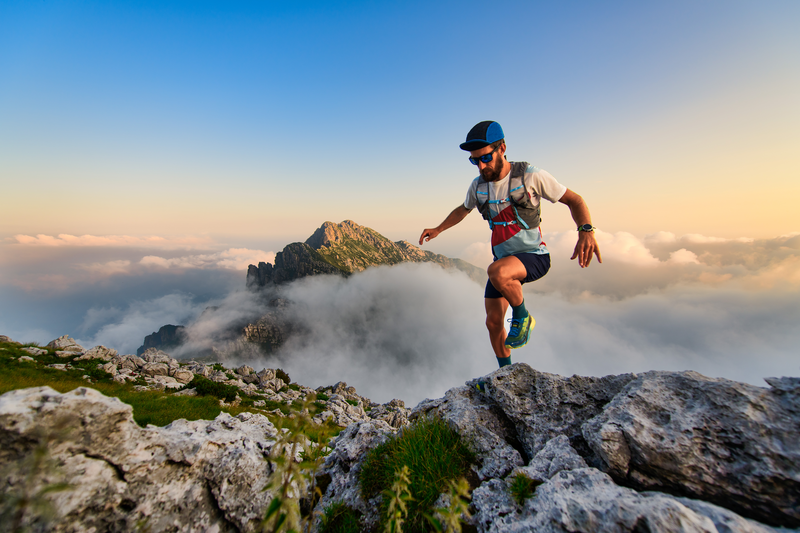
95% of researchers rate our articles as excellent or good
Learn more about the work of our research integrity team to safeguard the quality of each article we publish.
Find out more
MINI REVIEW article
Front. Microbiol. , 29 November 2022
Sec. Aquatic Microbiology
Volume 13 - 2022 | https://doi.org/10.3389/fmicb.2022.1063594
This article is part of the Research Topic Women in Aquatic Microbiology: 2022 View all 9 articles
Pit lakes are one of the greatest legacies of open-cut mining. Despite the potential hazards of these lakes, they represent newly formed ecosystems with great scientific and ecological potential. Although thousands of pit lakes occur on every inhabited continent, with more being created, the microbial ecology of pit lakes is relatively under-researched. We evaluated the current state of microbial research in pit lakes by performing a Web of Science search and creating a literature database. Study lakes were categorized according to location and water quality (pH and conductivity) which is a key community and environmental concern. Research technology employed in the study was also categorized. We compared research effort in lakes, rivers, and streams which are the more “charismatic” inland aquatic ecosystems. Pit lake publications on microbes from 1987 to 2022 (n = 128) were underrepresented in the literature relative to rivers and streams (n = 321) and natural lakes (n = 948). Of the 128 pit lake publications, 28 were within the field of geochemistry using indirect measures of microbial activity. Most pit lake microbial research was conducted in a few acidic lakes in Germany due to social pressure for remediation and government initiative. Relatively few studies have capitalized on emerging technology. Pit lake microbial research likely lags other more charismatic ecosystems given that they are viewed as performing “ecosystem disservices,” but this is socially complex and requires further research. Improving understanding of microbial dynamics in pit lakes will allow scientists to deliver safer pit lakes to communities.
Mining is the backbone of civilization (Younger et al., 2002; Coulson, 2012), and mine-pit lakes (“pit lakes”) are created when open-cut mines are flooded with water at the end of mining operations. When first formed, pit lakes are often low in biodiversity and nutrients, spatially homogeneous, lack higher trophic levels, and contain little organic matter (Lund et al., 2020). Pit lakes may have poor water quality as a result of groundwater and surrounding geology (e.g., Younger and Wolkersdorfer, 2004; Falagán et al., 2014; Soni et al., 2014; Marszelewski et al., 2017; Sakellari et al., 2021). Poor water quality has caused public alarm (Woodbury, 1998; Kean, 2009; Robbins, 2016), although where pit lakes have been actively rehabilitated, they are spectacular examples of recreation and cultural renewal (Weber, 2020).
Charismatic ecosystems tend to receive more scientific and public attention (Duarte et al., 2008), and pit lakes are often perceived as non-charismatic waterbodies responsible for “ecosystem disservices” (e.g., Zeide, 1998; von Döhren and Haase, 2015; Blanco et al., 2019) mainly related to poor water quality and physical safety of the site (McCullough and Lund, 2006; Graupner, 2009; Mantero et al., 2020; Newman et al., 2020; Sánchez-España et al., 2020b; Rönicke et al., 2021). However, every pit lake is different, and if well managed and designed, some pit lakes could represent a “transitional ecosystem disservice” (Saunders and Luck, 2016) with future benefits for communities and the environment in terms of recreation, conservation, and industry (Blanchette and Lund, 2016; Palit and Kar, 2019; Williams et al., 2020). Pit lakes also provide scientists a platform from which to discover new species, metabolic functions, biogeochemical processes, and interactions between and within trophic levels, often in extreme environments (e.g., Falagán et al., 2014, 2017a; Falagán and Johnson, 2014, 2016). However, factors such as the environmental stigma surrounding pit lakes, a lack of interdisciplinary scientific collaboration, and unpublished data held by the mining industry have likely hindered scientific progress in the field of pit lake research relative to other inland aquatic ecosystems (Blanchette and Lund, 2020).
Although research on microbial taxonomic diversity in pit lakes has been limited, pit lakes host a diverse microbial community within their waters and sediments (Blanchette et al., 2020; Grettenberger et al., 2020; Blanchette and Lund, 2021; Xin et al., 2021). From our knowledge of other aquatic ecosystems, microbes will play a key role in pit lake ecosystem function through nutrient cycling, the fate and transport of metals and metalloids, mineral formation, biogeochemical processes, decomposition of organic matter, and interactions with other organisms (Oren, 2004; Pace et al., 2016; Gupta et al., 2017; Flemming and Wuertz, 2019; Morris et al., 2020). Despite the growing popularity of microbial diversity and function research in terrestrial and aquatic environments, less is known about the overall taxonomic and functional diversity of the microbial community and their roles in pit lakes.
This mini review synthesizes the current state-of-the-art on prokaryotic microbial research in pit lakes via a literature review and analysis. We also provide metrics on other inland water bodies (rivers and naturally formed lakes) and categorize the pit lakes in terms of pH and conductivity because water quality is a key concern for communities. We provide a synthesis on the most-researched lakes and technologies employed and how to advance the field. This research is important because understanding microbial function is critical for restoring and providing ecosystem services (Singh et al., 2019; Dutta and Sen, 2021). Improving the scope of microbial research in pit lakes will allow scientists to manage risks and provide benefits to catchments and communities.
To determine the number of pit lake papers investigating microbes, a data set was created using Web of Science (WOS).1 Two broad pit lake literature searches were conducted on August 31, 2022 “Across all the databases” searching the “Topic field.” The first search method used a combination of 16 terms (i.e., “pit lake” AND “bacteria”): “pit lake (s),” “mine (lake (s)),” “Anthropocene (lake),” “(aquatic) ecosystem,” “bacteria,” “microbial community (ies),” “microbe (s),” “microbiome,” “16S,” “amplicon,” “sequencing,” “meteganomics,” “metaproteomics,” “metatrascriptomics,” “sulphate reducing bacteria,” and “SRB.” The second search method used the following searching strategy: (bacteria OR microbial community OR microbial communities OR microbe OR microbes OR microbiome OR 16S OR amplicon OR sequencing OR metagenomics OR metaproteomics OR metatrascriptomics OR sulphate reducing bacteria OR SRB OR microorganism) AND “pit lake” NOT (“crater lake” OR “bioreactor” OR “natural lake”). The second search method was conducted six times, changing the terms after the AND using the terms “pit lake (s)” (search one and two), “mining lake (s)” (search three and four), and “mine lake (ML) (s)” (search five and six). Additionally, if any papers were identified from reference lists of searched papers, they were added to the data set. Results from all searches were combined to form one data set (Supplementary Table 1). The dataset contains 221 data points extracted from 128 pit lakes microbial papers. Each data point refers to data extracted from each lake within a publication. Non-English papers, reviews, abstracts, meetings, books, and irrelevant papers were excluded from the data set. Tailing ponds were also excluded from the data set, although Base Mine Lake (BML, Alberta, Canada) was included. Compared to “common” tailing ponds, BML has a deeper freshwater and mine water “cap” (∼10 m vs. <5 m) over the tailings (Arriaga et al., 2019).
From each pit lake publication we extracted data related to the pit lake: country, lake name, latitude and longitude (decimal degrees), target resource, water pH, and conductivity. The research study was categorized in terms of broad microbial methodology (e.g., most probable number, sequencing) and type of sample collected (e.g., water, sediment, “iron snow”). Depending on the reported data, which may have been collected over time, environmental parameters such as pH and conductivity were extracted either as a single value or minimum and maximum values available. Pit lakes were classified according to pH as (a) acid (pH 1–6.4), (b) circumneutral (pH 6.5–7.5), (c) neutral (pH 7), or (d) alkaline (pH 7.6–14). Based on conductivity lakes were either (a) fresh (< 1499 uS/cm), (b) brackish (1500–1999 uS/cm), or (c) saline (> 2000 uS/cm). The salinity of most acidic pit lakes, particularly in the deeper anoxic layers, is predominantly caused by high concentration of SO42–), Ca, Mg, Al, and dissolved iron (Wollmann et al., 2000; Falagán et al., 2016) rather than sodium chloride (NaCl) characteristic of seawater or saline lakes. Conductivity and pH of pit lakes included in the data set were summarized using a Sankey diagram.2
In order to compare the relative number of pit lake microbe papers to research in other inland water bodies, three separate literature searches for microbial research in lakes, rivers, or streams was conducted in WOS broadly following the pit lake search format across “All Databases” searching the topic field: (1) (bacteria OR microbial community OR microbial communities OR microbe OR microbes OR microbiome OR 16S OR amplicon OR sequencing OR metagenomics OR metatranscriptomics OR sulphate reducing bacteria OR SRB) AND lake*, (2) (bacteria OR microbial community OR microbial communities OR microbe OR microbes OR microbiome OR 16S OR amplicon OR sequencing OR metagenomics OR metatranscriptomics OR sulphate reducing bacteria OR SRB) AND river*, and (3) (bacteria OR microbial community OR microbial communities OR microbe OR microbes OR microbiome OR 16S OR amplicon OR sequencing OR metagenomics OR metatranscriptomics OR sulphate reducing bacteria OR SRB) AND stream*. The search was limited to papers published between 1987 and 2022 corresponding to the period of pit lake microbial research and results were refined as above. The following terms were excluded from the searches: “pit lake,” “ML,” “mining lake,” quarry lake, “floodplain lake,” “reservoir,” “cave river,” “karst river,” “estuary,” and “delta.” Due to the large volume of global research on inland water bodies and different local names for these ecosystems, we limited our search to the terms “lake,” “river,” and “stream.” Web of Science research areas included: “Environmental Sciences Ecology,” “Biochemistry Molecular Biology,” “Marine Freshwater Biology,” “Microbiology,” Life Sciences Biomedicine Other,” “Microscopy,” “Biodiversity Conservation,” “Water Resources,” and “Biotechnology Applied Microbiology.”
Over the past 35 years (1987–2022) 687 papers on pit lakes have been published and 128 of these papers were microbial research (Supplementary Table 1). In the pit lake data base (n = 128 papers), 28 papers investigated geochemistry, which by nature inferred microbial activity through elemental and chemical analysis. Therefore, in the context of the search methodology, 100 published papers have directly measured microbial assemblage, activity, function, or density in pit lakes (Supplementary Table 1). The number of pit lake microbe papers per year between 1987 and 2022 ranged between zero and eight in 2021. During the same period, a greater number of papers on microbial research were published in rivers and streams (n = 321) and lakes (n = 948).
Our results support the idea that there is a positive relationship between research effort and ecosystem charisma (Duarte et al., 2008; He et al., 2021). Pit lake ecosystems are often associated with environmental stigma and “ecosystem disservices” such as poor water quality and visual disruptions in the landscape (Birkhofer et al., 2015; Lopez and Blanchette, 2020). It was surprising that research effort (measured in terms of “papers published”) conducted in rivers and streams was low relative to lakes, given that rivers were more often studied from 2000 to 2010 compared to lakes, wetlands, and ponds (Stendera et al., 2012). However, other researchers have found riverine microbe studies to be similarly underrepresented (de Oliveira and Margis, 2015), and aquatic microbes in general were underrepresented relative to other taxa such as fish and macroinvertebrates (Stendera et al., 2012). While determining the broad drivers of ecological research is outside the scope of this study, microbes may be considered non-charismatic taxa and therefore generally overlooked in terms of research (McGinlay et al., 2017).
In addition to a potentially biased perception of pit lake microbial ecology, the lack of published papers in this area may be due to more practical reasons. Pit lakes are created through mining, and “data loss” in industry reports or unpublished student research, as well as a lack of collaboration between industry and scientists of different disciplines are bottlenecks to research publication (Blanchette and Lund, 2020). Further, freshwater ecologists and microbiologists are not exposed to pit lake ecosystems (sensu Duarte et al., 2008; He et al., 2021), often because pit lake microbial research is published in “specialized” journals (e.g., Aquatic Geochemistry, Geobiology, Mine Water and the Environment, and Hydrobiologia) and thus unlikely to reach a broad microbiologist audience. However, given the advancement and adoption of new technology (e.g., “-omics”) and increased collaboration, microbial research in pit lakes is an emerging field with great potential.
Despite the presence of thousands of pit lakes on all inhabited continents (Castendyk and Eary, 2009), most pit lake microbial research (n = 128 papers, Supplementary Table 1), has been conducted in Germany (n = 60 papers) and Spain (n = 29 papers). Fewer studies were conducted in Canada (n = 13 papers), Australia (n = 7 papers), and the United States (n = 4 papers). The 128 microbial papers yielded “data points” (n = 221) from 99 pit lakes as different authors sampled the same pit lake (Supplementary Table 1). Of the 221 data points, 63 were extracted from German pit lakes, particularly ML 111 (n = 39), ML 77 (n = 14), and ML 117, (n = 10), followed by the Spanish Iberian Pyrite Belt (IPB) lakes Cueva de la Mora (n = 15) and Guadiana (n = 9).
Pit lake research in Germany and Spain has been driven by geography and water quality which is underpinned by political and social demand (Weber, 2020). In Germany, pit lake rehabilitation and research were national priorities after reunification (Benthaus et al., 2020). The nationally owned company Lausitzer und Mitteldeutsche Bergbau-Verwaltungsgesellschaft (LMBV) gained control of the flooded lignite mines in populated areas and was tasked with remediating East Germany’s acidic pit lakes (Benthaus et al., 2020), specifically Mine Lake 111 in Lusatia (East Germany) (Karakas et al., 2003; Kleinsteuber et al., 2008). The social, political, and environmental investments in German pit lake remediation have established and driven global pit lake research.
Although the microbiology of Spain’s pit lakes (e.g., Guadiana and Cueva de la Mora) are the second-most studied relative to Germany’s, they have undergone less research and remediation likely due in part to their remote locations (Sánchez-España, pers. comm.). Therefore, social pressure was less important in Spanish pit lake research than in Germany due to population density, with Spanish pit lake microbial research largely driven by the interests of scientists (Sánchez-España, pers. comm.). Further, many Spanish pit lakes are located on private active mine sites, meaning that unlike in Germany, the Spanish government had no broad legal authorization to conduct remediation or research, with some lakes eventually being drained and re-mined (Sánchez-España, pers. comm.). Mining is a key feature of the Anthropocene, and it follows that pit lake research would also be driven by social and population dynamics.
Most microbiological research in pit lakes has been conducted in acidic lakes (pH < 6.5; n = 179 data points), with fewer data points from alkaline (pH = > 7.5; n = 20), and circumneutral (pH = 6.5–7.5; n = 1) pit lakes (Figure 1). Acidic pit lakes form in abandoned coal, gold, base metal, and uranium pits due to a combination of factors such as the exposure of sulphides and minerals to water and oxygen (van der Graaf et al., 2020), an absence of neutralizing carbonates, groundwater chemistry, and the activity of sulphur and iron-oxidizing bacteria (e.g., Castro and Moore, 2000; Frömmichen et al., 2003; González-Toril et al., 2013). Often, acidic pit lakes are characterized by high concentrations of dissolved metals (e.g., iron and aluminium), sulphate (SO42–), and ammonium (NH4+), and low concentrations of nitrate, phosphorous, and organic carbon (Castro and Moore, 2000; Soni et al., 2014; Lund and McCullough, 2015). As a result, acidic pit lakes generally contain low levels of primary production and have microbial communities dominated by extremophiles involved in the cycling of sulphur and iron (Kamjunke et al., 2005; Fyson et al., 2006; Wendt-Potthoff et al., 2011).
Figure 1. Water quality (pH and conductivity) of 221 data points extracted from a Web of Science (WOS) literature search (n = 128 papers) for research on pit lake microbiology (Supplementary Table 1). Most data points (n = 179) were extracted from acidic pit lakes (pH < 6.4) vs. circumneutral, neutral, and alkaline pit lakes (pH > 6.5; n = 27 data points).
Acidic pit lakes and associated mines are considered hostile environments posing potential threats for wildlife and humans (McCullough and Lund, 2006; Hadjipanagiotou et al., 2020; Sergeant et al., 2022). Unsurprisingly, understanding and rehabilitating these lakes has become a priority for industry, government agencies and consequently researchers (Meier et al., 2004; Gammons and Icopini, 2020). Although abiotic approaches such as limestone addition and flooding with alkaline water have been used successfully (e.g., Neil et al., 2009; Benthaus et al., 2020), many rehabilitation strategies have focused on microbially mediated neutralization processes (e.g., Geller et al., 2009).
Pit lake microbial research has been dominated by laboratory studies conducted at micro- and mesocosm scales to determine what active interventions may improve pit lake water quality (e.g., Castro and Moore, 2000; Costa and Duarte, 2005; McCullough et al., 2006; Mccullough and Lund, 2008; Kumar et al., 2011; McCullough and Lund, 2011; Lund and McCullough, 2015; Falagán et al., 2017b). These remediation studies mainly focused on the presumed water quality effects of specific microbial groups and processes such as iron- or sulphur-reducing bacteria or dissimilatory reduction (Fauville et al., 2004; Costa and Duarte, 2005), rather than the microbial ecology of pit lakes.
Most data points (n = 124) did not report conductivity data for pit lake studied. The remaining 97 data points were predominantly saline (>2000 uS/cm, n = 60) (Figure 1). Saline lakes were also likely to be acidic (pH < 6.4) (Figure 1) and therefore salinity was probably due to high concentrations of SO42–, Fe (II), Ca, and Mg (e.g., Wollmann et al., 2000; Falagán et al., 2016) rather than NaCl. In contrast to acidic pit lakes, non-acidic (pH > 6.5) moderately saline pit lakes are often considered benign environments with low rehabilitation priority. Thus, like natural saline lakes (Williams, 1996), saline pit lakes are under-researched (Williams, 1996; Mori et al., 2019; Blanchette and Lund, 2021). Similar to acid pit lakes, saline pit lake rehabilitation strategies would focus on water quality improvement or minimization of pit outflows (Mehanna et al., 2010; Mouhamad et al., 2017; Nielsen, 2020). Natural saline lakes provide ecosystem services such as minerals, water, habitats, aquaculture, tourism, and recreational activities (Williams, 1996; Wurtsbaugh et al., 2017; Edwards and Null, 2019). Similar services may be found in saline pit lakes, although this may require changing public perception (Lopez and Blanchette, 2020; Rosa et al., 2020; Svobodova et al., 2021).
Until recently, pit lake microbial science was slow to capitalize on emerging technology (Supplementary Table 1). Many papers in our data base used most probable number to determine the concentration of specific microbial groups (Kumar et al., 2011; Wendt-Potthoff et al., 2011, Koschorreck and Wendt-Potthoff, 2012). Community composition was often determined using gradient gel electrophoresis (DGGE) or terminal restriction fragment length polymorphism (T-FRLP) which limit the detection of rare taxa (<1% of the total abundance) (Kampe et al., 2010; Falagán et al., 2013; González-Toril et al., 2013). Although only a few studies have been conducted on metatrascriptomics, metagenomics, and metaproteomics in pit lakes (White et al., 2015; Mori et al., 2019; Ayala-Muñoz et al., 2020, 2022; Sánchez-España et al., 2020a; Ayala-Muñoz et al., 2022) papers utilizing emerging technology have introduced the field of pit lake microbial research to the wider microbiological community.
Pit lakes provide a unique opportunity to investigate colonization, succession, temporal changes of newly formed ecosystems, and novel microbial metabolic pathways (Kampe et al., 2010; Marszelewski et al., 2017, Sánchez-España et al., 2018) (Figure 2). Like any ecosystem, pit lakes host a rare microbial biosphere that plays key roles in nutrient cycling, pollutant degradation, and protection from pathogens (Pascoal et al., 2021) and hosts unique extremophile organisms (Stierle et al., 2006). However, we found that pit lake microbial papers were underrepresented in the literature relative to rivers/streams and lakes. This may have been due to the correlation between research effort and ecosystem charisma given the environmental stigma and ecosystem disservices associated with pit lakes (real and perceived), or for more practical reasons given that pit lake research has been traditionally performed by industry. We also were unable to include non-English papers, which may have underrepresented actual research effort. Regardless, pit lake research has been driven by population and therefore social pressure for remediation in acidic lakes as seen in Germany, which has produced the bulk of papers on pit lake microbiology. Advancing the field of pit lake microbiology will require capitalizing on new technology and publishing in broad microbiological journals in order to promote interdisciplinary and international collaboration. With thousands of pit lakes on all inhabited continents, raising the profile of this research area will benefit communities and the environment.
Figure 2. Microbes can be used in pit lakes for environmental monitoring and identification of processes and taxa. Harnessing new technology will advance the field of pit lake microbiology. Symbols curtesy of Integration and Application Network (ian.umces.edu/symbols/) and NESP Resilient Landscapes Hub (nesplandscapes.edu.au).
All authors conceptualized, wrote the manuscript, contributed to the article, and approved the submitted version.
RB and MB were supported by the Australian Coal Association Research Program (ACARP). We thank to Javier Sánchez-España [Spanish Institute of Geology and Mining (IGME-CSIC) and National Centre Spanish Research Council (CSIC)] for conversations on the history of Spanish pit lake research. Jonas Polifka assisted with the literature search.
The authors declare that the research was conducted in the absence of any commercial or financial relationships that could be construed as a potential conflict of interest.
All claims expressed in this article are solely those of the authors and do not necessarily represent those of their affiliated organizations, or those of the publisher, the editors and the reviewers. Any product that may be evaluated in this article, or claim that may be made by its manufacturer, is not guaranteed or endorsed by the publisher.
The Supplementary Material for this article can be found online at: https://www.frontiersin.org/articles/10.3389/fmicb.2022.1063594/full#supplementary-material
Arriaga, D., Nelson, T. C., Risacher, F. F., Morris, P. K., Goad, C., Slater, G. F., et al. (2019). The co-importance of physical mixing and biogeochemical consumption in controlling water cap oxygen levels in Base Mine Lake. Appl. Geochem. 111:104442. doi: 10.1016/j.apgeochem.2019.104442
Ayala-Muñoz, D., Burgos, W. D., Sánchez-España, J., Couradeau, E., Falagán, C., and Macalady, J. L. (2020). Metagenomic and metatranscriptomic study of microbial metal resistance in an acidic pit lake. Microorganisms 8:1350. doi: 10.3390/microorganisms8091350
Ayala-Muñoz, D., Macalady, J. L., Sánchez-España, J., Falagán, C., Couradeau, E., and Burgos, W. D. (2022). Microbial carbon, sulfur, iron, and nitrogen cycling linked to the potential remediation of a meromictic acidic pit lake. ISME J [Epub ahead of print]. doi: 10.1038/s41396-022-01320-w
Benthaus, F. C., Totsche, O., and Luckner, L. (2020). In-lake neutralization of East German lignite pit lakes: Technical history and new approaches from LMBV. Mine Water Environ. 39, 603–617. doi: 10.1007/s10230-020-00707-5
Birkhofer, K., Diehl, E., Andersson, J., Ekroos, J., Früh-Müller, A., Machnikowski, F., et al. (2015). Ecosystem services-current challenges and opportunities for ecological research. Front. Ecol. Evol. 2:87. doi: 10.3389/fevo.2014.00087
Blanchette, M. L., Allcock, R., Gonzalez, J., Kresoje, N., and Lund, M. (2020). Macroinvertebrates and microbes (Archaea, Bacteria) offer complementary insights into mine-pit lake ecology. Mine Water Environ. 39, 589–602. doi: 10.1007/s10230-019-00647-9
Blanchette, M. L., and Lund, M. A. (2016). Pit lakes are a global legacy of mining: An integrated approach to achieving sustainable ecosystems and value for communities. Curr. Opin. Environ. Sustain. 23, 28–34. doi: 10.1016/j.cosust.2016.11.012
Blanchette, M. L., and Lund, M. A. (2020). Foreword to the special issue on pit lakes: The current state of pit lake science. Mine Water Environ. 39, 425–426. doi: 10.1007/s10230-020-00706-6
Blanchette, M. L., and Lund, M. A. (2021). Aquatic ecosystems of the Anthropocene: Limnology and microbial ecology of mine pit lakes. Microorganisms 9:1207. doi: 10.3390/microorganisms9061207
Blanco, J., Dendoncker, N., Barnaud, C., and Sirami, C. (2019). Ecosystem disservices matter: Towards their systematic integration within ecosystem service research and policy. Ecosyst. Serv. 36:100913. doi: 10.1016/j.ecoser.2019.100913
Castendyk, D., and Eary, L. (2009). “The nature and global distribution of pit lakes,” in Mine pit lakes: Characteristics, predictive modelling, and sustainability, eds D. N. Castendyk and L. E. Eary (Littleton, CO: Society of Mining, Metallurgy, and Exploration), 1–11. doi: 10.3897/BDJ.8.e54959
Castro, J. M., and Moore, J. N. (2000). Pit lakes: Their characteristics and the potential for their remediation. Environ. Geol. 39, 1254–1260. doi: 10.1007/s002549900100
Costa, M., and Duarte, J. (2005). Bioremediation of acid mine drainage using acidic soil and organic wastes for promoting sulphate-reducing bacteria activity on a column reactor. Water Air Soil Pollut. 165, 325–345. doi: 10.1007/s11270-005-6914-7
Coulson, M. (2012). The history of mining: The events, technology and people involved in the industry that forged the modern world. Petersfield: Harriman House Limited.
de Oliveira, L. F. V., and Margis, R. (2015). The source of the river as a nursery for microbial diversity. PLoS One 10:e0120608. doi: 10.1371/journal.pone.0120608
Duarte, C. M., Dennison, W. C., Orth, R. J. W., and Carruthers, T. J. B. (2008). The charisma of coastal ecosystems: Addressing the imbalance. Estuaries Coast. 31, 233–238. doi: 10.1007/s12237-008-9038-7
Dutta, J., and Sen, T. (2021). “Ecosystem services of microbes,” in Metagenomics and microbial ecology, 1st Edn, eds S. De Mandal, A. K. Panda, N. S. Kumar, S. S. Bisht, and F. Jin (Abingdon: Taylor & Francis Group).
Edwards, E. C., and Null, S. E. (2019). The cost of addressing saline lake level decline and the potential for water conservation markets. Sci. Total Environ. 651, 435–442. doi: 10.1016/j.scitotenv.2018.09.006
Falagán, C., Foesel, B., and Johnson, B. (2017a). Acidicapsa ferrireducens sp. nov., Acidicapsa acidiphila sp. nov., and Granulicella acidiphila sp. nov.: Novel acidobacteria isolated from metal-rich acidic waters. Extremophiles 21, 459–469. doi: 10.1007/s00792-017-0916-4
Falagán, C., Yusta, I., Sánchez-España, J., and Johnson, D. (2017b). Biologically-induced precipitation of aluminium in synthetic acid mine water. Miner. Eng. 106, 79–85. doi: 10.1016/j.mineng.2016.09.028
Falagán, C., and Johnson, D. (2016). Acidithiobacillus ferriphilus sp. nov., a facultatively anaerobic iron- and sulfur-metabolizing extreme acidophile. Int. J. Syst. Evol. Microbiol. 66, 206–211. doi: 10.1099/ijsem.0.000698
Falagán, C., and Johnson, D. B. (2014). Acidibacter ferrireducens gen. nov., sp. nov.: An acidophilic ferric iron-reducing gammaproteobacterium. Extremophiles 18, 1067–1073. doi: 10.1007/s00792-014-0684-3
Falagán, C., Sánchez-España, F. J., and Johnson, B. D. (2013). Microbiological communities in two acidic mine pit lakes in the Iberian Pyrite Belt (IPB), Spain. Adv. Mater. Res. 825, 19–22. doi: 10.1371/journal.pone.0066746
Falagán, C., Sánchez-España, J., and Johnson, D. B. (2014). New insights into the biogeochemistry of extremely acidic environments revealed by a combined cultivation-based and culture-independent study of two stratified pit lakes. FEMS Microbiol. Ecol. 87, 231–243. doi: 10.1111/1574-6941.12218
Falagán, C., Sánchez-España, J., Yusta, I., Barrie Johnson, D., Calera, L., and Cantos, T. (2016). “New insights into the microbiology of meromictic acidic pit lakes in the Iberian Pyrite Belt (Spain),” in Proceedings of the IMWA 2016, ‘mining meets water – conflicts and solutions’, eds C. Drebenstedt and M. Paul (Lepzig: IMWA), 192–198.
Fauville, A., Mayer, B., Frömmichen, R., Friese, K., and Veizer, J. (2004). Chemical and isotopic evidence for accelerated bacterial sulphate reduction in acid mining lakes after addition of organic carbon: Laboratory batch experiments. Chem. Geol. 204, 325–344. doi: 10.1016/j.chemgeo.2003.11.010
Flemming, H. C., and Wuertz, S. (2019). Bacteria and archaea on Earth and their abundance in biofilms. Nat. Rev. Microbiol. 17, 247–260. doi: 10.1038/s41579-019-0158-9
Frömmichen, R., Kellner, S., and Friese, K. (2003). Sediment conditioning with organic and/or inorganic carbon sources as a first step in alkalinity generation of acid mine pit lake water (pH 2-3). Environ. Sci. Technol. 37, 1414–1421. doi: 10.1021/es026131c
Fyson, A., Nixdorf, B., and Kalin, M. (2006). The acidic lignite pit lakes of Germany-microcosm experiments on acidity removal through controlled eutrophication. Ecol. Eng. 28, 288–295. doi: 10.1016/j.ecoleng.2006.06.012
Gammons, C. H., and Icopini, G. A. (2020). Improvements to the water quality of the acidic Berkeley pit lake due to copper recovery and sludge disposal. Mine Water Environ. 39, 427–439. doi: 10.1007/s10230-019-00648-8
Geller, W., Koschorreck, M., Wendt-Potthoff, K., Bozau, E., Herzsprung, P., Büttner, O., et al. (2009). A pilot-scale field experiment for the microbial neutralization of a holomictic acidic pit lake. J. Geochem. Explor. 100, 153–159. doi: 10.1016/j.gexplo.2008.04.003
González-Toril, E., Santofimia, E., López-Pamo, E., Omoregie, E. O., Amils, R., and Aguilera, Á. (2013). Microbial ecology in extreme acidic pit lakes from the Iberian pyrite belt (SW Spain). Adv. Mater. Res. 825, 23–27.
Graupner, H. (2009). Nachterstedt landslide raises worries for other former mine areas. Bonn: Deutsche Welle.
Grettenberger, C. L., Mccauley Rench, R. L., Gruen, D. S., Mills, D. B., Carney, C., Brainard, J., et al. (2020). Microbial population structure in a stratified, acidic pit lake in the Iberian Pyrite Belt. Geomicrobiol. J. 37, 623–634. doi: 10.1080/01490451.2020.1751748
Gupta, A., Gupta, R., and Singh, R. L. (2017). “Microbes and environment,” in Principles and applications of environmental biotechnology for a sustainable future, ed. R. Singh (Singapore: Springer), 43–84. doi: 10.1007/978-981-10-1866-4_3
Hadjipanagiotou, C., Christou, A., Zissimos, A. M., Chatzitheodoridis, E., and Varnavas, S. P. (2020). Contamination of stream waters, sediments, and agricultural soil in the surroundings of an abandoned copper mine by potentially toxic elements and associated environmental and potential human health–derived risks: A case study from Agrokipia, Cyprus. Environ. Sci. Pollut. Res. 27, 41279–41298. doi: 10.1007/s11356-020-10098-3
He, F., Jähnig, S. C., Wetzig, A., and Langhans, S. D. (2021). More exposure opportunities for promoting freshwater conservation. Aquat. Conserv. Mar. Freshw. Ecosyst. 31, 3626–3635. doi: 10.1002/aqc.3725
Kamjunke, N., Tittel, J., Krumbeck, H., Beulker, C., and Poerschmann, J. (2005). High heterotrophic bacterial production in acidic, iron-rich mining lakes. Microb. Ecol. 49, 425–433. doi: 10.1007/s00248-004-0270-9
Kampe, H., Dziallas, C., Grossart, H. P., and Kamjunke, N. (2010). Similar bacterial community composition in acidic mining lakes with different pH and lake chemistry. Microb. Ecol. 60, 618–627. doi: 10.1007/s00248-010-9679-5
Karakas, G., Brookland, I., and Boehrer, B. (2003). Physical characteristics of acidic mining lake 111. Aquat. Sci. 65, 297–307. doi: 10.1007/s00027-003-0651-z
Kleinsteuber, S., Müller, F. D., Chatzinotas, A., Wendt-Potthoff, K., and Harms, H. (2008). Diversity and in situ quantification of Acidobacteria subdivision 1 in an acidic mining lake. FEMS Microbiol. Ecol. 63, 107–117. doi: 10.1111/j.1574-6941.2007.00402.x
Koschorreck, M., and Wendt-Potthoff, K. (2012). A sediment exchange experiment to assess the limiting factors of microbial sulfate reduction in acidic mine pit lakes. J. Soils Sediments 12, 1615–1622. doi: 10.1007/s11368-012-0547-4
Kumar, R. N., McCullough, C. D., and Lund, M. A. (2011). How does storage affect the quality and quantity of organic carbon in sewage for use in the bioremediation of acidic mine waters? Ecol. Eng. 37, 1205–1213. doi: 10.1016/j.ecoleng.2011.02.021
Lopez, X. P. S., and Blanchette, M. L. (2020). Understanding public perceptions of a new pit lake in As Pontes, Spain. Mine Water Environ. 39, 647–656. doi: 10.1007/s10230-020-00651-4
Lund, M., van Etten, E., Polifka, J., Vasquez, M., Ramessur, R., Yangzom, D., et al. (2020). The importance of catchments to mine-pit lakes: Implications for closure. Mine Water Environ. 39, 572–588. doi: 10.1007/s10230-020-00704-8
Lund, M. A., and McCullough, C. D. (2015). “Addition of bulk organic matter to acidic pit lakes may facilitate closure,” in Proceedings of the 10th international conference on acid rock drainage & IMWA annual conference, Santiago, 1–11.
Mantero, J., Thomas, R., Holm, E., Rääf, C., Vioque, I., Ruiz-Canovas, C., et al. (2020). Pit lakes from Southern Sweden: Natural radioactivity and elementary characterization. Sci. Rep. 10:13712. doi: 10.1038/s41598-020-70521-0
Marszelewski, W., Dembowska, E. A., Napiórkowski, P., and Solarczyk, A. (2017). Das verständnis abiotischer und biotischer verhältnisse in bergbauseen im dienst einer effizienten bewirtschaftung: Eine Fallstudie (Polen). Mine Water Environ. 36, 418–428. doi: 10.1007/s10230-017-0434-8
McCullough, C., and Lund, M. (2006). Opportunities for sustainable mining pit lakes in Australia. Mine Water Environ. 25, 220–226. doi: 10.1007/s10230-006-0136-0
McCullough, C., and Lund, M. (2011). “Limiting factors for crayfish and finfish in acidic coal pit lake,” in Proceedings of the international mine water conference (IMWA) congress, Aachen.
Mccullough, C. D., and Lund, M. A. (2008). “Field scale trials treating acidity in coal pit lakes using sewage and green waste,” in Proceedings of the 10th international mine water association (IMWA) congress, Karlsbad, 599–602.
McCullough, C. D., Lund, M. A., and May, J. M. (2006). “Microcosm testing of municipal sewage and green waste for full-scale remediation of an acid coal pit lake, in semi-arid tropical Australia,” in Proceedings of the 7th international conference on acid rock drainage 2006, ICARD, Vol. 2, St. Louis, MO, 1177–1197. doi: 10.21000/jasmr06021177
McGinlay, J., Parsons, D. J., Morris, J., Hubatova, M., Graves, A., Bradbury, R. B., et al. (2017). Do charismatic species groups generate more cultural ecosystem service benefits? Ecosyst. Serv. 27, 15–24. doi: 10.1016/j.ecoser.2017.07.007
Mehanna, M., Saito, T., Yan, J., Hickner, M., Cao, X., Huang, X., et al. (2010). Using microbial desalination cells to reduce water salinity prior to reverse osmosis. Energy Environ. Sci. 3, 1114–1120. doi: 10.1039/c002307h
Meier, J., Babenzien, H.-D., and Wendt-Potthoff, K. (2004). Microbial cycling of iron and sulfur in sediments of acidic and pH-neutral mining lakes in Lusatia (Brandenburg, Germany). Biogeochemistry 67, 135–156. doi: 10.1023/B:BIOG.0000015324.2890.b7
Mori, J. F., Chen, L. X., Jessen, G. L., Rudderham, S. B., McBeth, J. M., Lindsay, M. B. J., et al. (2019). Putative mixotrophic nitrifying-denitrifying gammaproteobacteria implicated in nitrogen cycling within the ammonia/oxygen transition zone of an oil sands pit lake. Front. Microbiol. 10:2435. doi: 10.3389/fmicb.2019.02435
Morris, A., Meyer, K., and Bohannan, B. (2020). Linking microbial communities to ecosystem functions: What we can learn from genotype-phenotype mapping in organisms. Philos. Trans. R. Soc. B Biol. Sci. 375:20190244. doi: 10.1098/rstb.2019.0244
Mouhamad, R. S., Mutlag, L. A., Al-Khateeb, M. T., Iqbal, M., Nazir, A., Ibrahim, K. M., et al. (2017). Reducing water salinity using effective microorganisms. Net J. Agric. Sci. 5, 114–120. doi: 10.30918/NJAS.53.17.048
Neil, L. L., McCullough, C. D., Lund, M. A., Evans, L. H., and Tsvetnenko, Y. (2009). Toxicity of acid mine pit lake water remediated with limestone and phosphorus. Ecotoxicol. Environ. Saf. 72, 2046–2057. doi: 10.1016/j.ecoenv.2009.08.013
Newman, C. P., Poulson, S. R., and McCrea, K. W. (2020). Contaminant generation and transport from mine pit lake to perennial stream system: Multidisciplinary investigations at the Big Ledge Mine, Nevada, USA. Chem. Erde 80:125552. doi: 10.1016/j.chemer.2019.125552
Nielsen, B. L. (2020). Scientists use bacteria to help plants grow in salty soil. Douglas: Phys.Org.
Oren, A. (2004). Prokaryote diversity and taxonomy: Current status and future challenges. Philos. Trans. R. Soc. B Biol. Sci. 359, 623–638. doi: 10.1098/rstb.2003.1458
Pace, A., Borillot, R., Bouton, A., Vennin, E., Galaup, S., Bundeleva, I., et al. (2016). Microbial and diagenetic steps leading to the mineralization of Great Salt Lake microbialites. Sci. Rep. 6:31495. doi: 10.1038/srep31495
Palit, D., and Kar, D. (2019). “A contemplation on pit lakes of Raniganj Coalfield Area: West Bengal, India,” in Sustainable agriculture, forest and environmental management, eds M. K. Jhariya, A. Banerjee, R. S. Meena, and D. K. Yadav (Singapore: Springer), 517–571.
Pascoal, F., Costa, R., and Magalhães, C. (2021). The microbial rare biosphere: Current concepts, methods and ecological principles. FEMS Microbiol. Ecol. 97:fiaa227. doi: 10.1093/femsec/fiaa227
Robbins, J. (2016). Hordes of geese die on a toxic lake in Montana. New York, NY: The New York Times.
Rönicke, H., Frassl, M. A., Rinke, K., Tittel, J., Beyer, M., Kormann, B., et al. (2021). Suppression of bloom-forming colonial cyanobacteria by phosphate precipitation: A 30 years case study in Lake Barleber (Germany). Ecol. Eng. 162:106171. doi: 10.1016/j.ecoleng.2021.106171
Rosa, J. C. S., Morrison-Saunders, A., Hughes, M., and Sánchez, L. E. (2020). Planning mine restoration through ecosystem services to enhance community engagement and deliver social benefits. Restor. Ecol. 28, 937–946. doi: 10.1111/rec.13162
Sakellari, C., Roumpos, C., Louloudis, G., and Vasileiou, E. (2021). A review about the sustainability of pit lakes as a rehabilitation factor after mine closure. Mater. Proc. 5:52. doi: 10.3390/materproc2021005052
Sánchez-España, J., Yusta, I., Ilin, A., van der Graaf, C., and Sánchez-Andrea, I. (2020b). Microbial geochemistry of the acidic saline pit lake of Brunita Mine (La Unión, SE Spain). Mine Water Environ. 39, 535–555. doi: 10.1007/s10230-020-00655-0
Sánchez-España, J., Falagán, C., Ayala, D., and Wendt-Potthoff, K. (2020a). Adaptation of Coccomyxa sp. To extremely low light conditions causes deep chlorophyll and oxygen maxima in acidic pit lakes. Microorganisms 8:1218. doi: 10.3390/microorganisms8081218
Sánchez-España, J., Wang, K., Falagán, C., Yusta, I., and Burgos, W. D. (2018). Microbially mediated aluminosilicate formation in acidic anaerobic environments: A cell-scale chemical perspective. Geobiology 16, 88–103. doi: 10.1111/gbi.12269
Saunders, M. E., and Luck, G. W. (2016). Limitations of the ecosystem services versus disservices dichotomy. Conserv. Biol. 30, 1363–1365. doi: 10.1111/cobi.12740
Sergeant, C., Sexton, E., Moore, J., Westwood, A., Nagorski, S., Ebersole, J., et al. (2022). Risks of mining to salmonid-bearing watersheds. Sci. Adv. 8:eabn0929. doi: 10.1126/sciadv.abn0929
Singh, A. K., Sisodia, A., Sisodia, V., and Padhi, M. (2019). “Role of microbes in restoration ecology and ecosystem services,” in New and future developments in microbial biotechnology and bioengineering: Microbial biotechnology in agro-environmental sustainability, eds V. K. Gupta and A. Pandey (Amsterdam: Elsevier), 57–68. doi: 10.1016/B978-0-444-64191-5.00004-3
Soni, A., Mishra, B., and Singh, S. (2014). Pit lakes as an end use of mining: A review. J. Min. Environ. 5, 99–111. doi: 10.1016/j.scitotenv.2018.05.279
Stendera, S., Adrian, R., Bonada, N., Cañedo-Argüelles, M., Hugueny, B., Januschke, K., et al. (2012). Drivers and stressors of freshwater biodiversity patterns across different ecosystems and scales: A review. Hydrobiologia 696, 1–28. doi: 10.1007/s10750-012-1183-0
Stierle, A. A., Stierle, D. B., and Kelly, K. (2006). Berkelic acid, a novel spiroketal with selective anticancer activity from an acid mine waste fungal extremophile. J. Org. Chem. 71, 5357–5360. doi: 10.1021/jo060018d
Svobodova, K., Owen, J. R., and Harris, J. (2021). The global energy transition and place attachment in coal mining communities: Implications for heavily industrialized landscapes. Energy Res. Soc. Sci. 71:101831. doi: 10.1016/j.erss.2020.101831
van der Graaf, C. M., Sánchez-España, J., Yusta, I., Ilin, A., Shetty, S. A., Bale, N. J., et al. (2020). Biosulfidogenesis mediates natural attenuation in acidic mine pit lakes. Microorganisms 8, 1–26. doi: 10.3390/microorganisms8091275
von Döhren, P., and Haase, D. (2015). Ecosystem disservices research: A review of the state of the art with a focus on cities. Ecol. Indic. 52, 490–497. doi: 10.1016/j.ecolind.2014.12.027
Weber, A. (2020). Surrounded by pit lakes: New landscapes after lignite mining in the former German democratic republic. Mine Water Environ. 39, 658–665. doi: 10.1007/s10230-020-00696-5
Wendt-Potthoff, K., Koschorreck, M., Diez Ercilla, M. D., and Sánchez-España, J. (2011). Microbial activity and biogeochemical cycling in nutrient-rich meromictic acid pit lake. Limnologica 42, 175–188. doi: 10.1016/j.limno.2011.10.004
White, R. A., Power, I. M., Dipple, G. M., Southam, G., and Suttle, C. A. (2015). Metagenomic analysis reveals that modern microbialites and polar microbial mats have similar taxonomic and functional potential. Front. Microbiol. 6:966. doi: 10.3389/fmicb.2015.00966
Williams, M., Oyedotun, T., and Simmons, D. (2020). Assessment of water quality of lakes used for recreational purposes in abandoned mines of Linden, Guyana. Geol. Ecol. Landsc. 4, 269–281. doi: 10.1080/24749508.2019.1633220
Williams, W. (1996). The largest, highest and lowest lakes of the world: Saline lakes. SIL Proc. 26, 61–79. doi: 10.1080/03680770.1995.11900693
Wollmann, K., Deneke, R., Nixdorf, B., and Packroff, G. (2000). Dynamics of planktonic food webs in three mining lakes across a pH gradient (pH 2-4). Hydrobiologia 433, 3–14. doi: 10.1023/A:1004060732467
Wurtsbaugh, W. A., Miller, C., Null, S. E., Justin De Rose, R., Wilcock, P., Hahnenberger, M., et al. (2017). Decline of the world’s saline lakes. Nat. Geosci. 10, 816–821. doi: 10.1038/NGEO3052
Xin, R., Banda, J. F., Hao, C., Dong, H., Pei, L., Guo, D., et al. (2021). Contrasting seasonal variations of geochemistry and microbial community in two adjacent acid mine drainage lakes in Anhui Province, China. Environ. Pollut. 268:115826. doi: 10.1016/j.envpol.2020.115826
Younger, P., Banwart, S., and Hedin, R. (2002). Mining and the water environment. Mine Water 5, 1–63. doi: 10.1007/978-94-010-0610-1_1
Younger, P., and Wolkersdorfer, C. (2004). Mining impacts on the freshwater environment: Technical and managerial guidelines for catchment scale management ERMITE-Consortium. Mine Water Environ. 23, S2–S80.
Keywords: prokaryotic microorganisms, global data set, Anthropocene, research effort, ecological restoration, mine water
Citation: Bernasconi R, Lund MA and Blanchette ML (2022) Non-charismatic waterbodies and ecosystem disservices: Mine pit lakes are underrepresented in the literature. Front. Microbiol. 13:1063594. doi: 10.3389/fmicb.2022.1063594
Received: 07 October 2022; Accepted: 07 November 2022;
Published: 29 November 2022.
Edited by:
Alessandra Adessi, University of Florence, ItalyReviewed by:
Javier Sánchez-España, Spanish National Research Council (CSIC), SpainCopyright © 2022 Bernasconi, Lund and Blanchette. This is an open-access article distributed under the terms of the Creative Commons Attribution License (CC BY). The use, distribution or reproduction in other forums is permitted, provided the original author(s) and the copyright owner(s) are credited and that the original publication in this journal is cited, in accordance with accepted academic practice. No use, distribution or reproduction is permitted which does not comply with these terms.
*Correspondence: Rachele Bernasconi, ci5iZXJuYXNjb25pQGVjdS5lZHUuYXU=
Disclaimer: All claims expressed in this article are solely those of the authors and do not necessarily represent those of their affiliated organizations, or those of the publisher, the editors and the reviewers. Any product that may be evaluated in this article or claim that may be made by its manufacturer is not guaranteed or endorsed by the publisher.
Research integrity at Frontiers
Learn more about the work of our research integrity team to safeguard the quality of each article we publish.