- Department of Food and Drug, University of Parma, Parma, Italy
Lactic Acid Bacteria (LAB) exert a fundamental activity in cheese production, as starter LAB in curd acidification, or non-starter LAB (NSLAB) during ripening, in particular in flavor formation. NSLAB originate from the farm and dairy environment, becoming natural contaminants of raw milk where they are present in very low concentrations. Afterward, throughout the different cheesemaking processes, they withstand chemical and physical stresses becoming dominant in ripened cheeses. However, despite a great body of knowledge is available in the literature about NSLAB effect on cheese ripening, the investigations regarding their presence and abundance in raw milk are still poor. With the aim to answer the initial question: “which and how many LAB are present in cow raw milk used for cheese production?,” this review has been divided in two main parts. The first one gives an overview of LAB presence in the complex microbiota of raw milk through the meta-analysis of recent taxonomic studies. In the second part, we present a collection of data about LAB quantification in raw milk by culture-dependent analysis, retrieved through a systematic review. Essentially, the revision of data obtained by plate counts on selective agar media showed an average higher concentration of coccoid LAB than lactobacilli, which was found to be consistent with meta-taxonomic analysis. The advantages of the impedometric technique applied to the quantification of LAB in raw milk were also briefly discussed with a focus on the statistical significance of the obtainable data. Furthermore, this approach was also found to be more accurate in highlighting that microorganisms other than LAB are the major component of raw milk. Nevertheless, the variability of the results observed in the studies based on the same counting methodology, highlights that different sampling methods, as well as the “history” of milk before analysis, are variables of great importance that need to be considered in raw milk analysis.
1. Introduction
Microbiological and biochemical changes in the curd are crucial factors for the production of raw-milk, ripened cheeses. The microbial ecology of this cheese variety consists of a complex interaction between starter lactic acid bacteria (SLAB, usually deliberately added for curd acidification) and non-starter LAB (NSLAB, adventitious milk contaminants from farm and dairy environments) from milking to ripening (Gatti et al., 2014; Blaya et al., 2018).
NSLAB frequently recovered from cheese are facultative heterofermentative lactobacilli (Beresford et al., 2001). Lacticaseibacillus [formerly Lactobacillus casei group, (Zheng et al., 2020)] is one of the most prevalent genera found in hard cooked, long-ripened cheeses. It includes the species Lacticaseibacillus casei, Lacticaseibacillus paracasei, and Lacticaseibacillus rhamnosus, which are of particular interest because of their proven role in cheese flavor formation during ripening (Bottari et al., 2018), as well as their potential health benefits through the consumption of fermented foods (Hill et al., 2018; De Filippis et al., 2020). Their trend from low abundance in raw milk, to dominance in ripened cheese, involves adaptation to chemical and physical stresses throughout the cheesemaking and ripening processes (i.e., heat-related, acidic, osmotic, and oxidative stresses), besides their ability to grow using energy sources other than lactose (Gatti et al., 2014). These are the physiological criteria used for the delineation of the name of the new genus Lacticaseibacillus from caseus (cheese; Zheng et al., 2020).
The mechanisms responsible for NSLAB survival to stresses encountered during cheesemaking might be attributed to various strategies of adaptation, such as the capability to utilize different energy sources (Lazzi et al., 2014; Papadimitriou et al., 2016) or the activation of strategies that can increase their tolerance to the food manufacturing process, such as the recently described toxin-antitoxin systems (Levante et al., 2019, 2021). Although NSLAB have been extensively studied for their role in cheese ripening (De Dea Lindner et al., 2008; Solieri et al., 2012; Sgarbi et al., 2013, 2014; Levante et al., 2017; Bottari et al., 2020), and reviews are available on this topic (Beresford et al., 2001; Settanni and Moschetti, 2010; Montel et al., 2014; Gobbetti et al., 2015; Bottari et al., 2018), the source of their origin and their relative abundance in raw milk are not well clarified yet.
Further research is necessary to demonstrate whether live microorganisms are present in milk inside a healthy mammary gland (Oikonomou et al., 2020), although milk endogenous bacterial contamination via enteromammary pathway has been hypothesized (Addis et al., 2016). However, it is known that microbial colonization occurs from different sources throughout the route from farm to cheese factory (Gobbetti et al., 2018) and raw milk represents an ideal environment for the growth of many microorganisms (Quigley et al., 2013). To shed light on which and how many of these biotypes will be part of the ripened cheese microbiota, it is of primary importance to know their initial abundance in raw milk, since the number of bacterial cells is known to be one of the most important factors in determining microbial activity in food (Fleet, 1999; Giraffa, 2004). It is expected that in the next future quantitative methods such as flow cytometry will help understanding how microbial loads of certain species in raw milk may affect the microbiome of resulting cheeses (Skeie et al., 2019; Porcellato et al., 2021), as it was also shown for other complex microbiomes (Vandeputte et al., 2017).
Although many studies deal with the description of the complex dairy ecosystem focusing on LAB in raw milk, often reporting isolation, identification, and relative quantification of the most abundant species, they rarely provide absolute quantification. With the aim to answer the initial question: “which and how many lactic acid bacteria are present in cow raw milk used for cheese production?,” this review analyses and comments on the data of the studies available in the literature addressing LAB quantification in raw milk used for the production of different cow’s milk cheeses.
The different analytical techniques used for LAB evaluation in milk can be divided into two methodological approaches: (I) conventional culture-dependent methods which analyze microorganisms after their growth in liquid or solid media; (II) more recent culture-independent methods which directly detect microbial nucleic acids, avoiding the culturing step. A prior careful consideration of numerous factors (e.g., taxonomic resolution, workload) is necessary to define the appropriate methodology for a certain purpose (Temmerman et al., 2004). These different approaches are complementary, thus their combination in a polyphasic approach is suggested to set up more complete and accurate studies (Ercolini et al., 2001; Delbès et al., 2007; Agrimonti et al., 2019) that better describe the dynamic evolution of microbial communities in food ecosystems, in particular in the complex transformation of raw milk to ripened cheese.
In both methods, the qualitative information (i.e., microorganisms taxonomic identification) can be addressed by common molecular analysis (such as PCR-and sequencing-based methods). On the other hand, the quantitative results differ: most of the culture-independent studies on LAB in raw milk report the relative abundance (%) of prevalent taxa; culture-dependent studies mainly based on plate counts, report instead results as Colony Forming Units (CFU/mL).
For this reason, data collected from the studies applying different approaches have been discussed separately in the following two chapters: the first one aims at giving an overview of LAB’s abundance within the complex microbiota of cow raw milk through the review of recent taxonomic studies; the second includes a collection of data about quantification of LAB by culture-dependent analysis in cow raw milk.
2. Culture-independent quantification
Culture-independent techniques available for milk and cheese microbiota analysis are constantly evolving and have been extensively reviewed elsewhere (Randazzo et al., 2009; Ndoye et al., 2011; Quigley et al., 2011; Neviani et al., 2013; Addis et al., 2016; Levante et al., 2020; Tilocca et al., 2020). Common outlines of these reviews are: (I) the usefulness of the culture-independent approach in describing complex ecosystems such as raw milk and cheese, and the ability to overcome culture-dependent limits (e.g., ability to distinguish between dead or viable cells by a combination of DNA-and RNA-based approaches), although a polyphasic approach is recommended; (II) -omics approaches are expected to greatly contribute in shedding light on microbial ecology dynamics.
Methods for absolute quantification at the species level have been implemented, such as Fluorescence in-situ Hybridization [FISH (Bottari et al., 2006)], quantitative PCR [qPCR (Agrimonti et al., 2019)], and Total Bacterial Count computed with the percentage of taxon after 16S rRNA Amplicon Sequencing (Props et al., 2017; Skeie et al., 2019; Porcellato et al., 2021). However, to the authors’ knowledge, no studies applied these methods for the absolute quantification of the Lacticaseibacillus group in cow raw milk used for cheese production, except for Masoud and colleagues who quantified Lacticaseibacillus rhamnosus in raw milk used for Danish cheese production (Masoud et al., 2012).
Different studies are instead available in the literature about the characterization of raw milk microbiota by amplicon-based high-throughput sequencing (HTS) analysis. This method is successful to describe changes in microbiota related to seasonality, geographical origin, and the microbiota evolution at different steps of cheese making, but it suffers from bias because results are usually reported as relative abundance of taxa which are not converted to quantitative values (Skeie et al., 2019), and identification of bacteria beyond the genus level is often not possible (Claesson et al., 2010; Dreier et al., 2022). On the other hand, a fundamental advantage of these studies is the possibility to use the generated raw sequences to perform meta-analysis.
A very useful tool to conduct the meta-analysis is FoodMicrobionet [FMBN (Parente et al., 2016, 2019; De Filippis et al., 2018)], a collection of datasets created by 16S rRNA gene amplicon HTS studies of food bacterial communities. This tool was already used to widely review the microbiota of dairy milk, discussing comparisons between the pasture and feed, farm environments, teat skin, teat milk (from different species, also affected by diseases), bulk tank milk and finally also HTST (high-temperature short time) milk (Parente et al., 2020).
In the present review, the updated version of FMBN 4.1.2 (Parente et al., 2022), integrated with the taxonomy reclassification for the genus Lactobacillus (Zheng et al., 2020) and further recent studies on the raw milk microbiota, has been used. Among the studies available in the database on cow raw milk, those analyzing cow whole raw milk used for cheese production were selected (De Pasquale et al., 2014; Dolci et al., 2014; Calasso et al., 2016; De Filippis et al., 2016; Giello et al., 2017; Falardeau et al., 2019; Cremonesi et al., 2020; Kamimura et al., 2020; Nikoloudaki et al., 2021; Supplementary Tables 1, 2). This dataset included reads from 250 raw milk samples, classified in 1,594 total taxa belonging to 45 different phyla (Chloroplast, Eukaryota, Mithocondria, and unidentified OTUs at the domain level were removed). Nodes and edges tables (.gml file generated by FMBN Shiny app) were then imported into Gephi software [v 0.9.2 (Bastian et al., 2009)] for bipartite network analysis, i.e., network with nodes belonging to OTUs and samples.
Nodes and edge statistics were calculated in Gephi: the degree is the number of connections to a certain node, meaning that for a sample, it represents the number of present OTUs, vice versa for an OTU, it represents the number of milk samples where it was found; the weight is a value assigned to an edge, corresponding to the OTU relative abundance in the sample; the weighted degree is the sum of weights for a node (Supplementary Table 3). Nodes and taxa label size are proportional to their weighted degree, hence the bigger they are, the more the OTU is abundant in raw milk. Only nodes with a weighted degree > 10 were considered for the network (Figure 1). ForceAtlas2 algorithm (Jacomy et al., 2014) was applied for the layout: as a consequence, taxa are closer to samples where they are present with a greater abundance (thus, taxa to the periphery are less abundant); also, taxa close to each other were present more frequently in the same samples.
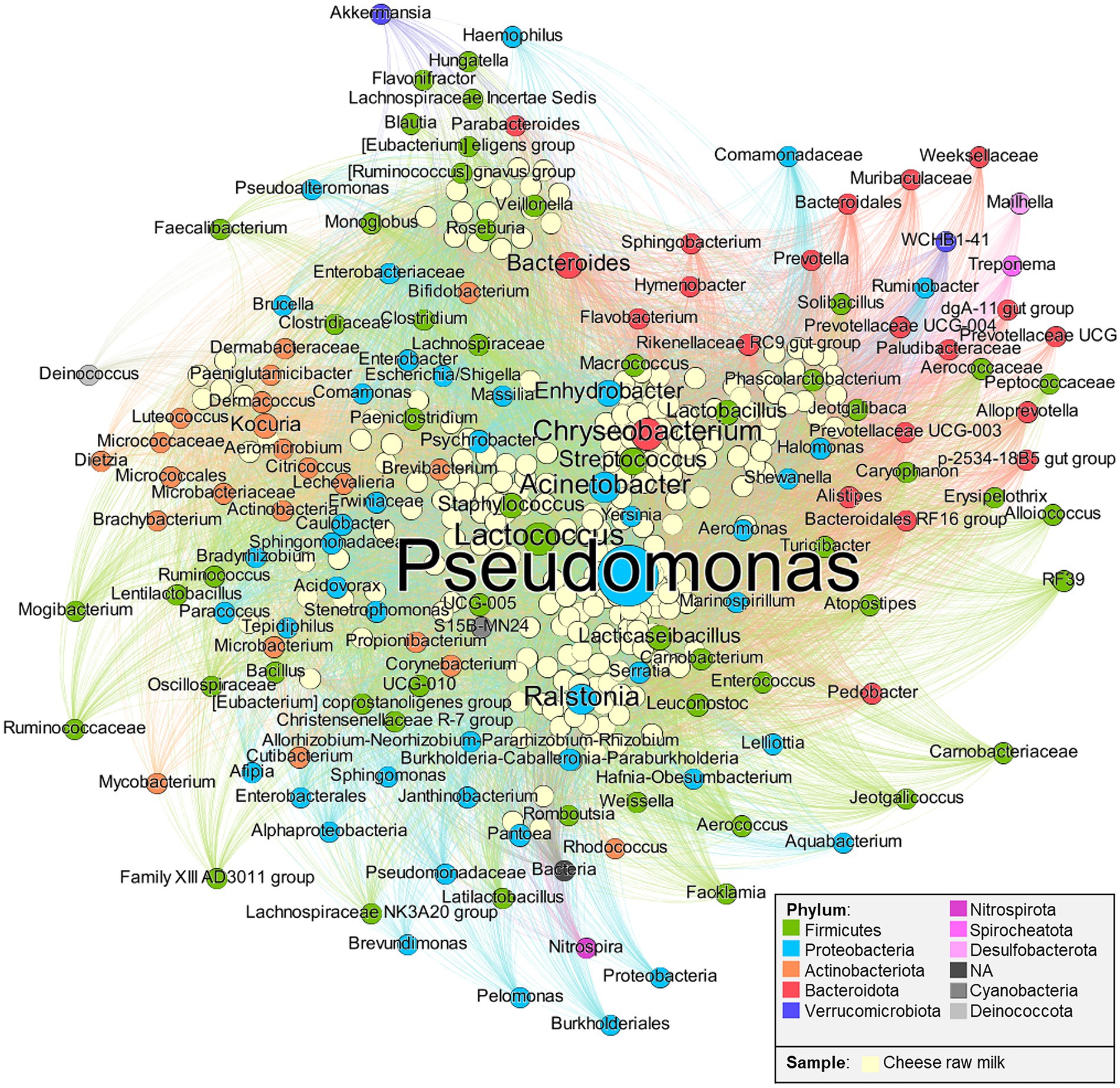
Figure 1. Bipartite network. Nodes represent cheese raw milk samples and Operational Taxonomic units (OTUs), linked to each other by edges. OTUs’ label and node size are directly proportional to their relative abundance in all the raw milk samples. Data were extracted from studies listed in Supplementary Table 2.
After the filtering step, 149 taxa were retained (Supplementary Table 3), which belong to 10 different phyla. Part of the OTUs were classified only at the kingdom level (Bacteria, NA dark gray node). Among the most abundant genera, the psychrotrophic bacteria are present in significant proportions: these include Pseudomonas (23.85% of the tot weighted degree in the network), Chryseobacterium (6.56%), and Acinetobacter (6.01%). It is reasonable to assume that their dominance could be due to the refrigerating temperature usually applied for raw milk storage which decreases bacterial diversity (Raats et al., 2011).
According to these data, LAB relevant for cheese production are present as dominant taxa such as Lactococcus (7.24%), or subdominant such as Streptococcus (3.6%), Lacticaseibacillus (2.42%), Lactobacillus (2.35%), Leuconostoc (1.15%), and Enterococcus (0.41%). Despite their relatively low abundance in the raw milk ecosystem, these subdominant taxa can develop in subsequent cheese manufacturing. An example is found in long ripened cheeses, where the Lacticaseibacillus genus is known to become dominant throughout the ripening of raw milk, hard cooked, long-ripened cheeses (Bottari et al., 2018).
The low abundance of some subdominant species makes their isolation from raw milk difficult, limiting thus a potential targeted use. A strategy to overcome this limit could be the enrichment of the autochthonous raw milk microbiota through its spontaneous fermentation. Following this approach, Bancalari and colleagues carried out a spontaneous fermentation of Parmigiano Reggiano raw milk samples to isolate LAB strains potentially usable as adjunctive aromatic starters (Bancalari et al., 2017), while Galli and colleagues incubated the raw milk for 24 and 48 h, at 30°C and 40°C, with the purpose to isolate additional LAB with potentially high GABA (γ-aminobutyric acid) producing capabilities (Galli et al., 2022).
3. Culture-dependent quantification
3.1. A systematic review of plate counts on agar media of LAB in raw milk
3.1.1. Search strategy
Articles were identified by searching in Scopus1 onthe10th of October 2022, using the following key words: TITLE-ABS-KEY ((({raw milk}) OR ({raw-milk}) OR ({bulk milk}) OR ({tank milk}) OR ({vat milk}) OR ({milk microbiota}) OR ({milk microflora}) OR ({Raw cows’ milk}) OR ({Raw milk/cheese})) AND (({lactic acid bacteria}) OR (lab) OR (lactobacilli) OR (lactobacillus) OR (lacticaseibacillus) OR (nslab)) AND (cheese) AND NOT (({human milk}) OR (camel) OR (goat) OR (ewe) OR (sheep) OR (deer) OR (donkey))). After a titles/abstract screening, articles were included in the review on the base of the following criteria about the milk analyzed: (1) it must be raw [as defined by (EC, 2004)]; (2) it must derive from cow; (3) it must be used for cheese production; (4) it must be analyzed for LAB Colony Forming Unit. In the Figure 2, the four-phase PRISMA flow diagram (Page et al., 2021) schematizing the studies search process is reported.
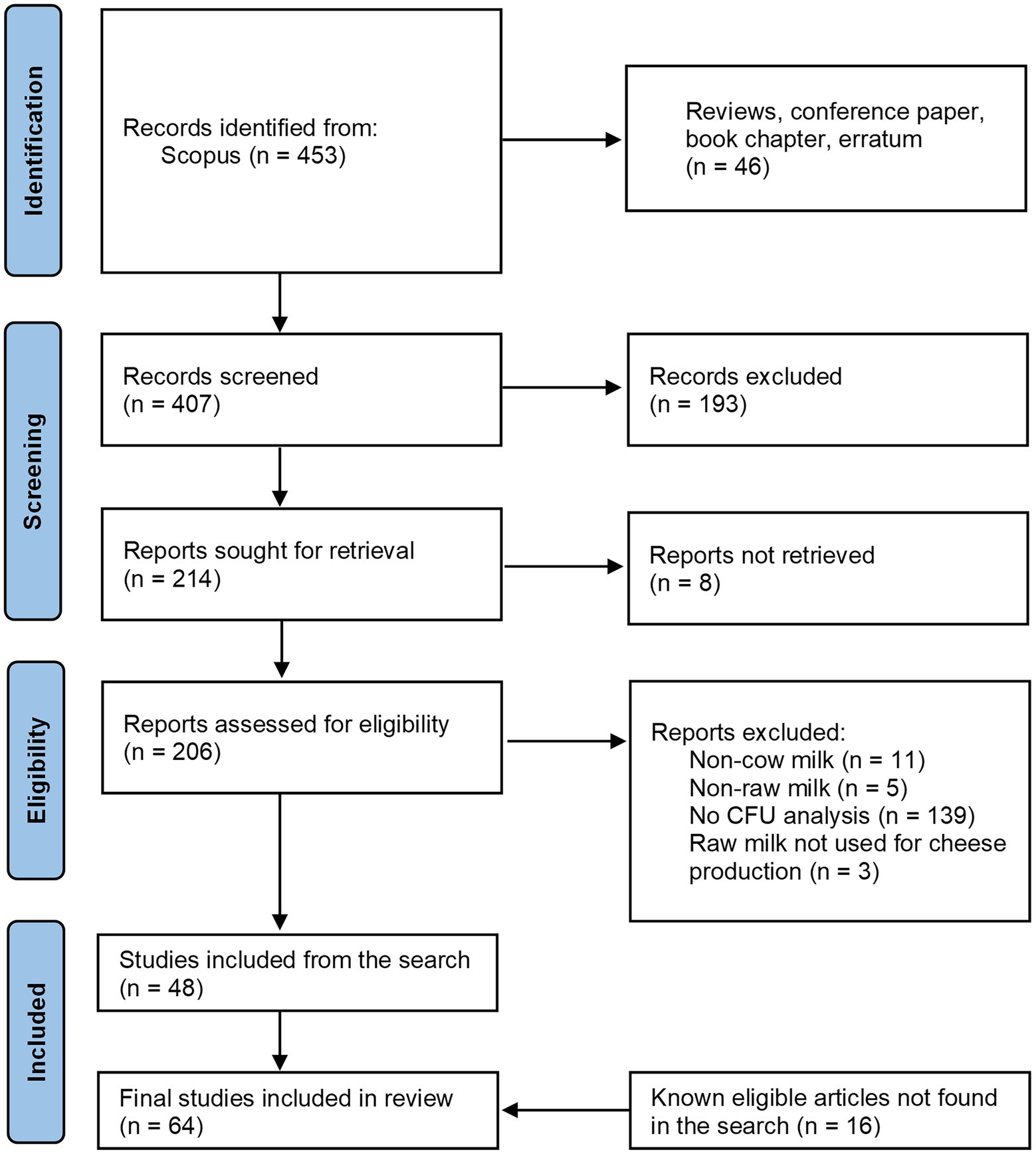
Figure 2. Flow diagram of the studies search for the systematic review of plate counts on agar media of Lactic Acid Bacteria in raw milk. CFU, Colony forming unit.
3.1.2. Data elaboration
According to the definition of raw milk (EC, 2004), we considered only data from the analysis of milk samples which were not heat treated or undergone any treatment that has an equivalent effect. LAB quantification data (CFU/mL) evaluated by plate counts on elective or selective agar media after incubation at different temperatures were extracted and transformed in log10 scale when necessary. Quantification in broth media using the Most Probable Number (MPN) was rarely used (Mucchetti et al., 2009; Cremonesi et al., 2020). Results of standard total bacterial count through Plate Count Agar (PCA; EC, 2006; ISO 4833-2, 2013) have been also extracted when available. Data were grouped based on the LAB group analyzed, i.e., by growth media and incubation temperature used. Only the two largest, more robust datasets underwent a statistical analysis for the evaluation of a significant difference among the two LAB groups: (I) Lactobacilli, cultivated on Man-Rogosa-Sharpe (MRS) at 30–32°C (n = 82); (II) Coccoid LAB, cultivated on M17 at 30–32°C (n = 70). The two datasets (Supplementary Data 1) were checked for their normal distribution through the Shapiro–Wilk test; the homogeneity of variance was assessed with a F-test; finally, a t-test (α = 0.05) was applied. The data elaboration and statistical analysis were done in the R environment (R Core Team, 2022) using the packages “stats” and “tidyverse” (Wickham et al., 2019).
3.1.3. Lactic acid bacteria concentration in raw milk for cheese production
Out of the 453 records retrieved from the systematic search (Figure 2), the first country in terms of publication number in this field is Italy (n = 102), followed by France (57), and Spain (48) (Scopus stats, data not shown). Together with Ireland, Switzerland, and Portugal, they represent the 59% of the articles retrieved by this search. This is evidence of the high research interest of the European Institutions in the dairy sector, reflecting the historic tradition of cheesemaking of the continent (Montel et al., 2014). The rest of the records were from Brazil, Turkey, Mexico and the United States of America.
Raw milk cheeses are produced worldwide. However, different cow raw milk cheeses analyzed in the studies retrieved through the literature search, were excluded from this review because the authors did not evaluated the LAB quantification in the raw milk by plate counts. Some examples are: Cotija [Mexico (Escobar-Zepeda et al., 2016)], Tulum [Turkey (Gezginc et al., 2022)], Fontina [Italy (Giannino et al., 2009)], Kraški and Tolminc [Slovenia (Trmčić et al., 2011)], Zlatar [Serbia (Terzic-Vidojevic et al., 2007)], Sir iz Mišine [Croatia (Vrdoljak et al., 2022)], Bitto [Italy (Colombo et al., 2009)], São Jorge [Portugal (Kongo et al., 2009)], and Poro de Tabasco [Mexico (De la Rosa-Alcaraz et al., 2020)].
Table 1 reports the list of the 64 studies available in the literature that quantified LAB in cow raw milk used for the production of 42 types of cheese. Mean values of log CFU/mL have been grouped by media type and incubation temperature to define the core LAB raw milk microbiota (Figure 3). Thus, the observed variability is likely explained by the fact that other factors take part in the modulation of LAB load in raw milk, such as sampling procedures, milk pre-treatments (e.g., skimming), and seasonality. Furthermore, although the studies considered eligible in this review have in common the use of the same growth media and incubation temperature, they may have slight differences in the analysis procedure, such as plate incubation time, degree of aerobic/anaerobic conditions, use of selective additives (Supplementary Data 2).
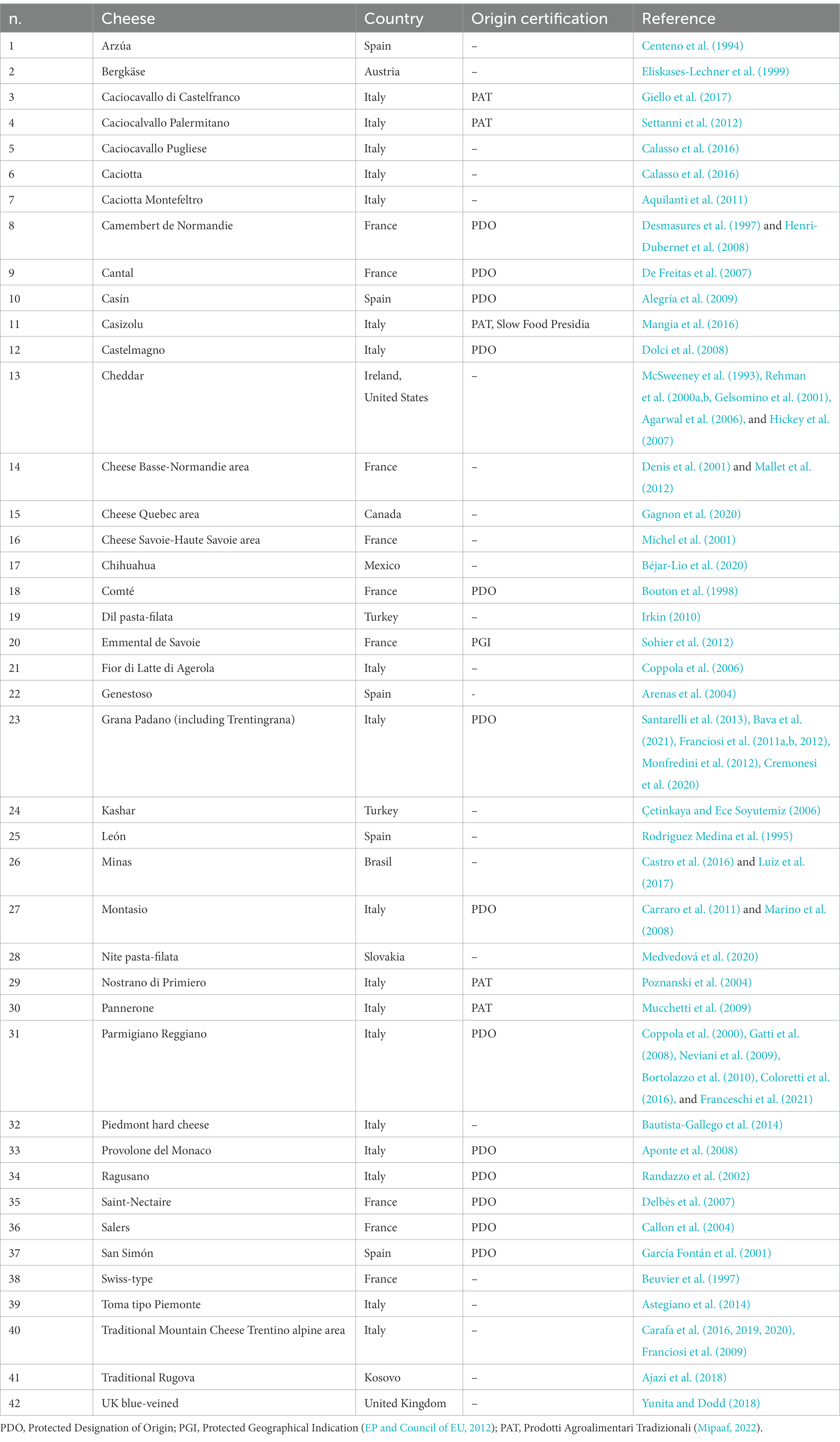
Table 1. List of cheeses whose raw milk was analyzed for LAB concentration through culture-dependent techniques.
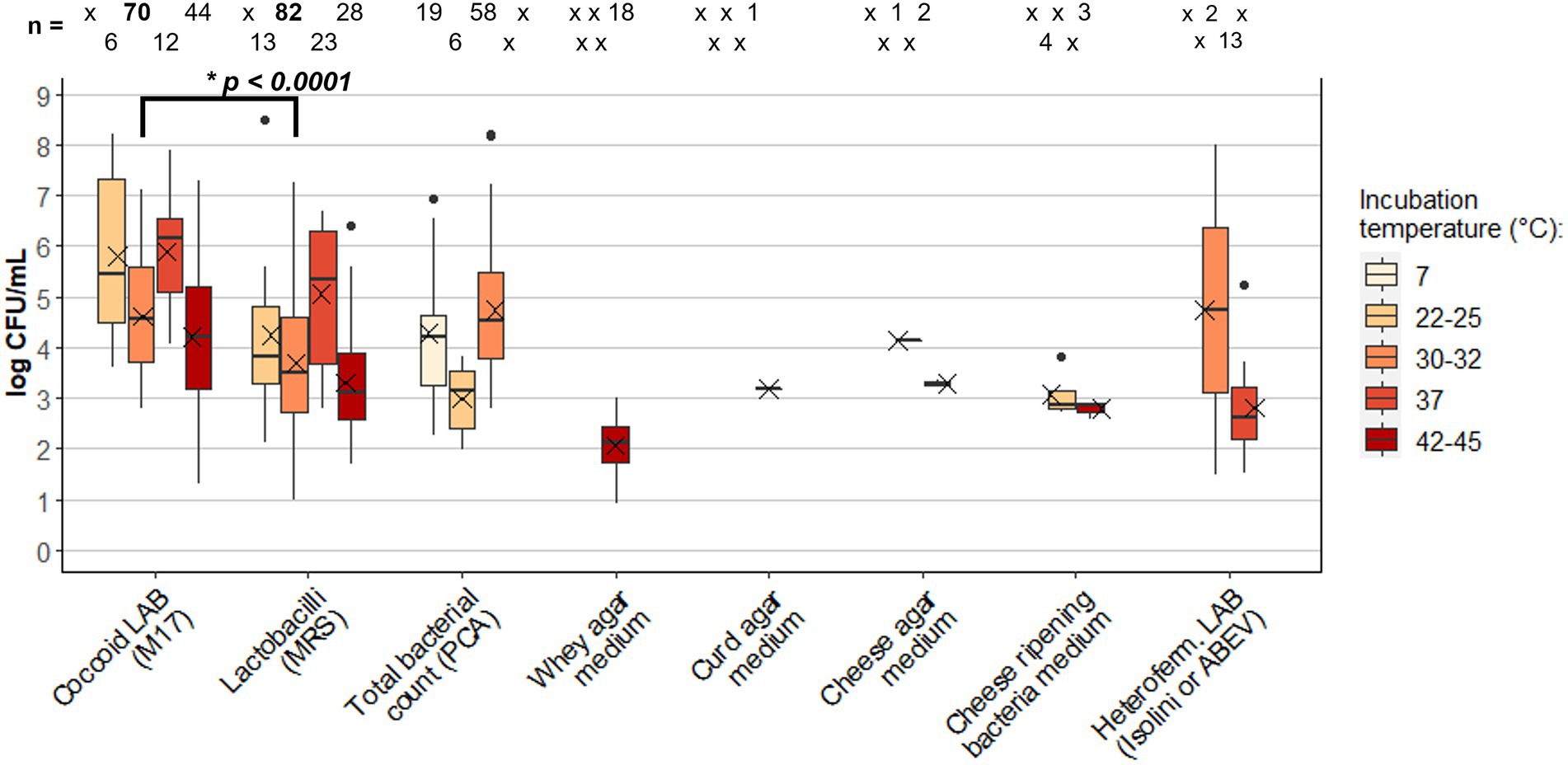
Figure 3. Box plot of Lactic Acid Bacteria (LAB) concentration (log CFU/mL) in cheese raw milk: comparison between different growth media and incubation temperatures. Extremes of the bars represent the max. and min. Values; the box lines indicate the 1st quartile, the median, and the 3rd quartile; the mean is indicated by the X within the box; isolated data points are outliers. The number of data (n) is reported at the top of each box (x = missing data). Data were extracted from studies listed in Table 1. The value of p (*p) resulting from the t-test applied to Coccoid LAB and Lactobacilli results incubated at 30–32°C is reported (datasets are reported in Supplementary Data 1). MRS, Man-Rogosa-Sharpe; PCA, plate count agar; ABEV, Arginine Bromocresol Meat Extract Vancomycin.
The total number of values found in literature about LAB quantification in raw milk used for Grana Padano cheese is 103, the highest among the values found for the other cheeses addressed in this review. This indicates a high research interest in this cheese type. The raw milk used for Grana Padano PDO (Protected designation of origin) cheese (including Trentingrana) was analyzed by sampling whole evening raw milk and skimmed morning raw milk, thus testing the effect of overnight creaming (Franciosi et al., 2011a; Santarelli et al., 2013). With a similar sampling procedure, also the microbial composition of morning whole raw milk and vat raw milk was evaluated (Monfredini et al., 2012), investigating also different storage temperatures (Franciosi et al., 2011b, 2012). More recently, the effect of the use of different machine cleaning detergents on the raw milk microbiota was investigated by sampling bulk tank raw milk at the farm and skimmed raw milk (Cremonesi et al., 2020), whereas Bava and colleagues analyzed bulk tank raw milk sampled at farms which had different management practices (Bava et al., 2021). In total, six studies quantified the LAB in raw milk used for Parmigiano Reggiano (Table 1), which allowed the collection of 28 values, the highest data number after Grana Padano and Traditional Mountain Cheese from Trentino alpine area (103 data in seven studies and 70 data in four studies, respectively). Raw milk used for Parmigiano Reggiano PDO is also skimmed by overnight creaming, and different studies analyzed evening whole and morning skimmed raw milk (CRPA, 2011; Coloretti et al., 2016). Franceschi and colleagues sampled bulk raw milk from tanks directly at the farm after storage at two different temperatures (Franceschi et al., 2021). Other studies analyzed vat raw milk, obtained by mixing whole evening raw milk and morning skimmed raw milk (Coppola et al., 2000; Gatti et al., 2008; Neviani et al., 2009; CRPA, 2011).
The vat raw milk has also been studied in several other types of cheese: Provolone del Monaco (Aponte et al., 2008), Castelmagno (Dolci et al., 2008), Saint-Nectaire (Delbès et al., 2007), Swiss-type (Beuvier et al., 1997), Traditional Mountain cheese (Carafa et al., 2016, 2019), Cheddar (Gelsomino et al., 2001), Arzúa (Centeno et al., 1994), Cantal (De Freitas et al., 2007) and Fior di Latte di Agerola (Coppola et al., 2006). In the case of Caciotta, Caciocavallo Pugliese, and Emmental de Savoie the raw milk was sampled from tanks at the cheese factory (Sohier et al., 2012; Calasso et al., 2016), while for other cheeses directly at the farm, sometimes evaluating the effect of seasonality (Desmasures et al., 1997; Arenas et al., 2004; Franciosi et al., 2009; Mallet et al., 2012; Castro et al., 2016; Luiz et al., 2017), breeding practices (Giello et al., 2017; Gagnon et al., 2020), or transhumance period (Carafa et al., 2020).
Many studies, instead, did not report detailed information about the raw milk samples analyzed (McSweeney et al., 1993; Rodriguez Medina et al., 1995; Rehman et al., 2000a,b; Denis et al., 2001; García Fontán et al., 2001; Randazzo et al., 2002; Agarwal et al., 2006; Çetinkaya and Ece Soyutemiz, 2006; Aquilanti et al., 2011; Carraro et al., 2011; Yunita and Dodd, 2018).
The main growth media used in the 64 reviewed studies were de Man-Rogosa-Sharpe (MRS; De Man et al., 1960) elective for lactobacilli (55 studies), M17 (Terzaghi and Sandine, 1975) elective for coccoid LAB (40 studies), and PCA (31 studies) for total bacterial count. The results of 5 growth temperature ranges are shown in Figure 3: 7°C for psychrotrophs; 22–25, 30–32 and 37°C for mesophiles; 42–45°C for thermophiles.
PCA medium was modified for coccoid LAB count (PCA-BCP, Supplementary Data 2), to analyze raw milk used to produce Camembert de Normandie [2.91 log CFU/mL raw milk in winter period; 2.84 log CFU/mL in spring/summer period (Desmasures et al., 1997)] and cheese of Basse Normandie area [1.91 log CFU/mL raw milk in winter period; 1.87 log CFU/mL in spring period (Mallet et al., 2012)]; for this reason, these values were not included in Figure 3. Since the average of total bacterial counts at 30–32°C (4.74 ± 1.26 log CFU/mL) is similar in comparison with LAB counts, especially for M17 counts, this may indicate that LAB represent the majority of mesophiles among culturable bacteria in raw milk. The slightly higher average concentration of total psychrotrophs in comparison with total mesophiles at 22–25°C is likely caused by the low number of data found in the literature for this temperature range (n = 19 and n = 6, respectively), in addition to the high values of 6.53 and 6.93 log CFU/mL found, respectively, in the raw milk used for the Spanish cheeses Leòn (Rodriguez Medina et al., 1995) and Genestoso (Arenas et al., 2004; the latest was indeed considered an outlier).
Focusing on the LAB counts, cocci were found in very high concentrations at 37°C (5.88 log CFU/mL on average). However, this value is influenced by the high counts of raw milk used to produce artisanal cheeses from Sicily [7.9 log CFU/mL (Randazzo et al., 2002)], from Brasil [6.53 and 6.86 log CFU/mL in raw milk sampled in dry and rainy season, respectively, (Castro et al., 2016)], and from Turkey [6.64 log CFU/mL (Çetinkaya and Ece Soyutemiz, 2006)]. The raw milk used for artisanal cheese Caciotta di Montefeltro (Aquilanti et al., 2011) was also found to have a particular high value of mesophiles (22–25°C) coccoid LAB of 7.61 log CFU/mL. The same artisanal productions, together with raw milk used for artisanal Rugova cheese from Kosovo (Ajazi et al., 2018), Kashar from Turkey (Çetinkaya and Ece Soyutemiz, 2006), and Minas from Brazil (Castro et al., 2016), showed the highest count of lactobacilli at the same incubation temperature of 37°C, expanding the value range of LAB found in MRS (1st quartile = 3.69 log CFU/mL; 3rd quartile = 6.29 log CFU/mL), which had an average of 5.05 log CFU/mL (Figure 3). The concentration of lactobacilli able to grow at this temperature in raw milk used for Cheddar cheese was found to be 2.28 log CFU/mL (McSweeney et al., 1993), even if the authors performed the cultivation on a different growth medium named Lactobacillus Selection Agar (LBS, Baltimore Biological Laboratories, Rockville, MD, United States; Becton Dickinson Microbiology Systems, United States). The same medium incubated at 30°C resulted in a lactobacilli concentration of 1.94 log CFU/mL in the raw milk used for the same cheese type (Gelsomino et al., 2001; Hickey et al., 2007).
More common and thus more useful for comparison was the incubation of MRS and M17 at 30–32°C, performed in 33 (n = 82) and 26 (n = 70) studies respectively, and representing the raw milk used for 24 of the 42 types of cheese analyzed in the review. The average concentration of lactobacilli (3.69 ± 1.21 log CFU/mL) was found significantly lower (p < 0.001) than coccoid LAB (4.62 ± 1.07 log CFU/mL). This result is consistent with what we found in the meta-analysis of HTS studies on the raw milk microbiota, where Lactococcus and Streptococcus genera were present in greater abundance compared to Lacticaseibacillus and Lactobacillus genera.
At the same growth temperature, total acidifying mesophiles had similar counts in raw milk used for cheese in Savoie-Haute Savoie using Elliker medium (Michel et al., 2001). Few works instead quantified coccoid LAB at 22–25°C, thus the higher concentration found in comparison with counts at 30–32°C may not be relevant.
The thermophiles population was counted in raw milk used for Swiss-type (Beuvier et al., 1997), Fior di Latte di Agerola (Coppola et al., 2006), Caciotta di Montefeltro (Aquilanti et al., 2011), Piedmont hard cheese (Bautista-Gallego et al., 2014), Caciocavallo di Castelfranco (Giello et al., 2017), Caciocavallo Palermitano (Settanni et al., 2012), and PDO cheeses such as Saint-Nectair (Delbès et al., 2007), Montasio (Marino et al., 2008; Carraro et al., 2011), Provolone del Monaco (Aponte et al., 2008), Salers (Callon et al., 2004), Comté (Bouton et al., 1998) and Parmigiano Reggiano (Coppola et al., 2000; Gatti et al., 2008). Thermophilic streptococci were particularly high in raw milk used for Fior di Latte di Agerola (6.7 log CFU/mL on average), causing a wider data distribution in comparison with thermophilic lactobacilli, searched in the same cheeses, except for Saint-Nectaire, which accounted an average of 3.06 log CFU/mL.
With the intention to discriminate LAB present in raw milk that better adapt to the biochemical transformation of the substrate during cheesemaking, some authors have developed specific media: Whey Agar Medium (WAM; Gatti et al., 2003), Curd Agar Medium (CURDAM; Lazzi et al., 2007), Cheese Agar Medium (CAM; Neviani et al., 2009); Heterofermentative Isolini Agar (Isolini et al., 1990) and Arginine Bromocresol Meat Extract Vancomycin (ABEV; Sohier et al., 2012) where used instead to discriminate facultative and obligate heterofermentative lactobacilli, which are known to differently adapt and grow in cheese during ripening; finally, Cheese Ripening Bacteria Media (CRBM; Denis et al., 2001) was proposed to selectively count LAB growing on the cheese surface. Low concentration of thermophiles was found in raw milk used for Parmigiano Reggiano (Gatti et al., 2008; Neviani et al., 2009) and Grana Padano (Franciosi et al., 2011b, 2012; Monfredini et al., 2012) when cultivated on WAM. The raw milk used for the same cheeses showed a slightly higher concentration of LAB able to grow in curd and cheese media (Gatti et al., 2008; Neviani et al., 2009; Santarelli et al., 2013). Heterofermentative lactobacilli were mainly analyzed at 37°C using Isolini agar and ABEV, resulting in a mean concentration of 2.82 ± 0.98 log CFU/mL. Although these specific media were used by few studies for the quantification of LAB in raw milk, they have the potential to be applied for the selective recovery of subdominant LAB species often difficult to isolate (Neviani et al., 2009).
3.2. Other culture-dependent methods
An alternative method that enables the quantification of LAB in milk, based on their duplicative capacity, is the impedometric analysis. Similarly to plate count, this is a culture-dependent method, but differently, it is not based on the quantification of formed colony but on the detection and estimation of LAB metabolites during their growth.
Impedometric analysis measures the resistance that an alternating current finds when passing through a conductive culture medium, where microorganisms are developing (Yang and Bashir, 2008; Bancalari et al., 2016). When this method is applied to the study of LAB growth in milk, the system can quantify the presence of ions resulting from the degradation of lactose into lactic acid, between two electrodes (Lanzanova et al., 1993; Mucchetti et al., 1994).
Using an instrument such as the BacTrac 4300®, two impedance components can be revealed and measured over time. The first component is the overall relative change in conductivity compared to an initially recorded value (M%) and the second is the variation of the ionic double layer near the surface of the two electrodes (E%; Futschik and Pfutzner, 1995; Bancalari et al., 2019). Both measures are recorded every 10 min, and incubation time and temperature can be set depending on the chosen conditions.
By plotting these measures against time, conductimetric or impedometric curves can be obtained. If these curves are then fitted with the Gompertz equation, three different parameters can be obtained: Lag, Rate and Yend (Bancalari et al., 2016). Particularly, the first parameter, Lag, is measured in hours and is defined as the time that the LAB cells need to adapt to the analysis conditions, before starting to duplicate. This value is inversely correlated with the amount of LAB cells: the smaller the time, the higher the number of LAB cells (Bancalari et al., 2016).
However, even if both impedance analysis and plate counts depend on the physiological state of the cells and their concentration, the Lag value cannot be converted into CFU. In fact, the Lag value is a more complex concept that depends on several factors such as the LAB genus, the species, biotype and, definitely, the physiological state of LAB cells at the beginning of the analysis. As compared to plate count, this method allows to collect more information about the behaviors of LAB strains, and even small differences can be highlighted within the same species (Bancalari et al., 2016).
Taking into consideration all these aspects, the impedometric method was recently used to quantify the mesophilic LAB in raw milk used to produce Grana Padano by measuring M% every 10 min for 48 consecutive hours at 25°C (D’Incecco et al., 2020). The obtained results allow underlining differences between samples in Lag values, ranging from 15 to 20 h, that were attributed to the different initial concentrations of LAB cells (D’Incecco et al., 2020).
One of the most evident advantages of this technique applied to the analysis of raw milk is the possibility of increasing the throughput, by analyzing many samples and variables at the same time and easily having more than two repetitions of each sample, which makes subsequent statistical data analysis and interpretation more reliable, especially if compared to plate counts.
4. Conclusion and perspectives
The route of some LAB bearing positive features for cheese production, begins when they reach the raw milk. With the intention of defining which and how many LAB are present in cow raw milk used for cheese production, this review compared and discussed the results of the available studies that addressed this topic through culture-dependent and-independent methods.
The critical review of the literature highlighted an inconsistency in the definition of “raw milk,” a term that can be referred to milk sampled at the farm rather than from the cheese vat after skimming. Together with the sampling procedures, other factors such as different farming systems, milk pre-treatments, and seasonality, take part in the modulation of the raw milk microbiota.
The meta-analysis conducted with the use of FMBN was very effective to highlight the complexity of the microbiota of cow raw milk used for cheese production which is composed of over 45 phyla and showed how the genera belonging to LAB are not the most abundant in this microbial ecosystem. It also indicated how LAB belonging to Lactobacillus and Lacticaseibacillus genera are present in lower relative abundance in comparison with Lactococcus and Streptococcus.
This result is consistent when compared with LAB concentration evaluated by means of plate counts on agar media: lactobacilli were in fact found on average in lower concentration than coccoid LAB in the raw milk used for the production of 24 different cheese types.
This allows once more to conclude that both culture-dependent and-independent techniques complementarily describe the raw milk microbiota, and the combination of the two approaches restores a complete picture of which and how many LAB are present in the raw milk.
A better knowledge of both the amount and the species of LAB present in the raw milk could be useful in the perspective of monitoring and maintaining the biodiversity of the microbiome of this complex substrate through the managing of parameters at the farm and cheese making level and finally predict the expected outcomes in the resulting raw milk cheeses.
Author contributions
LB and MG conceptualized the study. LB performed the investigation, data curation and visualization, and wrote the original draft. MG supervised the study. AL and MG contributed to the methodology of data elaboration and acquired the fundings. EB and MG cured the Section 3.2 of the manuscript. BB critically revised the paper. All authors contributed to manuscript revision, read, and approved the submitted version.
Funding
This research has financially been supported by the Programme “FIL-Quota Incentivante” of University of Parma and co-sponsored by Fondazione Cariparma.
Acknowledgments
The authors would like to thank Giulia Mariotti for the help in the investigation.
Conflict of interest
The authors declare that the research was conducted in the absence of any commercial or financial relationships that could be construed as a potential conflict of interest.
Publisher’s note
All claims expressed in this article are solely those of the authors and do not necessarily represent those of their affiliated organizations, or those of the publisher, the editors and the reviewers. Any product that may be evaluated in this article, or claim that may be made by its manufacturer, is not guaranteed or endorsed by the publisher.
Supplementary material
The Supplementary material for this article can be found online at: https://www.frontiersin.org/articless/10.3389/fmicb.2022.1092224/full#supplementary-material.
Footnotes
References
Addis, M. F., Tanca, A., Uzzau, S., Oikonomou, G., Bicalho, R. C., and Moroni, P. (2016). The bovine milk microbiota: insights and perspectives from-omics studies. Mol. BioSyst. 12, 2359–2372. doi: 10.1039/c6mb00217j
Agarwal, S., Sharma, K., Swanson, B. G., Yüksel, G. Ü., and Clark, S. (2006). Nonstarter lactic acid bacteria biofilms and calcium lactate crystals in cheddar cheese. J. Dairy Sci. 89, 1452–1466. doi: 10.3168/jds.S0022-0302(06)72213-5
Agrimonti, C., Bottari, B., Sardaro, M. L. S., and Marmiroli, N. (2019). Application of real-time PCR (qPCR) for characterization of microbial populations and type of milk in dairy food products. Crit. Rev. Food Sci. Nutr. 59, 423–442. doi: 10.1080/10408398.2017.1375893
Ajazi, F. C., Kurteshi, K., Ehrmann, M. A., Gecaj, R., Ismajli, M., Berisha, B., et al. (2018). Microbiological study of traditional cheese produced in Rugova region of Kosovo. Bulgarian J. Agr. Sci. 24, 321–325.
Alegría, Á., Álvarez-Martín, P., Sacristán, N., Fernández, E., Delgado, S., and Mayo, B. (2009). Diversity and evolution of the microbial populations during manufacture and ripening of Casín, a traditional Spanish, starter-free cheese made from cow’s milk. Int. J. Food Microbiol. 136, 44–51. doi: 10.1016/j.ijfoodmicro.2009.09.023
Aponte, M., Fusco, V., Andolfi, R., and Coppola, S. (2008). Lactic acid bacteria occurring during manufacture and ripening of provolone del Monaco cheese: detection by different analytical approaches. Int. Dairy J. 18, 403–413. doi: 10.1016/j.idairyj.2007.10.011
Aquilanti, L., Babini, V., Santarelli, S., Osimani, A., Petruzzelli, A., and Clementi, F. (2011). Bacterial dynamics in a raw cow’s milk Caciotta cheese manufactured with aqueous extract of Cynara cardunculus dried flowers. Lett. Appl. Microbiol. 52, 651–659. doi: 10.1111/j.1472-765X.2011.03053.x
Arenas, R., González, L., Bernardo, A., Fresno, J. M., and Tornadijo, M. E. (2004). Microbiological and physico-chemical changes in Genestoso cheese, a Spanish acid curd variety, throughout ripening. Food Control 15, 271–279. doi: 10.1016/S0956-7135(03)00067-7
Astegiano, S., Bellio, A., Adriano, D., Bianchi, D. M., Gallina, S., Gorlier, A., et al. (2014). Evaluation of hygiene and safety criteria in the production of a traditional piedmont cheese. Ital. J. Food Saf. 3, 5–8. doi: 10.4081/ijfs.2014.1705
Bancalari, E., Bernini, V., Bottari, B., Neviani, E., and Gatti, M. (2016). Application of impedance microbiology for evaluating potential acidifying performances of starter lactic acid bacteria to employ in milk transformation. Front. Microbiol. 7, 1–11. doi: 10.3389/fmicb.2016.01628
Bancalari, E., D’Incecco, P., Savo Sardaro, M. L., Neviani, E., Pellegrino, L., and Gatti, M. (2019). Impedance microbiology to speed up the screening of lactic acid bacteria exopolysaccharide production. Int. J. Food Microbiol. 306:108268. doi: 10.1016/j.ijfoodmicro.2019.108268
Bancalari, E., Savo Sardaro, M. L., Levante, A., Marseglia, A., Caligiani, A., Lazzi, C., et al. (2017). An integrated strategy to discover lactobacillus casei group strains for their potential use as aromatic starters. Food Res. Int. 100, 682–690. doi: 10.1016/j.foodres.2017.07.066
Bastian, M., Heymann, S., and Jacomy, M. (2009). Gephi: an open source software for exploring and manipulating networks. Proc. Int. AAAI Conf. Web Social Media 3, 361–362. doi: 10.1609/icwsm.v3i1.13937
Bautista-Gallego, J., Alessandria, V., Fontana, M., Bisotti, S., Taricco, S., Dolci, P., et al. (2014). Diversity and functional characterization of Lactobacillus spp. isolated throughout the ripening of a hard cheese. Int. J. Food Microbiol. 181, 60–66. doi: 10.1016/j.ijfoodmicro.2014.04.020
Bava, L., Zucali, M., Tamburini, A., Morandi, S., and Brasca, M. (2021). Effect of different farming practices on lactic acid bacteria content in cow milk. Animals 11:522. doi: 10.3390/ani11020522
Béjar-Lio, G. I., Gutiérrez-Méndez, N., and Nevárez-Moorillón, G. V. (2020). Microbiological and physicochemical characteristics of Chihuahua cheese manufactured with raw milk. AIMS Agric. Food 5, 86–101. doi: 10.3934/AGRFOOD.2020.1.86
Beresford, T. P., Fitzsimons, N. A., Brennan, N. L., and Cogan, T. M. (2001). Recent advances in cheese microbiology. Int. Dairy J. 11, 259–274. doi: 10.1016/S0958-6946(01)00056-5
Beuvier, E., Berthaud, K., Cegarra, S., Dasen, A., Pochet, S., Buchin, S., et al. (1997). Ripening and quality of Swiss-type cheese made from raw, pasteurized or microfiltered milk. Int. Dairy J. 7, 311–323. doi: 10.1016/S0958-6946(97)00015-0
Blaya, J., Barzideh, Z., and LaPointe, G. (2018). Symposium review: interaction of starter cultures and nonstarter lactic acid bacteria in the cheese environment. J. Dairy Sci. 101, 3611–3629. doi: 10.3168/jds.2017-13345
Bortolazzo, E., Coloretti, F., Garavaldi, A., Nocetti, M., and Vecchia, P. (2010). Influenza Dei BATTERI LATTICI MESOFILI SULLE PROPRIETÀ SENSORIALI del FORMAGGIO PARMIGIANO REGGIANO. Scienza e tecnica lattiero-casearia 66, 5–9.
Bottari, B., Ercolini, D., Gatti, M., and Neviani, E. (2006). Application of FISH technology for microbiological analysis: current state and prospects. Appl. Microbiol. Biotechnol. 73, 485–494. doi: 10.1007/s00253-006-0615-z
Bottari, B., Levante, A., Bancalari, E., Sforza, S., Bottesini, C., Prandi, B., et al. (2020). The interrelationship between microbiota and peptides during ripening as a driver for Parmigiano Reggiano cheese quality. Front. Microbiol. 11, 1–14. doi: 10.3389/fmicb.2020.581658
Bottari, B., Levante, A., Neviani, E., and Gatti, M. (2018). How the fewest become the greatest. L. casei’s Impacton long ripened cheeses. Front. Microbiol. 9, 2014–2019. doi: 10.3389/fmicb.2018.02866
Bouton, Y., Guyot, P., and Grappin, R. (1998). Preliminary characterization of microflora of Comte cheese. J. Appl. Microbiol. 85, 123–131. doi: 10.1046/j.1365-2672.1998.00476.x
Calasso, M., Ercolini, D., Mancini, L., Stellato, G., Minervini, F., Di Cagno, R., et al. (2016). Relationships among house, rind and core microbiotas during manufacture of traditional Italian cheeses at the same dairy plant. Food Microbiol. 54, 115–126. doi: 10.1016/j.fm.2015.10.008
Callon, C., Millet, L., and Montel, M. C. (2004). Diversity of lactic acid bacteria isolated from AOC Salers cheese. J. Dairy Res. 71, 231–244. doi: 10.1017/S0022029904000159
Carafa, I., Clementi, F., Tuohy, K., and Franciosi, E. (2016). Microbial evolution of traditional mountain cheese and characterization of early fermentation cocci for selection of autochtonous dairy starter strains. Food Microbiol. 53, 94–103. doi: 10.1016/j.fm.2015.09.001
Carafa, I., Navarro, I. C., Bittante, G., Tagliapietra, F., Gallo, L., Tuohy, K., et al. (2020). Shift in the cow milk microbiota during alpine pasture as analyzed by culture dependent and high-throughput sequencing techniques. Food Microbiol. 91:103504. doi: 10.1016/j.fm.2020.103504
Carafa, I., Stocco, G., Franceschi, P., Summer, A., Tuohy, K. M., Bittante, G., et al. (2019). Evaluation of autochthonous lactic acid bacteria as starter and non-starter cultures for the production of Traditional Mountain cheese. Food Res. Int. 115, 209–218. doi: 10.1016/j.foodres.2018.08.069
Carraro, L., Maifreni, M., Bartolomeoli, I., Martino, M. E., Novelli, E., Frigo, F., et al. (2011). Comparison of culture-dependent and-independent methods for bacterial community monitoring during Montasio cheese manufacturing. Res. Microbiol. 162, 231–239. doi: 10.1016/j.resmic.2011.01.002
Castro, R. D., Oliveira, L. G., Sant Anna, F. M., Luiz, L. M. P., Sandes, S. H. C., Silva, C. I. F., et al. (2016). Lactic acid microbiota identification in water, raw milk, endogenous starter culture, and fresh Minas artisanal cheese from the campo das Vertentes region of Brazil during the dry and rainy seasons. J. Dairy Sci. 99, 6086–6096. doi: 10.3168/jds.2015-10579
Centeno, J. A., Rodriguez-Otero, J. L., and Cepeda, A. (1994). Microbiological study of Arzúa cheese (NW Spain) throughout cheesemaking and ripening. J. Food Saf. 14, 229–241. doi: 10.1111/j.1745-4565.1994.tb00596.x
Çetinkaya, F., and Ece Soyutemiz, G. (2006). Microbiological and chemical changes throughout the manufacture and ripening of Kashar: a traditional Turkish cheese. Turk. J. Vet. Anim. Sci. 30, 397–404.
Claesson, M. J., Wang, Q., O’Sullivan, O., Greene-Diniz, R., Cole, J. R., Ross, R. P., et al. (2010). Comparison of two next-generation sequencing technologies for resolving highly complex microbiota composition using tandem variable 16S rRNA gene regions. Nucleic Acids Res. 38:e200. doi: 10.1093/nar/gkq873
Colombo, F., Borgo, F., and Fortina, M. G. (2009). Genotypic characterization of non starter lactic acid bacteria involved in the ripening of artisanal Bitto PDO cheese. J. Basic Microbiol. 49, 521–530. doi: 10.1002/jobm.200800381
Coloretti, F., Chiavari, C., Nocetti, M., Reverberi, P., Bortolazzo, E., Musi, V., et al. (2016). Whey starter addition during maturation of evening milk: effects on some characteristics of cheese milk and Parmigiano–Reggiano cheese. Dairy Sci. Technol. 96, 185–197. doi: 10.1007/s13594-015-0257-y
Coppola, S., Fusco, V., Andolfi, R., Aponte, M., Blaiotta, G., Ercolini, D., et al. (2006). Evaluation of microbial diversity during the manufacture of Fior di latte di Agerola, a traditional raw milk pasta-filata cheese of the Naples area. J. Dairy Res. 73, 264–272. doi: 10.1017/S0022029906001804
Coppola, R., Nanni, M., Iorizzo, M., Sorrentino, A., Sorrentino, E., Chiavari, C., et al. (2000). Microbiological characteristics of Parmigiano Reggiano cheese during the cheesemaking and the first months of the ripening. Lait 80, 479–490. doi: 10.1051/lait:2000139
Cremonesi, P., Morandi, S., Ceccarani, C., Battelli, G., Castiglioni, B., Cologna, N., et al. (2020). Raw Milk microbiota modifications as affected by chlorine usage for cleaning procedures: the Trentingrana PDO case. Front. Microbiol. 11, 1–14. doi: 10.3389/fmicb.2020.564749
D’Incecco, P., Bancalari, E., Gatti, M., Ranghetti, A., and Pellegrino, L. (2020). Low-temperature centrifugation of milk for manufacture of raw milk cheeses: impact on milk debacterization and cheese yield. Lwt 118:108789. doi: 10.1016/j.lwt.2019.108789
De Dea Lindner, J., Bernini, V., De Lorentiis, A., Pecorari, A., Neviani, E., and Gatti, M. (2008). Parmigiano Reggiano cheese: evolution of cultivable and total lactic microflora and peptidase activities during manufacture and ripening. Dairy Sci. Technol. 88, 511–523. doi: 10.1051/dst:2008019
De Filippis, F., Genovese, A., Ferranti, P., Gilbert, J. A., and Ercolini, D. (2016). Metatranscriptomics reveals temperature-driven functional changes in microbiome impacting cheese maturation rate. Sci. Rep. 6:21871. doi: 10.1038/srep21871
De Filippis, F., Parente, E., Zotta, T., and Ercolini, D. (2018). A comparison of bioinformatic approaches for 16S rRNA gene profiling of food bacterial microbiota. Int. J. Food Microbiol. 265, 9–17. doi: 10.1016/j.ijfoodmicro.2017.10.028
De Filippis, F., Pasolli, E., and Ercolini, D. (2020). The food-gut axis: lactic acid bacteria and their link to food, the gut microbiome and human health. FEMS Microbiol. Rev. 44, 454–489. doi: 10.1093/femsre/fuaa015
De Freitas, I., Pinon, N., Thierry, A., Lopez, C., Maubois, J.-L., and Lortal, S. (2007). In depth dynamic characterisation of French PDO Cantal cheese made from raw milk. Lait 87, 97–117. doi: 10.1051/lait:2007007
De la Rosa-Alcaraz, M., de Los, Á., Ortiz-Estrada, Á. M., Heredia-Castro, P. Y., Hernández-Mendoza, A., Reyes-Díaz, R., et al. (2020). Poro de Tabasco cheese: chemical composition and microbiological quality during its artisanal manufacturing process. J. Dairy Sci. 103, 3025–3037. doi: 10.3168/jds.2019-17363
De Man, J. C., Rogosa, M., and Sharpe, M. E. (1960). A medium for the cultivation of lactobacilli. J. Appl. Bacteriol. 23, 130–135. doi: 10.1111/j.1365-2672.1960.tb00188.x
De Pasquale, I., Di Cagno, R., Buchin, S., De Angelis, M., and Gobbetti, M. (2014). Microbial ecology dynamics reveal a succession in the core microbiota involved in the ripening of pasta filata Caciocavallo Pugliese cheese. Appl. Environ. Microbiol. 80, 6243–6255. doi: 10.1128/AEM.02097-14
Delbès, C., Ali-Mandjee, L., and Montel, M. C. (2007). Monitoring bacterial communities in raw milk and cheese by culture-dependent and-independent 16S rRNA gene-based analyses. Appl. Environ. Microbiol. 73, 1882–1891. doi: 10.1128/AEM.01716-06
Denis, C., Gueguen, M., Henry, E., and Levert, D. (2001). New media for the numeration of cheese surface bacteria. Lait 81, 365–379. doi: 10.1051/lait:2001138
Desmasures, N., Bazin, F., and Guéguen, M. (1997). Microbiological composition of raw milk from selected farms in the camembert region of Normandy. J. Appl. Microbiol. 83, 53–58. doi: 10.1046/j.1365-2672.1997.00166.x
Dolci, P., Alessandria, V., Rantsiou, K., Rolle, L., Zeppa, G., and Cocolin, L. (2008). Microbial dynamics of Castelmagno PDO, a traditional Italian cheese, with a focus on lactic acid bacteria ecology. Int. J. Food Microbiol. 122, 302–311. doi: 10.1016/j.ijfoodmicro.2007.12.018
Dolci, P., De Filippis, F., La Storia, A., Ercolini, D., and Cocolin, L. (2014). rRNA-based monitoring of the microbiota involved in fontina PDO cheese production in relation to different stages of cow lactation. Int. J. Food Microbiol. 185, 127–135. doi: 10.1016/j.ijfoodmicro.2014.05.021
Dreier, M., Meola, M., Berthoud, H., Shani, N., Wechsler, D., and Junier, P. (2022). High-throughput qPCR and 16S rRNA gene amplicon sequencing as complementary methods for the investigation of the cheese microbiota. BMC Microbiol. 22, 48–18. doi: 10.1186/s12866-022-02451-y
EC (2004). Corrigendum to Regulation (EC) no 853/2004 of the European Parliament and of the Council of 29 April 2004 laying down specific hygiene rules for food of animal origin (OJ L 139, 30.4.2004). Off. J. Eur. Union
EC (2006). Commission Regulation (EC) no 1664/2006 of 6 November 2006 amending Regulation (EC) no 2074/2005 as regards implementing measures for certain products of animal origin intended for human consumption and repealing certain implementing measures. Official J. Eur. Union L 320, 13–45.
Eliskases-Lechner, F., Ginzinger, W., Rohm, H., and Tschager, E. (1999). Raw milk flora affects composition and quality of Bergkäse. 1. Microbiology and fermentation compounds. Lait 79, 385–396. doi: 10.1051/lait:1999432
EP and Council of EU (2012). REGULATION (EU) no 1151/2012 OF THE EUROPEAN PARLIAMENT AND OF THE COUNCIL of 21 November 2012 on quality schemes for agricultural products and foodstuffs. Off. J. Eur. Union L 343/1
Ercolini, D., Moschetti, G., Blaiotta, G., and Coppola, S. (2001). The potential of a polyphasic PCR-DGGE approach in evaluating microbial diversity of natural whey cultures for water-buffalo mozzarella cheese production: bias of culture-dependent and culture-independent analyses. Syst. Appl. Microbiol. 24, 610–617. doi: 10.1078/0723-2020-00076
Escobar-Zepeda, A., Sanchez-Flores, A., and Quirasco Baruch, M. (2016). Metagenomic analysis of a Mexican ripened cheese reveals a unique complex microbiota. Food Microbiol. 57, 116–127. doi: 10.1016/j.fm.2016.02.004
Falardeau, J., Keeney, K., Trmčić, A., Kitts, D., and Wang, S. (2019). Farm-to-fork profiling of bacterial communities associated with an artisan cheese production facility. Food Microbiol. 83, 48–58. doi: 10.1016/j.fm.2019.04.002
Fleet, G. H. (1999). Microorganisms in food ecosystems. Int. J. Food Microbiol. 50, 101–117. doi: 10.1016/S0168-1605(99)00080-X
Franceschi, P., Malacarne, M., Formaggioni, P., Faccia, M., and Summer, A. (2021). Effects of milk storage temperature at the farm on the characteristics of parmigiano reggiano cheese: chemical composition and proteolysis. Animals 11, 1–12. doi: 10.3390/ani11030879
Franciosi, E., De Sabbata, G., Gardini, F., Cavazza, A., and Poznanski, E. (2011a). Changes in psychrotrophic microbial populations during milk creaming to produce grana Trentino cheese. Food Microbiol. 28, 43–51. doi: 10.1016/j.fm.2010.08.003
Franciosi, E., Gardini, F., Monfredini, L., Tabanelli, G., Fabris, A., Endrizzi, I., et al. (2012). Does milk treatment before cheesemaking affect microbial and chemical traits of ripened cheese? Grana Trentino as a case study. J. Dairy Sci. 95, 5485–5494. doi: 10.3168/jds.2011-4693
Franciosi, E., Settanni, L., Cavazza, A., and Poznanski, E. (2009). Biodiversity and technological potential of wild lactic acid bacteria from raw cows’ milk. Int. Dairy J. 19, 3–11. doi: 10.1016/j.idairyj.2008.07.008
Franciosi, E., Settanni, L., Cologna, N., Cavazza, A., and Poznanski, E. (2011b). Microbial analysis of raw cows’ milk used for cheese-making: influence of storage treatments on microbial composition and other technological traits. World J. Microbiol. Biotechnol. 27, 171–180. doi: 10.1007/s11274-010-0443-2
Futschik, K., and Pfutzner, H., (1995). “Electrode and media impedance for detection and characterization of microorganisms,” in IEEE/Engineering in Medicine and Biology Society Annual Conference 75–76.
Gagnon, M., Ouamba, A. J. K., LaPointe, G., Chouinard, P. Y., and Roy, D. (2020). Prevalence and abundance of lactic acid bacteria in raw milk associated with forage types in dairy cow feeding. J. Dairy Sci. 103, 5931–5946. doi: 10.3168/jds.2019-17918
Galli, V., Venturi, M., Mari, E., Guerrini, S., and Granchi, L. (2022). Gamma-aminobutyric acid (GABA) production in fermented milk by lactic acid bacteria isolated from spontaneous raw milk fermentation. Int. Dairy J. 127:105284. doi: 10.1016/j.idairyj.2021.105284
García Fontán, M. C., Franco, I., Prieto, B., Tornadijo, M. E., and Carballo, J. (2001). Microbiological changes in “san Simón” cheese throughout ripening and its relationship with physico-chemical parameters. Food Microbiol. 18, 25–33. doi: 10.1006/fmic.2000.0351
Gatti, M., Bottari, B., Lazzi, C., Neviani, E., and Mucchetti, G. (2014). Invited review: microbial evolution in raw-milk, long-ripened cheeses produced using undefined natural whey starters. J. Dairy Sci. 97, 573–591. doi: 10.3168/jds.2013-7187
Gatti, M., De Dea Lindner, J., De Lorentiis, A., Bottari, B., Santarelli, M., Bernini, V., et al. (2008). Dynamics of whole and lysed bacterial cells during Parmigiano-Reggiano cheese production and ripening. Appl. Environ. Microbiol. 74, 6161–6167. doi: 10.1128/AEM.00871-08
Gatti, M., Lazzi, C., Rossetti, L., Mucchetti, G., and Neviani, E. (2003). Biodiversity in lactobacillus helveticus strains present in natural whey starter used for Parmigiano Reggiano cheese. J. Appl. Microbiol. 95, 463–470. doi: 10.1046/j.1365-2672.2003.01997.x
Gelsomino, R., Vancanneyt, M., Condon, S., Swings, J., and Cogan, T. M. (2001). Enterococcal diversity in the environment of an Irish Cheddar-type cheesemaking factory. Int. J. Food Microbiol. 71, 177–188. doi: 10.1016/S0168-1605(01)00620-1
Gezginc, Y., Karabekmez-Erdem, T., Tatar, H. D., Dağgeçen, E. C., Ayman, S., and Akyol, İ. (2022). Metagenomics and volatile profile of Turkish artisanal Tulum cheese microbiota. Food Biosci. 45:101497. doi: 10.1016/j.fbio.2021.101497
Giannino, M. L., Marzotto, M., Dellaglio, F., and Feligini, M. (2009). Study of microbial diversity in raw milk and fresh curd used for fontina cheese production by culture-independent methods. Int. J. Food Microbiol. 130, 188–195. doi: 10.1016/j.ijfoodmicro.2009.01.022
Giello, M., La Storia, A., Masucci, F., Di Francia, A., Ercolini, D., and Villani, F. (2017). Dynamics of bacterial communities during manufacture and ripening of traditional Caciocavallo of Castelfranco cheese in relation to cows’ feeding. Food Microbiol. 63, 170–177. doi: 10.1016/j.fm.2016.11.016
Giraffa, G. (2004). Studying the dynamics of microbial populations during food fermentation. FEMS Microbiol. Rev. 28, 251–260. doi: 10.1016/j.femsre.2003.10.005
Gobbetti, M., De Angelis, M., Di Cagno, R., Mancini, L., and Fox, P. F. (2015). Pros and cons for using non-starter lactic acid bacteria (NSLAB) as secondary/adjunct starters for cheese ripening. Trends Food Sci. Technol. 45, 167–178. doi: 10.1016/j.tifs.2015.07.016
Gobbetti, M., Di Cagno, R., Calasso, M., Neviani, E., Fox, P. F., and De Angelis, M. (2018). Drivers that establish and assembly the lactic acid bacteria biota in cheeses. Trends Food Sci. Technol. 78, 244–254. doi: 10.1016/j.tifs.2018.06.010
Henri-Dubernet, S., Desmasures, N., and Guéguen, M. (2008). Diversity and dynamics of lactobacilli populations during ripening of RDO camembert cheese. Can. J. Microbiol. 54, 218–228. doi: 10.1139/W07-137
Hickey, D. K., Kilcawley, K. N., Beresford, T. P., and Wilkinson, M. G. (2007). Lipolysis in Cheddar cheese made from raw, thermized, and pasteurized milks. J. Dairy Sci. 90, 47–56. doi: 10.3168/jds.S0022-0302(07)72607-3
Hill, D., Sugrue, I., Tobin, C., Hill, C., Stanton, C., and Ross, R. P. (2018). The lactobacillus casei group: history and health related applications. Front. Microbiol. 9, 1–12. doi: 10.3389/fmicb.2018.02107
Irkin, R. (2010). Determination of microbial contamination sources for use in quality management of cheese industry: Dil cheese as an example. J. Verbraucherschutz Lebensmittelsicherh. 5, 91–96. doi: 10.1007/s00003-009-0525-y
ISO 4833-2 (2013). Microbiology of the Food Chain — Horizontal method for the enumeration of microorganisms — Part 2: Colony count at 30°C by the surface plating technique.
Isolini, D., Grand, M., and Glättli, H.-U. (1990). Selektivmedien zum Nachweis von obligat und fakultativ heterofermentative Laktobazillen. Forschungsanstalt für Milchwirtschaft 19, 57–59.
Jacomy, M., Venturini, T., Heymann, S., and Bastian, M. (2014). ForceAtlas2, a continuous graph layout algorithm for handy network visualization designed for the Gephi software. PLoS One 9, 1–12. doi: 10.1371/journal.pone.0098679
Kamimura, B. A., Cabral, L., Noronha, M. F., Baptista, R. C., Nascimento, H. M., and Sant Ana, A. S. (2020). Amplicon sequencing reveals the bacterial diversity in milk, dairy premises and Serra da Canastra artisanal cheeses produced by three different farms. Food Microbiol. 89:103453. doi: 10.1016/j.fm.2020.103453
Kongo, J. M., Gomes, A. M., Malcata, F. X., and McSweeney, P. L. H. (2009). Microbiological, biochemical and compositional changes during ripening of São Jorge – a raw milk cheese from the Azores (Portugal). Food Chem. 112, 131–138. doi: 10.1016/j.foodchem.2008.05.067
Lanzanova, M., Mucchetti, G., and Neviani, E. (1993). Analysis of conductance changes as a growth index of lactic acid bacteria in Milk. J. Dairy Sci. 76, 20–28. doi: 10.3168/jds.S0022-0302(93)77319-1
Lazzi, C., Gatti, M., Bernini, V., De Dea Lindner, J., and Neviani, E. (2007). Impiego di nuovi terreni colturali a base di cagliata e di formaggio per il recupero e la differenziazione della microflora caratteristica di formaggi a lunga stagionatura. Scienza e tecnica lattiero-casearia 58, 55–69.
Lazzi, C., Turroni, S., Mancini, A., Sgarbi, E., Neviani, E., Brigidi, P., et al. (2014). Transcriptomic clues to understand the growth of lactobacillus rhamnosus in cheese. BMC Microbiol. 14:28. doi: 10.1186/1471-2180-14-28
Levante, A., Bertani, G., Bottari, B., Bernini, V., Lazzi, C., Gatti, M., et al. (2020). How new molecular approaches have contributed to shedding light on microbial dynamics in Parmigiano Reggiano cheese. Curr. Opin. Food Sci. 38, 131–140. doi: 10.1016/j.cofs.2020.11.005
Levante, A., De Filippis, F., La Storia, A., Gatti, M., Neviani, E., Ercolini, D., et al. (2017). Metabolic gene-targeted monitoring of non-starter lactic acid bacteria during cheese ripening. Int. J. Food Microbiol. 257, 276–284. doi: 10.1016/j.ijfoodmicro.2017.07.002
Levante, A., Folli, C., Montanini, B., Ferrari, A., Neviani, E., and Lazzi, C. (2019). Expression of DINJ-YAFQ system of lactobacillus casei group strains in response to food processing stresses. Microorganisms 7, 438–453. doi: 10.3390/microorganisms7100438
Levante, A., Lazzi, C., Vatsellas, G., Chatzopoulos, D., Dionellis, V. S., Makrythanasis, P., et al. (2021). Genome sequencing of five Lacticaseibacillus strains and analysis of type I and II toxin-antitoxin system distribution. Microorganisms 9:648. doi: 10.3390/microorganisms9030648
Luiz, L. M. P., Castro, R. D., Sandes, S. H. C., Silva, J. G., Oliveira, L. G., Sales, G. A., et al. (2017). Isolation and identification of lactic acid bacteria from Brazilian Minas artisanal cheese. CYTA – J. Food 15, 1–4. doi: 10.1080/19476337.2016.1219392
Mallet, A., Guéguen, M., Kauffmann, F., Chesneau, C., Sesboué, A., and Desmasures, N. (2012). Quantitative and qualitative microbial analysis of raw milk reveals substantial diversity influenced by herd management practices. Int. Dairy J. 27, 13–21. doi: 10.1016/j.idairyj.2012.07.009
Mangia, N. P., Fancello, F., and Deiana, P. (2016). Microbiological characterization using combined culture dependent and independent approaches of Casizolu pasta filata cheese. J. Appl. Microbiol. 120, 329–345. doi: 10.1111/jam.13001
Marino, M., Maifreni, M., Bartolomeoli, I., and Rondinini, G. (2008). Evaluation of amino acid-decarboxylative microbiota throughout the ripening of an Italian PDO cheese produced using different manufacturing practices. J. Appl. Microbiol. 105, 540–549. doi: 10.1111/j.1365-2672.2008.03793.x
Masoud, W., Vogensen, F. K., Lillevang, S., Abu Al-Soud, W., Sørensen, S. J., and Jakobsen, M. (2012). The fate of indigenous microbiota, starter cultures, Escherichia coli, listeria innocua and Staphylococcus aureus in Danish raw milk and cheeses determined by pyrosequencing and quantitative real time (qRT)-PCR. Int. J. Food Microbiol. 153, 192–202. doi: 10.1016/j.ijfoodmicro.2011.11.014
McSweeney, P. L. H., Fox, P. F., Lucey, J. A., Jordan, K. N., and Cogan, T. M. (1993). Contribution of the indigenous microflora to the maturation of cheddar cheese. Int. Dairy J. 3, 613–634. doi: 10.1016/0958-6946(93)90104-8
Medvedová, A., Koňuchová, M., Kvočiková, K., Hatalová, I., and Valík, L. (2020). Effect of lactic acid bacteria addition on the microbiological safety of pasta-Filata types of cheeses. Front. Microbiol. 11, 1–16. doi: 10.3389/fmicb.2020.612528
Michel, V., Hauwuy, A., and Chamba, J.-F. (2001). La flore microbienne de laits crus de vache: diversité et influence des conditions de production. Lait 81, 575–592. doi: 10.1051/lait:2001151
Mipaaf, (2022). D.M. 25 febbraio 2022. Aggiornamento dell’elenco nazionale dei prodotti agroalimentari tradizionali ai sensi dell’articolo 12, comma 1, della legge 12 dicembre 2016, n. 238 (XXI revisione) (22A01528). Gazzetta Ufficiale della Repubblica Italiana 67.
Monfredini, L., Settanni, L., Poznanski, E., Cavazza, A., and Franciosi, E. (2012). The spatial distribution of bacteria in grana-cheese during ripening. Syst. Appl. Microbiol. 35, 54–63. doi: 10.1016/j.syapm.2011.07.002
Montel, M. C., Buchin, S., Mallet, A., Delbes-Paus, C., Vuitton, D. A., Desmasures, N., et al. (2014). Traditional cheeses: rich and diverse microbiota with associated benefits. Int. J. Food Microbiol. 177, 136–154. doi: 10.1016/j.ijfoodmicro.2014.02.019
Mucchetti, G., Gatti, M., and Neviani, E. (1994). Electrical conductivity changes in Milk caused by acidification: determining factors. J. Dairy Sci. 77, 940–944. doi: 10.3168/jds.S0022-0302(94)77029-6
Mucchetti, G., Ghiglietti, R., Locci, F., Francolino, S., Bonvini, B., Remagni, M. C., et al. (2009). Technological, microbiological and chemical characteristics of Pannerone, a traditional Italian raw milk cheese. Dairy Sci. Technol. 89, 419–436. doi: 10.1051/dst/2009017
Ndoye, B., Rasolofo, E. A., LaPointe, G., and Roy, D. (2011). A review of the molecular approaches to investigate the diversity and activity of cheese microbiota. Dairy Sci. Technol. 91, 495–524. doi: 10.1007/s13594-011-0031-8
Neviani, E., Bottari, B., Lazzi, C., and Gatti, M. (2013). New developments in the study of the microbiota of raw-milk, long-ripened cheeses by molecular methods: the case of grana Padano and Parmigiano Reggiano. Front. Microbiol. 4, 1–14. doi: 10.3389/fmicb.2013.00036
Neviani, E., De Dea Lindner, J., Bernini, V., and Gatti, M. (2009). Recovery and differentiation of long ripened cheese microflora through a new cheese-based cultural medium. Food Microbiol. 26, 240–245. doi: 10.1016/j.fm.2009.01.004
Nikoloudaki, O., Lemos Junior, W. J. F., Borruso, L., Campanaro, S., De Angelis, M., Vogel, R. F., et al. (2021). How multiple farming conditions correlate with the composition of the raw cow’s milk lactic microbiome. Environ. Microbiol. 23, 1702–1716. doi: 10.1111/1462-2920.15407
Oikonomou, G., Addis, M. F., Chassard, C., Nader-Macias, M. E. F., Grant, I., Delbès, C., et al. (2020). Milk microbiota: what are we exactly talking about? Front. Microbiol. 11, 1–15. doi: 10.3389/fmicb.2020.00060
Page, M. J., McKenzie, J. E., Bossuyt, P. M., Boutron, I., Hoffmann, T. C., Mulrow, C. D., et al. (2021). The PRISMA 2020 statement: an updated guideline for reporting systematic reviews. BMJ 372:n71. doi: 10.1136/bmj.n71
Papadimitriou, K., Alegría, Á., Bron, P. A., de Angelis, M., Gobbetti, M., Kleerebezem, M., et al. (2016). Stress physiology of lactic acid bacteria. Microbiol. Mol. Biol. Rev. 80, 837–890. doi: 10.1128/mmbr.00076-15
Parente, E., Cocolin, L., De Filippis, F., Zotta, T., Ferrocino, I., O’Sullivan, O., et al. (2016). FoodMicrobionet: a database for the visualisation and exploration of food bacterial communities based on network analysis. Int. J. Food Microbiol. 219, 28–37. doi: 10.1016/j.ijfoodmicro.2015.12.001
Parente, E., De Filippis, F., Ercolini, D., Ricciardi, A., and Zotta, T. (2019). Advancing integration of data on food microbiome studies: FoodMicrobionet 3.1, a major upgrade of the FoodMicrobionet database. Int. J. Food Microbiol. 305:108249. doi: 10.1016/j.ijfoodmicro.2019.108249
Parente, E., Ricciardi, A., and Zotta, T. (2020). The microbiota of dairy milk: a review. Int. Dairy J. 107:104714. doi: 10.1016/j.idairyj.2020.104714
Parente, E., Zotta, T., and Ricciardi, A. (2022). FoodMicrobionet v4: a large, integrated, open and transparent database for food bacterial communities. BioRXiv :2022.01.19.476946. doi: 10.1101/2022.01.19.476946
Porcellato, D., Smistad, M., Bombelli, A., Abdelghani, A., Jørgensen, H. J., and Skeie, S. B. (2021). Longitudinal study of the bulk tank Milk microbiota reveals major temporal shifts in composition. Front. Microbiol. 12:616429. doi: 10.3389/fmicb.2021.616429
Poznanski, E., Cavazza, A., Cappa, F., and Cocconcelli, P. S. (2004). Indigenous raw milk microbiota influences the bacterial development in traditional cheese from an alpine natural park. Int. J. Food Microbiol. 92, 141–151. doi: 10.1016/j.ijfoodmicro.2003.09.006
Props, R., Kerckhof, F.-M., Rubbens, P., De Vrieze, J., Hernandez Sanabria, E., Waegeman, W., et al. (2017). Absolute quantification of microbial taxon abundances. ISME J. 11, 584–587. doi: 10.1038/ismej.2016.117
Quigley, L., O’Sullivan, O., Beresford, T. P., Ross, R. P., Fitzgerald, G. F., and Cotter, P. D. (2011). Molecular approaches to analysing the microbial composition of raw milk and raw milk cheese. Int. J. Food Microbiol. 150, 81–94. doi: 10.1016/j.ijfoodmicro.2011.08.001
Quigley, L., O’Sullivan, O., Stanton, C., Beresford, T. P., Ross, R. P., Fitzgerald, G. F., et al. (2013). The complex microbiota of raw milk. FEMS Microbiol. Rev. 37, 664–698. doi: 10.1111/1574-6976.12030
R Core Team (2022). R: A language and environment for statistical computing. Vienna, Austria: R Foundation for Statistical Computing. Available at: https://www.R-project.org/
Raats, D., Offek, M., Minz, D., and Halpern, M. (2011). Molecular analysis of bacterial communities in raw cow milk and the impact of refrigeration on its structure and dynamics. Food Microbiol. 28, 465–471. doi: 10.1016/j.fm.2010.10.009
Randazzo, C. L., Caggia, C., and Neviani, E. (2009). Application of molecular approaches to study lactic acid bacteria in artisanal cheeses. J. Microbiol. Methods 78, 1–9. doi: 10.1016/j.mimet.2009.04.001
Randazzo, C. L., Torriani, S., Akkermans, A. D. L., De Vos, W. M., and Vaughan, E. E. (2002). Diversity, dynamics, and activity of bacterial communities during production of an artisanal sicilian cheese as evaluated by 16S rRNA analysis. Appl. Environ. Microbiol. 68, 1882–1892. doi: 10.1128/AEM.68.4.1882-1892.2002
Rehman, S. U., Banks, J. M., McSweeney, P. L. H., and Fox, P. F. (2000a). Effect of ripening temperature on the growth and significance of non-starter lactic acid bacteria in Cheddar cheese made from raw or pasteurised milk. Int. Dairy J. 10, 45–53. doi: 10.1016/S0958-6946(00)00022-4
Rehman, S. U., McSweeney, P. L. H., Banks, J. M., Brechany, E. Y., Muir, D. D., and Fox, P. F. (2000b). Ripening of Cheddar cheese made from blends of raw and pasteurised milk. Int. Dairy J. 10, 33–44. doi: 10.1016/S0958-6946(00)00024-8
Rodriguez Medina, M. L., Tornadijo, M. E., Carballo, J., and Sarmiento, R. M. (1995). Microbiological study of león raw cow-milk cheese, a spanish craft variety. J. Food Prot. 58, 998–1006. doi: 10.4315/0362-028X-58.9.998
Santarelli, M., Bottari, B., Lazzi, C., Neviani, E., and Gatti, M. (2013). Survey on the community and dynamics of lactic acid bacteria in grana Padano cheese. Syst. Appl. Microbiol. 36, 593–600. doi: 10.1016/j.syapm.2013.04.007
Settanni, L., Di Grigoli, A., Tornambé, G., Bellina, V., Francesca, N., Moschetti, G., et al. (2012). Persistence of wild Streptococcus thermophilus strains on wooden vat and during the manufacture of a traditional Caciocavallo type cheese. Int. J. Food Microbiol. 155, 73–81. doi: 10.1016/j.ijfoodmicro.2012.01.022
Settanni, L., and Moschetti, G. (2010). Non-starter lactic acid bacteria used to improve cheese quality and provide health benefits. Food Microbiol. 27, 691–697. doi: 10.1016/j.fm.2010.05.023
Sgarbi, E., Bottari, B., Gatti, M., and Neviani, E. (2014). Investigation of the ability of dairy nonstarter lactic acid bacteria to grow using cell lysates of other lactic acid bacteria as the exclusive source of nutrients. Int. J. Dairy Technol. 67, 342–347. doi: 10.1111/1471-0307.12132
Sgarbi, E., Lazzi, C., Tabanelli, G., Gatti, M., Neviani, E., and Gardini, F. (2013). Nonstarter lactic acid bacteria volatilomes produced using cheese components. J. Dairy Sci. 96, 4223–4234. doi: 10.3168/jds.2012-6472
Skeie, S. B., Håland, M., Thorsen, I. M., Narvhus, J., and Porcellato, D. (2019). Bulk tank raw milk microbiota differs within and between farms: a moving goalpost challenging quality control. J. Dairy Sci. 102, 1959–1971. doi: 10.3168/jds.2017-14083
Sohier, D., Jamet, E., Le Dizes, A. S., Dizin, M., Pavan, S., Postollec, F., et al. (2012). Polyphasic approach for quantitative analysis of obligately heterofermentative lactobacillus species in cheese. Food Microbiol. 31, 271–277. doi: 10.1016/j.fm.2012.01.009
Solieri, L., Bianchi, A., and Giudici, P. (2012). Inventory of non starter lactic acid bacteria from ripened Parmigiano Reggiano cheese as assessed by a culture dependent multiphasic approach. Syst. Appl. Microbiol. 35, 270–277. doi: 10.1016/j.syapm.2012.04.002
Temmerman, R., Huys, G., and Swings, J. (2004). Identification of lactic acid bacteria: culture-dependent and culture-independent methods. Trends Food Sci. Technol. 15, 348–359. doi: 10.1016/j.tifs.2003.12.007
Terzaghi, B. E., and Sandine, W. E. (1975). Improved medium for lactic streptococci and their bacteriophages. Appl. Microbiol. 29, 807–813. doi: 10.1128/am.29.6.807-813.1975
Terzic-Vidojevic, A., Vukasinovic, M., Veljovic, K., Ostojic, M., and Topisirovic, L. (2007). Characterization of microflora in homemade semi-hard white Zlatar cheese. Int. J. Food Microbiol. 114, 36–42. doi: 10.1016/j.ijfoodmicro.2006.10.038
Tilocca, B., Costanzo, N., Morittu, V. M., Spina, A. A., Soggiu, A., Britti, D., et al. (2020). Milk microbiota: characterization methods and role in cheese production. J. Proteome 210:103534. doi: 10.1016/j.jprot.2019.103534
Trmčić, A., Obermajer, T., Majhenič, A. Č., Matijašić, B. B., and Rogelj, I. (2011). Competitive advantage of bacteriocinogenic strains within lactic acid bacteria consortium of raw milk cheese; [Kompetitivna prednost bakteriocinogenih sojeva konzorcija bakterija mliječne kiseline izoliranih iz sira proizvedenog od sirovog mlijeka]. Mljekarstvo 61, 26–32.
Vandeputte, D., Kathagen, G., Dhoe, K., Vieira-Silva, S., Valles-Colomer, M., Sabino, J., et al. (2017). Quantitative microbiome profiling links gut community variation to microbial load. Nature 551, 507–511. doi: 10.1038/nature24460
Vrdoljak, M., Tudor Kalit, M., Dolenčić Špehar, I., Radeljević, B., Jelić, M., Mandinić, S., et al. (2022). Effects of the autochthonous probiotic bacteria lactobacillus plantarum B and Lactococcus lactis Subsp. lactis S1 on the proteolysis of Croatian cheese ripened in a lambskin sack (sir iz Mišine). Fermentation 8, 382–392. doi: 10.3390/fermentation8080382
Wickham, H., Averick, M., Bryan, J., Chang, W., McGowan, L. D., François, R., et al., (2019). Welcome to the tidyverse. J. Open Source Softw. 4:1686. doi: 10.21105/joss.01686
Yang, L., and Bashir, R. (2008). Electrical/electrochemical impedance for rapid detection of foodborne pathogenic bacteria. Biotechnol. Adv. 26, 135–150. doi: 10.1016/j.biotechadv.2007.10.003
Yunita, D., and Dodd, C. E. R. (2018). Microbial community dynamics of a blue-veined raw milk cheese from the United Kingdom. J. Dairy Sci. 101, 4923–4935. doi: 10.3168/jds.2017-14104
Zheng, J., Wittouck, S., Salvetti, E., Franz, C. M. A. P., Harris, H. M. B., Mattarelli, P., et al. (2020). A taxonomic note on the genus lactobacillus: description of 23 novel genera, emended description of the genus lactobacillus Beijerinck 1901, and union of Lactobacillaceae and Leuconostocaceae. Int. J. Syst. Evol. Microbiol. 70, 2782–2858. doi: 10.1099/ijsem.0.004107
Keywords: raw milk cheese, raw milk microbiota, cheese microbiota, cheese ripening, LAB, NSLAB, Lacticaseibacillus
Citation: Bettera L, Levante A, Bancalari E, Bottari B and Gatti M (2023) Lactic acid bacteria in cow raw milk for cheese production: Which and how many? Front. Microbiol. 13:1092224. doi: 10.3389/fmicb.2022.1092224
Edited by:
Denis Roy, Laval University, CanadaReviewed by:
Athanasios Alexopoulos, Democritus University of Thrace, GreeceFlavio Tidona, Council for Agricultural and Economics Research (CREA), Italy
Copyright © 2023 Bettera, Levante, Bancalari, Bottari and Gatti. This is an open-access article distributed under the terms of the Creative Commons Attribution License (CC BY). The use, distribution or reproduction in other forums is permitted, provided the original author(s) and the copyright owner(s) are credited and that the original publication in this journal is cited, in accordance with accepted academic practice. No use, distribution or reproduction is permitted which does not comply with these terms.
*Correspondence: Monica Gatti, ✉ bW9uaWNhLmdhdHRpQHVuaXByLml0