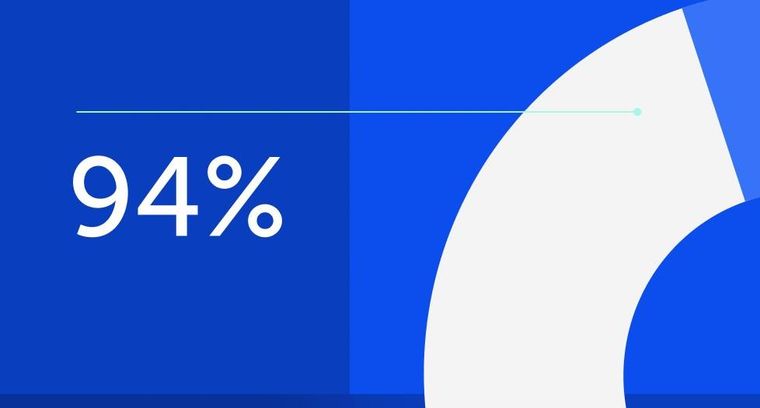
94% of researchers rate our articles as excellent or good
Learn more about the work of our research integrity team to safeguard the quality of each article we publish.
Find out more
REVIEW article
Front. Microbiol., 07 April 2022
Sec. Food Microbiology
Volume 13 - 2022 | https://doi.org/10.3389/fmicb.2022.684730
This article is part of the Research TopicInterspecies Interactions Within Fermented Food Systems and Their Impact on Process Efficiency and Product QualityView all 11 articles
The benefits derived from fermented foods and beverages have placed great value on their acceptability worldwide. Food fermentation technologies have been employed for thousands of years and are considered essential processes for the production and preservation of foods, with the critical roles played by the autochthonous fermenting food-grade microorganisms in ensuring food security and safety, increased shelf life, and enhanced livelihoods of many people in Africa, particularly the marginalized and vulnerable groups. Many indigenous fermented foods and beverages of Africa are of plant origin. In this review, the predominance, fermentative activities, and biopreservative role of Lactobacillus spp. during production of indigenous foods and beverages, the potential health benefit of probiotics, and the impact of these food-grade microorganisms on food safety and prolonged shelf life are discussed. During production of African indigenous foods (with emphasis on cereals and cassava-based food products), fermentation occurs in succession; the first group of microorganisms to colonize the fermenting substrates are lactic acid bacteria (LAB) with the diversity and dominance of Lactobacillus spp. The Lactobacillus spp. multiply rapidly in the fermentation matrix, by taking up nutrients from the surrounding environments, and cause rapid acidification in the fermenting system via the production of organic compounds that convert fermentable sugars into mainly lactic acid. Production of these compounds in food systems inhibits spoilage microorganisms, which has a direct effect on food quality and safety. The knowledge of microbial interaction and succession during food fermentation will assist the food industry in producing functional foods and beverages with improved nutritional profiling and technological attributes, as Lactobacillus strains isolated during fermentation of several African indigenous foods have demonstrated desirable characteristics that make them safe for use as probiotic microorganisms and even as a starter culture in small- and large-scale/industrial food production processes.
Fermented foods have been in existence since antiquity and are consumed by different regions globally because of the numerous nutritional values conferred on humans. For decades, the physiology and the functionalities of many important microorganisms associated with fermented foods have been studied, and this has assisted in the development of foods with improved flavor, taste, texture, and consistency (Marco et al., 2020). In Africa, fermented foods constitute the main dietary components, as many foods are fermented spontaneously before consumption with the predominance of lactic acid bacteria (LAB). These fermented foods and beverages serve as a vehicle for important microorganisms, thus playing essential roles in humans when consumed. Traditional fermentation of food is a natural method used in preserving different foods and beverages produced and thus extends the food shelf life, improves food palatability and food digestibility, and inhibits undesirable microorganisms, which results in improvement of food nutritional values. All these positive characteristics that impacted the fermented foods are dependent on the functional and technological roles displayed by autochthonous microorganisms present in the food substrates. Yeast and LAB are the groups of microorganisms that perform fermentative roles and flavor production during food fermentation, with LAB being the predominant microbes isolated from African indigenous fermented foods (cereals and tubers), although other microorganisms such as Bacillus spp., acetic acid bacteria, and molds have been reportedly isolated during production of some other fermented foods: legumes, wine, etc. (Adesulu-Dahunsi et al., 2017a, 2020; Chaves-López et al., 2020). Studies have shown that during fermentation of African fermented cereal-based and cassava-based foods, growth is firstly initiated by the LAB.
In Africa, production of several foods consumed by the populace relies on the fermentation processes; food security and shelf life are improved because of the biopreservative role played by the autochthonous microorganisms, which is advantageous to the community where there is poor access to electricity and refrigerating systems (Adesulu and Awojobi, 2014). Fermentation in food production is simply brought about by different activities of microorganisms and their enzymes to enhance the bio-accessibility and bioavailability of nutrients present in the raw food substrates, thus improving the organoleptic properties, improving digestibility, extending the food shelf life, inhibiting undesirable microorganisms, and serving as a vehicle for delivery of probiotics in humans (Diaz et al., 2019; Marco et al., 2020). Fermentation causes changes in both the physical and chemical properties of foods. It is described as the natural way of enhancing vitamin contents, essential amino acids, and sensorial properties of foods (Sharma et al., 2020). Food fermentation technologies are carried out at the household level via spontaneous fermentation consisting of mixed cultures of different species of autochthonous microbes, and back-slopping (re-inoculation of a previous successful fermentation batch into the new set to be fermented, which results in the dominance of best-adapted strains) has been carried out for several decades. In the last 2–3 decades, with increasing research and development, discoveries in the use of industrial-scale technology are preferred, i.e., inoculation of “defined starter culture” (pre-cultured single or mixed strains of microorganisms with functional and probiotics characteristics) in controlled fermentation processes; the latter is becoming a necessary under-regulated process to increase automated production, leading to improved and consistent fermented food quality for commercial purposes.
Several advantages of these fermented food products have been reviewed by various researchers (Melini et al., 2019; Şanlier et al., 2019; Sharma et al., 2020; Tsafrakidou et al., 2020), including the following:
• Production of safe food products.
• Reduction in cooking time.
• Production of foods with extended shelf life.
• Improvement of the organoleptic properties of fermented food products/enhancement of food sensorial characteristics.
• Increases mineral and trace element bioavailability in food/enrichment of food nutritional contents and digestibility.
• Elimination of cyanide compounds in cassava tubers and other harmful substances that may be present in cereals.
• Prevention of diseases or infections such as diarrhea and salmonellosis.
The population dynamics of microbes during fermentation using phenotypic and culture-dependent techniques have resulted in the understanding of different important roles that exist in the microbial consortia (Oguntoyinbo and Narbad, 2015; Adesulu-Dahunsi et al., 2017b). The invention of meta-omic methods (metagenomics, metatranscriptomics, metaproteomics, and metabolomics) has greatly complemented the culture-dependent techniques for strain-level characterization of food microbiota during fermentation processes and has also assisted in the studying of strains’ performances and microbial interactions of the important microorganisms within the food matrix and the systematic analysis of microbial metabolism and responses to the external/environmental factors (Liu et al., 2005; Makarova et al., 2006; Walsh et al., 2017; Taylor et al., 2020). The understanding of different roles displayed by food-grade microorganisms present in spontaneously fermented food products is crucial and has helped in the optimization of the final food quality, bringing about improved food safety. This review gives an overview of the impact of the activities and predominance of Lactobacillus microbiota during the production of African indigenous foods and the industrial importance of their interactions in cereal-based and cassava-based fermentation, their application in optimized or controlled food fermentation over mixed culture/uncontrolled fermentation, and the impact of Lactobacillus species co-occurrence in the fermenting food matrix on product development and safety.
Lactic acid bacteria perform the function of converting carbohydrate in food substrates into organic acids (principally lactic acid) and can also produce a wide range of metabolites (Rodríguez et al., 2019). Lactic acid bacteria is a common name given to the family Lactobacillaceae (Pfeiler and Klaenhammer, 2007). These groups of microorganisms are Gram-positive, acid-tolerant, mainly lactic acid producers, non-sporulating groups of important bacteria widely reported to perform fermentative roles in several African fermented foods and beverages, and some genera, most importantly the genus Lactobacillus, have a “Generally Recognized As Safe” (GRAS) status; these characteristics displayed by LAB affect the storage quality, thereby extending the shelf life and safety of many fermented foods and beverages (Oguntoyinbo and Narbad, 2015; Adesulu-Dahunsi et al., 2018). The most common LAB genera frequently reported to be associated with African indigenous fermented foods are Lactobacillus, Lactococcus, Leuconostoc, Pediococcus, and Weissella. Lactobacillus constitute an important genus within the phylum Firmicutes, class Bacilli, order Lactobacillales, and family Lactobacillaceae. Recently, the International Scientific Association for Prebiotics and Probiotics (Marco et al., 2021) reported that the genus Lactobacillus comprises over 250 species; the species that are more closely related (i.e., those that share similar physiological traits) are grouped under the same genus, Lactobacillus spp., with desirable probiotic attributes, and are now renamed, including the following: Lactobacillus casei (renamed as Lacticaseibacillus casei), Lactobacillus paracasei (renamed as Lacticaseibacillus paracasei), Lactobacillus rhamnosus (renamed as Lacticaseibacillus rhamnosus), Lactobacillus plantarum (renamed as Lactiplantibacillus plantarum), Lactobacillus brevis (renamed as Levilactobacillus brevis), Lactobacillus salivarius (renamed as Ligilactobacillus salivarius), Lactobacillus fermentum (renamed as Limosilactobacillus fermentum), and Lactobacillus reuteri (renamed as Limosilactobacillus reuteri) (Marco et al., 2021). Zheng et al. (2020) also proposed the reclassification of the genus Lactobacillus into 25 genera; the classification includes the genus Lactobacillus (Lactobacillus delbrueckii group), Paralactobacillus, and 23 other novel genera. Lactobacilli are predominant organisms involved in the fermentation of cereal-based foods and beverages in Africa. During lactic acid fermentation, LAB at the initial stage of fermentation improve the flavor, shelf life, nutritional values, and digestibility of fermented foods. The predominance and the involvement of Lactobacilli especially [L. plantarum (Lactiplantibacillus plantarum) and L. fermentum (Limosilactobacillus fermentum)] during food fermentation have been reported by several researchers (Oguntoyinbo and Narbad, 2015; Adesulu-Dahunsi et al., 2017a; Naghmouchi et al., 2019).
Lactobacillus species are widely applied in food production and are known for their fermentative ability, health, and nutritional benefits. The role of LAB in the safety and quality of fermented foods around the globe has been reviewed by several researchers (Antara et al., 2019; Chaves-López et al., 2020; Goel et al., 2020). Strains of Lactobacillus play key roles during food fermentation by contributing immensely to the microbiological safety and extension of food shelf life. During fermentation, Lactobacilli usually outcompete other microorganisms through the production of organic acids, which are inhibitory to potential competitors, leading to the elimination of pathogenic microorganisms. The co-occurrence and activities of the LAB species in the fermenting food matrix and their functional and technological roles during food production have a major impact on the food safety, nutrition, sensory characteristics, and shelf life extension, as these LAB species have been found to inhibit pathogenic microorganisms that may be present in the fermentation through increased pH and production of lactic acid, and removal of toxic compounds (Behera et al., 2018; Douillard and de Vos, 2019; Virdis et al., 2021). In Africa, diverse foods are produced from plant sources and animal milk, and these foods are naturally fermented into different types of edible products. Some of these plant-based fermented foods have their sources from cereal (maize, sorghum, and millet), cassava (tubers), African oil palm, fruits, and leafy vegetables (African locust beans, melon, castor oil seed, sesame, cotton seeds, fluted pumpkin bean, ripe plantain, the fruit of sand apple, fresh leaves of Cassia obtusifolia, etc.).
Although other types of microorganisms may be involved during the production of foods and beverages, the predominance of Lactobacilli during indigenous food fermentation is commonly associated with cereal-based and cassava-based foods; these substrates are processed into different types of foods and beverages. Most of the fermented cereal-based foods are consumed as complementary infant food and as cereal beverages in adults, while the fermented cassava-based foods are consumed as main course meals. Different fermented foods from cereals and cassava tubers include mawé (Beninese fermented sour dough); gowé (Beninese malted and fermented cereal-based beverage); tchoukoutou (Beninese home-brewed beer from sorghum); ben-saalga (Burkinabé fermented cereal gruel); dolo (Burkinabé fermented sorghum beer); Bikédi (Congolese fermented cassava tubers); poto poto (Congolese fermented maize dough); cingwada (East African retted cassava); kisk (Egyptian fermented cereal mixture); Bikédi, foufou, chikwangue, mbalampinda, tutu, tsaba, minsela, and tsiya borde (Ethiopian fermented beverages); injera (Ethiopian sour fermented flatbread); kocho (Ethiopian fermented bread); koko and koko sour water (Ghanaian fermented millet porridge and drink); agbelima and kenkey (Ghanaian fermented cassava-based food); kisra dégué (Ivorian fermented cereal gruel); wômi, baca, and doklu (Ivorian fermented cereal-based foods); attiéké, placali, and attoupkou (Ivorian fermented cassava-based foods); uji (Kenyan millet-based fermented porridge); ogi (Nigerian fermented cereal gruel); gari, fufu, and lafun (Nigerian fermented retted cassava); pito and kunun zaki (Nigerian fermented cereal-based beverages); ikigage (Rwandan fermented sorghum beer); hussuwa (Sudanese fermented sorghum food); (Sudanese fermented sorghum bread); mbege, togwa, and kivunde (Tanzanian retted cassava); komé (Togolese fermented cereal-based foods); koko and bushera (Ugandan fermented cereal beverage); and mahewu (Zimbabwean fermented cereal beverages) (Aboua et al., 1989; Keleke, 1996; Olasupo et al., 1997; Mestres et al., 1999; Kimaryo et al., 2000; Blandino et al., 2003; Lei and Jakobsen, 2004; Abriouel et al., 2006; Sawadogo-Lingani et al., 2007; Ndiaye et al., 2008; Hama et al., 2009; Ray and Sivakumar, 2009; Mukisa et al., 2012; Owusu-Kwarteng et al., 2012; Greppi et al., 2013; Sanni and Adesulu, 2013; Soro-Yao et al., 2013; Obinna-Echem et al., 2014; Djeni et al., 2015; Adesulu-Dahunsi et al., 2017b; Aka-Gbezo et al., 2017). Several examples of these African indigenous fermented foods and beverages (AIFFBs) ranging from cereal-based to cassava-based foods and beverages and the predominant Lactobacillus spp. associated are shown in Table 1.
Table 1. Africa indigenous cereal-based and cassava-based fermented foods and beverages and the predominant Lactobacillus spp. associated.
Diversity of LAB intraspecies interaction was reported to be associated with Ghanaian spontaneously fermented millet porridge and drink (koko and koko sour water), with L. fermentum as the predominant organisms. Assohoun et al. (2012) revealed the succession and predominance of LAB species during spontaneous fermentation of maize for the production of doklu (Ivorian maize dough). It was reported that after 24 h of fermentation, the percentage occurrence of L. plantarum was 64%, which was the highest, followed by other LAB: Weissella cibaria (22%), Pediococcus acidilactici (7%), and L. fermentum (7%); at 48 h fermentation time, L. plantarum spp. still predominate, having 56% of occurrence, and at the end of fermentation, the percentage of occurrence of L. fermentum was 100%. The predominance of LAB species especially L. plantarum in Nigeria traditional fermented foods and beverages is well documented (Oguntoyinbo and Narbad, 2015); this Lactobacillus strain plays significant roles during fermentation, and has been selected as a potential probiotic and starter culture due to the different properties it displayed, such as good acidification, hydrogen peroxide production, and variation in carbohydrate fermentation patterns (Rozos et al., 2018). From our previous study, the intraspecies differentiation of L. plantarum strains isolated from indigenous fermented foods was carried out using different molecular techniques for the selection of strains that can be used to produce foods with desirable functional properties or as an adjunct and/or starter culture during food fermentation processes. It is well established that the predominance of Lactobacillus spp. and their enzyme production capacity in African fermented foods have assisted in the breaking down of molecules of high-molecular-weight organic acids and compounds, bacteriocins, and hydrogen peroxide (Mokoena et al., 2016).
Lactic acid bacteria have been reportedly isolated at different fermentation stages, and researchers have recommended that the quality of the final food products is dependent on the microbial diversity, dynamics, and frequency of occurrence of these LAB species. Oyedeji et al. (2013) isolated and characterized the predominant LAB species during spontaneous fermentation of Nigerian cassava-based and cereal-based foods, fufu and ogi; the dominance in the population of the L. plantarum strains was observed, and the use of these LAB strains as a starter culture for production of fermented foods was suggested. Karaca et al. (2017) reported the predominance of Lactobacillus spp. (Lactobacillus gasseri, L. fermentum, L. brevis, and L. casei) during fermentation of Dégué (fermented millet beverages popularly consumed in some parts of African countries, namely, Burkina Faso, Ivory Coast, Senegal, Mali, Guinea, and Benin Republic).
In food processing industries, Lactobacilli constitute an important group of LAB, and the industrial importance of this Lactobacillus spp. cannot be overemphasized; they are commonly recommended as a starter culture for controlled fermentation of African indigenous fermented foods (Giraud and Cuny, 1997; Olasupo et al., 1997). The increase in demand for consistent and quality fermented products has resulted in the use of a starter culture for a more controlled fermentation process (Adebo et al., 2018; Masebe and Adebo, 2019). Controlled fermentation using a well-defined starter culture guarantees fast, consistent fermentation, the introduction of specific strains with exceptional functional properties, and food safety, though this technology is not widely practiced/used in many developing countries. Lactobacillus spp. associated with African indigenous fermented foods are known to exhibit many interesting beneficial traits and functional properties such as the production of the desired flavor, and antimicrobial production results in improved nutritional quality and safety of the food products and ultimately promotes the health status of the consumers. The roles and applications of Lactobacillus spp. are shown in Figure 1.
The use of a carefully selected functional starter culture with added nutritional benefits during microbial fermentation will enhance the production of fermented foods with improved quality (Nkhata et al., 2018). The technological effectiveness of LAB during food fermentation must be taken into consideration before selection as a functional starter culture or co-culture; some of these attributes include rapid acidification, development of antimicrobial compounds, probiotic features, and ability to improve the nutritional quality of fermented foods (Soro-Yao et al., 2014; Banwo et al., 2021). Many plant-based food substrates fermented with lactobacilli are reported to have high percentages of rhamnose (a naturally occurring deoxy sugar), and these sugars possess antioxidant properties that can be used as a substrate during Lactobacillus metabolism in cereal fermentation to produce vitamin-enriched foods and in combating nutritional deficiency in many African fermented cereal-based foods. During cereal-based fermentation, the dominance of Lactobacilli and other LAB with extracellular amylase production has resulted in the provision of metabolizable starch via hydrolysis and can be utilized for industrial fermentation processes, because of its acid production, which simultaneously improves the preservation and safety of fermented foods.
In cereal fermentation, the enzymatic activity of amylase displayed by Lactobacillus spp. in catalyzing the hydrolysis of amylose and amylopectin to yield fermentable maltose makes each strain desirable, important, and useful as a starter culture. In traditional food fermentation, using amylolytic lactic acid bacteria as a starter culture offers an advantage by combining both amylase production and acidification properties in a single strain. The raw starch binding ability of the α-amylase producing gene (amyA) of L. plantarum A6 (amylolytic strain isolated from retted cassava) with an unusual structure of the 3′ end of the α-amylase gene and the ability to break down raw starch has been successfully cloned and sequenced (Giraud et al., 1991; Giraud and Cuny, 1997). The L. plantarum A6 strain can synthesize large amounts of α-amylase that can be used as a starter culture during fermentation processes, resulting in the production of more lactic acid in the fermentation matrix.
Though beneficial starter cultures have not been fully utilized during the traditional fermentation of many cereal- and cassava-based products, fermented foods have probiotic potentials due to the Lactobacillus species present in the fermenting matrix. There are reports on the quality of some African fermented products that have been greatly improved upon using beneficial cultures (Nyanzi et al., 2013). Ogi was enhanced with a lactic acid starter culture to produce a functional food called Dogik. Nyanzi et al. (2013) reported the use of LAB associated with fermented foods to produce a starter culture possessing antimicrobial potentials; this starter culture has antimicrobial activities against diarrheagenic bacteria. During spontaneous fermentation of cereals to produce African fermented beverages, akamu and ogi, several LAB species such as L. plantarum, Lactobacillus pentosus, Lactobacillus cellobiosus, Pediococcus pentosaceus, and Leuconostoc mesenteroides were associated with L. plantarum, displaying the potential of being used as a starter culture (Nwachukwu et al., 2010). Oguntoyinbo and Narbad (2012) reported the multifunctional properties displayed by two strains of L. plantarum isolated from Nigerian cereal-based beverages (L. plantarum ULAG11 and L. plantarum ULAG24) and the potential of using these LAB strains for industrial processing of West African cereal-based foods and beverages, due to the probiotic function, amylase enzyme, and bacteriocin-producing ability exhibited by the strains (Mokoena et al., 2016).
The protective functions of many food-grade LAB in terms of improved food safety and food quality, against pathogenic and spoilage microorganisms during food fermentation, have attracted the attention of researchers. Biopreservatives are naturally occurring compounds that can be produced from microorganisms, plants, or animals. Some of the compounds produced by food-grade Lactobacillus spp. can be used to extend the food’s shelf life, because the antimicrobial metabolites released by these microorganisms may have some potential usage as natural preservatives that can be used to control or inactivate spoilage or pathogenic microorganisms in the fermenting food matrix; inhibition of the pathogenic or spoilage organisms that may be present in foods will result in increased food functionality and food quality (Pisoschi et al., 2018). Biopreservation using LAB and their metabolites connotes an alternative for improving food shelf life and safety (Reis et al., 2012). Many studies on the bacteriocinogenic effect of Lactobacillus spp. and its several applications in food preservations have been carried out. Many probiotic lactobacilli are reported to display inhibitory effects, which makes them useful as biological preservatives (Naghmouchi et al., 2019). The shelf life and safety of fermented foods can be enhanced through the biopreservative’s role as demonstrated by several LAB species in the form of competition to obtain nutrients during fermentation; this antagonistic form of interaction produces antimicrobial substances. Bacteriocin production by LAB is of great interest to the fermentation industry as some well-characterized bacteriocins have been reported to show a broad spectrum of activity on pathogenic and spoilage microorganisms (Cebrián et al., 2012; Naghmouchi et al., 2019; Vieco-Saiz et al., 2019).
Bacteriocins can be used as “biologically derived” preservatives; thus, food shelf life can be extended, and pathogenic organisms are inhibited without altering the product’s nutritional quality (Hugas et al., 2002; Ross et al., 2002). Lactic acid bacteria isolated from African fermented food are reported to demonstrate antimicrobial activity toward many pathogenic organisms, by the production of lactic acid and reduction of pH in vivo (Sanni et al., 2002). Many Lactobacillus species are known to be bacteriocin producers, and their presence in food inhibits the growth of pathogenic microorganisms. Olsen et al. (1995) reported the occurrence of antimicrobial compounds displayed by Lactobacillus species during fermentation of maize dough; the antagonistic interactions against pathogenic organisms in the fermented dough showed that the inhibitory compounds produced by the synergistic relationship between the strains of L. plantarum and L. fermentum were effective against both Gram-positive and Gram-negative bacteria, because of their ability to produce acids and bacteriocins. Adebayo et al. (2014) reported the use of bacteriogenic Lactobacillus strains (Lactobacillus fermentum and Lactobacillus casei) isolated from naturally fermented foods in Nigeria for effective control of spoilage and pathogenic microorganisms; the strains showed a broad range of activities and had a significant effect on the selected pathogenic microorganisms. Adedokun et al. (2016) demonstrated the use of a Lactobacillus strain as a food preservative agent. The antifungal activity of L. fermentum YML014 isolated from cassava-based fermented foods against food spoilage molds using tomato puree was investigated by Adedokun et al. (2016). It was found that the Lactobacillus fermentum YML104 showed strong antifungal activity against Aspergillus niger, Aspergillus flavus, and Penicillium expansum, and thus caused an increase in the shelf life of the tomato puree.
Production of antimicrobial compounds such as lactic acid, propionic acid, and diacetyl by LAB during fermentation results in a decrease in pH and inhibition of many pathogenic microorganisms. Rattanachaikunsopon and Phumkhachorn (2010) reported the inhibitory activity of reuterin produced by Lactobacillus reuteri against protozoa and fungi. Other inhibitory compounds such as organic acids, bacteriocins, and antifungal peptides produced by L. plantarum have been reportedly isolated from silage (De Vuyst and Leroy, 2007). Ben Omar et al. (2008) isolated 135 Lactobacillus species during the production of poto poto (Congolese fermented maize product) using species-specific PCR and 16S rRNA gene sequencing; 31 strains were identified as bacteriocin producers, and the bacteriocins produced by these LAB strains, L. plantarum (28) and L. fermentum (3), were identified as plantaricin and were found to display a broad spectrum of inhibition against the following pathogens: Escherichia coli, Salmonella enterica, Enterobacter aerogenes, Bacillus cereus, Staphylococcus aureus, Listeria monocytogenes, and Enterococcus faecalis. Lactobacillus spp. (L. plantarum F1 and L. brevis OG1) isolated from Nigerian fermented foods were reported to produce bacteriocins, which have a broad spectrum of inhibition against pathogenic and spoilage organisms; the Lactobacillus strains exhibited activities of 6,400 and 3,200 AU/ml, respectively, against Escherichia coli NCTC10418 and Enterococcus faecalis EF1, but did not inhibit Candida albicans ATCC10231 and Klebsiella sp. UCH15 (Ogunbanwo et al., 2003).
In another study, one L. rhamnosus and several L. plantarum strains isolated from sha’a (fermented maize beverage produced in Cameroon) produced bacteriocins that were able to inhibit both Gram-positive and Gram-negative bacteria, including species of the genera Lactobacillus, Streptococcus, Bacillus, Salmonella, Shigella, Pseudomonas, and Klebsiella and multidrug-resistant strains of the pathogens E. coli and S. aureus (Kaktcham et al., 1995). During food fermentations, Lactobacillus species have been reported to perform protective functions. Kaktcham et al. (1995) investigated the antimicrobial properties and protective functions of Lactobacillus spp. isolated during the fermentation of Cameroonian traditional cereal-based beverages and cow milk (Sha’a and Kossam). It was observed that 12 strains out of the 21 LAB species were reported to display inhibitory substances to proteolytic enzymes (Trypsin and Proteinase K) and the bacteriocins produced showed broad inhibitory activity against pathogenic microbes.
In cereal-based foods, LAB generally produces enzymes that assist in the breaking down of polysaccharides and other high-molecular-weight substances such as bacteriocins and hydrogen peroxide, thereby making it inhibitory for pathogenic organisms to survive (Mokoena et al., 2016). These LAB in fermented cereals also cause an increase in free amino acids and vitamin B groups, by breaking down antinutritional compounds, thus leading to the availability of iron, zinc, and calcium and production of gas and other volatile compounds and resulting in improved sensorial properties of the cereal-based foods and beverages (Blandino et al., 2003). Production of bacteriocins by LAB has also provided a good alternative to synthetic drugs and antibiotics. Lactobacillus produces antimicrobial ribosomally synthesized peptides, or “bacteriocins.” The LAB-producing bacteriocin has been reported to possess biopreservative characteristics, which makes them ideal food biopreservatives; some of these characteristics include the bacteriocin proteinaceous nature, in vivo non-toxicity and non-immunogenicity, inactivity against eukaryotic cells, thermoresistance against heat treatment, and broadened bactericidal activity (Naghmouchi et al., 2019; Vieco-Saiz et al., 2019). Several classes of LAB bacteriocins have their application in foods as food additives and control undesirable microorganisms in food (Heng et al., 2007). Nisin is the only FDA-approved bacteriocin produced from LAB species, and Lactococcus lactis is a widely studied biopreservative and has numerous applications as a food additive effective against foodborne pathogens and Gram-positive spoilage organisms during the production of cheese and dairy products. The effectiveness and application of bacteriocin in the food system have been widely reviewed (Cleveland et al., 2001; Naghmouchi et al., 2019). In the selection of bacteriocin-producing strains in food applications, the following must be put into consideration:
• The bacteriocin-producing strain should possess a “GRAS” status.
• The strain must display a broad spectrum of inhibition.
• The strain must be thermostable.
• The strain must possess beneficial effects and improve safety.
Probiotics are live microbes that impart health benefits to humans when an adequate amount is consumed (Hill et al., 2014). The significant effect of Lactobacillus as probiotic strains was first established at the beginning of the 20th century (1908) by the Russian scientist and Nobel Prize winner Elie Metchnikoff while working in Bulgaria; he hypothesized that the longevity of the Bulgarian centenarians was a result of the improved health benefits conferred upon daily intake of fermented yogurt containing Lactobacillus bulgaricus (Metchnikoff, 1908). Several indigenous fermented foods and dairy products have been studied to contain live microorganisms with potential health benefits (Lei and Jakobsen, 2004; Salmerón et al., 2015). Obiolor and kunu-zaki are examples of African fermented beverages with probiotic potentials and have indirectly impacted beneficial effects on the host; several attempts have been made to introduce probiotic drinks and beverages containing L. rhamnosus GR-1 and Streptococcus thermophilus in the form of a starter culture in the production of yogurt in Uganda, Tanzania, and Kenya (Salmerón et al., 2015; Nath et al., 2016; Westerik et al., 2020). The importance of probiotic microorganisms is so enormous to the development of the gastrointestinal tract (GIT), as probiotics have been described as a live microbial feed supplement that beneficially affects the host via improving the intestinal microbial balance (Steinkraus, 1995; Rezac et al., 2018). Lactobacillus has been well researched and is reported to be the most common microorganism associated with fermented foods, and most strains of this LAB species can reasonably confer a health benefit when an adequate amount is ingested (Hill et al., 2014; Sanders et al., 2018).
Lactobacilli have been reported as potential probiotics or employed as a starter culture, because of the essential roles they played during food fermentation. Up to 20 LAB species, consisting mainly of the genus Lactobacillus, were recognized by Canadian Food Inspection Agency [CFIA] (2013). Health Claims. Probiotic Claims. Summary table of acceptable non-strain specific claims for probiotics and eligible species for the claims. in Section 8.7.3 (Canadian Food Inspection Agency [CFIA], 2013). Many African fermented foods have been reported to confer probiotic attributes and enormous health benefits when consumed. These foods have probiotic potentials as so many isolated and characterized LAB are capable of surviving at low pH (2.5) and tolerating 0.3% bile salt for several hours. Application of viable cultures in controlling diarrhea among infants and their use as functional foods have been reported. Lei and Jakobsen (2004) reported the ameliorative effect in consuming koko sour water (Ghanaian spontaneously fermented millet drink) among diarrhea patients (diarrhea is the main cause of infant morbidity and mortality in developing countries). The koko and koko sour water millet porridge and drink were reported to contain an abundance of viable LAB, which are responsible for the beneficial effect conferred when these beverages are consumed. A similar report was obtained among Tanzanian children that were fed with togwa (lactic acid fermented cereal gruel) (Svanberg et al., 1992). A large number of LAB strains of the genus Lactobacillus and Bifidobacterium from fecal or vaginal sources are frequently reported compared to LAB of food origin, as many food-origin LAB are likely to be recovered from fecal sources.
Lactobacillus have been reported to have the ability to adhere to intestinal cells via coaggregation to form normal balanced flora and also produce compounds such as organic acids, hydrogen peroxides, and bacteriocins that are inhibitory to pathogenic organisms (Adeyemo et al., 2018; Ajayeoba and Ijabadeniyi, 2019). Several benefits are associated with the consumption of foods containing sufficient amounts of probiotic-containing well-defined and viable food-grade microorganisms (Dicks and Botes, 2010; Yadav et al., 2011; Furtado-Martins et al., 2013; Pasolli et al., 2020); these benefits include the following:
• Regulation of gut microbiota, which, in turn, leads to the improvement of the intestinal health of the host.
• Immune system development and prevention of infectious diseases.
• Improved nutritional quality of fermented products.
• Enhancement of the bioavailability of nutrients.
• Alleviation of allergies and of symptoms that may be associated with the lactose-intolerant individuals.
• Development of nutraceutical and/or functional foods.
• Production of bioactive compounds that enhances the functionality of foods and beverages.
• Lowering of serum cholesterol levels.
• Reduction in the risk of contracting some diseases.
• Prevention of cancer.
To harness the full LAB potentials of food origin, daily consumption of fermented foods is recommended. In the consensus statement on fermented foods issued by the International Scientific Association for Probiotics and Prebiotics (Marco et al., 2021), the panelists recommended that the term “Probiotic” should be used when demonstrated health benefits are conferred on humans by well-defined and characterized live and active microbes. From the conclusion, it is not enough that fermented foods contain viable and active microbes, but foods that will be labeled as probiotics must possess additional health benefits with proven safety and should ensure that such strain-specific products must be able to confer the required benefits on the host when the adequate amount is consumed.
The inclusion and activities of bacteria as part of the human gut microbiota play valuable roles in the general health and wellbeing of man. The consumption of fermented foods is very crucial to gut health, as the LAB associated with these foods assist in boosting the good/beneficial microflora in the intestinal tract, resulting in the increased gut microbiome in the digestive system through which the human immune system is enhanced. Several investigators have recommended the consumption of traditional fermented foods as a veritable source of probiotics in humans (Mahasneh and Abbas, 2010; Jafari et al., 2011). Daily consumption of fermented foods containing live microorganisms will aid the delivery of substantial beneficial microorganisms to the GITs, as the presence of microorganisms associated with fermented foods in the GIT is sparsely documented (Zhang et al., 2016; Rezac et al., 2018). Some probiotic LAB isolated from the human gut have displayed their functionality in health improvement and have also been found to display some characteristics such as immune system improvement, inhibition of pathogenic bacteria, and modulation of epithelial cell permeability, which is relevant to therapeutic and/or prophylactic treatments against various diseases (Anderson et al., 2010; Ashida et al., 2011; Diaz Heijtz et al., 2011; Ahern et al., 2014; Wang et al., 2018).
Investigations have shown that fermented foods are the source of LAB represented in the gut microbiome. The predominance of these LAB in the human gut is a result of different contributing factors, age, lifestyle, geographical location, diet, and use of antibiotics, as the gut microbial composition is directly influenced by main dietary composition (Brewster et al., 2019). Several LAB species associated with fermented foods have been reported to have similar physiological traits with the strains known for improving gut health. The increasing interest in the use of probiotic LAB as a vehicle for drug delivery and treatment of GIT diseases has also been well documented (Mokoena et al., 2016; Wang et al., 2018). The probiotic microbes associated with fermented foods have immensely contributed to the protective roles in the gut as they are found to exhibit a strong inhibitory effect in vitro via organic acid production. Lactobacillus spp. also contribute to the healthy microbiota of human mucosal surfaces.
Gut bacteria perform several functions in the maintenance of human health, including production of vitamins, regulation of gut motility and development, maintenance of epithelial integrity by regulating tight junction permeability, inhibition of pathogenic microorganisms, and development of the central nervous system (CNS; Ashida et al., 2011; Erny et al., 2015; Mangalam et al., 2017). It has been proposed that gut bacteria are required to maintain epithelial integrity by regulating tight junction permeability. Karczewski et al. (2010) reported the ability of the LAB strain L. plantarum WCFS1 in enhancing the intestinal barrier of the host with intestinal disorders; the experiment showed the ability of the L. plantarum strain to regulate tight-junction proteins and to protect against chemical-induced disruption of the epithelial barrier. L. rhamnosus species have also been reported to demonstrate adherence to the gut epithelial tissue, resulting in the colonization of human GIT (Collins et al., 1998; Dunne et al., 2001).
Factors such as excessive antibiotic usage, stress, and diseases can alter the gut microbiota and may lead to dysbacteriosis. Consumption of fermented foods has been proven to be the easiest way of introducing potentially beneficial microorganisms to the GIT, though persistency of the probiotic LAB of food origin in the gut may not occur for a long time; it is recommended that regular consumption of these fermented foods will enhance the ability of the food-associated microorganisms to confer probiotic potentials in human (Derrien and Vlieg, 2015; Zhang et al., 2016). Thus, daily consumption of fermented foods is recommended, as it will enhance a healthy gut because of the innate beneficial microorganisms present in these foods.
In Africa, the biotechnological application, development of bioreactor technology, and the use of a starter culture for food fermentation processes should be prioritized through technical skills training, provision of infrastructures, and government willingness in funding research in the area of upgrading fermentation processes and fortification of these foods with bio-enriched vitamins. Also, synergism among research institutes, universities, and manufacturing sectors will play a major role in bringing starter culture development and usage to the limelight in the food industry; this will guarantee consistent and safe food with improved shelf life. Several foods produced in Africa are fermented before consumption, and it is highly recommended that among the household food producers, good manufacturing practices should be effectuated from the point of collection of the raw materials/substrates because of the risks that are associated with improperly/unhygienically produced foods during the production of the final food products. The use of safe water should also be emphasized throughout the production/fermentation process; food should not be manufactured under poor hygienic conditions, and all these can be achieved by properly educating the food producers. The activities of fermentative LAB and most especially Lactobacillus strains have played substantial roles in ensuring food security and extension of food shelf life. Production of antimicrobial substances (most especially bacteriocins) by Lactobacillus spp. and the spectrum of antibacterial activity displayed by these species can find their wide applications in the food industry. As applications of Lactobacillus are enormous and wide usage as a probiotic and/or as a starter culture due to their ability to improve the product’s nutritional and technological features cannot be neglected, this LAB group occupies a central role during the fermentation process and significantly possesses many benefits as discussed in this review, with long and safe usage in the food industry. Lactobacillus species (those associated with African fermented foods) are described as key players during fermentation technology and have been reportedly involved in the enhancement of flavor, texture, and improved rheology with health-promoting characteristics, which can be applied in the production of functional foods, thus conferring beneficial attributes and consistency in food quality and thereby making the African food market/industry attractive. However, the use of appropriate metagenomic tools that will provide insights into the gene, structure, and functions of microbial strains with multi-functional properties during controlled fermentation will guarantee product quality and consistency, which will be accessible to all. In developing products with improved quality and safety, the inclusion of technologically relevant microorganisms (such as Lactobacillus strains) is crucial and will serve as sustainable interventions for the development of African-specific starter cultures. Development of starter culture technology for both small-scale and industrial-scale food production will lead to products of greater consistency, safe quality, and global acceptance, and all these should be the focus of African researchers and scientists. It can be observed that despite the rich diversity of food-grade microorganisms present in many African indigenous fermented foods, most of the well-defined starter cultures available in the shops and markets are not manufactured in Africa; therefore, there is a need to produce well-defined and well-characterized microbial cultures from autochthonous microorganisms peculiar to African foods.
AA-D: conceptualization and writing—original draft preparation. TA: writing part of the primary draft. AA-D and SD: technical editing of the manuscript and reviewing the manuscript for submission. All authors have read and agreed to the published version of the manuscript.
AA-D acknowledges and appreciates the grant received for this research from the African-German Network of Excellence in Science (AGNES), supported by the Federal Ministry of Education and Research (BMBF), and Alexander von Humboldt Foundation (AvH). The authors also acknowledge the financial support of Bowen University Iwo, Nigeria, in the payment of the article Open Access Publication Fees.
The authors declare that the research was conducted in the absence of any commercial or financial relationships that could be construed as a potential conflict of interest.
All claims expressed in this article are solely those of the authors and do not necessarily represent those of their affiliated organizations, or those of the publisher, the editors and the reviewers. Any product that may be evaluated in this article, or claim that may be made by its manufacturer, is not guaranteed or endorsed by the publisher.
Aboua, F., Nemlin, J., Kossa, A., and Kamenan, A. (1989). Transformation traditionnelle de quelques céréales cultivées en Côte d’Ivoire. Colloque International de Technologie: Céréales en régions chaudes. Centre universitaire de N’gaoundéré, Cameroon, 22–26 February 1988. France: John Libbey Eurotext.
Abriouel, H., Ben, Omar N, López, R. L., Martínez-Can̄amero, M., Keleke, S., and Gálvez, A. (2006). Culture-independent analysis of the microbial composition of the African traditional fermented foods poto poto and dèguè by using three different DNA extraction methods. Int. J. Food Microbiol. 111, 228–233. doi: 10.1016/j.ijfoodmicro.2006.06.006
Adebayo, F. A., Afolabi, O. R., and Akintokun, A. K. (2014). Antimicrobial properties of purified bacteriocins produced from Lactobacillus casei and Lactobacillus fermentum against selected pathogenic microorganisms. J. Adv. Med. Medical Res. 4, 3415–3431. doi: 10.9734/BJMMR/2014/8584
Adebo, O. A., Njobeh, P. B., Adeboye, A. S., Adebiyi, J. A., Sobowale, S. S., Ogundele, O. M., et al. (2018). “Advances in fermentation technology for novel food products,” in Innovations in Technologies for Fermented Food and Beverage Industries, Vol. 2018, eds S. Panda and P. Shetty (Switzerland: Springer), 71–87.
Adedokun, E. O., Rather, I. A., Bajpai, V. K., and Park, Y. H. (2016). Biocontrol efficacy of Lactobacillus fermentum YML014 against food spoilage moulds using the tomato puree model. Front. Life Sci. 9, 64–68. doi: 10.1080/21553769.2015.1084951
Adesulu, A. T., and Awojobi, K. O. (2014). Enhancing sustainable development through indigenous fermented food products in Nigeria. Afr. J. Microbiol. Res. 8, 1338–1343. doi: 10.5897/AJMR2013.5439
Adesulu-Dahunsi, A. T., Dahunsi, S. O., and Olayanju, A. (2020). Synergistic microbial interactions between lactic acid bacteria and yeasts during production of Nigerian indigenous fermented foods and beverages. Food Cont. 110:106963. doi: 10.1016/j.foodcont.2019.106963
Adesulu-Dahunsi, A. T., Jeyaram, K., and Sanni, A. I. (2018). Probiotic and technological properties of exopolysaccharide producing lactic acid bacteria isolated from some cereal- based Nigerian indigenous fermented food products. Food Cont. 92, 225–231. doi: 10.1016/j.foodcont.2018.04.062
Adesulu-Dahunsi, A. T., Sanni, A. I., Jeyaram, K., and Banwo, K. (2017a). Genetic diversity of Lactobacillus plantarum strains from some indigenous fermented foods in Nigeria. LWT Food Sci. Technol. 82, 199–206. doi: 10.1016/j.lwt.2017.04.055
Adesulu-Dahunsi, A. T., Sanni, A. I., and Jeyaram, K. (2017b). Rapid differentiation among Lactobacillus, Pediococcus and Weissella species from some Nigerian indigenous fermented foods. LWT Food Sci. Technol. 77, 39–44. doi: 10.1016/j.lwt.2016.11.007
Adeyemo, S. M., Agun, T. F., and Ogunlusi. (2018). Antimicrobial activity of lactic acid bacteria isolated from ‘pupuru’: an African fermented staple against food borne- pathogens. J. Mol. Biol. Biotechnol. 3, 1–6. doi: 10.9734/ajrb/2019/v5i330090
Ahern, P. P., Faith, J. J., and Gordon, J. I. (2014). Mining the human gut microbiota for effector strains that shape the immune system. Immunity 40, 815–823. doi: 10.1016/j.immuni.2014.05.012
Ajayeoba, T. A., and Ijabadeniyi, O. A. (2019). Characterization and antioxidant ability of potential probiotic lactic acid bacteria in ogi liquor and lemon juice-ogi liquor. Microbiology 69, 777–786. doi: 10.1007/s13213-019-01469-4
Aka-Gbezo, Konan, A. G., Achi, P., Koffi-Nevry, R., Koussemon-Camara, M., and Bbonfoh, B. (2017). Screening of antimicrobial activity of lactic acid bacteria isolated from Anango baca slurry, a spontaneously fermented maize product used in Côte d’Ivoire. Int. J. Biol. Chem. Sci. 11, 2616–2629. doi: 10.4314/ijbcs.v11i6.6
Anderson, R. C., Cookson, A. L., McNabb, W. C., Park, Z., McCann, M. J., Kell, W. J., et al. (2010). Lactobacillus plantarum MB452 enhances the function of the intestinal barrier by increasing the expression levels of genes involved in tight junction formation. BMC Microbiol. 10:316. doi: 10.1186/1471-2180-10-316
Antara, N. S., Gunam, I. B. W., Kencana, F. K. D., and Utama, I. S. (2019). “The role of lactic acid bacteria on safety and quality of fermented foods,” in AIP Conference Proceedings 2155, 020005 (Maryland, US: AIP), doi: 10.1063/1.5125509
Ashida, H., Ogawa, M., Kim, M., Mimuro, H., and Sasakawa, C. (2011). Bacteria and host interactions in the gut epithelial barrier. Nat. Chem. Biol. 8, 36–45. doi: 10.1038/nchembio.741
Assohoun, M. C. N., Djeni, T. N., N’Guessan, F. K., and Koussemon, M. (2012). Preliminary study on antimicrobial properties of lactic acid bacteria involved in the fermentation of corn dough during Doklu processing in Cote D’ivore. Food 6, 65–70.
Banwo, K., Asogwa, F. C., Ogunremi, O. R., Adesulu-Dahunsi, A., and Sanni, A. (2021). Nutritional profile and antioxidant capacities of fermented millet and sorghum gruels using lactic acid bacteria and yeasts. Food Biotechnol. 35, 1–22. doi: 10.1080/08905436.2021.1940197
Bationo, F., Humblot, C., Songre-Ouattara, L. T., Hama-Ba, F., Le Merrer, M., Chapron, M., et al. (2019). Total folate in West African cereal-based fermented foods: bioaccessibility and influence of processing. J. Food Comp. Anal 85:103309. doi: 10.1016/j.jfca.2019.103309
Behera, S. S., Ray, R. C., and Zdolec, N. (2018). Lactobacillus plantarum with functional properties: an approach to increase safety and shelf-life of fermented foods. Biomed. Res. Int. 2018:9361614. doi: 10.1155/2018/9361614
Ben Omar, N., Abriouel, H., Keleke, S., Valenzuela, A. S., Martínez-Cañamero, M., López, R. L., et al. (2008). Bacteriocin-producing Lactobacillus strains isolated from poto poto, a Congolese fermented maize product, and genetic fingerprinting of their plantaricin operons. Int. J. Food Microbiol. 127, 18–25. doi: 10.1016/j.ijfoodmicro.2008.05.037
Blandino, A., Al-Aseeri, M. E., Pandiella, S. S., Cantero, D., and Webb, C. (2003). Cereal-based fermented foods and beverages. Food Res. Int. 36, 527–543. doi: 10.1111/1750-3841.13422
Brewster, R., Tamburini, F. B., Asiimwe, E., Oduaran, O., Hazelhurst, S., and Bhatt, A. S. (2019). Surveying gut microbiome research in Africans: toward improved diversity and representation. Trends Microbiol. 27, 824–835. doi: 10.1016/j.tim.2019.05.006
Canadian Food Inspection Agency [CFIA] (2013). Section 8.7.3 Health Claims. Probiotic Claims. Summary Table of Acceptable Non-strain Specific Claims for Probiotics and Eligible Species for the Claims. Available online at: http://www.inspection.gc.ca/english/fssa/labeti/guide/ch8ae.shtml#a8_7
Cebrián, R., Baños, A., Valdivia, E. R., Pérez-Pulido, R., Martínez-Bueno, M., and Maqueda, M. (2012). Characterization of functional, safety, and probiotic properties of Enterococcus faecalis UGRA10, a new AS-48-producer strain. Food Microbiol. 30, 59–67. doi: 10.1016/j.fm.2011.12.002
Chaves-López, C., Rossi, C., Maggio, F., Paparella, A., and Serio, A. (2020). Changes occurring in spontaneous maize fermentation: An overview fermentation. MDPI 6:36. doi: 10.3390/fermentation6010036
Cleveland, J., Montville, T. J., Nes, I. F., and Chikindas, M. L. (2001). Bacteriocins: safe, natural antimicrobials for food preservation Int. J. Food Microbiol. 71, 1–20. doi: 10.1016/S0168-1605(01)00560-8
Collins, J., Thornton, G., and Sullivan, G. (1998). Selection of probiotic strains for human applications. Int. Dairy J. 8:48790.
De Vuyst, L., and Leroy, F. (2007). Bacteriocins from lactic acid bacteria: production, purification, and food applications. J. Mol. Microbiol. Biotechnol. 13:1949. doi: 10.1159/000104752
Derrien, M., and Vlieg, J. E. T. (2015). Fate, activity, and impact of ingested bacteria within the human gut microbiota. Trends Microbiol. 23, 354–366. doi: 10.1016/j.tim.2015.03.002
Diaz, M., Kellingray, L., Akinyemi, N., Adefiranye, O. O., Olaonipekun, A. B., and Bayili, G. R. (2019). Comparison of the microbial composition of African fermented foods using amplicon sequencing. Scientific Rep. 9:13863. 1 doi: 10.1038/s41598-019-50190-4
Diaz Heijtz, R., Wang, S., Anuar, F., Qian, Y., Björkholm, B., Samuelsson, A., et al. (2011). Normal gut microbiota modulates brain development and behavior. Proc. Natl. Acad. Sci. U.S. A. 108, 3047–3052. doi: 10.1073/pnas.1010529108
Dicks, L. M. T., and Botes, M. (2010). Probiotic lactic acid bacteria in the gastrointestinal tract: health benefits, safety and mode of action. Ben. Microbes 1, 11–29. doi: 10.3920/BM2009.0012
Djeni, N. T., Bouatenin, K. M. J.-P., Assohoun, N. M. C., Toka, D. M., Menan, E. H., Dousset, X., et al. (2015). Biochemical and microbial characterization of cassava inocula from the three main attieke production zones in Côte d’Ivoire. Food Cotrol 50, 133–140. doi: 10.1016/j.foodcont.2014.08.046
Douillard, F. P., and de Vos, W. M. (2019). Biotechnology of health-promoting bacteria. Biotechnol. Adv. 37:107369. doi: 10.1016/j.biotechadv.2019.03.008
Dunne, C., O’Mahony, L., Murphy, L., Thornton, G., Morrissey, D., O’halloran, S., et al. (2001). In vitro selection criteria for probiotic bacteria of human origin: correlation with in vivo findings. Am. J. Clin. Nutr. 73:386S92S. doi: 10.1093/ajcn/73.2.386s
Erny, D., Hrabe, de Angelis, A. L. H., Jaitin, D., Wieghofer, P., Staszewski, O., et al. (2015). Host microbiota constantly control maturation and function of microglia in the CNS. Nat. Neurosci. 18, 965–977. doi: 10.1038/nn.4030
Furtado-Martins, E. M., MotaRamos, A., Vanzela, E. S. L., Stringheta, P. C., Pinto, C. L. O., and Martins, J. M. (2013). Products of vegetable origin: A new alternative for the consumption of probiotic bacteria. Food Res. Int. 51, 764–770. doi: 10.1016/j.foodres.2013.01.047
Giraud, E., Brauman, A., Keleke, S., Lelong, B., and Raimbault, M. (1991). Isolation and physiological study of an amylolytic strain of Lactobacillus plantarum. Appl. Microbiol. Biotechnol. 36, 379–383. doi: 10.1007/BF00208160
Giraud, E., and Cuny, G. (1997). Molecular characterization of the α-amylase genes of Lactobacillus plantarum A6 and Lactobacillus amylovorus reveals an unusual 3’ end structure with direct tandem repeats and suggests a common evolutionary origin. Gene 198, 149–157. doi: 10.1016/S0378-1119(97)00309-0
Goel, A., Halami, P. M., and Tamang, J. P. (2020). Genome Analysis of Lactobacillus plantarum Isolated from some Indian fermented foods for bacteriocin production and probiotic marker genes. Front. Microbiol. 11:40. doi: 10.3389/fmicb.2020.00040
Greppi, A., Rantsiou, K., Padonou, W., Hounhouigan, J., Jespersen, L., Jakobsen, M., et al. (2013). Determination of yeast diversity in ogi, mawè, gowè and tchoukoutou by using culture-dependent and -independent methods. Int. J. Food Microbiol. 165, 84–88. doi: 10.1016/jijfoodmicro.2013.05.005
Hama, F., Savadogo, A., Ouattara, C. A. T., and Traore, A. (2009). Biochemical, microbial and processing study of dèguè a fermented food (from pearl millet dough) from Burkina Faso. Pakist. J. Nutri. 8, 759–764.
Heng, N. C. K., Wescombe, P. A., Burton, J. P., Jack, R. W., and Tagg, J. R. (2007). “The diversity of bacteriocins in Grampositive bacteria,” in Bacteriocins: Ecology and Evolution, eds M. A. Riley and M. A. Chavan (Germany: Springer-Verlag Heidelberg), 45–92.
Hill, C., Guarner, F., Gibson, G. R., Merenstein, D. J., Pot, B., Morelli, L., et al. (2014). The International Scientific Association for Probiotics and Prebiotics consensus statement on the scope and appropriate use of the term probiotic. Nat. Rev. Gastroenterol. Hepatol. 11, 506–514. doi: 10.1038/nrgastro.2014.66
Hugas, M., Garriga, M., Pascual, M., Aymerich, M. T., and Monfort, J. M. (2002). Enhancement of sakacin K activity against Listeria monocytogenes in fermented sausages with pepper or manganese as ingredients. Food Microbiol. 19, 519–528. doi: 10.1006/fmic.2002.0497
Jafari, B., Rezaie, A., and Alizadeh, S. (2011). Isolation and identification of potentially probiotic bacteria from traditional dairy products of Ardabil region in Iran. Ann. Biol. Res. 2011:31117.
Kaktcham, P. M., Zambou, N. F., Tchouanguep, F. M., El-Soda, M., and Choudhary, M. I. (1995). Antimicrobial and Safety Properties of Lactobacilli Isolated from two Cameroonian Traditional Fermented Foods. Sci. Pharm. MDPI 80, 189–203.
Karaca, O. B., Agani, B., and Erginkaya, Z. (2017). “Cereal based fermented dairy beverage of West Africa: Déguê,” in Proceedings of the 4th North and East European Congress on Food (NEEFood), Kaunas.
Karczewski, J., Troost, F. J., Konings, I., Deker, J., Kleerembezem, M., Bumer, R., et al. (2010). Regulation of human epithelial tight junction proteins by Lactobacillus plantarum in vivo and protective effects on the epithelial barrier. Am. J. Physiol. Gastrointest Liver Physiol. 298, G851–G859. doi: 10.1152/ajpgi.00327.2009
Keleke, S. (1996). Le rouissage du manioc: contribution à l’étude du phénomène de ramollissement des racines de manioc. France: UPEC.
Kimaryo, V., Massawe, G., Olasupo, N., et al. (2000). The use of a starter culture in the fermentation of cassava for the production of kivunde, a traditional Tanzanian food product. Int. J. Food Microbiol. 56, 179–190. doi: 10.1016/s0168-1605(00)00159-8
Lei, V., and Jakobsen, M. (2004). Microbiological characterization and probiotic potential of koko and koko sour water, African spontaneously fermented millet porridge and drink. J. Appl. Microbiol. 96, 384–397. doi: 10.1046/j.1365-2672.2004.02162.x
Liu, M., van Enckevort, F. H., and Siezen, R. J. (2005). Genome update: lactic acid bacteria genome sequencing is booming. Microbiology 151, 3811–3814. doi: 10.1099/mic.0.28557-0
Mahasneh, A. M., and Abbas, M. M. (2010). Probiotics and traditional fermented foods: the eternal connection. Jordan J. Biol. Sci. 3:13340.
Makarova, K., Slesarev, A., Wolf, Y., Sorokin, A., Mirkin, B., Koonin, E. A., et al. (2006). Comparative genomics of the lactic acid bacteria. Proc. Natl. Acad. Sci. U.S.A. 103, 15611–15616.
Mangalam, A., Shahi, S. K., Luckey, D., Karau, M., Marietta, E., Luo, N., et al. (2017). Human gut derived commensal bacteria suppress CNS inflammatory and demyelinating disease. Cell Rep. 20, 1269–1277. doi: 10.1016/j.celrep.2017.07.031
Marco, M. L., Hill, C., Hutkins, R., Slavin, J., Tancredi, D. J., Merenstein, D., et al. (2020). Should there be a recommended daily intake of microbes? J. Nutr. 150, 3061–3067. doi: 10.1093/jn/nxaa323
Marco, M. L., Sanders, M. E. Gänzle, M., Arrieta, M. C., Cotter, P. D. De Vuyst, L., et al. (2021). The international scientific association for probiotics and prebiotics (ISAPP) consensus statement on fermented foods article. Nat. Rev. Gastroenterol. Hepatol. 18, 196–208. doi: 10.1038/s41575-020-0390-395
Masebe, K. M., and Adebo, O. A. (2019). “Production and quality characteristics of a probiotic beverage from watermelon (Citrullus lanatus),” in Engineering, Technology and Waste Management (SETWM-19), Proceedings of the 17th Johannesburg International Conference on Science, Johannesburg, South Africa, eds E. Fosso-Kankeu, F. Waanders, and H. K. P. Bulsara (Johannesburg: Eminent Association of Pioneers and North-West University), 42–49.
Melini, F., Melini, V., Luziatelli, F., Ficca, A. G., and Ruzzi, M. (2019). Health-promoting components in fermented foods: An up-to-date systematic review. Nutrients 11:1189. doi: 10.3390/nu11051189
Mestres, C., Hounhouigan, J. D., Nago, M. C., and Barro, C. (1999). L’aklui sec: Un nouveau produit de petit déjeuner prêt à l’emploi: Expérience d’une production artisanale au Bénin. Agricult. Dével. 23, 108–117.
Mokoena, M. P., Mutanda, T., and Olaniran, A. O. (2016). Perspectives on the probiotic potential of lactic acid bacteria from African traditional fermented foods and beverages. Food Nut. Res. 60:29630. doi: 10.3402/fnr.v60.29630
Mukisa, I. M., Porcellato, D., Byaruhanga, Y. B., Muyanja, C. M., Rudi, K., Langsrud Tand, et al. (2012). The dominant microbial community associated with fermentation of Obushera (sorghum and millet beverages) determined by culture-dependent and culture-independent methods. Int. J. Food Microbiol. 160, 1–10. doi: 10.1016/j.ijfoodmicro.2012.09.023
Muyanja, M. B. K., Narvhus, J. A., Treimo, J., and Langsrud, T. (2003). Isolation, characterisation and identification of lactic acid bacteria from bushera: a Ugandan traditional fermented beverage Int. J. Food Microbiol. 80, 201–210. doi: 10.1016/S0168-1605(02)00148-4
Naghmouchi, K., Belguesmia, Y., Bendali, F., Spano, G., Seal, B. S., and Drider, D. (2019). Lactobacillus fermentum: a bacterial species with potential for food preservation and biomedical applications. Crit. Rev. Food Sci. Nut. 60, 3387–3399. doi: 10.1080/10408398.2019.1688250
Nath, A. K., Gupta, A., Niopany, B., Vyas, G., Maneesri, J., Thakur, N., et al. (2016). “Biotechnology and traditional fermented foods,” in Indigenous Fermented Foods of South East Asia, ed. V. K. Joshi (Boca Raton: CRC Press).
Ndiaye, C., Xu, S. Y., Ngom, P. M., and Ndoye, A. S. (2008). Malting germination effect on rheological properties and cooking time of millet (P. typhoïdes) and sorghum (S. bicolor) flours and rolled flour products (arraw). Am. J. Food Technol. 3, 373–383.
Nkhata, S. G., Ayua, E., Kamau, E. H., and Shingiro, J. (2018). Fermentation and germination improve nutritional value of cereals and legumes through activation of endogenous enzymes. Food Sci. Nutr. 6, 2446–2458. doi: 10.1002/fsn3.846
Nwachukwu, E., Achi, O. K., and Ijeoma, I. O. (2010). Lactic acid bacteria in fermentation of cereals for the production of indigenous Nigerian foods. Afr. J. Food Sci. Technol. 1, 021–026.
Nyanzi, R., Jooste, P. T., Cameron, M., and Witthuhn, C. (2013). Comparison of rpoA and pheS Gene Sequencing to 16S rRNA Gene Sequencing in Identification and Phylogenetic Analysis of LAB from Probiotic Food Products and Supplements. Food Biotechnol. 27, 303–327. doi: 10.1080/08905436.2013.838783
Obinna-Echem, P. C., Kuri, V., and Beal, J. (2014). Evaluation of the microbial community, acidity and proximate composition of akamu, a fermented maize food. J. Sci. Food Agric. 94, 331–340. doi: 10.1002/jsfa.6264
Ogunbanwo, S. T., Sanni, A. I., and Onilude, A. A. (2003). Characterization of bacteriocin produced by Lactobacillus plantarum F1 and Lactobacillus brevis OG1. Afr. J. Biotechnol. 2, 219–227. doi: 10.5897/ajb2003.000-1045
Oguntoyinbo, F. A., and Narbad, A. (2012). Molecular characterization of lactic acid bacteria and in situ amylase expression during traditional fermentation of cereal foods. Food Microbiol. 31, 254–262.
Oguntoyinbo, F. A., and Narbad, A. (2015). Multifunctional properties of Lactobacillus plantarum strains isolated from fermented cereal foods. J. Funct. Foods 17, 621–631. doi: 10.1016/j.jff.2015.06.022
Olasupo, N. A., Olukoya, D. K., and Odunfa, S. A. (1997). Identification of Lactobacillus Species Associated with Selected African Fermented Foods. Z. Naturforsch. 52, 105–108.
Olsen, A., Halm, M., and Jakobsen, M. (1995). The antimicrobial activity of lactic acid bacteria from fermented maize (kenkey) and their interactions during fermentation. J. Appl. Bacteriol. 79, 506–512. doi: 10.1111/j.1365-2672.1995.tb03170.x
Owusu-Kwarteng, J., Akabanda, F., Nielsen, D. S., Tano-Debrah, K., Glover, R. L., and Jespersen, L. (2012). Identification of lactic acid bacteria isolated during traditional fura processing in Ghana. Food Microbiol. 32, 72–78. doi: 10.1016/jfm.2012.04.010
Oyedeji, O., Ogunbanwo, S. T., and Onilude, A. A. (2013). Predominant lactic acid bacteria involved in the traditional fermentation of fufu and ogi, two Nigerian fermented food products. Food Nutr. Sci. 4, 40–46. doi: 10.4236/fns.2013.411A006
Pasolli, E., De Filippis, F., Mauriello, I. E., Cumbo, F., Walsh, A. M., Leech, J., et al. (2020). Large-scale genome-wide analysis links lactic acid bacteria from food with the gut microbiome. Nat. Commun. 11:2610. doi: 10.1038/s41467-020-16438-8
Pfeiler, E. A., and Klaenhammer, T. R. (2007). The genomics of lactic acid bacteria. Trends Microbiol. 15, 546–553.
Pisoschi, A. M., Pop, A., Georgescu, C., et al. (2018). An overview of natural antimicrobials role in food. Europ. J. Med. Chem. 143, 922–935. doi: 10.1016/j.ejmech.2017.11.095
Pswarayi, F., and Ganzle, M. G. (2019). Composition and origin of the fermentation microbiota of Mahewu, a Zimbabwean fermented cereal beverage. Appl. Environ. Microbiol. 85, 3130–3118. doi: 10.1128/AEM.03130-18
Rattanachaikunsopon, P., and Phumkhachorn, P. (2010). Lactic acid bacteria: their antimicrobial compounds and their uses in food production. Ann. Biol. Res. 1:21828.
Ray, R. C., and Sivakumar, P. S. (2009). Traditional and novel fermented foods and beverages from tropical root and tuber crops: review. Int. J. Food Sci. Technol. 44, 1073–1087.
Reis, J. A., Paula, A. T., and Casarotti, S. N. (2012). Lactic acid bacteria antimicrobial compounds: Characteristics and applications. Penna Food Eng. Rev. 4, 124–140. doi: 10.1007/s12393-012-9051-2
Rezac, S., Kok, C. R., Heermann, M., and Hutkins, R. (2018). Fermented Foods as a Dietary Source of Live Organisms. Front. Microbiol. 9:1785. doi: 10.3389/fmicb.2018.01785
Rodríguez, L. G. R., Mohamed, F., Bleckwedel, J., Medina, R., De Vuyst, L., Hebert, E. M., et al. (2019). Diversity and functional properties of lactic acid bacteria isolated from wild fruits and flowers present in northern Argentina. Front. Microbiol. 21:1091. doi: 10.3389/fmicb.2019.01091
Ross, R. P., Morgan, S., and Hill, C. (2002). Preservation and fermentation: past, present and future Int. J. Food Microbiol. 79, 3–16. doi: 10.1016/s0168-1605(02)00174-5
Rozos, G., Voidarou, C., Stavropoulou, E., Skoufos, I., Tzora, A., Alexopoulos, A., et al. (2018). Biodiversity and microbial resistance of lactobacilli isolated from the traditional Greek cheese kopanisti. Front. Microbiol. 9:517. doi: 10.3389/fmicb.2018.00517
Salmerón, I., Thomas, K., and Pandiella, S. S. (2015). Effect of potentially probiotic lactic acid bacteria on the physicochemical composition and acceptance of fermented cereal beverages. J. Funct. Foods 15, 106–115. doi: 10.1016/j.jff.2015.03.012
Sanders, M. E., Benson, A., Lebeer, S., Merenstein, D. J., and Klaenhammer, T. R. (2018). Shared mechanisms among probiotic taxa: implications for general probiotic claims. Curr. Opin. Biotechnol. 49, 207–216. doi: 10.1016/j.copbio.2017.09.007
Şanlier, N. Gökcen, B. B., and Sezgin, A. C. (2019). Health benefits of fermented foods. Crit. Rev. Food Sci. Nutr. 59, 506–527. doi: 10.1080/10408398.2017.1383355
Sanni, A. I., and Adesulu, A. T. (2013). Microbiological and physico-chemical changes during fermentation of maize for masa production. Afr. J. Microbiol. Res. 7, 4355–4362. doi: 10.5897/AJMR12.1362
Sanni, A. I., Morlon-Guyot, J., and Guyot, J. P. (2002). New efficient amylase-producing strains of Lactobacillus plantarum and L. fermentum isolated from different Nigerian traditional fermented foods. Int. J. Food Microbiol. 72, 53–62. doi: 10.1016/s0168-1605(01)00607-9
Sawadogo-Lingani, H., Lei, V., Diawara, B., Nielsen, D. S., Møller, P. L., Traoré, A. S., et al. (2007). The biodiversity of predominant lactic acid bacteria in dolo and pito wort for the production of sorghum beer. J. Appl. Microbiol. 103, 765–777. doi: 10.1111/j.1365-2672.2007.03306.x
Sharma, R., Garg, P., Kumar, P., Bhatia, S. K., and Kulshrestha, S. (2020). Microbial fermentation and its role in quality improvement of fermented foods. MDPI Ferm. 6:106. doi: 10.3390/fermentation6040106
Soro-Yao, A. A., Brou, K., Amani, G., Thonart, P., and Djè, K. M. (2014). The use of lactic acid bacteria starter cultures during the processing of fermented cereal-based foods in West Africa: A Review. Trop Life Sci. Res. 25, 81–100.
Soro-Yao, A. A., Brou, K., Koffi-Nevry, R., and Djè, K. M. (2013). Microbiology of Ivorian fermented products: A review. Asian J. Agric. Food Sci. 1, 37–34.
Svanberg, U., Sjögren, E., Lorri, W., Svennerholm, A. M., and Kaijser, B. (1992). Inhibited growth of common enteropathogenic bacteria in lactic-fermented cereal gruels. World J. Microbiol. Biotechnol. 8, 601–606. doi: 10.1007/BF01238797
Taylor, B. C., Lejzerowicz, F., Poirel, M., Shaffer, J. P., Jiang, L., Aksenov, A., et al. (2020). Consumption of fermented foods is associated with systematic differences in the gut microbiome and metabolome. mSystems 5, e901–e919. doi: 10.1128/mSystems.00901-19
Tsafrakidou, P., Michaelidou, A., and Biliaderis, C. G. (2020). Fermented Cereal-based Products: Nutritional Aspects, Possible Impact on Gut Microbiota and Health Implications. MDPI Foods 9:734. doi: 10.3390/foods9060734
Vieco-Saiz, N., Belguesmia, Y., Raspoet, R., Auclair, E., Gancel, F., Kempf, I., et al. (2019). Benefits and inputs from lactic acid bacteria and their bacteriocins as alternatives to antibiotic growth promoters during food-animal production. Front. Microbiol. 10:57. doi: 10.3389/fmicb.2019.00057
Vieira-Dalodé, G., Jespersen, L., Hounhouigan, D. J., Moller, P. L., Nago, C. M., and Jakobsen, M. (2007). Lactic acid bacteria and yeasts associated with gowé production from sorghum in Bénin. J. Appl. Microbiol. 103, 342–349. doi: 10.1111/j.1365-2672.2006.03252
Virdis, C., Sumby, K., Bartowsky, E., and Jiranek, V. (2021). Lactic Acid Bacteria in Wine: Technological Advances and Evaluation of Their Functional Role. Front. Microbiol. 11:612118. doi: 10.3389/fmicb.2020.612118
Walsh, A. M., Crispie, F., Claesson, M. J., and Cotter, P. D. (2017). Translating omics to food microbiology. Ann. Rev. Food Sci. Technol. 8, 113–134. doi: 10.1146/annurev-food-030216-025729
Wang, H., Wei, C., Min, L., and Zhu, L. (2018). Good or bad: gut bacteria in human health and diseases. Biotechnol. Biotechnol. Equip 32, 1075–1080. doi: 10.1080/13102818.2018.1481350
Westerik, N., Nelson, A., Wacoo, A. P., Sybesma, W., and Kort, R. (2020). A comparative interrupted times series on the health impact of probiotic yogurt consumption among school children from three to six years old in Southwest Uganda. Front. Nutr. 7:574792. doi: 10.3389/fnut.2020.574792
Yadav, H., Jain, S., Rastamanesh, R., Bomba, A., Catanzaro, R., and Marotta, F. (2011). Fermentation technology in the development of functional foods for human health: where we should head. Ferment Technol. 1:1102. doi: 10.4172/2167-7972.1000-1102
Yousif, N. M. K., Dawyndt, P., Abriouel, H., Wijaya, A., Schillinger, U., Vancanneyt, J., et al. (2005). Molecular characterization, technological properties and safety aspects of Enterococci from ‘Hussuwa,’ an African fermented sorghum product. J. Appl. Microbiol. 98, 216–228. doi: 10.1111/j.1365-2672.2004.02450
Zhang, G., Hu, H., Liu, C., Zhang, Q., Shakya, S., and Li, Z. (2016). Probiotics for Prevention of Atopy and Food Hypersensitivity in Early Childhood. A PRISMA-Compliant Systematic Review and Meta-Analysis of Randomized Controlled Trials. Medicine 95:2562. doi: 10.1097/MD.0000000000002562
Zheng, J., Wittouck, S., Salvetti, E., Franz, C. M. A. P., Harris, H. M. B., and Mattarelli, P. (2020). Taxonomic note on the genus Lactobacillus: Description of 23 novel genera, emended description of the genus Lactobacillus Beijerinck 1901, and union of Lactobacillaceae and Leuconostocaceae. Int. J. Syst. Evol. Microbiol. 70, 2782–2858. doi: 10.1099/ijsem.0.004107
Keywords: Africa, fermented foods, starter culture, food security, Lactobacillus species
Citation: Adesulu-Dahunsi AT, Dahunsi SO and Ajayeoba TA (2022) Co-occurrence of Lactobacillus Species During Fermentation of African Indigenous Foods: Impact on Food Safety and Shelf-Life Extension. Front. Microbiol. 13:684730. doi: 10.3389/fmicb.2022.684730
Received: 23 March 2021; Accepted: 31 January 2022;
Published: 07 April 2022.
Edited by:
Brian Gibson, Technical University of Berlin, GermanyReviewed by:
Koshy Philip, University of Malaya, MalaysiaCopyright © 2022 Adesulu-Dahunsi, Dahunsi and Ajayeoba. This is an open-access article distributed under the terms of the Creative Commons Attribution License (CC BY). The use, distribution or reproduction in other forums is permitted, provided the original author(s) and the copyright owner(s) are credited and that the original publication in this journal is cited, in accordance with accepted academic practice. No use, distribution or reproduction is permitted which does not comply with these terms.
*Correspondence: Adekemi Titilayo Adesulu-Dahunsi, YWRla2VtaS5kYWh1bnNpQGJvd2VuLmVkdS5uZw==
Disclaimer: All claims expressed in this article are solely those of the authors and do not necessarily represent those of their affiliated organizations, or those of the publisher, the editors and the reviewers. Any product that may be evaluated in this article or claim that may be made by its manufacturer is not guaranteed or endorsed by the publisher.
Research integrity at Frontiers
Learn more about the work of our research integrity team to safeguard the quality of each article we publish.