- 1State Key Laboratory of Environmental Geochemistry, Institute of Geochemistry, Chinese Academy of Sciences, Guiyang, China
- 2College of Life Sciences, College of Marine Science and Engineering, Nanjing Normal University, Nanjing, China
- 3Institute of Medical Plant Physiology and Ecology, School of Pharmaceutical Sciences, Guangzhou University of Chinese Medicine, Guangzhou, China
Introduction: Applications of organomineral fertilizer (OMF) are important measures for developing organic agriculture in karst mountain areas. However, the influence of OMF on the structure and function of soil microbial diversity and their relationship with crop yield and quality are still unclear.
Methods: Based on soil science, crop science, and high-throughput sequencing methods, we investigated the changes of rhizosphere soil microbial communities of Perilla frutescens under different fertilization measures. Then, the relationship between P. frutescens yield and quality with soil quality was analyzed.
Results: The results showed that the addition of OMF increased the amount of total carbon and total potassium in soil. OF, especially OMF, improved P. frutescens yield and quality (e.g., panicle number per plant, main panicle length, and unsaturated fatty acid contents). Both OF and OMF treatments significantly increased the enrichment of beneficial microorganism (e.g., Bacillus, Actinomadura, Candidatus_Solibacter, Iamia, Pseudallescheria, and Cladorrhinum). The symbiotic network analysis demonstrated that OMF strengthened the connection among the soil microbial communities, and the community composition became more stable. Redundancy analysis and structural equation modeling showed that the soil pH, available phosphorus, and available potassium were significantly correlated with soil microbial community diversity and P. frutescens yield and quality.
Discussion: Our study confirmed that OMF could replace CF or common OF to improve soil fertility, crop yield and quality in karst mountain soils.
Introduction
Application of chemical fertilizers (CFs) can significantly increase soil fertility and crop yield in a short time. However, long-term excessive application of CFs damages soil microbial communities and biological activities, which results in decreased soil quality, increased dependence of crop growth on fertilizer nutrients, and aggravated agricultural surface source pollution (Gomiero et al., 2011; Kour et al., 2020; Ren et al., 2020). To reduce the negative effects caused by excessive application of CFs, organic fertilizers (OFs) are usually used to replace or partially replace CFs to protect soil biodiversity and maintain soil ecological balance (Megali et al., 2013; Steffen et al., 2015). Increased OFs application can increase soil carbon storage and plant nutrients, and improve soil biological activity, which are of great significance for mitigating climate warming and developing sustainable agricultural production (Gattinger et al., 2012; Seufert et al., 2012). Many studies have shown that OFs application is an effective way to improve crop yield and quality (Liu et al., 2020, 2021; Du et al., 2022), and increase soil microbial richness and diversity (Zhou et al., 2015; Cui et al., 2018; Li et al., 2020). However, the contents of mineral nutrients such as nitrogen, phosphorus, and potassium in OFs are low, and the increased amount of fertilizer needed increases the cost and application difficulty. Therefore, it is very necessary to improve the contents of mineral elements in traditional OFs.
As a new fertilizer combining the advantages of organic fertilizer and inorganic fertilizer, the nutrient release effect of OMFs occurs simultaneously with the crop growth process, making the agronomic efficiency higher when compared with the inorganic fertilizer (Kiehl, 2008). Compared with CFs, OMFs can reduce the loss of some nutrients, such as nitrogen volatilization, phosphorus fixation and potassium leaching (Aguilar et al., 2019). Compared with common OFs, OMFs are rich in mineral elements necessary for crop growth. OMFs are usually composed of natural organic matter sources and inorganic element sources. Organomineral fertilizer ingredients are mostly agricultural wastes such as chicken manure, coffee shell, wood waste, sewage sludge and sugarcane cake, combined with urea, calcium superphosphate, potassium chloride, magnesium silicate, calcium sulfate and other inorganic chemical fertilizers (Efanov et al., 2001; Carvalho et al., 2014; Grohskopf et al., 2019; Gonçalves et al., 2021; Hawrot-Paw et al., 2022; Ngo et al., 2022). According to the published articles, the research on the application effect of organic mineral fertilizer mainly focuses on the agronomic efficiency of crops. Compared with the crop response to inorganic fertilizers, the response to organomineral fertilizers is quite variable. The reported results are gains (Efanov et al., 2001; Deeks et al., 2013; Carvalho et al., 2014; Sakurada et al., 2016; Vollú et al., 2018; Ngo et al., 2022), losses (Antille et al., 2017; Frazão et al., 2019), or equivalent efficiency (Corrêa et al., 2018; Dias et al., 2020; Mumbach et al., 2020). Those varied results may be related to the different ingredients of OMFs, the amount of fertilizer applied and the environment of the study sites. Limited research literature showed that OMF, as a source of organic carbon and mineral elements, had a positive effect on the quantity and activity of soil microorganisms (Hawrot-Paw et al., 2022), but had little effect on the rhizosphere bacterial diversity of crops (Vollú et al., 2018).
The staggering production of cuttings and quarry by-products from mining activities results in a huge environmental burden; however, the combined use of these cuttings and low-grade mineral rocks can help reduce this pollution (Basak et al., 2021; Syed et al., 2021). The OMF formed by the combination fermentation of low-grade mineral powder and agricultural waste can more fully reflect the production concept of energy saving and environmental protection, and reduce the cost in the production process of inorganic fertilizers such as urea and phosphate fertilizer. Previous studies have shown that OMF produced by mixing rock powder containing potassium and phosphate with OF or mixing rock powder during OF fermentation can provide beneficial mineral nutrients for crop growth without introducing toxic heavy metal pollution (Theodoro and Leonardos, 2006; Biswas et al., 2009; Lian et al., 2020; Basak et al., 2021; Syed et al., 2021). As an important support of soil quality, soil microorganisms are sensitive to fertilization managements (Wang et al., 2020; Bello et al., 2021). However, there have been no reports on the effects of OMFs application on soil microbial community diversity and composition in karst mountain soils.
P. frutescens is an annual herb in the Labiaceae family and is a widely cultivated cash crop in Asian countries (Hu et al., 2010; Yu et al., 2017). In China, it has traditionally been used in medicine and food, and has been cultivated for more than 2000 years (Lee and Kim, 2007). The oil-rich seeds of P. frutescens are used to make condiments in traditional Asian cuisines (Luitel et al., 2017). As the raw material of cooking oil, perilla grains are rich in unsaturated fatty acids and have a high content of ɑ-linolenic acid, up to 50–70%; this is the highest ɑ-linolenic acid content known in plants (Yu et al., 2017). In addition to value as a food item, P. frutescens is also used in traditional Chinese medicine, and as food decoration and a coloring agent (Tian et al., 2014). Moreover, P. frutescens is an important agricultural crop in karst areas of southwest China (Tian et al., 2017).
Potassium is one of the main elements necessary for plant growth. According to the standard of the Second Soil Survey of China (China Soil Science Database1), the soil in karst areas is in a state of potassium deficiency (total K < 10 g·kg−1 and available K < 100 mg·kg−1). Therefore, potassium supplementation is necessary to improve soil fertility in karst areas of southwest China. For this reason, our OMF was fermented from potassium-containing rocks (potassium feldspar) together with agricultural wastes such as chicken manure and straw. We hypothesized that the application of potassium-containing OMF was beneficial to improving P. frutescens quality and yield, and had positive effects on the distribution and structure of soil microbial communities. To test this hypothesis, the yield, quality, and rhizosphere soil microbial community characteristics of P. frutescens were analyzed and studied based on soil science, crop science, and high-throughput sequencing technology methods. The aim of this study was to reveal: (1) differences in soil microbial diversity and community structure of the P. frutescens rhizosphere under different fertilization treatments; (2) the relationship among P. frutescens yield and quality, soil characteristics, and microbial community under different fertilization treatments; and (3) the effects of OMF on soil and crop quality. This study investigated for the first time the feasibility of using OMFs to improve the quality of P. frutescens and the abundance of soil beneficial microbial community in the potassium-deficient karst areas. The results will be conducive to the application of new OMFs in karst areas, and improve the yield and quality of P. frutescens which is widely cultivated in this area.
Materials and methods
Experimental design and sample collection
The experimental field site was located in Changzhai Village, Changshun County, Guizhou Province, China (26°01′25″ N, 106°30′55″ E; elevation, 1,004 m). The strata are mainly light-colored limestone of the Permian Qixia Formation and Maokou Formation. The soil type is yellow soil according to the Chinese soil classification system (Gong, 1999) and Orthic Acrisols according to the World Reference Base (WRB) soil classification system (USS Working Group WRB, 2015). The region has a subtropical monsoon humid climate. The annual average temperature is 15.1°C, the annual average precipitation is 1396.7 mm, the annual sunshine duration is 1202.1 h, and the annual frost-free period is 275 days.
Four treatments were set up in the field experiment: blank control group (CK), no fertilization; CF group, compound CF was applied; OF group, ordinary OF was applied; OMF group, potassium-containing OMF was applied. Four parallel plots (5 m × 5 m) were set for each treatment group (total, 16 plots). The randomized complete block design was used to divide the sample area blocks (Supplementary Figure S1). On 7 May 2019, P. frutescens was planted and fertilized. The P. frutescens seeds were the 1st generation hybrids cultivated by the Oil Materials Research Institute of Guizhou Academy of Agricultural Sciences (China).
The fertilizer specifications used were as follows:
The raw materials for OF fermentation were mushroom residue, distiller’s grains, straw, and chicken manure, which were mixed according to a mass ratio of 1:1:1:2 and EM microbial agent was added for fermentation (1 kg bacterial agent was added for every 10 T substrate). The mixture was then fermented in a fermentation tank for 30 days with periodic stirring. The total nutrient content (N + P2O5 + K2O) was 7.2%, among which the content ratio of N:P2O5:K2O was 0.9%:2.9%:3.4%, organic matter was 48.3%, and pH was 7.7.
Potassium-containing OMF was made by mixing the raw fermentation materials of the OF and potassium-containing rock powder, which contained 76% potassium feldspar, and passing the mixture through a 2-mm sieve. In accordance with a mass ratio of 3:1, the chemical composition was as follows: Al2O3, 17.11%; SiO2, 54.06%; K2O, 9.09%; CaO, 1.9%; Fe2O3, 6.15%; and MgO, 3.41% (Sun et al., 2019). Then, EM microbial agent was added for fermentation (1 kg bacterial agent per 10 T substrate). The raw materials were thoroughly mixed and fermented for 30 days with periodic stirring. The total nutrient content of OMF was 6.4%, with a content ratio of N: P2O5: K2O of 1.6%:1.1%:3.7%, the content of organic matter was 56.4%, and the pH was 7.6. OF and OMF were produced by Guizhou Guifu Ecological Fertilizer Co., LTD. (China).
The compound CF was produced by Guizhou Xiyang Fertilizer Co., LTD. (China) and had a total nutrient content ≥45%, N:P2O5:K2O content ratio was 15%:15%:15%. Urea was produced by Guizhou Chitianhua Tongzi Chemical Co., LTD. (China) with a total N ≥ 46.4%. Phosphate fertilizer was produced by Fuda Phosphorus Chemical Co., LTD. (China) with P2O5 ≥ 12%. The proportions of N, P, and K in OF and OMF groups were balanced by urea and phosphate fertilizer. All treatment groups had fertilizer applied with a content ratio of N:P2O5:K2O of 15:15:15, and application amounts are shown in Table 1.
Rhizosphere soil sampling was conducted on 19 August 2019, which was the 105th day of P. frutescens growth. Rhizosphere soil samples were arbitrarily collected from eight P. frutescens plants in each plot and mixed into one sample. A total of 16 mixed soil samples were collected. When collecting rhizosphere soil, the whole plant was first dug up, and the scattered soil at the root was gently shaken off. The remaining soil attached to the root system was considered the rhizosphere soil (Sun et al., 2021). The soil samples were frozen and transported to the laboratory on dry ice, passed through a 2-mm sterile sieve, and the plant roots were removed. Each sample was divided into two parts: one part was naturally air-dried to determine physicochemical properties, and the other was stored in a − 80°C freezer for DNA extraction.
Soil physicochemical analysis
Soil pH was analyzed by vibrating slurry with a water:soil ratio of 2.5:1 (v/w) and determined using a pH meter (Mettler-Toledo FE28, Switzerland; Marcos et al., 2019). Soil total organic carbon (TOC), total organic nitrogen (TON), total carbon (TC), and total nitrogen (TN) were determined using an elemental analyzer (Vario MACRO Cube, Germany; Liu et al., 2014). Total phosphorus (TP) and total potassium (TK) were determined by sodium hydroxide melting flame spectrophotometry (Ren et al., 2016). Available phosphorus (AP) was determined by the NaHCO3 method, and available potassium (AK) was determined by ammonium acetate extraction–flame spectrophotometry (Lu, 1999).
P. frutescens yield and quality analysis
On 21 September 2019, P. frutescens were harvested on the 137th day of growth. The P. frutescens yield was measured after harvest. The main indicators were plant height, biomass per plant, number of stem nodes, number of effective branches at one time, length of effective branches at one time, number of branch angles, number of panicles per plant, length of the main panicle, number of fruits in a single row of the main panicle, number of fruits on the main ear, and number of grains in the 10 main ears. After the perilla seeds were harvested, seed quality was inferred by determining fatty acid content (e.g., palmitic acid content, stearic acid content, oleic acid content, linoleic acid content, and linolenic acid content), total lipid content, and crude protein content. The fatty acid content was determined by gas chromatography–mass spectrometry (GCMS-QP2010, Schimadzu, Japan; Javier et al., 2018; Ko et al., 2018). Fat content was determined by sequential Soxhlet extraction according to ISO 659:2009 (Kourimska et al., 2018). The crude protein content was determined by the Kjeldahl method, the nitrogen concentration of the sample was calculated with a conversion factor (6.25), and the total nitrogen and protein mass were determined to obtain the crude protein content (Yaldiz and Camlica, 2020).
DNA extraction and high-throughput sequencing
Total soil DNA was extracted from 0.5 g soil according to the manufacturer’s instructions for the E.Z.N.A.® Soil DNA Kit (Omega Bio-Tek, USA). The DNA extraction quality was detected using 1% agarose gel electrophoresis, and the DNA concentration and purity were determined using a NanoDrop2000 spectrophotometer (Thermo Fisher Scientific Co., LTD., USA). Using the extracted DNA as a template, the V3–V4 region of the bacterial 16 s rRNA gene was amplified using the primers 338F (5′-ACTCCTACGGGAGGCAGCAG-3′) and 806R (5′-GGACTACHVGGGTWTCTAAT-3′; Xu et al., 2016). The fungal ITS region was amplified using the primers ITS1F (5′-CTTGGTCATTTAGAGGAAGTAA-3′) and ITS2R (5′-GCTGCGTTCTTCATCGATGC-3′; Adams et al., 2013). PCR amplification conditions and high-throughput sequencing were conducted as described in Li et al. (2021). Sequencing was performed on Illumina’s MiSeq PE300 platform (Shanghai Majorbio Bio-pharm Technology Co., LTD., China). The raw sequence data reported in this paper were deposited in the NCBI SRA database (serial numbers: bacteria, PRJNA836163; fungi, PRJNA836186).
Trimmomatic (version 0.332) was used for quality control of the original sequences (Bolger et al., 2014), and FLASH (version 1.2.113) was used for splicing (Magoc and Salzberg, 2011). The splicing sequence data were analyzed using UPARSE (version 7.1;4 Edgar, 2013), and sequences with a similarity of ≥97% were assigned to the same operational taxonomic unit (OTU). After quality control and concatenation of the original sequences of all samples, 1,088,672 and 976,526 high-quality sequences of bacteria and fungi were obtained, respectively. At the 97% sequence similarity level, the sequences clustered into 5,685 and 2,585 OTUs, respectively. For each representative sequence, the SILVA (bacteria5) and UNITE (fungi6) databases were used to annotate taxonomic information (Fan et al., 2020; Kang et al., 2022).
Statistical analysis
Mean value, standard deviation, and variance analysis of soil physicochemical properties and Perilla yield and quality were analyzed using Microsoft Excel 2010 and SPSS Statistics (version 20.0, IBM, USA). Differences between mean values were determined by one-way ANOVA and LSD post-hoc test (p < 0.05). All bioinformatic analyses were performed using R (version 3.6.1; https://cran.r-project.org/bin/windows/base/old/3.6.1/).
The alpha diversity (Sobs, Chao, and Shannon indices) of Perilla rhizosphere soil microbial communities was estimated based on OTUs. All indices were calculated by the “vegan” (Dixon, 2003) and “picante” (Kembel et al., 2010) packages in R (version 3.6.3). The linear discriminant analysis (LDA) effect size (LEfSe) method was used to assess potential bacterial and fungal biomarkers (from phylum to genus) within soil microbiomes that were specifically enriched under different fertilization management types based on p < 0.05 and an LDS score > 4.0 (Segata et al., 2011). Principal coordinates analysis (PCoA) was performed to calculate the gradient of compositional changes for bacterial and fungal microbial communities (based on Weighted-Unifrac distance matrix) using the ggplot2 package (Lozupone and Knight, 2005). Differences in bacterial and fungal communities between different samples were analyzed by Adonis test.
After variance inflation factor (VIF) analysis, pH, TC, TOC, TN, TK, AP, and AK with a VIF threshold less than 10 were selected for redundancy analysis (RDA) between environmental factors and soil microbial communities. Variance partitioning analysis (VPA) was used to quantitatively evaluate the individual and common explainability of environmental factor variables for microbial community differences. In addition, FAPROTAX (Louca et al., 2016) and FUNGuild (Nguyen et al., 2016) were used to analyze the ecological functions of soil bacteria and fungi, respectively. Kruskal–Wallis H test was used to test the significance of differences between groups.
Co-occurrence network analysis
To study the effect of different fertilization management techniques on the relationship of soil microbial communities, soil bacterial and fungal communities were combined based on fertilization management technique, and a soil microbial co-occurrence network based on genus classification was constructed. The co-occurrence network was constructed with genera that had a relative abundance greater than 0.1% based on random matrix theory (Deng et al., 2012). To simplify the networks for better visualization, a Spearman’s correlation between the two genera was considered statistically significant if the Spearman’s correlation coefficient (r) was >0.6 and the p value was <0.05. Moreover, p values were adjusted using the Benjamini–Hochberg FDR method (Benjamini and Hochberg, 1995). Spearman’s correlation and network attributes were calculated using the WGCNA, Psych, Igraph, and fdrci packages in R (version 3.6.3) (Csardi and Nepusz, 2006). The network attributes included the number of edges, average clustering coefficient, average degree, modularity, average path length, graph density, and betweenness centrality. Higher numbers of nodes and edges, graph density, average degree, and lower average path length indicate a more complex and connected network (Ma et al., 2016; Jiao et al., 2021). The higher the betweenness centrality value of microbial species, the greater the critical role of the species in the network. The Fruchterman–Reingold layout algorithm was used in the interactive platform Gephi (version 0.9.27) for network visualization and network topology parameter calculation (Bastian et al., 2009). The network stability was evaluated by removing the nodes in the static network to estimate the speed of robustness decline, and the network robustness was evaluated by the natural connectivity of the nodes (Yuan et al., 2021; Zhu et al., 2022).
Structural equation model analysis
A structural equation model (SEM) was used to identify the direct and indirect effects of soil physicochemical properties (such as pH, TC, AK, and AP) on bacterial and fungal diversity (Shannon index), and P. frutescens yield (biomass per plant) and quality (linoleic acid content). To reduce SEM complexity, the representative indices of soil physicochemical properties were calculated by PCoA (Sun et al., 2021). All variables were standardized using Z-transformation (mean = 0, standard deviation = 1; Du et al., 2022).
The theoretical model assumed that: (1) soil pH has a direct impact on soil nutrient content, microbial community diversity, and P. frutescens quality or yield, (2) the soil available phosphorus, potassium, and total carbon had direct or indirect effects on the P. frutescens quality and yield and the soil microbial community, and (3) the P. frutescens yield has a direct effect on the soil microbial community. Model fitting was performed using root mean square error of approximation (RMSEA), probability level p value, Bentler comparative fit index (CFI), maximum likelihood goodness of fit (χ2), and degrees of freedom (df) tests (Du et al., 2022). The SEM was constructed using Amos Graphics (version 24.0, IBM Corp., USA; Liu, L. et al., 2019).
Results
Soil physicochemical properties
Compared with the CK group, the OMF group significantly increased soil TC and TK contents (p < 0.05; Table 2). Fertilization also significantly increased soil AK content (p < 0.05; Table 2). In addition, there were no significant differences in the physicochemical properties between the different fertilization treatment groups. The measurement results of different fertilization treatment groups indicated that short-term fertilization treatments may not significantly improve soil physicochemical properties. However, numerical analysis demonstrated that OF and OMF treatments tended to produce better results than no fertilization and CF treatment.
P. frutescens yield and quality
The analysis of P. frutescens yield during the harvest period showed that, compared with the CK group, the CF, OF, and OMF groups all significantly improved the yield indicators, including the number of stem nodes, number of effective branches at one time, and number of branch angles. However, there were no significant differences among the three fertilization treatments (Table 3). In addition, the number of panicles per plant and length of the main panicle in the OMF group were significantly greater than those in the other treatment groups.
Analysis of P. frutescens seed quality showed that the contents of oleic acid, linoleic acid, α-linolenic acid, and fat in the OF and OMF groups were significantly higher than those in the CK and CF groups. Additionally, the OMF group had the highest contents of oleic acid, α-linolenic acid, and fat (Figure 1). The crude protein content of the OMF group was significantly higher than that of the other treatment groups (p < 0.05). This indicated that the application of OFs, especially OMF, helped improve P. frutescens quality.
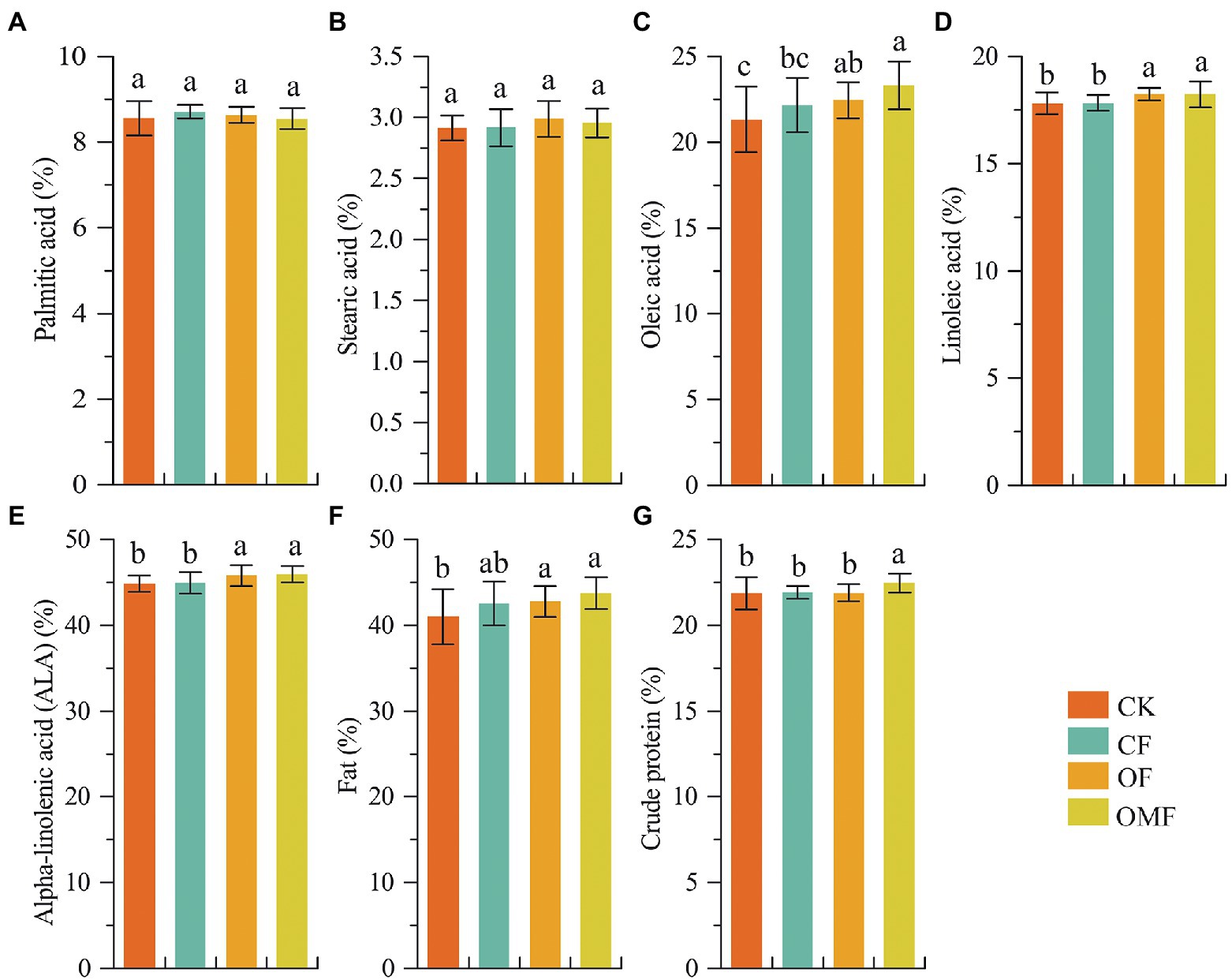
Figure 1. Perilla frutescens quality index under different fertilization treatments. Based on one-way ANOVA and LSD post hoc test, different letters (a,b) indicated significant differences among fertilization treatment groups (P < 0.05).
Effects of fertilization treatments on soil microbial community composition
Alpha diversity analysis showed that different fertilization treatments did not significantly affect soil bacterial community richness and diversity, whereas OMF significantly increased soil fungal community richness (Figure 2). PCoA showed that there was no clear distinction between samples from different treatment groups on the PC1 and PC2 axes (Figures 3A,B); this indicated that there was no significant difference in soil bacterial community and fungal community composition among different fertilizer treatment groups in the short term.
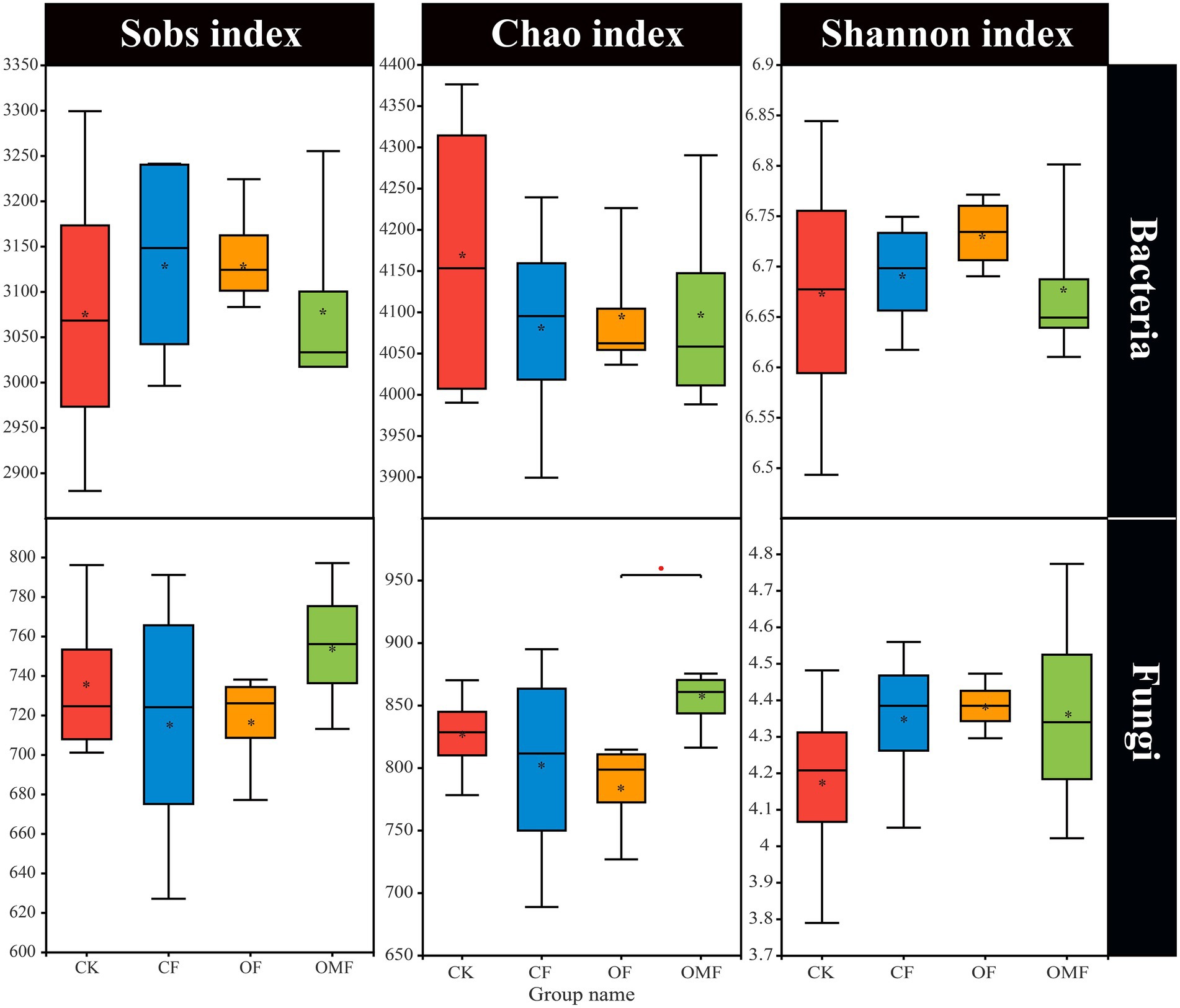
Figure 2. Alpha diversity index of soil microbial community in different treatment groups. *indicates a significant difference at the level of 0.01 < p ≤ 0.05 (Student t test).

Figure 3. Principal coordinates analysis of the Weighted-Unifrac distance matrix for bacteria (A), and fungi (B), in different fertilizer treatment groups. Bar plots of relative abundance of bacterial phyla (C), and fungal phyla (D), in different fertilization groups.
Fertilization management did not cause significant changes in phylum-level bacterial composition (p > 0.05, ANOVA analysis; Figure 3C). Proteobacteria, Actinobacteria, Acidobacteria, and Chloroflexi were the main dominant bacterial phyla in each fertilization treatment group. Fertilization management also did not significantly affect the fungal community composition at the phylum level (p > 0.05, ANOVA; Figure 3D). Ascomycota, Mortierellomycota, Basidiomycota, and Glomeromycota were the dominant fungal phyla in each treatment group. The taxonomic analysis of the dominant bacterial genera with relative abundance >1% showed that different short-term fertilization treatments had no obvious effect on most dominant bacterial genera (Supplementary Table S1). However, the relative abundances of Bacillus and Candidatus_Solibacter significantly increased after fertilization, especially in the OF and OMF groups; the relative abundance of Bryobacter significantly decreased in the OF and OMF groups. The relative abundances of Clonostachys and Gonytrichum in the OMF group were significantly lower than those in the other groups, and the relative abundance of Penicillium in the OF group was significantly lower than that in the other treatment groups. In addition, there were no significant differences in other dominant fungal genera among the treatment groups (Supplementary Table S2).
The LEFSe results showed that Bryobacter was significantly enriched in the CK group; the nitrogen-fixing bacteria Saccharimonadia and iron-reducing bacteria Desulfobacca were significantly enriched in the CF group; Actinomadura, Nakamurellaceae and Nakamurella were significantly enriched in the OF group; and Iamia and Iamiaceae was significantly enriched in the OMF group (Figure 4A). The LEFSe analysis of the fungal community showed (Figure 4B) that Cyphellaceae, Piskurozymaceae, Filobasidiales, Solicoccozyma, and Funneliformis were mainly enriched in the CK group, and Mycosphaerellaceae, Didymosphaeriaceae, Paraphaeosphaeria, and Pseudopithomyces were mainly enriched in the CF group. Helotiales, Glomerales, Cladorrhinum, and Pseudallescheria in the OF group, and Phaeosphaeriaceae, Bulleribasidiaceae, and Sodiomyces were mainly enriched in the OMF group.
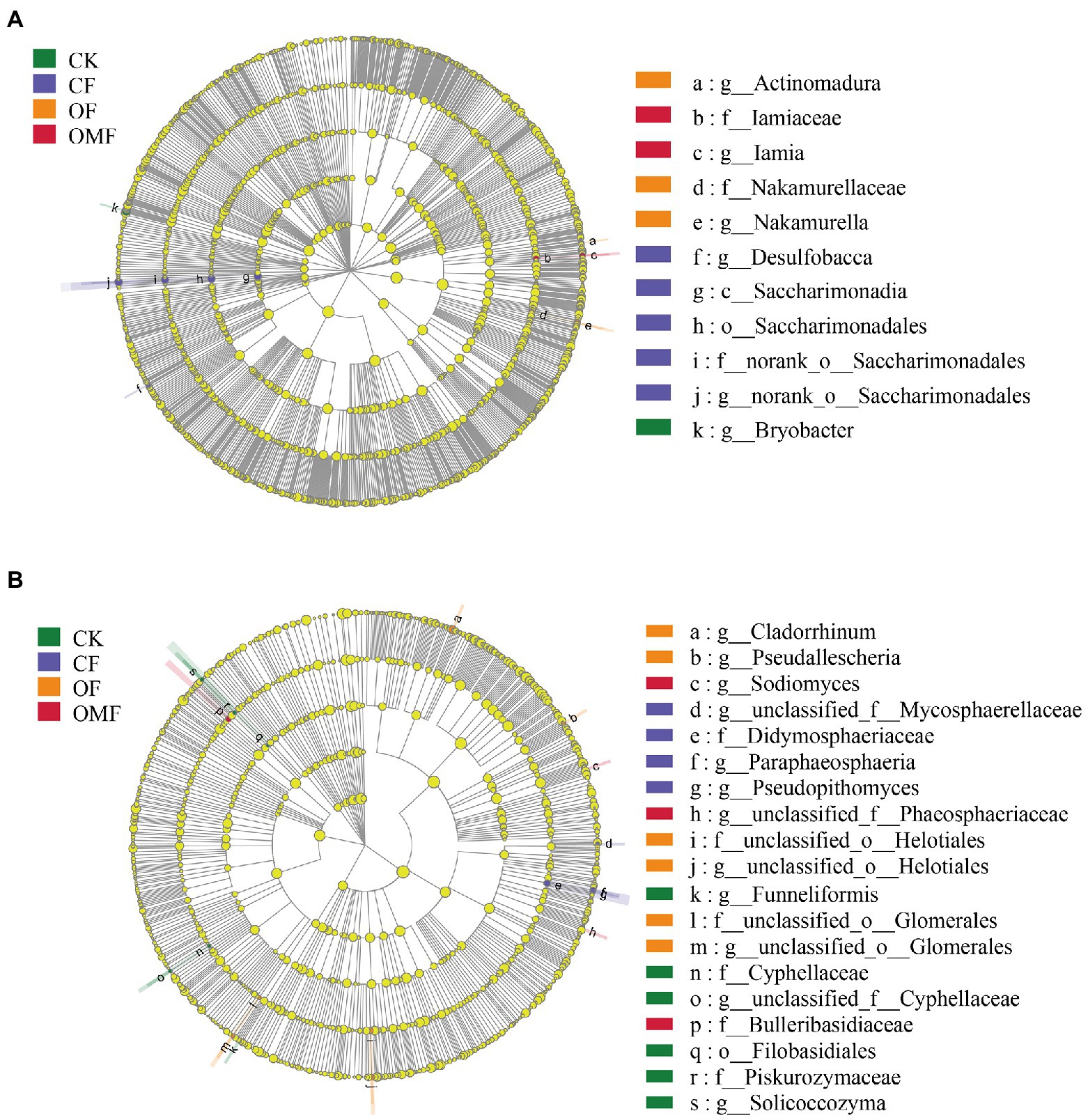
Figure 4. Effects of different fertilization treatments on the relative abundance of soil bacterial (A), and fungal (B), lineages. The linear discriminant analysis (LDA) effect size analysis was performed to identify the indicator taxa representing each group, and the values were significant (p < 0.05) when the LDA score was greater than 4. There are five rings in the cladogram, that represent the phylum, class, order, family, and genus from inside to outside, respectively. The different color nodes (except yellow, which indicates no significant changes) on the ring represent significant changes in taxonomic composition due to the treatments. Abbreviations for classification levels: P, phylum; C class; O, order; F, family; G, genus.
Co-occurrence network analysis of soil microbial communities under different fertilization management techniques
Analysis of the topological indicators of the bacterial community co-occurrence network revealed that the OMF group had the highest increase in the number of network edges and average degree (Table 4). The OF group had the highest modularity index, followed by the OMF group. This finding indicated that the application of OF and OMF could enhance the association of soil bacterial communities because the degree of modularity was higher. The robustness analysis results showed that the robustness of OF and OMF treatment groups were higher than that of CK and OF groups, and OMF groups was highest (Figure 5C).
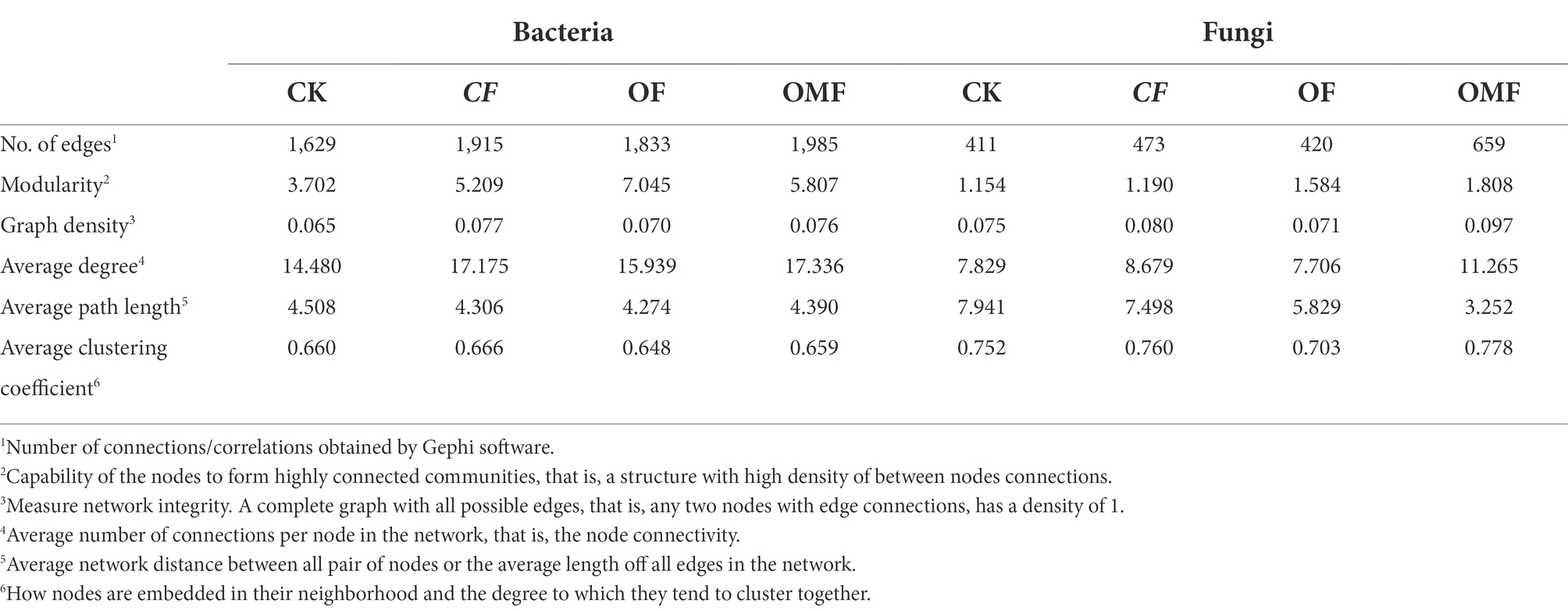
Table 4. Topological indices of each co-occurrence network in Figure 5.
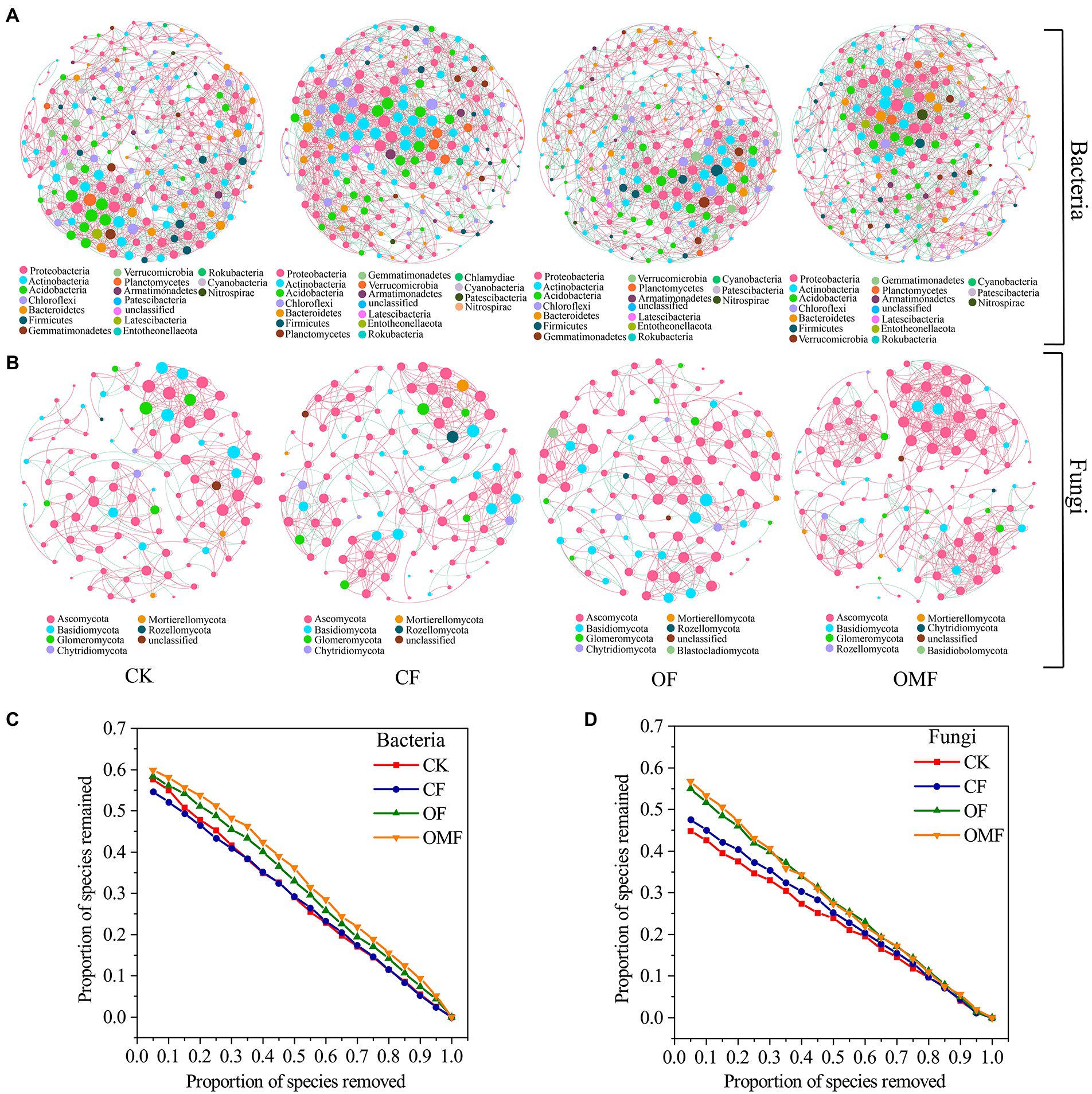
Figure 5. Co-occurrence networks of the soil microbial communities at the genus level in different fertilization treatment groups (A,B); and the robustness of bacterial network (C), and fungal network (D). The node size is proportional to the taxon abundance, and the nodes represent bacterial or fungi taxa at the genus level (genera with relative abundances greater than 0.1%). The node colors represent different bacterial and fungal phyla. The edges are colored according to interaction types; positive correlations are labeled in pink and negative correlations are labeled in green.
Analysis of the network topology indicators of the fungal community showed that, compared with other treatment groups, the OMF group significantly increased the total number of edges in the network, number of positive correlation edges, number of nodes, graph density, and average degree (Table 4). The OMF group had the highest modularity index; this demonstrated that OMF can improve the association of fungal communities, and OMF can make the fungal community structure more modular and more stable. The robustness analysis results showed that the robustness of OMF and CF groups had little difference, but was higher than that of CK and OF groups (Figure 5D).
Analysis of the scale proportions of the top three modules in each group revealed that the proportion of the top three modules of bacterial and fungal communities was greatest in the OF group followed by the OMF group (Supplementary Figure S2). This indicated that the bacterial communities and the fungal communities of the OF and OMF groups were more closely related.
The main nodes in the bacterial community network of each treatment group belonged to Proteobacteria, Actinobacteria, Acidobacteria, Chlorobacteria, Bacteroidetes, and Firmicutes; the main nodes in the fungal community network belonged to Ascomycota, Basidiomycota, and Glomeromycota. This finding indicated that these bacterial and fungal phyla were keystone microbiota in all treatment groups (Figure 5). According to the analysis of the betweenness centrality values (Supplementary Table S3), the top 10 genus-level species were different in each treatment group, which indicated that the genus-level microbiota that played a key role in the co-occurrence network differed among treatment groups.
Effects of different fertilizer treatments on the ecological function of soil microbial communities
According to FAPROTAX functional analysis, chemoheterotrophy, aerobic chemoheterotrophy, nitrification, aerobic ammonia oxidation, and nitrogen fixation were the top five bacterial community functions in each treatment group (Figure 6A). Among the top 50 functions, only human pathogens all significantly differed among different treatments, and the OF and OMF treatments reduced the proportion of human pathogenic bacteria in soil compared with CK and CF treatments. There were no significant differences in other bacterial community functions among different treatment groups.
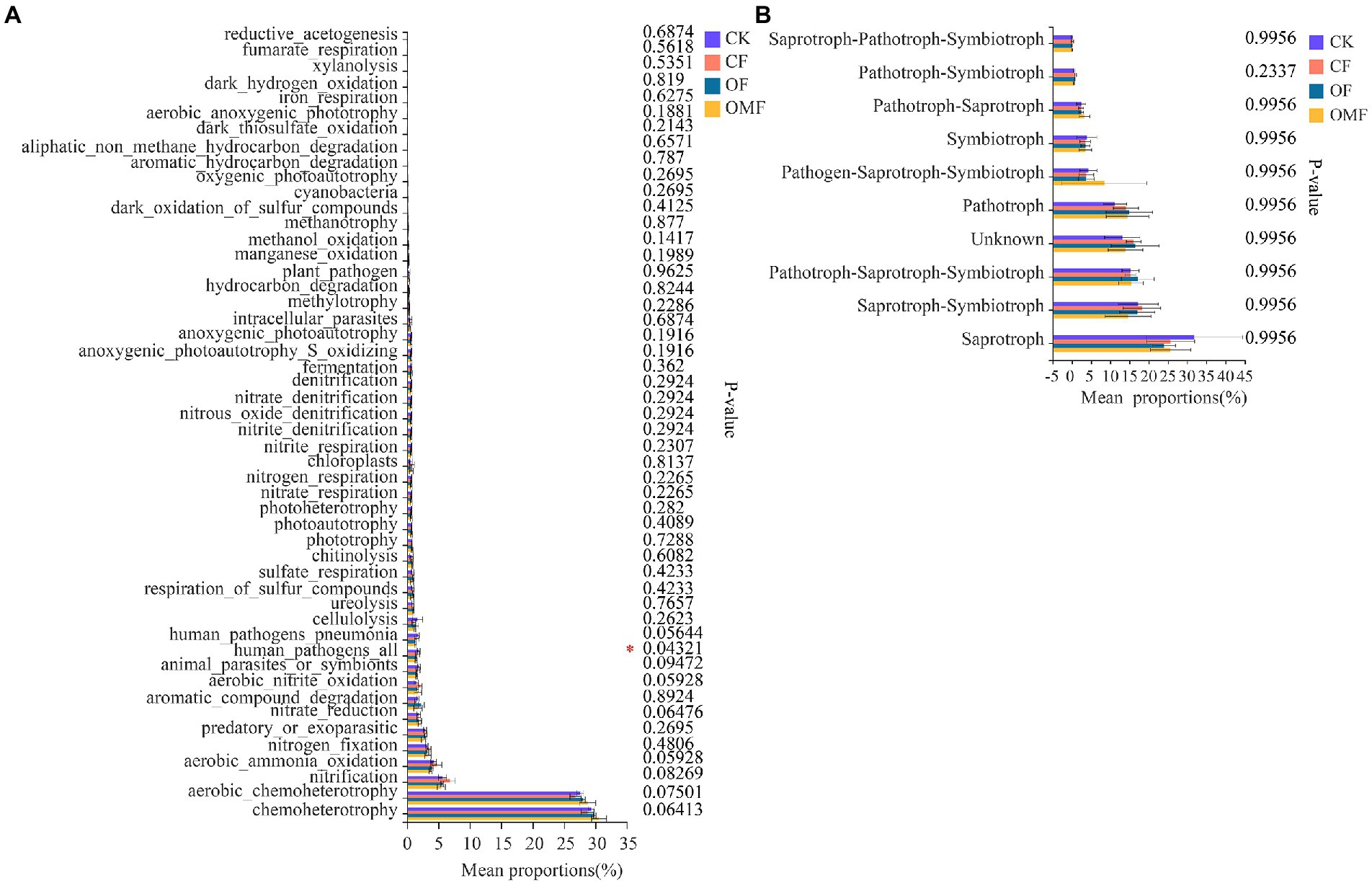
Figure 6. Functional analysis of soil microbial community in different treatment groups. FAPROTAX function analysis of bacterial communities (A), and FUNGuild function analysis of fungal communities (B). The abscissa indicates the function name, the ordinate indicates the percentage value of a function abundance of the sample, and different colors indicate different groups. Based on Kruskal–Wallis H test, the rightmost values represent p values, where *indicates 0.01 < p ≤ 0.05.
FUNGuild functional analysis indicated that Saprotroph, Saprotroph–Symbiotroph, Pathotroph–Saprotroph–Symbiotroph, Pathotroph, and Symbiotroph were the top five trophic modes of the fungal community (Figure 6B). Analysis of the relative abundance of arbuscular mycorrhizal fungi (AMF) in symbiotic trophic fungi showed that the AMF abundance was significantly reduced in the CF group (Supplementary Figure S3A); this indicated that CFs inhibited AMF growth. F_unclassified_o_Paraglomerales, Glomeraceae, Diversisporales_fam_Incertae_sedis, f_unclassified_o_GS24, and Paraglomeraceae were the dominant families in AMF, but there were significant differences in their relative abundances among different fertilization treatments groups (Supplementary Figure S3B).
Effects of soil environmental factors on microbial community composition and diversity, and P. frutescens yield and quality
RDA indicated that the selected environmental factors explained 38.20% of the total change in bacterial communities (Figure 7A) and 31.32% of total changes in fungal communities (Figure 7B). The results of RDA showed that pH (ANOVA, p = 0.021) and AP (ANOVA, p = 0.024) were the main factors affecting the soil bacterial community composition, and pH (ANOVA, p = 0.034) and AK (ANOVA, p = 0.011) were the main factors affecting the soil fungal community composition (Figure 7).
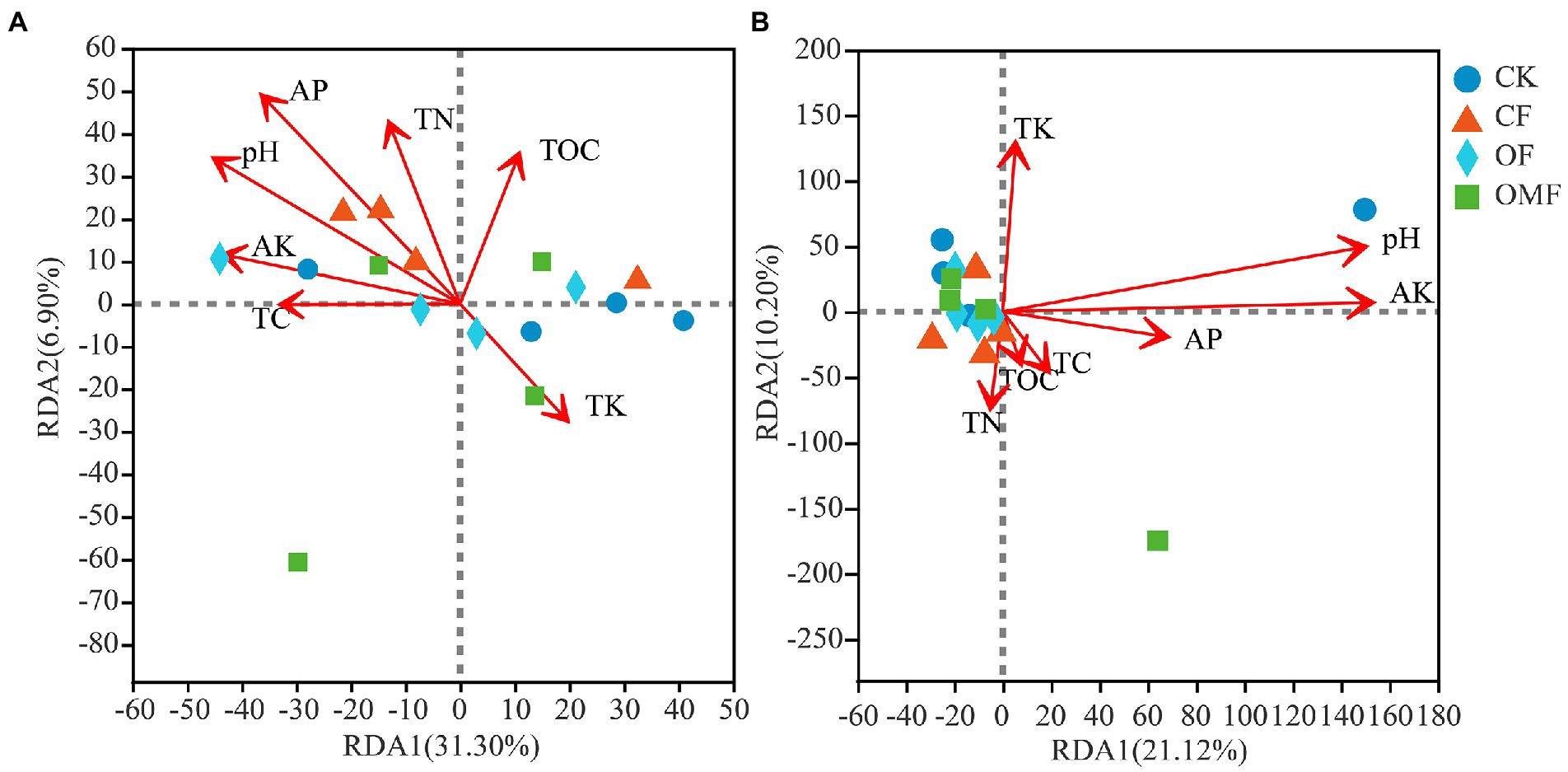
Figure 7. Distance-based redundancy analysis (RDA) among bacterial (A), or fungal (B), communities and environmental factors.
The SEM fit the measured data well (bacteria, χ2/df = 0.658, p = 0.764, CFI = 1.000, RMSEA = 0.000; fungi, χ2/df = 1.493, p = 0.135, CFI = 0.920, RMSEA = 0.181); this indicated high consistency between the hypothesized model and the observed data (Figure 8). The SEM showed that soil physicochemical properties accounted for 68% of P. frutescens quality, among which AK and TC were significantly positively correlated with P. frutescens quality, and pH and AP were significantly negatively correlated with P. frutescens quality (Figure 8). AK and AP were significantly positively correlated with bacterial diversity, and P. frutescens yield was significantly negatively correlated with bacterial diversity; these factors accounted for 68% of bacterial diversity (Figure 8A). AP was significantly positively correlated with and explained 61% of P. frutescens yield. TC and P. frutescens yield were directly and significantly positively correlated with fungal community diversity, whereas AK was directly and significantly negatively correlated with fungal community diversity (Figure 8B).
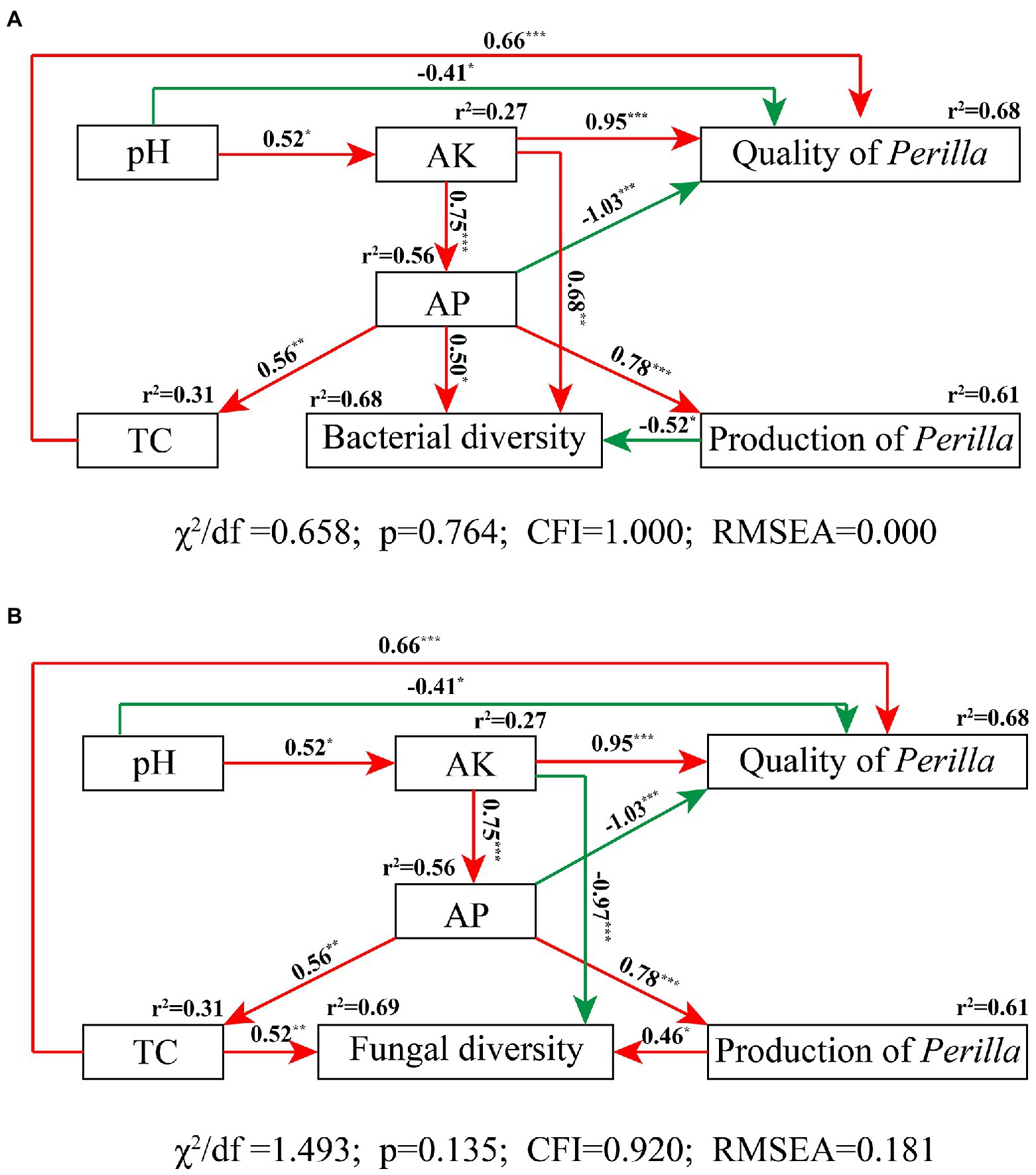
Figure 8. Structural equation modeling results describing the relationship among soil nutrients, microbial diversity, and P. frutescens yield and quality. The relationship among soil pH value, total soil carbon content (TC), available potassium (AK), available phosphorus (AP), P. frutescens production, and P. frutescens quality with bacterial diversity (A), and fungal diversity (B). Red lines: positive correlation; green lines: negative correlation. The numbers above the arrows indicate correlation strength. r2 values indicate the proportion of variance explained for each variable. χ2, Chi-square; df, degrees of freedom; p, probability level; RMSEA, goodness-of-fit statistics for each model. Significance levels of each predictor are shown as *for p < 0.05, **for p < 0.01, and ***for p < 0.001.
Discussion
Effects of organomineral fertilizer on soil properties and P. frutescens yield and quality
Compared with the CK group, OMF application significantly increased the TC and TK contents of the soil (Table 2). The experiment proved that although short-term application of OMF could not significantly improve most of the soil physicochemical properties, it could guarantee the same effect as the same amount of inorganic fertilizer. Numerical analysis showed that OF especially OMF application had a trend of improving soil fertility compared with no fertilization and CF application, which was consistent with previous continuous fertilization results (Zhao et al., 2016; Du et al., 2022).
The yield test results of P. frutescens at harvest showed that the number of panicles per plant and length of the main panicle were significantly greater in the OMF group compared with the other treatment groups (Table 3); this indicated that OMF increased P. frutescens yield to a certain extent. The quality inspection results of P. frutescens showed that OF and OMF treatments significantly increased the contents of unsaturated fatty acids, total fat, and total protein in P. frutescens, and the OMF treatment values were higher (Figure 1). These results were similar to those of a previous study that investigated the effect of OMF on Purslane growth, which also showed that OMF treatment increased unsaturated fatty acid content (Yang et al., 2020). The SEM results also demonstrated that soil AK content was significantly positively correlated with P. frutescens quality (Figure 8). Therefore, OMF application was beneficial for improving P. frutescens quality.
Effects of different fertilization treatments on soil microbial communities
Microorganisms are the driver of soil fertility changes; they can directly indicate soil quality and play an important role in plant growth and crop yield (Fan et al., 2020; Finkel et al., 2020). In this study, short-term fertilization treatments did not significantly affect alpha diversity (Figure 2). Similar studies also showed that soil microbial alpha diversity was stable and not easily affected by agricultural management practices (Coller et al., 2019; Gui et al., 2021; Kang et al., 2022). How fertilization management affects soil microbial diversity depends on soil properties (Mendes et al., 2015), such as soil pH, which is generally considered a decisive factor underlying microbial diversity (Bissett et al., 2011).
Actinobacteria and Firmicutes are generally considered to be beneficial microorganisms for plants (Yang et al., 2017). Actinobacteria can control plant bacterial diseases by producing various antibiotics, secreting cell wall-degrading enzymes, and inducing host resistance (Conn et al., 2008; Chater et al., 2010; Liu et al., 2012). LEFSe analysis showed that the treatment of OF and OMF promoted the enrichment of some bacteria belong to Actinobacteria, including Actinomadura, Nakamurellaceae, Nakamurella, Iamiaceae, and Iamia (Figure 4). Actinomadura can produce several antibiotics that inhibit the growth of soil pathogens and reduce the occurrence of crop diseases and insect pests (Li et al., 2022). Bacillus and Candidatus_Solibacter were also significantly increased in OF and OMF groups (Supplementary Table S1). Bacillus is commonly formulated as biocontrol agents because they secrete antibiotics or antimicrobial proteins (Ahimou et al., 2000; Weller et al., 2002; Moyne et al., 2004), and improve soil fertility by increasing soil mineral nutrient availability (Chen et al., 2016). Additionally, Candidatus_Solibacter is a bacterium that decomposes organic matter (Rime et al., 2015).
LEFSe analysis showed that the main enriched fungal species (e.g., Glomerales, Cladorrhinum, and Pseudallescheria) in the OF groups belonged to the phyla Ascomycota and Glomeromycota (Figure 4B). Among them, Pseudallescheria is a biocontrol fungus; it is an important natural enemy of some plant parasitic nematodes that can parasitize eggs and infect larvae and females, and can significantly reduce the damage of plant nematode diseases such as those caused by root-knot, cyst, and stem nematodes of various crops (Wang et al., 1997; Ko et al., 2010; Zhu et al., 2020). Cladorrhinum is an effective biocontrol fungus for controlling the soil-borne Rhizoctonia solani pathogen (Liu, H. et al., 2019). Ascomycota is a key driver of the degradation of organic residues in soil (Richardson, 2009; Ma et al., 2013); therefore, the Ascomycota abundance may increase with increasing organic matter content (Du et al., 2022). Glomeromycota can undergo symbiosis with terrestrial plants to form arbuscular mycorrhizae, and this symbiosis can help plants absorb inorganic salts in soil, especially phosphorus (Smith and Read, 2008; Calaca and Bustamante, 2022). Our results showed that, OF treatments promoted increase in the number of beneficial fungi in the karst soil.
In conclusion, short-term fertilization treatments affected soil microbial communities, and OF and OMF had advantages over CF. However, compared with previous long-term experimental results, some differences in this study were not significant. Therefore, extending the fertilization period and intensifying fertilization may produce significant fertilization effects (Kox et al., 2020).
OMF treatment increased connectivity and structural stability of soil microbial communities
There is a complex association network among soil microbial communities, and they do not exist alone. When soil microbial community composition changes because of fertilization management, the microbial co-occurrence network also changes (Kang et al., 2022). The OF and OMF groups had higher modularity indices, which indicated that OF and OMF improved the soil microbial community connectivity and made the community connected more closely (Figure 5; Supplementary Figure S2; Table 4). This result is generally consistent with those of other studies on OF application (Ling et al., 2016; Wang et al., 2017; Liu et al., 2020; Kang et al., 2022).
Complex networks with higher connectivity are more tolerant of environmental disturbances than simple networks with lower connectivity (Santolini and Barabási, 2018). In this study, the network connectivity of both bacterial and fungal communities was highest in the OMF group (Table 4); this indicated that OMF treatment resulted in higher anti-interference ability of soil microorganisms. As the core components of soil organic matter degradation, bacteria and fungi usually form different functional groups and change the interaction between their ecological networks because of the decomposition or utilization of organic and inorganic nutrients (Wang et al., 2017; Dai et al., 2018; Samaddar et al., 2019), and they tend to maintain a complex network structure (Kang et al., 2022). The robustness analysis showed that the application of OF, especially OMF, could improve the stability of bacterial and fungal network structures (Figures 5C,D).
Keystone microbial groups play an important role in maintaining ecosystem homeostasis (Banerjee et al., 2018; Fan et al., 2020). According to the degree values of the network nodes, it was found that the key bacterial and fungal phyla in the nodes were not significantly different among the treatment groups (Figure 5); this was consistent with the composition and distribution of dominant species in the community (Figures 3C,D). At the phylum level, microorganisms had strong stability and were not easily affected by fertilization management. The genera with the highest betweenness centrality scores are generally considered keystone taxa (González et al., 2010; Vick-Majors et al., 2014). In this study, the keystone genera differed among fertilization treatment groups. The keystone genus of the bacterial community in the OMF group was Pseudomonas, and the keystone genus of the fungal community was Metarhizium (Supplementary Table S3), both of which are recognized as biocontrol microorganisms. Pseudomonas can adsorb heavy metals in soil and promote plant growth (Costa-Gutierrez et al., 2020; Ghorbanzadeh et al., 2020; Wu et al., 2022), whereas Metarhizium can kill plant pests and mitigate plant diseases (Riguetti Zanardo Botelho et al., 2019; Gebremariam et al., 2021; González-Pérez et al., 2022). In conclusion, this study demonstrated that the application of organic fertilizer, especially OMF, can not only enhance the connectivity, cohesiveness and stability of microbial community network structure, but also increase the abundance of beneficial microorganisms.
Relationship among soil physicochemical properties, soil microbial community, and P. frutescens yield and quality
The RDA results showed that soil pH was the most important factor affecting bacterial and fungal communities (Figure 7). This results was consistent with the previous study (Cho et al., 2016; Gu et al., 2019). Changes in soil pH can alter soil structure, fertility, and vegetation communities, thereby directly or indirectly affecting soil microbial community composition (Lauber et al., 2009; Qi et al., 2018). In addition, in this study, AP was also the main factor affecting bacterial communities, and AK was the main factor affecting fungal communities (Figure 7). Fertilizers may be absorbed and used by plants after entering the soil, or they may remain in the soil, leading to changes in the composition of bacterial communities (Sun et al., 2016) and fungal communities (Zhou et al., 2016). Therefore, different fertilization treatments affected soil microbial community composition by mediating the effects of soil physicochemical properties, especially pH, and AP and AK.
SEM results showed that both soil TC and AK content was positively correlated to the quality (linoleic acid content) of P. frutescens (Figure 8), indicated that improve soil carbon and available potassium content could improve the quality of P. frutescens. There was a direct and significant positive correlation between P. frutescens yield and AP (Figure 8); this indicated that P. frutescens yield was mainly affected by the AP content in soil, and increasing the AP content can improve P. frutescens yield. Soil bacterial diversity was significantly positively correlated with soil AK (Figure 8A), but fungal diversity was significantly negatively correlated with soil AK (Figure 8B). Some previous studies showed that soil bacteria and fungi exhibited different patterns in response to fertilization treatments (Álvarez-Martín et al., 2016; Ai et al., 2018), and our study also supported this conclusion. Fungi are generally considered more closely related to plants and they are able to provide nutrients to plants in a symbiotic relationship (Chen et al., 2017), whereas bacteria are more affected by soil properties and environmental factors (Singh et al., 2008; Delgado-Baquerizo et al., 2016, 2018); our study obtained similar results (Figures 7, 8). In this study, we found that soil bacterial diversity was significantly negatively correlated with P. frutescens yield, and fungal diversity was significantly positively correlated with P. frutescens yield. This result may be opposite to many previous long-term fertilization studies. Because, in this study, short-term application of OF and OMF significantly increased P. frutescens yield (biomass per plant), but there was no significant change in bacterial diversity (Shannon index), and the value of OMF group even showed a downward trend (Figure 2). The application of organic fertilizer may stimulate the rapid growth of some dominant bacteria and beneficial bacteria in soil in a short time, while the abundance of some oligotrophic microorganisms that are not adapted to the existence of organic fertilizer will decline, resulting in the decrease of bacterial diversity. Through the correlation analysis among soil physicochemical properties, microbial communities and P. frutescens agronomic efficiency, it can be concluded that the application of OF or OMFs can improve the physicochemical properties of soil, especially the contents of total carbon, available potassium and available phosphorus, which can promote the quality and yield of P. frutescens.
Conclusion
The results of this study demonstrated that, under short-term fertilization management, OMF increased the total carbon and total potassium contents of soil. OF, especially OMF, improved measures of P. frutescens yield and quality, including the number of panicles per plant, length of the main panicle, and contents of unsaturated fatty acids such as α-linolenic acid, total fat, and total protein, while significantly increasing the number of beneficial microbial communities in the soil. The co-occurrence network analysis also revealed that OF and OMF improved the connectivity and stability of soil microbial communities. In conclusion, application of OF, especially OMF, is a good strategy to shape the composition of beneficial bacterial communities in the soil, and to improve soil fertility and crop yield and quality in karst areas.
Data availability statement
The datasets presented in this study can be found in online repositories. The names of the repository/repositories and accession number(s) can be found at: https://www.ncbi.nlm.nih.gov/, PRJNA836163; https://www.ncbi.nlm.nih.gov/, PRJNA836186.
Author contributions
BL and XL designed the study and modified the manuscript. YL and QS did the experimental work, carried out the data analysis, and wrote the manuscript. XA and YX contributed to the data analysis and original draft writing. All authors contributed to the article and approved the submitted version.
Funding
This work was supported by the Strategic Priority Research Program of the Chinese Academy of Science (XDA23060102), the Project on Social Development by Department of Science and Technology of Guizhou Province (SY-[2014]3041), the talents of Guizhou Science and Technology Cooperation Platform (2016-5648) and the Opening Fund of the State Key Laboratory of Environmental Geochemistry (SKLEG2021XXX).
Acknowledgments
Thanks to the editors and reviewers for their professional revision suggestions, which greatly improved the quality of this manuscript. We thank Mallory Eckstut, PhD, from Liwen Bianji (Edanz) (www.liwenbianji.cn) for editing the English text of a draft of this manuscript.
Conflict of interest
The authors declare that the research was conducted in the absence of any commercial or financial relationships that could be construed as a potential conflict of interest.
Publisher’s note
All claims expressed in this article are solely those of the authors and do not necessarily represent those of their affiliated organizations, or those of the publisher, the editors and the reviewers. Any product that may be evaluated in this article, or claim that may be made by its manufacturer, is not guaranteed or endorsed by the publisher.
Supplementary material
The Supplementary material for this article can be found online at: https://www.frontiersin.org/articles/10.3389/fmicb.2022.1058067/full#supplementary-material
Footnotes
References
Adams, R. I., Miletto, M., Taylor, J. W., and Bruns, T. D. (2013). Dispersal in microbes: fungi in indoor air are dominated by outdoor air and show dispersal limitation at short distances. ISME J. 7, 1262–1273. doi: 10.1038/ismej.2013.28
Aguilar, A. S., Cardoso, A. F., Lima, L. C., Luz, J. M. Q., Rodrigues, T., and Lana, R. M. Q. (2019). Influence of organomineral fertilization in the development of the potato crop CV. Cupid. Biosci. J. 35, 199–210. doi: 10.14393/BJ-v35n1a2019-41740
Ahimou, F., Jacques, P., and Deleu, M. (2000). Surfactin and iturin a effects on Bacillus subtilis surface hydrophobicity. Enzyme Microb. Technol. 27, 749–754. doi: 10.1016/S0141-0229(00)00295-7
Ai, C., Zhang, S., Zhang, X., Guo, D., Zhou, W., and Huang, S. (2018). Distinct responses of soil bacterial and fungal communities to changes in fertilization regime and crop rotation. Geoderma 319, 156–166. doi: 10.1016/j.geoderma.2018.01.010
Álvarez-Martín, A., Hilton, S. L., Bending, G. D., Rodriguez-Cruz, M. S., and SanchezMartin, M. J. (2016). Changes in activity and structure of the soil microbial community after application of azoxystrobin or pirimicarb and an organic amendment to an agricultural soil. Appl. Soil Ecol. 106, 47–57. doi: 10.1016/j.apsoil.2016.05.005
Antille, D. L., Godwin, J., Sakrabani, R., Seneweera, S., Tyrrel, S. F., and Johnstron, A. E. (2017). Field-scale evaluation of biosolids derived organomineral fertilizers applied to winter wheat in England. Agron. J. 109, 654–674. doi: 10.2134/agronj2016.09.0495
Banerjee, S., Schlaeppi, K., and van der Heijden, M. G. A. (2018). Keystone taxa as drivers of microbiome structure and functioning. Nat. Rev. Microbiol. 16, 567–576. doi: 10.1038/s41579-018-0024-1
Basak, B. B., Sarkar, B., and Naidu, R. (2021). Environmentally safe release of plant available potassium and micronutrients from organically amended rock mineral powder. Environ. Geochem. Health 43, 3273–3286. doi: 10.1007/s10653-020-00677-1
Bastian, M., Heymann, S., and Jacomy, M. (2009). “Gephi: an open source software for exploring and manipulating networks.” In The Third International AAAI Conference on Weblogs and Social Media LCWSM, San Jose, 361–362.
Bello, A., Wang, B., Zhao, Y., Yang, W., Ogundeji, A., Deng, L., et al. (2021). Composted biochar affects structural dynamics, function and co-occurrence network patterns of fungi community. Sci. Total Environ. 775:145672. doi: 10.1016/j.scitotenv.2021.145672
Benjamini, Y., and Hochberg, Y. (1995). Controlling the false discovery rate - a practical and powerful approach to multiple testing. J. R. Stat. Soc. B 57, 289–300. doi: 10.1111/j.2517-6161.1995.tb02031.x
Bissett, A., Richardson, A. E., Baker, G., and Thrall, P. H. (2011). Long-term land use effects on soil microbial community structure and function. Appl. Soil Ecol. 51, 66–78. doi: 10.1016/j.apsoil.2011.08.010
Biswas, D. R., Narayanasamy, G., Datta, S. C., Singh, G., Begum, M., Maiti, D., et al. (2009). Changes in nutrient status during preparation of enriched organomineral fertilizers using rice straw, low-grade rock phosphate, waste mica, and phosphate solubilizing microorganism. Commun. Soil Sci. Plant Anal. 40, 2285–2307. doi: 10.1080/00103620902961243
Bolger, A. M., Lohse, M., and Usadel, B. (2014). Trimmomatic: a flexible trimmer for Illumina sequence data. Bioinformatics 30, 2114–2120. doi: 10.1093/bioinformatics/btu170
Calaca, F. J. S., and Bustamante, M. M. C. (2022). Richness of arbuscular mycorrhizal fungi (Glomeromycota) along a vegetation gradient of Brazilian Cerrado: responses to seasonality, soil types, and plant communities. Mycol. Prog. 21:27. doi: 10.1007/s11557-022-01785-1
Carvalho, R. P., Moreira, R. A., Cruz, M. C. M., Fernandes, D. R., and Oliveira, A. F. (2014). Organomineral fertilization on the chemical characteristics of Quartzarenic Neosol cultivated with olive tree. Sci. Hortic. 176, 120–126. doi: 10.1016/j.scienta.2014.07.006
Chater, K. F., Biro, S., Lee, K. J., Palmer, T., and Schrempf, H. (2010). The complex extracellular biology of Streptomyces. FEMS Microbiol. Rev. 34, 171–198. doi: 10.1111/j.1574-6976.2009.00206.x
Chen, L., Liu, Y., Wu, G., Veronican Njeri, K., Shen, Q., Zhang, N., et al. (2016). Induced maize salt tolerance by rhizosphere inoculation of Bacillus amyloliquefaciens SQR9. Physiol. Plant. 158, 34–44. doi: 10.1111/ppl.12441
Chen, Y. L., Xu, T. L., Veresoglou, S. D., Hu, H. W., Hao, Z. P., Hu, Y. J., et al. (2017). Plant diversity represents the prevalent determinant of soil fungal community structure across temperate grasslands in northern China. Soil Biol. Biochem. 110, 12–21. doi: 10.1016/j.soilbio.2017.02.015
Cho, S. J., Kim, M. H., and Lee, Y. O. (2016). Effect of pH on soil bacterial diversity. J. Environ. Prot. Ecol. 40, 75–83. doi: 10.1186/s41610-016-0004-1
Coller, E., Cestaro, A., Zanzotti, R., Bertoldi, D., Pindo, M., Larger, S., et al. (2019). Microbiome of vineyard soils is shaped by geography and management. Microbiome 7:170. doi: 10.1186/s40168-019-0758-7
Conn, V., Walker, A., and Franco, C. (2008). Endophytic actinobacteria induce defense pathways in Arabidopsis thaliana. Mol. Plant-Microbe Interact. 21, 208–218. doi: 10.1094/MPMI-21-2-0208
Corrêa, J. C., Rebellatto, A., Grohskopf, M. A., Cassol, P. C., Hentz, P., and Rigo, A. Z. (2018). Soil fertility and agriculture yield with the application of organomineral or mineral fertilizers in solid and fluid forms. Pesqui. Agropecu. Bras. 53, 633–640. doi: 10.1590/S0100-204X2018000500012
Costa-Gutierrez, S. B., Raimondo, E. E., Lami, M. J., Vincent, P. A., Espinosa-Urgel, M., and de Cristóbal, R. E. (2020). Inoculation of Pseudomonas mutant strains can improve growth of soybean and corn plants in soils under salt stress. Rhizosphere 16:100255. doi: 10.1016/j.rhisph.2020.100255
Csardi, G., and Nepusz, T. (2006). The igraph software package for complex network research. InterJ. Complex Syst. 1695, 1–9.
Cui, X., Zhang, Y., Gao, J., Peng, F., and Gao, P. (2018). Long-term combined application of manure and chemical fertilizer sustained higher nutrient status and rhizospheric bacterial diversity in reddish paddy soil of Central South China. Sci. Rep. 8:16554. doi: 10.1038/s41598-018-34685-0
Dai, Z., Su, W., Chen, H., Barberán, A., Zhao, H., Yu, M., et al. (2018). Long-term nitrogen fertilization decreases bacterial diversity and favors the growth of Actinobacteria and Proteobacteria in agroecosystems across the globe. Glob. Chang. Biol. 24, 3452–3461. doi: 10.1111/gcb.14163
Deeks, L., Chaney, K., Murray, C., Sakrabani, R., Gedara, S., Le, M., et al. (2013). A new sludge-derived organo-mineral fertilizer gives similar crop yields as conventional fertilizers. Agron. Sustain. Dev. 33, 539–549. doi: 10.1007/s13593-013-0135-z
Delgado-Baquerizo, M., Oliverio, A. M., Brewer, T. E., Benavent-Gonzalez, A., Eldridge, D. J., Bardgett, R. D., et al. (2018). A global atlas of the dominant bacteria found in soil. Science 359, 320–325. doi: 10.1126/science.aap9516
Delgado-Baquerizo, M., Reich, P. B., Khachane, A. N., Campbell, C. D., Thomas, N., Freitag, T. E., et al. (2016). It is elemental: soil nutrient stoichiometry drives bacterial diversity. Environ. Microbiol. 19, 1176–1188. doi: 10.1111/1462-2920.13642
Deng, Y., Jiang, Y. H., Yang, Y. F., He, Z. L., Luo, F., and Zhou, J. Z. (2012). Molecular ecological network analyses. BMC Bioinf. 13:113. doi: 10.1186/1471-2105-13-113
Dias, M. A. D., Lana, R. M. Q., Mageste, J. G., Marques, O. J., Silva, A. D., Lemes, E. M., et al. (2020). Mineral and organomineral sources of nitrogen to maize agronomic performance. Biosci. J. 36, 1528–1534. doi: 10.14393/BJ-v36n5a2020-45632
Dixon, P. (2003). VEGAN, a package of R functions for community ecology. J. Veg. Sci. 14, 927–930. doi: 10.1111/j.1654-1103.2003.tb02228.x
Du, T. Y., He, H. Y., Zhang, Q., Lu, L., Mao, W. J., and Zhai, M. Z. (2022). Positive effects of organic fertilizers and biofertilizers on soil microbial community composition and walnut yield. Appl. Soil Ecol. 175:104457. doi: 10.1016/j.apsoil.2022.104457
Edgar, R. C. (2013). UPARSE: highly accurate OTU sequences from microbial amplicon reads. Nat. Methods 10, 996–998. doi: 10.1038/Nmeth.2604
Efanov, M. V., Galochkin, A. I., Schott, P. R., Dudkin, D. V., and Klepikov, A. G. (2001). Nitrogen-containing organomineral fertilizer based on wood waste. Russ. J. Appl. Chem. 74, 1774–1776. doi: 10.1023/A:1014850528793
Fan, K., Delgado-Baquerizo, M., Guo, X., Wang, D., Zhu, Y., and Chu, H. (2020). Biodiversity of key-stone phylotypes determines crop production in a 4-decade fertilization experiment. ISME J. 15, 550–561. doi: 10.1038/s41396-020-00796-8
Finkel, O. M., Salas-Gonzalez, I., Castrillo, G., Conway, J. M., Law, T. F., Teixeira, P. J. P. L., et al. (2020). A single bacterial genus maintains root growth in a complex microbiome. Nature 587, 103–108. doi: 10.1038/s41586-020-2778-7
Frazão, J. J., Benites, V. M., Ribeiro, J. V. S., Pierobon, V. M., and Lavres, J. (2019). Agronomic effectiveness of a granular poultry litter-derived organomineral phosphate fertilizer in tropical soils: soil phosphorus fractionation and plant responses. Geoderma 337, 582–593. doi: 10.1016/j.geoderma.2018.10.003
Gattinger, A., Muller, A., Haeni, M., Skinner, C., Fliessbach, A., Buchmann, N., et al. (2012). Enhanced top soil carbon stocks under organic farming. Proc. Natl. Acad. Sci. U. S. A. 109, 18226–18231. doi: 10.1073/pnas.1209429109
Gebremariam, A., Chekol, Y., and Assefa, F. (2021). Phenotypic, molecular, and virulence characterization of entomopathogenic fungi, Beauveria bassiana (Balsam) Vuillemin, and Metarhizium anisopliae (Metschn.) Sorokin from soil samples of Ethiopia for the development of mycoinsecticide. Heliyon 7:e07091. doi: 10.1016/j.heliyon.2021.e07091
Ghorbanzadeh, N., Mahsefat, M., Farhangi, M. B., Khalili Rad, M., and Proietti, P. (2020). Short-term impacts of pomace application and Pseudomonas bacteria on soil available phosphorus. Biocatal. Agric. Biotechnol. 28:101742. doi: 10.1016/j.bcab.2020.101742
Gomiero, T., Pimentel, D., and Paoletti, M. G. (2011). Environmental impact of different agricultural management practices: conventional vs. organic agriculture. Crit. Rev. Plant Sci. 30, 95–124. doi: 10.1080/07352689.2011.554355
Gonçalves, C. A., de Camargo, R., de Sousa, R. T. X., Soares, N. S., de Oliveira, R. C., Stanger, M. C., et al. (2021). Chemical and technological attributes of sugarcane as functions of organomineral fertilizer based on filter cake or sewage sludge as organic matter sources. PLoS One 16:e0236852. doi: 10.1371/journal.pone.0236852
Gong, Z. T. (1999). Soil system classification in China-theory, method and practiceBeijing: Science press.
González, A. M. M., Dalsgaard, B., and Olesen, J. M. (2010). Centrality measures and the importance of generalist species in pollination networks. Ecol. Complex. 7, 36–43. doi: 10.1016/j.ecocom.2009.03.008
González-Pérez, E., Ortega-Amaro, M. A., Bautista, E., Delgado-Sanchez, P., and Jimenez-Bremont, J. F. (2022). The entomopathogenic fungus Metarhizium anisopliae enhances Arabidopsis, tomato, and maize plant growth. Plant Physiol. Biochem. 176, 34–43. doi: 10.1016/j.plaphy.2022.02.008
Grohskopf, M. A., Corrêa, J. C., Fernandes, D. M., Teixeira, P. C., and Mota, S. C. A. (2019). Mobility of nitrogen in the soil due to the use of organomineral fertilizers with different concentrations of phosphorus. Commun. Soil Sci. Plant Anal. 51, 208–220. doi: 10.1080/00103624.2019.1705321
Gu, S., Hu, Q., Cheng, Y., Bai, L., Liu, Z., Xiao, W., et al. (2019). Application of organic fertilizer improves microbial community diversity and alters microbial network structure in tea (Camellia sinensis) plantation soils. Soil Tillage Res. 195:104356. doi: 10.1016/j.still.2019.104356
Gui, H., Fan, L., Wang, D., Yan, P., Li, X., Zhang, L., et al. (2021). Organic management practices shape the structure and associations of soil bacterial communities in tea plantations. Appl. Soil Ecol. 163:103975. doi: 10.1016/j.apsoil.2021.103975
Hawrot-Paw, M., Mikiciuk, M., Koniuszy, A., and Meller, E. (2022). Influence of organomineral fertiliser from sewage sludge on soil microbiome and physiological parameters of maize (Zea mays L.). Agronomy 12:1114. doi: 10.3390/agronomy12051114
Hu, Y., Sun, L. W., Mokgolodi, N. C., Zhang, Y. X., Wen, C. X., Xie, X. L., et al. (2010). Primary identifications and palynological observations of Perilla in China. J. Syst. Evol. 48, 133–145. doi: 10.1111/j.1759-6831.2010.00067.x
Javier, S., Canales, F., Tweed, J., and Lee, M. (2018). Fatty acid profile changes during gradual soil water depletion in oats suggests a role for jasmonates in coping with drought. Front. Plant Sci. 9:1007. doi: 10.3389/fpls.2018.0107
Jiao, S., Lu, Y., and Wei, G. (2021). Soil multitrophic network complexity enhances the link between biodiversity and multifunctionality in agricultural systems. Glob. Chang. Biol. 28, 140–153. doi: 10.1111/gcb.15917
Kang, Y., Ma, Y., Wu, W., Zeng, S., Jiang, S., Yang, H., et al. (2022). Bioorganic and silicon amendments alleviate early defoliation of pear trees by improving the soil nutrient bioavailability, microbial activity, and reshaping the soil microbiome network. Appl. Soil Ecol. 173:104383. doi: 10.1016/j.apsoil.2021.104383
Kembel, S. W., Cowan, P. D., Helmus, M. R., Cornwell, W. K., Morlon, H., Ackerly, D. D., et al. (2010). Picante: R tools for integrating phylogenies and ecology. Bioinformatics 26, 1463–1464. doi: 10.1093/bioinformatics/btq166
Ko, O., Jn, O., and Hc, O. (2018). Pharmacological potentials, characterization and fatty acids profile of Persea americana mill. (Avocardo) seed oil using gas chromatography-mass spectroscopy. Biochem. Anal. Biochem. 7, 1–3. doi: 10.4172/2161-1009.100036
Ko, W. H., Tsou, Y. J., Ju, Y. M., Hsieh, H. M., and Ann, P. J. (2010). Production of a fungistatic substance by Pseudallescheria boydii isolated from soil amended with vegetable tissues and its significance. Mycopathologia 169, 125–131. doi: 10.1007/s11046-009-9237-1
Kour, D., Rana, K. L., Yadav, A. N., Yadav, N., Kumar, M., Kumar, V., et al. (2020). Microbial biofertilizers: bioresources and ecofriendly technologies for agricultural and environmental sustainability. Biocatal. Agric. Biotechnol. 23:101487. doi: 10.1016/j.bcab.2019.101487
Kourimska, L., Sabolova, M., Horcicka, P., Rys, S., and Bozik, M. (2018). Lipid content, fatty acid profile, and nutritional value of new oat cultivars. J. Cereal Sci. 84, 44–48. doi: 10.1016/j.jcs.2018.09.012
Kox, M., Elzen, E., Lamers, L. P. M., Jetten, M. S. M., and Kessel, M. (2020). Microbial nitrogen fixation and methane oxidation are strongly enhanced by light in Sphagnum mosses. AMB Expr. 10:61. doi: 10.1186/s13568-020-00994-9
Lauber, C. L., Hamady, M., Knight, R., and Fierer, N. (2009). Pyrosequencing–based assessment of soil pH as a predictor of soil bacterial community structure at the continental scale. Appl. Environ. Microbiol. 75, 5111–5120. doi: 10.1128/AEM.00335-09
Lee, J. K., and Kim, N. S. (2007). Genetic diversity and relationships of cultivated and weedy types of Perilla frutescens collected from East Asia revealed by SSR markers. Korean J. Breed. Sci 39:491–499.
Li, Y., Liu, X. M., Yin, Z. Y., Chen, H., Cai, X. L., Xie, Y. H., et al. (2021). Changes in soil microbial communities from exposed rocks to arboreal rhizosphere during vegetation succession in a karst mountainous ecosystem. J. Plant Interact. 16, 550–563. doi: 10.1080/17429145.2021.2002955
Li, Y., Liu, X. M., Zhang, L., Xie, Y. H., Cai, X. L., Wang, S. J., et al. (2020). Effects of short-term application of chemical and organic fertilizers on bacterial diversity of cornfield soil in a karst area. J. Soil Sci. Plant Nut. 20, 2048–2058. doi: 10.1007/s42729-020-00274-2
Li, Q., Zhang, D., Song, Z., Ren, L., Jin, X., Fang, W., et al. (2022). Organic fertilizer activates soil beneficial microorganisms to promote strawberry growth and soil health after fumigation. Environ. Pollut. 295:118653. doi: 10.1016/j.envpol.2021.118653
Lian, B., Xiao, B., Xiao, L. L., Wang, W. J., and Sun, Q. B. (2020). Molecular mechanism and carbon sink effects of microbial transformation in potassium- bearing rocks. Earth Sci. Front. 27, 238–246. doi: 10.13745/j.esf.sf.2020.5.38
Ling, N., Zhu, C., Xue, C., Chen, H., Duan, Y., Peng, C., et al. (2016). Insight into how organic amendments can shape the soil microbiome in long-term field experiments as revealed by network analysis. Soil Biol. Biochem. 99, 137–149. doi: 10.1016/j.soilbio.2016.05.005
Liu, X., Bolla, K., Ashforth, E. J., Zhou, Y., Gao, H., and Huang, P. (2012). Systematics-guided bioprospecting for bioactive microbial natural products. Antonie Van Leeuwenhoek 101, 55–66. doi: 10.1007/s10482-011-9671-1
Liu, L., Chen, H., Liu, M., Yang, J. R., Xiao, P., Wilkinson, D. M., et al. (2019). Response of the eukaryotic plankton community to the cyanobacterial biomass cycle over 6 years in two subtropical reservoirs. ISME J. 13, 2196–2208. doi: 10.1038/s41396-019-0417-9
Liu, Z., Guo, Q., Feng, Z. Y., Liu, Z. D., Li, H. Y., Sun, Y. F., et al. (2020). Long-term organic fertilization improves the productivity of kiwifruit (Actinidia chinensis Planch.) through increasing rhizosphere microbial diversity and network complexity. Appl. Soil Ecol. 147:103426. doi: 10.1016/j.apsoil.2019.103426
Liu, H., Pan, F., Han, X., Song, F., Zhang, Z., Yan, J., et al. (2019). Response of soil fungal community structure to long-term continuous soybean cropping. Front. Microbiol. 9:3316. doi: 10.3389/fmicb.2018.03316
Liu, J., Shu, A., Song, W., Shi, W., and Gao, Z. (2021). Long-term organic fertilizer substitution increases rice yield by improving soil properties and regulating soil bacteria. Geoderma 404:115287. doi: 10.1016/j.geoderma.2021.115287
Liu, J., Sui, Y., Yu, Z., Shi, Y., Chu, H., Jin, J., et al. (2014). High throughput sequencing analysis of biogeographical distribution of bacterial communities in the black soils of Northeast China. Soil Biol. Biochem. 70, 113–122. doi: 10.1016/j.soilbio.2013.12.014
Louca, S., Parfrey, L. W., and Doebeli, M. (2016). Decoupling function and taxonomy in the global ocean microbiome. Science 353, 1272–1277. doi: 10.1126/science.aaf4507
Lozupone, C., and Knight, R. (2005). UniFrac: a new phylogenetic method for comparing microbial communities. Appl. Environ. Microbiol. 71, 8228–8235. doi: 10.1128/AEM.71.12.8228-8235.2005
Lu, R. K. (1999). Methods of agrochemical soil analysis vol China Agricultural Science Press, Beijing.
Luitel, B. P., Ko, H. C., Hur, O. S., Rhee, J. H., Baek, H. J., Ryu, K. Y., et al. (2017). Variation for morphological characters in cultivated and weedy types of Perilla frutescens Britt. germplasm. Korean J. Plant Resour. 30, 298–310. doi: 10.7732/kjpr.2017.30.3.298
Ma, B., Wang, H. Z., Dsouza, M., Lou, J., He, Y., Dai, Z. M., et al. (2016). Geographic patterns of co-occurrence network topological features for soil microbiota at continental scale in eastern China. ISME J. 10, 1891–1901. doi: 10.1038/ismej.2015.261
Ma, A., Zhuang, X., Wu, J., Cui, M., Lv, D., Liu, C., et al. (2013). Ascomycota members dominate fungal communities during straw residue decomposition in arable soil. PLoS One 8:e66146. doi: 10.1371/journal.pone.0066146
Magoc, T., and Salzberg, S. L. (2011). FLASH: fast length adjustment of short reads to improve genome assemblies. Bioinformatics 27, 2957–2963. doi: 10.1093/bioinformatics/btr507
Marcos, M. S., Bertiller, M. B., and Olivera, N. L. (2019). Microbial community composition and network analyses in arid soils of the Patagonian Monte under grazing disturbance reveal an important response of the community to soil particle size. Appl. Soil Ecol. 138, 223–232. doi: 10.1016/j.apsoil.2019.03.001
Megali, L., Glauser, G., and Rasmann, S. (2013). Fertilization with beneficial microorganisms decreases tomato defenses against insect pests. Agron. Sustain. Dev. 34, 649–656. doi: 10.1007/s13593-013-0187-0
Mendes, L. W., de Lima Brossi, M. J., Kuramae, E. E., and Tsai, S. M. (2015). Land-use system shapes soil bacterial communities in southeastern Amazon region. Appl. Soil Ecol. 95, 151–160. doi: 10.1016/j.apsoil.2015.06.005
Moyne, A. L., Cleveland, T. E., and Tuzun, S. (2004). Molecular characterization and analysis of the operon encoding the antifungal lipopeptide bacillomycin D. FEMS Microb. Lett. 234, 43–49. doi: 10.1016/j.femsle.2004.03.011
Mumbach, G. L., Gatiboni, L. C., Bona, F. D., Schmitt, D. E., Corrêa, J. C., Gabriel, C. A., et al. (2020). Agronomic efficiency of organomineral fertilizer in sequential grain crops in southern Brazil. Agron. J. 112, 3037–3049. doi: 10.1002/agj2.20238
Ngo, H. T. T., Watts-Williams, S. J., Panagaris, A., Baird, R., McLaughlin, M. J., and Cavagnaro, T. R. (2022). Development of an organomineral fertiliser formulation that improves tomato growth and sustains arbuscular mycorrhizal colonisation. Sci. Total Environ. 815:151977. doi: 10.1016/j.scitotenv.2021.151977
Nguyen, N. H., Song, Z. W., Bates, S. T., Branco, S., Tedersoo, L., Menke, J., et al. (2016). FUNGuild: an open annotation tool for parsing fungal community datasets by ecological guild. Fungal Ecol. 20, 241–248. doi: 10.1016/j.funeco.2015.06.006
Qi, D., Wieneke, X., Tao, J., Zhou, X., and Desilva, U. (2018). Soil pH is the primary factor correlating with soil microbiome in karst rocky desertification regions in the Wushan County, Chongqing, China. Front. Microbiol. 9:1027. doi: 10.3389/fmicb.2018.01027
Ren, H., Qin, X., Huang, B., Fernández-García, V., and Lv, C. (2020). Responses of soil enzyme activities and plant growth in a eucalyptus seedling plantation amended with bacterial fertilizers. Arch. Microbiol. 202, 1381–1396. doi: 10.1007/s00203-020-01849-4
Ren, C. J., Zhao, F. Z., Kang, D., Yang, G. H., Han, X. H., Tong, X. G., et al. (2016). Linkages of C:N:P stoichiometry and bacterial community in soil following afforestation of former farmland. Forest Ecol. Manag. 376, 59–66. doi: 10.1016/j.foreco.2016.06.004
Richardson, M. (2009). The ecology of the zygomycetes and its impact on environmental exposure. Clin. Microbiol. Infect. 15, 2–9. doi: 10.1111/j.1469-0691.2009.02972.x
Riguetti Zanardo Botelho, A. B., Alves-Pereira, A., Colonhez Prado, R., Zucchi, M. I., and Delalibera Júnior, I. (2019). Metarhizium species in soil from Brazilian biomes: a study of diversity, distribution, and association with natural and agricultural environments. Fungal Ecol. 41, 289–300. doi: 10.1016/j.funeco.2019.07.004
Rime, T., Hartmann, M., Brunner, I., Widmer, F., Zeyer, J., and Frey, B. (2015). Vertical distribution of the soil microbiota along a successional gradient in a glacier forefield. Mol. Ecol. 24, 1091–1108. doi: 10.1111/mec.13051
Sakurada, L. R., Batista, M. A., Inoue, T. T., Muniz, A. S., and Pagliari, P. H. (2016). Organomineral phosphate fertilizers: agronomic efficiency and residual effect on initial corn development. Agron. J. 108, 2050–2059. doi: 10.2134/agronj2015.0543
Samaddar, S., Truu, J., Chatterjee, P., Truu, M., Kim, K., Kim, S., et al. (2019). Long-term silicate fertilization increases the abundance of actinobacterial population in paddy soils. Biol. Fertil. Soils 55, 109–120. doi: 10.1007/s00374-018-01335-6
Santolini, M., and Barabási, A. L. (2018). Predicting perturbation patterns from the topology of biological networks. Proc. Natl. Acad. Sci. U. S. A. 115, E6375–E6383. doi: 10.1073/pnas.1720589115
Segata, N., Izard, J., Waldron, L., Gevers, D., Miropolsky, L., Garrett, W. S., et al. (2011). Metagenomic biomarker discovery and explanation. Genome Biol. 12:R60. doi: 10.1186/gb-2011-12-6-r60
Seufert, V., Ramankutty, N., and Foley, J. A. (2012). Comparing the yields of organic and conventional agriculture. Nature 485, 229–232. doi: 10.1038/nature11069
Singh, B. K., Nunan, N., Ridgway, K. P., McNicol, J., Young, J. P. W., Daniell, T. J., et al. (2008). Relationship between assemblages of mycrorrhizal fungi and bacteria on grass roots. Environ. Microbiol. 10, 534–541. doi: 10.1111/j.1462-2920.2007.01474.x
Smith, S. E., and Read, D. J. (2008). “The symbionts forming arbuscular mycorrhizas,” in Mycorrhizal Symbiosis. eds. S. E. Smith and D. J. Read (San Diego: Academic Press), 13–41.
Steffen, W., Richardson, K., Rockstrom, J., Cornell, S. E., Fetzer, I., Bennett, E. M., et al. (2015). Planetary boundaries: guiding human development on a changing planet. Science 347:1259855. doi: 10.1126/science.1259855
Sun, R., Dsouza, M., Gilbert, J. A., Guo, X., Wang, D., Guo, Z., et al. (2016). Fungal community composition in soils subjected to long-term chemical fertilization is most influenced by the type of organic matter. Environ. Microbiol. 18, 5137–5150. doi: 10.1111/1462-2920.13512
Sun, A., Jiao, X. Y., Chen, Q., Wu, A. L., Zheng, Y., Lin, Y. X., et al. (2021). Microbial communities in crop phyllosphere and root endosphere are more resistant than soil microbiota to fertilization. Soil Biol. Biochem. 153:108113. doi: 10.1016/j.soilbio.2020.108113
Sun, Q. B., Ruan, Y. L., Chen, P., Wang, S. J., Liu, X. M., and Lian, B. (2019). Effects of mineral-organic fertilizer on the biomass of green Chinese cabbage and potential carbon sequestration ability in karst areas of Southwest China. Acta Geochim. 38, 430–439. doi: 10.1007/s11631-019-00320-6
Syed, S., Wang, X., Prasad, T. N. V. K. V., and Lian, B. (2021). Bio-organic-mineral fertilizer for sustainable agriculture: current trends and future perspectives. Fortschr. Mineral. 11:1336. doi: 10.3390/min11121336
Theodoro, S. H., and Leonardos, O. H. (2006). The use of rocks to improve family agriculture in Brazil. An. Acad. Bras. Cienc. 78, 721–730. doi: 10.1590/S0001-37652006000400008
Tian, S. G., Guo, P., Shen, Q., Wang, X. P., Yang, S., Shang, Z. W., et al. (2017). Main traits and quality of seven Perilla frutescens varieties (lines) in Guizhou. Guizhou Agric. Sci. 45, 107–109.
Tian, J., Zeng, X., Zhang, S., Wang, Y., Zhang, P., Lü, A., et al. (2014). Regional variation in components and antioxidant and antifungal activities of Perilla frutescens essential oils in China. Ind. Crop. Prod. 59, 69–79. doi: 10.1016/j.indcrop.2014.04.048
USS Working Group WRB. (2015). World reference base for soil resources 2014, update 2015 international soil classification system for naming soils and creating legends for soil maps. World Soil Resources Reports No. 106. FAO, Rome.
Vick-Majors, T. J., Priscu, J. C., and Amaral-Zettler, L. A. (2014). Modular community structure suggests metabolic plasticity during the transition to polar night in ice-covered Antarctic lakes. ISME J. 8, 778–789. doi: 10.1038/ismej.2013.190
Vollú, R. E., Cotta, S. R., Jurelevicius, D., Leite, D. C. A., Parente, C. E. T., Malm, O., et al. (2018). Response of the bacterial communities associated with maize rhizosphere to poultry litter as an organomineral fertilizer. Front. Environ. Sci. 6:118. doi: 10.3389/fenvs.2018.00118
Wang, Y., Chen, G. W., Sun, Y. F., Zhu, K., Jin, Y., Li, B. G., et al. (2020). Different agricultural practices specify bacterial community compositions in the soil rhizosphere and root zone. Soil Ecol. Lett. 4, 18–31. doi: 10.1007/s42832-020-0058-y
Wang, L., Li, J., Yang, F., E, Y., Raza, W., Huang, Q., et al. (2017). Application of bioorganic fertilizer significantly increased apple yields and shaped bacterial community structure in orchard soil. Microb. Ecol. 73, 404–416. doi: 10.1007/s00248-016-0849-y
Wang, C. J., Song, C. Y., Zhang, X. D., Xie, Y. Q., Liu, X. Z., and Wang, M. Z. (1997). Sustainable control efficacy of Paecilomyces lilacinus against Heterodera glycines. Chin. J. Biol. Contr. 13:26.
Weller, D., Raaijmakers, J., Gardener, B., and Thomashow, L. (2002). Microbial populations responsible for specific soil suppressiveness to plant pathogens. Annu. Rev. Phytopathol. 40, 309–348. doi: 10.1146/annurev.phyto.40.030402.110010
Wu, S., Zhou, Z., Zhu, L., Zhong, L., Dong, Y., Wang, G., et al. (2022). Cd immobilization mechanisms in a Pseudomonas strain and its application in soil Cd remediation. J. Hazard. Mater. 425:127919. doi: 10.1016/j.jhazmat.2021.127919
Xu, N., Tan, G. C., Wang, H. Y., and Gai, X. P. (2016). Effect of biochar additions to soil on nitrogen leaching, microbial biomass and bacterial community structure. Eur. J. Soil Biol. 74, 1–8. doi: 10.1016/j.ejsobi.2016.02.004
Yaldiz, G., and Camlica, M. (2020). Yield components and some quality properties of fenugreek cultivar and lines. Banat′s. J. Biotechnol. XI, 40–47. doi: 10.7904/2068-4738-XI(22)-40
Yang, H. W., Li, J., Xiao, Y. H., Gu, Y. B., Liu, H. W., and Liang, Y. L. (2017). An integrated insight into the relationship between soil microbial community and tobacco bacterial wilt disease. Front. Microbiol. 8:2179. doi: 10.3389/fmicb.2017.02179
Yang, Y. R., Syed, S., Mao, S. X., Li, Q., Ge, F., Lian, B., et al. (2020). Bioorganic–mineral fertilizer can remediate chemical fertilizer-oversupplied soil: Purslane planting as an example. J. Soil Sci. Plant Nutr. 20, 892–900. doi: 10.1007/s42729-020-00175-4
Yu, H., Qiu, J. F., Ma, L. J., Hu, Y. J., Li, P., and Wan, J. B. (2017). Phytochemical and phytopharmacological review of Perilla frutescens L. (Labiatae), a traditional edible-medicinal herb in China. Food Chem. Toxicol. 108, 375–391. doi: 10.1016/j.fct.2016.11.023
Yuan, M. M., Guo, X., Wu, L., Zhang, Y., Xiao, N., Ning, D., et al. (2021). Climate warming enhances microbial network complexity and stability. Nat. Clim. Chang. 11, 343–348. doi: 10.1038/s41558-021-00989-9
Zhao, J., Ni, T., Li, J., Lu, Q., Fang, Z., Huang, Q., et al. (2016). Effects of organic–inorganic compound fertilizer with reduced chemical fertilizer application on crop yields, soil biological activity and bacterial community structure in a rice–wheat cropping system. Appl. Soil Ecol. 99, 1–12. doi: 10.1016/j.apsoil.2015.11.006
Zhou, J., Guan, D., Zhou, B., Zhao, B., Ma, M., Qin, J., et al. (2015). Influence of 34-years of fertilization on bacterial communities in an intensively cultivated black soil in Northeast China. Soil Biol. Biochem. 90, 42–51. doi: 10.1016/j.soilbio.2015.07.005
Zhou, J., Jiang, X., Zhou, B. K., Zhao, B. S., Ma, M. C., Guan, D. W., et al. (2016). Thirty-four years of nitrogen fertilization decreases fungal diversity and alters fungal community composition in black soil in Northeast China. Soil Biol. Biochem. 95, 135–143. doi: 10.1016/j.soilbio.2015.12.012
Zhu, J., Ren, Z., Huang, B., Cao, A., Wang, Q., Yan, D., et al. (2020). Effects of fumigation with allyl isothiocyanate on soil microbial diversity and community structure of tomato. J. Agric. Food Chem. 68, 1226–1236. doi: 10.1021/acs.jafc.9b07292
Keywords: karst soil, organomineral fertilizer, Perilla frutescens, nutritional quality, rhizosphere microbial community
Citation: Li Y, Shen Q, An X, Xie Y, Liu X and Lian B (2022) Organomineral fertilizer application enhances Perilla frutescens nutritional quality and rhizosphere microbial community stability in karst mountain soils. Front. Microbiol. 13:1058067. doi: 10.3389/fmicb.2022.1058067
Edited by:
Hongchen Jiang, China University of Geosciences Wuhan, ChinaReviewed by:
Xiaojing Hu, Northeast Institute of Geography and Agroecology (CAS), ChinaXiubing Gao, Guizhou Tea Research Institute, China
Copyright © 2022 Li, Shen, An, Xie, Liu and Lian. This is an open-access article distributed under the terms of the Creative Commons Attribution License (CC BY). The use, distribution or reproduction in other forums is permitted, provided the original author(s) and the copyright owner(s) are credited and that the original publication in this journal is cited, in accordance with accepted academic practice. No use, distribution or reproduction is permitted which does not comply with these terms.
*Correspondence: Xiuming Liu, bGl1eGl1bWluZ0B2aXAuc2tsZWcuY24=; Bin Lian, YmluMjM2OEB2aXAuMTYzLmNvbQ==
†These authors have contributed equally to this work and share first authorship