- 1Division of Crop Protection, Indian Council of Agricultural Research (ICAR)-Directorate of Onion and Garlic Research, Pune, Maharashtra, India
- 2School of Soil Stress Management, Indian Council of Agricultural Research (ICAR)-National Institute of Abiotic Stress Management, Baramati, Maharashtra, India
Waterlogging and anthracnose-twister disease are significant obstacles in rainy-season onion cultivation. As a shallow-rooted crop, onions are highly sensitive to waterlogging. Wherever rainy-season onion cultivation has been undertaken, the anthracnose-twister disease complex is also widespread across the world in addition to waterlogging. Waterlogging is the major predisposing factor for anthracnose and other fungal diseases. However, studies on the combined stress impact on onions have been ignored. In the present review, we have presented an overview of the anthracnose-twister disease, the waterlogging effect on host physiology, host-pathogen interaction under waterlogging stress, and appropriate management strategies to mitigate the combined stress effects. Crucial soil and crop management strategies can help cope with the negative impact of concurrent stresses. Raised bed planting with drip irrigation, the use of plant bio-regulators along with nutrient management, and need-based fungicide sprays would be the most reliable and feasible management options. The most comprehensive solution to withstand combined stress impacts would be a genetic improvement of commercial onion cultivars.
Introduction
Onions (Allium cepa L.), belonging to the family Alliaceae, are a major commodity in international trade due to their culinary and therapeutic uses. It has long been valued worldwide for its nutraceutical properties, including its anticancer, antidiabetic, antimicrobial, cardiovascular, and antioxidant effects. Onions are grown on more than 5.1 million hectares worldwide, yielding 99.9 million tons. Asia is a major contributor to the total production (64%), largely from China, followed by India (FAOSTAT, 2019). Despite the increased demand for onions in the international market, production is exhibiting a decreasing trend (Figure 1). The production, productivity, and innate nutritional potential of onions are immensely affected by the intervention of biotic and abiotic stresses. Diseases like Purple Blotch, Stemphylium Blight, the Anthracnose-Twister Complex, Fusarium Basal Rot, and insect pests such as thrips are the challenging biotic stresses affecting onion production. Among abiotic stresses, drought, waterlogging, extreme temperatures, salinity, and nutrient stress affect onion production; however, due to its shallow-rooted nature, it is extremely sensitive to waterlogging stress (Rao, 2016).
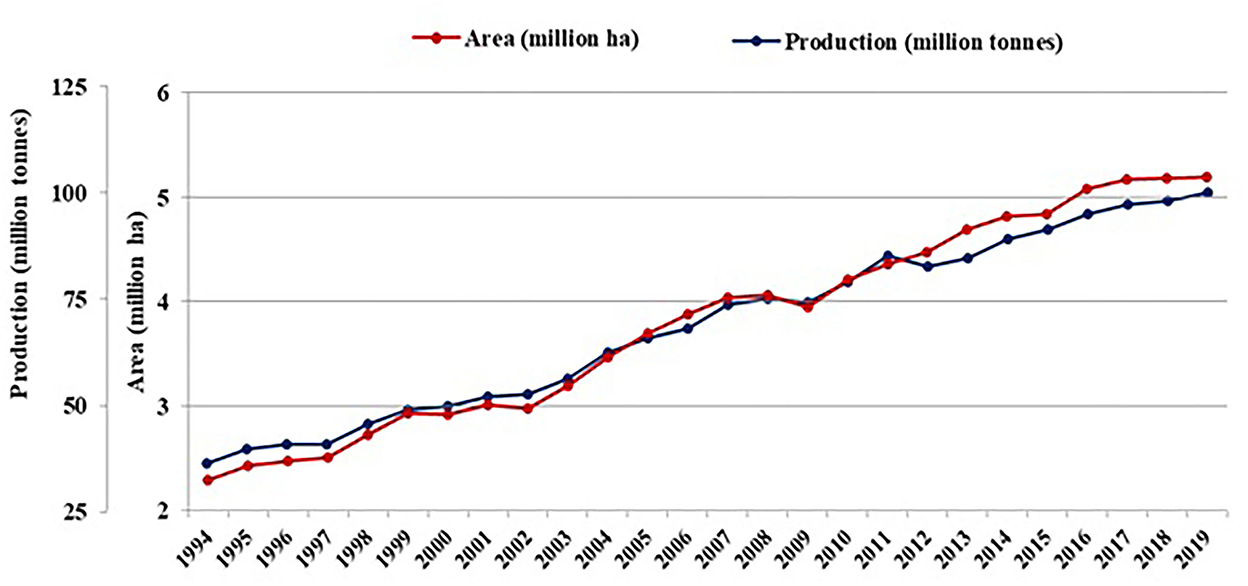
Figure 1. World status of onion on area harvested and production (Source: FAOSTAT, 2019).
Further, owing to global climate change, there is a discernible change in the onset and withdrawal of the monsoon and erratic rainfall patterns, resulting in frequent and prolonged waterlogging events [Jackson and Colmer, 2005; Intergovernmental Panel on Climate Change (IPCC)., 2014]. These climatic aberrations encouraged abiotic stresses, which, in turn, affected biotic stresses in onions; the classic example is the co-occurrence of waterlogging and anthracnose-twister disease.
The first and foremost impact of waterlogging in a plant is anoxia and hypoxia within the roots and their effects on the shoots (Arduini et al., 2016; Herzog et al., 2016). In addition to changes in host physiology, waterlogging may attract various facultative parasites/saprophytes by increasing the host's susceptibility to pathogen attack and is thus believed to be one of the major predisposing factors in many plant disease infections (Hsu et al., 2013; Tacconi et al., 2015; Tosi et al., 2015; Urban et al., 2015; Sorrenti et al., 2019). Similarly, in onions, waterlogging may have a significant role in the development of debilitating anthracnose or twister disease. However, until today, the interference of waterlogging with anthracnose-twister development has gone unnoticed. Though the predictable losses due to the disease vary with its severity and crop growth stage, the pathogen Colletotrichum sp. has been reported to result in up to 80–100% onion bulb yield loss in several countries (Ebenebe, 1980a; Chawda and Rajasab, 1996; Alberto et al., 2001, 2019; Alberto, 2014). Moreover, this disease has forced the majority of farmers to abandon rainy-season onion cultivation in India, Sri Lanka, Indonesia, and many west African countries (Wiyono, 2007; Sikirou et al., 2011; Chowdappa et al., 2015; Herath et al., 2021).
India, the second-largest onion-growing country in the world, has a high demand for its onions in the global market due to their distinctive pungency and year-round availability. In India, onions are grown three times a year: during the rainy season (kharif: July to October) (20%), during the late rainy season (late kharif : October to January) (20%), and during the post-rainy season (rabi: December to April) (60%). Although the share of rainy season onions in the country's total production is relatively small (up to 20%), it has a significant impact on price stability, as it can ensure a continuous supply in the market in October, November, December, and January, when stored onions from the post-monsoon season (rabi) are not available in the market (Samra et al., 2006; Srinivas and Lawande, 2007; Gopal, 2015; Gedam et al., 2021). In the major onion-growing states of India, i.e., Maharashtra and Karnataka, anthracnose is a major obstacle during the rainy season. Intense rainfall leading to soil flooding/waterlogging coupled with anthracnose disease are the major factors affecting rainy-season onion production.
So far, the available literature focuses on the individual stress impacts (either waterlogging or anthracnose) on onions (Chawda and Rajasab, 1996; Alberto et al., 2001, 2019; Yiu et al., 2009; Alberto, 2014; Ghodke et al., 2018; Dubey et al., 2020, 2021; Gedam et al., 2021). Most of the time, multiple stresses coexist during the crop growth cycle. The impact of multiple stress co-occurrences and their interaction has not been studied. Therefore, through this review article, we highlighted an overview of anthracnose-twister disease, the effect of waterlogging stress on host physiology, host-pathogen interaction under waterlogging stress, and appropriate management strategies to mitigate the combined stress effects.
Global and spatial distribution of anthracnose/twister
Earlier studies discovered that this disease is of major concern wherever onions are grown during the rainy or monsoon season (Ebenebe, 1980a; Qadri and Srivastava, 1985; Qadri, 1988; Singh and Sinha, 1994; Galvan et al., 1997; Weeraratne, 1997; Kuruppu, 1999; Alberto et al., 2001, 2002; Wiyono, 2007; Kim et al., 2008; Nischwitz et al., 2008; Sikirou et al., 2011; Rodriguez-Salamanca et al., 2012; Baysal-Gurel et al., 2014; Chowdappa et al., 2015; Santana et al., 2015). Figure 2 shows that anthracnose-twister disease is widespread throughout the world and occurs most regularly in the tropics and subtropics.
Taxonomic and molecular characterization of Colletotrichum sp. and genetic diversity of the pathogen
Conventional detection and characterization of Colletotrichum species were typically based on morphological characteristics, which were ineffective for distinguishing between Colletotrichum species. Hence, molecular characterization is much preferred and essential to examine genetic variation within and between species and populations. The internal transcribed spacer region (ITS) of ribosomal DNA has been normally used to separate Colletotrichum species (Freeman et al., 2000). However, it is not always appropriate (Damm et al., 2012). Therefore, molecular markers, actin (ACT), β-tubulin (TUB2), chitin synthase 1 (CHS-1), and glyceraldehyde-3-phosphate dehydrogenase (GAPDH) gene, have been used to identify the Colletotrichum species (Weir et al., 2012). Several studies of morphological and molecular characterization by earlier researchers were conducted on onions to determine the cause of the anthracnose-twister complex. Before 1997, C. gloeosporioides was known as the only pathogen responsible for onion anthracnose. Later, C. gloeosporioides and Fusarium oxysporum were reported to be the predominant microorganisms associated with the leaf twister disease (LTD) (Weeraratne, 1997; Kuruppu, 1999). Further, a detailed etiological study revealed that the onion anthracnose is a disease complex caused by the collective infection of C. gloeosporioides, C. acutatum, and Gibberella moniliformis (Alberto and Aquino, 2010). Among these, C. gloeosporioides and C. acutatum were reported to be responsible for leaf anthracnose, while G. moniliformis was responsible for abnormal neck elongation and leaf twisting, which might be due to excessive accumulation of phytohormone gibberellins in onions (Alberto and Aquino, 2010; Alberto, 2014). Several other Colletotrichum spp. such as C. circinans (Kim et al., 2008; Kiehr et al., 2012; Chen et al., 2022), C. coccodes (Rodriguez-Salamanca et al., 2012; Baysal-Gurel et al., 2014; Hay et al., 2016), C. siamense, C. truncatum (Chowdappa et al., 2015; Salunkhe et al., 2018a), C. spaethianum (Santana et al., 2015; Salunkhe et al., 2018b), and recently three members of C. acutatum species complex (C. nymphaeae, C. scovillei, and C. tamarilloi), and two members of C. gloeosporioides species complex (C. fructicola, C. theobromicola) (Lopes et al., 2021) have been reported in onion crop and their wild relatives. Thus, all earlier studies revealed that onion anthracnose is a disease complex predominantly caused by the Colletotrichum species complex and the association of G. moniliformis, which helps to increase symptom severity.
Symptomatology
This disease affects both the aboveground and underground parts of the onion. Typical anthracnose-affected plants showed leaf curling, twisting, chlorosis, necrotic leaves and sheath with black acervuli, and neck/pseudostem elongation followed by bulb rotting (Ebenebe, 1980a; Chawda and Rajasab, 1996; Weeraratne, 1997; Madushani et al., 2012; Chowdappa et al., 2015) (Figure 3). We observed that initially, small, whitish, water-soaked sunken lesions appeared on leaf blades and leaf sheaths. Further, the lesions became oval to elliptical and were surrounded by chlorotic margins, which turned into necrotic regions along the leaf axis. On necrotic tissues, prominent, black acervuli were formed in concentric rings. Later, the lesions became thin, brittle, and easily detached at the point of the lesion. Under high inoculum pressure, lesions can be seen on the leaf sheath near the soil, leading to bulb rotting. If the disease occurs at an early crop stage, there is no bulb formation, and it can cause complete crop loss. If it occurs at a later stage, even if there is bulb formation, the quality of the bulb deteriorates (Alberto et al., 2001, 2002; Wiyono, 2007; Nischwitz et al., 2008).
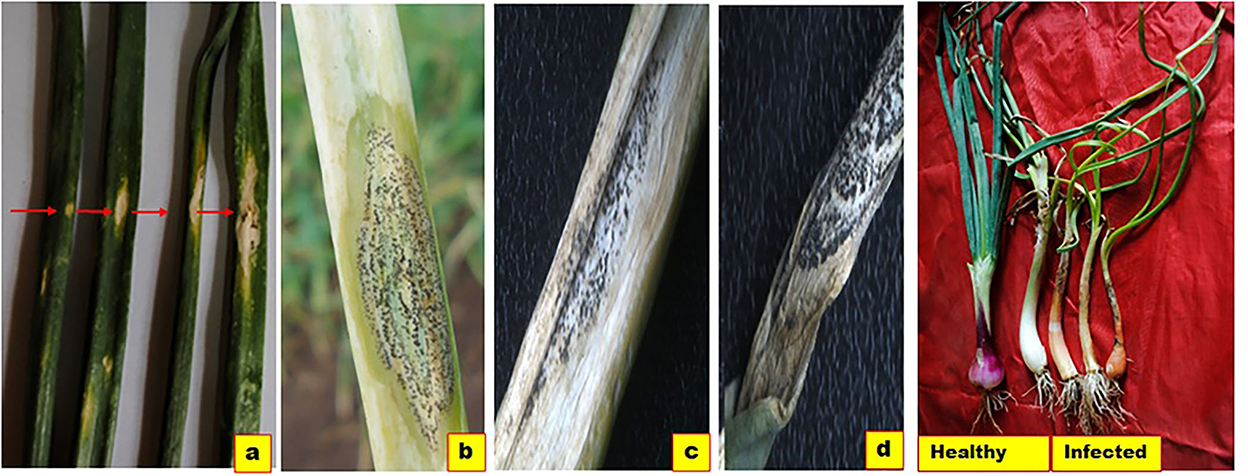
Figure 3. Symptoms of Anthracnose-Twister. (a) Initial white sunken lesions on leaves enlarges to form straw color patches surrounded by chlorotic margin. (b) Lesion advancement with formation of black color, fruiting bodies (acervuli). (c) Necrotic tissues covered with acervuli. (d) Leaf twisting and necrosis (d) seedling chlorosis, neck elongation and twisting with undersized bulbs. Red arrows indicate lesion enlargement.
Pathogen infection strategy
In nature, Colletotrichum spp. can overwinter in the form of acervuli/dormant mycelium on infected plant debris in the soil. With the onset of monsoon rains or sometimes off-season showers, resting structures enhance conidia formation (Ebenebe, 1980b), which establishes contact with the host for establishment. These conidia are usually water-borne, and quiescent infection is at its utmost during the wettest days of the cropping season (Denham and Waller, 1981; Fitzell and Peak, 1984; Darvas and Kotze, 1987). A detailed study of conidial behavior showed that since conidia were embedded in a mucilaginous mass of glycoproteins and proline-rich substances, they remained protected from desiccation and toxic plant metabolites (Gregory et al., 1959; Nicholson, 1992). Mucilage also has the self-inhibiting compound gloeosporone, which prevents conidial germination in thick suspensions (Lax et al., 1985). With rainfall and high humidity, mucilage absorbs water, and thus conidial concentrations become diluted.
Moreover, the liberation and dispersal of conidia take place through a splash and wash-off mechanism (Rajasab and Chawda, 1994). Lower leaves receive conidial inoculum with rain splashes; hence, the maximum infected area was observed on the lower leaves. Later, conidia were transported by wash-off mechanism, along with rain splashes, to the upper leaves and were released from the leaves, moved downward, and deposited over the neck and bulbs where anthracnose symptoms develop. Since the conidial inoculum is distributed in several loci, the disease initially appears in patches and is equally distributed throughout the field (Ebenebe, 1980b).
After reaching the infection site, conidia rapidly adhere to the aerial parts of the host plant (Nicholson, 1992, 1996; Mercure et al., 1994a,b) and apply intracellular colonization and subcuticular intramural colonization strategies for infection. Besides this, the fungus develops infection structures such as germ tubes, appressoria, intracellular hyphae, and secondary necrotrophic hyphae (Bailey et al., 1992). Pre- and post-penetration steps of infection, colonization, and anthracnose symptom appearance in onions could be completed within 120 h after incubation (Panday et al., 2012). Thus, the pathogen with a short life cycle (Figure 4) may trigger disease outbursts under a conducive environment and has the potential to destroy the crop in a very short span.
Epidemic favoring climatic conditions
Studies regarding anthracnose favorable weather parameters reported that heavy rainfall, high relative humidity (nearly 100%), and moderate temperature (20–31°C) appear to be congenial for spore germination, pathogen establishment, and disease epidemics (Mordue, 1971; Weeraratne, 1997; Sharma and Kulshrestha, 2015). Similar studies in tomato and pepper also concluded that anthracnose severity was found to be associated with intense rainfall or overhead irrigation, which encourages conidial dispersal and provides leaf wetness favorable for pathogen infection and disease development (Dillard, 1989, 1992; Sanogo and Pennypacker, 1997). Interaction between temperature and relative humidity plays an important role in anthracnose symptom severity, and a combination of high (95 ± 5%) RH for ≥24 h and temperatures ≥25°C creates highly conducive conditions for anthracnose (Rodriguez-Salamanca et al., 2018). Thus, it is obvious that rainfall causing high relative humidity and prolonged leaf wetness are the primary contributors to disease formation and progression. Waterlogging stress and anthracnose co-occur during the onion crop cycle. Therefore, their co-occurrence might have a certain interaction that needs to be systematically investigated.
Waterlogging stress physiology and its impact on anthracnose
Soil flooding/waterlogging is a widespread, unavoidable seasonal phenomenon that limits onion production globally. The damage due to waterlogging is severe in onion crops because of their shallow root systems, where maximum root penetration is about 75 cm, with higher root density only in the topsoil layer (Drinkwater and Janes, 1955). It adversely affects plant growth and development both in the nursery and in a standing crop and, consequently, the yield potential of onions (Ghodke et al., 2018). It also limits plant growth by interfering with various physiological and biochemical processes regulated by a network of genes and plant hormones (Figure 5). Its impact is complex and varies according to the variety, season, soil type, growth stage, extent, and rainfall duration.
Waterlogging stress leads to oxygen scarcity in the soil as it reduces soil aeration. Roots are the primary organ that experiences the damaging effects of flooding stress. Waterlogging stress often creates hypoxia (low oxygen) or anoxia (absence of oxygen) in the roots that limits photosynthetic oxygen production. Consequently, this affects the internal oxygen level and diffusion, which shifts the root respiration from aerobic to a low-ATP, anaerobic fermentation pathway. This induced ethanolic and lactic fermentation pathway via mitochondrial oxidative phosphorylation depletes the overall plant energy level. This energy crisis inhibits root growth and its functioning, leading to root mortality. Along with soil physicochemical properties (pH and redox potential) low oxygen level also affects the soil microbiota. Waterlogging lowers the concentration of oxidized nutrient elements (NO3-, SO42-, Fe3+) and increases the abundance of reduced elements (Mn2+, Fe2+, H2S, NH4+). These elements accumulate in the root in addition to the internally synthesized ethylene and carbon dioxide that are highly toxic to plants (Greenway et al., 2006; Kreuzwieser and Rennenberg, 2014). It further induces oxidative stress in plants by stimulating a progressive reduction in soil oxygen levels. This causes a burst in the production of reactive oxygen species (hydrogen peroxide, superoxide, and hydroxyl radical), resulting in photo-oxidative damage that leads to cell membrane damage, enzyme deactivation, impaired photosynthesis, and other vital processes that interrupt the normal water and nutrient translocation toward the shoots (Herzog et al., 2016). Eventually, the overall plant growth is stunted due to energy limitation; under severe anaerobic conditions, the accumulation of toxic products further leads to high plant mortality.
Photosynthesis is the key physiological process that is severely affected due to waterlogging stress. The net carbon assimilation rate is hampered in many crops due to waterlogging stress (Vu and Yelenosky, 1991). The decrease in photosynthesis under hypoxia or anoxia is mainly due to stomatal and non-stomatal limitations. Reduced chlorophyll pigments, accumulation of carbohydrates, and alteration in Rubisco carboxylase/oxygenase and PEP carboxylase enzyme activities are associated with non-stomatal limitations (Iglesias et al., 2002). However, low stomatal conductance (Stomata closer) due to reduced root hydraulic conductivity is the stomatal limitation that impaired the water and nutrient absorption during the stress period. Plants with these limitations exhibited stunted shoot growth, declining leaf area, and premature leaf senescence, resulting in the yellowing of the leaves, wilting, and epinasty. In addition, the synthesis and signaling of plant hormones, photosynthesis, assimilation partitioning, and translocation are also negatively affected (Ferrer et al., 2005). These physiological impedances reduce crop yield (maize and okra) under waterlogged conditions (Vwioko et al., 2017). Nutrient deficiency is one of the major effects of waterlogging stress in plants, resulting in reduced photosynthesis and net carbon fixation, ultimately leading to a reduction in growth and yield (Bange et al., 2004) (Figure 5).
Waterlogging interferes with host physiology, pathogen biology, and plant-pathogen interaction and influences the incidence and severity of foliar diseases. Prolonged mild, wet weather and wet soil create high humidity within the crop canopy, which causes the pathogen to spread (Lawyer, 1985). Even though there have been several reports of the effects of leaf wetness or the amount and duration of rainfall on foliar diseases (Murray et al., 1990; Trapero-Casas and Kaiser, 1992), we have few reports regarding the effect of saturated soils on foliar diseases in the database. Generally, abiotic stress may influence plant-pathogen interaction positively or negatively, thus increasing or decreasing the disease severity (Chojak-Kozniewska et al., 2018). Seed-to-seedling transmission of bacterial blight (Pseudomonas syringae pv. pisi) in pea crops increases extensively as the soil approaches saturation (Roberts, 1992). Diseases of cereals and lupin crops are more severe in waterlogged soils (Belford et al., 1992). Waterlogging was found to increase the prevalence of banana vascular wilt (F. oxysporum f. sp. cubense), crown and root rot (Phytophthora spp.) of apple and raspberry damping off (Pythium irregulare) in beans, and verticillium wilt (Verticillium dahlia) in chili pepper (Duncan and Kennedy, 1989; Wilcox, 1993; Shivas et al., 1995; Aguilar et al., 2000; Sanogo et al., 2008; Li et al., 2015). Pathogens' ability to grow under anaerobic conditions in waterlogged soils contributes to disease severity (Rao and Li, 2003). The duration of waterlogging is an important component of an interaction study that can alter combined stress consequences. Waterlogging for more than 6 days stressed Cape gooseberry plants, making them more prone to wilt (F. oxysporum f. sp. physali.).
Onset and progression of onion anthracnose relies heavily on favorable environmental conditions such as., high humidity, leaf wetness, persistent precipitation and warm temperature (Mordue, 1971; Dillard, 1989, 1992; Sanogo and Pennypacker, 1997; Weeraratne, 1997; Sharma and Kulshrestha, 2015; Rodriguez-Salamanca et al., 2018). Abiotic stress usually increases vulnerability to hemibiotrophic or necrotrophic pathogens. However, it decreases susceptibility to biotrophic pathogens (Saijo and Loo, 2020). Since C. gloeosporiodes is a hemibiotrophic pathogen, waterlogging may increase the host's susceptibility to onion anthracnose. In addition to causing root anoxia, waterlogging raises canopy humidity, making the plant more susceptible to foliar diseases (Jolly et al., 2017).
It is evident from the studies conducted during 2012–13 at the research station of ICAR-DOGR, Rajgurunagar, Pune, where the incidence of foliar diseases was monitored on 15 planting dates starting from June 15 to January 31, each at a 15-day interval. The peak incidence of anthracnose was during August and September, irrespective of the crop age/planting date. The highest significant positive correlation was recorded between disease severity and rainfall, i.e., 0.976 (p < 0.001). Even though several weather parameters are present, rainfall alone is able to play a significant role in anthracnose severity (Figure 6). During these months, high levels of soil saturation and frequent and long spells of water stagnation were observed in the field. These results support the fact that waterlogging aids in the development of anthracnose.
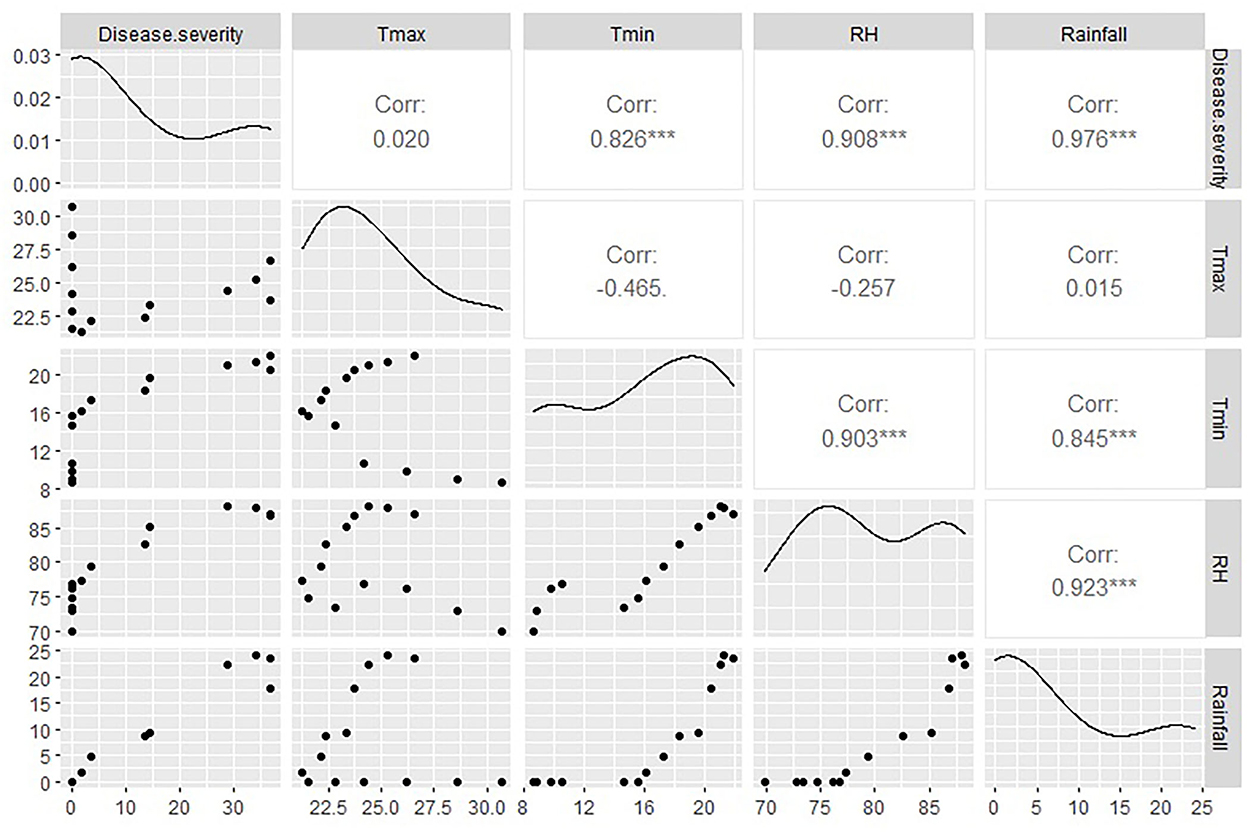
Figure 6. Anthracnose disease severity in relation to weather parameters (rainfall, humidity, temperature) during 2012-13 at ICAR-DOGR. ***Highly significant at < 0.001.
Generally, if abiotic stress happens before infection, it acts as predisposing stress, and predisposition implies an effect on the host rather than the pathogen; this also changes the host's resistance to susceptibility or, rarely, vice versa (Figure 7). In peas, pre-infection waterlogging reduced black spot (Mycosphaerella pinodes) severity, whereas post-infection waterlogging increased disease severity and caused a greater reduction in plant growth (McDonald and Dean, 1996). Pre-infection waterlogging might cause certain changes in host plants that hamper the pathogen establishment, and this might be the possible reason for reduced disease severity. Usually, there is a marked reduction in leaf water content (free water and relative water content) in waterlogged plants (Kuai et al., 2014; An et al., 2016; Zhang et al., 2019). The leaf water content is always associated with the water uptake capacity of the root, leaf transpiration, and leaf anatomy structure, which can alter due to waterlogging stress—the leaf temperature of waterlogged plants increases due to reduced transpiration rate and gas exchange.
Additionally, many plants close their stomata in response to waterlogging. Thus, in such situations, initial establishment and host penetration by the foliar pathogens may become difficult, reducing the possibility of anthracnose infection. However, under this condition, a possible increase in root infection may give a twister appearance to onion (Shivas et al., 1995; Alberto and Aquino, 2010; Alberto, 2014). In contrast, if the foliar infection by C. gloeosporiodes has already been established in the host and later the host is exposed to waterlogging, chances of enhanced growth, sporulation, and the spread of foliar pathogen may increase (Figure 7).
Moreover, nutritional imbalance due to waterlogging causes leaf chlorosis, senescence, wilting, necrosis, and stunted plant growth, which may affect the susceptibility of a plant to disease and increase the severity of infection (Graham, 1983; Yiu et al., 2008). The physiological response of plants to waterlogging may also make them more susceptible to disease. Increased ethylene levels in the shoots of waterlogged plants may be responsible for chlorosis and senescence (Smith, 1987). Stem/neck elongation is an escape strategy by short-term waterlogged plants (Zhang et al., 2021). In onions, abnormal neck elongation and leaf twisting are supposed to be due to excessive accumulation of gibberellins produced by secondary infection of G. moniliformis (Alberto and Aquino, 2010; Alberto, 2014). Nevertheless, the role of waterlogging in onion neck elongation is still unclear, and studies in this line may provide a clear picture.
In several cases, exposure of plants to abiotic stress weakens disease resistance, while pathogen infections often enhance abiotic stress responses (Atkinson and Urwin, 2012). Plants' response to combined stress is a more complex phenomenon than individual stress response and warrants an understanding of the crop-specific response. Thus, in addition to other factors such as a virulent pathogen, an initial amount of inoculum, susceptible hosts, a congenial environment, and crop management practices (planting method and plant density), waterlogging has a role in disease development that needs to be systemically studied. Knowledge of pre- and post-infectional waterlogging impact on onion anthracnose development will help combat combined stress.
Concurrent waterlogging and anthracnose-twister management strategies
Adopting site-specific soil and crop management practices can improve soil drainage and trigger plant resistance, respectively, and may help mitigate the negative impact of combined stress. Although specific research work in this direction is yet to be carried out, possible management options that might help to combat the concurrent stress in onion are discussed below.
Raised bed with drip irrigation
Raised bed planting should be followed for planting rainy season onions to minimize the damage due to water stagnation and anthracnose (Govaerts et al., 2007; Gadge and Lawande, 2012; Laxman et al., 2020). In raised beds, as the top 15 cm of the soil layer remained unsaturated, it decreased the waterlogging effect and improved soil drainage by creating a favorable environment for root growth and its proper functioning (Bakker et al., 2005; Blessitt, 2007; Velmurugan et al., 2016). Further, bioaerosols (small water droplets with conidia masses) were easily disseminated, and disease incidence might increase with overhead irrigation (Raniere and Crossan, 1959). Hence, the adoption of drip irrigation was found to be more effective in avoiding water stagnation in the root zone and minimizing disease incidence (Tripathi et al., 2017). Thus, a raised bed with drip irrigation could be an efficient technology for mitigating waterlogging and anthracnose stress in rainy onions.
Enhanced crop vigor
Crop vigor during the initial growth stages can be essential for waterlogging and disease tolerance (Sundgren et al., 2018). Seed priming is the most effective method for improving seedling establishment and early crop vigor (Taylor et al., 1998). Various crop seed priming agents have been explored for abiotic and biotic stress tolerance. In onions, vermicompost seed priming enhanced germination, and healthy plants withstood drought, salinity, and temperature stresses (Muhie et al., 2020). This was due to the improved activities of enzymes such as catalase, superoxide dismutase, and ascorbate peroxidase. Priming with hydrogen peroxide (H2O2) enhanced waterlogging tolerance in soybean seedlings (Andrade et al., 2018), which can be explored in onions. Nevertheless, more research is needed to determine the impact of vigor-stimulating compounds and their use for concurrent stress alleviation in onions.
Further, there are reports of managing early-stage crop stress with emerging plasma technology (Song et al., 2020). Limited in vitro studies regarding the induction of crop tolerance to pathogen stress (Jiang et al., 2014; Ochi et al., 2017), water deficit (Ling et al., 2015; Guo et al., 2017; Feng et al., 2018), salinity (Iranbakhsh et al., 2018), and oxidative stress (Bubler et al., 2015; Iranbakhsh et al., 2017) by plasma technology have been documented. However, validation of in vitro results through field experiments is needed to confirm the use of plasma technology in tackling the mentioned concurrent stress in onions.
Nutrient management
Soil nutrient losses due to waterlogging can cause significant impact on plant heath. Proper nutrient management is needed to mitigate the harmful effects of waterlogging and attain better crop performance (Noreen et al., 2018). In waterlogged conditions, soil nitrogen (N) is lost due to leaching, resulting in reduced uptake by plants and N deficiency in both plants and soil (Nguyen et al., 2018). Early crop vigor may be linked to enhanced nitrogen uptake (Sundgren et al., 2018). Additionally, plant growth and development can be improved with the application of slow-release N fertilizers, as they release nitrogen over a prolonged period during crop growth and make it available as per crop demand (Shaviv, 2001; Dinnes et al., 2002; Lubkowski and Grzmil, 2007; Varadachari and Goertz, 2010; Trenkel, 2021). Though there are several reports on the advantages and disadvantages of the application of N fertilizer during or immediately after waterlogging on crop yield (Swarup and Sharma, 1993; Najeeb et al., 2015; Mondal et al., 2018), the efficiency of the same in onions needs to be studied in detail.
Similarly, potassium is an essential nutrient in various physiological and biochemical plant mechanisms and can trigger abiotic and biotic stress tolerance/resistance in plants. Mitigating K+ loss due to hypoxia or anoxia stress is an important strategy for building up waterlogging resistance in plants (Mancuso and Marras, 2006; Pang et al., 2006; Mugnai et al., 2011; Teakle et al., 2013). Potassium supplementation under waterlogging stress enhances plant growth, photosynthetic pigments, rate of photosynthesis, and plant nutrient uptake (Ashraf et al., 2011). However, the efficiency of exogenous potassium application in alleviating the negative effects of waterlogging and disease incidence is variable depending on the source and amount of K, application time, and plant and pathogen species (Prabhu et al., 2007). Therefore, the role of potassium supplementation in mitigating waterlogging and anthracnose stress in onions is still unclear and needs focused research.
Use of plant growth regulators (biostimulants)
Plant bio-regulators may alleviate the harmful effects of waterlogging stress if applied at the correct growth stage (Nguyen et al., 2018; Ren et al., 2018; Wu et al., 2018). Foliar application of spermidine and spermine in Welsh onions (Allium fistulosum) stimulated biochemical and physiological adaptations during waterlogged stress (Yiu et al., 2009). However, evidence suggests an efficient use of plant bio-regulators such as 1-naphthaleneacetic acid (1-NAA), cytokinin, 6-benzyl adenine (6-BA), 5-aminolevulinic acid (ALA), glycine betaine (GB), abscisic acid (ABA), gibberellic acid, ethylene, and melatonin in enhancing waterlogging tolerance in various crops (Pandey et al., 2002; Pang et al., 2007; An et al., 2016; Ren et al., 2016, 2018; Kim et al., 2018a,b; Rasheed et al., 2018; Zhang et al., 2019). Adequate studies in this field need to be carried out in onions to depict the exact hormonal and enzymatic cross-talk, metabolic pathways, and physiological, morphological, and anatomical adaptations governing waterlogging tolerance. Further, the efficiency of growth regulators activating the onion defense mechanism under waterlogged conditions needs to be explored.
As nutrients can build up plant immunity and growth regulators help in the recovery of physiological injury, integrated application of nutrient and growth regulators at the proper crop stage could be a worthwhile strategy in ameliorating waterlogging and anthracnose stress in onion.
Biological management
The damage caused by waterlogging stress can be managed to some extent by treating plants with ACC deaminase–producing PGPR (Barnawal et al., 2012; Li et al., 2013). Pseudomonas putida UW4, Bradyrhizobium, and Enterobacter cloacae are some ACC deaminase producers that reduce the damage caused by waterlogging (Grichko and Glick, 2001; Fougnies et al., 2007; Grover et al., 2011). However, all these studies were conducted on other crops and needed to be investigated in onion. Moreover, the anthracnose management potential of these PGPR bacteria needs to be explored.
Use of fungicides
Periodic, need-based fungicide sprays are one of the promising methods for mitigating biotic stress in plants. The selection of fungicide, timing of application, and spray frequency are critical for satisfactory anthracnose management. Predictive models based on rainfall and humidity will provide the necessary directions for fungicide application. Generally, copper fungicides, dithiocarbamates, benzimidazoles, and triazoles are recommended for anthracnose management (Waller, 1992). New-generation chemicals like strobilurins are also used for anthracnose management. Although the application of fungicides in managing onion anthracnose is widely popular, it is poorly documented. The disease can be treated with protective fungicides, namely mancozeb or chlorothalonil. Spraying 10 or 15 g/20 liters of Carbendazim at 5- or 10-day intervals effectively controlled anthracnose in onions (Anonymous., 1996). Besides this, strobilurins (Quadris and Cabrio) are also effective when used in rotation with a protectant to prevent fungicide resistance (Miller, 2013).
Further, there are a few reports of C. gloeosporiodes resistance to benzimidazole in papaya and mango anthracnose (Spalding, 1982; Astua et al., 1994; Barquero and Arauz, 1996). To avoid fungicidal resistance among the pathogen population, benomyl was included in the spray schedule (Jimenez et al., 1989). Triazoles can perform a dual role as fungicides and plant growth regulators to defend plants from various stresses (Leul and Zhou, 1998, 1999; Habibzadeh et al., 2013; Rademacher, 2015). It was found that Paclobutrazol (PBZ) pre-treatment enhanced antioxidants and antioxidative enzymes in sweet potato under flooding stress (Lin et al., 2006). Paclobutrazol (PBZ) application improved waterlogging stress tolerance in Welsh onions (Yiu et al., 2008). PBZ and carbendazim can perform well and show the lowest disease severity. Additionally, PBZ alone and with benomyl showed the shortest neck elongation (Perez and Alberto, 2020). Although few triazoles are already in use in managing anthracnose in onion, their role in the mitigation of both waterlogging and disease stress needs to be validated.
Crop improvement
A cost-effective, eco-friendly, and widely adopted approach for diminishing the losses caused by waterlogging and disease is to introduce waterlogging as well as disease tolerance/resistance into existing plant varieties through breeding approaches (Zhou, 2010; Tewari and Mishra, 2018; Wani et al., 2018; Gedam et al., 2021). Preliminary screening of onion accessions against waterlogging and anthracnose was carried out at ICAR-DOGR. Among the available accessions, red onion line 1666 and DOGR Hybrid-50 were moderately resistant to both stresses (Directorate of Onion Garlic Research, 2015, 2017). Nevertheless, the tolerance to waterlogging is a complicated characteristic that is governed by several different mechanisms, such as aerenchyma formation in roots (Zhang et al., 2015; Luan et al., 2018; Pujol and Wissuwa, 2018), tolerance to secondary metabolites (Pang et al., 2006), ion toxicities (Huang et al., 2018), the maintenance of membrane potential (Gill et al., 2018), and control of ROS production under waterlogging stress, with many QTL reported to be controlling these characteristics (Li et al., 2008; Zhou, 2011; Zhang et al., 2017; Gill et al., 2018; Huang et al., 2018). Recently, omics technologies like transcriptomics, proteomics, genomics, and metagenomics have created new opportunities in crop improvement research. These technologies help us identify the key genes, traits, and metabolic pathways associated with stress tolerance in onion crops (Ghodke et al., 2020; Khandagale et al., 2020). This information will open a new avenue that will assist in developing tolerant onion varieties. Similarly, the anthracnose resistance breeding program in onions has hardly been carried out. Detecting the resistance source is a crucial step for successful disease resistance breeding. Up till today, very little research in this direction has been conducted. As per existing data, limited resistant sources are available on Allium cepa; therefore, introducing resistance genes from wild relatives will be a better option. Previous researchers found that anthracnose resistance was polygenic and could be controlled by several genes (Melo and Costa, 1983; Galvan et al., 1997). Currently, no fully resistant cultivars or wild relatives against anthracnose are available.
During pathogenesis, the pathogen may secrete enzymes that could cause rapid hydrolysis of total sugars in the host tissues, resulting in decreased resistance (Jayapal and Mahadevan, 1968). Low levels of total phenols in leaves might be the reason for decreased resistance (Arora and Wagle, 1985; Bashan, 1986; Mishra et al., 2009; Alberto, 2014). Further, cultivars with high levels of protein, sugar, and phenols may show increased resistance (Alberto et al., 2002). The success of a breeding program relies on the identification of genes and related markers for various tolerance mechanisms, which enable breeders to identify tolerant or resistant genes. Since the major part of the onion growing region is assumed to be strongly exposed to waterlogging and anthracnose stress during Kharif cultivation, emphasis should be given to the large-scale screening of resistance sources for both stresses and their utilization for the development of stress-tolerant varieties.
Conclusion and future prospects
Knowing and understanding abiotic (waterlogging) and biotic (anthracnose-twister) stress impact on rainy season onion performance is critical. Although research has pointed to individual stresses, concurrent stress possibilities should not be overlooked. Even if humidity, rainfall, temperature, and prolonged leaf wetness are key drivers for anthracnose outbreaks, waterlogging interactions are likely to significantly affect disease severity. Waterlogging impact may be either positive or negative and depends on the sequence of the stress events that should be critically analyzed. The scope of critical crop management options for enhancing host resistance should be widely explored. Exogenous application of several plant growth regulators (PGR) could be considered one of the leading methods for enhancing stress tolerance in onions. Together, foliar nutrient and growth regulator applications provide opportunities for future research. Genetic enhancement could be achieved through genetic engineering and modern molecular breeding platforms. Thus, significant attempts are required to breed onion cultivars with enhanced tolerance to stress combinations.
Author contributions
VS and SG conceived idea, designed the study, and contributed to anthracnose related portion of the manuscript. PG, BG, AP, and RK contributed to waterlogging part of the manuscript. VS, AP, BG, and RK contributed in analysis and visualizations. VS, PG, AP, BG, and SG wrote the manuscript. All authors played role in revising manuscript and approved the final version.
Funding
Project code IXX16061 Biotechnological approaches for biotic stress management.
Conflict of interest
The authors declare that the research was conducted in the absence of any commercial or financial relationships that could be construed as a potential conflict of interest.
Publisher's note
All claims expressed in this article are solely those of the authors and do not necessarily represent those of their affiliated organizations, or those of the publisher, the editors and the reviewers. Any product that may be evaluated in this article, or claim that may be made by its manufacturer, is not guaranteed or endorsed by the publisher.
References
Aguilar, E. A., Turner, D. W., and Sivasithamparam, K. (2000). Fusarium oxysporum f. sp. cubense inoculation and hypoxia alter peroxidase and phenylalanine ammonia-lyase activities in nodal roots of banana cultivars (Musa sp.) differing in their susceptibility to Fusarium wilt. Aust. J. Bot. 48, 589–596. doi: 10.1071/BT99009
Alberto, R. T. (2014). Pathological response and biochemical changes in Allium cepa L. (bulb onions) infected with anthracnose-twister disease. Plant Pathol. Quar. J. 4, 23–31. doi: 10.5943/ppq/4/1/4
Alberto, R. T., and Aquino, V. M. (2010). Characterization of Colletotrichum gloeosporioides (Penzig) Penzig and Sacc. (anthracnose) and Gibberella moniliformis Wineland (twister) infecting onions in the Philippines. Asia Life Sci. 19, 23–58.
Alberto, R. T., Duca, M. S. V., Santiago, S. E., Miller, S. A., and Black, L. L. (2001). First report of anthracnose of onion (Allium cepa L.) caused by Colletotrichum gloeosporioides (Penzig) Penzig and Sacc. in the Philippines. Trop. Plant Pathol. 37, 46–51.
Alberto, R. T., Isip, M. F., Biagtan, A. R., and Tagaca, R. C. (2019). Disease risk map of anthracnose-twister of onion based on previous disease locations as a future predictors. Spat. Inf. Res. 3, 259–265. doi: 10.1007/s41324-018-0229-4
Alberto, R. T., Santiago, S. E., Black, L. L. M., and Miller, S. A. (2002). Screening commercial onion cultivars for resistance to anthracnose. Final Report. Integrated Pest Management-Collaborative Research Support Program. Office of International Research and Development, Virginia Tech, VA. USA.
An, Y., Qi, L., and Wang, L. A. L. A. (2016). pretreatment improves waterlogging tolerance of fig plants. PLoS ONE. 11, e0147202. doi: 10.1371/journal.pone.0147202
Andrade, C. A., de Souza, K. R., de Oliveira Santos, M., da Silva, D. M., and Alves, J. D. (2018). Hydrogen peroxide promotes the tolerance of soybeans to waterlogging. Sci. Hortic. 232, 40–45. doi: 10.1016/j.scienta.2017.12.048
Anonymous. (1996). Pest Control in Tropical Onions. PANS Manual. London, UK: Tropical Development Research Centre, 109.
Arduini, I., Orlandi, C., Pampana, S., and Masoni, A. (2016). Waterlogging at tillering affects spike and spikelet formation in wheat. Crop Pasture Sci. 67, 703–711. doi: 10.1071/CP15417
Arora, Y. K., and Wagle, D. S. (1985). Interrelationship between peroxidase, polyphenol oxidase activities, and phenolic content of wheat for resistance to loose smut. Biochem. Physiol. Pflanzen 180, 75–78. doi: 10.1016/S0015-3796(85)80081-0
Ashraf, M. A., Ahmad, M. S., Ashraf, M., Al-Qurainy, F., and Ashraf, M. Y. (2011). Alleviation of waterlogging stress in upland cotton (Gossypium hirsutum L.) by exogenous application of potassium in soil and as a foliar spray. Crop Pasture Sci. 62, 25–38. doi: 10.1071/CP09225
Astua, G., Arauz Cavallini, L. F., and Umaña, G. (1994). Sensibilidad reducida al tiabendazole en Colletotrichum gloesporioides aislado de papayaReduced sensibility to thiabendazole in Colletotrichum gloesporioides isolated from papaya. Agron Costarric 18, 35–39.
Atkinson, N. J., and Urwin, P. E. (2012). The interaction of plant biotic and abiotic stresses: from genes to the field. J. Exp. Bot. 63, 3523–3543. doi: 10.1093/jxb/ers100
Bailey, J. A., O'connell, R. J., Pring, R. J., and Nash, C. (1992). “Infection strategies of Colletotrichum species,” in Colletotrichum: Biology, Pathology and Control, eds J. A. Bailey and M. J. Jeger (, Wallingford, UK: CAB International), 88–120. doi: 10.1079/9780851987569.0000
Bakker, D. M., Hamilton, G. J., Houlbrooke, D. J., and Spann, C. (2005). The effect of raised beds on soil structure, waterlogging, and productivity on duplex soils in Western Australia. Soil Res. 43, 575–585. doi: 10.1071/SR03118
Bange, M. P., Milroy, S. P., and Thongbai, P. (2004). Growth and yield of cotton in response to waterlogging. Field Crops Res. 88, 129–142. doi: 10.1016/j.fcr.2003.12.002
Barnawal, D., Bharti, N., Maji, D., and Chanotiya, C. S. (2012). and Kalra A. 1-Aminocyclopropane-1-carboxylic acid (ACC) deaminase-containing rhizobacteria protect Ocimum sanctum plants during waterlogging stress via reduced ethylene generation. Plant Physiol. Biochem. 58, 227–223. doi: 10.1016/j.plaphy.2012.07.008
Barquero, C., and Arauz, L. F. (1996). Variacion de la sensibilidad a benomil en poblaciones de Colletotrichum gloeosporioides aislado de mango en Costa Rica. Memorias IX Congress Nacional Agron. Recursos Naturales, III Congress Nacional Fitopatol. San Jose, Costa Rica: II Congress Nacional Suelos, Vol. 2, 133.
Bashan, Y. (1986). Phenols in cotton seedlings resistant and susceptible to Alternaria macrospora. J. Phytopathol. 116, 1–10. doi: 10.1111/j.1439-0434.1986.tb00888.x
Baysal-Gurel, F., Subedi, N., Mamiro, D. P., and Miller, S. A. (2014). First report of Anthracnose of onion caused by Colletotrichum coccodes in Ohio. Plant Dis. 98, 1271. doi: 10.1094/PDIS-01-14-0032-PDN
Belford, R. K., Dracup, M., and Tennant, D. (1992). Limitations to growth and yield of cereal and lupin crops on duplex soils. Aust. J. Exp. Agric. 32, 929–945. doi: 10.1071/EA9920929
Blessitt, J. B. (2007). Productivity of raised seedbeds for soybean [Glycine max.(L.) Merr.] production on clayey soils of the Mississippi delta (PhD thesis). Mississippi State University, Mississippi.
Bubler, S., Herppich, W. B., Neugart, S., Schreiner, M., Ehlbeck, J., Rohn, S., et al. (2015). Impact of cold atmospheric pressure plasma on physiology and flavonol glycoside profile of peas (Pisum sativum ‘Salamanca'). Food Res. Int. 76, 132–142. doi: 10.1016/j.foodres.2015.03.045
Chawda, H. T., and Rajasab, A. H. (1996). Onion anthracnose disease symptoms: a review. Onion Newslett. Trop. 7, 82–84.
Chen, A., Najeeb, S., Wang, Y., Khan, R. A., Ping, X., Ling, J., et al. (2022). First report of Colletotrichum circinans causing anthracnose in Allium fistulosum var. giganteum in Gansu Province, China. Plant Dis. 106, 1985. doi: 10.1094/PDIS-09-21-2011-PDN
Chojak-Kozniewska, J., Kuzniak, E., and Zimny, J. (2018). The effects of combined abiotic and pathogen stress in plants: insights from salinity and Pseudomonas syringae pv lachrymans interaction in cucumber. Front. Plant Sci. 9, 1691. doi: 10.3389/fpls.2018.01691
Chowdappa, P., Chethana, C. S., and Pavani, K. V. (2015). Colletotrichum siamense and C. truncatum are responsible for severe outbreaks of anthracnose on onion in southwest India. J. Plant Pathol. 97, 77–86. doi: 10.4454/JPP.V97I1.015
Damm, U., Cannon, P. F., Woudenberg, J. H., and Crous, P. W. (2012). The Colletotrichum acutatum species complex. Stud. Mycol. 73, 37–113. doi: 10.3114/sim0010
Darvas, J. M., and Kotze, J. M. (1987). Avocado fruit diseases and their control in South Africa. South Afr. Avocado Growers Assoc. Yearbook 10, 117–119.
Denham, T. G., and Waller, J. M. (1981). Some epidemiological aspects of post-bloom fruit drop disease (Colletotrichum gloeosporioides) in citrus. Ann. Appl. Biol. 98, 65–77. doi: 10.1111/j.1744-7348.1981.tb00423.x
Dillard, H. R. (1989). Effect of temperature, wetness duration, and inoculum density on infection and lesion development of Colletotrichum coccodes on tomato fruit. Phytopathology 79, 1063–1066. doi: 10.1094/Phyto-79-1063
Dillard, H. R. (1992). “Colletotrichum coccodes: the pathogen and its hosts,” in Colletotrichum: Biology, Pathology and Control, eds J. A. Bailey and M. J. Jeger (Wallingford, UK: CAB International), 225–36.
Dinnes, D. L., Karlen, D. L., Jaynes, D. B., Kaspar, T. C., Hatfield, J. L., Colvin, T. S., et al. (2002). Nitrogen management strategies to reduce nitrate leaching in tile-drained Midwestern soils. Agronomy 94, 153–171. doi: 10.2134/agronj2002.1530
Directorate of Onion and Garlic Research. (2015). Annual Report 2015–2016. Rajgurunagar, India: ICAR-DOGR.
Directorate of Onion and Garlic Research. (2017). Annual Report 2017–2018. Rajgurunagar, India: ICAR-DOGR.
Drinkwater, W. O., and Janes, B. E. (1955). “Effects of irrigation and soil moisture on maturity, yield and storage of two onion hybrids,” in: Proceedings of the American Society for Horticultural Science (American Society for Horticultural Science), Vol. 66, 267–278.
Dubey, S., Kuruwanshi, V. B., Bhagat, K. P., and Ghodke, P. H. (2021). Impact of excess moisture in onion genotypes (Allium cepa L.) under climate change scenario. Int. J. Curr. Microbiol. Appl. Sci. 10, 166–175.
Dubey, S., Kuruwanshi, V. B., Ghodke, P. H., and Mahajan, V. (2020). Biochemical and yield evaluation of onion (Allium cepa L.) genotypes under waterlogging condition. Int. J. Chem. Stud. 8, 2036–2040. doi: 10.22271/chemi.2020.v8.i4v.9926
Duncan, J. M., and Kennedy, D. M. (1989). The effect of waterlogging on Phytophthora root rot of red raspberry. Plant Pathol. 38, 161–168. doi: 10.1111/j.1365-3059.1989.tb02129.x
Ebenebe, A. C. (1980a). Search of the causal agent of the'onion twister'disorder. Samaru Miscell. Paper. 96, 1–16.
Ebenebe, A. C. (1980b). Onion twister disease caused by Glomerella cingulata in northern Nigeria. Plant Dis. 64, 1030–1032. doi: 10.1094/PD-64-1030
FAOSTAT (2019). Food and Agriculture Organization of the United Nations. Production: Crops. Available online at: http://faostat.fao.org (accessed on 29 June, 2022).
Feng, J., Wang, D., Shao, C., Zhang, L., and Tang, X. (2018). Effects of cold plasma treatment on alfalfa seed growth under simulated drought stress. Plasma Sci. Technol. 20, 035505. doi: 10.1088/2058-6272/aa9b27
Ferrer, J. L. R., Magalhaes, P. C., Alves, J. D., Vasconcellos, C. A., Delu Filho, N., Fries, D. D., et al. (2005). Calcium partially relieves the deleterius effects of hypoxia on a maize cultivar selected for waterlogging tolerance. Rev. Brasil. Milho e Sorgo Sete Lagoas. 4, 381–89. 4, 381–389. doi: 10.18512/1980-6477/rbms.v4n3p381-389
Fitzell, R. D., and Peak, C. M. (1984). The epidemiology of anthracnose disease of mango: inoculum sources, spore production and dispersal. Ann. Appl. Biol. 104, 53–59. doi: 10.1111/j.1744-7348.1984.tb05586.x
Fougnies, L., Renciot, S., Muller, F., Plenchette, C., Prin, Y., De Faria, S. M., et al. (2007). Arbuscular mycorrhizal colonization and nodulation improve flooding tolerance in Pterocarpus officinalis Jacq. seedlings. Mycorrhiza. 17, 159–166. doi: 10.1007/s00572-006-0085-2
Freeman, S., Minz, D., Jurkevitch, E., Maymon, M., and Shabi, E. (2000). Molecular analyses of Colletotrichum species from almond and other fruits. Phytopathology 90, 608–614. doi: 10.1094/PHYTO.2000.90.6.608
Gadge, S. S., and Lawande, K. E. (2012). Crop damage due to climatic change: a major constraint in onion farming. Indian Res J. Extension Educ. II Special. 2, 38–41.
Galvan, G. A., Wietsma, W. A., Putrasemedja, S., Permadi, A. H., and Kik, C. (1997). Screening for resistance to anthracnose (Colletotrichum gloeosporioides Penz.) in Allium cepa and its wild relatives. Euphytica 95, 173–178. doi: 10.1023/A:1002914225154
Gedam, P. A., Thangasamy, A., Shirsat, D. V., Ghosh, S., Bhagat, K. P., Sogam, O. A., et al. (2021). Screening of onion (Allium cepa L.) genotypes for drought tolerance using physiological and yield based indices through multivariate analysis. Front. Plant Sci. 12, 600371. doi: 10.3389/fpls.2021.600371
Ghodke, P., Khandagale, K., Thangasamy, A., Kulkarni, A., Narwade, N., Shirsat, D., et al. (2020). Comparative transcriptome analyses in contrasting onion (Allium cepa L.) genotypes for drought stress. PLoS ONE. 15, e0237457. doi: 10.1371/journal.pone.0237457
Ghodke, P. H., Shirsat, D. V., Thangasamy, A., Mahajan, V., Salunkhe, V. N., Khade, Y., et al. (2018). Effect of water logging stress at specific growth stages in onion crop. Int. J. Curr. Microbiol. Appl. Sci. 7, 3438–3448. doi: 10.20546/ijcmas.2018.701.405
Gill, M. B., Zeng, F., Shabala, L., Böhm, J., Zhang, G., Zhou, M., et al. (2018). The ability to regulate voltage-gated K+-permeable channels in the mature root epidermis is essential for waterlogging tolerance in barley. J. Expt. Bot. 69, 667–680. doi: 10.1093/jxb/erx429
Gopal, J. (2015). Onion research in India: Status and challenges. Progressive Horticulture. 47, 1–19. doi: 10.5958/2249-5258.2015.00001.9
Govaerts, B., Sayre, K. D., Lichter, K., Dendooven, L., and Deckers, J. (2007). Influence of permanent raised bed planting and residue management on physical and chemical soil quality in rain fed maize/wheat systems. Plant Soil. 291, 39–54. doi: 10.1007/s11104-006-9172-6
Graham, R. D. (1983). “Effects of nutrient stress on susceptibility of plants to disease with particular reference to the trace elements,” in Advances in Botanical Research, Vol. 10 (Glen Osmond: Academic Press), 221–276. doi: 10.1016/S0065-2296(08)60261-X
Greenway, H., Armstrong, W., and Colmer, T. D. (2006). Conditions leading to high CO2 (>5kPa) in waterlogged–flooded soils and possible effects on root growth and metabolism. Ann. Bot. 98, 9–32. doi: 10.1093/aob/mcl076
Gregory, P. H., Guthrie, E. J., and Bunce, M. E. (1959). Experiments on splash dispersal of fungus spores. Microbiology 20, 328–354. doi: 10.1099/00221287-20-2-328
Grichko, V. P., and Glick, B. R. (2001). Amelioration of flooding stress by ACC deaminase-containing plant growth-promoting bacteria. Plant Physiol. Biochem. 39, 11–17. doi: 10.1016/S0981-9428(00)01212-2
Grover, M., Ali, S. Z., Sandhya, V., Rasul, A., and Venkateswarlu, B. (2011). Role of microorganisms in adaptation of agriculture crops to abiotic stresses. World J. Microbiol. Biotechnol. 27, 1231–1240. doi: 10.1007/s11274-010-0572-7
Guo, Q., Wang, Y., Zhang, H., Qu, G., Wang, T., Sun, Q., et al. (2017). Alleviation of adverse effects of drought stress on wheat seed germination using atmospheric dielectric barrier discharge plasma treatment. Sci. Rep. 7, 1–4. doi: 10.1038/s41598-017-16944-8
Habibzadeh, F., Sorooshzadeh, A., Pirdashti, H., and Modarres-Sanavy, S. A. (2013). Alleviation of waterlogging damage by foliar application of nitrogen compounds and tricyclazole in canola. Aust. J. Crop Sci. 7, 401–406. doi: 10.3316/informit.261180914141065
Hay, F. S., Strickland, D., Maloney, E., Hoepting, C., and Pethybridge, S. J. (2016). Anthracnose of onion caused by Colletotrichum coccodes in New York. Plant Dis. 100, 2171. doi: 10.1094/PDIS-05-16-0607-PDN
Herath, I. S., Udayanga, D., Miriyagalla, S., Castlebury, L. A., and Manamgoda, D. S. (2021). Colletotrichum siamense causing anthracnose-twister disease of onion (Allium cepa) in Sri Lanka. Australas. Plant Dis. Notes 16, 1–6. doi: 10.1007/s13314-021-00444-w
Herzog, M., Striker, G. G., Colmer, T. D., and Pedersen, O. (2016). Mechanisms of waterlogging tolerance in wheat–a review of root and shoot physiology. Plant Cell Environ. 39, 1068–1086. doi: 10.1111/pce.12676
Hsu, F. C., Chou, M. Y., Chou, S. J., Li, Y. R., Peng, H. P., Shih, M. C., et al. (2013). Submergence confers immunity mediated by the WRKY22 transcription factor in Arabidopsis. Plant Cell. 25, 2699–2713. doi: 10.1105/tpc.113.114447
Huang, X., Fan, Y., Shabala, L., Rengel, Z., Shabala, S., Zhou, M. X. A., et al. (2018). major QTL controlling the tolerance to manganese toxicity in barley (Hordeum vulgare L.). Mol Breeding 38, 1–9. doi: 10.1007/s11032-017-0767-9
Iglesias, D. J., Lliso, I., Tadeo, F. R., and Talon, M. (2002). Regulation of photosynthesis through source: sink imbalance in citrus is mediated by carbohydrate content in leaves. Physiol. Plant. 116, 563–572. doi: 10.1034/j.1399-3054.2002.1160416.x
Intergovernmental Panel on Climate Change (IPCC). (2014). Climate Change 2014. Synthesis Report. Contribution of working groups I, II and III to the fifth assessment report of the Intergovernmental Panel on Climate Change. Geneva: IPCC.
Iranbakhsh, A., Ardebili, N. O., Ardebili, Z. O., Shafaati, M., and Ghoranneviss, M. (2018). Non-thermal plasma induced expression of heat shock factor A4A and improved wheat (Triticum aestivum L.) growth and resistance against salt stress. Plasma Chem. Plasma Process. 38, 29–44. doi: 10.1007/s11090-017-9861-3
Iranbakhsh, A., Ghoranneviss, M., Oraghi Ardebili, Z., Oraghi Ardebili, N., Hesami Tackallou, S., Nikmaram, H., et al. (2017). Non-thermal plasma modified growth and physiology in Triticum aestivum via generated signaling molecules and UV radiation. Biol. Plant. 61, 702–708. doi: 10.1007/s10535-016-0699-y
Jackson, M. B., and Colmer, T. (2005). Response and adaptation by plants to flooding stress. Ann. Bot. 96, 501–505. doi: 10.1093/aob/mci205
Jayapal, R., and Mahadevan, A. (1968). Biochemical changes in banana leaves in response to leaf spot pathogens. IndiaFn Phytopathol. 21, 43–48.
Jiang, J., Lu, Y., Li, J., Li, L., He, X., Shao, H., et al. (2014). Effect of seed treatment by cold plasma on the resistance of tomato to Ralstonia solanacearum (bacterial wilt). PLoS ONE. 9, e97753. doi: 10.1371/journal.pone.0097753
Jimenez, R., Hernandez, R. L., and Arauz, L. F. (1989). Prueba de fungicidas para prevenir enfermedades en el fruto del mango (Mangifera indica L.). Bol. Tec. Estacion Exp. Fabio Baudrit 22, 11–20.
Jolly, E., Johnson, P., Glen, M., and Barry, K. (2017). “Interactions of waterlogging and fungal infection in lentil (Lens culinaris) cultivars,” in Science Protecting Plant Health, Brisbane, Australia, 161.
Khandagale, K., Krishna, R., Roylawar, P., Ade, A. B., Benke, A., Shinde, B., et al. (2020). Omics approaches in Allium research: progress and way ahead. Peer J. 8, e9824. doi: 10.7717/peerj.9824
Kiehr, M., Delhey, R., and Azpilicueta, A. (2012). Smudge and other diseases of onion caused by Colletotrichum circinans, in southern Argentina. Phyton 81, 161–164. doi: 10.32604/phyton.2012.81.161
Kim, W. G., Hong, S. K., and Kim, J. H. (2008). Occurrence of anthracnose on welsh onion caused by Colletotrichum circinans. Mycobiology 36, 274–276. doi: 10.4489/MYCO.2008.36.4.274
Kim, Y., Seo, C. W., Khan, A. L., Mun, B. G., Shahzad, R., Ko, J. W., et al. (2018a). Ethylene mitigates waterlogging stress by regulating glutathione biosynthesis-related transcripts in soybeans. bioRxiv. 252312. doi: 10.1101/252312
Kim, Y., Seo, C. W., Khan, A. L., Mun, B. G., Shahzad, R., Ko, J. W., et al. (2018b). Exo-ethylene application mitigates waterlogging stress in soybean (Glycine max L.). BMC Plant Biol. 18, 254. doi: 10.1186/s12870-018-1457-4
Kreuzwieser, J., and Rennenberg, H. (2014). Molecular and physiological responses of trees to waterlogging stress. Plant Cell Environ. 37, 2245–2259. doi: 10.1111/pce.12310
Kuai, J., Liu, Z., Wang, Y., Meng, Y., Chen, B., Zhao, W., et al. (2014). Waterlogging during flowering and boll forming stages affects sucrose metabolism in the leaves subtending the cotton boll and its relationship with boll weight. Plant Sci. 223, 79–98. doi: 10.1016/j.plantsci.2014.03.010
Kuruppu, P. U. (1999). First report of Fusarium oxysporum causing a leaf twisting disease on Allium cepa var. ascalonicum in Sri Lanka. Plant Dis. 83, 695–698. doi: 10.1094/PDIS.1999.83.7.695C
Lawyer, A. S. (1985). “Diseases caused by Ascochyta spp.,” in Compendium of Pea Diseases, ed D. J. Hagedorn (St Paul, MN, USA, American Phytopathological Society), 11–15.
Lax, A. R., Templeton, G. E., and Meyer, W. L. (1985). Isolation, purification, and biological activity of a self-inhibitor from conidia of Colletotrichum gloeosporioides. Phytopathology 75, 386–390. doi: 10.1094/Phyto-75-386
Laxman, R. H., Upreti, K. K., Shivashankara, K. S., Sadashiva, A. T., Madhavi Reddy, K., Aghora, T. S., et al. (2020). “Management strategies for alleviating abiotic stresses in vegetable crops,” in New Frontiers in Stress Management for Durable Agriculture (Singapore: Springer), 523–542. doi: 10.1007/978-981-15-1322-0_27
Leul, M., and Zhou, W. (1998). Alleviation of waterlogging damage in winter rape by application of uniconazole: effects on morphological characteristics, hormones and photosynthesis. Field Crops Res. 59, 121–127. doi: 10.1016/S0378-4290(98)00112-9
Leul, M., and Zhou, W. J. (1999). Alleviation of waterlogging damage in winter rape by uniconazole application: effects on enzyme activity, lipid peroxidation, and membrane integrity. J. Plant Growth Regul. 18, 9–14. doi: 10.1007/PL00007046
Li, H., Vaillancourt, R., Mendham, N., and Zhou, M. (2008). Comparative mapping of quantitative trait loci associated with waterlogging tolerance in barley (Hordeum vulgare L.). BMC Genom. 9, 401. doi: 10.1186/1471-2164-9-401
Li, J., McConkey, B. J., Cheng, Z., Guo, S., and Glick, B. R. (2013). Identification of plant growth-promoting bacteria-responsive proteins in cucumber roots under hypoxic stress using a proteomic approach. J. Proteom. 84, 119–131. doi: 10.1016/j.jprot.2013.03.011
Li, Y. P., You, M. P., Colmer, T. D., and Barbetti, M. J. (2015). Effect of timing and duration of soil saturation on soilborne Pythium diseases of common bean (Phaseolus vulgaris). Plant Dis. 99, 112–118. doi: 10.1094/PDIS-09-13-0964-RE
Lin, K. H., Tsou, C. C., Hwang, S. Y., Chen, L. F., and Lo, H. F. (2006). Paclobutrazol pre-treatment enhanced flooding tolerance of sweet potato. J. Plant Physiol. 163, 750–760. doi: 10.1016/j.jplph.2005.07.008
Ling, L., Jiangang, L., Minchong, S., Chunlei, Z., and Yuanhua, D. (2015). Cold plasma treatment enhances oilseed rape seed germination under drought stress. Sci. Rep. 5, 1–10. doi: 10.1038/srep13033
Lopes, L. H., Boiteux, L. S., Rossato, M., Aguiar, F. M., Fonseca, M. E., Oliveira, V. R., et al. (2021). Diversity of Colletotrichum species causing onion anthracnose in Brazil. Eur. J. Plant Pathol. 159, 339–357. doi: 10.1007/s10658-020-02166-8
Luan, H., Guo, B., Pan, Y., Lv, C., Shen, H., Xu, R., et al. (2018). Morpho-anatomical and physiological responses to waterlogging stress in different barley (Hordeum vulgare L.) genotypes. Plant Growth Regul. 85, 399–409. doi: 10.1007/s10725-018-0401-9
Lubkowski, K., and Grzmil, B. (2007). Controlled release fertilizers. Pol. J. Chem. Technol. 9, 81–84. doi: 10.2478/v10026-007-0096-6
Madushani, R. L., Vengadaraman, A., and De Costa, D. M. (2012). “Pathogenic variations of Colletotrichum spp. causing the leaf twister disease of onion,” in Book of Abstracts of the Peradeniya University Research Sessions, Sri Lanka, 17.
Mancuso, S., and Marras, A. M. (2006). Adaptative response of Vitis root to anoxia. Plant Cell Physiol. 47, 401–409. doi: 10.1093/pcp/pcj007
McDonald, G. K., and Dean, G. (1996). Effect of waterlogging on the severity of disease caused by Mycosphaerella pinodes in peas (Pisum sativum L.). Aust. J. Exp. Agric. 36, 219–222. doi: 10.1071/EA9960219
Melo, I. G., and Costa, C. P. (1983). Estimativas de parametros geneticos em cebola (Allium cepa L.) populac,oes Pira Rosa e Pira Tropical, para o car'ater resistencia a Colletotrichum gloeosporioides Penz. Sensu ARX, 1957. Summa Phytopathol. 9, 207–13.
Mercure, E. W., Kunoh, H., and Nicholson, R. L. (1994a). Adhesion of Colletotrichum graminicola conidia to corn leaves, a requirement for disease development. Physiol. Mol. Plant Pathol. 45, 407–420. doi: 10.1016/S0885-5765(05)80039-6
Mercure, E. W., Leite, B., and Nicholson, R. L. (1994b). Adhesion of ungerminated conidia of Colletotrichum graminicola to artificial hydrophobic surfaces. Physiol. Mol. Plant Pathol. 45, 421–440. doi: 10.1016/S0885-5765(05)80040-2
Mishra, K. K., Kolte, S. J., Nashaat, N. I., and Awasthi, R. P. (2009). Pathological and biochemical changes in Brassica juncea (mustard) infected with Albugo candida (white rust). Plant Pathol. 58, 80–86. doi: 10.1111/j.1365-3059.2008.01939.x
Mondal, T., Mitra, B., and Das, S. (2018). Precision nutrient management in wheat (Triticum aestivum) using nutrient expert®: growth phenology, yield, nitrogen-use efficiency and profitability under eastern sub-Himalayan plains. Indian J. Agron 63, 174–180.
Mordue, J. E. (1971). Colletotrichum lindemuthianum. [Descriptions of Fungi and Bacteria]. CMI descriptions of pathogenic fungi and bacteria. doi: 10.1079/DFB/20056400316
Mugnai, S., Marras, A. M., and Mancuso, S. (2011). Effect of hypoxic acclimation on anoxia tolerance in Vitis roots: response of metabolic activity and K+ fluxes. Plant Cell Physiol. 52, 1107–1116. doi: 10.1093/pcp/pcr061
Muhie, S. H., Yildirim, E., Memis, N., and Demir, I. (2020). Vermicompost priming stimulated germination and seedling emergence of onion seeds against abiotic stresses. Seed Sci. Technol. 48, 153–157. doi: 10.15258/sst.2020.48.2.02
Murray, G. M., Martin, R. H., and Cullis, B. R. (1990). Relationship of the severity of Septoria tritici blotch of wheat to sowing time, rainfall at heading and average susceptibility of wheat cultivars in the area. Aust. J. Agric. Res. 41, 307–315. doi: 10.1071/AR9900307
Najeeb, U., Bange, M. P., Tan, D. K., and Atwell, B. J. (2015). Consequences of waterlogging in cotton and opportunities for mitigation of yield losses. AoB Plants 7, plv080. doi: 10.1093/aobpla/plv080
Nguyen, H. C., Lin, K. H., Ho, S. L., Chiang, C. M., and Yang, C. M. (2018). Enhancing the abiotic stress tolerance of plants: from chemical treatment to biotechnological approaches. Physiol. Plant. 164, 452–466. doi: 10.1111/ppl.12812
Nicholson, R. L. (1992). “Colletotrichum graminicola and the anthracnose diseases of maize and sorghum,” in Colletotrichum. Biology, Pathology and Control, eds J. A. Bailey and M. J. Jeger (Wallingford, UK: CABI), 186–202.
Nicholson, R. L. (1996). “Adhesion of fungal propagules,” in Histology, Ultrastructure and Molecular Cytology of Plant-Microorganism Interactions (Dordrecht: Springer), 117–134. doi: 10.1007/978-94-009-0189-6_7
Nischwitz, C., Langston, D. Jr., Sanders, H. F., Torrance, R., Lewis, K. J., Gitaitis, R. D., et al. (2008). First report of Colletotrichum gloeosporioides causing ‘twister disease'of onion (Allium cepa) in Georgia. Plant Dis. 92, 974. doi: 10.1094/PDIS-92-6-0974C
Noreen, S., Fatima, Z., Ahmad, S., Athar, H. U., and Ashraf, M. (2018). “Foliar application of micronutrients in mitigating abiotic stress in crop plants,” in Plant Nutrients and Abiotic Stress Tolerance (Singapore: Springer), 95–117. doi: 10.1007/978-981-10-9044-8_3
Ochi, A., Konishi, H., Ando, S., Sato, K., Yokoyama, K., Tsushima, S., et al. (2017). Management of bakanae and bacterial seedling blight diseases in nurseries by irradiating rice seeds with atmospheric plasma. Plant Pathol. 66, 67–76. doi: 10.1111/ppa.12555
Panday, S. S., Alberto, R. T., and Labe, M. S. (2012). Ultrastructural characterization of infection and colonization of Colletotrichum gloeosporioides in onion. Plant Pathol. Quar. J. 2, 168–177. doi: 10.5943/ppq/2/2/10
Pandey, D. M., Goswami, C. L., Kumar, B., and Jain, S. (2002). Effect of growth regulators on photosynthetic metabolites in cotton under water stress. Biol. Plant. 45, 445–448. doi: 10.1023/A:1016286121451
Pang, J., Ross, J., Zhou, M., Mendham, N., and Shabala, S. (2007). Amelioration of detrimental effects of waterlogging by foliar nutrient sprays in barley. Funct. Plant Biol. 34, 221–227. doi: 10.1071/FP06158
Pang, J. Y., Newman, I. A., Mendham, N., Zhou, M., and Shabala, S. (2006). Microelectrode ion and O2 fluxes measurements reveal differential sensitivity of barley root tissues to hypoxia. Plant Cell Environ. 29, 1107–1121. doi: 10.1111/j.1365-3040.2005.01486.x
Perez, P. M., and Alberto, R. T. (2020). Chemical management of anthracnose-twister (Colletotrichum gloeosporioides and Fusarium fujikuroi) disease of onion (Allium cepa). Plant Pathol. Quar. J. 10, 198–216. doi: 10.5943/ppq/10/1/19
Prabhu, A. S., Fageria, N. K., and Huber, D. M. (2007). “Potassium nutrition and plant diseases,” in Mineral Nutrition and Plant Disease, eds L. E. Datnoff, W. H. Elmer, D. M. Huber [Saint Paul: American Phytopathological Society (APS Press)], 57–78.
Pujol, V., and Wissuwa, M. (2018). Contrasting development of lysigenous aerenchyma in two rice genotypes under phosphorus deficiency. BMC Res. Notes. 11, 60. doi: 10.1186/s13104-018-3179-y
Qadri, S. M. H. (1988). Two onion diseases new to Andhra Pradesh and Karnataka. Indian Phytopathol. 41, 293.
Qadri, S. M. H., and Srivastava, K. J. (1985). “Leaf blight of onion (Allium cepa L.) caused by Colletotrichum gloeosporioides Penz. and Sacc. Hitherto unrecorded from India,” in Proceedings of 72nd Indian Science Congress Association, Lucknow, 74–75.
Rademacher, W. (2015). Plant growth regulators: backgrounds and uses in plant production. J. Plant Growth Regul. 34, 845–872. doi: 10.1007/s00344-015-9541-6
Rajasab, A. H., and Chawda, H. T. (1994). Dispersal of the conidia of Colletotrichum gloeosporioides by rain and the development of anthracnose on onion. Grana 33, 162–165. doi: 10.1080/00173139409428994
Raniere, L. C., and Crossan, D. F. (1959). The influence of overhead irrigation and microclimate on Colletotrichum phomoides. Phytopathology 49, 72–74.
Rao, N. S. (2016). “Onion,” in Abiotic Stress Physiology of Horticultural Crops, eds N. S. Rao, K. S. Shivashankara, R. H. Laxman (New Delhi: Springer), 133–149. doi: 10.1007/978-81-322-2725-0_8
Rao, R., and Li, Y. (2003). Management of flooding effects on growth of vegetable and selected field crops. Horttechnology 13, 610–616. doi: 10.21273/HORTTECH.13.4.0610
Rasheed, R., Iqbal, M., Ashraf, M. A., Hussain, I., Shafiq, F., Yousaf, A., et al. (2018). Glycine betaine counteracts the inhibitory effects of waterlogging on growth, photosynthetic pigments, oxidative defence system, nutrient composition, and fruit quality in tomato. J. Hortic. Sci. Biotechnol. 93, 385–391. doi: 10.1080/14620316.2017.1373037
Ren, B., Zhang, J., Dong, S., Liu, P., and Zhao, B. (2018). Exogenous 6-benzyladenine improves antioxidative system and carbon metabolism of summer maize waterlogged in the field. J. Agron Crop. Sci. 204, 175–184. doi: 10.1111/jac.12253
Ren, B., Zhu, Y., Zhang, J., Dong, S., Liu, P., Zhao, B., et al. (2016). Effects of spraying exogenous hormone 6-benzyladenine (6-BA) after waterlogging on grain yield and growth of summer maize. Field Crops Res. 188, 96–104. doi: 10.1016/j.fcr.2015.10.016
Roberts, S. J. (1992). Effect of soil moisture on the transmission of pea bacterial blight (Pseudomonas syringae pv. pisi) from seed to seedling. Plant Pathol. 41, 136–140. doi: 10.1111/j.1365-3059.1992.tb02330.x
Rodriguez-Salamanca, L. M., Enzenbacher, T. B., Derie, M. L., du Toit, L. J., Feng, C., Correll, J. C., et al. (2012). First report of Colletotrichum coccodes causing leaf and neck anthracnose on onions (Allium cepa) in Michigan and the United States. Plant Dis. 96, 769. doi: 10.1094/PDIS-01-12-0022-PDN
Rodriguez-Salamanca, L. M., Naegele, R. P., Quesada-Ocampo, L. M., and Hausbeck, M. K. (2018). Inoculation method, temperature, and relative humidity affect leaf and neck anthracnose, a new onion disease in Michigan. Plant Health Prog. 19, 64–68. doi: 10.1094/PHP-10-17-0063-RS
Saijo, Y., and Loo, E. P. (2020). Plant immunity in signal integration between biotic and abiotic stress responses. New Phytol. 225, 87–104. doi: 10.1111/nph.15989
Salunkhe, V. N., Anandhan, S., Gawande, S. J., Ikkar, R. B., Bhagat, Y. S., Mahajan, V., et al. (2018a). First report of Colletotrichum truncatum causing anthracnose of mouse garlic (Allium angulosum) in India. Plant Dis. 102, 240. doi: 10.1094/PDIS-04-17-0566-PDN
Salunkhe, V. N., Anandhan, S., Gawande, S. J., Ikkar, R. B., Bhagat, Y. S., Mahajan, V., et al. (2018b). First report of Anthracnose caused by Colletotrichum spaethianum on Allium ledebourianum in India. Plant Dis. 102, 2031. doi: 10.1094/PDIS-01-18-0104-PDN
Samra, J. S., Ramakrishna, Y. S., Desai, S., Subba Rao, A. V., Rama Rao, C. A., Reddy, Y. V., et al. (2006). Impact of excess rains on yield, market availability and prices of onion. Information Bulletin. Hyderabad: Central Research Institute for Dryland Agriculture (ICAR), 52.
Sanogo, S., El-Sebai, O. I., and Sanderson, R. (2008). Severity of Verticillium wilt, plant growth, and spectral reflectance indices of chile pepper under periodic flooding and no-flooding conditions. Hort Sci. 43, 414–419. doi: 10.21273/HORTSCI.43.2.414
Sanogo, S., and Pennypacker, S. P. (1997). Factors affecting sporogenic and myceliogenic germination of sclerotia of Colletotrichum coccodes. Plant Dis. 81, 333–336. doi: 10.1094/PDIS.1997.81.4.333
Santana, K. F., Garcia, C. B., Matos, K. S., Hanada, R. E., da Silva, G. F., and Sousa, N. R. (2015). NR. First report of Anthracnose caused by Colletotrichum spaethianum on Allium fistulosum in Brazil. Plant Dis. 100, 224. doi: 10.1094/PDIS-07-15-0737-PDN
Sharma, M., and Kulshrestha, S. (2015). Colletotrichum gloeosporioides: an anthracnose causing pathogen of fruits and vegetables. Biosci. Biotechnol. Res. Asia 12, 1233–1246. doi: 10.13005/bbra/1776
Shaviv, A. (2001). Advances in controlled-release fertilizers. Adv Agron 71, 1–49. doi: 10.1016/S0065-2113(01)71011-5
Shivas, R. G., Wood, P. M., Darcey, M. W., and Peg, K. G. (1995). First record of Fusarium oxysporum f. sp. cubense on Cavendish bananas in Western Australia. Aust. Plant Pathol. 24, 38–43. doi: 10.1071/APP9950038
Sikirou, R., Beed, F., Hotègni, J., Winter, S., Assogba-Komlan, F., Reeder, R., et al. (2011). First report of anthracnose caused by Colletotrichum gloeosporioides on onion (Allium cepa) in Benin. New Dis. Rep. 23, 583–584. doi: 10.5197/j.2044-0588.2011.023.007
Singh, A., and Sinha, J. (1994). Severe curls or twister disease of onion in India. Indian Phytopathol. 47, 214.
Smith, K. A. (1987). “The effects of waterlogging on root growth and on symbiotic nitrogen fixation by legumes,” in ‘Adaptation of chickpea and pidgeon pea to abiotic stresses' Proceedings of the Consultant's Workshop, 19-21 December 1984, eds N. P. Saxena and C. Johansen (Patancheru, India: ICRISAT), 77–89.
Song, J. S., Kim, S. B., Ryu, S., Oh, J., and Kim, D. S. (2020). Emerging plasma technology that alleviates crop stress during the early growth stages of plants: a review. Front. Plant Sci. 11, 988. doi: 10.3389/fpls.2020.00988
Sorrenti, G., Tacconi, G., and Tosi, L. (2019). Avanza la “moria del kiwi”: evoluzione e primi riscontri della ricerca. Riv. Frutticoltura Ortofloricoltura 83, 34–43.
Spalding, D. H. (1982). Resistance of mango pathogens to fungicides used to control postharvest diseases. Plant Dis. 66, 1185–1186. doi: 10.1094/PD-66-1185
Srinivas, P. S., and Lawande, K. E. (2007). Seedling root dip method for protecting onion plants from thrips. Indian J. Plant Prot. 35, 206–209.
Sundgren, T. K., Uhlen, A. K., Lillemo, M., Briese, C., and Wojciechowski, T. (2018). Rapid seedling establishment and a narrow root stele promotes waterlogging tolerance in spring wheat. J. Plant Physiol. 227, 45–55. doi: 10.1016/j.jplph.2018.04.010
Swarup, A., and Sharma, D. P. (1993). Influence of top-dressed nitrogen in alleviating adverse effects of flooding on growth and yield of wheat in a sodic soil. Field Crops Res. 35, 93–100. doi: 10.1016/0378-4290(93)90142-A
Tacconi, G., Paltrinieri, S., Mejia, J. F., Fuentealba, S. P., Bertaccini, A., Tosi, L., et al. (2015). Vine decline in kiwifruit: climate change and effect on waterlogging and Phytophthora in North Italy. Acta Hortic. 93–97. doi: 10.17660/ActaHortic.2015.1096.7
Taylor, A. G., Allen, P. S., Bennett, M. A., Bradford, K. J., Burris, J. S., Misra, M. K., et al. (1998). Seed enhancements. Seed Sci. Res. 8, 245–256. doi: 10.1017/S0960258500004141
Teakle, N. L., Bazihizina, N., Shabala, S., Colmer, T. D., Barrett-Lennard, E. G., Rodrigo-Moreno, A., et al. (2013). Differential tolerance to combined salinity and O2 deficiency in the halophytic grasses Puccinellia ciliata and Thinopyrum ponticum: the importance of K+ retention in roots. Environ. Exp. Bot. 87, 69–78. doi: 10.1016/j.envexpbot.2012.09.006
Tewari, S., and Mishra, A. (2018). “Flooding stress in plants and approaches to overcome,” in Plant Metabolites and Regulation Under Environmental Stress (New Delhi: Academic Press), 355–366. doi: 10.1016/B978-0-12-812689-9.00018-2
Tosi, L., Tacconi, G., and Giacopini, A. (2015). La moria del kiwi, situazione e prospettive. Informatore Agrario 44, 67–70. Available online at: http://www.ediagroup.it/BDO/BDO_popupAbstract.asp?D=123393
Trapero-Casas, A., and Kaiser, W. J. (1992). Influence of temperature, wetness period, plant age, and inoculum concentration on infection and development of Ascochyta blight of chickpea. Phytopathology 82, 589–596. doi: 10.1094/Phyto-82-589
Trenkel, M. E. T. (2021). Slow-and Controlled-Release and Stabilized Fertilizers: An Option for Enhancing Nutrient Use Efficiency in Agriculture. Paris: International Fertilizer Industry Association (IFA).
Tripathi, P. C., Sankar, V., and Lawande, K. E. (2017). Microirrigation in onion (Allium cepa) and garlic (A. sativum)-a review. Curr. Hortic. 5, 3–14.
Urban, D. W., Roberts, M. J., Schlenker, W., and Lobell, D. B. (2015). The effects of extremely wet planting conditions on maize and soybean yields. Clim. Change 130, 247–260. doi: 10.1007/s10584-015-1362-x
Varadachari, C., and Goertz, H. M. (2010). “Slow-release and controlled-release nitrogen fertilizers,” in ING Bulletins on Regional Assessment of Reactive Nitrogen, ed S. Bijay (New Delhi, Indian Nitrogen Group, Society) pp.1–42.
Velmurugan, A., Swarnam, T. P., Ambast, S. K., and Kumar, N. (2016). Managing waterlogging and soil salinity with a permanent raised bed and furrow system in coastal lowlands of humid tropics. Agric. Water Manag. 168, 56–67. doi: 10.1016/j.agwat.2016.01.020
Vu, J. C., and Yelenosky, G. (1991). Photosynthetic responses of citrus trees to soil flooding. Physiol. Plant. 81, 7–14. doi: 10.1111/j.1399-3054.1991.tb01705.x
Vwioko, E., Adinkwu, O., and El-Esawi, M. A. (2017). Comparative physiological, biochemical, and genetic responses to prolonged waterlogging stress in okra and maize given exogenous ethylene priming. Front. Physiol. 8, 632. doi: 10.3389/fphys.2017.00632
Waller, J. M. (1992). Colletotrichum Diseases of Perennial and Other Cash Crops, in Colletotrichum: Biology, Pathology and Control, eds J. A. Bailey and M. J. Jeger (Wallingford, CT: CAB International), 167–185.
Wani, S. H., Choudhary, M., Kumar, P., Akram, N. A., Surekha, C., Ahmad, P., et al. (2018). “Marker-assisted breeding for abiotic stress tolerance in crop plants,” in Biotechnologies of Crop Improvement, Volume 3 (Cham: Springer), 1–23. doi: 10.1007/978-3-319-94746-4_1
Weir, B. S., Johnston, P. R., and Damm, U. (2012). The Colletotrichum gloeosporioides species complex. Stud. Mycol. 73, 115–180. doi: 10.3114/sim0011
Wilcox, W. F. (1993). Incidence and severity of crown and root rots on four apple rootstocks following exposure to Phytophthora species and waterlogging. J. Am. Soc. Hortic. Sci. 118, 63–67. doi: 10.21273/JASHS.118.1.63
Wiyono, S. (2007). Climate Change and Pests and Diseases Explosion. Pro. Biodiversity in the middle of global warming. Kheti Foundation.
Wu, H., Xiang, J., Chen, H. Z., Zhang, Y. P., Zhang, Y. K., Zhu, F., et al. (2018). Effects of exogenous growth regulators on plant elongation and carbohydrate consumption of rice seedlings under submergence. J. Appl. Ecol. 29, 149–157. doi: 10.13287/j.1001-9332.201801.021
Yiu, J. C., Liu, C. W., Fang, D. Y., and Lai, Y. S. (2009). Waterlogging tolerance of Welsh onion (Allium fistulosum L.) enhanced by exogenous spermidine and spermine. Plant Physiol. Biochem. 47, 710–716. doi: 10.1016/j.plaphy.2009.03.007
Yiu, J. C., Liu, C. W., Kuo, C. T., Tseng, M. J., Lai, Y. S., Lai, W. J., et al. (2008). Changes in antioxidant properties and their relationship to paclobutrazol-induced flooding tolerance in Welsh onion. J. Sci. Food Agric. 88, 1222–1230. doi: 10.1002/jsfa.3209
Zhang, Q., Liu, X., Zhang, Z., Liu, N., Li, D., Hu, L., et al. (2019). Melatonin improved waterlogging tolerance in alfalfa (Medicago sativa) by reprogramming polyamine and ethylene metabolism. Front. Plant Sci. 10, 44. doi: 10.3389/fpls.2019.00044
Zhang, R., Zhou, Y., Yue, Z., Chen, X., Cao, X., Ai, X., et al. (2019). The leaf-air temperature difference reflects the variation in water status and photosynthesis of sorghum under waterlogged conditions. PLoS ONE. 14, e0219209. doi: 10.1371/journal.pone.0219209
Zhang, X., Fan, Y., Shabala, S., Koutoulis, A., Shabala, L., Johnson, P., et al. (2017). new major-effect QTL for waterlogging tolerance in wild barley (Hordeum spontaneum). Theor. Appl. Genet. 130, 1559–1568. doi: 10.1007/s00122-017-2910-8
Zhang, X., Shabala, S., Koutoulis, A., Shabala, L., Johnson, P., Hayes, D., et al. (2015). Waterlogging tolerance in barley is associated with faster aerenchyma formation in adventitious roots. Plant Soil 394, 355–372. doi: 10.1007/s11104-015-2536-z
Zhang, Y., Liu, G., Dong, H., and Waterlogging, L.i. C. (2021). stress in cotton: damage, adaptability, alleviation strategies, and mechanisms. Crop J. 9, 257–270. doi: 10.1016/j.cj.2020.08.005
Zhou, M. (2010). “Improvement of plant waterlogging tolerance,” in Waterlogging Signalling and Tolerance in Plants (Berlin, Heidelberg: Springer), 267–285. doi: 10.1007/978-3-642-10305-6_13
Keywords: anthracnose, biotic stress, concurrent stresses, onion, twister disease, waterlogging
Citation: Salunkhe VN, Gedam P, Pradhan A, Gaikwad B, Kale R and Gawande S (2022) Concurrent waterlogging and anthracnose-twister disease in rainy-season onions (Allium cepa): Impact and management. Front. Microbiol. 13:1063472. doi: 10.3389/fmicb.2022.1063472
Received: 07 October 2022; Accepted: 16 November 2022;
Published: 08 December 2022.
Edited by:
Chetan Keswani, Southern Federal University, RussiaReviewed by:
Richa Raghuwanshi, Banaras Hindu University, IndiaArpan Mukherjee, Banaras Hindu University, India
Copyright © 2022 Salunkhe, Gedam, Pradhan, Gaikwad, Kale and Gawande. This is an open-access article distributed under the terms of the Creative Commons Attribution License (CC BY). The use, distribution or reproduction in other forums is permitted, provided the original author(s) and the copyright owner(s) are credited and that the original publication in this journal is cited, in accordance with accepted academic practice. No use, distribution or reproduction is permitted which does not comply with these terms.
*Correspondence: Suresh Gawande, c3VyZXNoZ2F3YW5kZTc2JiN4MDAwNDA7Z21haWwuY29t