- 1Affiliated Zhoupu Hospital, Shanghai University of Medicine and Health Sciences, Shanghai, China
- 2Microbial Pharmacology Laboratory, Shanghai University of Medicine and Health Sciences, Shanghai, China
- 3School of Pharmacy, Shanghai University of Medicine and Health Sciences, Shanghai, China
- 4Shanghai University of Medicine and Health Science, Shanghai University of Traditional Chinese Medicine, Shanghai, China
- 5School of Medical Instrument and Food Engineering, University of Shanghai for Science and Technology, Shanghai, China
- 6School of Chemistry and Life Sciences, Suzhou University of Science and Technology, Suzhou, China
- 7School of Medical Technology, Shanghai University of Medicine and Health Sciences, Shanghai, China
- 8Department of Critical Care Medicine, The Third Xiangya Hospital, Central South University, Changsha, Hunan, China
α-Glucosidase and α-amylase are the two main glycosidases that participate in the metabolism of carbohydrates. Inhibitors of these two enzymes are considered an important medical treatment for carbohydrate uptake disorders, such as diabetes and obesity. Microbes are an important source of constituents that have the potential to inhibit glycosidases and can be used as sources of new drugs and dietary supplements. For example, the α-glucosidase inhibitor acarbose, isolated from Actinoplanes sp., has played an important role in adequately controlling type 2 diabetes, but this class of marketed drugs has many drawbacks, such as poor compliance with treatment and expense. This demonstrates the need for new microorganism-derived resources, as well as novel classes of drugs with better compliance, socioeconomic benefits, and safety. This review introduces the literature on microbial sources of α-glucosidase and α-amylase inhibitors, with a focus on endophytes and marine microorganisms, over the most recent 5 years. This paper also reviews the application of glycosidase inhibitors as drugs and dietary supplements. These studies will contribute to the future development of new microorganism-derived glycosidase inhibitors.
Introduction
Enzyme inhibitors have attracted increasing attention for medical applications. The common targets of such inhibitors are glycosidases, β-lactamases, lipases, proteases, and xanthine oxidase (Usman et al., 2019). Glycosidases (EC 3.2.1) contain α-glucosidase (EC 3.2.1.20) and α-amylase (EC 3.2.1.1), which are mainly involved in carbohydrate metabolism (Aslan et al., 2018; Demir et al., 2018). α-Glucosidases hydrolyze terminal non-reducing residues of various carbohydrate substrates, resulting in the release of α-glucopyranose (Baron, 1998; Heacock et al., 2005). Meanwhile, α-amylases hydrolyze α-D-(1,4)-glucan linkages in starch to form polymers composed of glucose units (Paul et al., 2021). Since glycosidases are widely used to hydrolyze carbohydrates, inhibitors of these two enzymes are beneficial to treatment carbohydrate-dependent diseases like diabetes, obesity and other diseases (Asano, 2003; Usman et al., 2019). Some glycosidase inhibitors are also considered potential useful tools for the treatment of viral infections and lysosomal-storage-diseases (Block et al., 1998; Chang et al., 2013).
Glycosidase inhibitors hitherto have mostly been derived from microbial sources, which can be easily handled on a large scale, as compared to that with plants (Shah et al., 2018). Since the classic α-glucosidase inhibitor nojirimycin, derived from microbial resources, was first reported by Niwa et al. (1970), more and more glycosidase inhibitors have been isolated from microorganisms (Niwa et al., 1970). However, the search for novel microorganisms with new glycosidase inhibitors was previously concentrated on general environment, which has led to microbial resource scarcity (Bisaria et al., 2020). Therefore, the discovery of new inhibitors from uncommon environments, such as the marine ecosystem and endophytic microorganisms, has been the focus in recent years (Davies-Bolorunduro et al., 2021; Karthikeyan et al., 2022).
The marine environment has not been fully explored, and metabolites of marine microbial origins have a more important role in drug discovery (Pathak et al., 2022). These natural products from marine microorganisms have various bioactivities, such as anti-bacterial (Yang et al., 2018), anti-fungal (Sangkanu et al., 2021), anti-viral (Richard et al., 2018), anti-parasitic (Santos et al., 2019), anti-tumor (Yan et al., 2020), and anti-diabetic properties (Elaiyaraja et al., 2018). The survival of bacteria that live in unusual marine ecosystem depends on these active metabolites (Davies-Bolorunduro et al., 2021).
Endophytic microorganisms, which have symbiotic relationships with plants without causing harm, are a prospective source of novel drugs with various bioactivities (Manganyi et al., 2019). Through their interactions with plants, endophytes generate several metabolites with pharmaceutical applications, such as anti-bacterial (Deshmukh et al., 2022), anti-fungal (Deshmukh et al., 2018), anti-viral (Lacerda et al., 2022), anti-cancer (Gangadevi and Muthumary, 2009; Gill and Vasundhara, 2019), anti-oxidant (Toghueo and Boyom, 2019), and anti-diabetic compounds (Agrawal et al., 2022). The utilization of these endophytes for discovering new active metabolites has been increasing, and it ultimately will be of major importance for pharmaceutical, medical, and industrial applications (Lopéz et al., 2019; Qiu et al., 2019).
The aim of the present review was to consolidate the literature on microbial sources of α-glucosidase and α-amylase inhibitors from 2018 to 2022. Peer-reviewed articles were retrieved from searches using the following databases: PubMed, Google Scholar, and Web of Science.
Inhibitors of glycosidases from different microbes
Inhibitors of glycosidases from bacteria
Bacteria are the main traditional sources of glycosidase inhibitors and bacteria with inhibitory activity against glycosidase in recent 5 years are listed in Table 1.
Actinomycetes
In general, secondary metabolites obtained from bacteria exhibit various activities (Solecka et al., 2012). Actinomycetes, especially Streptomyces species, such as Streptomyces hygroscopicus (validamycin; Kameda et al., 1984), Streptomyces lavendulae (1-deoxynojirimycin, DNJ; Murao and Miyata, 1980; Ezure et al., 1985), and Streptomyces dimorphogenes (trestatin; Yokose et al., 1983), are well known to produce novel glycosidase inhibitors of therapeutic value.
Streptomyces
In the most recent 5 years, most glycosidase inhibitors were still produced by Streptomyces species, and these are very potent. α-Glucosidase inhibitors from Streptomyces costaricanus EBL.HB6 were isolated and purified by Nguyen et al. (2021) in Vietnam. The IC50 value of purified inhibitors was 9.59 mg/ml. They also optimized cultivation conditions to increase the yield of inhibitors. However, very few Streptomyces strains with glycosidase inhibitory activity have originated from the general environment in recent years, and most of them are derived from the marine ecosystem and endophytic microorganisms.
The deep-ocean interior contains many new Streptomyces strains, which show strong inhibitory activity against glycosidases. Kumar and Rao (2018) confirmed that the extract of the marine organism Streptomyces coelicoflavus SRBVIT13 exhibits remarkable inhibitory activity against α-glucosidase of yeast and mammals in vitro. In vivo, postprandial blood glucose levels decreased after the oral administration of this extract in diabetic rats. The main compound with activity was identified as 2-t-butyl-5-chloromethyl-3-methyl-4-oxoimidazolidine-1-carboxylic acid, t-butyl ester (1). Marine-derived Streptomyces sp.S2A was isolated from the gulf of India by Siddharth and Vittal. The active metabolites were extracted, and the α-glucosidase and α-amylase inhibitory activities were tested, with IC50 values of 21.17 μg/ml and 20.46 μg/ml, respectively. The bioactive compound was determined to be pyrrolo[1–a]pyrazine-1,4-dione,hexahydro-3-(2-methylpropyl) (2) (Siddharth and Vittal, 2018). Siddharth and Vittal (2019) further extracted a bioactive fraction from marine Streptomyces sp.SCA29 in India. The fraction was assayed for inhibitory activity against α-glucosidase and α-amylase, and the IC50 values were 44.26 μg/ml and 53.19 μg/ml, respectively. 4-Methoxyacetanilide (3), an acetamide derivative, was also purified based on a bioassay-guided method (Figure 1).
Endophytes from Murraya koenigii comprise a library of novel Streptomyces strains. M. koenigii is a small, deciduous shrub that possesses notable pharmacological effects, such as anti-diabetic, anti-microbial, and anti-diarrheal (Tembhurne and Sakarkar, 2010). Saini confirmed that the endophytic Streptomyces koyangensis strain B025 isolated from M. koenigii has remarkable inhibitory activity against α-amylase. The active compounds were considered to be phenols (Saini and Gangwar, 2018).
For several years, marine endophytic Streptomyces strains isolated from different marine sources comprise one of the most popular research subjects (Chen et al., 2016). The isobutylhexapeptide TXS-2(4) was isolated from the marine Streptomycetes SCSIO 40064 by Chen et al. (2022). They found that this compound significantly inhibited the activity of α-glucosidase, and the IC50 value was 18.67 ± 1.27 mM. El-Gendy et al. (2022) demonstrated the effects of a new endophytic Streptomyces species from the marine Sarcophyton convolutum. The strain displayed α-glucosidase inhibitory activity with an IC50 value ≥ 84.34 ± 2.25% and α-amylase inhibitory activity ≥ 88.20 ± 1.33%.
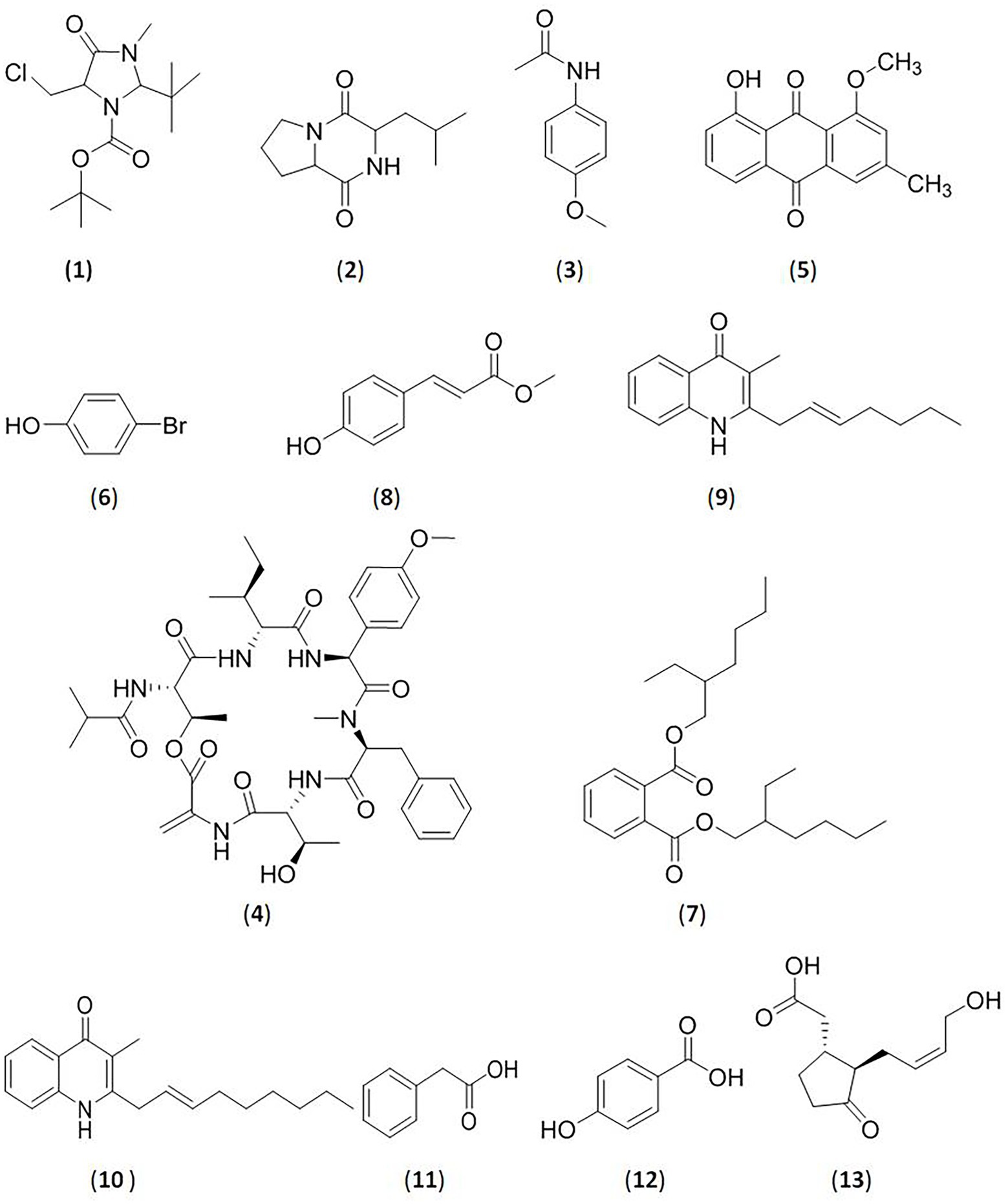
Figure 1. Glycosidase inhibitors compounds from bacteria. (1).2-t-butyl-5-chloromethyl-3-methyl-4-oxoimidazolidine-1-carboxylic acid, t-butyl ester; (2).pyrrolo[1–a]pyrazine-1,4-dione,hexahydro-3-(2-methylpropyl); (3).4-methoxyacetanilide; (4).isobutyryl hexapeptide TXS-2; (5).1-O-methyl chrysophanol (OMC); (6).bromophenol derivative; (7).bis (2-ethylhexyl) phthalate; (8).methoxy ethyl cinnamate (ethyl (E)-3-(4-methoxyphenyl)acrylate; (9)2-(2-heptenyl)-3-methyl-4-quinolinone; (10).3-methyl-2-(2-nonenyl)-4-quinolinone; (11).2-phenylacetic acid; (12).4-hydroxybenzoic acid; (13). (−)-jasmonic acid.
Non-Streptomyces-actinomycetes
In addition to Streptomyces species, glycosidases inhibitors can also be derived from non-Streptomyces-actinomycetes, such as Actinoplanes utahensis (acarbose; Schwientek et al., 2012) and others. In recent years, researchers have isolated inhibitors from Amycolatopsis, Nocardiopsis, Arthrobacter, Saccharomonospora, and other strains in succession. Most of them have come from marine or plant endophytic microorganisms, which are similar to Streptomyces species.
An active compound was isolated from Amycolatopsis thermoflava SFMA-103 by Chandrasekhar et al. (2021). The compound was identified as 1-O-methyl chrysophanol (OMC, 5), a class of hydroxyanthraquinones. OMC inhibited carbohydrate metabolizing enzymes in vitro, with IC50 values of 38.49 μg/ml (α-glucosidase) and 3.4 mg/ml (α-amylase). When orally administered Wistar rats, OMC was demonstrated to inhibit the increase of blood sugar levels in vivo.
Nocardiopsis SCA21 was isolated from marine sediment in India by Siddharth and Rai (2019). Two bioactive compounds were first purified and identified as 4-bromophenol, a bromophenol derivative (6), and Bis (2-ethylhexyl) phthalate, a phthalate ester (7). These two compounds exhibited strong enzyme inhibitory activities against α-glucosidase, with IC50 values of 94.61 μg/ml (compound 6) and 202.33 μg/ml (compound 7). Compound 6 could also could inhibit the activity of α-amylase with an IC50 value of 103.23 μg/ml, whereas compound 7 was less active against α-amylase with an IC50 value >250 μg/ml. Mawlankar et al. (2020) reported a bioactive substance from the novel marine species Arthrobacter enclensis. The purified compound was found to be a α-glucosidase inhibitor, and its IC50 value was 500 ± 0.142 μg/ml. It was identified as a C7N aminocyclitol, that displayed high similarity to acarbose, but the structure has not been completely solved. Researchers have also demonstrated that its encoding gene is similar to the biosynthetic gene cluster encoding acarbose (7%).
Indupalli et al. (2018) first reported an unusual actinobacterium Saccharomonas oceani VJDS-3 isolated from the mangrove forest in India. The ethyl methoxycinnamate (ethyl (E)-3-4-methoxyphenyl) acrylate (8) was purified. It exhibited significant inhibitory activity against α-glucosidase at 20 μg/ml with an IC50 value of 66.8 ± 1.2 μg/ml and moderate to weak inhibitory activity against α-amylase at 40 μg/ml with an IC50 value of 11.5 ± 0.5 μg/ml. Saini and Gangwar (2022) obtained an endophytic actinomycete strain derived from Aegle marmelos. The ethyl acetate extract was assayed to have IC50 values of 1950.71 ± 0.11 μg/ml against α-amylase, and 391.38 ± 0.09 μg/ml against α-glucosidase. The results indicated that the crude extract has the potential to reduce postprandial blood glucose. However, further studies are needed to identify the strain and the active substance.
Other bacteria
In addition to actinomycetes, other bacteria were reported to produce glycosidase inhibitors, such as Bacillus sp. (nojirimycin; Iida et al., 1987), Vibrio sp. (El-Hady et al., 2017), and lactic acid bacteria (Nurhayati et al., 2017). Scientists have discovered many glycosidase inhibitors from Bacillus sp., Lactobacillus sp., and other bacteria in the past 5 years.
Bacillus species can produce secondary metabolites with various activities, which enable the bacterium to resist external harmful factors (Sansinenea and Ortiz, 2011; Yang et al., 2014). Five endophytes from Rhizophora stylosa roots with high α-amylase inhibitory activity were reported by Dat and Thuy. They were identified as Bacillus, Streptomyces and Pseudomvibrio. The ethyl acetate extracts of five isolated strains showed inhibitory activities against α-amylase with values ranging from 31.4 ± 3.1% to 59.7 ± 6.4% (Dat and Thuy, 2021). Further, Dat et al. (2022) investigated an endophytic Bacillus sp. RAR_M1_45 derived from the mangrove plant Rhizophora apiculata Blume. They isolated five compounds from the crude extract of Bacillus sp., that is 2-(2-heptenyl)-3-methyl-4-quinolinone (9), 3-methyl-2-(2-nonenyl)-4-quinolinone (10), 2-phenylacetic acid (11), 4-hydroxybenzoic acid (12), and (−)-jasmonic acid (13). All five compounds showed inhibitory activity against α-glucosidase with IC50 values ranging from 163.3 ± 10.66 μg/ml to 960.4 ± 8.62 μg/ml and inhibitory activity against α-amylase with IC50 values ranging from 63.87 ± 4.23 μg/ml to 649.9 ± 17.5 μg/ml.
Lactobacillus sp. is another important source of glycosidase inhibitors. Frediansyah et al. (2019) found that all three cell extracts and cell-free supernatants of Lactobacillus pentosus strains isolated from Muntingia calabura L. have inhibitory activity against α-glucosidase and α-amylase in vitro. Moreover, compared to that in the cell-free supernatant group, the cell extracts exhibited higher inhibition of α-glucosidase. However, it seemed to have the opposite effect on the inhibition of α-amylase. In addition, Gulnaz et al. (2021) reported that an extract of Lactobacillus sakei Probio65 and Lactobacillus plantarum Probio-093 has inhibitory activity against α-glucosidase and α-amylase. These two Lactobacillus strains could change the intestinal microbiota diversity in high-fat diet-induced diabetic mice, and Probio-093 had a more significant effect on the intestinal microbiota. Together, this showed that Lactobacillus comprises potential candidates to treat type 2 diabetes.
Chi et al. (2020) screened five microbial strains in Vietnam with glycosidase inhibitory effects. The strains were identified as and named Enterobacter cloacae TD-V20, Bacillus sp. TD-V21, Bacillus sp. TD-V24, Streptomyces sp. TD-X13, and Streptomyces sp. TD-X10. All extracts of the five strains exhibited inhibitory activities against α-glucosidase, and Streptomyces sp. TD-X10 showed stronger inhibitory activity against α-amylase. Eleven endophytic bacterial strains from Annona muricata were found to inhibit α-amylase activity. One strain (DS21) exhibited the highest activity with a 72.22% inhibition rate. The strain was a gram-negative bacterium, and needed to be further identified (Pujiyanto et al., 2018).
Inhibitors of glycosidases from fungus
Saito reported an α-amylase inhibitor produced by the fungus Cladosporium herbarum F-28, in contrast to the traditional opinions that only bacteria can produce glycosidase inhibitors, and this inhibitor was found to have high specificity for mammalian amylase (Saito, 1982). Further, glycosidase inhibitory activities were tested using Penicillium (Kwon et al., 2000), the endophytic fungus Stemphylium globuliferum from Trigonella foenum-graceum (Pavithra et al., 2014), Aspergillus awamori isolated from Acacia nilotica (proteinaceous α-glycosidase inhibitor; Singh and Kaur, 2016; Singh et al., 2021), and other fungi. Compared to bacteria, more fungal resources have been found in the past 5 years, including Aspergillus, Penicillium, Mycosphaerella, Alternaria and mushrooms (macrofungi), which make many novel compounds with high inhibitory activity against glycosidases (Table 2).
Aspergillus
Aspergillus species are a source of bioactive secondary metabolites, especially marine-derived and endophytic strains. Sun et al. (2018) identified new meroterpenoids (R,E)-3-(2,2-dimethyl chroman6-yl)-4-hydroxy-5-((2-(2-hydroxypropan-2-yl)-2,3-dihydrobenzofuran-5-yl)methylene) furan-2(5H)-one (14) isolated from the marine fungus Aspergillus terreus OUCMDZ-2739, which showed significant inhibitory activity against α-glucosidase with an IC50 value of 24.8 μM. They also tested the known compounds rubrolide S and butyrolactone I from A. terreus, which also exhibited stronger α-glucosidase inhibitory activity, with IC50 values of 1.2 μM and 61.6 μM, respectively (Figure 2).
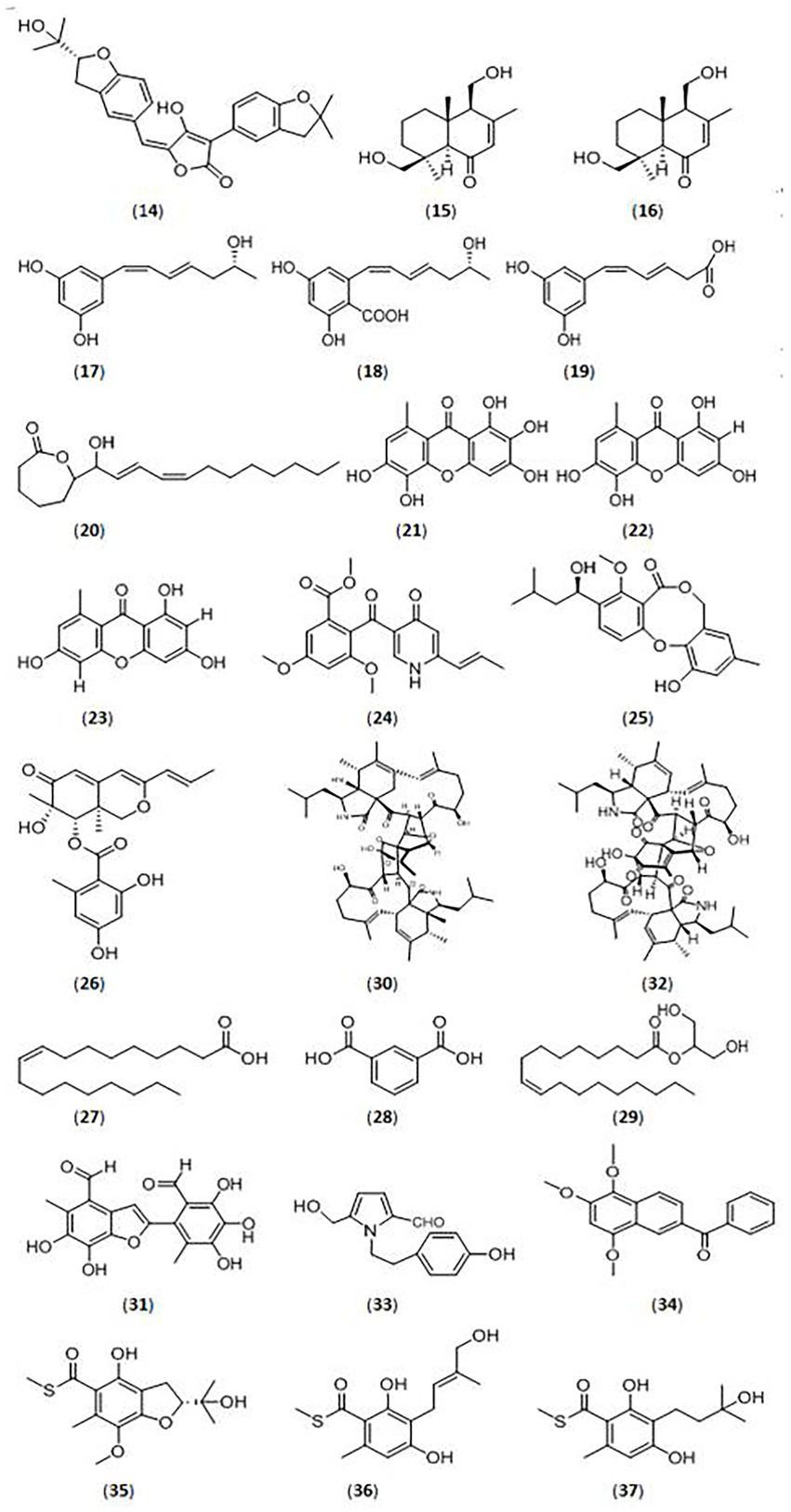
Figure 2. Glycosidase inhibitors compound from fungi. (14).(R,E)-3-(2,2-dimethyl chroman6-yl)-4-hydroxy-5-((2-(2-hydroxypropan-2-yl)-2,3-dihydrobenzofuran-5-yl)methylene) furan-2(5H)-one; (15).(4S,5R,9S,10R)-11,13-dihydroxy-drim-7-en-6-one; (16).5-((R,1Z,3E)-6-hydroxy-1,3-heptadien-1-yl)-1,3-benzenediol; (17).4-carboxy-5-((R,1Z,3E)-6-hydroxy-1,3-heptadien-1-yl)- 1,3-benzenediol; (18).4-carboxy-5-((1Z,3E)-1,3-heptadien1-yl)-1,3-benzenediol; (19). (1Z,3E)-4-carboxy-1,3-butadienyl-1-yl)-1,3-benzenediol; (20).ε-caprolactone derivative; (21).unreported xanthone; (22–23).xanthones; (24).vermistatin; (25).penicillide; (26).Sch725680; (27).oleic acid; (28).1,3-benzenedicarboxylic acid; (29).hexadecanoic acid; (30).asperchalasine I; (31).epicoccolide B; (32).asperchalasine A; (33).5-hydroxymethyl-1-[2-(4-hydroxyphenyl)-ethyl]-1H-pyrrole-2-carbaldehyde; (34).nigronapthaphenyl; (35).eurothiocins C; (36).eurothiocins F; (37).eurothiocins G.
The Aspergillus egypticus HT166S (endophytic fungus) was isolated from the plant Helianthus tuberosus by Ruzieva et al. (2020). Methanol extracts were found to possess α-amylase inhibitory activity with 75.4% inhibition rate. The methanol component contained flavonoids, terpenoids, anthraquinones, tannins and other bioactive compounds, and flavonoids had the highest activity.
Penicillium
Penicillium species are traditional glycosidase inhibitor-producing bacteria. Gou et al. (2021) identified 18 compounds (eight new and ten known compounds) from a marine fungus Penicillium sp. TW58-16. Among them, five new compounds ((4S,5R,9S,10R)-11,13-dihydroxy-drim-7-en-6-one (15), 5-((R,1Z,3E)-6-hydroxy-1,3-heptadien-1-yl)-1,3-benzenediol (16), 4-carboxy-5-((R,1Z,3E)-6-hydroxy-1,3-heptadien-1-yl)- 1,3-benzenediol(17), 4-carboxy-5-((1Z,3E)-1,3-heptadien1-yl)-1,3-benzenediol(18), and 5-((1Z,3E)-4-carboxy-1,3-butadienyl-1-yl)-1,3-benzenediol (19)) showed significant α-glucosidase inhibitory activities, with inhibition rates from 32.0 to 74.4%, and a ε-caprolactone derivative (20) showed the highest inhibitory effects (91.1%).
Malik et al. (2020) investigated an endophytic Penicillium canescens strain isolated from Juniperus polycarpos fruits, and identified three xanthones (one novel xanthone (21) and another two known xanthones (22–23)) with inhibitory effects against α-glucosidase. The three compounds displayed α-glucosidase inhibitory activities, with IC50 values from 32.32 ± 1.01 μM to 75.20 ± 1.02 μM.
The endophytic fungus Penicillium pinophilum SCAU037, isolated from mangrove, was studied by He et al. (2019). Three compounds, vermistatin (24), penicillide (25), and Sch725680 (26), were purified and showed stronger α-glucosidase inhibitory activity with IC50 values of 51.9 μM, 78.4 μM, and 33.8 μM, respectively.
Siregar et al. isolated four endophytic fungi (Penicillium and Aspergillus) from the Raru plant (Situmorang et al., 2015). The Penicillium TR3 strain displayed significant α-amylase inhibitory activity with an inhibition rate of 96.34%. They separated and identified several compounds, and compound 27 (oleic acid; Ahmad et al., 2012; Indrianingsih et al., 2018), compound 28 (1,3-benzenedicarboxylic acid, bis (2-ethylhexyl) ester, isophthalic acid group; Rajan and Aanandhi, 2017; Abusufyan et al., 2018), compound 29 (hexadecanoic acid, 2-hydroxy-1-(hydroxymethyl) ethyl ester (CAS), palmitin group; Venkatramanan et al., 2020) showed inhibitory activities against α-glucosidase and α-amylase (Siregar et al., 2022).
Mycosphaerellaceae
Researchers have reported endophytic Mycosphaerellaceae strains as a source of glycosidase inhibitors, and have isolated novel compounds from them in the most recent 5 years. Qiu et al. (2019) isolated three compounds (asperchalasine I(30), epicoccolide B(31), asperchalasine A(32)) from the mangrove fungus Mycosphaerella sp. SYSU-DZG01, which showed stronger α-glucosidase inhibitory activity with IC50 values 17.1, 26.7, and 15.7 μM, respectively. Moreover, asperchalasine I (30) was a novel compound with significant α-glucosidase inhibitory activity, indicating its medicinal potential.
Lopéz et al. (2019) studied the endophytic Mycosphaerellaceae fungus Zasmidium sp. EM5-10 isolated from mangrove leaves. The crude extract of this fungus showed stronger α-glucosidase inhibitory activity, with an inhibition rate of 91.3%.
Alternaria
Endophytic Alternaria species were also found to be active against different glycosidases. The endophytic fungus Alternaria sp. QPS 05, with strong inhibitory activity against α-glucosidase, was isolated from Quercus phillyraeoides A. Gray by Indrianingsih et al. Researchers separated a fatty acid extract with significant α-glucosidase inhibitory activity. Further studies revealed that the mixture contained linoleic acid, oleic acid, palmitic acid, and linolenic acid (Indrianingsih et al., 2018).
Khan et al. (2019) reported an aqueous extract of the endophytic fungus JCO (Alternaria sp.), isolated from Syzygium cumini L (jambolana), which had stronger inhibitory activity against α-amylase (62%). Further, the endophytic fungi MAN (Aspergillus sp.), MBR, isolated from Mangifera indica (mango), and NGU, NTH, isolated from Azadirachta indica (neem) exhibited α-amylase inhibitory activity from 49 to 59%.
Mushrooms
Mushrooms are fungi which have broad application as functional foods and medicinal products based on their active compounds (Papoutsis et al., 2021). Many scientists have demonstrated that different mushrooms have strong anti-diabetic, antioxidant, anti-viral, anti-tumor, and immunoregulatory bioactivities (Thakur, 2020). Chen et al. isolated many compounds from Grifola frondosa, a type of wild mushroom. Further studies demonstrated that compound 5-hydroxymethyl-1-[2-(4-hydroxyphenyl)-ethyl]-1H-pyrrole-2-carbaldehyde (33) showed significant inhibitory activities against α-glucosidase from baker’s yeast and mammalian intestines, with IC50 values of 44.42 μM ± 5.11 μM and 28.65 μM ± 3.14 μM, respectively (Chen et al., 2018; Papoutsis et al., 2021).
An edible oyster mushroom was also collected from India, and its inhibitory activity against α-amylase was studied by Tamboli et al. (2018). Different extracts were prepared, and methanol, acetone, and chloroform extracts showed inhibitory activities against α-amylase, with IC50 values of 383, 224, and 1.71 μg/ml, respectively. Further studies on its active composition showed that flavonoids in the acetone extract and glycoproteins in the chloroform extract could be the active components.
Kumar et al. (2018) tested extracts from two edible macrofungi (Dacryopinax spathularia and Schizophyllumcommune), for their inhibitory activities against α-amylase. The extract of D. spathularia showed an α-amylase inhibition rate of 38.24% at 1000 μg/ml, and the extract of S. commune had a rate of 48.19%.
Stojkovic et al. (2019) investigated the in vitro anti-diabetic properties of six edible and medicinal mushroom species, Agaricus blazei Murrill, Coprinus comatus, Cordyceps militaris, Inonotus obliquus, Morchella conica and Phellinus linteus. The methanol extract of C. comatus showed the highest inhibitory activity against α-amylase with an IC50 value of 714.45 μg/ml. All of the six tested macrofungi had inhibitory effects against α-glucosidase, and the strongest inhibitory effect was found with I. obliquus, with an IC50 value of 220.31 μg/ml, which was the most potent strain.
Other fungi
A variety of other fungi, especially endophytic fungi, were also discovered for their inhibitory activities against glycosidases in recent years. Nigronapthaphenyl (34), a new compound extracted from the endophytic fungus Nigrospora sphaerica derived from the mangrove Bruguiera gymnorrhyza was reported by Ukwatta et al. (2019). The new substance displayed strong inhibitory activity against α-glucosidase (IC50 value of 6.9 ± 0.5 μM). Sharma et al. (2021) isolated the endophytic fungus Schizophyllum commune Fr. from Aloe vera and its extract showed more than 90% inhibitory activity against α-glucosidase. Treatment of STZ-induced diabetic rats with the fungus extract reduced blood glucose levels. Phenols and terpenoids were identified in the ethyl acetate extract, which could be the active ingredients. Saravanakumar et al. (2021) surveyed the glycosidase inhibitory activities of the endophyte Diaporthe eres (SPEF004) derived from the Ligustrum obtusifolium leaf. The ethyl acetate extract of D. eres displayed α-glucosidase inhibitory activity of 13.28 ± 0.94% and α-amylase inhibitory activity of 41.11 ± 1.52%. An endophytic fungal Colletotrichum species derived from Salacia macrosperma was detected by Roopa et al. (2022). The fungal extract showed inhibitory effects against α-glucosidase and α-amylase with IC50 values of 124.62 and 106.11 μg/ml, respectively.
The marine fungus Talaromyces indigoticus FS688 was studied by Li et al. (2021), and many bioactive compounds were isolated. The compound eurothiocins C (35) had stronger α-glucosidase inhibitory activity (IC50 value of 5.4 μM), but eurothiocins F (36) and eurothiocins G (37) displayed lower activities with IC50 values of 33.6 μM and 72.1 μM, respectively.
Alcantara et al. (2019) reported the anti-diabetic effects of various extracts of Fuscoporia torulosa MFSLP-12. A methanol extract of this fungus had significant α-glucosidase inhibitory activity, with an inhibition rate of 56%, and α-amylase inhibitory activity with inhibition rate of 38%. The IC50 value was about 5-fold and 9-fold higher for α-glucosidase and α-amylase than the control drug acarbose.
Applications of microbial α-glucosidase and α-amylase inhibitors
Diabetes
Type 2 diabetes mellitus is a genetically heterogeneous metabolic disorder characterized by high blood glucose levels, and insulin is the pancreatic hormone that controls blood sugar (DeFronzo, 2004; Artasensi et al., 2020). There is no cure for diabetes currently, but recent studies proves that diabetes morbidity and mortality can be controlled with optimal medical therapy, a healthy diet, and physical exercise (Asano, 2003).
Pharmacological therapy
Reducing postprandial hyperglycemia is the most important treatment for diabetes. The researchers realized that the inhibition of glucosidase with inhibitors could regulate the absorption of carbohydrates and prevent postprandial hyperglycemia (Asano, 2003). Those inhibitors could cause the release of GLP-1 and reduce glycated hemoglobin levels (Kumar and Sinha, 2012). Glucosidase inhibitors, such as acarbose, voglibose, and miglitol, are important first-line agents for type 2 diabetes patients (Hossain and Pervin, 2018; Dhatariya, 2019). Also, these drugs can be used as second-line agents in combination with metformin, which could reduce the dosage of metformin and improve safety (Clissold and Edwards, 1988; Chan et al., 2018).
Acarbose (38), isolated from Actinoplanes sp. SE50, was the first commercialized glucosidase inhibitor launched in 1990 (Schmidt et al., 1977; Joshi et al., 2014). It is one of the most common glycosidase inhibitors, and also the most widely studied one. Acarbose inhibits many glycosidases, such as α-amylase, maltase, and glucoamylase, which could reduce the hydrolysis of starch in intestine (Liu and Ma, 2017; Li et al., 2022). Voglibose (39), isolated from Streptomyces hygroscopicies limonons, was discovered in 1981 (Japan), and marketed for clinical use since 1994 (Horii et al., 1986; Saito et al., 1998). It is a more tolerated and potent inhibitor of α-glycosidase than acarbose with fewer side effects and higher activities (Dabhi et al., 2013). Miglitol (40) was developed by Bayer and first marketed in 1998 (Kumar and Sinha, 2012). It is a derivative of 1-desoxynojirimycin (DNJ). DNJ can be isolated from S. lavendulae or other strains, and then chemically synthesized to form miglitol (Sels et al., 1999). Miglitol is completely absorbed by the small intestine with high bioavailability, whereas acarbose and voglibose are poorly absorbed with low bioavailability (Akmal and Wadhwa, 2022; Figure 3).

Figure 3. Clinical medicine of glycosidase inhibitors. (38).acarbose; (39).voglibose; (40).miglitol.
Dietary supplements
The presence of glycosidase inhibitors in the diet can inhibit the activity of human glycosidase and reduce the absorption of dietary carbohydrates (Park et al., 2021). In addition, there has been increasing concern about the possibility of using dietary supplements to prevent diabetes. For example, Salacia reticulata is used as a diabetic supplement in Japan (Asano, 2003; Zou et al., 2019).
Obesity
Diet control is an important way to control obesity. Glycosidase are responsible for carbohydrate digestion, and increase postprandial blood sugar levels. Glycosidase inhibitors are potential compounds that can be used in weight loss. They inhibit glucosidase, delay the absorption of carbohydrates, and reduce people’s postprandial blood sugar levels and insulin responses to dietary carbohydrates (Peddio et al., 2022).
Also, another important factor for obesity is the abnormal differentiation or adipocytes dysfunction. Li et al. illustrated that DNJ, an α-glucosidase inhibitor, can inhibit adipogenesis during the differentiation of white preadipocytes, providing a new approach to explain the beneficial effects of α-glucosidase inhibitor on obesity (Li et al., 2019).
Antiviral treatment
N-nonyl-deoxynojirimycin (NN-DNJ, α-glucosidase inhibitor derivative) is a potential antiviral drug. Block et al. reported that NN-DNJ induces misfolding of the hepatitis B virus envelope glycoproteins and further prevents virus formation (Block et al., 1998). Moreover, Zitzmann et al. demonstrated that NN-DNJ could prevent the formation and secretion of bovine viral diarrhea virus, a model for human hepatitis C virus (Zitzmann et al., 1999; Whitby et al., 2004). Also, N-butyl-deoxynojirimycin (α-glucosidase inhibitor) and its derivatives show significant antiviral against Ebola virus in vitro. It inhibits assembly and secretion of virus particle (Chang et al., 2013; Yuan, 2015).
Lysosomal storage diseases
The lysosomal storage diseases are a group of inherited diseases that lead to metabolic disorders of the lysosomes. The diseases mainly include Fabry disease, Gaucher disease, Niemann-Pick disease and so on (Khanna et al., 2010; Mechtler et al., 2012). Giraldo et al. reported the treatment of type 1 Gaucher disease with N-butyl-deoxynojirimycin (α-glucosidase inhibitor) over 12 years. Eventually 80% of patients achieved the treatment goals, with stable levels of hematologic counts and volumes of the liver and spleen (Giraldo et al., 2018).
Conclusion and further research
In recent years, the incidence of type 2 diabetes has been growing rapidly. Glucose, which is hydrolyzed by glycosidases, is absorbed into the blood, and then caused severe postprandial hyperglycemia. So glycosidase is an important therapeutic target for diabetes (Usman et al., 2019). However, marketed inhibitors of glycosidase have many side effects. Therefore, novel glycosidase inhibitors that are safer, more effective, and more cost-effective are needed.
Glycosidases are produced by microbes, animals and plants (Aslan et al., 2018; Demir et al., 2018). However, inhibitors of glycosidase are mainly derived from microbes. In the most recent 5 years, increasing research on new microorganisms producing inhibitors of glycosidase has been reported. Compared to general microorganisms, most new microorganisms are extremophiles, which is reflected by the number of papers on new microorganisms. This new focus on extremophiles expands the scope of the search glycosidase inhibitors. Five reports introduced nine new compounds with inhibitory activities against glycosidases in recent 5 years. They are compound 14 isolated from the marine fungus Aspergillus terreus OUCMDZ-2739 by Sun et al.; five new compounds 15–19 from a marine fungus Penicillium sp. by Guo et al.; novel xanthone (21) isolated from endophytic Penicillium canescens strain by Malik et al.; asperchalasine I (30) isolated from the mangrove fungus Mycosphaerella sp. by Qiu et al.; and Nigronapthaphenyl (34) extracted from the endophytic fungus Nigrospora sphaerica by Ukwatta et al. All of them come from marine and endophytic fungus. It would be very useful if a database for microorganisms and its glycosidase inhibitors was established.
In addition to applications in the treatment of diabetes and obesity, several applications for glycosidase inhibitors have been reported but have not yet been industrially developed. We look forward to seeing the use of these inhibitors expand.
Author contributions
XW, JL, and JS reviewed conceptualization and wrote the manuscript. JB, KW, JL, and ZY collected the data from previous researches and prepared the figures and tables. HO designed of the work and reviewed critically for important intellectual content. LS designed and supervised the paper. All authors contributed to the article and approved the submitted version.
Funding
This research was financially supported by the Natural Science Foundation of Shanghai (20ZR1424600), the National Natural Science Foundation of China (81773616), the Shanghai Excellent Technology Leader Program (17XD1423200), and Nature Science Foundation of Jiangsu Higher Education Institutions of China (20KJB180002).
Conflict of interest
The authors declare that the research was conducted in the absence of any commercial or financial relationships that could be construed as a potential conflict of interest.
Publisher’s note
All claims expressed in this article are solely those of the authors and do not necessarily represent those of their affiliated organizations, or those of the publisher, the editors and the reviewers. Any product that may be evaluated in this article, or claim that may be made by its manufacturer, is not guaranteed or endorsed by the publisher.
References
Abusufyan, S., Ibrahim, M., and Mohib, K. (2018). Comparative in vitro antidiabetic and antioxidant activity of various extracts of Ficus species. Pharm. J. 10, 349–354. doi: 10.5530/pj.2018.2.59
Agrawal, S., Samanta, S., and Deshmukh, S. K. (2022). The antidiabetic potential of endophytic fungi: future prospects as therapeutic agents. Biotechnol. Appl. Biochem. 69, 1159–1165. doi: 10.1002/bab.2192
Ahmad, Z., Zamhuri, K. F., Yaacob, A., Siong, C. H., Selvarajah, M., Ismail, A., et al. (2012). In vitro anti-diabetic activities and chemical analysis of polypeptide-k and oil isolated from seeds of Momordica charantia (bitter gourd). Molecules 17, 9631–9640. doi: 10.3390/molecules17089631
Akmal, M., and Wadhwa, R. (2022). “Alpha glucosidase inhibitors,” in Stat pearls [internet]. eds. M. Akmal and R. Wadhwa (Treasure Island, FL: Stat Pearls Publishing)
Alcantara, M., Kimberlee, F., and Calupitan, J. R. H. F. (2019). Inhibitory potential of Fuscoporia torulosa MFSLP-12 extracts on enzymes α-amylase and α-glucosidase in vitro. Doctoral dissertation 27, 631–642.
Artasensi, A., Pedretti, A., Vistoli, G., and Fumagalli, L. (2020). Type 2 diabetes mellitus: a review of multi-target drugs. Molecules 25:1987. doi: 10.3390/molecules25081987
Asano, N. (2003). Glycosidase inhibitors: update and perspectives on practical use. Glycobiology 13, 93R–104R. doi: 10.1093/glycob/cwg090
Aslan, T. P., Demir, H. E., Oztaskin, Y. N., Maraş, A., Gulçin, İ., and Goksu, S. (2018). Diarylmethanon, bromophenol and diarylmethane compounds: discovery of potent aldose reductase, α-amylase and α-glycosidase inhibitors as new therapeutic approach in diabetes and functional hyperglycemia. Int. J. Biol. Macromol. 119, 857–863. doi: 10.1016/j.ijbiomac.2018.08.004
Baron, A. D. (1998). Postprandial hyperglycaemia and alpha-glucosidase inhibitors. Diabetes Res. Clin. Pract. 40, S51–S55. doi: 10.1016/s0168-8227(98)00043-6
Bisaria, K., Sinha, S., Srivastava, A., and Singh, R. (2020). “Marine pharmacognosy: an overview of marine-derived pharmaceuticals,” in Marine Niche: Applications in Pharmaceutical Sciences. eds. K. Bisaria, S. Sinha, A. Srivastava, and R. Singh (Singapore: Springer). 361–381.
Block, T. M., Lu, X., Mehta, A. S., Blumberg, B. S., Tennant, B., Ebling, M., et al. (1998). Treatment of chronic hepadnavirus infection in a woodchuck animal model with an inhibitor of protein folding and trafficking. Nat. Med. 4, 610–614. doi: 10.1038/nm0598-610
Chan, C. W., Yu, C. L., Lin, J. C., Hsieh, Y. C., Lin, C. C., Hung, C. Y., et al. (2018). Glitazones and alpha-glucosidase inhibitors as the second-line oral anti-diabetic agents added to metformin reduce cardiovascular risk in type 2 diabetes patients: a nationwide cohort observational study. Cardiovasc. Diabetol. 17, 1–13. doi: 10.1186/s12933-018-0663-6
Chandrasekhar, C., Rajpurohit, H., Javaji, K., Kuncha, M., Setti, A., Ali, A. Z., et al. (2021). Anti-hyperglycemic and genotoxic studies of 1-O-methyl chrysophanol, a new anthraquinone isolated from Amycolatopsis thermoflava strain SFMA-103. Drug Chem. Toxicol. 44, 148–160. doi: 10.1080/01480545.2018.1551406
Chang, J., Warren, T. K., Zhao, X., Gill, T., Guo, F., Wang, L., et al. (2013). Small molecule inhibitors of ER α-glucosidases are active against multiple hemorrhagic fever viruses. Antivir. Res. 98, 432–440. doi: 10.1016/j.antiviral.2013.03.023
Chen, Z., Hao, J., Wang, L., Wang, Y., Kong, F., and Zhu, W. (2016). New α-glucosidase inhibitors from marine algae-derived Streptomyces sp. OUCMDZ-3434. Sci. Rep. 6, 1–9. doi: 10.1038/srep20004
Chen, S., Yong, T., Xiao, C., Su, J., Zhang, Y., Jiao, C., et al. (2018). Pyrrole alkaloids and ergosterols from Grifola frondosa exert anti-α-glucosidase and anti-proliferative activities. J. Funct. Foods 43, 196–205. doi: 10.1016/j.jff.2018.02.007
Chen, S., Zhang, Q., Zhang, X., Jiang, X., Zhang, H., Zhu, Y., et al. (2022). A new xanthostatin analog from the marine sponge-associated actinomycete Streptomyces sp. SCSIO 40064. Nat. Prod. Res. 36, 3529–3537. doi: 10.1080/14786419.2020.1867131
Chi, H. K., Quang, T. H., and Hang, T. T. N. (2020). Screening and priliminary study on microbial strains with inhibitory activity against enzymes relating to diabetes in Viet Nam. Vietnam J. Sci. Technol. 58, 209–217. doi: 10.15625/2525-2518/58/6A/15528
Clissold, S. P., and Edwards, C. (1988). Acarbose. A preliminary review of its pharmacodynamic and pharmacokinetic properties, and therapeutic potential. Drugs 35, 214–243. doi: 10.2165/00003495-198835030-00003
Dabhi, A. S., Bhatt, N. R., and Shah, M. J. (2013). Voglibose: an alpha glucosidase inhibitor. J. Clin. Diagn. Res. 7, 3023–3027. doi: 10.7860/JCDR/2013/6373.3838
Dat, T. T. H., Ha, D. V., Oanh, P. T. T., Nhi, N. P. K., Anh, H. L. T., Quy, P. T., et al. (2022). The study on biological activity and molecular docking of secondary metabolites from Bacillus sp. isolated from the mangrove plant Rhizophora apiculata Blume. Reg. Stud. Mar. Sci. 55:102583. doi: 10.1016/j.rsma.2022.102583
Dat, T. T. H., and Thuy, O. P. T. (2021). In vitro antioxidant, α-amylase and α-glucosidase inhibitory activities of endophytic bacteria from the roots of the mangrove plant Griffith. Acad. J. Biol. 43, 125–135. doi: 10.15625/2615-9023/16143
Davies-Bolorunduro, O., Osuolale, O., Saibu, S., Adeleye, I., and Aminah, N. (2021). Bioprospecting marine actinomycetes for antileishmanial drugs: current perspectives and future prospects. Heliyon 7:e07710. doi: 10.1016/j.heliyon.2021.e07710
DeFronzo, R. A. (2004). Pathogenesis of type 2 diabetes mellitus. Med. Clin. 88, 787–835. doi: 10.1016/j.mcna.2004.04.013
Demir, Y., Taslimi, P., Ozaslan, M. S., Oztaskin, N., Cetinkaya, Y., Gulcin, I., et al. (2018). Antidiabetic potential: in vitro inhibition effects of bromophenol and diarylmethanones derivatives on metabolic enzymes. Arch. Pharm (Weinheim) 351:e1800263. doi: 10.1002/ardp.201800263
Deshmukh, S. K., Dufossé, L., Chhipa, H., Saxena, S., Mahajan, G. B., and Gupta, M. K. (2022). Fungal endophytes: a potential source of antibacterial compounds. J. Fungi 8:164. doi: 10.3390/jof8020164
Deshmukh, S. K., Gupta, M. K., Prakash, V., and Saxena, S. (2018). Endophytic fungi: a source of potential antifungal compounds. J. Fungi 4:77. doi: 10.3390/jof4030077
Dhatariya, K. (2019). Diabetes: the place of new therapies. Ther. Adv. Endocrinol. Metab. 10:204201881880759. doi: 10.1177/2042018818807599
Elaiyaraja, V., Latha, M. H., and Devi, C. S. (2018). Optimization and production of anti-inflammatory and anti-diabetic metabolites from marine Streptomyces sp. VITJS8. Res. J. Pharm. Technol. 11, 2866–2868. doi: 10.5958/0974-360X.2018.00528.0
El-Gendy, M. M. A. A., Yahya, S. M., Hamed, A. R., and El-Bondkly, A. M. A. (2022). Assessment of the phylogenetic analysis and antimicrobial, antiviral, and anticancer activities of marine endophytic Streptomyces species of the soft coral Sarcophyton convolutum. Int. Microbiol. 25, 133–152. doi: 10.1007/s10123-021-00204-x
El-Hady, A., Faten, K., Fayad, W., Iodice, C., El-Shahid, Z. A., Abdel-Aziz, M. S., et al. (2017). Investigating on the correlation between some biological activities of marine sponge-associated bacteria extracts and isolated diketopiperazines. Curr. Microbiol. 74, 6–13. doi: 10.1007/s00284-016-1144-3
Ezure, Y., Maruo, S., Miyazaki, K., and Kawamata, M. (1985). Moranoline (1-deoxynojirimycin) fermentation and its improvement. Agric. Biol. Chem. 49, 1119–1125. doi: 10.1080/00021369.1985.10866866
Frediansyah, A., Nurhayati, R., and Sholihah, J. (2019). “Lactobacillus pentosus isolated from Muntingia calabura shows inhibition activity toward alpha-glucosidase and alpha-amylase in intra and extracellular level.” in IOP Conference Series: Earth and Environmental Science. (Bristol, Indonesia: IOP Publishing).
Gangadevi, V., and Muthumary, J. (2009). A novel endophytic taxol-producing fungus Chaetomella raphigera isolated from a medicinal plant, Terminalia arjuna. Appl. Biochem. Biotechnol. 158, 675–684. doi: 10.1007/s12010-009-8532-0
Gill, H., and Vasundhara, M. (2019). Isolation of taxol producing endophytic fungus Alternaria brassicicola from non-Taxus medicinal plant Terminalia arjuna. World J. Microbiol. Biotechnol. 74, 83–87. doi: 10.1016/j.tet.2017.11.039
Giraldo, P., Andrade-Campos, M., Alfonso, P., Irun, P., Atutxa, K., Acedo, A., et al. (2018). Twelve years of experience with miglustat in the treatment of type 1 Gaucher disease: the Spanish ZAGAL project. Blood Cell Mol. Dis. 68, 173–179. doi: 10.1016/j.bcmd.2016.10.017
Gou, X., Tian, D., Wei, J., Ma, Y., Zhang, Y., Chen, M., et al. (2021). New drimane sesquiterpenes and polyketides from marine-derived fungus Penicillium sp. TW58-16 and their anti-inflammatory and α-glucosidase inhibitory effects. Mar. Drugs 19:416. doi: 10.3390/md19080416
Gulnaz, A., Nadeem, J., Han, J. H., Lew, L. C., Son, J. D., Park, Y. H., et al. (2021). Lactobacillus SPS in reducing the risk of diabetes in high-fat diet-induced diabetic mice by modulating the gut microbiome and inhibiting key digestive enzymes associated with diabetes. Biology 10:348. doi: 10.3390/biology10040348
He, F., Li, X., Yu, J. H., Zhang, X., Nong, X., Chen, G., et al. (2019). Secondary metabolites from the mangrove sediment-derived fungus Penicillium pinophilum SCAU037. Fitoterapia 136:104177. doi: 10.1016/j.fitote.2019.104177
Heacock, P. M., Hertzler, S. R., Williams, J. A., and Wolf, B. W. (2005). Effects of a medical food containing an herbal alpha-glucosidase inhibitor on postprandial glycemia and insulinemia in healthy adults. J. Am. Diet. Assoc. 105, 65–71. doi: 10.1016/j.jada.2004.11.001
Horii, S., Fukase, H., Matsuo, T., Kameda, Y., Asano, N., and Matsui, K. (1986). Synthesis and. Alpha.-D-glucosidase inhibitory activity of N-substituted valiolamine derivatives as potential oral antidiabetic agents. J. Med. Chem. 29, 1038–1046. doi: 10.1021/jm00156a023
Hossain, M. A., and Pervin, R. (2018). “Current antidiabetic drugs: review of their efficacy and safety” in Nutritional and Therapeutic Interventions for Diabetes and Metabolic Syndrome (Cambridge, MA: Academic Press), 455–473.
Iida, H., Yamazaki, N., and Kibayashi, C. (1987). Total synthesis of (+)-nojirimycin and (+)-1-deoxynojirimycin. J. Org. Chem. 52, 3337–3342. doi: 10.1021/jo00391a031
Indrianingsih, A. W., Prihantini, A. I., and Tachibana, S. (2018). Isolation of endophytic fungi QPS 05 from quercus phillyraeoides a. gray and its potential for ±−glucosidase inhibitory activity. Jurnal Kimia Terapan Indonesia 20, 1–7. doi: 10.14203/jkti.v20i1.372
Indupalli, M., Muvva, V., Mangamuri, U., Munaganti, R. K., and Naragani, K. (2018). Bioactive compounds from mangrove derived rare actinobacterium Saccharomonospora oceani VJDS-3. Biotechnology 8, 103–109. doi: 10.1007/s13205-018-1093-6
Joshi, S. R., Ramachandran, A., Chadha, M., Chatterjee, S., Rathod, R., and Kalra, S. (2014). Acarbose plus metformin fixed-dose combination in the management of type 2 diabetes. Expert. Opin. Pharmacother. 15, 1611–1620. doi: 10.1517/14656566.2014.932771
Kameda, Y., Asano, N., Yoshikawa, M., Takeuchi, M., Yamaguchi, T., Matsui, K., et al. (1984). Valiolamine, a new alpha-glucosidase inhibiting aminocyclitol produced by Streptomyces hygroscopicus. J. Antibiot. (Tokyo) 37, 1301–1307. doi: 10.7164/antibiotics.37.1301
Karthikeyan, A., Joseph, A., and Nair, B. G. (2022). Promising bioactive compounds from the marine environment and their potential effects on various diseases. J. Genet. Eng. Biotechnol. 20, 14–38. doi: 10.1186/s43141-021-00290-4
Khan, R., Naqvi, S. T. Q., Fatima, N., and Muhammad, S. A. (2019). 30. Study of antidiabetic activities of endophytic fungi isolated from plants. Pure Appl. Biol. 8, 1287–1295. doi: 10.19045/bspab.2019.80071
Khanna, R., Soska, R., Lun, Y., Feng, J., Frascella, M., Young, B., et al. (2010). The pharmacological chaperone 1-deoxygalactonojirimycin reduces tissue globotriaosylceramide levels in a mouse model of Fabry disease. Mol. Ther. 18, 23–33. doi: 10.1038/mt.2009.220
Kumar, A., Dandapat, S., Kumar, M., and Sinha, M. (2018). Anti-inflammatory and amylase inhibitory properties of indian edible macrofungi Dacryopinax spathularia (Schwein) and Schizophyllum commune (fries). Bioscan 13, 715–718.
Kumar, S. R. S., and Rao, K. V. B. (2018). Efficacy of alpha glucosidase inhibitor from marine Actinobacterium in the control of postprandial hyperglycaemia in Streptozotocin (STZ) induced diabetic male albino Wister rats. Iran. J. Pharm. Res. 17, 202–214. doi: 10.22037/ijpr.2018.2182
Kumar, R. V., and Sinha, V. R. (2012). Newer insights into the drug delivery approaches of α-glucosidase inhibitors. Expert Opin. Drug Deliv. 9, 403–416. doi: 10.1517/17425247.2012.663080
Kwon, O. S., Park, S. H., Yun, B. S., Pyun, Y. R., and Kim, C. J. (2000). Cjcclo (Dehydroala-L-Leu), an α-glucosidase inhibitor from Penicillium sp. F7Q6l4. J. Antibiot. 53, 954–958. doi: 10.7164/antibiotics.53.954
Lacerda, I. C. D. S., Polonio, J. C., and Golias, H. C. (2022). Endophytic fungi as a source of antiviral compounds–a review. Chem. Biodivers. 19:e202100971. doi: 10.1002/cbdv.202100971
Li, X., Bai, Y., Jin, Z., and Svensson, B. (2022). Food-derived non-phenolic α-amylase and α-glucosidase inhibitors for controlling starch digestion rate and guiding diabetes-friendly recipes. LWT 153:112455. doi: 10.1016/j.lwt.2021.112455
Li, A. N., Chen, J. J., Li, Q. Q., Zeng, G. Y., Chen, Q. Y., Chen, J. L., et al. (2019). Alpha-glucosidase inhibitor 1-Deoxynojirimycin promotes beige remodeling of 3T3-L1 preadipocytes via activating AMPK. Biochem. Biophys. Res. Commun. 509, 1001–1007. doi: 10.1016/j.bbrc.2019.01.023
Li, M., Li, S., Hu, J., Gao, X., Wang, Y., Liu, Z., et al. (2021). Thioester-containing benzoate derivatives with α-glucosidase inhibitory activity from the deep-sea-derived fungus Talaromyces indigoticus FS688. Mar. Drugs 20:33. doi: 10.3390/md20010033
Liu, Z., and Ma, S. (2017). Recent advances in synthetic α-glucosidase inhibitors. Chem. Med. Chem. 12, 819–829. doi: 10.1002/cmdc.201700216
Lopéz, D., Cherigo, L., Mejia, L. C., Loza-Mejía, M. A., and Martínez-Luis, S. (2019). α-Glucosidase inhibitors from a mangrove associated fungus, Zasmidium sp. strain EM5-10. BMC Chem. 13, 22–11. doi: 10.1186/s13065-019-0540-8
Malik, A., Ardalani, H., Anam, S., McNair, L. M., Kromphardt, K. J., Frandsen, R. J. N., et al. (2020). Antidiabetic xanthones with α-glucosidase inhibitory activities from an endophytic Penicillium canescens. Fitoterapia 142:104522. doi: 10.1016/j.fitote.2020.104522
Manganyi, M. C., Tchatchouang, C. D. K., Regnier, T., Bezuidenhout, C. C., and Ateba, C. N. (2019). Bioactive compound produced by endophytic fungi isolated from pelargonium sidoides against selected bacteria of clinical importance. Mycobiology 47, 335–339. doi: 10.1080/12298093.2019.1631121
Mawlankar, R. B., Dharne, M. S., and Dastager, S. G. (2020). Isolation of potent alpha-glucosidase inhibitor from a novel marine bacterium Arthrobacter enclensis. SN Applied sciences 2, 1–11. doi: 10.1007/s42452-020-2285-3
Mechtler, T. P., Stary, S., Metz, T. F., De Jesús, V. R., Greber-Platzer, S., Pollak, A., et al. (2012). Neonatal screening for lysosomal storage disorders: feasibility and incidence from a nationwide study in Austria. Lancet 379, 335–341. doi: 10.1016/S0140-6736(11)61266-X
Murao, S., and Miyata, S. (1980). Isolation and characterization of a new trehalase inhibitor, S-GI. Agric. Biol. Chem. 44, 219–221. doi: 10.1271/bbb1961.44.219
Nguyen, T. T., Phan, T. H. T., Nguyen, P. D. N., Dao, T. M. A., and Do, T. T. (2021). Optimization, purification and characterization of α-glucosidase inhibitors from Streptomyces costaricanus EBL. HB6 isolated. Vietnam 9. doi: 10.21203/rs.3.rs-931347/v1
Niwa, T., Inouye, S., Tsuruoka, T., Koaze, Y., and Niida, T. (1970). “Nojirimycin” as a potent inhibitor of glucosidase. Agric. Biol. Chem. 34, 966–968. doi: 10.1271/bbb1961.34.966
Nurhayati, R., Frediansyah, A., and Rachmah, D. L. (2017). Lactic acid bacteria producing inhibitor of alpha glucosidase isolated from Ganyong (Canna edulis) and Kimpul (Xanthosoma sagittifolium). in Paper Presented at: IOP Conference Series: Earth and Environmental Science (Bristol: IOP Publishing).
Papoutsis, K., Zhang, J., Bowyer, M. C., Brunton, N., Gibney, E. R., and Lyng, J. (2021). Fruit, vegetables, and mushrooms for the preparation of extracts with α-amylase and α-glucosidase inhibition properties: a review. Food Chem. 338:128119. doi: 10.1016/j.foodchem.2020.128119
Park, Y. S., Lee, J. Y., Hwang, K. Y., and Kim, K. (2021). Production of α-glucosidase inhibitor and 1-deoxynojirimycin by Bacillus subtilis MORI. Microbiol. Biotechnol. Lett. 49, 566–575. doi: 10.48022/mbl.2111.11008
Pathak, K., Gogoi, U., Saikia, R., Pathak, M. P., and Das, A. (2022). Marine-derived antidiabetic compounds: an insight into their sources, chemistry, SAR, and molecular mechanisms. Stud. Nat. Prod. Chem. 73, 467–504. doi: 10.1016/B978-0-323-91097-2.00004-2
Paul, J. S., Gupta, N., Beliya, E., Tiwari, S., and Jadhav, S. K. (2021). Aspects and recent trends in microbial α-amylase: a review. Appl. Biochem. Biotechnol. 193, 2649–2698. doi: 10.1007/s12010-021-03546-4
Pavithra, N., Sathish, L., Babu, N., Venkatarathanamma, V., Pushpalatha, H., Reddy, G. B., et al. (2014). Evaluation of α-amylase, α-glucosidase and aldose reductase inhibitors in ethyl acetate extracts of endophytic fungi isolated from antidiabetic medicinal plants. Int. J. Pharm. Sci. Res. 5, 5334–5341. doi: 10.13040/IJPSR.0975-8232.5(12).5334-41
Peddio, S., Padiglia, A., Cannea, F. B., Crnjar, R., Zam, W., Sharifi-Rad, J., et al. (2022). Common bean (Phaseolus vulgaris L.) α-amylase inhibitors as safe nutraceutical strategy against diabetes and obesity: An update review. Phytother. Res. doi: 10.1002/ptr.7480
Pujiyanto, S., Resdiani, M., Raharja, B., and Ferniah, R. S. (2018). α-Amylase inhibitor activity of endophytic bacteria isolated from Annona muricata L. J. Phys. 1025:012085. doi: 10.1088/1742-6596/1025/1/012085
Qiu, P., Liu, Z., Chen, Y., Cai, R., Chen, G., and She, Z. (2019). Secondary metabolites with α-glucosidase inhibitory activity from the mangrove fungus Mycosphaerella sp. SYSU-DZG01. Mar. Drugs 17:483. doi: 10.3390/md17080483
Rajan, T. S., and Aanandhi, V. M. (2017). Phytochemical evaluation and in vitro antidiabetic activity of ethanolic extract of Amaranthus tristis Linn. J. Pharm. Sci. Res. 10:2981. doi: 10.5958/0974-360X.2017.00527.3
Richard, K., Williams, D. E., De Silva, E. D., Brockman, M. A., Brumme, Z. L., Andersen, R. J., et al. (2018). Identification of novel HIV-1 latency-reversing agents from a library of marine natural products. Viruses 10:348. doi: 10.3390/v10070348
Roopa, G., Madhusudhan, M., Nischita, R., and Geetha, N. (2022). In vitro studies on anti-diabetic, anti-bacterial and phytochemical activities of endophytic fungal extracts from Salacia species. J. Med. Plants 10, 31–37. doi: 10.22271/plants.2022.v10.i4a.1434
Ruzieva, D., Gulyamova, T., and Abdulmyanova, L. (2020). Idenfication of secondary metabolites of the endophytic fungus aspergillus egypticus-HT166S inhibiting the activity of pancreatic α-amylase. Plant Cell Biotechnol. Mol. Biol. 81, 81–100. doi: 10.1016/S0378-8741(02)00059-4
Saini, P., and Gangwar, M. (2018). Inhibition spectrum, purification and kinetic study of alpha-amylase inhibitor from Murraya koenigii endophytic actinobacterium (Streptomyces koyangensis strain B025). Curr. Sci. 115, 393–395. doi: 10.18520/cs/v115/i3/393-395
Saini, P., and Gangwar, M. (2022). In vitro enzyme inhibitory and free radical scavenging potentials of an Aegle marmelos endophytic actinomycete extract. Res. J. Recent Sci. 11, 24–27.
Saito, N. (1982). Alpha-amylase inhibitor from fungus Cladosporium herbarum F-828. J. Biol. Chem. 257, 3120–3125. doi: 10.1016/S0021-9258(19)81083-9
Saito, N., Sakai, H., Suzuki, S., Sekihara, H., and Yajima, Y. (1998). Effect of an α-glucosidase inhibitor (voglibose), in combination with sulphonylureas, on glycaemic control in type 2 diabetes patients. J. Int. Med. Res. 26, 219–232. doi: 10.1177/030006059802600501
Sangkanu, S., Rukachaisirikul, V., Suriyachadkun, C., and Phongpaichit, S. (2021). Antifungal activity of marine-derived actinomycetes against Talaromyces marneffei. J. Appl. Microbiol. 130, 1508–1522. doi: 10.1111/jam.14877
Sansinenea, E., and Ortiz, A. (2011). Secondary metabolites of soil Bacillus spp. Biotechnol. Lett. 33, 1523–1538. doi: 10.1007/s10529-011-0617-5
Santos, J. D., Vitorino, I., De la Cruz, M., Díaz, C., Cautain, B., Annang, F., et al. (2019). Bioactivities and extract dereplication of Actinomycetales isolated from marine sponges. Front. Microbiol. 10:727. doi: 10.3389/fmicb.2019.00727
Saravanakumar, K., Sriram, B., Sathiyaseelan, A., Hu, X., Mariadoss, A. V. A., Mubarak Ali, D., et al. (2021). Molecular identification, volatile metabolites profiling, and bioactivities of an indigenous endophytic fungus (Diaporthe sp.). Process Biochem. 102, 72–81. doi: 10.1016/j.procbio.2020.12.002
Schmidt, D. D., Frommer, W., Junge, B., Muller, L., Wingender, W., Truscheit, E., et al. (1977). Alpha-glucosidase inhibitors. New complex oligosaccharides of microbial origin. Naturwissenschaften 64, 535–536. doi: 10.1007/BF00483561
Schwientek, P., Szczepanowski, R., Rückert, C., Kalinowski, J., Klein, A., Selber, K., et al. (2012). The complete genome sequence of the acarbose producer Actinoplanes sp. SE50/110. BMC Genom. 13, 1–18. doi: 10.1186/1471-2164-13-112
Sels, J. P. J., Huijberts, M. S., and Wolffenbuttel, B. H. (1999). Miglitol, a new α-glucosidase inhibitor. Expert. Opin. Pharmacother. 1, 149–156. doi: 10.1517/14656566.1.1.149
Shah, B., Sartaj, L., Ali, F., Shah, A., and Khan, T. (2018). Plant extracts are the potential inhibitors of α-amylase: a review. MOJ Bioequiv. Bioavailab. 5, 270–273. doi: 10.15406/mojbb.2018.05.00113
Sharma, A., Kaur, R., Kaur, J., Garg, S., Bhatti, R., and Kaur, A. (2021). An endophytic Schizophyllum commune Fr. Exhibits in-vitro and in-vivo antidiabetic activity in streptozotocin induced diabetic rats. AMB Express 11, 58–11. doi: 10.1186/s13568-021-01219-3
Siddharth, S., and Rai, V. R. (2019). Isolation and characterization of bioactive compounds with antibacterial, antioxidant and enzyme inhibitory activities from marine-derived rare actinobacteria, Nocardiopsis sp. SCA21. Microb. Pathog. 137:103. doi: 10.1016/j.micpath.2019.103775
Siddharth, S., and Vittal, R. R. (2018). Evaluation of antimicrobial, enzyme inhibitory, antioxidant and cytotoxic activities of partially purified volatile metabolites of marine Streptomyces sp.S2A. Microorganisms 6:603. doi: 10.3390/microorganisms6030072
Siddharth, S., and Vittal, R. R. (2019). Isolation, characterization, and structural elucidation of 4-methoxyacetanilide from marine actinobacteria Streptomyces sp. SCA29 and evaluation of its enzyme inhibitory, antibacterial, and cytotoxic potential. Arch. Microbiol. 201, 737–746. doi: 10.1007/s00203-019-01634-y
Singh, B., Dhaliwal, R. S., Kumar, P., and Singh, A. (2021). Insecticidal activity of a proteinaceous α-glycosidase inhibitor isolated from an endophytic Aspergillus awamori and its biosafety evaluation. Physiol. Mol. Plant Pathol. 116:101707. doi: 10.1016/j.pmpp.2021.101707
Singh, B., and Kaur, A. (2016). Antidiabetic potential of a peptide isolated from an endophytic Aspergillus awamori. J. Appl. Microbiol. 120, 301–311. doi: 10.1111/jam.12998
Siregar, P. I., Pujiyanto, S., and Lunggani, A. T. (2022). Inhibitory activity of endophytic fungi against alpha-amylase isolated from raru (Cotylelobium melanoxylon). Berkala Penelitian Hayati 28, 44–50. doi: 10.23869/bphjbr.28.1.20227
Situmorang, R. O., Harianja, A. H., and Silalahi, J. (2015). Karo’s local wisdom: the use of woody plants for traditional diabetic medicines. Indones. J. For. Res. 2, 121–130. doi: 10.20886/ijfr.2015.2.2.121-130
Solecka, J., Zajko, J., Postek, M., and Rajnisz, A. (2012). Biologically active secondary metabolites from Actinomycetes. Open Life Sci. 7, 373–390. doi: 10.2478/s11535-012-0036-1
Stojkovic, D., Smiljkovic, M., Ciric, A., Glamoclija, J., Van Griensven, L., Ferreira, I. C., et al. (2019). An insight into antidiabetic properties of six medicinal and edible mushrooms: inhibition of α-amylase and α-glucosidase linked to type-2 diabetes. S. Afr. J. Bot. 120, 100–103. doi: 10.1016/j.sajb.2018.01.007
Sun, K., Zhu, G., Hao, J., Wang, Y., and Zhu, W. (2018). Chemical-epigenetic method to enhance the chemodiversity of the marine algicolous fungus, Aspergillus terreus OUCMDZ-2739. Tetrahedron 74, 83–87. doi: 10.4314/njnpm.v8i1.11817
Tamboli, E., Bhatnagar, A., and Mishra, A. (2018). Alpha-amylase inhibitors from mycelium of an oyster mushroom. Prep. Biochem. Biotechnol. 48, 693–699. doi: 10.1080/10826068.2018.1487849
Tembhurne, S., and Sakarkar, D. (2010). Protective effect of Murraya koenigii (L) leaves extract in streptozotocin induced diabetics rats involving possible antioxidant mechanism. J. Med. Plants Res. 4, 2418–2423. doi: 10.5897/JMPR10.349
Thakur, M. (2020). Advances in mushroom production: key to food, nutritional and employment security: a review. Indian Phytopathol. 73, 377–395. doi: 10.1007/s42360-020-00244-9
Toghueo, R. M. K., and Boyom, F. F. (2019). Endophytes from ethno-pharmacological plants: sources of novel antioxidants-a systematic review. Biocatal. Agric. Biotechnol. 22:101430. doi: 10.1016/j.bcab.2019.101430
Ukwatta, K. M., Lawrence, J. L., and Wijayarathna, C. (2019). The study of antimicrobial, anti-cancer, anti-inflammatory and α-glucosidase inhibitory activities of Nigronapthaphenyl, isolated from an extract of Nigrospora sphaerica. Mycology 10, 222–228. doi: 10.1080/21501203.2019.1620892
Usman, B., Sharma, N., Satija, S., Mehta, M., Vyas, M., Khatik, G. L., et al. (2019). Recent developments in alpha-glucosidase inhibitors for management of type-2 diabetes: an update. Curr. Pharm. Des. 25, 2510–2525. doi: 10.2174/1381612825666190717104547
Venkatramanan, M., Sankar, G. P., Senthil, R., Akshay, J., Veera Ravi, A., Langeswaran, K., et al. (2020). Inhibition of quorum sensing and biofilm formation in Chromobacterium violaceum by fruit extracts of Passiflora edulis. ACS Omega 5, 25605–25616. doi: 10.1021/acsomega.0c02483
Whitby, K., Taylor, D., Patel, D., Ahmed, P., and Tyms, A. S. (2004). Action of celgosivir (6 O-butanoyl castanospermine) against the pestivirus BVDV: implications for the treatment of hepatitis C. Antivir. Chem. Chemother. 15, 141–151. doi: 10.1177/095632020401500304
Yan, D., Yu-xin, S., and Qi-hua, P. (2020). Research progress on the bioactive substances of marine actinomycetes and their antitumor activities. Nat. Prod. Res. Dev. 32:323. doi: 10.16333/j.1001-6880.2019.2.017
Yang, S. Q., Li, X. M., Li, X., Li, H. L., Meng, L. H., and Wang, B. G. (2018). New citrinin analogues produced by coculture of the marine algal-derived endophytic fungal strains Aspergillus sydowii EN-534 and Penicillium citrinum EN-535. Phytochem. Lett. 25, 191–195. doi: 10.1016/j.phytol.2018.04.023
Yang, G. J., Wang, Y., and Zheng, X. Y. (2014). Effect of low-voltage static electric field on growth of Bacillus sp. Appl. Mech. Mater. 644-650, 5415–5418.
Yokose, K., Ogawa, K., Sano, T., Watanabe, K., Maruyama, H. B., and Suhara, Y. (1983). New α-amylase inhibitor, trestatins I. isolation, characterization and biological activities of Trestatins a, B and C. J. Antibiot. 36, 1157–1165. doi: 10.7164/antibiotics.36.1157
Yuan, S. (2015). Possible FDA-approved drugs to treat Ebola virus infection. Infect. Dis. Poverty 4, 23–11. doi: 10.1186/s40249-015-0055-z
Zitzmann, N., Mehta, A. S., Carrouée, S., Butters, T. D., Platt, F. M., McCauley, J., et al. (1999). Imino sugars inhibit the formation and secretion of bovine viral diarrhea virus, a pestivirus model of hepatitis C virus: implications for the development of broad spectrum anti-hepatitis virus agents. Proc. Natl. Acad. Sci. 96, 11878–11882. doi: 10.1073/pnas.96.21.1187
Keywords: α-glucosidase inhibitor, α-amylase inhibitor, microbes, endophytes, marine microorganism, medical application
Citation: Wang X, Li J, Shang J, Bai J, Wu K, Liu J, Yang Z, Ou H and Shao L (2022) Metabolites extracted from microorganisms as potential inhibitors of glycosidases (α-glucosidase and α-amylase): A review. Front. Microbiol. 13:1050869. doi: 10.3389/fmicb.2022.1050869
Edited by:
Xue Qiao, Peking University, ChinaReviewed by:
Wensheng Qin, Lakehead University, CanadaMin Wang, Stanford University, United States
Jun Liu, Center of Advanced European Studies and Research (CAESAR), Germany
Copyright © 2022 Wang, Li, Shang, Bai, Wu, Liu, Yang, Ou and Shao. This is an open-access article distributed under the terms of the Creative Commons Attribution License (CC BY). The use, distribution or reproduction in other forums is permitted, provided the original author(s) and the copyright owner(s) are credited and that the original publication in this journal is cited, in accordance with accepted academic practice. No use, distribution or reproduction is permitted which does not comply with these terms.
*Correspondence: Lei Shao, ZmVpaHVzbEAxNjMuY29t; Hao Ou, NzkxNTg5MkBxcS5jb20=