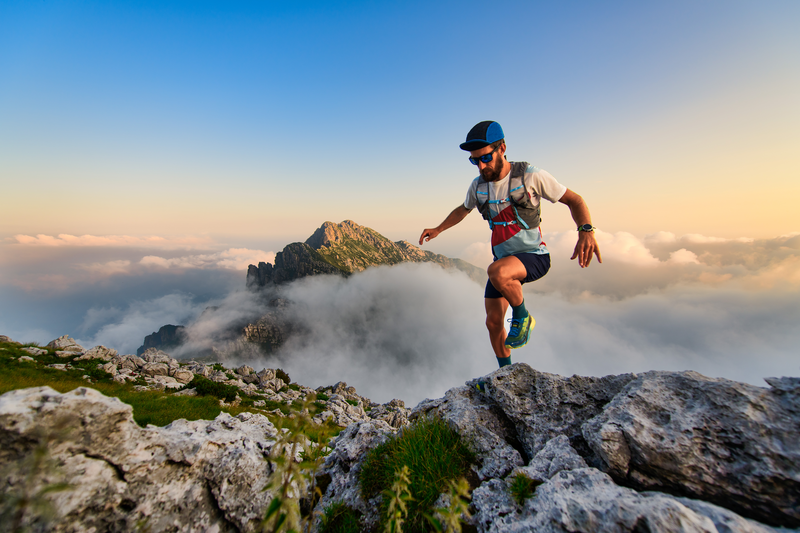
94% of researchers rate our articles as excellent or good
Learn more about the work of our research integrity team to safeguard the quality of each article we publish.
Find out more
ORIGINAL RESEARCH article
Front. Microbiol. , 22 December 2022
Sec. Microbial Symbioses
Volume 13 - 2022 | https://doi.org/10.3389/fmicb.2022.1079550
Associated microbes of several herbivorous insects can improve insect fitness. However, the contribution of specific insect gut bacterium to plant toxin toxification for its host fitness remains scarce. Here, a gut bacterium Raoultella terrigena from the ghost moth Thitarodes xiaojinensis larvae was identified. This bacterium grew unhindered in the presence of Polygonum viviparum, which is a natural food for ghost moth larvae but showed significant growth inhibition and toxicity against Spodoptera litura. S. litura reared on artificial diets containing 5, 15 and 25% P. viviparum powder after 7 days coculture with R. terrigena were found to have shorter larval and pupal durations than on the diets containing P. viviparum powder but without R. terrigena coculture. HPLC analysis revealed that the content of quercetin in mineral medium containing 15% P. viviparum powder after 7 days coculture with R. terrigena was significantly decreased (79.48%) as compared with that in P. viviparum powder without R. terrigena coculture. In vitro fermentation further verified that R. terrigena could degrade 85.56% quercetin in Lucia-Bertani medium. S. litura reared on artificial diets containing 0.01, 0.05 and 0.1 mg/g quercetin after 48 h coculture with R. terrigena were also found to have shorter larval, prepupal and pupal durations, as well as higher average pupal weight and adult emergence rate than on the diets containing quercetin, but without R. terrigena coculture. In addition, R. terrigena was detected in the bud and root tissues of the sterilized P. viviparum, indicating that T. xiaojinensis larvae might acquire this bacterium through feeding. These results demonstrate that the gut bacteria contribute to the degradation of plant toxic molecules to improve the development of herbivorous insects and provide fundamental knowledge for developing effective methods for beneficial insect rearing and pest control.
The interaction between insect and plant is often mediated by plant secondary metabolites. These molecules not only act as feeding attractants, but also as feeding deterrents or toxins to herbivorous insects by impeding digestion, hindering normal metabolism, or blocking nutrient transport, etc., (Mithöefer and Boland, 2012). In order to use plants as food sources, insect herbivores have to equip weapons to overcome a variety of plant defenses, including detoxifying plant secondary compounds. An array of xenobiotic detoxification enzymes such as P450, carboxylesterase and glutathione S-transferases in insects play an indispensable role in antioxidant defense and detoxification of plant secondary metabolites and exogenous xenobiotics (Li et al., 2007; Zhang et al., 2013). Recent evidence suggest that their microbiome vary across life stages and may affect a wide variety of host traits including fitness and immunity (Beran and Gershenzon, 2016; Ali et al., 2018; Itoh et al., 2018; Ali et al., 2019; Zaynab et al., 2019; Khan et al., 2020). Furthermore, insect gut is the main site of action of toxic plant metabolites and detoxification reaction. To help counter toxic effects of these defense compounds, several insects such as generalist lepidopteran herbivores cotton leafworm Spodoptera littoralis, coffee berry borer Hypothenemus hampei, pine weevil Hylobius abietis, leaf-cutter ant Atta colombica and A. cephalotes have been found to harbor gut microbiota with the metabolic ability to degrade plant secondary metabolites (Schramm et al., 2012; Ceja-Navarro et al., 2015; Berasategui et al., 2017; Francoeur et al., 2020).
The traditional medicinal plant Polygonum viviparum, belonging to Polygonaceae family, is a perennial herb and widely distributed in the alpine meadows at an altitude of 2,220–5,100 (Li and Shen, 1996). Most Polygonum plants have insecticidal, antifeedant and repellent activities, among which P. hydropiper has long been applied as plant insecticide and antifeedant (Zeng et al., 2008). It is deemed to be an effective biocide against many insect pests such as aphid Myzus persicae, armyworm Mythimna separata, diamond back moth Plutella xylostella, cabbage caterpillar Pieris rapae and rice planthopper Nilaparvata lugens, as well as confused flour beetle Tribolium confusum (Zeng et al., 2008). The acetone extracts from the whole plant of P. viviparum showed certain insecticidal activity against M. seperata (Li et al., 2009). The root of P. viviparum also showed significant growth inhibition and toxicity against the two important agricultural pests P. xylostella and Spodoptera litura (Qiu and Han, 2016). However, which secondary metabolites from P. viviparum inhibit insect growth remains unknown.
Major secondary metabolites of P. viviparum are flavonoids, include groups of bioactive gallic acid, quercetin and chlorogenic acid (Zhang et al., 2005; Li et al., 2014). Flavonoids are one of the largest and most diverse groups of plant secondary metabolites, with more than 6,500 diverse flavonoids being identified in plants (Ververidis et al., 2007). These compounds have been found to negatively affect development and behavior of insect herbivores from Hemiptera, Coleoptera, Lepidoptera, and Hymenoptera, by acting as feeding deterrents, growth inhibitors or toxins (Elliger et al., 1981; Lattanzio et al., 2000; Nyman and Julkunen-Tiitto, 2000; Salunke et al., 2005; Schnarr et al., 2022). Thus, these metabolites might be involved in P. viviparum resistance to herbivores.
However, the root of P. viviparum is the main food for the ghost moth larvae (Lepidoptera: Hepialidae: Thitarodes), an obligate host of the medicinal fungus Ophiocordyceps sinensis in the Tibetan plateau. The Chinese cordyceps formed by this parasitism has been considered as one of the most valued health food and traditional Asian medicine since the 15th century (Sung et al., 2007; Zhou et al., 2014; Han et al., 2019). Previous studies have demonstrated the flavonoids degradation by some bacterial species such as Bacteroides fragilis, B. uniformis, Bifidobacterium pseudocatenulatum, Clostridium perfringens, Enterococcus avium and Flavonifractor plautii in the human gut (Zhang et al., 2014; Braune and Blaut, 2016). However, whether flavonoids of P. viviparum exhibit inhibitory effect against S. litura, and whether gut microorganisms from the ghost moth larvae are involved in the detoxificationof the compounds in P. polygonum is still unknown.
In this study, R. terrigena was isolated from P. viviparum and the gut of Thitarodes xiaojinensis larvae which consumed the root powder of P. viviparum as a medium component. This bacterium significantly decreased the content of quercetin in P. viviparum root powder and was further demonstrated to degrade 85.56% quercetin by in vitro fermentation. This bacterium also improved the growth of S. litura which fed on the artificial medium containing the root powder of P. viviparum or quercetin. These results suggested that gut bacteria of ghost moth T. xiaojinensis larvae would involve in detoxifying P. viviparum flavonoids and thereby contributing to the adaptation of herbivorous insect to host plant. This study will not only help to understand the coevolutionary relationship between the insect and plant, but also provide new insights for artificial rearing of beneficial insects and for pest control.
Thitarodes xiaojinensis larvae, seeds and roots of P. viviparum were obtained from the known distribution areas of the Chinese cordyceps in the Xiaojin County, Sichuan Province, China (Liu et al., 2021). The larvae were collected alive and placed one larva in each vial filled with moss. All the larvae and roots were sent to the laboratory in foam boxes with ice packs wrapped with cotton to maintain the low temperature immediately after the collection. The collected larvae were identified by the amplification of the mitochondrial cytochrome b gene (cytb) sequence with the primers CB1 (TATGTACTACCATGAGGACAAATATC) and CB2 (ATTACACCTCCTAATTTATTAGGAAT) (Zou et al., 2010). S. litura larvae were artificially reared on a diet containing 15% wheatgerm powder, 4% yeast powder, 0.5% sucrose, 0.4% vitamin C, 14% agar, 0.4% sorbic acid and 0.4% methylparaben at 25°C, 70% relative humidity and a 12: 12 h L: D photoperiod.
Bacteria were isolated from the gut of T. xiaojinensis larvae and screened for the utilization of P. viviparum roots. Twenty T. xiaojinensis larvae were dissected and gut contents were macerated in 200 μl of 1 × phosphate buffer (pH = 7.4) and further diluted at a proportion of 1: 10 with 1 × phosphate buffer. Fifty microliters of the diluted gut suspension were spread on agar plates with mineral medium (9.5 mM KH2PO4, 4.8 mM MgSO4, 0.1 mM CaCl2, 0.8 mM Na2HPO4 and 10 g/l bacto agar), and a gradient of P. viviparum root powder ranging from 10 to 25% in weight. The P. viviparum root powder was prepared by a blender after the roots were washed by distilled water and dried at 25°C for 1 h. The agar plates were incubated in the dark at 13°C and 25°C, respectively. The growth of bacteria was examined every day and the morphologically distinct colonies were picked and further purified by quadrant streaking on LB (Lucia-Bertani) agar plates. Purified colonies were transferred to liquid mineral media containing 25% P. viviparum powder for confirming their growth. Each treatment was repeated three times.
The culture-dependent endophytes of P. viviparum were isolated for the first time by using the blendor method. Seeds of P. viviparum were surface sterilized using 10% NaClO for 30 min following by 10% formaldehyde for 20 min, and then washed using sterilized water for 5 times. Sterilized seeds were cultivated on mineral media at 15°C. Then, bud and root tissues from the sterilized seeds were used to isolate endophytic microbes. Prior to mortar and pestle maceration, unsterilized and surface-sterilized seeds were placed on LB medium to determine the effectiveness of the sterilization. An abundance of bacterial and fungal colonies was observed on the medium with unsterilized seeds, but no colony was observed on the medium plates with surface-sterilized seeds (Supplementary Figure S1). The bud and root tissues from surface sterilized seeds were then macerated and used as inoculum on plates containing modified Jensen’s medium (Gushgari-Doyle et al., 2021) and LB medium. Distinct colonies were purified by streaking on LB plates and identified as described above.
Bacterial isolates which grew on both agar plates and liquid fermentation of mineral medium containing P. viviparum powder were further characterized. For physiological and biochemical characterization, the bacteria were cultured on LB agar plates at 37°C for 24 h in the dark. Gram staining, casein hydrolysis, catalase, citrate utilization, gelatin liquefaction, hydrogen sulfide, indole, methyl red, nitrate reduction, oxidation and fermentation of glucose, phenylalanine deaminase, starch hydrolysis, urease and Voges-Proskauer tests were performed using standardized procedures of Bergey’s Manual of Systematic Bacteriology (Buchanan and Gibbons, 1984; Dong and Cai, 2001). For molecular characterization, DNA was extracted from bacterial cultures grown at 37°C for 24 h using an Ezup Column Bacteria Genomic DNA Purification kit (Sangon Biotech, Shanghai, China) according to the instructions. The prokaryotic V4 region of 16S rRNA was amplified with primers 27F (5’-AGAGTTTGATCCTGGCTCAG-3′) and 1492R (5’-TACGGYTACCTTGTTACGACTT-3′). The PCR product was separated by electrophoresis and the band was cut from the gels for purification, and then sequenced in Sangon Biotech (Shanghai) Co., Ltd., (Guangzhou, China). Sequence of the isolated bacterium was compared with non-redundant GenBank library using nucleotide blast search.1 The taxonomically related sequences were collected from NCBI Taxonomy Homepage. Phylogenetic tree was constructed by using Neighbor-Joining analysis in MEGA 5.10 software. The confidence at each node was assessed by 1,000 bootstrap replicates.
A gut bacterial isolate identified as R. terrigena was found to grow well in mineral medium containing 25% P. viviparum powder. Thus, this bacterial species was used for further experiments. Since no proper artificial media have been formulated for T. xiaojinensis larvae, a worldwide vegetable pest S. litura which can be reared on artificial media was used instead of T. xiaojinensis to determine the effect of R. terrigena. One milliliter of bacterial suspension with an optical density of 0.8 at 600 nm was inoculated in a 250 ml flask containing 100 ml mineral media supplemented with 20% P. viviparum powder. The flasks were then incubated for 7 days in a shaker at 200 rpm at 37°C. The fermented broths were divided into two parts: one part contained living R. terrigena bacteria and another part was autoclaved at 121°C for 10 min. The autoclaved and unautoclaved bacterial cultures were centrifuged at 2000 g for 5 min at 25°C. The precipitates from autoclaved and unautoclaved bacterial cultures were directly mixed with the artificial diet of S. litura according to the proportions of 5, 15 and 25%, respectively. The autoclaved and unautoclaved P. viviparum deposits without R. terrigena inoculation were mixed with the artificial diet in the above ratios and used as the control diets.
Twenty newly molted fourth instar larvae of S. litura were shifted to a sterile plastic container (diameter = 9 cm) padded with two sheets of sterile filter paper (Whatman no. 3). The larvae were fed on the diets containing 0, 5, 15 and 25% of the autoclaved and unautoclaved P. viviparum deposits, respectively. The diets were replaced every 24 h. Five replicates were established for each diet with 20 larvae for each replicate. The mortality of larvae and pupae, and the developmental duration in each larval and pupal stage were recorded. All the pupae were weighed on the first day post pupation.
To investigate whether the growth improvement of S. litura fed on the diets containing R. terrigena-fermented P. viviparum root powder was caused by the reduction of flavonoids content, the contents of quercetin and gallic acid in P. viviparum precipitates in the cultures with and without R. terrigena inoculation post 7 days at a 200 rpm shaker at 37°C were analyzed by high performance liquid chromatography (HPLC). The precipitates prepared by centrifugation as described above were freeze-dried by using Savant Modulyo D freeze drier (Thermo Fisher Scientific, Waltham, MA, United States). For each treatment, 0.5 g of dry sample was diluted to 50 ml with 30% methanol, and left for 2 h before ultrasonic extraction for 30 min and then used for HPLC analysis with a photodiode array Detector (1,100 series equipment, Agilent Technologies, Santa Clara, CA, United States). A C18 reversed-phase column (3.0 by 150 mm, 2.7 um) was used with the following parameters: 370 nm, 0.4 ml/min flow rate, and 10 μl injection volume for quercetin analysis, and 272 nm, 0.25 ml/min flow rate, and 1 μl injection volume for gallic acid analysis. The mobile phase consisted of 0.1% acetic acid in water and methanol. The peaks of standard quercetin (Macklin, Shanghai, China) and gallic acid (Macklin, Shanghai, China) under the above conditions were identified (Supplementary Figure S2).
Quercetin degradation by R. terrigena was further verified with the following procedures. R. terrigena was inoculated into LB medium at a 200 rpm shaker at 37°C for 16 h. Then 10 μl of R. terrigena culture with an optical density of 0.8 at 600 nm was inoculated into a vial of 990 μl LB medium supplemented with and without 0.004% quercetin. Ten microliters LB medium was inoculated into the control vial with 0.004% quercetin as a control. The vials were shaken at 200 rpm at 37°C for 48 h, and the resulting cultures were centrifuged at 5000 rpm for 5 min at 25°C to pellet bacterial cells and the supernatants were taken for quercetin analysis according to the method (Juan and Chou, 2010; Zhang et al., 2014). Briefly, 1 ml supernatant was mixed with 2.5 ml methanol. The centrifuged methanol extraction was then mixed with the same volume of 2% aluminium trichloride (AlCl3; Macklin, Shanghai, China) in methanol. The absorbance of supernatant was measured under 428 nm with UV Spectrophotometer (Unico UV-2800A, Shanghai, China). The degradation rate of quercetin by R. terrigena was determined using a standard curve with quercetin (Macklin, Shanghai, China; 0–0.05 mg/ml) as the standard. All tests were replicated three times.
Both bacterial and human pirins exhibit quercetinase activity, which can be inhibited by the addition of inhibitors of the quercetin 2,3-dioxygenase reaction (Adams and Jia, 2005). To investigate the transcription of the two genes in T. xiaojinensis, yhhw1 coding a pirin protein and yhhw3 coding a quercetin 2,3-dioxygenase were firstly cloned from R. terrigena and RT-PCR was performed on different templates including total RNA, cDNA and DNA. Total RNA and DNA were co-extracted and separated from whole T. xiaojinensis larvae collected from the natural location by TRIzol reagent (Invitrogen, Carlsbad, United States) and 1 μg RNA was used to synthesize the first strand cDNA in 20 μl-reaction system following the manufacturer’s instruction for the TransScript™ One-Step gDNA Removal and cDNA Synthesis SuperMix (TransGen, Beijing, China). The primers used for RT-PCR amplifications were TGGCTCGATTCCTGGCATAC and TGACGACCTGAATCCACACG (yhhw1), TGCCGGCCAAAGACAAAATG and AAATGGGCCATAGCCGACAA (yhhw3).
To assess the effect of quercetin on the development of S. litura, the contact and feeding toxicities of quercetin against the newly molted fourth instar larvae of S. litura were investigated. For the contact toxicity assay, quercetin at three different concentrations (1, 5 and 10 mg/ml) in 1 ml methanol was, respectively, sprayed onto a sterile filter paper (Whatman no. 3: 9 cm diameter). One milliliter methanol was used as a control. After being dried under a fume hood for 10 min at 25°C, two filter papers were placed on the bottom of a sterile plastic box (14.6 × 8.5 × 5.0 cm). Fifteen fourth instar larvae of S. litura were introduced on the plastic box and fed with the artificial diet after 24 h. Each treatment was replicated six times. The treated larvae were maintained at 25°C in the dark. The diets were renewed and the larval mortality was observed every 24 h.
For the feeding toxicity assay, the fourth instar S. litura larvae were fed with 11 different types of diets containing three concentrations of quercetin without R. terrigena, and three concentrations of quercetin with living or dead (autoclaved) R. terrigena. 0.05% dimethyl sulfoxide (DMSO) as a solvent and LB medium without bacteria and quercetin were used as controls. Fifty microliters 5 mg/ml, 25 mg/ml and 50 mg/ml quercetin were added to 940 μl LB medium, respectively, and 10 μl R. terrigena culture (OD600 = 0.6) or 10 μl LB medium were added to give quercetin concentrations of 0.25, 1.25 and 2.50 mg/ml, respectively. Inhibition zone experiment had shown that quercetin at 0.25, 1.25 and 2.50 mg/ml had no inhibitory effect on the growth of R. terrigena (Supplementary Figure S3). After shaken at 200 rpm and 37°C for 48 h, 4 ml bacterial culture containing different concentrations of quercetin were transferred, respectively, to 96 g artificial diets which were homogenously mixed and ready to feed on the insect larvae. Each treatment was replicated five times. The treated larvae were maintained at 25°C and a 12: 12 h L: D photoperiod. The diets which were uneaten were removed and replaced with fresh diet every 24 h. The developmental duration of each larval and pupal stage was recorded. All the pupae were weighed on the first day post pupation.
Results were presented as mean values with their standard deviation (SD). PROC Nonparametric Tests with the command ‘Sample K-S’ were used for the analysis of the normal distribution of data (SPSS17.0, SPSS Inc., Chicago, IL, United States). To determine if variables differed among treatments, data were analyzed by one-way ANOVA, using Tukey’s honestly significant difference test (p < 0.05) or t-tests for experiments with two treatments. GraphPad Prism 7.04 (GraphPad Software, Inc., United States) was used for creating scientific graphs.
More than 300 bacterial colonies including 9 bacterial species from the larval gut of T. xiaojinensis grew on the mineral agar medium containing 10% P. viviparum powder (Figure 1A), and 4 species including Raoultella terrigena (MW555198), Pantoea agglomerans (MW555193), Serratia plymuthica (MW555201) and Rahnella aquatilis (MW555197) on the mineral agar medium containing 25% P. viviparum powder. However, only R. terrigena normally proliferated in liquid mineral medium containing 25% P. viviparum powder.
Figure 1. Characterization of the bacteria subsisting on Polygonum viviparum. (A) Identified bacterial species from the gut of Thitarodes xiaojinensis larvae on the mineral medium (MM) containing 10% P. viviparum root powder and from surface sterilized P. viviparum roots or seeds on LB medium (Lucia-Bertani) agar plates, (B) Phylogenetic tree of R. terrigena based on 16S rRNA by using the Neighbor-Joining method and 1,000 bootstrap replicates in MEGA 5.0 software, (C) Morphology of R. terrigena on MM containing 25% P. viviparum and LB after 24 h of culture, and a gram stain (×100).
Dozens of colonies comprising 5 bacterial species were obtained from bud and root tissues of the surface sterilized P. viviparum seeds. R. terrigena was also detected in bud and root tissues of P. viviparum (Figure 1A). The isolated R. terrigena strain B49 shared 100% identity to R. terrigena strains JH01 and NCTC9189, and clustered into a clade with other species of Raoultella (Figure 1B).
The isolated R. terrigena was cultured on mineral medium containing 25% P. viviparum and LB medium at 37°C for 24 h. The colonies were regular round, opaque and convex. The bacterial cells were rod-shaped, single or short chain arranged. The cell length and width were about (1 ~ 2) μm and 0.5 μm, respectively (Figure 1C). This bacterium was Gram-negative, and catalase, indole, nitrate reduction, oxidation and fermentation of glucose, starch hydrolysis and urease tests were all positive. Casein hydrolysis, citrate utilization, gelatin, hydrogen sulfide and phenylalanine deaminase tests were negative.
Polygonum viviparum exhibits inhibitory activity for the growth of S. litura larvae (Qiu and Han, 2016), which was confirmed in this study. The growth of the 4th instar S. litura larvae fed with the artificial diet containing 5, 15 and 25% P. viviparum root powder was significantly hampered, compared with those fed with the artificial diet without P. viviparum. Surprisingly, the growth was significantly improved when the artificial diet contained P. viviparum root powder cocultured with R. terrigena (Figure 2). Notably, the percentages of the 6th instar larvae at day 4 (Figure 2A), pupae at day 12 (Figure 2B) and adults at day 30 (Figure 2C) in six treatments with R. terrigena-fermented P. viviparum root powder were all significantly higher than those without R. terrigena. The final adult emergence rate at day 34 in the control without P. viviparum in the artificial medium was significantly higher than those fed with the artificial diet containing P. viviparum, no matter whether R. terrigena was present or not (Figure 2D).
Figure 2. Development of the fourth instar larvae of Spodoptera litura reared on the artificial diets supplemented with 5, 15 or 25% P. viviparum root powder which was cocultured with or without R. terrigena for 7 days. Percentages of different developmental stages of S. litura for 4 day (A), 12 day (B), 30 day (C) and 34 day (D), developmental durations of the different developmental stages of S. litura (E) and average pupal weight of S. litura on the first day post pupation (F).
The developmental durations of the 4th, 5th, 6th instar larvae and pupae were further compared in details among the artificial media with or without R. terrigena. No significant difference was observed in the developmental duration of the 4th instar larvae fed with different diets. However, for the 5th, 6th instar larvae and pupae, the developmental durations in the treatments without P. viviparum root powder were 3.2, 5.6 and 9.9 days, respectively, which were significantly shorter than those in the treatments containing different concentrations of P. viviparum powder. The presence of autoclaved or unautoclaved R. terrigena cultures containing three concentrations of P. viviparum in the diets significantly shortened the developmental durations of the 5th, 6th instar larvae and pupae of S. litura (Figure 2E). Furthermore, the average pupal weight in the control without P. viviparum in the artificial medium was significantly higher (0.29 g) than those fed with the artificial diet containing P. viviparum, no matter whether R. terrigena was present or not and the average pupal weight was significantly higher in the artificial diet mixed with autoclaved R. terrigena culture containing 15% P. viviparum powder or in the artificial diets mixed with unautoclaved R. terrigena culture containing 15% or 25% P. viviparum powder, compared with that in the diets without R. terrigena coculture (Figure 2F).
In all, these results indicated that the growth of S. litura with diets containing P. viviparum powder was improved by the presence of R. terrigena bacteria.
To investigate whether growth improvement of S. litura by R. terrigena with diets containing P. viviparum powder was caused by the reduced flavonoid content, the content of quercetin and gallic acid in P. viviparum powder with or without R. terrigena was analyzed by HPLC. The average concentration of quercetin in P. viviparum root powder was 76.70 mg/kg, but only 15.74 mg/kg in the culture with R. terrigena after 7 days, in which 79.48% quercetin were degraded (Figure 3A). Although the concentration of gallic acid was higher than that of quercetin in P. viviparum powder, no significant difference was observed in the content of gallic acid in P. viviparum powder between the cultures with or without R. terrigena (Figure 3B). These results showed that R. terrigena could degrade quercetin but not gallic acid in P. viviparum powder.
Figure 3. Quercetin degradation by R. terrigena in P. viviparum powder and in LB medium. Contents of quercetin (A) and gallic acid (B) analyzed by HPLC-DAD, in 25% P. viviparum root powder fermented with or without R. terrigena for 7 days, standard curve of quercetin added in the LB culture medium (C) and quercetin degradation in LB medium with or without R. terrigena cultured for 48 h (D), expression of the genes yynw1 and yynw3 in natural larvae of T. xiaojinensis (E). Electrophoresis of the products for the different templates used for RT-PCR of the genes yynw1 and yynw3. No product was detected for the total RNA indicating the RNA without contamination of DNA. A band of ~500 bp for yynw1 and A band of ~400 bp for yynw3 were obtained for both cDNA and DNA. (+) positive control, DNA product from R. terrigena. (−) non-template control.
Decreased concentration of quercetin by R. terrigena was further demonstrated in LB medium containing quercetin only. The supernatants from the bacterial culture with quercetin were colorless, while LB medium with quercetin but without R. terrigena were yellow (Supplementary Figure S4). According to the quantitative test, a standard curve of quercetin was established. The linear regression equation of concentration logarithm was y = 7.794 × −0.0026 and the correlation co-efficiency R was 0.9975 (Figure 3C). The reduction rate of quercetin by R. terrigena reached 85.56% (Figure 3D). Together with the isolated strain B49, R. terrigena ATC700372 (GDMCC1.452) also exhibited the ability of degrading quercetin. The supernatants from the R. terrigena ATC700372 bacterial culture with quercetin were also colorless (Supplementary Figure S5) and its quercetin reduction rate reached 75.80% (Supplementary Table S1). The results further indicated that R. terrigena could degrade quercetin.
To further determine the contribution of R. terrigena to quercetin degradation in T. xiaojinensis, the genes yynw1 and yynw3 with quercetinase activity were screened in R. terrigena and the expression of these two genes was investigated in T. xiaojinensis. The obtained sequences of yynw1 and yynw3 were listed in Supplementary Table S2. The results showed that R. terrigena possessed the genes yynw1 and yynw3. The expression of the genes yynw1 and yynw3 was also confirmed by using reverse transcriptase PCR from RNA extracts of field-collected larvae (Figure 3E), indicating a contribution of R. terrigena to the process of quercetin degradation in natural populations of T. xiaojinensis.
Growth improvement of S. litura by R. terrigena was further confirmed in diets containing quercetin at three concentrations (1, 5 and 10 mg/ml). Quercetin at three concentrations showed no toxicity against S. litura in filter papers, as larval mortality, pupation rate, emergence rate (Figure 4A) and pupal weight (Figure 4B) of S. litura were not significantly different from those in the negative and methanol solvent control. However, the results from the feeding toxicity assay showed inhibitory effect of quercetin on the growth of S. litura. The 4th instar larvae fed with the artificial diets, respectively, with 0.01, 0.05 and 0.1 mg/g quercetin exhibited significantly longer developmental durations for later larvae, prepupa and pupa than those fed with the artificial diet without quercetin (Figure 4C). Furthermore, the larval survival rate, adult emergence rate (Figure 4D) and pupal weight (Figure 4E) significantly decreased in the diets containing quercetin at three concentrations.
Figure 4. Toxicity of quercetin against S. litura. Effects of quercetin on larval mortality, pupation rate, adult emergence rate (A) and average pupal weight (B) of S. litura using filter paper diffusion assay, developmental durations of the different developmental stages of S. litura (C), percentages of survival larvae and adult emergence (D), and average pupal weight (E) of S. litura reared on the artificial diets containing 0.01, 0.05 or 0.1 mg/g quercetin which was cocultured with or without R. terrigena for 48 h.
When the artificial diet was mixed with quercetin at 0.1 mg/g degraded by R. terrigena and used to feed on S. litura 4th instar larvae, insect growth was improved, including shortened larval, prepupal, pupal durations and increased larval survival rate, adult emergence rate as well as average pupal weight, no matter if the cultures of quercetin and R. terrigena were autoclaved or not (Figure 4). Likewise, larval, prepupal and pupal durations were significantly shortened with quercetin at both 0.05 mg/g and 0.1 mg/g (Figure 4C). Both adult emergence rate (Figure 4D) and average pupal weight (Figure 4E) were significantly increased although larval survival rate was not improved when S. litura 4th instar larvae were fed with the diets containing quercetin at 0.05 and 0.1 mg/g.
Taken together, these results further confirmed that quercetin in P. viviparum root powder was involved in the growth hamper of S. litura and co-culture of quercetin and gut bacterium R. terrigena could relieve the growth hamper.
Herbivorous insect microbiota has been shown to mediate the outcome of insect-plant interaction in different ways, such as essential nutrient supplementation, xenobiotic compounds degradation and plant defense suppression (Douglas, 2009; Kohl and Dearing, 2012; Cheng et al., 2017; Shukla and Beran, 2020). Microbiota of some herbivorous insects have been demonstrated to contribute to the detoxification of plant secondary metabolites (Kohl et al., 2014; Ceja-Navarro et al., 2015; Berasategui et al., 2017), however, bacterial involvement of quercetin degradation in plants as insect food remain unclear. This study demonstrated that isolated gut bacterium R. terrigena from T. xiaojinensis larvae are involved in the degradation of quercetin in P. viviparum root powder to relieve the growth suppression of herbivorous insects such as S. litura and T. xiaojinensis.
Nine bacterial species were isolated from the guts of field species of T. xiaojinensis on mineral medium containing 10% P. viviparum as the sole source of carbon and nitrogen, but only R. terrigena could normally multiply in liquid mineral medium containing 25% P. viviparum. R. terrigena was also detected from the sterilized bud and root tissues of P. viviparum, indicating R. terrigena as a gut bacterium from T. xiaojinensis larvae and an endophyte bacterium in P. viviparum.
Microbial communities from Thitarodes larvae have been investigated using traditional culture-dependent and culture-independent methods. Eight bacterial genera (Staphylococcus, Bacillus, Klebsiella, Pseudomonas, Aeromonas, Plesiomonas, Sporosarcina and Neisseria) are isolated from the guts of wild T. gonggaensis larvae (Zhuo et al., 2004). Eight bacterial genera (Enterobacter, Carnobacterium, Novosphingobium, Acinetobacter, Pseudomonas, Klebsiella, Pantoea and Delftia are also obtained from the guts of wild T. gonggaensis larvae (Liu et al., 2008). Several fungal genera (Mortierella, Trichosporon, Mucor, Rhinocladiella, Cephalosporium, Rhodiola and Mastigobasidium) are identified by RFLP analysis from this ghost moth species, and Cryptococcus magnus, Geomyces sp. and Trichosporon porosum by culture method (Yu et al., 2008). The microbiota of the hemolymph and gut from T. xiaojinensis larvae with or without injected O. sinensis blastospores are investigated by culture-dependent and-independent methods, and 25 culturable bacterial species and 14 fungal species, together with 537 bacterial OTUs and 218 fungal OTUs, are identified from the hemolymph and gut of samples from five infection stages (Wu et al., 2020). However, R. terrigena has not been obtained from all these reports. This may reflect the differences in sampling locations, isolation methods and larval culture methods. R. terrigena isolated from the three wild-collected Thitarodes population but not from the three corresponding artificial rearing population further indicated the difference of larval gut microbiota caused by sample source (Liu et al., 2021).
Endophytes as a ubiquitous associate of the plant are considered to play a pivotal role in regulating the primary and secondary metabolism of their host plant, and in providing elicitors to improve plant health, ameliorating stress tolerance in plants and source of therapeutically important secondary metabolites (Pandey et al., 2022). R. terrigena is considered to be an environmental bacterium, which is isolated mainly from soil and aquatic environments (Fazal et al., 2019). It is also isolated from plant roots of Nicotiana tabacum and switchgrass Panicum virgatum, and considered to be an endophytic nitrogen-fixing bacterium (Schicklberger et al., 2015; Gushgari-Doyle et al., 2021). Furthermore, R. terrigena is detected from the gut of Dendroctonus rhizophagus and D. valens, exhibiting high acetylene reduction activity (Morales-Jimenez et al., 2013), as well as from the reproductive system of the female oriental fruit fly Bactrocera dorsalis, and its metabolites are found to be attractive to adults in field trapping assays (Shi et al., 2012). Surprisingly, in this study, R. terrigena was firstly verified to effectively degrade quercetin in P. viviparum roots, which are natural food for Thitarodes larvae, and to improve S. litura growth when the larval medium contained P. viviparum root powder or quercetin. Because no artificial medium is available for Thitarodes larvae, whether the quercetin carried by P. viviparum roots is toxic to Thitarodes larvae is not validated. Why this endophyte bacterium from P. viviparum metabolizes quercetin inside the plant for the improved herbivorous insect growth needs further exploration.
Quercetin is considered to be a kind of flavonoid widely present in terrestrial plants and herbal medicines which helps plants resist herbivore attack (Simmonds, 2003; Selin-Rani et al., 2016; Shi et al., 2021), and induces expressions of some detoxification enzymes in insects (Zhang et al., 2012; Shi et al., 2021). Quercetin is assumed to be an ecofriendly alternative compound not only for S. litura but also for Lymantria dispar, Helicoverpa zea, Oedaleus asiaticus, Aphis spp. and Callosobruchus chinensis (Lattanzio et al., 2000; Salunke et al., 2005; Selin-Rani et al., 2016; Cui et al., 2019). The synergistic effect of quercetin and nucleopolyhedrovirus was also found (Shi et al., 2021). However, quercetin metabolism by microbes is still unclear (Aboshi et al., 2014). In this study, quercetin degradation by R. terrigena was discovered, which provides new techniques for mass production of Thitarodes larvae by employing this bacterium onto P. viviparum roots and seeds, and for the control of insect pest by inhibiting this gut bacterium when the pests feed on the plants full of quercetin.
Polygonum viviparum roots are toxic to S. litura, but favorable food for T. xiaojinensis larvae in the Himalaya Mountain. R. terrigena was firstly isolated from the gut of T. xiaojinensis larvae and P. viviparum roots and seeds. This bacterium grew in liquid mineral medium containing 25% P. viviparum root powder. The artificial medium containing 5, 15 and 25% P. viviparum powder showed significant growth inhibition of S. litura, but the growth was significantly improved when R. terrigena was present. Quercetin in P. viviparum powder was found to involve in the suppressed growth of S. litura. Quercetin degradation by R. terrigena was demonstrated to reduce the growth suppression of S. litura. These results provide insight into the detoxification of gut microbes from insects in the interaction between herbivores and defensed host plants.
The datasets presented in this study can be found in online repositories. The names of the repository/repositories and accession number(s) can be found at: GenBank accession number MW555198.1, https://www.ncbi.nlm.nih.gov/nuccore/MW555198.1/.
GL and RH designed the research and wrote or revised the manuscript. GL and LC conducted the research. All authors contributed to the article and approved the submitted version.
This study was supported by Guangdong Basic and Applied Basic Research Foundation (2021A1515010520), Guangzhou Municipal Science and Technology Bureau (202102080238), Qinghai Science and Technology Major Project (2021-SF-A4-1) and GDAS Special Project of Science and Technology Development (2020GDASYL-20200103097 and 2022GDASZH-2022010106).
The authors declare that the research was conducted in the absence of any commercial or financial relationships that could be construed as a potential conflict of interest.
All claims expressed in this article are solely those of the authors and do not necessarily represent those of their affiliated organizations, or those of the publisher, the editors and the reviewers. Any product that may be evaluated in this article, or claim that may be made by its manufacturer, is not guaranteed or endorsed by the publisher.
The Supplementary material for this article can be found online at: https://www.frontiersin.org/articles/10.3389/fmicb.2022.1079550/full#supplementary-material
Aboshi, T., Ishida, M., Matsushita, K., Hirano, Y., Nishida, R., and Mori, N. (2014). Stage-specific quercetin sulfation in the gut of Mythimna separata larvae (Lepidoptera: Noctuidae). Biosci. Biotechnol. Biochem. 78, 38–40. doi: 10.1080/09168451.2014.877835
Adams, M., and Jia, Z. (2005). Structural and biochemical analysis reveal pirins to possess quercetinase activity. J. Biol. Chem. 280, 28675–28682. doi: 10.1074/jbc.M501034200
Ali, H., Muhammad, A., Islam, S. U., Islam, W., and Hou, Y. (2018). A novel bacterial symbiont association in the hispid beetle, Octodonta nipae (Coleoptera: Chrysomelidae), their dynamics and phylogeny. Microb. Pathog. 118, 378–386. doi: 10.1016/j.micpath.2018.03.046
Ali, H., Muhammad, A., Sanda, N. B., Huang, Y., and Hou, Y. (2019). Pyrosequencing uncovers a shift in bacterial communities across life stages of Octodonta nipae (Coleoptera: Chrysomelidae). Front. Microbiol. 10:466. doi: 10.3389/fmicb.2019.00466
Beran, F., and Gershenzon, J. (2016). Microbes matter: herbivore gut endosymbionts play a role in breakdown of host plant toxins. Environ. Microbiol. 18, 1306–1307. doi: 10.1111/1462-2920.13258
Berasategui, A., Salem, H., Paetz, C., Santoro, M., Gershenzon, J., Kaltenpoth, M., et al. (2017). Gut microbiota of the pine weevil degrades conifer diterpenes and increases insect fitness. Mol. Ecol. 26, 4099–4110. doi: 10.1111/mec.14186
Braune, A., and Blaut, M. (2016). Bacterial species involved in the conversion of dietary flavonoids in the human gut. Gut. Microbes. 7, 216–234. doi: 10.1080/19490976.2016.1158395
Buchanan, R.E., and Gibbons, N.E. (1984). Bergey's Mannual of Determinative Bacteriology. 8th Edn. Science Press, Beijing.
Ceja-Navarro, J. A., Vega, F. E., Karaoz, U., Hao, Z., Jenkins, S., Lim, H. C., et al. (2015). Gut microbiota mediate caffeine detoxification in the primary insect pest of coffee. Nat. Commun. 6:7618. doi: 10.1038/ncomms8618
Cheng, D. F., Guo, Z. J., Riegler, M., Xi, Z. Y., Liang, G. W., and Xu, Y. J. (2017). Gut symbiont enhances insecticide resistance in a significant pest, the oriental fruit fly Bactrocera dorsalis (Hendel). Microbiome 5:13. doi: 10.1186/s40168-017-0236-z
Cui, B. Y., Huang, X. B., Li, S., Hao, K., Chang, B. H., Tu, X. B., et al. (2019). Quercetin affects the growth and development of the grasshopper Oedaleus asiaticus (Orthoptera: Acrididae). J. Econ. Entomol. 112, 1175–1182. doi: 10.1093/jee/toz050
Dong, X.Z., and Cai, M.Y. (2001). Manual for Identification of Common Bacterial Systems. Science, Beijing.
Douglas, A. E. (2009). The microbial dimension in insect nutritional ecology. Funct. Ecol. 23, 38–47. doi: 10.1111/j.1365-2435.2008.01442.x
Elliger, C. A., Wong, Y., Chan, B. G., and Waiss, A. C. (1981). Growth-inhibitors in tomato (Lycopersicon) to tomato fruitworm (Heliothis zea). J. Chem. Ecol. 7, 753–758. doi: 10.1007/BF00990307
Fazal, M. A., Alexander, S., Grayson, N. E., Deheer-Graham, A., Oliver, K., Holroyd, N., et al. (2019). Complete whole-genome sequences of two Raoultella terrigena strains, NCTC 13097 and NCTC 13098, isolated from human cases. Microbiol. Resour. Ann. 8:e00239-19. doi: 10.1128/MRA.00239-19
Francoeur, C. B., Khadempour, L., Moreira-Soto, R. D., Gotting, K., Book, A. J., Pinto-Tomás, A. A., et al. (2020). Bacteria contribute to plant secondary compound degradation in a generalist herbivore system. mBio 11:e02146-20. doi: 10.1128/mBio.02146-20
Gushgari-Doyle, S., Schicklberger, M., Li, Y. V., Walker, R., and Chakraborty, R. (2021). Plant growth promotion diversity in switchgrass-colonizing, diazotrophic endophytes. Front. Microbiol. 12:730440. doi: 10.3389/fmicb.2021.730440
Han, R. C., Wu, H., Tao, H. P., Qiu, X. H., Liu, G. Q., Rao, Z. C., et al. (2019). Research on Chinese cordyceps during the past 70 years in China. Chin. J. Appl. Entomol. 56, 849–883. doi: 10.7679/j.issn.2095/1353.2019.099
Itoh, H., Tago, K., Hayatsu, M., and Kikuchi, Y. (2018). Detoxifying symbiosis: microbe-mediated detoxification of phytotoxins and pesticides in insects. Nat. Prod. Rep. 35, 434–454. doi: 10.1039/c7np00051k
Juan, M. Y., and Chou, C. C. (2010). Enhancement of antioxidant activity, total phenolic and flavonoid content of black soybeans by solid state fermentation with Bacillus subtilis BCRC 14715. Food Microbiol. 27, 586–591. doi: 10.1016/j.fm.2009.11.002
Khan, K. A., Al-Ghamdi, A. A., Ghramh, H. A., Ansari, M. J., Ali, H., Alamri, S. A., et al. (2020). Structural diversity and functional variability of gut microbial communities associated with honey bees. Microb. Pathog. 138:103793. doi: 10.1016/j.micpath.2019.103793
Kohl, K. D., and Dearing, M. D. (2012). Experience matters: prior exposure to plant toxins enhances diversity of gut microbes in herbivores. Ecol. Lett. 15, 1008–1015. doi: 10.1111/j.1461-0248.2012.01822.x
Kohl, K. D., Weiss, R. B., Cox, J., Dale, C., and Dearing, M. D. (2014). Gut microbes of mammalian herbivores facilitate intake of plant toxins. Ecol. Lett. 17, 1238–1246. doi: 10.1111/ele.12329
Lattanzio, V., Arpaia, S., Cardinali, A., Di Venere, D., and Linsalata, V. (2000). Role of endogenous flavonoids in resistance mechanism of Vigna to aphids. J. Agr. Food Chem. 48, 5316–5320. doi: 10.1021/jf000229y
Li, X., Schuler, M. A., and Berenbaum, M. R. (2007). Molecular mechanisms of metabolic resistance to synthetic and natural xenobiotics. Annu. Rev. Entomol. 52, 231–253. doi: 10.1146/annurev.ento.51.110104.151104
Li, Y. Z., and Shen, S. D. (1996). A preliminary morphological and anatomical investigation in the vegetative and reproductive organs of Polygonum viviparum. J. Qinghai Norm Univ. 1, 34–41. doi: 10.1146/10.16229/j.cnki.issn1001-7542.1996.01.011
Li, G., Shen, H. M., and Zhang, X. H. (2009). Preliminary research of insecticidal activity of acetone extracts from sixteen species of poisonous plants in the Tianzhu grassland of Gansu Province to Mythimna seperata. J Gansu Agr. Univ. 44, 101–104. doi: 10.13432/j.cnki.jgsau.2009.02.033
Li, J. A., Wang, L., and Yang, T. S. (2014). Simultaneous determination of gallic acid, chlorogenic acid and quercetin in Polygonum viviparum L. by HPLC. J. Ningxia Normal Univ. 35, 74–81.
Liu, L., Wang, Z. K., Yu, H. W., Chen, S. J., Yan, G. F., Xia, Y. X., et al. (2008). Analysis of the bacterial diversity in intestines of Hepialus gonggaensis larvae. Acta Microbiol. Sinica 48, 616–622. doi: 10.13343/j.cnki.wsxb.2008.05.004
Liu, G. Q., Zheng, X. H., Long, H., Rao, Z. C., Cao, L., and Han, R. C. (2021). Gut bacterial and fungal communities of the wild and laboratory-reared Thitarodes larvae, host of the Chinese medicinal fungus Ophiocordyceps sinensis on Tibetan plateau. Insects 12:327. doi: 10.3390/insects12040327
Mithöefer, A., and Boland, W. (2012). Plant defense against herbivores: chemical aspects. Annu. Rev. Plant Biol. 63, 431–450. doi: 10.1146/annurev-arplant-042110-103854
Morales-Jimenez, J., Vera-Ponce De Leon, A., Garcia-Dominguez, A., Martinez-Romero, E., Zuniga, G., and Hernandez-Rodriguez, C. (2013). Nitrogen-fixing and uricolytic bacteria associated with the gut of Dendroctonus rhizophagus and Dendroctonus valens (Curculionidae: Scolytinae). Microb. Ecol. 66, 200–210. doi: 10.1007/s00248-013-0206-3
Nyman, T., and Julkunen-Tiitto, R. (2000). Manipulation of the phenolic chemistry of willows by gall-inducing sawflies. Proc. Natl. Acad. Sci. U. S. A. 97, 13184–13187. doi: 10.1073/pnas.230294097
Pandey, S. S., Jain, R., Bhardwaj, P., Thakur, A., Kumari, M., Bhushan, S., et al. (2022). Plant probiotics - endophytes pivotal to plant health. Microbiol. Res. 263:127148. doi: 10.1016/j.micres.2022.127148
Qiu, X., and Han, R. (2016). The effect of Polygonum viviparum on the development of Plutella xylostella and Spodoptera litura. J. Environ. Entomol. 38, 54–60. doi: 10.3969/j.issn.1674-0858.2016.01.7
Salunke, B. K., Kotkar, H. M., Mendki, P. S., Upasani, S. M., and Maheshwari, V. L. (2005). Efficacy of flavonoids in controlling Callosobruchus chinensis (L.) (Coleoptera: Bruchidae), a post-harvest pest of grain legumes. Crop Prot. 24, 888–893. doi: 10.1016/j.cropro.2005.01.013
Schicklberger, M., Shapiro, N., Loque, D., Woyke, T., and Chakraborty, R. (2015). Draft genome sequence of Raoultella terrigena R1Gly, a diazotrophic endophyte. Genome Announc. 3:e00607-15. doi: 10.1128/genomeA.00607-15
Schnarr, L., Segatto, M. L., Olsson, O., Zuin, V. G., and Kummerer, K. (2022). Flavonoids as biopesticides - systematic assessment of sources, structures, activities and environmental fate. Sci. Total Environ. 824:153781. doi: 10.1016/j.scitotenv.2022.153781
Schramm, K., Vassao, D. G., Reichelt, M., Gershenzon, J., and Wittstock, U. (2012). Metabolism of glucosinolate-derived isothiocyanates to glutathione conjugates in generalist lepidopteran herbivores. Insect Biochem. Mol. Biol. 42, 174–182. doi: 10.1016/j.ibmb.2011.12.002
Selin-Rani, S., Senthil-Nathan, S., Thanigaivel, A., Vasantha-Srinivasan, P., Edwin, E. S., Ponsankar, A., et al. (2016). Toxicity and physiological effect of quercetin on generalist herbivore, Spodoptera litura fab. And a non-target earthworm Eisenia fetida Savigny. Chemosphere 165, 257–267. doi: 10.1016/j.chemosphere.2016.08.136
Shi, G., Kang, Z., Liu, H., Ren, F., and Zhou, Y. (2021). The effects of quercetin combined with nucleopolyhedrovirus on the growth and immune response in the silkworm (Bombyx mori). Arch. Insect Biochem. Physiol. 108:e21839. doi: 10.1002/arch.21839
Shi, Z. H., Wang, L. L., and Zhang, H. Y. (2012). Low diversity bacterial community and the trapping activity of metabolites from cultivable bacteria species in the female reproductive system of the oriental fruit fly, Bactrocera dorsalis Hendel (Diptera: Tephritidae). Int. J. Mol. Sci. 13, 6266–6278. doi: 10.3390/ijms13056266
Shukla, S. P., and Beran, F. (2020). Gut microbiota degrades toxic isothiocyanates in a flea beetle pest. Mol. Ecol. 29, 4692–4705. doi: 10.1111/mec.15657
Simmonds, M. S. J. (2003). Flavonoid–insect interactions: recent advances in our knowledge. Phytochemistry 64, 21–30. doi: 10.1016/s0031-9422(03)00293-0
Sung, G. H., Hywel-Jones, N. L., Sung, J. M., Luangsa-Ard, J. J., Shrestha, B., and Spatafora, J. W. (2007). Phylogenetic classification of Cordyceps and the clavicipitaceous fungi. Stud. Mycol. 57, 5–59. doi: 10.3114/sim.2007.57.01
Ververidis, F., Trantas, E., Douglas, C., Vollmer, G., Kretzschmar, G., and Panopoulos, N. (2007). Biotechnology of flavonoids and other phenylpropanoid-derived natural products. Part I: chemical diversity, impacts on plant biology and human health. Biotechnol. J. 2, 1214–1234. doi: 10.1002/biot.200700084
Wu, H., Rao, Z., Cao, L., De Clercq, P., and Han, R. (2020). Infection of Ophiocordyceps sinensis fungus causes dramatic changes in the microbiota of its Thitarodes host. Front. Microbiol. 11:577268. doi: 10.3389/fmicb.2020.577268
Yu, H. W., Wang, Z. K., Liu, L., Xia, Y. X., Yin, Y. P., Yuan, Q., et al. (2008). Analysis of fungal diversity in intestines of Hepialus gonggaensis larvae. Acta Microbiol. Sinica 48, 439–445. doi: 10.13343/j.cnki.wsxb.2008.04.003
Zaynab, M., Fatima, M., Sharif, Y., Zafar, M. H., Ali, H., and Khan, K. A. (2019). Role of primary metabolites in plant defense against pathogens. Microb. Pathog. 137:103728. doi: 10.1016/j.micpath.2019.103728
Zeng, W. A., Zhou, G. S., Deng, Z. P., Cheng, J. P., Shi, X. B., Huang, Y. N., et al. (2008). Polygonum hydropiper L. a pesticide plant of Polygonaceae. Chin. Agr. Sci. Bull. 24, 450–452.
Zhang, M. X., Fang, T. T., Pu, G. L., Sun, X. Q., Zhou, X. G., and Cai, Q. N. (2013). Xenobiotic metabolism of plant secondary compounds in the English grain aphid, Sitobion avenae (F.) (Hemiptera: Aphididae). Pestic. Biochem. Phys. 107, 44–49. doi: 10.1016/j.pestbp.2013.05.002
Zhang, C. X., Li, Y. L., and Zu, H. F. (2005). Studies on the chemical constituents from herba Polygonum viviparum L. Nat. Prod. Res. Dev. 17, 177–178. doi: 10.16333/j.1001-6880.2005.02.015
Zhang, Y. E., Ma, H. J., Feng, D. D., Lai, X. F., Chen, Z. M., Xu, M. Y., et al. (2012). Induction of detoxification enzymes by quercetin in the silkworm. J. Econ. Entomol. 105, 1034–1042. doi: 10.1603/ec11287
Zhang, Z. C., Peng, X. C., Li, S. T., Zhang, N., Wang, Y., and Wei, H. (2014). Isolation and identification of quercetin degrading bacteria from human fecal microbes. PLoS One 9:e90531. doi: 10.1371/journal.pone.0090531
Zhou, X. W., Li, L. J., and Tian, E. W. (2014). Advances in research of the artificial cultivation of Ophiocordyceps sinensis in China. Crit. Rev. Biotechnol. 34, 233–243. doi: 10.3109/07388551.2013.791245
Zhuo, F. P., Chen, S. J., Yin, Y. P., Wang, Z. K., and Xia, Y. X. (2004). Analysis on the Hepialus gonggaensis's intestinal bacterial flora. J. Chongqing Univ. 27, 26–29.
Keywords: Spodoptera litura, Thitarodes xiaojinensis, Raoultella terrigena, herbivory, plant secondary metabolites, adaptation, microbiota, detoxification
Citation: Liu G, Cao L and Han R (2022) Plant quercetin degradation by gut bacterium Raoultella terrigena of ghost moth Thitarodes xiaojinensis. Front. Microbiol. 13:1079550. doi: 10.3389/fmicb.2022.1079550
Received: 25 October 2022; Accepted: 07 December 2022;
Published: 22 December 2022.
Edited by:
Yijuan Xu, South China Agricultural University, ChinaReviewed by:
Habib Ali, Khwaja Fareed University of Engineering and Information Technology (KFUEIT), PakistanCopyright © 2022 Liu, Cao and Han. This is an open-access article distributed under the terms of the Creative Commons Attribution License (CC BY). The use, distribution or reproduction in other forums is permitted, provided the original author(s) and the copyright owner(s) are credited and that the original publication in this journal is cited, in accordance with accepted academic practice. No use, distribution or reproduction is permitted which does not comply with these terms.
*Correspondence: Richou Han, aGFucmNAZ2l6LmdkLmNu
Disclaimer: All claims expressed in this article are solely those of the authors and do not necessarily represent those of their affiliated organizations, or those of the publisher, the editors and the reviewers. Any product that may be evaluated in this article or claim that may be made by its manufacturer is not guaranteed or endorsed by the publisher.
Research integrity at Frontiers
Learn more about the work of our research integrity team to safeguard the quality of each article we publish.