction of the collection, suggesting the presence of other resistance mechanisms. Genomic analysis revealed a series of ribosomal mutations, among which A2043T, A2043G, and A2044G in rrl, associated with a higher level of resistance than the mutation C2596T, as previously reported in CF P. aeruginosa (Mustafa et al., 2017; Colque et al., 2020). Other mutations (A1284G and T1325C) have not been described so far. In addition, in two isolates with an MIC of 512 mg/L, we found mutations in the ribosomal protein 4, namely, Q65R (never described) and G69R, previously reported in macrolide-resistant Streptococcus pneumoniae (Clark et al., 2007; Kosowska-Shick et al., 2008), linezolid-resistant Staphylococcus epidermidis (Mendes et al., 2012), and CF macrolide-resistant Burkholderia multivorans (G70R; corresponding position) (Silva et al., 2016).
Concerning fluoroquinolones, we could not evidence a clear role of efflux in resistance, since the level of expression of axyF was low in most of the isolates. Of note, however, two isolates from our collection with high level of expression in axyF (19.2, 10.3) also show high ciprofloxacin MICs, in the absence of target mutations (10.3) or in the presence of mutations similar to those observed in a more susceptible isolate with low axyF expression (19.2 vs. 9.1). This is coherent with the recent description of mutations in axyT (putative regulator of AxyEF-OprN) associated with overexpression of axyF in strains harboring high ciprofloxacin MIC even in the absence of QRDR mutations (Magallon et al., 2021). In the rest of the collection, we cannot exclude that efflux-mediated resistance could be masked by the impact of target mutations on MICs. Some mutations (Q83L and D87N in the QRDR of gyrA) have been previously reported as hot spots in Escherichia coli and P. aeruginosa (Bagel et al., 1999; Takenouchi et al., 1999), Stenotrophomonas maltophilia (Zhao et al., 2015), and environmental isolates of Achromobacter spp. (Furlan et al., 2018), while others have never been reported in gyrA (L454M and T881M) and gyrB (I683V), but are located outside of the QRDR regions. We note here that the mutation D87N was associated with an elevated ciprofloxacin MIC (32 mg/L) in the absence of overexpression of axyF, while D87G was associated with even higher MICs (64–128 mg/L) in isolates overexpressing axyF to high levels (Magallon et al., 2021). The mutations we reported in parC/parE were not previously described in A. xylosoxidans or other species, to the best of our knowledge, but are not located in the QRDR regions. It is established that mutations in the QRDR confer higher levels of resistance to fluoroquinolones than those in other regions (Yoshida et al., 1990; Belland et al., 1994). Conversely, most of the mutations reported by Magallon et al. (2021) and considered as not relevant for resistance were not seen here. It is also interesting to note that many mutations are evidenced in the isolates from patient 18, which we suspect to belong to a new species, and may thus simply represent variation in the gene sequence.
We did not study in details resistance mechanisms to tetracyclines and chloramphenicol, but it is remarkable that all isolates with elevated MICs to chloramphenicol were overexpressing axyF. We cannot exclude the concomitant presence of other resistance mechanisms, but only notice that chloramphenicol is also described as a good substrate for MexEF-OprN in P. aeruginosa (Maseda et al., 2000).
This study suffers from some limitations. First, the number of isolates remains limited, but this is due to the still relatively low proportion of patients colonized by this bacterial genus in the collecting centers. Second, we could not establish a link between resistance development and antibiotic use in each individual patient, which could be the topic of further investigations. Third, in close relationship with the two previous limitations, we could not study in details the evolution of resistance over time because the number of samples and the period of time during which they were collected was highly variable among patients, rendering difficult a statistically meaningful analysis. Fourth, we could not confirm all our hypotheses at the molecular level because this would require the construction of a large number of deletion mutants or of complemented strains, which would represent a work by itself. In particular, we noticed that the deletion of efflux pumps in reference strains is not sufficient to cause a significant phenotypic change in susceptibility, highlighting the interest of rather working with clinical isolates that show increased levels of expression for these transporters. Specifically, the comparison of successive isogenic isolates from the same patients allowed us to unambiguously evidence the role of resistance mechanisms that were expressed in one isolate from the pair and associated with a change in MIC, partially alleviating this limitation. Fifth, the only available ATCC reference strain does not show a wild-type profile of susceptibility for all antibiotics. It appeared nevertheless to us as an adequate control, being easily available to anyone, in the absence of fully sequenced isolate from human specimen harboring a wild-type phenotype. The AXX-A strain (considered as wild type) has been recently reclassified as A. insuavis (NCBI:txid1003200) but has nevertheless been used as a reference sequence in our genomic analyses, in order to prevent missing the identification of some mutations associated with resistance, especially for fluoroquinolones. Lastly, the definition and evaluation of a novel cgMLST scheme for WGS-based typing of A. xylosoxidans should be further investigated.
Nevertheless, the present work is among the first studies to shed some light on a number of different mechanisms that most likely contribute to explain the unusually high level of resistance to conventional antibiotics in A. xylosoxidans or the closely related species A. insuavis. Our data should therefore help to better apprehend bacterial response to antibiotic exposure and adapt antibiotherapy accordingly.
Data Availability Statement
The original contributions presented in the study are included in the article/Supplementary Material, further inquiries can be directed to the corresponding author/s.
Author Contributions
HC, BK, and FVB: conceptualization. HC and SK: methodology, formal analysis, and investigation. SK and BK: resources. HC, SK, and FVB: writing—original draft preparation, funding acquisition. BK: writing—review and editing. BK and FVB: supervision. All authors contributed to the article and approved the submitted version.
Funding
This research was funded by a grant from the King Baudouin Foundation (Fonds Forton) and the Belgian Mucovereniging/Association Muco to FVB and HC and a grant for infectiology by Gilead to SK.
Conflict of Interest
The authors declare that the research was conducted in the absence of any commercial or financial relationships that could be construed as a potential conflict of interest.
Publisher’s Note
All claims expressed in this article are solely those of the authors and do not necessarily represent those of their affiliated organizations, or those of the publisher, the editors and the reviewers. Any product that may be evaluated in this article, or claim that may be made by its manufacturer, is not guaranteed or endorsed by the publisher.
Acknowledgments
FV is Research Director from the Belgian Fonds de la Recherche Scientifique (FRS-FNRS). We warmly thank Julien Bador, Dijon, France and Niels Norskov-Lauritsen, Aarhus University, Aahrus, Denmark, for the kind gift of reference strains and their mutants, Hector Rodriguez-Villalobos, Laboratoire de microbiologie, cliniques universitaires Saint-Luc, Université catholique de Louvain, Brussels, Belgium, for performing MALDI-TOF analyses, Albert Ruiz-Sorribas for help in sequence alignments, and Alix Mangin, Virginie Mohymont, and Romain Tricot for excellent technical assistance.
Supplementary Material
The Supplementary Material for this article can be found online at: https://www.frontiersin.org/articles/10.3389/fmicb.2022.762307/full#supplementary-material
Footnotes
References
Amoureux, L., Bador, J., Bounoua Zouak, F., Chapuis, A., de Curraize, C., and Neuwirth, C. (2016). Distribution of the species of Achromobacter in a French Cystic Fibrosis Centre and multilocus sequence typing analysis reveal the predominance of A. xylosoxidans and clonal relationships between some clinical and environmental isolates. J. Cyst. Fibros. 15, 486–494. doi: 10.1016/j.jcf.2015.12.009
Amoureux, L., Bador, J., Siebor, E., Taillefumier, N., Fanton, A., and Neuwirth, C. (2013). Epidemiology and resistance of Achromobacter xylosoxidans from cystic fibrosis patients in Dijon, Burgundy: first French data. J. Cyst. Fibros. 12, 170–176. doi: 10.1016/j.jcf.2012.08.005
Bador, J., Amoureux, L., Blanc, E., and Neuwirth, C. (2013). Innate aminoglycoside resistance of Achromobacter xylosoxidans is due to AxyXY-OprZ, an RND-type multidrug efflux pump. Antimicrob. Agents Chemother. 57, 603–605. doi: 10.1128/AAC.01243-12
Bador, J., Amoureux, L., Duez, J. M., Drabowicz, A., Siebor, E., Llanes, C., et al. (2011). First description of an RND-type multidrug efflux pump in Achromobacter xylosoxidans, AxyABM. Antimicrob. Agents Chemother. 55, 4912–4914. doi: 10.1128/AAC.00341-11
Bador, J., Neuwirth, C., Liszczynski, P., Mezier, M. C., Chretiennot, M., Grenot, E., et al. (2016). Distribution of innate efflux-mediated aminoglycoside resistance among different Achromobacter species. New Microbes. New Infect. 10, 1–5. doi: 10.1016/j.nmni.2015.11.013
Bagel, S., Hullen, V., Wiedemann, B., and Heisig, P. (1999). Impact of gyrA and parC mutations on quinolone resistance, doubling time, and supercoiling degree of Escherichia coli. Antimicrob. Agents Chemother. 43, 868–875. doi: 10.1128/AAC.43.4.868
Belland, R. J., Morrison, S. G., Ison, C., and Huang, W. M. (1994). Neisseria gonorrhoeae acquires mutations in analogous regions of gyrA and parC in fluoroquinolone-resistant isolates. Mol. Microbiol. 14, 371–380. doi: 10.1111/j.1365-2958.1994.tb01297.x
Chalhoub, H., Saenz, Y., Rodriguez-Villalobos, H., Denis, O., Kahl, B. C., Tulkens, P. M., et al. (2016). High-level resistance to meropenem in clinical isolates of Pseudomonas aeruginosa in the absence of carbapenemases: role of active efflux and porin alterations. Int. J. Antimicrob. Agents 48, 740–743. doi: 10.1016/j.ijantimicag.2016.09.012
Clark, C. L., Kosowska-Shick, K., Ednie, L. M., and Appelbaum, P. C. (2007). Capability of 11 antipneumococcal antibiotics to select for resistance by multistep and single-step methodologies. Antimicrob. Agents Chemother. 51, 4196–4201. doi: 10.1128/AAC.00827-07
Clinical and Laboratory Standards Institute (2020). Performance Standards for Antimicrobial Susceptibility Testing; 30th Informational Supplement. CLSI document M100-S30. Pennsylvania, PA: Clinical and Laboratory Standards Institute.
Colque, C. A., Albarracin Orio, A. G., Feliziani, S., Marvig, R. L., Tobares, A. R., Johansen, H. K., et al. (2020). Hypermutator Pseudomonas aeruginosa Exploits Multiple Genetic Pathways To Develop Multidrug Resistance during Long-Term Infections in the Airways of Cystic Fibrosis Patients. Antimicrob. Agents Chemother. 64, e2142–e2119. doi: 10.1128/AAC.02142-19
Cramer, C. L., Patterson, A., Alchakaki, A., and Soubani, A. O. (2017). Immunomodulatory indications of azithromycin in respiratory disease: a concise review for the clinician. Postgrad. Med. 129, 493–499. doi: 10.1080/00325481.2017.1285677
Cystic Fibrosis Foundation (2020). 2019 Patient Registry Annual Data Report. Maryland, US: Cystic Fibrosis Foundation.
de Been, M., Pinholt, M., Top, J., Bletz, S., Mellmann, A., van Schaik, W., et al. (2015). Core Genome Multilocus Sequence Typing Scheme for High- Resolution Typing of Enterococcus faecium. J. Clin. Microbiol. 53, 3788–3797. doi: 10.1128/JCM.01946-15
Dekker, J. P., and Frank, K. M. (2016). Next-Generation Epidemiology: Using Real-Time Core Genome Multilocus Sequence Typing To Support Infection Control Policy. J. Clin. Microbiol. 54, 2850–2853. doi: 10.1128/JCM.01714-16
Doi, Y., Poirel, L., Paterson, D. L., and Nordmann, P. (2008). Characterization of a naturally occurring class D beta-lactamase from Achromobacter xylosoxidans. Antimicrob. Agents Chemother. 52, 1952–1956. doi: 10.1128/AAC.01463-07
Duggan, J. M., Goldstein, S. J., Chenoweth, C. E., Kauffman, C. A., and Bradley, S. F. (1996). Achromobacter xylosoxidans bacteremia: report of four cases and review of the literature. Clin. Infect. Dis. 23, 569–576. doi: 10.1093/clinids/23.3.569
Dupont, C., Jumas-Bilak, E., Michon, A. L., Chiron, R., and Marchandin, H. (2017). Impact of High Diversity of Achromobacter Populations within Cystic Fibrosis Sputum Samples on Antimicrobial Susceptibility Testing. J. Clin. Microbiol. 55, 206–215. doi: 10.1128/JCM.01843-16
Edwards, B. D., Greysson-Wong, J., Somayaji, R., Waddell, B., Whelan, F. J., Storey, D. G., et al. (2017). Prevalence and Outcomes of Achromobacter Species Infections in Adults with Cystic Fibrosis: a North American Cohort Study. J. Clin. Microbiol. 55, 2074–2085. doi: 10.1128/JCM.02556-16
Fang, X., Fang, Z., Zhao, J., Zou, Y., Li, T., Wang, J., et al. (2012). Draft genome sequence of Pseudomonas aeruginosa strain ATCC 27853. J. Bacteriol. 194, 3755. doi: 10.1128/jb.00690-12
Furlan, J. P. R., Sanchez, D. G., Gallo, I. F. L., and Stehling, E. G. (2018). Replicon typing of plasmids in environmental Achromobacter sp. producing quinolone-resistant determinants. APMIS 126, 864–869. doi: 10.1111/apm.12896
Fyfe, C., Grossman, T. H., Kerstein, K., and Sutcliffe, J. (2016). Resistance to Macrolide Antibiotics in Public Health Pathogens. Cold Spring Harb. Perspect. Med. 6:a025395. doi: 10.1101/cshperspect.a025395
Gade, S. S., Norskov-Lauritsen, N., and Ridderberg, W. (2017). Prevalence and species distribution of Achromobacter sp. cultured from cystic fibrosis patients attending the Aarhus centre in Denmark. J. Med. Microbiol. 66, 686–689. doi: 10.1099/jmm.0.000499
Hoyle, N., Zhvaniya, P., Balarjishvili, N., Bolkvadze, D., Nadareishvili, L., Nizharadze, D., et al. (2018). Phage therapy against Achromobacter xylosoxidans lung infection in a patient with cystic fibrosis: a case report. Res. Microbiol. 169, 540–542. doi: 10.1016/j.resmic.2018.05.001
Hu, Y., Zhu, Y., Ma, Y., Liu, F., Lu, N., Yang, X., et al. (2015). Genomic insights into intrinsic and acquired drug resistance mechanisms in Achromobacter xylosoxidans. Antimicrob. Agents Chemother. 59, 1152–1161. doi: 10.1128/AAC.04260-14
Isler, B., Kidd, T. J., Stewart, A. G., Harris, P., and Paterson, D. L. (2020). Achromobacter Infections and Treatment Options. Antimicrob. Agents Chemother. 64, e1025–e1020. doi: 10.1128/AAC.01025-20
Kosowska-Shick, K., Clark, C., Credito, K., Dewasse, B., Beachel, L., Ednie, L., et al. (2008). In vitro capability of faropenem to select for resistant mutants of Streptococcus pneumoniae and Haemophilus influenzae. Antimicrob. Agents Chemother. 52, 748–752. doi: 10.1128/AAC.01389-07
Lomovskaya, O., Warren, M. S., Lee, A., Galazzo, J., Fronko, R., Lee, M., et al. (2001). Identification and characterization of inhibitors of multidrug resistance efflux pumps in Pseudomonas aeruginosa: novel agents for combination therapy. Antimicrob. Agents Chemother. 45, 105–116. doi: 10.1128/AAC.45.1.105-116.2001
Magallon, A., Amoureux, L., Garrigos, T., Sonois, M., Varin, V., Neuwirth, C., et al. (2022). Role of AxyABM overexpression in acquired resistance in Achromobacter xylosoxidans. J. Antimicrob. Chemother 13:dkab479. doi: 10.1093/jac/dkab479
Magallon, A., Roussel, M., Neuwirth, C., Tetu, J., Cheiakh, A. C., Boulet, B., et al. (2021). Fluoroquinolone resistance in Achromobacter spp.: substitutions in QRDRs of GyrA, GyrB, ParC and ParE and implication of the RND efflux system AxyEF-OprN. J. Antimicrob. Chemother. 76, 297–304. doi: 10.1093/jac/dkaa440
Marsac, C., Berdah, L., Thouvenin, G., Sermet-Gaudelus, I., and Corvol, H. (2021). Achromobacter xylosoxidans airway infection is associated with lung disease severity in children with cystic fibrosis. ERJ. Open. Res. 7, 00076–02021. doi: 10.1183/23120541.00076-2021
Maseda, H., Yoneyama, H., and Nakae, T. (2000). Assignment of the substrate-selective subunits of the MexEF-OprN multidrug efflux pump of Pseudomonas aeruginosa. Antimicrob. Agents Chemother. 44, 658–664. doi: 10.1128/AAC.44.3.658-664.2000
Mendes, R. E., Deshpande, L. M., Costello, A. J., and Farrell, D. J. (2012). Molecular epidemiology of Staphylococcus epidermidis clinical isolates from U.S. hospitals. Antimicrob. Agents Chemother. 56, 4656–4661. doi: 10.1128/AAC.00279-12
Morita, Y., Nakashima, K. I., Nishino, K., Kotani, K., Tomida, J., Inoue, M., et al. (2016). Berberine Is a Novel Type Efflux Inhibitor Which Attenuates the MexXY-Mediated Aminoglycoside Resistance in Pseudomonas aeruginosa. Front. Microbiol. 7:1223. doi: 10.3389/fmicb.2016.01223
Mustafa, M. H., Khandekar, S., Tunney, M. M., Elborn, J. S., Kahl, B. C., Denis, O., et al. (2017). Acquired resistance to macrolides in Pseudomonas aeruginosa from cystic fibrosis patients. Eur. Respir. J. 49:1601847. doi: 10.1183/13993003.01847-2016
Nei, M., and Kumar, S. (2000). Molecular Evolution and Phylogenetics. Oxford, UK: Oxford University, 1–348.
Nielsen, S. M., Penstoft, L. N., and Norskov-Lauritsen, N. (2019). Motility, Biofilm Formation and Antimicrobial Efflux of Sessile and Planktonic Cells of Achromobacter xylosoxidans. Pathogens. 8:14. doi: 10.3390/pathogens8010014
Nordmann, P., Dortet, L., and Poirel, L. (2012a). Rapid detection of extended-spectrum-beta-lactamase-producing Enterobacteriaceae. J. Clin. Microbiol. 50, 3016–3022.
Nordmann, P., Poirel, L., and Dortet, L. (2012b). Rapid detection of carbapenemase-producing Enterobacteriaceae. Emerg. Infect. Dis. 18, 1503–1507.
Ocaktan, A., Yoneyama, H., and Nakae, T. (1997). Use of fluorescence probes to monitor function of the subunit proteins of the MexA-MexB-oprM drug extrusion machinery in Pseudomonas aeruginosa. J. Biol. Chem. 272, 21964–21969. doi: 10.1074/jbc.272.35.21964
Okoliegbe, I. N., Hijazi, K., Cooper, K., Ironside, C., and Gould, I. M. (2020). Longitudinal surveillance and combination antimicrobial susceptibility testing of multidrug-resistant Achromobacter spp. From cystic fibrosis patients. Antimicrob. Agents Chemother. 64, e1467–e1420. doi: 10.1128/AAC.01467-20
Papalia, M., Steffanowski, C., Traglia, G., Almuzara, M., Martina, P., Galanternik, L., et al. (2020). Diversity of Achromobacter species recovered from patients with cystic fibrosis, in Argentina. Rev. Argent Microbiol. 52, 13–18. doi: 10.1016/j.ram.2019.03.004
Poole, K., Lau, C. H.-F., Gilmour, C., Hao, Y., and Lam, J. S. (2015). Polymyxin Susceptibility in Pseudomonas aeruginosa Linked to the MexXY-OprM Multidrug Efflux System. Antimicrob. Agents Chemother. 59, 7276–7289. doi: 10.1128/AAC.01785-15
Raidt, L., Idelevich, E. A., Dubbers, A., Kuster, P., Drevinek, P., Peters, G., et al. (2015). Increased Prevalence and Resistance of Important Pathogens Recovered from Respiratory Specimens of Cystic Fibrosis Patients During a Decade. Pediatr. Infect. Dis. J. 34, 700–705. doi: 10.1097/INF.0000000000000714
Raso, T., Bianco, O., Grosso, B., Zucca, M., and Savoia, D. (2008). Achromobacter xylosoxidans respiratory tract infections in cystic fibrosis patients. APMIS 116, 837–841. doi: 10.1111/j.1600-0463.2008.00995.x
Saiman, L., Chen, Y., Tabibi, S., San Gabriel, P., Zhou, J., Liu, Z., et al. (2001). Identification and antimicrobial susceptibility of Alcaligenes xylosoxidans isolated from patients with cystic fibrosis. J. Clin. Microbiol. 39, 3942–3945. doi: 10.1128/JCM.39.11.3942-3945.2001
Silva, I. N., Santos, P. M., Santos, M. R., Zlosnik, J. E. A., Speert, D. P., Buskirk, S. W., et al. (2016). Long-Term Evolution of Burkholderia multivorans during a Chronic Cystic Fibrosis Infection Reveals Shifting Forces of Selection. mSystems 1, e29–e16. doi: 10.1128/mSystems.00029-16
Somayaji, R., Stanojevic, S., Tullis, D. E., Stephenson, A. L., Ratjen, F., and Waters, V. (2017). Clinical Outcomes Associated with Achromobacter Species Infection in Patients with Cystic Fibrosis. Ann. Am. Thorac. Soc. 14, 1412–1418. doi: 10.1513/AnnalsATS.201701-071OC
Spilker, T., Vandamme, P., and LiPuma, J. J. (2012). A multilocus sequence typing scheme implies population structure and reveals several putative novel Achromobacter species. J. Clin. Microbiol. 50, 3010–3015. doi: 10.1128/JCM.00814-12
Steinberg, J. P., and Del Rio, C. (2005). “Other gram-negative and gram-variable bacilli,” in Principles and practices of infectious diseases, 6th ed, eds G. L. Mandell, J. E. Bennett, and R. Dolin (London, UK: Churchill Livingstone), 2751–2768.
Swenson, C. E., and Sadikot, R. T. (2015). Achromobacter respiratory infections. Ann. Am. Thorac. Soc. 12, 252–258.
Takenouchi, T., Sakagawa, E., and Sugawara, M. (1999). Detection of gyrA mutations among 335 Pseudomonas aeruginosa strains isolated in Japan and their susceptibilities to fluoroquinolones. Antimicrob. Agents Chemother. 43, 406–409. doi: 10.1128/AAC.43.2.406
Tamura, K., Stecher, G., and Kumar, S. (2021). MEGA11: Molecular Evolutionary Genetics Analysis Version 11. Mol. Biol. Evol. 38, 3022–3027. doi: 10.1093/molbev/msab120
Tetart, M., Wallet, F., Kyheng, M., Leroy, S., Perez, T., Le Rouzic, O., et al. (2019). Impact of Achromobacter xylosoxidans isolation on the respiratory function of adult patients with cystic fibrosis. ERJ. Open. Res. 5, 00051–2019. doi: 10.1183/23120541.00051-2019
Traglia, G. M., Almuzara, M., Merkier, A. K., Adams, C., Galanternik, L., Vay, C., et al. (2012). Achromobacter xylosoxidans: an emerging pathogen carrying different elements involved in horizontal genetic transfer. Curr. Microbiol. 65, 673–678. doi: 10.1007/s00284-012-0213-5
Wang, M., Ridderberg, W., Hansen, C. R., Hoiby, N., Jensen-Fangel, S., Olesen, H. V., et al. (2013). Early treatment with inhaled antibiotics postpones next occurrence of Achromobacter in cystic fibrosis. J. Cyst. Fibros. 12, 638–643. doi: 10.1016/j.jcf.2013.04.013
Yabuuchi, E., and Oyama, A. (1971). Achromobacter xylosoxidans n. sp. from human ear discharge. Jpn. J. Microbiol. 15, 477–481. doi: 10.1111/j.1348-0421.1971.tb00607.x
Yoshida, H., Bogaki, M., Nakamura, M., and Nakamura, S. (1990). Quinolone resistance-determining region in the DNA gyrase gyrA gene of Escherichia coli. Antimicrob. Agents Chemother. 34, 1271–1272. doi: 10.1128/AAC.34.6.1271
Zeiser, E. T., Becka, S. A., Barnes, M. D., Taracila, M. A., LiPuma, J. J., and Papp-Wallace, K. M. (2019). Resurrecting Old beta-Lactams: Potent Inhibitory Activity of Temocillin against Multidrug-Resistant Burkholderia Species Isolates from the United States. Antimicrob. Agents Chemother. 63, e2315–e2318. doi: 10.1128/AAC.02315-18
Keywords: Achromobacter, efflux, target mutation, macrolide, fluoroquinolone, aminoglycoside
Citation: Chalhoub H, Kampmeier S, Kahl BC and Van Bambeke F (2022) Role of Efflux in Antibiotic Resistance of Achromobacter xylosoxidans and Achromobacter insuavis Isolates From Patients With Cystic Fibrosis. Front. Microbiol. 13:762307. doi: 10.3389/fmicb.2022.762307
Received: 21 August 2021; Accepted: 07 February 2022;
Published: 28 March 2022.
Edited by:
Yuji Morita, Meiji Pharmaceutical University, JapanReviewed by:
S. M. Hossein Khademi, Lund University, SwedenHelene Marchandin, Université de Montpellier, France
Copyright © 2022 Chalhoub, Kampmeier, Kahl and Van Bambeke. This is an open-access article distributed under the terms of the Creative Commons Attribution License (CC BY). The use, distribution or reproduction in other forums is permitted, provided the original author(s) and the copyright owner(s) are credited and that the original publication in this journal is cited, in accordance with accepted academic practice. No use, distribution or reproduction is permitted which does not comply with these terms.
*Correspondence: Françoise Van Bambeke, ZnJhbmNvaXNlLnZhbmJhbWJla2VAdWNsb3V2YWluLmJl
†These authors have contributed equally to this work