- 1Department of Pharmaceutical Science and Health, Montepríncipe Campus, CEU San Pablo University, Madrid, Spain
- 2Medicine Faculty, Montepríncipe Campus, CEU San Pablo University, Madrid, Spain
Introduction: The overexploitation of natural ecosystems and the evolution of climate change currently force us to design new strategies for more sustainable agronomic uses. The recovery of plant residues, as an alternative to agrochemicals, can help alleviate these problems, for example, through its use for the synthesis of biofertilizers. In this work, the effect of the organic fertilizer matrix ORGAON® from the valorization of horticultural waste is tested, to which two strains of bacteria (and their consortium) are added (SAICEU11T identified as Bacillus pretiosus and SAICEU22T identified as Pseudomonas agronomica), selected for their demonstrated ability to promote plant growth (PGPB), on the lupine forage plant (Lupinus albus).
Methods: For the synthesis of the biofertilizer, both strains were added to the ORGAON® organic matrix separately, until reaching a final optical density (OD) of 0.5 McFarland in each case in the irrigation matrix. As a control, sterile ORGAON® (ORGAON®st) was used, also supplemented with the PGPB strains and a chemical fertilizer widely used in agronomy (Chem-F). With these treatments, a 6-week experiment was started under controlled laboratory conditions and on agricultural substrate, to recreate field conditions as accurately as possible. All the tests were carried out with 9 repetitions and 3 replicates of each treatment. After harvest, the improvements on the following biometric variables were studied for each treatment: total weight (Weight_T, g), shoot weight (Weight_S, g), root weight (Weight_R, g), number of leaves (Leaves, No.), shoot length (Length_S), root length (Length_R) and number of secondary roots (Roots, No.). Likewise, the identification of the tested strains and their description as new species was carried out. For this, they were studied from the phenotypic point of view (Transmission electron microscopy (TEM), metabolic profile, PGP activities, fatty acid profile and Matrix-assisted laser desorption/ionization time-of-flight (MALDI-TOF)) and genotypic (sequencing of the main housekeeping genes and sequencing of the whole genome, genomic characteristics (dDDH and ANI) and phylogenetic analysis).
Results and discussion: After the statistical analysis of the results, it is shown that the individual addition of both strains on the ORGAON® and ORGAON®st organic matrix improve certain biometric variables. In the case of the SAICEU11T (Bacillus pretiosus) strain, the variables root weight (Weight_R, g), total weight (Weight_T, g) and length of the plant, and number of secondary roots (Roots, No.) significantly improve, while in the case of the strain SAICEU22T (Pseudmonas agronomica), a significant improvement of root length (Length_R) and number of secondary roots (Roots, No.) is demonstrated. On the other hand, the genotaxonomic analysis showed that both species have not been described to date. The identification based on the main housekeeping genes, show that for the Bacillus strain (SAICEU11T) the sequence similarity of the 16S rRNA was 100%, gyrB 92.69%, rpoB 97.70% and rpoD 94.67%. For the Pseudomonas strain (SAICEU22T) the results were 100% for 16S rRNA, 98.43% for rpoD and 96.94% for gyrB. However, in both cases, the dDDH and ANI values, as well as the phylogenetic analysis, show that both species are below the species threshold, which would support the hypothesis that both are new species, in line with the chemotaxonomic results obtained by MALDI-TOF spectrometry and fatty acid profile. To verify the biosafety in their handling and release into the natural environment, we have ruled out the presence of genes that encode virulence factors or resistance to antibiotics, concluding that they are suitable for use in the field to improve the yield of crop plants. Type strains are SAICEU11T (= DSM 114702T = CECT30674T) for Bacillus pretiosus and SAICEU22T (= DSM 114959T = CECT30673T) for Pseudomonas agronomicae.
1. Introduction
The term “land resources” includes the physical, biotic, environmental, infrastructure and socio-economic components of a natural land unit, which includes the resource of surface fresh water, and close to the surface, essential for all human activity, including agricultural uses. Thus, the interaction between the components of environmental resources is vital for the determination of the productivity and sustainability of agroecosystems (Dubois, 2011). Unfortunately, the resilience of the system to resist and adapt to natural (such as climatology, availability of water resources) and human-induced (pollution, overexploitation, land uses and management) changes and fluctuations it fails to alleviate fatigue and impoverishment of soil and other natural resources. Thus, abiotic stress can cause the components of an ecosystem, such as the proportion of nutrients or microorganisms that live in it, to be affected (Fiodor et al., 2021). In this sense, the increase in the demand for food induces the use of a greater number of chemical fertilizers, which increase prices, overexploit resources, increase the pollution of natural environments and exacerbate the problem of soil impoverishment (Kumar et al., 2021). For these reasons, there is a scientific-technical interest in developing biotechnological tools that can contribute to alleviating this scarcity and supplies without impoverishing soils or negatively affecting ecosystems.
The recovery of agricultural plant residues to generate liquid organic fertilizers can offer an alternative to the abuse of chemical fertilizers (Witzgall et al., 2021). However, the complexity of the biomolecules that constitute them requires the participation of microorganisms for their transformation into simpler molecules, facilitating their absorption by plants. In this sense, the addition of plant growth-promoting bacteria (PGPB) strains can indirectly favor the transformation of organic matter favoring its root absorption (Katsenios et al., 2022). Likewise, its direct action in stimulating plant growth can effectively contribute to the improvement of agricultural production. Both effects seek to optimize the agronomic use of soils, reduce the demand for water and its corresponding abiotic pressure on aquifers, thus avoiding their contamination and minimizing their overexploitation (Lara Mantilla et al., 2011; Hakim et al., 2021).
Numerous potentially PGPB species are known in the Bacillus and Pseudomonas genera, especially for the great diversity of species that both possess. Bacillus genus was first described by Smith (1957). In turn, the Pseudomonas genus was first described by Migula (1894). The classification of both genera is detailed in the 2nd Edition of Bergey’s Manual of Systematic Bacteriology (Imhoff et al., 2005). The phylogeny of both genera is not entirely clear (Patel and Gupta, 2020; Lalucat et al., 2021) and its taxonomic ordering is being debated (La Duc et al., 2004; Torres Manno et al., 2020; Lalucat et al., 2021). The use of the housekeeping 16S rRNA gene for the identification of strains is not enough, given that strains with very high homology percentages are known with respect to species with which they can differ greatly in their phenotype and even gene content (Gomila et al., 2015; Dunlap, 2019). For this reason, in order to obtain a more robust phylogeny, analyses of a greater number of additional housekeeping genes have recently been incorporated, among which are gyrB, rpoB and rpoD in the case of the genus Bacillus (Yamada et al., 1999; La Duc et al., 2004), and gyrB and rpoD for the genus Pseudomonas (Yamada et al., 1999). However, the rise of Next Generation Sequencing (NGS), the precision and speed with which complete genomes are obtained, makes it necessary to provide more information to taxonomically classify species (Young and Gillung, 2020). The genomotaxonomy of new species includes the calculation of the whole genome DNA–DNA hybridization (dDDH) with evolutionarily close species, the average value of nucleotides (ANI) and the entire genome sequencing (Volpiano et al., 2021).
Lupinus albus is a fodder plant native to the Mediterranean area commonly used as food, due to its easy digestibility and high protein content (Hufnagel et al., 2020). Currently, the demand for vegetables with high protein content is increasing. A good example of this is lupine (Lupinus albus; Alkemade et al., 2021), an easy to grow plant with a root system that allows its growth in unfavorable environments and impoverished soils (Hufnagel et al., 2020). For these reasons, this species has been widely studied and is commonly used in biological tests. In the present work the effect of ORGAON® is tested, an organic fertilizer whose raw material is the recovered plant residues originating from horticultural plantations. Likewise, the collaborative effect that the PGPB SAICEU11T and SAICEU22T included in the matrix of said fertilizer exert on L. albus plants is tested.
Based on the phenotypic and genotypic analysis SAICEU11T and SAICEU22T are proposed as new species belonging to the Bacillus and Pseudomonas genera, respectively. Its genomic analysis allows us to verify that both are harmless to both the environment and living beings. Due to its innocuousness and potential PGP, biological tests were carried out on Lupinus albus var. Dorado irrigated with a matrix of organic fertilizer ORGAON® in comparison with a traditional chemical fertilizer, both supplemented with the PGPB strains.
2. Materials and methods
2.1. Isolates
2.1.1. Permission to reuse and copyright
SAICEU11T and SAICEU22T strains were isolated from the Medicago sativa rhizosphere grown mercury contaminated soils of the Almadén Mining District (Ciudad Real, Spain; Robas et al., 2021).
2.1.2. Plant growth promotion activities (PGP) characterization
To determine the in vitro production capacity of auxins (IAA), a colorimetric technique was used with the Van Urk Salkowski reagent, using the method described by Ehmann (1977). Bacteria were grown in LB medium (Texas, United States) at 28°C for 4 days without agitation and in darkness. The liquid medium was centrifuged after incubation, obtaining the supernatant. Then, 1 ml of it was mixed with 2 ml of Van Urk Salkowski reagent (2% FeCl3 in 35% of HClO4 solution) and kept in darkness. Optical density (OD) was measured at 530 nm after 30 min and 120 min. The results were quantified in ppm (g mL−1). Glick (1995) protocol was followed to differentiate the degradation of 1-aminocyclopropane-1-carboxylic acid (ACC) by the action of the enzyme ACC deaminase (ACCd) from bacteria having the ability to fix nitrogen. The culture medium contained 1.8% Bacto-Agar (Difco Laboratories, Detroit, United States), which has low nitrogen content, supplemented with ACC (30 mmol L−1). The Petri plates (90 × 14 mm) were then inoculated and cultured for 3 days at 28°C, checking the growth daily. The results were evaluated qualitatively (presence/absence of ACCd enzyme). Siderophore production was quantified using the Chrome Azurol S (CAS) agar, described by Alexander and Zuberer (1991). The interpretation was based on the quantitative analysis of the production of siderophores, manifested by the appearance of a halo around the bacterial colonies after 72 h of incubation at 28°C. The ability to solubilize phosphates was tested following the protocol described by De Freitas et al. (1997). Tricalcium-phosphate agar medium (TPM) (Nautiyal, 1999) was used, with a final pH adjusted to 7 with 1 mole L−1 HCl. After inoculation, plates were incubated at 28°C for 72 h. The inorganic phosphates solubilizer colonies showed distinct clarification halos that were evaluated qualitatively (presence/ absence). All PGPB activities were analyzed in triplicate.
2.2. Biological assays for promoting plant growth with ORGAON® biofertigation; an agriculture residue
2.2.1. General cultivation conditions of the two strains
The strains were obtained from the rhizosphere of Medicago sativa, from soils contaminated with mercury (Hg) from the mining district of Almadén (Ciudad Real, Spain) following the protocol described in Robas et al. (2021). Briefly, for their isolation, an extract of the rhizospheric soil was made and they were classified as mercuritolerant following the criteria established by Mathema et al. (2011). To measure its maximum bactericidal concentration (MBC) to Hg, the agar medium for standard methods (SMA, Pronadisa®, Madrid, Spain) supplemented with different concentrations of HgCl2 (400, 350, 200, 175, 150, 100, 87.5, 75, 50, 43.75, and 25 μg mL−1).
2.2.2. ORGAON® As recovered waste
ORGAON® is an organic fertilizer that comes from plant waste recovered by the company Biaqui S.L. (Almería, Spain). The agricultural plant remains are pressed and the liquid fraction constitutes a valuable extract that, after its transformation, is recovered as organic plant-based fertilizer. It is, therefore, a very valuable raw material, with a high content of complex organic compounds that must be transformed into inorganic forms for plant assimilation. Its enrichment with the SAICEU11T and SAICEU22T PGPB individually allows the elaboration of an organic biofertilizer, environmentally friendly. The physicochemical characteristics of ORGAON® are described in Robas Mora et al. (2022).
To carry out the biological assay, ORGAON® fertilizer was also tested sterilized (ORGAON®st) in parallel, by exposing a liquid sheet (2 mm) to UV light (265 nm) for 30 min.
For producing the biofertilizer, the inoculum of the SAICEU11T strain and SAICEU22T strain (optical density (OD) 0.5 McFarland) were added on this matrix, separately. To do this, 100 ml of a 5.0 McFarland suspension of SAICEU11T and SAICEU22T were added to 1 l of the 1/500 ORGAON®/ORGAON®st dilution, in each case and separately (Robas Mora et al., 2022). The pure cultures of the SAICEU11T and SAICEU22T strains were kept in cryo pearls at −80°C, prior to their use. They were recovered in SMA (Pronadisa®, Madrid, Spain), incubation 24 at 37°C, coinciding with the stationary phase of the culture. Subsequently, it was verified by Gram stain that the cultures were maintained in pure culture.
2.2.3. Biological assay
Pre-germinated Lupinus albus var. Dorado seeds, supplied by the Institute of Agricultural Research Finca La Orden-Valdesequera (Badajoz, Spain), were used. Prior to the growth assay, seeds were superficially sterilized by a 30 s ethanol washing (70% v/v). After that time, it was washed with plenty of sterile mineral water. Pre-germination was carried out on sterilized vermiculite substrate, at field capacity (at saturation, moment prior to the drainage of water), in PVC trays that were kept in darkness and stable room temperature (20°C ± 2°C) for 96 h until the appearance of a visible emerged radicle of 2.0 ± 0.2 cm. The agricultural soil was obtained from the plot where field trials are currently being carried out, located in Villanueva de San Mancio (GPS coordinates 21°92′N 5°03′O, Valladolid, Spain). It was sieved (0.5 cm) and the resulting product was mixed with sterile river sand 50/50 (w/w) to standardize granulometry in all treatments and replicates. Seedbeds of 10 cm × 8 cm were used on trays of 40 cm × 35 cm (three replicates per treatment and per tray) each with nine Lupinus albus pre-germinated seeds, per treatment (Table 1).
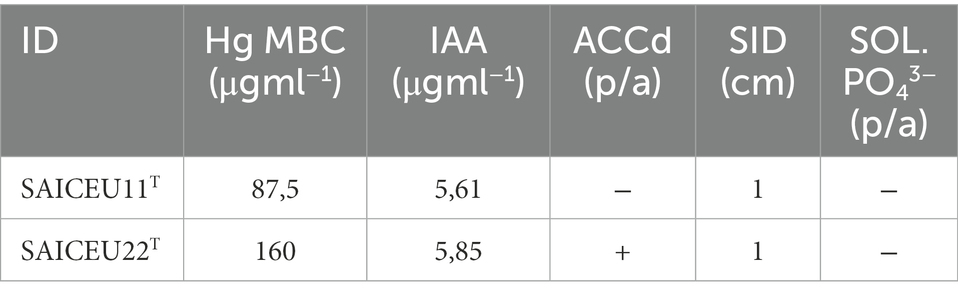
Table 1. Hg MBC: Hg maximum bactericidal concentration; IAA: 3-indoleacetic acid production; ACCd: 1-aminocyclopropane-1-carboxylic acid deaminase production; SID: siderophore production; SUN. PO43−: phosphate solubilizing capacity.
The irrigation matrix was ORGAON® (organic fertilizer), at an optimal experimental concentration of 1/500 (VORGAON/VH2O). Aditionally, ORGAON®st fertilizer was tested. The tests were carried out under controlled laboratory conditions (18°C ± 3°C, natural light contribution and relative humidity of 30%) and two weekly irrigations (experimental average volumes of 50 ml) with the biofertilizer (ORGAON®/ORGAON®st, supplemented with the SAICEU11T strain and ORGAON®/ORGAON®st, supplemented with the SAICEU22T strain). Controls were done with a traditional chemical fertilizer (Chem-F) or the organic fertilizer ORGAON®/ORGAON®st, in all cases without supplementation with the SAICEU11T strain or SAICEU22T strain. Chem-F consisted in a fertilizer low in chloride with the following formulation: (K2HPO4; 5%); (K 2SO4; 14%); (MgSO₄; 2.5%).
For the dilutions of the ORGAON® and the chemical fertilizer Chem_F, mineral water was used, with the composition detailed in Table 2.
The harvest was carried out after 6 weeks and involved the extraction of the shoot and root parts of each plant. The root part was washed with distilled water to remove all traces of substrate.
Nine pregerminated seeds (repetitions) were used for each treatment. Each fertigation treatment was tested in triplicate (replicates): ORGAON®, ORGAON®st and Chem_F added with SAICEU11T, SAICEU22T, the consortium of both or the control without addition, making a total of 27 pots per strain/ consortium, according to the experimental design shown in Table 3.
The following biometric parameters were analyzed: total weight (Weight_T, g), shoot weight (Weight_S, g), root weight (Weight_R, g), number of leaves (Leaves, No.), shoot length (Length_S), root length (Length_R) and number of secondary roots (Roots, No.). The two bacteria isolates were subjected to mutual compatibility test by cross streak method in SMA (Pronadisa®, Madrid, Spain).
2.2.4. Statistical analysis
To evaluate the effects of treatment on all biometric variables, for each irrigation matrix, an analysis of variance (one-way ANOVA) was performed. When significant differences appeared (value of p < 0.05), a Duncan post-hoc analysis was performed to identify those fertigation treatments that explain the difference between bacterial treatments. The SPSS v.27.0 program (IBM Corp, Armonk, NY, United States) was used.
2.3. New species/strains characterization and description
2.3.1. Transmission electron microscopy (TEM)
To determine the size and shape of the analyzed strains, the Prism E Scanning Electron Microscope (SEM) (Thermo Fisher Scientific Inc., Walthman, USA) was used. The culture was observed in suspension. A drop of Formvar on 200 mesh Cu TEM grids was used as a sample holder in the grid for TEM microscopy. The measurement conditions were: 10 mm working distance; 24 pA electron current; electron acceleration of 30 kV and 2-point size, pressure of 375 Pa, at 4°C and a humidity of 50%. The electron microscopy images were obtained by the research support service (SAI) of “X-ray diffraction and scanning electron microscopy” (SAI-DRX-MEB) of the CEU-San Pablo University (Madrid, Spain).
2.3.2. Biochemical tests
Oxi/Ferm Pluri Test® (Liofilchem, Italy) was used. The automatic characterization was then carried out using the VITEK®-2 GN identification cards (bioMérieux, Marcy-l’Étoile, France). The motility of the bacterium was tested in Motility Test Agar (Liofilchem, Italy). Antimicrobial sensitivity was determined using E-test in Müller Hinton agar (Pronadisa® Madrid, Spain) using different antibiotics for each strain. For SAICEU11T strain we used cefepime and sulfamethoxazole and trimethoprim (bioMérieux, Marcy-l’Étoile, France); amoxicillin, amoxicillin-clavulanic acid, cefotaxime, cefpirome ciprofloxacin and nalidixic acid (Liofilchem, Italy). For SAICEU22T strain we used piperacillin and piperacillin with tazobactam, cefepime (bioMérieux, Marcy-l’Étoile, France); ceftazidime, imipenem, imipenem with EDTA, amikacin, gentamicin, and ciprofloxacin (Liofilchem, Italy).
Mercury MBC was performed in Müller Hinton agar (Pronadisa®, Madrid, Spain), supplemented with different concentrations of HgCl2: 400, 350, 200, 175, 150, 100, 87.5, 75, 50, 43.75, and 25 μg mL−1. MBC was determined as the lowest concentration of HgCl2 capable of inhibiting > 99.9% of bacterial growth.
2.3.3. Fatty acids profile
Fatty acids spectrum was determined following the protocol recommended by MIDI Microbial Identification System (Sasser, 2001) at Spanish Collection of Type Cultures (CECT, Valencia, Spain). An Agilent 6,850 gas chromatograph was used, with the MIDI Microbial Identification System using the TSBA6 method (MIDI, 1999). For this, Trypticase soy broth (TSBA, Condalab®, Torrejón de Ardoz, Madrid) as culture medium was used, and an incubation temperature of 28°C for 24 h.
2.3.4. Matrix-assisted laser desorption/ionization time-of-flight (MALDI-TOF)
Matrix-Assisted Laser Desorption/Ionization Time-Of-Flight (MALDI-TOF) was used. It was carried out in the Vitek® MS IND system (BioMérieux, Marcy-l’Étoile, France) at the Carlos III Health Institute in Madrid. The slides were inoculated with a sterile loop. 1 l of the matrix solution (Vitek® MS-CHCA: mixture of 3.10 g of 2.5-dihydroxy 36 benzoic acid dissolved in 100 ml of water-ethanol-acetonitrile in 1/1/1 ratio) was added to each well and left to dry at room temperature. The mass spectra were generated with the Axima Assurance system (Shimadzu Corporation, Kyoto, Japan), using the Shimadzu Launchpad software program and the SARAMIS MS-ID v1 database application (AnagnosTee GmbH) for automatic measurement and identification. All strains were analyzed in duplicate. No pre-treatment was used before their inoculation on the slide. High confidence identification was considered when the assessment was equal to or greater than 97%.
2.3.5. Sequencing analysis
For genomic DNA extraction, QIAamp DNA Kit (QIAGEN®, Hilden, Germany) was used. The entire genome was sequenced in the genomics at Carlos III Health Institute (Madrid, Spain). With the obtained data, the de novo assembly and gene annotation was performed, corresponding to the Bacillus and Pseudomonas genus. Sequencing was carried out with the Illumina® platform in paired end format (2 × 300; Illumina®, Inc., San Diego, CA, United States). The quality control analysis was performed with the FastQC program (Andrews, 2010). Adapters were removed from FASTQ files using the CUTADAPT tool (Martin, 2011). The filtering was carried out with the Prinseq platform (Schmieder and Edwards, 2011). In the filtering, in addition to quality, a minimum size of 100 bp was used and those sequences with the presence of more than 5% of Ns were rejected. Neither the first 15 nucleotides nor the last 15 were considered due to low signal quality. The FASTQCollapser tool was used to remove duplicate readings and FASTQIntersect to remove sequences that were not in both readings (forward and reverse) of the file. For the sample de novo assembly the SPAdes assembly software (Bankevich et al., 2012) was used to achieve the scaffolds reconstruction. The metrics of the assemblies were obtained through SeqEditor (Hafez et al., 2021). Genome hybridization and identity percentages of the 16S rRNA, gyrB, rpoB and rpoD genes were analyzed using the basic local alignment search tool BLAST (Altschul et al., 1990).
The 16S rRNA gene was analyzed using the NCBI local alignment tool (BLAST). The search was restricted to Sequences from type material. The SAICEU11T and SAICEU22T strains genomes were analyzed using the TYGS server (type strain genome server; Meier-Kolthoff and Göker, 2019) and they were used as a basis for the realization of the phylogenetic trees. For this, the MASH algorithm was used, which gives an approximation to the possible intergenic relationships. (Ondov et al., 2016). Also, with the JSpeciesWS ANI value. When the software identifies that the species differ in any of the analyzed criteria, it identifies it with different colors in section “species cluster.” After that, dDDH was also performed with TIGS server (Meier-Kolthoff and Göker, 2019).
Several clinically important antimicrobial resistance genes were searched through functional annotation data generated from Rast, Comprehensive Antibiotic Resistance Database (CARD), ARG-ANNOT V4 and ResFinder 4.1 annotation lines.
2.3.6. Gene identification and annotation
The Uniprot platform (The Uniprot Consortium, 2021) and the NCBI database were used. For gene annotation, the Prokka program (rapid prokaryotic genome annotation) was used (Seemann, 2014). To check the presence of virulence genes and pathogenicity factors, the Virulence Factor Database (VDFB:) was used (Yang et al., 2007).
3. Results
3.1. Plant growth-promoting biological assays by biofertirigation
Biological tests were carried out to know the effect of biofertigation on the plant with two compound biofertilizers, one by the SAICEU11T strain and the other by the SAICEU22T strain added on ORGAON® and ORGAON®st (sterile). The consortium composed of both strains was also tested, once it was verified with the compatibility test that both strains do not exert interspecific competition phenomena between them. Its efficacy is compared to the traditional chemical fertilizer (Chem_F). In Table 4 the value of ps of the ANOVA results are shown. What is highlighted are those biometric parameters that underwent some significant variation (value of p < 0.05) with each fertigation condition. Only for these biometric parameters that are dependent on the type of irrigation, a post-hoc analysis (Duncan’s test) is performed to find out which irrigation is the one that justifies such variations. Full results can be found at length in the Supplementary Tables 1A–D.
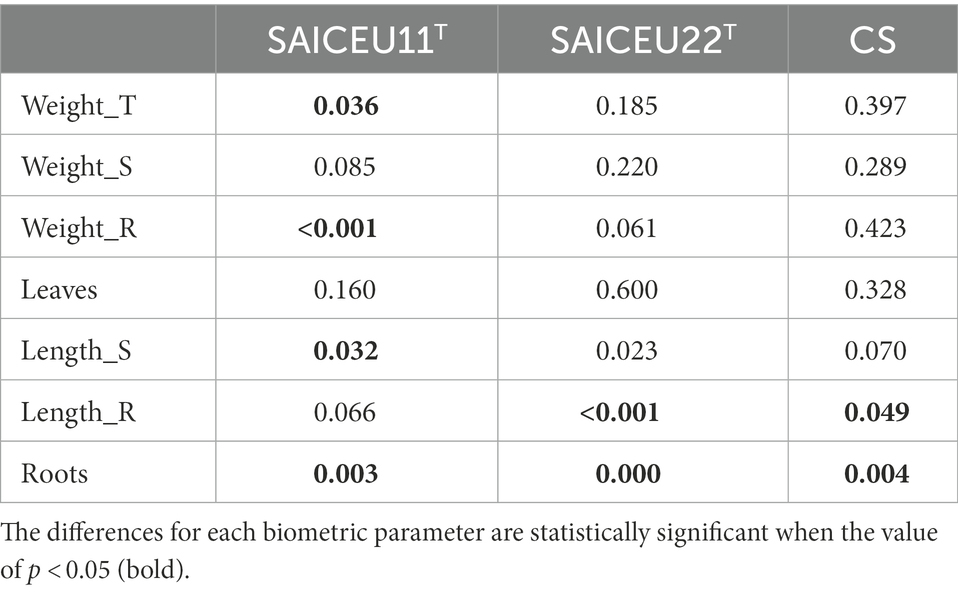
Table 4. Significance of the differences between values (value of p) after the ANOVA analysis, for each bacterial treatment and consortium.
Next, only those biometric parameters that are influenced by the type of fertigation are represented graphically. The post-hoc analysis carried out (Duncan’s test) allows the identification of those biometrical parameters that explain such differences (Figures 1–4).
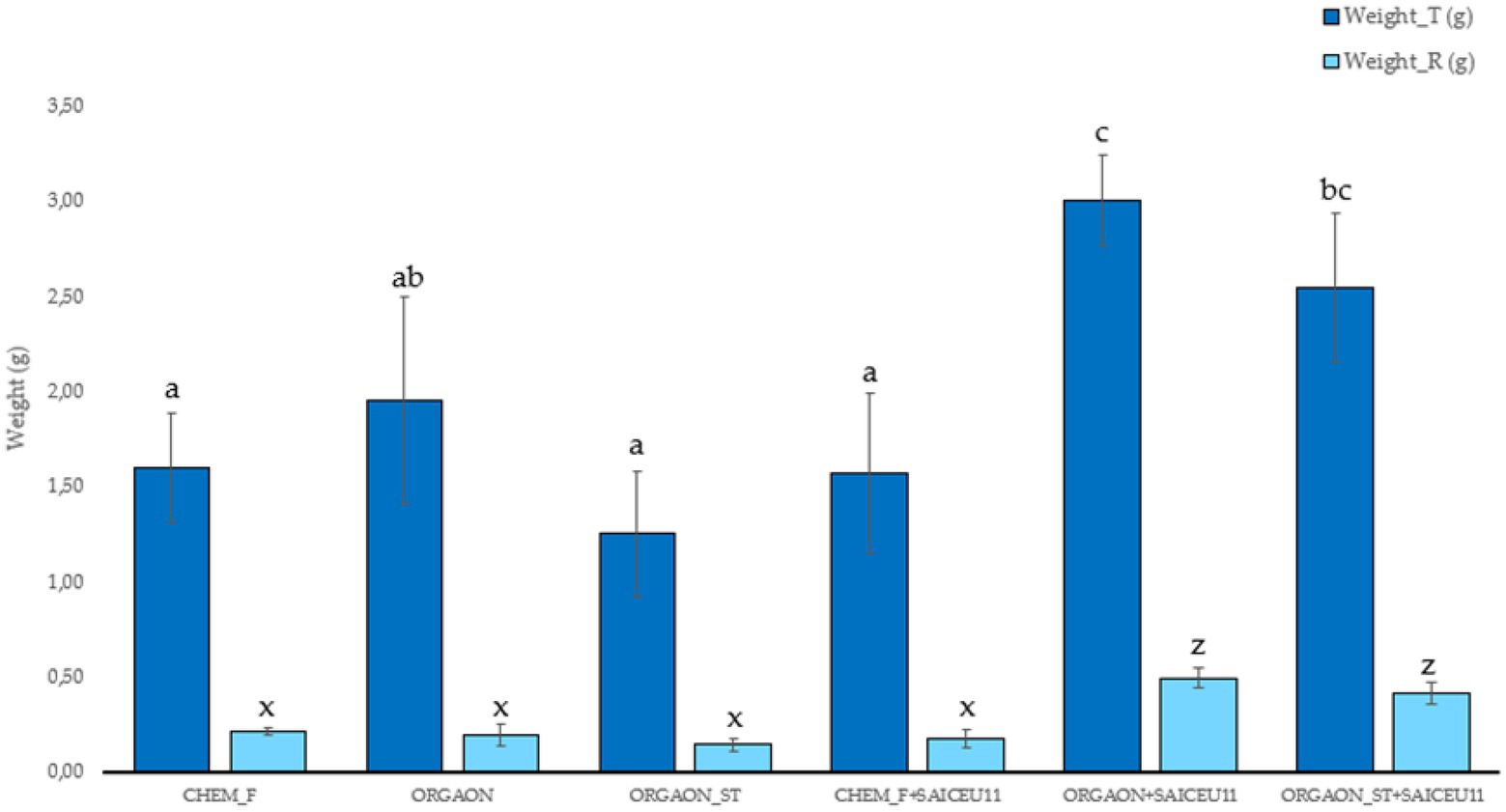
Figure 1. Weight biometrics of lupine plants subjected to different irrigation treatments with biofertilizer with SAICEU11T. Different letters denote statistically significant differences according to Duncan test (p < 0.05) for total plant weight (a, ab, and c) and root weight (x and z).
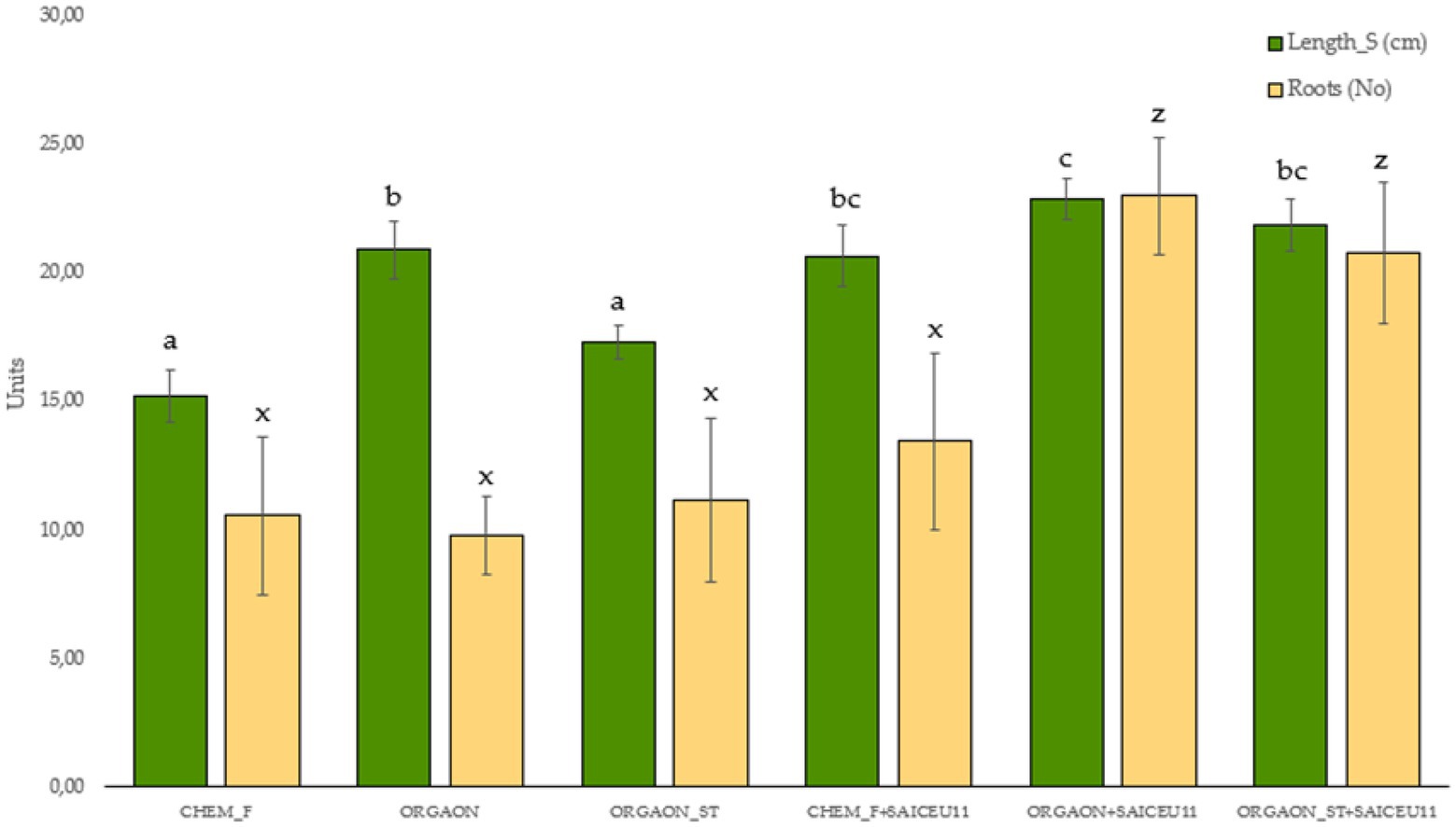
Figure 2. Biometrics of lupine plants subjected to different irrigation treatments with biofertilizer with SAICEU11T. Different letters denote statistically significant differences according to Duncan test (p < 0.05) for shoot length (a, b, bc, and c) and the number of secondary roots (x and z).
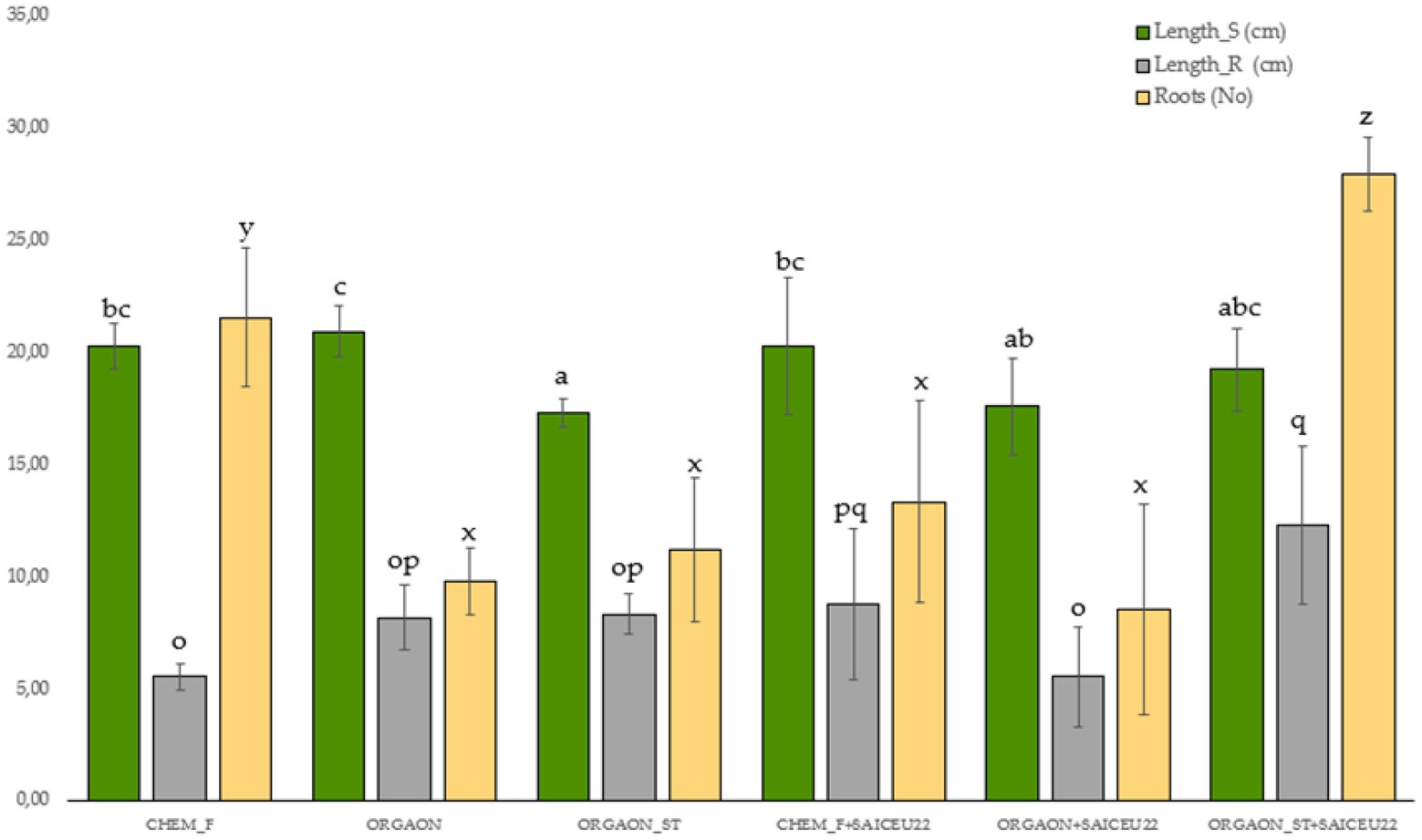
Figure 3. Weight biometrics of lupine plants subjected to different irrigation treatments with biofertilizer with SAICEU22T. Different letters denote statistically significant differences according to Duncan test (p < 0.05) for shoot length (a, ab, abc, bc, and c), the root length (o, op, pq, and q) and the number of secondary roots (x, y, and z).
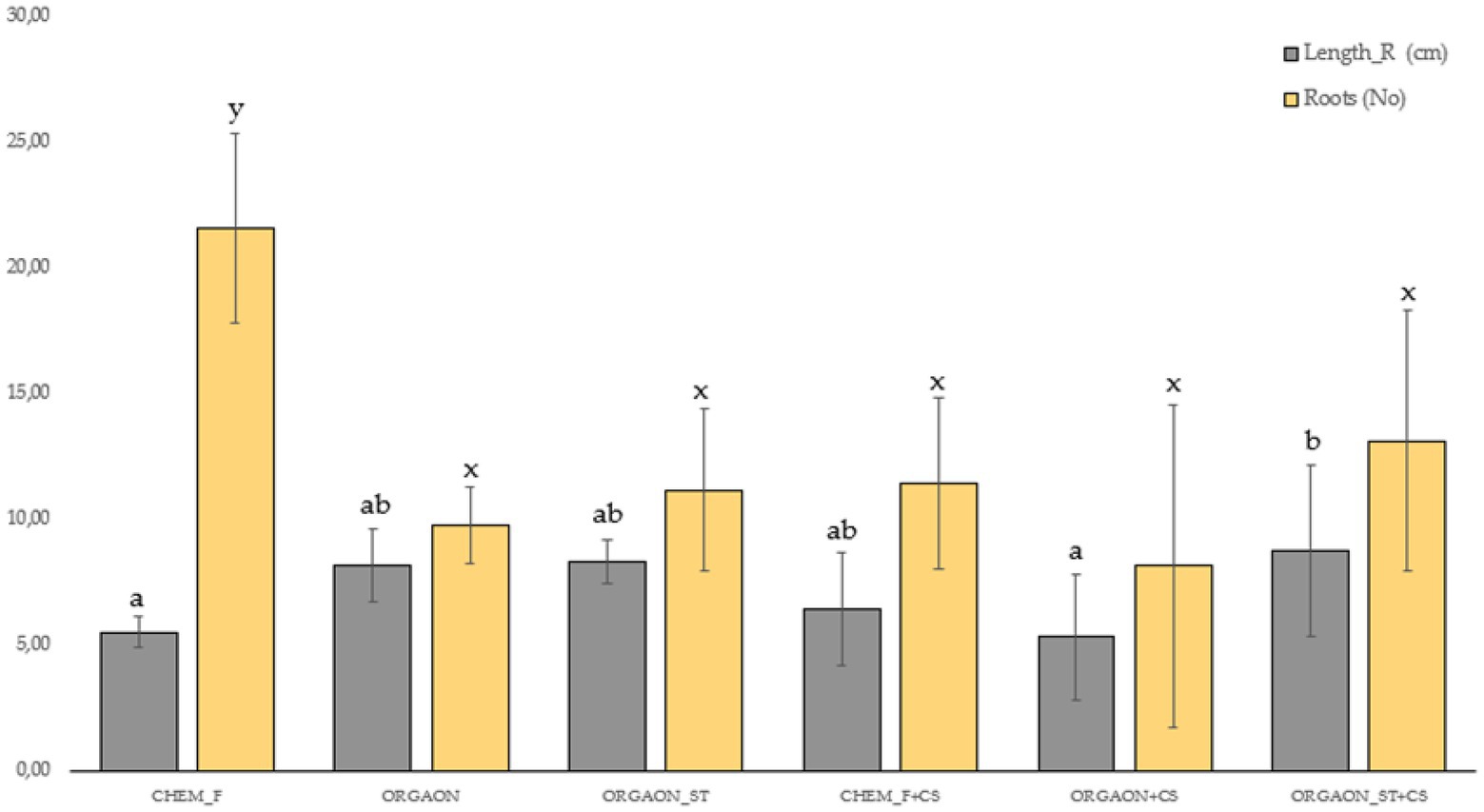
Figure 4. Weight biometrics of lupine plants subjected to different irrigation treatments with biofertilizer with both SAICEU11T and SAICEU22T (CS). Different letters denote statistically significant differences according to Duncan test (p < 0.05) for the root length (a, ab, and b) and the number of secondary roots (x and y).
After the post-hoc analysis with Duncan’s test, it was found that the treatments of ORGAON® and ORGAON®st supplemented with strains SAICEU11T and SAICEU22T showed statistical differences in biometrics, with respect to the plants treated with Chem-F as with fertilizers in the absence of added strains.
The sterilization of the ORGAON® fertilizer carried out to eliminate the native microbiota of the organic recovered residue, allowed to verify the promoting effect of the s strains SAICEU11T and SAICEU22T in the biometric variables (Figures 1–3).
In the compatibility test between SAICEU11T and SAICEU22T strains, no inhibition was observed on the cross point in any of the combinations, which indicate the compatibility among the isolates.
3.2. Species identification
The length of the 16S rRNA gene of the SAICEU11T strain was 1,359 bp; on the other hand, the length of this gene in the SAICEU22T strain was 1,125 bp. The percentages of similarity of the 16S rRNA gene greater than 98.65% were analyzed, calculating the dDDH and ANI values for each of them (Tables 5A,B).
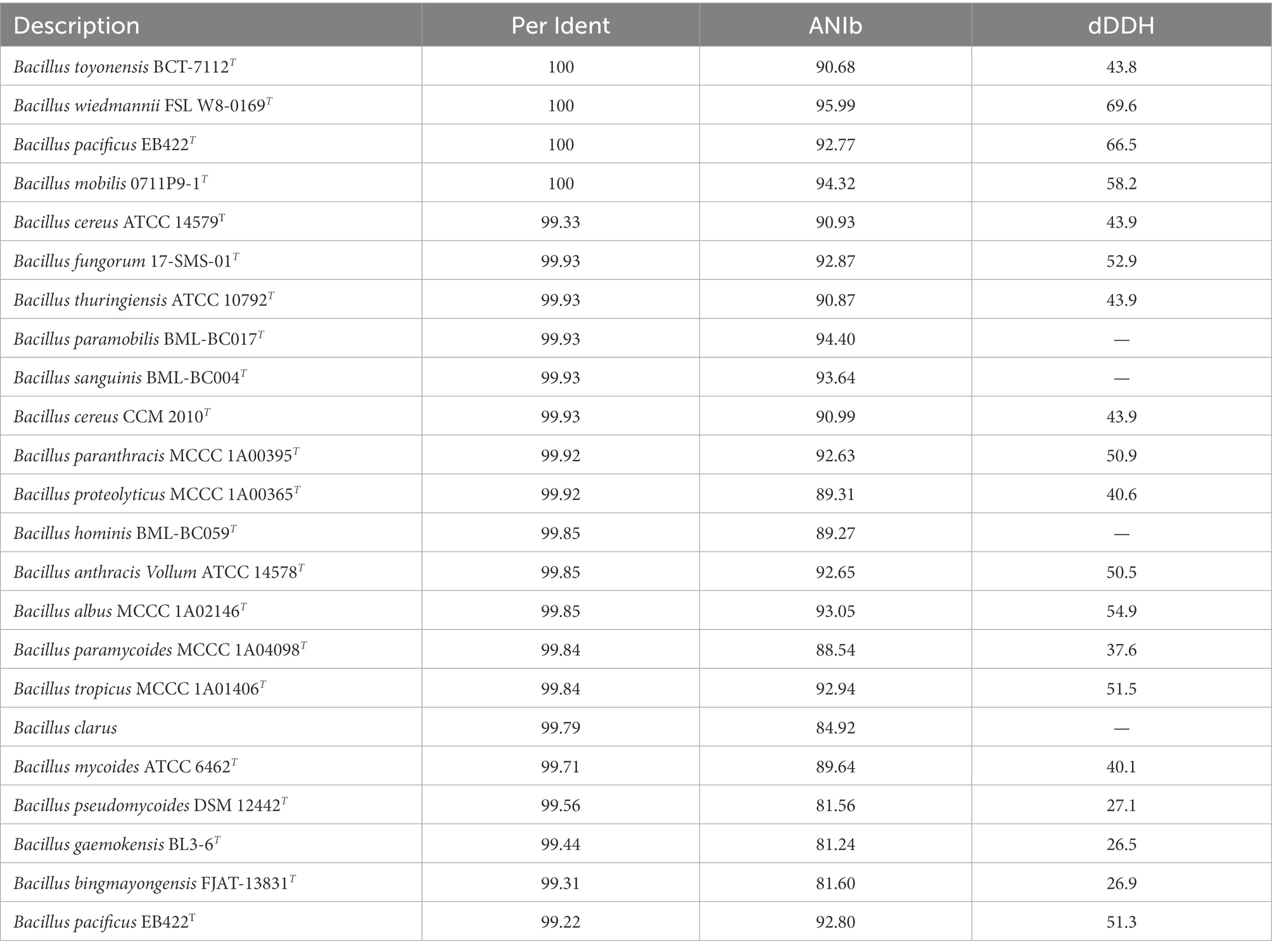
Table 5A. Similarity of the 16S rRNA gene greater than 98.65% of all type strains against SAICEU11T.
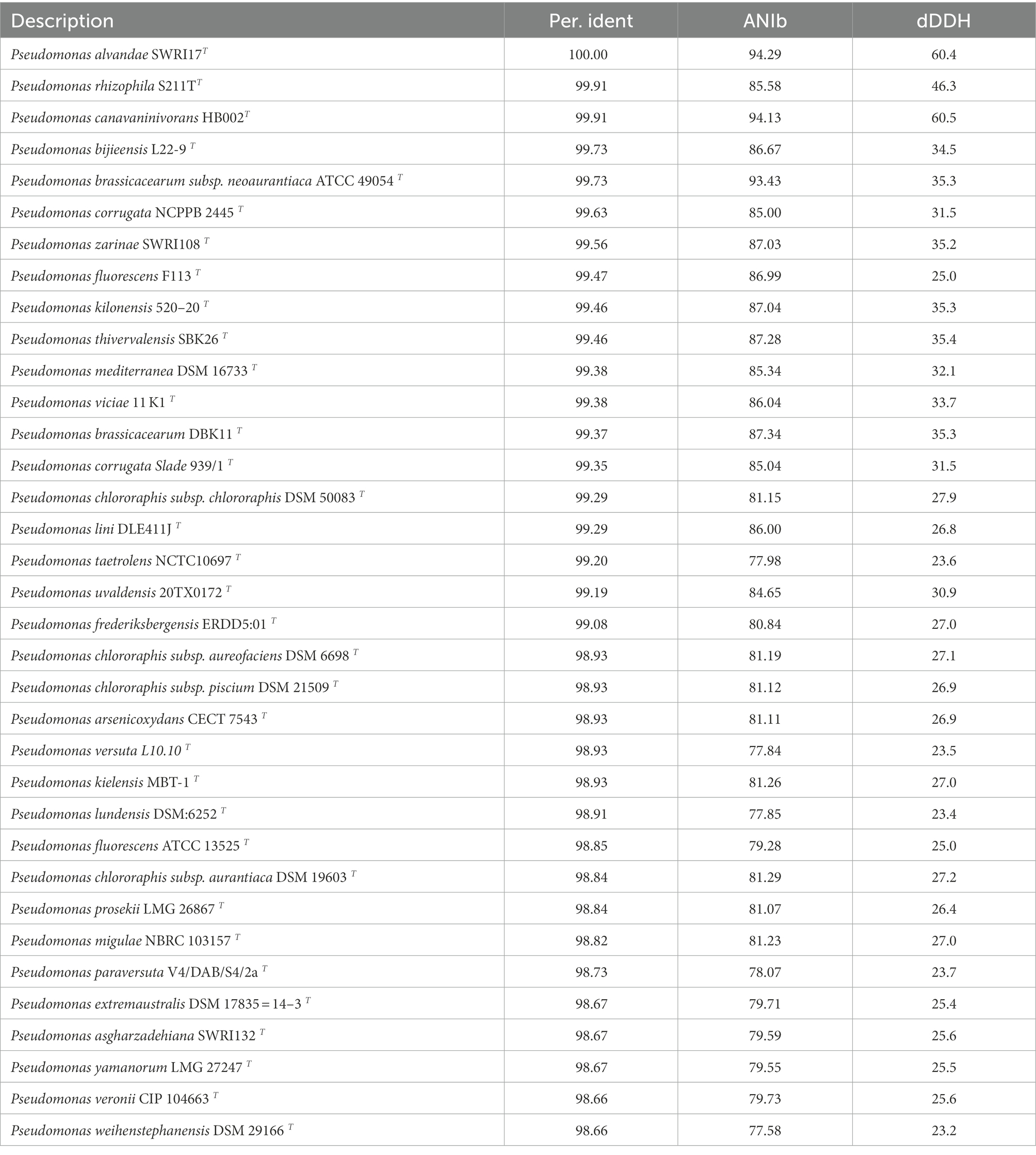
Table 5B. Similarity of the 16S rRNA gene greater than 98.65% of all type strains against SAICEU22T.
The percentage of homology of the sequence of the housekeeping genes gyrB, rpoB and rpoD in SAICEU11T, and gyrB and rpoD in SAICEU22T was obtained. Likewise, the homology values obtained are included in Tables 6A,B.
3.3. Plant growth-promoting characterization
The SAICEU11T strain presented PGP activities such as the production of auxins (concentration of 5.61 ± 5.61 acid 5.61 0.26 μg mL−1), production of the enzyme 1-aminocyclopropane-1-carboxylate deaminase (ACCd). The ability to solubilize phosphates was negative. The complete list of genes involved in promoting plant growth is shown in Supplementary Table 2.
The SAICEU22T strain presented the following PGP activities: production of auxins (concentration of acid-3-indoleacetic acid 5.85 ± 0.12 μg mL−1), production of ACCd and biosynthesis of siderophores (1.00 ± 0.30 cm). The ability to solubilize phosphates was negative. The complete list of genes involved in promoting plant growth is shown in Supplementary Table 3.
3.4. Description and phenotypic and genomic characterization of SAICEU11T
3.4.1. Chemotaxonomic features
The cellular fatty acid profiles (> 1%) of strain SAICEU11T and closely related strains are shown in Table 7. The mayor fatty acids (> 10%) of strain SAICEU11T was iso-C15:0 (30.96%). We find greater similarity with Bacillus wiedmannii FSL W8-0169T whose main fatty acids were iso-C15:0 (27.6%) and iso-C17:0 (10.1%). The major fatty acids of Bacillus pacificus EB422T were C16:0 (19.9%) and iso-C15:0 (13.8%), same as Bacillus mobilis 0711P9-1T with C16:0 (16.7%) and iso-C15:0 (10.8%). The SAICEU11T strain can be differentiated from major and minor fatty acids.
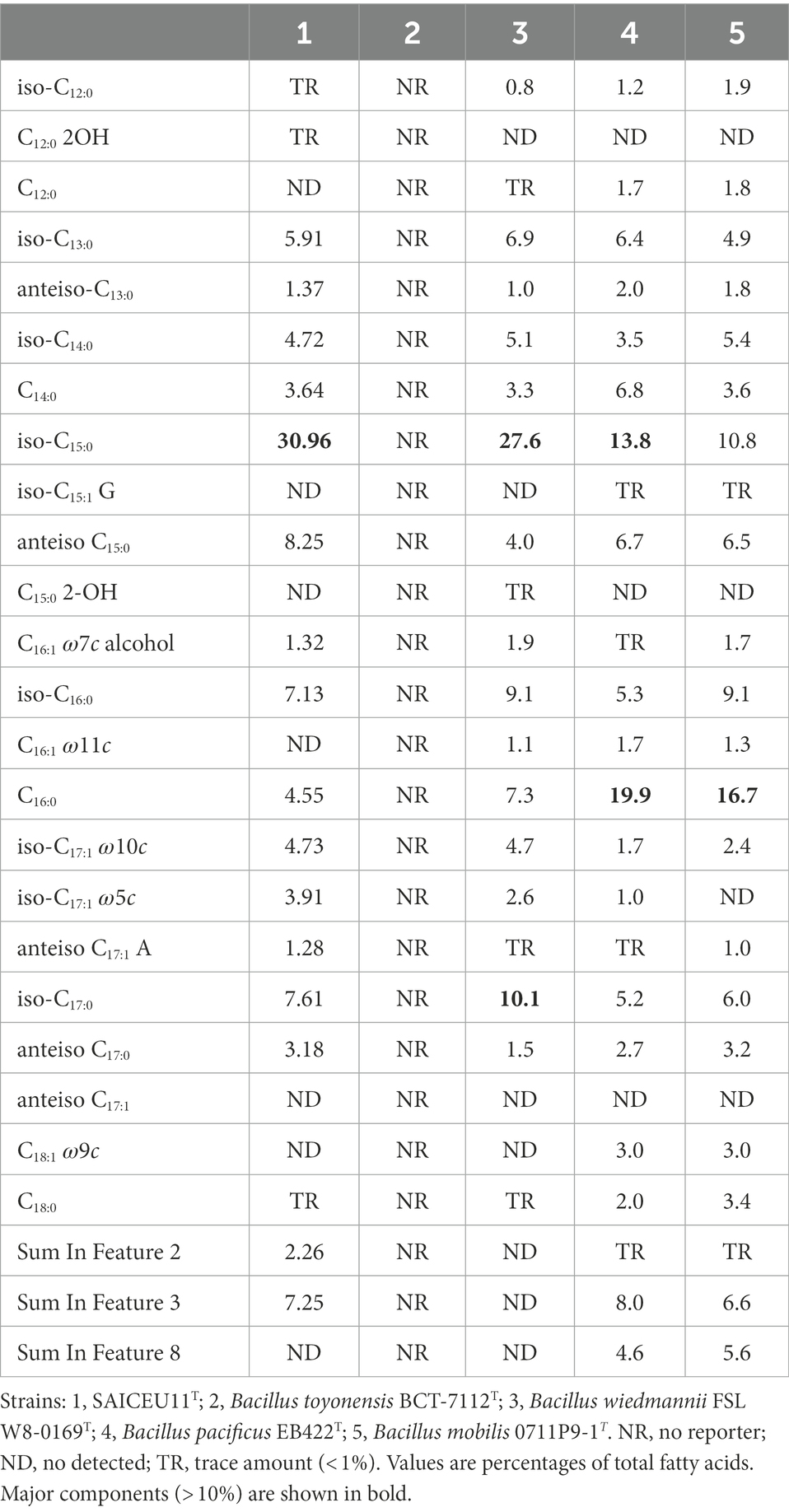
Table 7. Cellular fatty acid profiles (> 1%) of strain SAICEU11T and type strains of closely related species.
3.4.2. Phenotypic characterization
The SAICEU11T strain is a Gram-positive bacillus. In nutrient agar, the colonies are observed round (Ø 0. 6 ± 0.2 cm) and a creamy white color. It is catalase positive. In blood agar, the bacteria behave like beta hemolytic. It has motility. The rest of the phenotypic results and biochemical tests are found in Table 8.
Strains: 1. SAICEU11T; 2 Bacillus wiedmanni FSL W8-01 69T (Miller et al., 2016); 3. Bacillus mobilis MCCC 1 A05 942 (0711P9-1T; Liu et al., 2017). Data for the related strains obtained from indicated respective references/database. +, Positive; −, negative; NA, data not available; M. sativa: Medicago sativa.
The protein profile obtained with the mass spectrometry performed in MALDI-TOF Identifies SAICEU11T within the Bacillus cereus Group (Table 9). The spectrum can be consulted in the Supplementary Figure S1.
The antibiogram obtained shows that the SAICEU11T strain has low MIC (Supplementary Table 4) compared to the most widely used antibiotics against Bacillus spp. This phenotype is compatible with the resistome, which can be consulted at length in the Supplementary Tables 6A,B.
3.4.3. Sequence analysis: ANI and dDDH analysis
The genome size of SAICEU11T was 5,385,342 bp. A total of 90 contigs were found, with the N50 being 170,108 bp in size. The % GC of the strain was 35.30%. The genome was deposited at the NCBI, with the following access numbers (BioProyect PRJNA850797, BioSample SAMN29203362 and GenBank JAOXJG000000000).
The highest dDDH of the SAICEU11T strain was 69.6% versus Bacillus wiedmanni FSL W8-01 69T. Likewise, the ANI value (mean number of nucleotides) compared between Bacillus wiedmanni FSL W8-01 69T and SAICEU11T was 95.99%. Both values were below the recommended cut-off points for the definition of species, set at > 70% (dDDH) and 95–96% (ANI; Baek et al., 2019, Volpiano et al., 2021).
3.4.4. Phylogenetic analysis based on whole genome sequencing (WGS)
A phylogenetic tree was constructed based on the comparison of the complete genome sequences closer to SAICEU11T, available in the TYGS database (Figure 5). To do this, the ten strains with the smallest MASH distance were chosen. In the analysis of the phylogenetic tree of the complete genome of the SAICEU11T strain, it is observed that it is in a cluster shared with Bacillus wiedmannim and Bacillus mobilis.
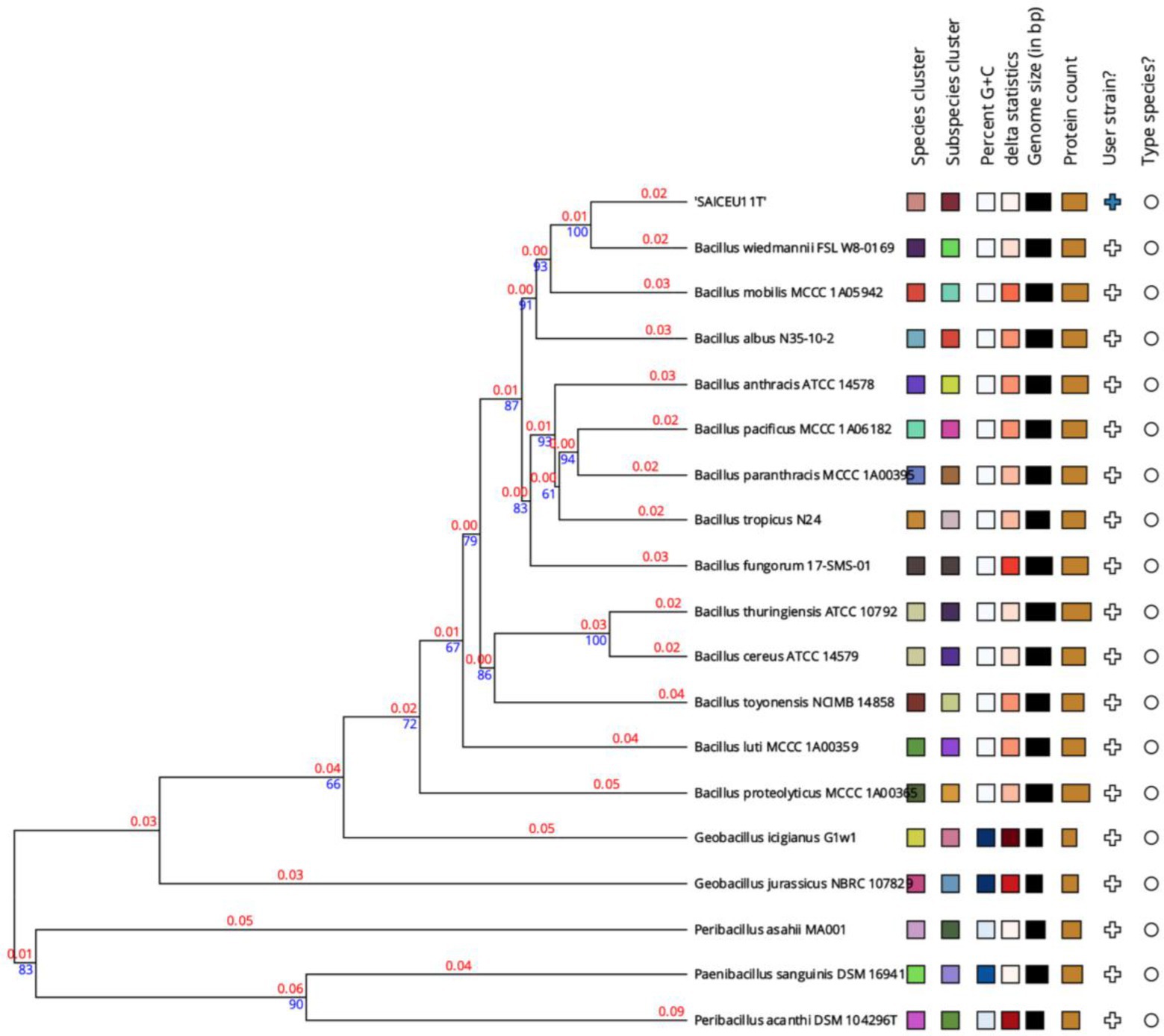
Figure 5. Phylogenetic tree from the complete genome of the species of the genus Bacillus. Numerical values indicate the relative distance between the analyzed species. The branch lengths are scaled in terms of GBDP distance d5 formula. The blue numbers are GBDP pseudo-bootstrap support values > 60% from 100 replications, with an average branch support of 55.2%. The branch length values (in red) represent the evolutionary time between two nodes. Unit: substitutions per sequence site. The tree was rooted at the midpoint (Farris, 1972).
3.5. Description and phenotypic and genomic characterization of SAICEU22T
3.5.1. Chemotaxonomic features
The cellular fatty acid profiles (> 1%) of strain SAICEU22T and closely related strains are shown in Table 10. The mayor fatty acids (> 10%) of strain SAICEU22T were C16:0 (31.53%), Sum In Feature 3 (25.65%) and Sum In Feature 8 (18.59%). We find greater similarity with Bacillus wiedmannii FSL W8-0169T whose main fatty acids were C15:0 iso (27.6%) and iso-C17:0 (10.1%). The major fatty acids of Bacillus pacificus EB422T were C16:0 (19.9%) and iso-C15:0 (13.8%), same as Bacillus mobilis 0711P9-1T with C16:0 (16.7%) and iso-C15:0 (10.8%). No data found for Pseudomonas alvandae SWRI17T and Pseudomonas rhizophila S211TT. In the case of Pseudomonas canavaninivorans HB002T they only refer to the main fatty acids found without percentage, these are C16:0, C16:1 cyclo ω7c and C18:1 ω7c. The same happens with Pseudomonas bijieensis L22-9 T, where they describe the main fatty acids, these are C16:0, Sum In Feature 3 and Sum In Feature 8. With the current data, more similarity would be found with Pseudomonas bijieensis L22-9 T.
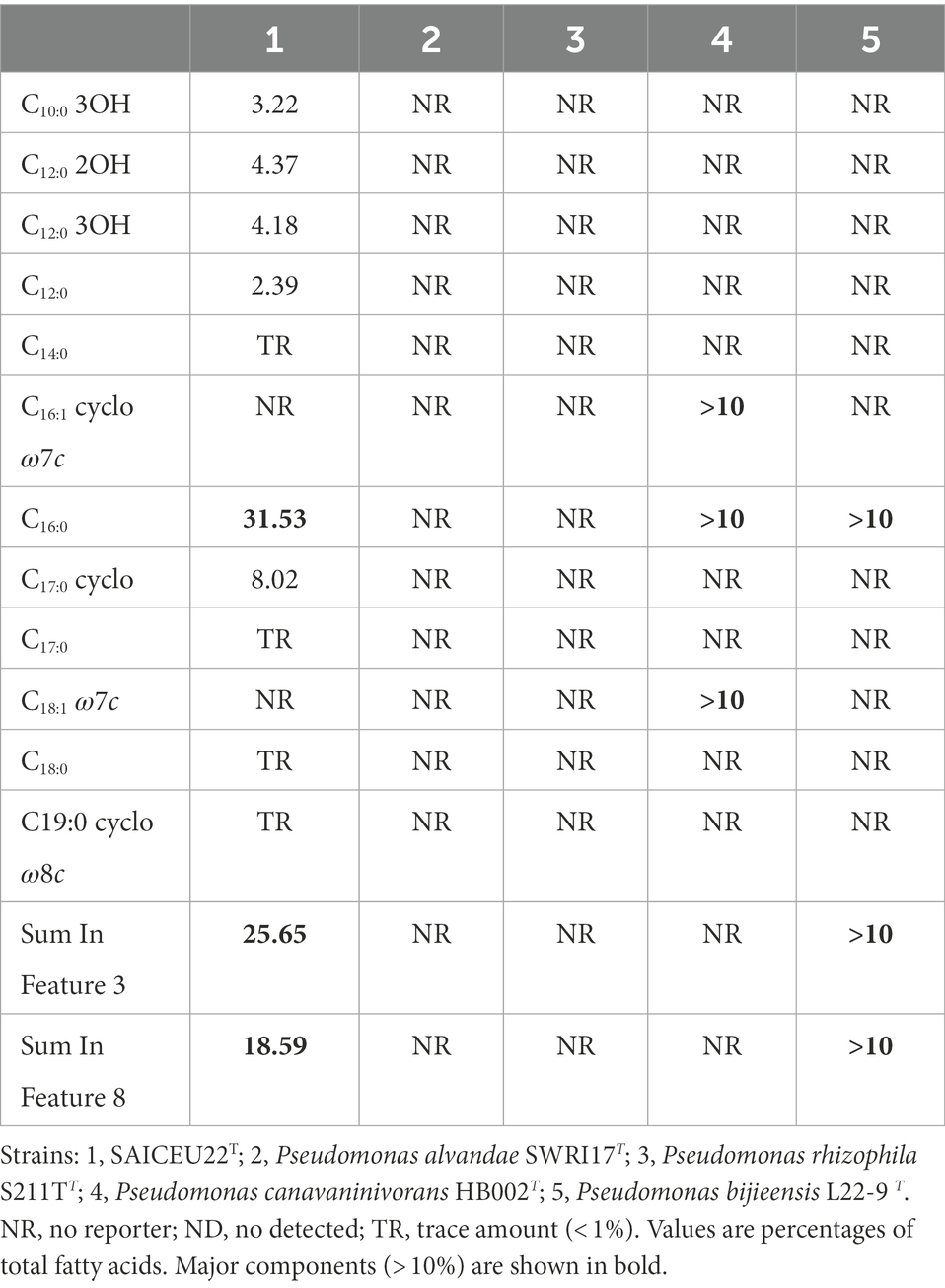
Table 10. Cellular fatty acid profiles (> 1%) of strain SAICEU22T and type strains of closely related species.
3.5.2. Phenotypic characterization
The SAICEU22T strain is a Gram-negative bacillus. In nutritive agar, the colonies are observed round and whitish (Ø 0.4 ± 0.2 cm). It is catalase positive and has no motility. The rest of the phenotypic results and biochemical tests are found in Table 11.
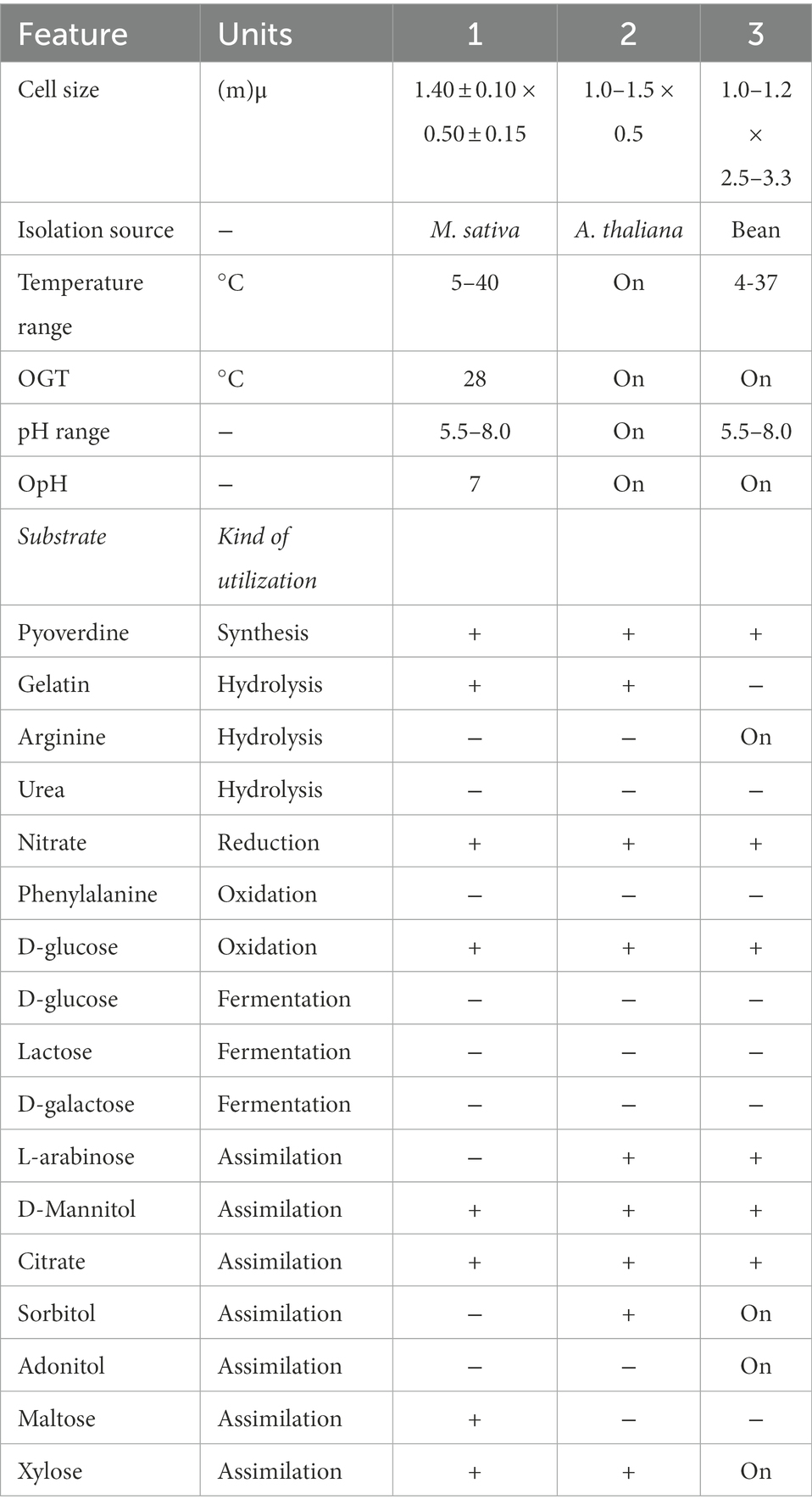
Table 11. Differential characteristic phenotype of Pseudomonas agronomica sp. nov. and closely related Pseudomonas spp.
Strains: 1. SAICEU22T; 2. Pseudomonas thivervalensis DSM13194T (Achouak et al., 2000); 3. Pseudomonas canavaninivorans HB002T (Hauth et al., 2022). Data for the related strains obtained from indicated respective references/database. +, Positive; −, negative; NA, data not available; M. sativa: Medicago sativa; A. thaliana: Arabidopsis thaliana.
The protein profile obtained with the mass spectrometry performed in MALDI-TOF did not correspond to any of the currently known species of the genus Pseudomonas (Table 12). The spectrum can be consulted in the Supplementary Figure 1.
The obtained antibiogram shows that the SAICEU22T strain presents low MIC (Supplementary Table 5) compared to the antibiotics of more widespread use against Pseudomonas spp. This phenotype is also compatible with the resistome, which can be consulted at length in the Supplementary Tables 7A,B.
3.5.3. Sequence analysis: ANI and dDDH analysis
The genome size of SAICEU22T was 6,158,284 bp. A total of 1,203 contigs were found, with the N50 being a size of 361,685 bp. The % GC of the strain was 61.10%. The genome was deposited at the NCBI, with the following access numbers (BioProject PRJNA853909, BioSample SAMN30035438, GenBank JAOSHO000000000).
For SAICEU22T strain, the highest dDDH rate was 35.40% compared to Pseudomonas thivervalensis 21629T. Likewise, the ANI value compared between Pseudomonas thivervalensis 21629T and SAICEU22T was 87.95%. Both values were below the recommended cut-off points for the definition of species, set at 70% (dDDH) and 95–96 (ANI; Baek et al., 2019; Volpiano et al., 2021).
3.5.4. Phylogenetic analysis based on whole genome sequencing (WGS)
A phylogenetic tree was constructed based on the comparison of the complete genome sequences closer to SAICEU22T, available in the TYGS database (Figure 6). To do this, the ten strains with the smallest MASH distance were chosen. It is observed that SAICEU22T is segregated from the rest of the species belonging to the Pseudomonas genus.
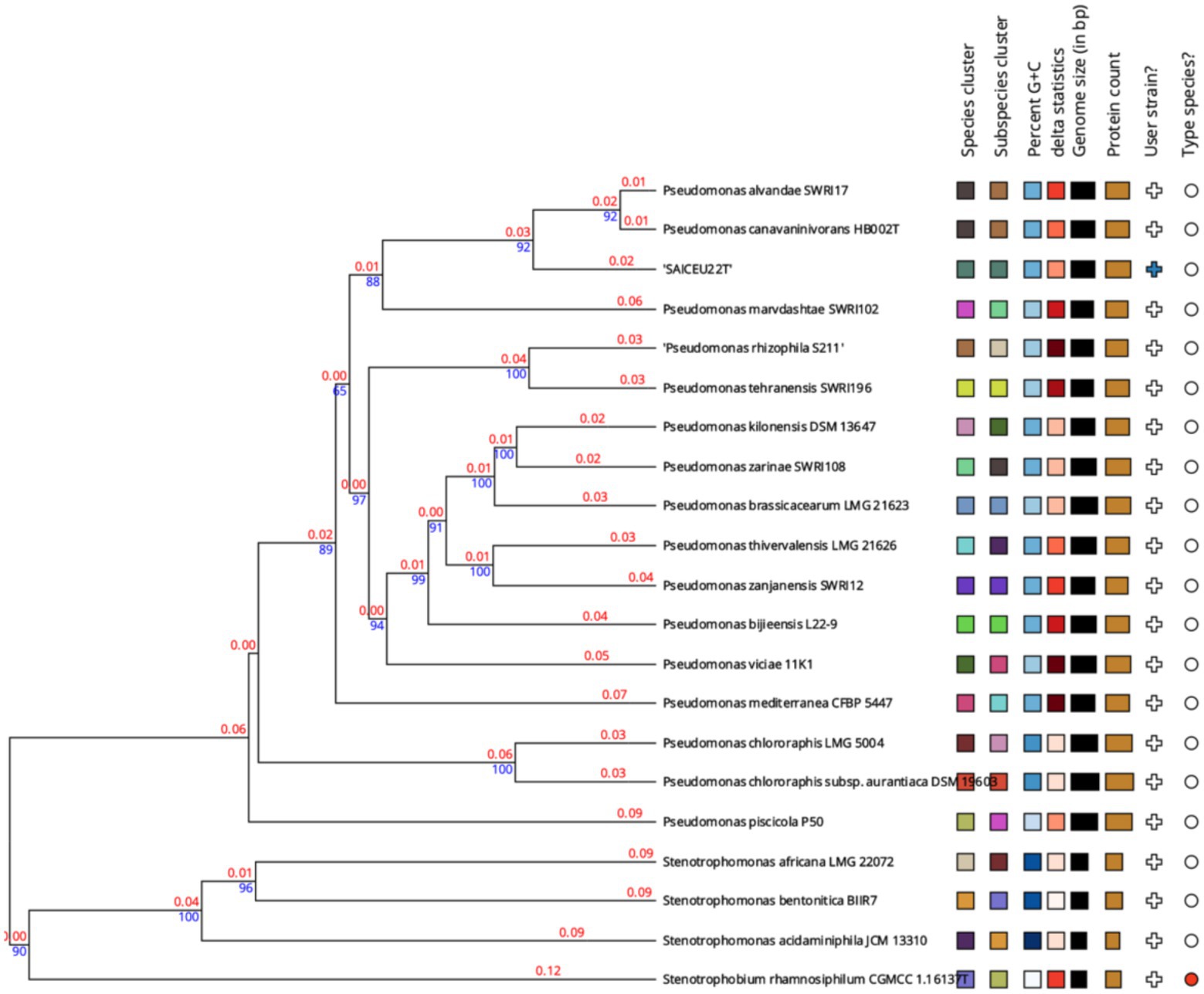
Figure 6. Phylogenetic tree from the complete genome of the species of Pseudomonas genus. Numerical values indicate the relative distance between the analyzed species. The branch lengths are scaled in terms of GBDP distance formula d5. The blue numbers are GBDP pseudo-bootstrap support values > 60% from 100 replications, with an average branch support of 55.2%. The branch length values (in red) represent the evolutionary time between two nodes. Unit: substitutions per sequence site. The tree was rooted at the midpoint (Farris, 1972).
4. Discussion
Unlike synthetic chemical fertilizers, organic fertilizers generated from recovered agronomic residues provide a set of nutrients in the form of complex biomolecules, which plants cannot always adsorb directly. However, the addition of PGPB to an organic fertilizer, giving rise to a biofertilizer, induces a double effect. Directly, PGP activities induce the promotion of plant growth. Indirectly, microbial participation in the hydrolysis and transformation of complex organic molecules into simpler nutrients makes them more accessible for root adsorption (Lara Mantilla et al., 2011; Velasco-Jiménez et al., 2020).
There are numerous strains belonging to Bacillus and Pseudomonas genera that present PGP activities, which allows their potential use to produce biofertilizers (Xue et al., 2021). PGP activities include, among others, the production of auxins (Pereira et al., 2019) and the enzyme ACCd (Kumar et al., 2021; Soumare et al., 2021). The biosynthesis of HCN, encoded by the hcnABC operon, induces a phytoprotective effect minimizing the ability to compete with other microorganisms that could behave as phytopathogens. Genetic analysis of SAICEU11T as SAICEU22T shows that both fully possess functional genes for these PGP activities. Additionally, both strains have many other genes that endow them with thermoprotective and phytoprotective capacity (Supplementary Tables 1, 2), not having found genes that encode pathogenicity factors in either of the two studied strains. Thus, the joint and orderly expression of PGP genes can explain their phenotypic profile in vitro, as the results of the biometric variables of plants treated with ORGAON® and ORGAON®st supplemented with these bacteria.
The comparative fertilization assay of Chem-F added with SAICEU11T to its unadded Chem-F control shows the absence of significant differences in most variables. This fact shows that the addition of the SAICEU11T strain does not induce a better use of nutrients by the plant when tested in a traditional chemical fertilizer. Likewise, there are no significant differences in biometric variables when comparing Chem-F treatments against ORGAON®. On the contrary, the use of the biofertilizer resulting from the addition of the SAICEU11T strain, both to ORGAON® and ORGAON®st, induces a significant increase in the variables root weight, weight and total length of the plant, and number of secondary roots. This fact shows the double effect that allows a PGPB to contribute to the complex organic fertilizer matrix (Hakim et al., 2021). This indirect activity of nutrient biotransformation has a second positive effect consisting of the microbial retention of biomolecules available to plants (Olanrewaju et al., 2017). In this way, unlike soluble chemical fertilizers, their soil loss by leachate is minimized by remaining longer in the soil and prolonging its availability. The optimization of use by plants can result in the reduction of fertilizer consumption and the minimization of its loss. In this way, the impact on ecosystems is reduced, compared to the use of chemical fertilizers (Stefan et al., 2022).
Treatment with ORGAON®st added with the SAICEU22T strain induces a significant increase in both the number of secondary roots and the length of the root. These effects are probably favored by the elimination of competition between the strains tested independently against the microorganisms that originally contained the organic fertilizer ORGAON®st prior to its sterilization (Abril et al., 2006). The increase in root size is a very relevant factor, since it allows the plant to acquire nutrients from different depths of the soil, favoring the retention of nutrients that could otherwise be lost by leachate (Olanrewaju et al., 2017).
Regarding the biometric results of the plants treated with the consortium strains, it is observed that there are no significant differences in the treatments with ORGAON®, ORGAON®st or Chem-F. The fact that the consortium is not effective compared to treatments in which strains are used independently appears to be due to incompatibility or competence phenomena between strains (Kato et al., 2005).
There is a scientific interest in the correct taxonomic ordering and characterization of PGPBs that allows an adequate biotechnological use. Thus, the phenotypic characteristics of the strains SAICEU11T and SAICEU22T place them within the genera Bacillus and Pseudomonas, respectively. The sequence of highly conserved regions and variable regions in the genome of housekeeping 16S rRNA has allowed for years the identification and classification of bacterial species. Although its use with known clinical strains is very efficient, this application loses reliability when it comes to environmental strains (Gomila et al., 2015). Therefore, although the sequence of a strain present homology of 100% for said 16S rRNA, the identification with a certain species does not necessarily mean they belong to the same taxon. To verify this, it is necessary to resort to the sequencing of a greater number of housekeeping genes. Currently, the genes gyrB, rpoB and rpoD are widely used both for taxonomic classification and the identification of Bacillus genus species (Yamada et al., 1999; La Duc et al., 2004) whilst gyrB and rpoD are normally used for Pseudomonas genus (Yamamoto et al., 2000). The analysis of the homology percentages of the 16S rRNA gene of the SAICEU11T strain reached a high identity value. However, the analysis of the housekeeping genes gyrB, rpoB and rpoD does not accurately satisfy the criteria for identification of the SAICEU11T strain. In this way, although in the sequence of the 16S rRNA gene like the rest of housekeeping they present a very high percentage of homology of SAICEU22T against Pseudomonas alvandae SWRI17, the identification criteria are not met either (Tables 2, 3). The chemotaxonomic analysis using the protein profile obtained with MALDI-TOF spectrometry, as well as the fatty acid profile do not allow the identification of the strains tested with any known species (Table 2).
The dDDH and ANI analyses show that both species are below the species threshold, which would support the hypothesis that two new species are being treated.
For all these reasons, the SAICEU11T and SAICEU22T strains are defined as not yet described species. Thus, the name of Bacillus pretiosus sp. nov is proposed for the strain SAICEU11T (= DSM 114702T = CECT 30674T) and Pseudomonas agronomica sp. nov for SAICEU22T (= DSM 114959T = CECT 30673T) for Pseudomonas agronomica.
5. Description of Bacillus pretiosus sp. nov
Bacillus pretiosus, was isolated from the rhizosphere of Medicago sativa in the mining district of Almadén (Ciudad Real, Spain), from an abandoned dump of a mercury exploitation. Gram-positive, motile bacillus, ranging from 2 ± 0.10 × 0.70 ± 0.15 μm, catalase positive and with colonies visible at 24 h with a creamy texture and dump color on nutrient agar and with beta-hemolysis on blood agar. Growth occurs between 4 and 37°C in 24 h. It grows in a pH range of 5.5–8.0. It is capable of assimilating D-mannitol, fermenting D-glucose and Lactose, and hydrolyzing gelatin. It can produce auxins and ADDd but does not solubilize phosphates or produce siderophores. The predominant fatty acids for SAICEU11T were C15:0 (39.21%), C16:0 (11.68%), C17:0 (10.79%), C14:0 (8.36%), C13:0 (7.28%) and C17:1 (7.21%). The G + C genomic DNA content of the type strain is 35.30%. The complete genome and the 16S rRNA gene sequence have been deposited in the NCBI Genbank (BioProject PRJNA850797, BioSample SAMN29203362 and GenBank JAOXJG000000000). The type strain is SAICEU11T (= DSM 114702T = CECT30674T).
6. Description of Pseudomonas agronómicas sp. nov
Pseudomonas agronomicas was isolated from the rhizosphere of Medicago sativa in the mining district of Almadén (Ciudad Real, Spain), from an abandoned dump of a mercury exploitation. Gram-negative, non-motile bacillus, ranging from 1.40 ± 0.10 × 0.50 ± 0.15 μm, catalase positive, whitish to translucent in color when grown on nutrient agar. In cultures of more than 72 h, it begins to produce pyoverdine, which gives it a characteristic green color. Growth occurs between 5 and 40°C in 24 h and in a pH range of 5.5–8.0. It is capable of assimilating D-mannitol, Citrate, Maltose and Xylose; oxidize D-glucose; reduce Nitrate and hydrolyze Galatin. It produces auxins, ACCd and siderophores but does not solubilize phosphates. The predominant fatty acids for SAICEU22T were C16:0 (31.53%), C12:0 (8.55%), and cyclo C17:0 (8.02%). The G + C genomic DNA content of the type strain is 61.10%. The complete genome and the 16S rRNA gene of the SAICEU22T strain have been deposited in the NCBI Genbank (BioProject PRJNA850797, BioSample SAMN29203362 and GenBank JAOXJG000000000). The type strain is SAICEU22T (= DSM 114959T = CECT30673T).
Data availability statement
The data presented in the study are deposited in the GenBank repository, accession number JAOSHO000000000 and JAOXJG000000000.
Author contributions
PJ, VF, and MR: conceptualization. PJ and VF: methodology. VF: software. PJ and MR: validation and supervision. VF and LG: formal analysis and resources. VF, LG, and MR: investigation. PJ, VF, and LG: data curation. LG and MR: writing—original draft preparation. PJ, AP, and MR: writing—review and editing. MR and LG: visualization. AP and PJ: project administration and funding acquisition. All authors contributed to the article and approved the submitted version.
Funding
This research has been funded by the Fundación Universitaria San Pablo CEU and Banco Santander, grant number FUSP- BS-PPC01/2014. Grants to recognized research groups 2021/2022 (Vice President for Re-search and Teaching Staff, CEU San Pablo University). Likewise, this project has been financed with the European Funds oriented to the ecological transition and digital transition, of the national plan for scientific, technical and innovation research 2021-2023, within the framework of the recovery, transformation and resilience plan. File number TED2021-132285A-I00.
Acknowledgments
We are grateful to the Seed Bank of the Center for Scientific and Technological Research of Extremadura, for its permanent availability in the supply of seeds for trials. Special thanks to the Carlos III Health Institute, Laboratory of Bacterial Taxonomy (Majadahonda, Madrid, Spain), for helping us in sequencing the complete genome of the strain.
Conflict of interest
The authors declare that the research was conducted in the absence of any commercial or financial relationships that could be construed as a potential conflict of interest.
Publisher’s note
All claims expressed in this article are solely those of the authors and do not necessarily represent those of their affiliated organizations, or those of the publisher, the editors and the reviewers. Any product that may be evaluated in this article, or claim that may be made by its manufacturer, is not guaranteed or endorsed by the publisher.
Supplementary material
The Supplementary material for this article can be found online at: https://www.frontiersin.org/articles/10.3389/fmicb.2022.1046201/full#supplementary-material
References
Abril, A., Biasutti, C., Maich, R., Dubbini, L., and Noe, L. (2006). Inoculation with Azospirillum spp. in the semiarid-central region of Argentina: factors involved in rhizosphere colonization.
Achouak, W., Sutra, L., Heulin, T., Meyer, J.-M., Fromin, N., Degraeve, S., et al. (2000). Pseudomonas brassicacearum sp. nov. and Pseudomonas thivervalensis sp. nov., two root-associated bacteria isolated from Brassica napus and Arabidopsis thaliana. Int. J. Syst. Evol. Microbiol. 50, 9–18. doi: 10.1099/00207713-50-1-9
Alexander, D. B., and Zuberer, D. A. (1991). Use of chrome azurol S reagents to evaluate siderophore production by rhizosphere bacteria. Biol. Fertil. Soils 12, 39–45. doi: 10.1007/BF00369386
Alkemade, J. A., Messmer, M. M., Arncken, C., Leska, A., Annicchiarico, P., Nazzicari, N., et al. (2021). A high-throughput phenotyping tool to identify field-relevant anthracnose resistance in white lupin. Plant Dis. 105, 1719–1727. doi: 10.1094/PDIS-07-20-1531-RE
Altschul, S. F., Gish, W., Miller, W., Myers, E. W., and Lipman, D. J. (1990). Basic local alignment search tool. J. Mol. Biol. 215, 403–410. doi: 10.1016/S0022-2836(05)80360-2
Andrews, S. (2010). FastQC: A quality control tool for high throughput sequence data. Available online at: https://www.bioinformatics.babraham.ac.uk/projects/fastqc/
Baek, I., Lee, K., Goodfellow, M., and Chun, J. (2019). Comparative genomic and phylogenomic analyses clarify relationships within and between Bacillus cereus and Bacillus thuringiensis: proposal for the recognition of two Bacillus thuringiensis Genomovars. Front. Microbiol. 10:1978. doi: 10.3389/fmicb.2019.01978
Bankevich, A., Nurk, S., Antipov, D., Gurevich, A. A., Dvorkin, M., Kulikov, A. S., et al. (2012). SPAdes: a new genome assembly algorithm and its applications to single-cell sequencing. J. Comput. Biol. 19, 455–477. doi: 10.1089/cmb.2012.0021
De Freitas, J., Banerjee, M., and Germida, J. (1997). Phosphate-solubilizing rhizobacteria enhance the growth and yield but not phosphorus uptake of canola (Brassica napus L.). Biol. Fertil. Soils 24, 358–364. doi: 10.1007/s003740050258
Dubois, O. (2011). The State of the World’s Land and Water Resources for Food and Agriculture: Managing Systems at Risk. London: Earthscan.
Dunlap, C. A. (2019). Taxonomy of registered bacillus spp. strains used as plant pathogen antagonists. Biol. Control 134, 82–86. doi: 10.1016/j.biocontrol.2019.04.011
Ehmann, A. (1977). The Van Urk-Salkowski reagent—a sensitive and specific chromogenic reagent for silica gel thin-layer chromatographic detection and identification of indole derivatives. J. Chromatogr. A 132, 267–276. doi: 10.1016/S0021-9673(00)89300-0
Farris, J. S. (1972). Estimating phylogenetic trees from distance matrices. Am. Nat. 106, 645–668. doi: 10.1086/282802
Fiodor, A., Singh, S., and Pranaw, K. (2021). The contrivance of plant growth promoting microbes to mitigate climate change impact in agriculture. Microorganisms 9:1841. doi: 10.3390/microorganisms9091841
Glick, B. R. (1995). The enhancement of plant growth by free-living bacteria. Can. J. Microbiol. 41, 109–117. doi: 10.1139/m95-015
Gomila, M., Peña, A., Mulet, M., Lalucat, J., and García-Valdés, E. (2015). Phylogenomics and systematics in pseudomonas. Front. Microbiol. 6:214. doi: 10.3389/fmicb.2015.00214
Hafez, A., Futami, R., Arastehfar, A., Daneshnia, F., Miguel, A., Roig, F. J., et al. (2021). SeqEditor: an application for primer design and sequence analysis with or without GTF/GFF files. Bioinformatics 37, 1610–1612. doi: 10.1093/bioinformatics/btaa903
Hakim, S., Naqqash, T., Nawaz, M. S., Laraib, I., Siddique, M. J., Zia, R., et al. (2021). Rhizosphere engineering with plant growth-promoting microorganisms for agriculture and ecological sustainability. Front. Sustain. Food Syst. 5:617157. doi: 10.3389/fsufs.2021.617157
Hauth, F., Buck, H., and Hartig, J. S. (2022). Pseudomonas canavaninivorans sp. nov., isolated from bean rhizosphere. Int. J. Syst. Evol. Microbiol. 72:005203. doi: 10.1099/ijsem.0.005203
Hufnagel, B., Marques, A., Soriano, A., Marquès, L., Divol, F., Doumas, P., et al. (2020). High-quality genome sequence of white lupin provides insight into soil exploration and seed quality. Nat. Commun. 11, 1–12. doi: 10.1038/s41467-019-14197-9
Imhoff, J. F., Garrity, G. M., Bell, J. A., Lilburn, T., Saddler, G. S., Bradbury, J. F., et al. (2005). Bergey’s manual of systematic bacteriology volume two the Proteobacteria part B the Gammaproteobacteria. Springer Science & Business Media
Kato, S., Haruta, S., Cui, Z. J., Ishii, M., and Igarashi, Y. (2005). Stable coexistence of five bacterial strains as a cellulose-degrading community. Appl. Environ. Microbiol. 71, 7099–7106. doi: 10.1128/AEM.71.11.7099-7106.2005
Katsenios, N., Andreou, V., and Sparangis, P. (2022). Assessment of plant growth promoting bacteria strains on growth, yield and quality of sweet corn. Sci. Rep. 12:11598. doi: 10.1038/s41598-022-16044-2
Kumar, S., Sindhu, S. S., and Kumar, R. (2021). Biofertilizers: an ecofriendly technology for nutrient recycling and environmental sustainability. Curr. Res. Microbial Sci. 3:100094. doi: 10.1016/j.crmicr.2021.100094
La Duc, M. T., Satomi, M., Agata, N., and Venkateswaran, K. (2004). gyrB as a phylogenetic discriminator for members of the bacillus anthracis–cereus–thuringiensis group. J. Microbiol. Methods 56, 383–394. doi: 10.1016/j.mimet.2003.11.004
Lalucat, J., Gomila, M., Mulet, M., Zaruma, A., and García-Valdés, E. (2021). Past, present and future of the boundaries of the Pseudomonas genus: proposal of Stutzerimonas gen. Nov. Syst. Appl. Microbiol. 45:126289. doi: 10.1016/j.syapm.2021.126289
Lara Mantilla, C., Oviedo Zumaqué, L. E., and Betancur Hurtado, C. A. (2011). Bacterias nativas con potencial en la producción de ácido indolacético para mejorar los pastos. Zootec. Trop. 29, 187–194.
Liu, Y., Du, J., Lai, Q., Zeng, R., Ye, D., Xu, J., et al. (2017). Proposal of nine novel species of the Bacillus cereus group. Int. J. Syst. Evol. Microbiol. 67, 2499–2508. doi: 10.1099/ijsem.0.001821
Martin, M. (2011). Cutadapt removes adapter sequences from high-throughput sequencing reads. EMBnet. J. 17, 10–12. doi: 10.14806/ej.17.1.200
Mathema, V. B., Thakuri, B. K. C., Sillanpää, M., and Shrestha, R. A. (2011). Study of mercury (II) chloride tolerant bacterial isolates from Baghmati River with estimation of plasmid size and growth variation for the high mercury (II) resistant Enterobacter spp. J. Biotech. Res. 3:72.
Meier-Kolthoff, J. P., and Göker, M. (2019). TYGS is an automated high-throughput platform for state-of-the-art genome-based taxonomy. Nat. Commun. 10:2182. doi: 10.1038/s41467-019-10210-3
Migula, W. (1894). Über ein neues System der Bakterien. Arbeiten aus dem Bakteriologischen Institut der Technischen Hochschule zu Karlsruhe, 1, 235–238.
Miller, R. A., Beno, S. M., Kent, D. J., Carroll, L. M., Martin, N. H., Boor, K. J., et al. (2016). Bacillus wiedmannii sp. nov., a psychrotolerant and cytotoxic Bacillus cereus group species isolated from dairy foods and dairy environments. Int. J. Syst. Evol. Microbiol. 66:4744. doi: 10.1099/ijsem.0.001421
Nautiyal, C. S. (1999). An efficient microbiological growth medium for screening phosphate solubilizing microorganisms. FEMS Microbiol. Lett. 170, 265–270. doi: 10.1111/j.1574-6968.1999.tb13383.x
Olanrewaju, O. S., Glick, B. R., and Babalola, O. O. (2017). Mechanisms of action of plant growth promoting bacteria. World J. Microbiol. Biotechnol. 33, 1–16. doi: 10.1007/s11274-017-2364-9
Ondov, B. D., Treangen, T. J., Melsted, P., Mallonee, A. B., Bergman, N. H., Koren, S., et al. (2016). Mash: fast genome and metagenome distance estimation using MinHash. Genome Biol. 17, 1–14. doi: 10.1186/s13059-016-0997-x
Patel, S., and Gupta, R. S. (2020). A phylogenomic and comparative genomic framework for resolving the polyphyly of the genus bacillus: proposal for six new genera of bacillus species, Peribacillus gen. Nov., Cytobacillus gen. Nov., Mesobacillus gen. Nov., Neobacillus gen. Nov., Metabacillus gen. Nov. and Alkalihalobacillus gen. Nov. Int. J. Syst. Evol. Microbiol. 70, 406–438. doi: 10.1099/ijsem.0.003775
Pereira, L. B., Andrade, G. S., Meneghin, S. P., Vicentini, R., and Ottoboni, L. M. (2019). Prospecting plant growth-promoting bacteria isolated from the rhizosphere of sugarcane under drought stress. Curr. Microbiol. 76, 1345–1354. doi: 10.1007/s00284-019-01749-x
Robas, M., Jiménez, P. A., González, D., and Probanza, A. (2021). Bio-mercury remediation suitability index: a novel proposal that compiles the PGPR features of bacterial strains and its potential use in phytoremediation. Int. J. Environ. Res. Public Health 18:4213. doi: 10.3390/ijerph18084213
Robas Mora, M., Probanza Lobo, A., and Jiménez Gómez, P. A. (2022). Valorization as a biofertilizer of an agricultural residue leachate: metagenomic characterization and growth promotion test by PGPB in the forage plant Medicago sativa (alfalfa). Front. Microbiol. Submitted.
Sasser, M. (2001). Identification of Bacteria by Gas Chromatography of Cellular Fatty Acids. Technical Note 101. Microbial ID, Inc., Newark, Del.
Schmieder, R., and Edwards, R. (2011). Quality control and preprocessing of metagenomic datasets. Bioinformatics 27, 863–864. doi: 10.1093/bioinformatics/btr026
Seemann, T. (2014). Prokka: rapid prokaryotic genome annotation. Bioinformatics 30, 2068–2069. doi: 10.1093/bioinformatics/btu153
Smith, N. (1957). Genus Bacillus subtilis Cohn, 1872. Bergey’s Manual of Determinative Bacteriology, 620–622.
Soumare, A., Diédhiou, A. G., Arora, N. K., Tawfeeq Al-Ani, L. K., Ngom, M., Fall, S., et al. (2021). Potential role and utilization of plant growth promoting microbes in plant tissue culture. Front. Microbiol. 12:649878. doi: 10.3389/fmicb.2021.649878
Stefan, D. S., Bosomoiu, M., Dancila, A. M., and Stefan, M. (2022). Review of soil quality improvement using biopolymers from leather waste. Polymers 14:1928. doi: 10.3390/polym14091928
Torres Manno, M. A., Repizo, G. D., Magni, C., Dunlap, C. A., and Espariz, M. (2020). The assessment of leading traits in the taxonomy of the Bacillus cereus group. Antonie Van Leeuwenhoek 113, 2223–2242. doi: 10.1007/s10482-020-01494-3
Velasco-Jiménez, A., Castellanos-Hernández, O., Acevedo-Hernández, G., Aarland, R. C., and Rodríguez-Sahagún, A. (2020). Rhizospheric bacteria with potential benefits in agriculture. Terra Latinoamericana 38, 333–345. doi: 10.28940/terra.v38i2.470
Volpiano, C. G., Sant'Anna, F. H., Ambrosini, A., de São José, J., Beneduzi, A., Whitman, W. B., et al. (2021). Genomic metrics applied to Rhizobiales (Hyphomicrobiales): species reclassification, identification of unauthentic genomes and false type strains. Front. Microbiol. 12:614957. doi: 10.3389/fmicb.2021.614957
Witzgall, K., Vidal, A., Schubert, D. I., Höschen, C., Schweizer, S. A., Buegger, F., et al. (2021). Particulate organic matter as a functional soil component for persistent soil organic carbon. Nat. Commun. 12:4115. doi: 10.1038/s41467-021-24192-8
Xue, L., Tang, L., Zhao, J., Fang, Z., Liu, H., Qiao, J., et al. (2021). Bacillus salipaludis sp. nov., isolated from saline–alkaline soil. Arch. Microbiol. 203, 2211–2217. doi: 10.1007/s00203-021-02239-0
Yamada, S., Ohashi, E., Agata, N., and Venkateswaran, K. (1999). Cloning and nucleotide sequence analysis of gyrB of Bacillus cereus, B. thuringiensis, B. mycoides, and B. anthracis and their application to the detection of B. cereus in rice. Appl. Environ. Microbiol. 65, 1483–1490. doi: 10.1128/AEM.65.4.1483-1490.1999
Yamamoto, S., Kasai, H., Arnold, D. L., Jackson, R. W., Vivian, A., and Harayama, S. (2000). Phylogeny of the genus Pseudomonas: intrageneric structure reconstructed from the nucleotide sequences of gyrB and rpoD genesThe GenBank accession numbers for the sequences determined in this work are: gyrB, D37926, D37297, D86005-D86019 and AB039381-AB039492; rpoD, D86020-D86036 and AB039493-AB039624. Microbiology 146, 2385–2394. doi: 10.1099/00221287-146-10-2385
Yang, J., Chen, L., Sun, L., Yu, J., and Jin, Q. (2007). VFDB 2008 release: an enhanced web-based resource for comparative pathogenomics. Nucleic Acids Res. 36, D539–D542. doi: 10.1093/nar/gkm951
Keywords: Bacillus pretiosus, biofertilizer, organic fertilizer, PGPB, Pseudomonas agronomica, rhizobacteria, plant residue valorization
Citation: Robas Mora M, Fernández Pastrana VM, Gutiérrez Oliva LL, Probanza Lobo A and Jiménez Gómez PA (2023) Plant growth promotion of the forage plant Lupinus albus Var. Orden Dorado using Pseudomonas agronomica sp. nov. and Bacillus pretiosus sp. nov. added over a valorized agricultural biowaste. Front. Microbiol. 13:1046201. doi: 10.3389/fmicb.2022.1046201
Edited by:
Justyna Bohacz, University of Life Sciences of Lublin, PolandReviewed by:
Kanika Bansal, Institute of Microbial Technology (CSIR), IndiaStefanie P. Glaeser, University of Giessen, Germany
Copyright © 2023 Robas Mora, Fernández Pastrana, Gutiérrez Oliva, Probanza Lobo and Jiménez Gómez. This is an open-access article distributed under the terms of the Creative Commons Attribution License (CC BY). The use, distribution or reproduction in other forums is permitted, provided the original author(s) and the copyright owner(s) are credited and that the original publication in this journal is cited, in accordance with accepted academic practice. No use, distribution or reproduction is permitted which does not comply with these terms.
*Correspondence: Marina Robas Mora, ✉ bWFyaW5hLnJvYmFzbW9yYUBjZXUuZXM=; Vanesa M. Fernández Pastrana, ✉ dmFuZXNhLmZlcm5hbmRlenBhc3RyYW5hQGNldS5lcw==; Pedro A. Jiménez Gómez, ✉ cGVkcm8uamltZW5lemdvbWV6QGNldS5lcw==