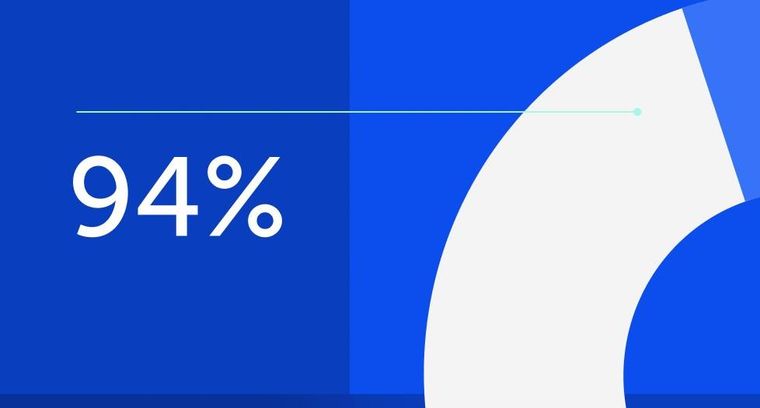
94% of researchers rate our articles as excellent or good
Learn more about the work of our research integrity team to safeguard the quality of each article we publish.
Find out more
ORIGINAL RESEARCH article
Front. Microbiol., 16 December 2022
Sec. Microorganisms in Vertebrate Digestive Systems
Volume 13 - 2022 | https://doi.org/10.3389/fmicb.2022.1054097
This article is part of the Research TopicRodent model organisms: Human diets and the gut microbiomeView all 23 articles
Microorganisms with probiotic properties are eliciting an increasing interest as coadjuvants in the prevention and treatment of obesity through modulation of the gut microbiota. In this study, a probiotic formulation based on Enterococcus faecium SF68 was administered to mice fed with a high-fat diet (HFD) to evaluate its efficacy in reducing body mass gain and in modulating the intestinal bacterial composition. Both stool and ileum samples were collected from untreated and treated mice and absolute abundances of specific taxa constituting the gut microbial consortium were evaluated. SF68 administration significantly reduced the HFD-induced weight gain. In these animals, the microbial gut composition shifted toward an enrichment in microbes positively correlated with mucus thickness, lower inflammation, lower glycemia levels, and SCFA production (i.e., Bifidobacterium, Akkermansia, and Faecalibacterium), as well as a depletion in bacterial phyla having a key role in obesity (i.e., Firmicutes, Proteobacteria). Our results demonstrate the efficacy of E. faecium SF68 in adjusting the composition of the dysbiotic microbiota of HFD-fed animals, thus ameliorating clinical conditions and exerting anti-obesity effects.
The administration of probiotics, “live microorganisms which, when administered in adequate amounts, confer a health benefit on the host” (Food and Agricultural Organization of the United Nations and World Health Organization, 2001), is often effective in ameliorating the symptoms of a wide variety of clinical disorders in humans. These include antibiotic-associated diarrhea (Mekonnen et al., 2020), infectious and Clostridioides difficile-associated diarrhea (Sánchez et al., 2017), irritable bowel syndrome (Moayyedi et al., 2010), type 2 diabetes (Ejtahed et al., 2011), inflammatory bowel diseases [e.g., ulcerative colitis (Ganji-Arjenaki and Rafieian-Kopaei, 2018) and obesity (Tenorio-Jiménez et al., 2020)], through countless but poorly investigated mechanisms of action.
Exploiting probiotic microorganisms as coadjuvants in the treatment or prevention of obesity is of clinical relevance. Obesity is a pathological condition that consists of abnormal or excessive fat accumulation, which in turn often drives to other chronic disorders (Cerdó et al., 2019), such as metabolic syndrome, insulin-resistance, and cardiovascular pathologies (Frasca et al., 2017). Moreover, this condition is typically associated with several other biomarkers. Due to aberrant intestinal permeability and mucus thickness, obese patients display high levels of lipopolysaccharide in the bloodstream (Green et al., 2020), a condition known as metabolic endotoxemia, which can lead to a constant state of systemic low-grade inflammation (Cani et al., 2007a). This prolonged inflammatory state contributes to insulin-resistance, triggering the onset of type-2 diabetes and metabolic issues (Cani et al., 2007b). Several studies showed how different probiotic strains were able to improve obesity-related conditions (Yadav et al., 2013; Bagarolli et al., 2017; Ejtahed et al., 2019). By restoring mucus thickness and tight junctions’ expression or regulating the production of different cytokines, probiotics can improve the gut barrier integrity and modulate immune functions (Cerdó et al., 2019), as well as stimulate the secretion of satiety-related hormones through the production of short chain fatty acids (SCFAs; Da Silva et al., 2021). Besides these specific mechanisms of action, probiotics can also modulate the gut microbiota composition, thus triggering cascading effects that affect the entire organism (Azad et al., 2018). Considering the severe alteration in the fecal microbiota observed in obese patients, with higher levels of Firmicutes (Abenavoli et al., 2019) and Actinobacteria (Bibbò et al., 2016) and lower abundances of Bifidobacterium and Akkermansia (Gérard, 2016) than normal-weight subjects, the administration of probiotics may be considered a potential therapeutic approach aimed at restoring eubiosis of the intestinal flora and counteracting the clinical scenario of obesity.
Previous studies demonstrated the efficacy of selected Enterococcus strains in a variety of conditions (i.e., intestinal dysbiosis, metabolic syndrome, high levels of serum cholesterol and triglycerides, and inflammation) due to their recognized ability to secrete propionate and butyrate, hydrolyze bile salts, inhibit the transcription of pro-inflammatory mediators, and modulate the composition of the gut microbiota (Allen et al., 2010; Avram-Hananel et al., 2010; Tarasova et al., 2010; Zhang et al., 2017; Greuter et al., 2020; Mishra and Ghosh, 2020; Huang et al., 2021). Considering the overall positive effects demonstrated in these studies, enterococci seem to be promising candidates for the prevention and treatment of obesity and amelioration of other obesity-related complications.
In this study, Enterococcus faecium SF68 was orally administered to mice fed with a high-fat diet (HFD) to evaluate its efficacy in reducing body mass gain, modulating the gut microbiota composition, and restoring a well-balanced intestinal flora. Quantitative analyses were performed to obtain information on the bacterial composition found in both fecal and ileal tissue samples, thus elucidating the role of E. faecium SF68 in the interaction with intestinal residing microorganisms and toward amelioration of the obesity condition.
In this study, C57BL/6 mice were used as an animal model. Animals were supplied by ENVIGO s.r.l (Italy). Mice were five-week-old and weighed 20 g upon reception. For the duration of the study, mice were housed in cages on a 12-h light cycle, with a room temperature of 22–24°C and 50–60% humidity. Animals were allowed free access to food and water ad libitum. They were handled and cared following the European Community Council Directive 2010/63/UE, transposed by the Italian Government. The study was approved by the Italian Ministry of Health (authorization 955/2018-PR). Mice were randomly divided in 4 groups, each constituted of 5 to 10 mice (Figure 1). Two of the four groups were fed with a standard diet (SD, TD.2018), which provided 58% kcal as carbohydrates, 24% kcal as proteins, and 18% kcal as fats (3.1 kcal/g), while the remaining groups were fed with a high-fat diet (HFD, TD.06414), which provided 21.4% kcal as carbohydrates, 18.3% kcal as proteins, and 60.8% kcal as fats (5.1 kcal/g). Both dietary regimes were purchased from ENVIGO s.r.l. Animals belonging to one of the two SD groups were treated with a probiotic formulation based on Enterococcus faecium SF68 (SD + SF68), and the same procedure was performed for animals fed with the HFD (HFD + SF68). E. faecium SF68 (Cerbios-Pharma SA, Switzerland) is currently available in worldwide commercialized probiotic formulations as a drug. To administer the probiotic formulation, 109 CFU of E. faecium SF68 were resuspended in 0.15 ml of methocel 3%. Animals received the product daily via oral gavage for 4 weeks. After this period, animals were weighed, then anaesthetized and sacrificed to collect feces (withdrawn directly from the sigmoid colon) and ileal samples (tissue and mucus layer deprived of luminal content). All samples were stored separately at −80°C until use.
To perform genomic DNA extraction from fecal and ileal samples, QIAmp PowerFecal Pro DNA Kit (QIAGEN, Germany) and QIAmp DNA Mini Kit (QIAGEN) were used, respectively. The extraction procedure was performed following the manufacturer’s protocol. Fecal and ileal samples to extract weighed 15 and 25 mg, respectively. DNA concentration was calculated by measuring the optical density at 260 nm (OD260) and DNA purity was estimated by determining the OD260/ OD280 ratio with Nanodrop Lite Spectrophotometer (Thermo Fisher Scientific, United States).
To assess the absolute abundances of total bacteria, and of the main phyla (i.e., Firmicutes, Bacteroidetes, Actinobacteria, Proteobacteria) and genera (i.e., Bacteroides, Bifidobacterium, Faecalibacterium, Lactobacillus, Prevotella) present in both fecal and ileal samples, 16S rRNA gene-based Real-Time qPCR reactions were performed. Quantification of Akkermansia muciniphila abundance in ileal samples was also carried out. Primer pairs used in this study, able to specifically anneal to phylum- or genus- specific regions of the gene encoding 16S rRNA, are listed in Tables 1, 2. A degenerate primer pair, used to determine the total bacteria abundance, is also reported (Table 1). PCR reactions were performed using the CFX96 Real-Time System (BioRad, United States), and absolute quantifications were extrapolated using the CFX Manager Software (Biorad). Bacterial quantifications were established using calibration curves, obtained with serial 10-fold dilutions of external standards with known concentration ranging from 102 to 108 DNA copies/μl. Reactions were carried out in duplicate in a 96-wells plate with a final reaction volume of 20 μl, containing 1 μl of 2.5 ng/μl DNA template, 10 μl of Luna Universal qPCR Master Mix (New England BioLabs, United States), 0.5 μl of each primer (0.25 μM), and 8 μl of sterile water. The amplification conditions were as follows: an initial denaturation step at 95°C for 1 min, followed by 45 cycles of denaturation at 95°C for 15 s, annealing at primers’ optimal temperature for 30 s (Tables 1, 2), and extension at 72°C for 10 s (Biagini et al., 2020). To check the amplification specificity, a melting curve analysis was carried out after PCR reactions by increasing the annealing temperature from 65 to 95°C.
Table 1. Primer pairs used in this study for the quantification of total bacterial load and microbial phyla.
Table 2. Primer pairs used in this study for the quantification of microbial genera and Akkermansia muciniphila.
All data are expressed as mean ± standard deviation. Statistical analyses were carried out with GraphPad Prism 8 (GraphPad Software Inc., United States). For all comparisons (SD vs. HFD; SD vs. SD + SF68; HFD vs. HFD + SF68) statistical significance was set at a p-value of 0.05 and assessed using Student t-tests for unpaired data.
Mice fed with HFD displayed a significant increase in body weight after 3 weeks (29.10 ± 2.56 g, +10.2%, p = 0.0038) and 4 weeks (31.60 ± 2.91 g, +20.2%, p < 0.0001) of dietary regimen compared to those fed with SD (3 W, 26.40 ± 0.84 g; 4 W: 26.30 ± 0.95 g), thus confirming the efficacy of the dietary regimen in setting up an obesity model (Figure 2). Interestingly, while no statistically significant weight differences were evidenced between SD and SD + SF68 during the entire experimental protocol (p > 0.05), mice receiving the probiotic formulation with the concomitant HFD gained less weight than the HFD control group at 2, 3, and 4 weeks (2 W, −12.4%, p = 0.0006; 3 W: −7.0%, p = 0.0376; 4 W: −16.2%, p = 0.0017).
Figure 2. Weight variations in groups SD, SD + SF68, HFD, and HFD + SF68 registered weekly up to 4 weeks. **p < 0.01; ****p < 0.0001.
In fecal samples of HFD-fed mice, significant increases were observed in the total amount of bacteria (p = 0.0095), Firmicutes (p = 0.0162), and Actinobacteria (p = 0.0024), while Bacteroidetes abundance resulted lower (p = 0.0395; Figure 3A). At genus level, these animals displayed an increase in Bifidobacterium (p = 0.0007) and Faecalibacterium (p = 0.0003), while a decrease was registered in Bacteroides (p = 0.0037; Figure 3A). The analysis of ileal samples provided different results. Firmicutes (p = 0.0397) and Proteobacteria (p = 0.0067) amounts were significantly lower in mice fed with HFD compared to SD and reductions in the absolute abundance of Lactobacillus (p = 0.0363), Bacteroides (p = 0.0133), and Faecalibacterium (p = 0.0021) were also evident in this group of animals (Figure 3B).
Figure 3. (A) Absolute abundances of total bacterial load, phyla, and genera in fecal samples of mice belonging to SD and HFD groups. (B) Absolute abundances of total bacterial load, phyla, and genera in ileal samples of mice belonging to SD and HFD groups. *p < 0.05; **p < 0.01; ***p < 0.001.
The administration of E. faecium SF68 to SD- and HFD-fed mice caused a significant reduction of the fecal bacterial load (SD + SF68 vs. SD, p = 0.0275; HFD + SF68 vs. HFD, p = 0.0021), Firmicutes (SD + SF68 vs. SD, p = 0.0318; HFD + SF68 vs. HFD, p = 0.0063), Bacteroidetes (SD + SF68 vs. SD, p = 0.0199; HFD + SF68 vs. HFD, p < 0.0001) and Proteobacteria (SD + SF68 vs. SD, p = 0.0088; HFD + SF68 vs. HFD, p = 0.0203) compared to untreated animals (Figure 4A). An increase in the abundance of Actinobacteria was observed in normal weight mice that received the formulation (p = 0.0001). Both groups receiving the probiotic displayed a significant increase in the amount of bacteria belonging to the Bifidobacterium genus (SD + SF68 vs. SD, p = 0.0042; HFD + SF68 vs. HFD, p = 0.0364) and a reduction in Prevotella (SD + SF68 vs. SD, p = 0.0008; HFD + SF68 vs. HFD, p < 0.0001) in comparison with SD and HFD controls. A significant reduction was also evidenced in the amount of Bacteroides in SD + SF68 animals compared to SD controls (p = 0.0002).
Figure 4. (A) Absolute abundances of total bacterial load, phyla, and genera in fecal samples of mice fed with SD or HFD with or without SF68 administration. (B) Absolute abundances of total bacterial load, phyla, and genera in ileal samples in both mice fed with SD or HFD with or without SF68 administration. Statistical comparisons were carried out between SD and SD + SF68, and HFD and HFD + SF68. *p < 0.05; **p < 0.01; ***p < 0.001; ****p < 0.0001.
Absolute quantifications of bacteria in ileal samples revealed increased abundance of the phylum Bacteroidetes (p = 0.0092) and the genus Faecalibacterium (p = 0.0352) in HFD + SF68 group in comparison with HFD controls (Figure 4B). In addition, an increase in Lactobacillus was observed after probiotic treatment in both SD- and HFD-fed mice (SD + SF68 vs. SD, p = 0.0255; HFD + SF68 vs. HFD, p = 0.0004).
The amount of Akkermansia was significantly lower in obese (HFD) than normal weight (SD) mice (p = 0.0026; Figure 5). Notably, a remarkable increase of this microorganism was revealed in HFD + SF68 mice (4.63 × 102 ± 3.68 × 102 of DNA copies/mg of ileum) compared to HFD-fed animals (7.45 × 10−1 ± 4.41 × 10−1 DNA copies/mg of ileum; p = 0.0341; Figure 5).
Figure 5. Variations in the absolute abundance of Akkermansia muciniphila in ileal tissues in both normal weight (SD) and obese (HFD) mice with or without SF68 administration. Statistical comparisons were carried out between SD and SD + SF68, and HFD and HFD + SF68. *p < 0.05; **p < 0.01.
Mice are the most commonly used animals to study obesity after inducing this condition with either monogenic mutations or high-fat diets (Fuchs et al., 2018). High-fat diets have been shown to mimic more faithfully the overall metabolic and physiological alterations observed in obese individuals (Antonioli et al., 2020). For this reason, we selected this procedure to establish a proper experimental model of obesity and to obtain a more representative analysis of the gut microbiota, which was performed on both fecal and ileal samples. In fact, to investigate the entire gut microbiota composition, the analysis of feces alone might not be sufficient (Raoult and Henrissat, 2014; Li et al., 2017), since the microbial community associated with the host’s mucus, in particular that residing in the ileal tract, is not adequately represented in the stool. In addition, mucus-associated microorganisms of the upper intestine are actively involved in several physiological processes, such as immune regulation, inflammatory responses (Raoult, 2017), nutrient absorption, particularly of simple sugars (Raoult and Henrissat, 2014), and energy intake (Aron-Wisnewsky et al., 2012), which altogether play a key role in the development of obesity.
In our study, mice fed with HFD displayed a remarkable weight gain and substantial variations in their gut microbiota composition, which is usually found dysbiotic in obese individuals (Abenavoli et al., 2019). In particular, obese animals showed an increased fecal abundance of Firmicutes and Actinobacteria. Previous studies already showed an increase of members belonging to these phyla in fecal samples of obese subjects (Turnbaugh et al., 2006; Clarke et al., 2012; Grigor'eva, 2020). These samples were also demonstrated to contain a higher abundance of bacterial genes commonly associated with obesity and mainly derived from Actinobacteria and Firmicutes (Turnbaugh et al., 2009). The enrichment in Firmicutes, the most abundant phylum in both murine and human gut, has also been associated with increased nutrient absorption, thus suggesting Firmicutes as main microbial actors in obesity (Jumpertz et al., 2011).
In accordance with studies performed on obese subjects, which report low intestinal levels of Bacteroides being associated with visceral adiposity and obesity (Yin et al., 2022), we observed a reduction in the abundance of Bacteroides in both the feces and ileal mucosa of obese mice. We also detected a relevant decrease in Lactobacillus and Faecalibacterium in ileal samples of obese mice, consistently with previous studies regarding obesity, non-alcoholic fatty liver disease, and inflammatory bowel diseases (Sokol et al., 2009; Million et al., 2013; Lopez-Siles et al., 2018; Iino et al., 2019). Lactobacilli represent an important component of the microbial community residing in the human gut and exert a variety of beneficial properties (Li et al., 2017; Villmones et al., 2018), which make them the most common probiotics available in the worldwide market (Slattery et al., 2019). On the other hand, F. prausnitzii, the unique species belonging to the genus Faecalibacterium up to date, is considered a bioindicator for human health (Ferreira-Halder et al., 2017), exerting anti-inflammatory activities (Martín et al., 2015), producing butyrate (Louis and Flint, 2009), and improving the gut barrier function (Carlsson et al., 2013). We can therefore strongly presume that the reduction of Lactobacillus and Faecalibacterium observed in the HFD group hampered the preservation of a healthy condition in mice. The overall weight gain in HFD mice alongside with the variations we observed in both fecal and ileal microbiota allowed us to assert the robustness of our murine model of obesity.
Since specific Enterococcus strains display beneficial effects and satisfactory safety profiles when administered in a variety of pathological conditions in humans (Holzapfel et al., 2018), we wondered whether the oral administration of a probiotic formulation based on E. faecium SF68 was able to counteract the excessive weight gain associated with the obesogenic diet and prevent the shift to a dysbiotic gut microbiota. Some strains of Enterococcus are known for their ability to lower serum cholesterol (i.e., E. faecium CRL 183, M74, and WEFA23) (Foulquié Moreno et al., 2006; Franz et al., 2011; Zhang et al., 2017), exert anti-inflammatory activities (i.e., E. faecium M-74 1, E. durans M4, and M5) (Avram-Hananel et al., 2010; Franz et al., 2011), increase insulin and leptin sensitivity and reduce adipocyte hypertrophy and fat accumulation (i.e., E. faecalis AG5; Mishra and Ghosh, 2020), and directly modulate the intestinal microbial composition (i.e., E. faecium L5, and R0026; Tarasova et al., 2010; Huang et al., 2021), thus showing endearing features in the management of obesity (De Carvalho Marchesin et al., 2018; Jiang et al., 2021). E. faecium SF68 is a well-known Enterococcus probiotic strains contained in commercialized formulations and has been proven to be safe and effective in the treatment of acute diarrhea (Allen et al., 2010; Greuter et al., 2020).
E. faecium SF68 administration to HFD-fed mice resulted in a remarkably lower weight gain. The concurrent variations observed in the intestinal microbiota were altogether encouraging. A reduction of fecal Firmicutes abundance was detected in both SD + SF68 and HFD + SF68 mice, thus suggesting an active role of the Enterococcus strain in the modulation of Firmicutes and in the preservation of an eubiotic state. Interestingly, in fecal samples of both SD- and HFD-fed mice a reduction in Proteobacteria after SF68 treatment was also detected. Proteobacteria are usually present in small percentages within a healthy gut microbiota. In fact, an association between high abundance of Proteobacteria and gut dysbiosis, which often occurs in case of metabolic disorders, intestinal inflammation, and obesity, has been proven (Shin et al., 2015; Litvak et al., 2017).
The abundance of Bifidobacterium notably increased in the fecal microbiota of SF68-treated SD- and HFD-fed mice. The correlation between the amount of bifidobacteria and obesity is well-established. A few studies reported higher Bifidobacterium abundances to be associated with normal weight conditions (Kalliomäki et al., 2008) and demonstrated that Bifidobacterium spp. negatively correlate with endotoxemia and positively correlate with improved glucose tolerance, reduced plasma levels of pro-inflammatory cytokines (Cani et al., 2007b), and higher mucus thickness (Moreira et al., 2012). Additionally, bifidobacteria have been proven to ameliorate obesity-related conditions, for example by reducing cholesterol and glucose levels (Yin et al., 2010; An et al., 2011).
A greater abundance of Prevotella in obese individuals (Stanislawski et al., 2019), as well as in patients with hypertension (Dan et al., 2019), has recently been reported. Our finding that Prevotella decreased in the fecal samples of both groups of mice after SF68 treatment supports the hypothesis that the reduction of this genus potentially corresponds to an improvement in mice’s overall wellbeing. The administration of SF68 also resulted in higher amounts of Lactobacillus and Faecalibacterium in the ileal mucosa of HFD-fed mice. Besides their aforementioned properties, microbes belonging to Bifidobacterium, Lactobacillus, and Faecalibacterium are additionally able to produce SCFAs. These compounds exert a variety of beneficial effects both at local and systemic level and can therefore ameliorate the clinical characteristics of obese patients (Canfora et al., 2015).
A. muciniphila is well-known for its inverse correlation with obesity, type 2 diabetes, and other metabolic conditions, such as hypercholesterolemia and fatty liver disease (Xu et al., 2020). Conversely, high levels of Akkermansia are associated with a better metabolic status, in particular with higher leptin sensitivity, lower fasting blood glucose and insulin resistance, and lower adipocyte size (Dao et al., 2016). Some of the molecular mechanisms of these associations are known. A. muciniphila is able to produce SCFAs as a result of mucin degradation (Ottman et al., 2017) and can counteract the onset of obesity by reducing appetite, energy intake, systemic inflammation, and blood pressure, and improve the gut barrier function (Le Blanc et al., 2017; Chambers et al., 2018). Similarly to results obtained in previous studies in which A. muciniphila was found to be decreased in obese mice (Everard et al., 2013), we assessed that the HFD-fed group displayed a lower abundance of this microorganism than animals receiving the standard diet. The administration of E. faecium SF68 to HFD-fed mice significantly increased Akkermansia levels, thus suggesting that the administration of such a probiotic could have a positive impact on the metabolism of mice fed with HFD.
In conclusion, relevant results were obtained when E. faecium SF68 was administered to mice fed with a high-fat diet, demonstrating its ability to positively influence the gut microbial community both at luminal and mucosal level and to counteract the weight gain that occurs with an obesogenic diet. Several variations in the microbiota composition were highlighted, such as the reduction in Firmicutes and Proteobacteria and the increase in Bifidobacterium in fecal samples, as well as an increase in Faecalibacterium, Lactobacillus, and Akkermansia in ileal samples. These findings evidence the potential of E. faecium SF68 as a promising approach for the co-treatment and prevention of obesity, also opening new perspectives in the study of the molecular mechanisms underlying its beneficial effect.
The datasets presented in this study can be found in online repositories. The names of the repository/repositories and accession number(s) can be found at: http://doi.org/10.17632/2syf8c7fsh.1.
The animal study was reviewed and approved by the Italian Ministry of Health (authorization 955/2018-PR).
MF, LA, and EG: conception and design of the study, validation, formal analysis, and supervision. AP, MC, LB, VD’A, CP, CS, DM, and FC: methodology and investigation. AP and MC: writing – original draft preparation. AP, MC, LB, VD’A, CP, CS, DM, FC, MF, LA, and EG: writing – review and editing. All authors have read and agreed to the published version of the manuscript.
This study was funded by grants from the University of Pisa (EG) and by Cerbios-Pharma SA (MF and LA).
The authors declare that the research was conducted in the absence of any commercial or financial relationships that could be construed as a potential conflict of interest.
All claims expressed in this article are solely those of the authors and do not necessarily represent those of their affiliated organizations, or those of the publisher, the editors and the reviewers. Any product that may be evaluated in this article, or claim that may be made by its manufacturer, is not guaranteed or endorsed by the publisher.
Abenavoli, L., Scarpellini, E., Colica, C., Boccuto, L., Salehi, B., Sharifi-Rad, J., et al. (2019). Gut microbiota and obesity: a role for probiotics. Nutrients 11:2690. doi: 10.3390/nu11112690
Allen, S. J., Martinez, E. G., Gregorio, G. V., and Dans, L. F. (2010). Probiotics for treating acute infectious diarrhoea. Cochrane Database Syst. Rev. 2010:CD003048. doi: 10.1002/14651858.CD003048.pub3
An, H. M., Park, S. Y., Lee, D. K., Kim, J. R., Cha, M. K., Lee, S. W., et al. (2011). Antiobesity and lipid-lowering effects of Bifidobacterium spp. in high fat diet-induced obese rats. Lipids Health Dis. 10:116. doi: 10.1186/1476-511X-10-116
Antonioli, L., D'Antongiovanni, V., Pellegrini, C., Fornai, M., Benvenuti, L., di Carlo, A., et al. (2020). Colonic dysmotility associated with high-fat diet-induced obesity: role of enteric glia. FASEB J. 34, 5512–5524. doi: 10.1096/fj.201901844R
Aron-Wisnewsky, J., Doré, J., and Clement, K. (2012). The importance of the gut microbiota after bariatric surgery. Nat. Rev. Gastroenterol. Hepatol. 9, 590–598. doi: 10.1038/nrgastro.2012.161
Avram-Hananel, L., Stock, J., Parlesak, A., Bode, C., and Schwartz, B. (2010). E. durans strain M4-5 isolated from human colonic flora attenuates intestinal inflammation. Dis. Colon Rectum 53, 1676–1686. doi: 10.1007/DCR.0b013e3181f4b148
Azad, M. A. K., Sarker, M., Li, T., and Yin, J. (2018). Probiotic species in the modulation of gut microbiota: an overview. Biomed. Res. Int. 2018:9478630. doi: 10.1155/2018/9478630
Bagarolli, R. A., Tobar, N., Oliveira, A. G., Araújo, T. G., Carvalho, B. M., Rocha, G. Z., et al. (2017). Probiotics modulate gut microbiota and improve insulin sensitivity in DIO mice. J. Nutr. Biochem. 50, 16–25. doi: 10.1016/j.jnutbio.2017.08.006
Biagini, F., Calvigioni, M., Lapomarda, A., Vecchione, A., Magliaro, C., De Maria, C., et al. (2020). A novel 3D in vitro model of the human gut microbiota. Sci. Rep. 10:21499. doi: 10.1038/s41598-020-78591-w
Bibbò, S., Ianiro, G., Giorgio, V., Scaldaferri, F., Masucci, L., Gasbarrini, A., et al. (2016). The role of diet on gut microbiota composition. Eur. Rev. Med. Pharmacol. Sci. 20, 4742–4749.
Canfora, E. E., Jocken, J. W., and Blaak, E. E. (2015). Short-chain fatty acids in control of body weight and insulin sensitivity. Nat. Rev. Endocrinol. 11, 577–591. doi: 10.1038/nrendo.2015.128
Cani, P. D., Amar, J., Iglesias, M. A., Poggi, M., Knauf, C., Bastelica, D., et al. (2007a). Metabolic endotoxemia initiates obesity and insulin resistance. Diabetes 56, 1761–1772. doi: 10.2337/db06-1491
Cani, P. D., Neyrinck, A. M., Fava, F., Knauf, C., Burcelin, R. G., Tuohy, K. M., et al. (2007b). Selective increases of bifidobacteria in gut microflora improve high-fat-diet-induced diabetes in mice through a mechanism associated with endotoxaemia. Diabetologia 50, 2374–2383. doi: 10.1007/s00125-007-0791-0
Carlsson, A. H., Yakymenko, O., Olivier, I., Håkansson, F., Postma, E., Keita, A. V., et al. (2013). Faecalibacterium prausnitzii supernatant improves intestinal barrier function in mice DSS colitis. Scand. J. Gastroenterol. 48, 1136–1144. doi: 10.3109/00365521.2013.828773
Cerdó, T., García-Santos, J. A., Bermúdez, M. G., and Campoy, C. (2019). The role of probiotics and prebiotics in the prevention and treatment of obesity. Nutrients 11:635. doi: 10.3390/nu11030635
Chambers, E. S., Preston, T., Frost, G., and Morrison, D. J. (2018). Role of gut microbiota-generated short-chain fatty acids in metabolic and cardiovascular health. Curr. Nutr. Rep. 7, 198–206. doi: 10.1007/s13668-018-0248-8
Clarke, S. F., Murphy, E. F., Nilaweera, K., Ross, P. R., Shanahan, F., O’Toole, P. W., et al. (2012). The gut microbiota and its relationship to diet and obesity: new insights. Gut Microbes 3, 186–202. doi: 10.4161/gmic.20168
Collado, M. C., Derrien, M., Isolauri, E., de Vos, W. M., and Salminen, S. (2007). Intestinal integrity and Akkermansia muciniphila, a mucin-degrading member of the intestinal microbiota present in infants, adults, and the elderly. Appl. Environ. Microbiol. 73, 7767–7770. doi: 10.1128/AEM.01477-07
Da Silva, T. F., Casarotti, S. N., de Oliveira, G. L. V., and Penna, A. L. B. (2021). The impact of probiotics, prebiotics, and synbiotics on the biochemical, clinical, and immunological markers, as well as on the gut microbiota of obese hosts. Crit. Rev. Food Sci. Nutr. 61, 337–355. doi: 10.1080/10408398.2020.1733483
Dan, X., Mushi, Z., Baili, W., Han, L., Enqi, W., Huanhu, Z., et al. (2019). Differential analysis of hypertension-associated intestinal microbiota. Int. J. Med. Sci. 16, 872–881. doi: 10.7150/ijms.29322
Dao, M. C., Everard, A., Aron-Wisnewsky, J., Sokolovska, N., Prifti, E., Verger, E. O., et al. (2016). Akkermansia muciniphila and improved metabolic health during a dietary intervention in obesity: relationship with gut microbiome richness and ecology. Gut 65, 426–436. doi: 10.1136/gutjnl-2014-308778
De Carvalho Marchesin, J., Celiberto, L. S., Orlando, A. B., De Medeiros, A. I., Pinto, R. A., Zuanon, J. A. S., et al. (2018). A soy-based probiotic drink modulates the microbiota and reduces body weight gain in diet-induced obese mice. J. Funct. Foods 48, 302–313. doi: 10.1016/j.jff.2018.07.010
Ejtahed, H. S., Angoorani, P., Soroush, A. R., Atlasi, R., Hasani-Ranjbar, S., Mortazavian, A. M., et al. (2019). Probiotics supplementation for the obesity management: a systematic review of animal studies and clinical trials. J. Funct. Foods 52, 228–242. doi: 10.1016/j.jff.2018.10.039
Ejtahed, H. S., Mohtadi-Nia, J., Homayouni-Rad, A., Niafar, M., Asghari-Jafarabadi, M., Mofid, V., et al. (2011). Effect of probiotic yogurt containing Lactobacillus acidophilus and Bifidobacterium lactis on lipid profile in individuals with type 2 diabetes mellitus. J. Dairy Sci. 94, 3288–3294. doi: 10.3168/jds.2010-4128
Everard, A., Belzer, C., Geurts, L., Ouwerkerk, J. P., Druart, C., Bindels, L. B., et al. (2013). Crosstalk between Akkermansia muciniphila and intestinal epithelium controls diet-induced obesity. Proc. Natl. Acad. Sci. U. S. A. 110, 9066–9071. doi: 10.1073/pnas.1219451110
Ferreira-Halder, C. V., Faria, A. V. S., and Andrade, S. S. (2017). Action and function of Faecalibacterium prausnitzii in health and disease. Best Pract. Res. Clin. Gastroenterol. 31, 643–648. doi: 10.1016/j.bpg.2017.09.011
Food and Agricultural Organization of the United Nations and World Health Organization. (2001). Health and Nutritional Properties of Probiotics in Food Including Powder Milk With Live Lactic Acid Bacteria. Rome: FAO.
Foulquié Moreno, M. R., Sarantinopoulos, P., Tsakalidou, E., and De Vuyst, L. (2006). The role and application of enterococci in food and health. Int. J. Food Microbiol. 106, 1–24. doi: 10.1016/j.ijfoodmicro.2005.06.026
Franz, C. M., Huch, M., Abriouel, H., Holzapfel, W., and Gálvez, A. (2011). Enterococci as probiotics and their implications in food safety. Int. J. Food Microbiol. 151, 125–140. doi: 10.1016/j.ijfoodmicro.2011.08.014
Frasca, D., Blomberg, B. B., and Paganelli, R. (2017). Aging, obesity, and inflammatory age-related diseases. Front. Immunol. 8:1745. doi: 10.3389/fimmu.2017.01745
Fuchs, T., Loureiro, M. P., Macedo, L. E., Nocca, D., Nedelcu, M., and Costa-Casagrande, T. A. (2018). Animal models in metabolic syndrome. Rev. Col. Bras. Cir. 45:e1975. doi: 10.1590/0100-6991e-20181975
Ganji-Arjenaki, M., and Rafieian-Kopaei, M. (2018). Probiotics are a good choice in remission of inflammatory bowel diseases: a meta-analysis and systematic review. J. Cell. Physiol. 233, 2091–2103. doi: 10.1002/jcp.25911
Gérard, P. (2016). Gut microbiota and obesity. Cell. Mol. Life Sci. 73, 147–162. doi: 10.1007/s00018-015-2061-5
Green, M., Arora, K., and Prakash, S. (2020). Microbial medicine: prebiotic and probiotic functional foods to target obesity and metabolic syndrome. Int. J. Mol. Sci. 21:2890. doi: 10.3390/ijms21082890
Greuter, T., Michel, M. C., Thomann, D., Weigmann, H., and Vavricka, S. R. (2020). Randomized, placebo-controlled, double-blind, and open-label studies in the treatment and prevention of acute diarrhea with Enterococcus faecium SF68. Front. Med. 7:276. doi: 10.3389/fmed.2020.00276
Grigor'eva, I. N. (2020). Gallstone disease, obesity and the Firmicutes/Bacteroidetes ratio as a possible biomarker of gut dysbiosis. J. Pers. Med. 11:13. doi: 10.3390/jpm11010013
Holzapfel, W., Arini, A., Aeschbacher, M., Coppolecchia, R., and Pot, B. (2018). Enterococcus faecium SF68 as a model for efficacy and safety evaluation of pharmaceutical probiotics. Benef. Microbes 9, 375–388. doi: 10.3920/BM2017.0148
Huang, J., Huang, J., Yin, T., Lv, H., Zhang, P., and Li, H. (2021). Enterococcus faecium R0026 combined with Bacillus subtilis R0179 prevent obesity-associated hyperlipidemia and modulate gut microbiota in C57BL/6 mice. J. Microbiol. Biotechnol. 31, 181–188. doi: 10.4014/jmb.2009.09005
Iino, C., Endo, T., Mikami, K., Hasegawa, T., Kimura, M., Sawada, N., et al. (2019). Significant decrease in Faecalibacterium among gut microbiota in non-alcoholic fatty liver disease: a large BMI- and sex-matched population study. Hepatol. Int. 13, 748–756. doi: 10.1007/s12072-019-09987-8
Jiang, J., Xiong, J., Ni, J., Chen, C., and Wang, K. (2021). Live combined B. subtilis and E. faecium alleviate liver inflammation, improve intestinal barrier function, and modulate gut microbiota in mice with non-alcoholic fatty liver disease. Med. Sci. Monit. 27:e931143. doi: 10.12659/MSM.931143
Jumpertz, R., Le, D. S., Turnbaugh, P. J., Trinidad, C., Bogardus, C., Gordon, J. I., et al. (2011). Energy-balance studies reveal associations between gut microbes, caloric load, and nutrient absorption in humans. Am. J. Clin. Nutr. 94, 58–65. doi: 10.3945/ajcn.110.010132
Kalliomäki, M., Collado, M. C., Salminen, S., and Isolauri, E. (2008). Early differences in fecal microbiota composition in children may predict overweight. Am. J. Clin. Nutr. 87, 534–538. doi: 10.1093/ajcn/87.3.534
Kim, M., Park, T., and Yu, Z. (2017). Metagenomic investigation of gastrointestinal microbiome in cattle. Asian Australas J. Anim. Sci. 30, 1515–1528. doi: 10.5713/ajas.17.0544
Le Blanc, J. G., Chain, F., Martín, R., Bermúdez-Humarán, L. G., Courau, S., and Langella, P. (2017). Beneficial effects on host energy metabolism of short-chain fatty acids and vitamins produced by commensal and probiotic bacteria. Microb. Cell Factories 16:79. doi: 10.1186/s12934-017-0691-z
Li, D., Chen, H., Mao, B., Yang, Q., Zhao, J., Gu, Z., et al. (2017). Microbial biogeography and core microbiota of the rat digestive tract. Sci. Rep. 8:45840. doi: 10.1038/srep45840
Litvak, Y., Byndloss, M. X., Tsolis, R. M., and Bäumler, A. J. (2017). Dysbiotic Proteobacteria expansion: a microbial signature of epithelial dysfunction. Curr. Opin. Microbiol. 39, 1–6. doi: 10.1016/j.mib.2017.07.003
Lopez-Siles, M., Enrich-Capó, N., Aldeguer, X., Sabat-Mir, M., Duncan, S. H., Garcia-Gil, L. J., et al. (2018). Alterations in the abundance and co-occurrence of Akkermansia muciniphila and Faecalibacterium prausnitzii in the colonic mucosa of inflammatory bowel disease subjects. Front. Cell. Infect. Microbiol. 8:281. doi: 10.3389/fcimb.2018.00281
Louis, P., and Flint, H. J. (2009). Diversity, metabolism, and microbial ecology of butyrate-producing bacteria from the human large intestine. FEMS Microbiol. Lett. 294, 1–8. doi: 10.1111/j.1574-6968.2009.01514.x
Martín, R., Miquel, S., Chain, F., Natividad, J. M., Jury, J., Lu, J., et al. (2015). Faecalibacterium prausnitzii prevents physiological damages in a chronic low-grade inflammation murine model. BMC Microbiol. 15:67. doi: 10.1186/s12866-015-0400-1
Matsuki, T., Watanabe, K., Fujimoto, J., Miyamoto, Y., Takada, T., Matsumoto, K., et al. (2002). Development of 16S rRNA-gene-targeted group-specific primers for the detection and identification of predominant bacteria in human feces. Appl. Environ. Microbiol. 68, 5445–5451. doi: 10.1128/AEM.68.11.5445-5451.2002
Mekonnen, S. A., Merenstein, D., Fraser, C. M., and Marco, M. L. (2020). Molecular mechanisms of probiotic prevention of antibiotic-associated diarrhea. Curr. Opin. Biotechnol. 61, 226–234. doi: 10.1016/j.copbio.2020.01.005
Million, M., Angelakis, E., Maraninchi, M., Henry, M., Giorgi, R., Valero, R., et al. (2013). Correlation between body mass index and gut concentrations of lactobacillus reuteri, Bifidobacterium animalis, Methanobrevibacter smithii, and Escherichia coli. Int. J. Obes. 37, 1460–1466. doi: 10.1038/ijo.2013.20
Mishra, A. K., and Ghosh, A. R. (2020). Probiotic Enterococcus faecalis AG5 mitigated high fat diet induced obesity and produced propionic acid stimulated apoptosis in 3T3-L1 pre-adipocyte. Life Sci. 261:118292. doi: 10.1016/j.lfs.2020.118292
Moayyedi, P., Ford, A. C., Talley, N. J., Cremonini, F., Foxx-Orenstein, A. E., Brandt, L. J., et al. (2010). The efficacy of probiotics in the treatment of irritable bowel syndrome: a systematic review. Gut 59, 325–332. doi: 10.1136/gut.2008.167270
Moreira, A. P., Texeira, T. F., Ferreira, A. B., Peluzio Mdo, C., and Alfenas Rde, C. (2012). Influence of a high-fat diet on gut microbiota, intestinal permeability and metabolic endotoxaemia. Br. J. Nutr. 108, 801–809. doi: 10.1017/S0007114512001213
Ottman, N., Geerlings, S. Y., Aalvink, S., de Vos, W. M., and Belzer, C. (2017). Action and function of Akkermansia muciniphila in microbiome ecology, health and disease. Best Pract. Res. Clin. Gastroenterol. 31, 637–642. doi: 10.1016/j.bpg.2017.10.001
Raoult, D. (2017). The study of microbiota needs both microbiologists and medical doctors. Clin. Microbiol. Infect. 23, 500–501. doi: 10.1016/j.cmi.2017.03.002
Raoult, D., and Henrissat, B. (2014). Are stool samples suitable for studying the link between gut microbiota and obesity? Eur. J. Epidemiol. 29, 307–309. doi: 10.1007/s10654-014-9905-4
Sánchez, B., Delgado, S., Blanco-Míguez, A., Lourenço, A., Gueimonde, M., and Margolles, A. (2017). Probiotics, gut microbiota, and their influence on host health and disease. Mol. Nutr. Food Res. 61:1600240. doi: 10.1002/mnfr.201600240
Shin, N. R., Whon, T. W., and Bae, J. W. (2015). Proteobacteria: microbial signature of dysbiosis in gut microbiota. Trends Biotechnol. 33, 496–503. doi: 10.1016/j.tibtech.2015.06.011
Slattery, C., Cotter, P. D., and O'Toole, P. W. (2019). Analysis of health benefits conferred by Lactobacillus species from kefir. Nutrients 11:1252. doi: 10.3390/nu11061252
Sokol, H., Seksik, P., Furet, J. P., Firmesse, O., Nion-Larmurier, I., Beaugerie, L., et al. (2009). Low counts of Faecalibacterium prausnitzii in colitis microbiota. Inflamm. Bowel Dis. 15, 1183–1189. doi: 10.1002/ibd.20903
Stach, J. E., Maldonado, L. A., Ward, A. C., Goodfellow, M., and Bull, A. T. (2003). New primers for the class Actinobacteria: application to marine and terrestrial environments. Environ. Microbiol. 5, 828–841. doi: 10.1046/j.1462-2920.2003.00483.x
Stanislawski, M. A., Dabelea, D., Lange, L. A., Wagner, B. D., and Lozupone, C. A. (2019). Gut microbiota phenotypes of obesity. NPJ Biofilms Microbiomes 5:18. doi: 10.1038/s41522-019-0091-8
Tarasova, E., Yermolenko, E., Donets, V., Sundukova, Z., Bochkareva, A., Borshev, I., et al. (2010). The influence of probiotic Enterococcus faecium strain L5 on the microbiota and cytokines expression in rats with dysbiosis induced by antibiotics. Benef. Microbes 1, 265–270. doi: 10.3920/BM2010.0008
Tenorio-Jiménez, C., Martínez-Ramírez, M. J., Gil, Á., and Gómez-Llorente, C. (2020). Effects of probiotics on metabolic syndrome: a systematic review of randomized clinical trials. Nutrients 12:124. doi: 10.3390/nu12010124
Turnbaugh, P. J., Hamady, M., Yatsunenko, T., Cantarel, B. L., Duncan, A., Ley, R. E., et al. (2009). A core gut microbiome in obese and lean twins. Nature 457, 480–484. doi: 10.1038/nature07540
Turnbaugh, P. J., Ley, R. E., Mahowald, M. A., Magrini, V., Mardis, E. R., and Gordon, J. I. (2006). An obesity-associated gut microbiome with increased capacity for energy harvest. Nature 444, 1027–1031. doi: 10.1038/nature05414
Villmones, H. C., Haug, E. S., Ulvestad, E., Grude, N., Stenstad, T., Halland, A., et al. (2018). Species level description of the human ileal bacterial microbiota. Sci. Rep. 8:4736. doi: 10.1038/s41598-018-23198-5
Xu, Y., Wang, N., Tan, H. Y., Li, S., Zhang, C., and Feng, Y. (2020). Function of Akkermansia muciniphila in obesity: interactions with lipid metabolism, immune response, and gut systems. Front. Microbiol. 11:219. doi: 10.3389/fmicb.2020.00219
Yadav, H., Lee, J. H., Lloyd, J., Walter, P., and Rane, S. G. (2013). Beneficial metabolic effects of a probiotic via butyrate-induced GLP-1 hormone secretion. J. Biol. Chem. 288, 25088–25097. doi: 10.1074/jbc.M113.452516
Yin, X. Q., An, Y. X., Yu, C. G., Ke, J., Zhao, D., and Yu, K. (2022). The association between fecal short-chain fatty acids, gut microbiota, and visceral fat in monozygotic twin pairs. Diabetes Metab. Syndr. Obes. 15, 359–368. doi: 10.2147/DMSO.S338113
Yin, Y. N., Yu, Q. F., Fu, N., Liu, X. W., and Lu, F. G. (2010). Effects of four Bifidobacteria on obesity in high-fat diet induced rats. World J. Gastroenterol. 16, 3394–3401. doi: 10.3748/wjg.v16.i27.3394
Keywords: probiotics, gut microbiota, Enterococcus, obesity, high-fat diet, ileum
Citation: Panattoni A, Calvigioni M, Benvenuti L, D’Antongiovanni V, Pellegrini C, Di Salvo C, Mazzantini D, Celandroni F, Fornai M, Antonioli L and Ghelardi E (2022) The administration of Enterococcus faecium SF68 counteracts compositional shifts in the gut microbiota of diet-induced obese mice. Front. Microbiol. 13:1054097. doi: 10.3389/fmicb.2022.1054097
Received: 26 September 2022; Accepted: 28 November 2022;
Published: 16 December 2022.
Edited by:
Bruce S. Seal, Oregon State University–Cascades, United StatesReviewed by:
Mohammad Altamimi, An-Najah National University, PalestineCopyright © 2022 Panattoni, Calvigioni, Benvenuti, D’Antongiovanni, Pellegrini, Di Salvo, Mazzantini, Celandroni, Fornai, Antonioli and Ghelardi. This is an open-access article distributed under the terms of the Creative Commons Attribution License (CC BY). The use, distribution or reproduction in other forums is permitted, provided the original author(s) and the copyright owner(s) are credited and that the original publication in this journal is cited, in accordance with accepted academic practice. No use, distribution or reproduction is permitted which does not comply with these terms.
*Correspondence: Matteo Fornai, bWF0dGVvLmZvcm5haUB1bmlwaS5pdA==
†These authors have contributed equally to this work and share first authorship
Disclaimer: All claims expressed in this article are solely those of the authors and do not necessarily represent those of their affiliated organizations, or those of the publisher, the editors and the reviewers. Any product that may be evaluated in this article or claim that may be made by its manufacturer is not guaranteed or endorsed by the publisher.
Research integrity at Frontiers
Learn more about the work of our research integrity team to safeguard the quality of each article we publish.