- 1Department of Botany and Microbiology, Faculty of Science, Zagazig University, Zagazig, Egypt
- 2Department of Microbiology, Faculty of Veterinary Medicine, Zagazig University, Zagazig, Egypt
- 3Department of Biochemistry, Faculty of Agriculture, Zagazig University, Zagazig, Egypt
- 4Department of Botany and Microbiology, Faculty of Science, Beni-Suef University, Beni-Suef, Egypt
- 5Department of Biology, College of Science, Princess Nourah Bint Abdul Rahman University, Riyadh, Saudi Arabia
- 6Department of Medical Microbiology and Immunology, Faculty of Medicine, Zagazig University, Zagazig, Egypt
In the present study, biologically active compounds such as phenolic-rich extract (PRE), 7S globulin (vicilin), and 11S globulin (legumin) from red kidney bean (Phaseolus vulgaris L.) seeds were extracted and evaluated as antibacterial agents against multidrug-resistant (MDR) Enterobacterales isolated from both animal and human sources. The overall occurrence rate of Enterobacterales was 43.6%, which significantly differed between animal (38.75%) and human (56.67%) sources. Antimicrobial susceptibility testing revealed that Enterobacterales isolates exhibited full resistance (100%) to amoxicillin-clavulanic acid, followed by ampicillin (75.44%), erythromycin (71.93%), cefoxitin (70.18%), amoxicillin (66.66%), ceftriaxone (64.91%), and trimethoprim/sulfamethoxazole (56.14%). Worthy of note, 97.92% of Enterobacterales isolates were MDR. The total phenolic contents (TPC; 53 ± 2 mg GAE g-1) and total flavonoid contents (TFC; 26 ± 1 mg QE g-1) were recorded. The major phenolic and flavonoid components were catechol (17.63 μg/mL) and hesperidin (11.37 μg/mL), respectively. Sodium dodecyl sulfate-polyacrylamide gel electrophoresis (SDS-PAGE) was performed to detect the 7S and 11S globulin‘s molecular mass. The data revealed that red kidney bean protein isolate (KPI) includes two major portions: 7S and 11S globulins. The bioactive compounds of Phaseolus vulgaris were investigated for their antibacterial activities against Enterobacterales for the first time. The protein component (MIC = 0.125 – 2 μg/mL; 53.85%) and its 7S and 11S globulin subunits (MIC = 0.5 – 2 μg/mL; 30.77% each) were the most potent extracts, whereas the methanolic extract was the least effective one (MIC = 2 μg/mL; 15.38%). The results displayed the potential of protein bioactive compounds as a hopeful candidate for enhancing future medication plans for the treatment of Enterobacterales originating from animal and human sources.
Introduction
Enterobacterales are the most challenging bacterial contaminant in raw and processed meat products worldwide, with a prominence of Escherichia coli (E. coli), Salmonella, Proteus, and Klebsiella species in food poisoning cases (Mohammed et al., 2014). The risk of this group comes from the high contamination level of examined meat at slaughterhouses, which begins from skinning, evisceration, washing, handling, and transportation that may cause foodborne illness (Duffy et al., 2003; Severgnini et al., 2011).
Antimicrobial resistance is a serious issue. It has evolved into a significant public health concern of the 21st century. The National Antimicrobial Resistance Monitoring System (NARMS) surveys indicated that retail meat is frequently contaminated with multidrug-resistant (MDR) bacteria such as Staphylococcus aureus, Salmonella species, and E. coli (Food and Drug Administration, 2007). Antimicrobial resistance and MDR bacterial strains are frequent in hospitals and might impair worldwide infectious disease management (Terreni et al., 2021).
Nowadays, increased mortalities per year are attributed to nosocomial infections with antimicrobial resistance; future years are expected to be considerably worse, as revealed recently (Zurabov and Zhilenkov, 2021). Hence, searching for novel antimicrobials derived from natural sources is an essential component of contemporary medicine to combat the socio-economic influence and health impact caused by MDR bacteria (Bakal et al., 2017; Khalid et al., 2021; Sabry et al., 2022).
A wide variety of secondary metabolites are produced by plants, many of which exhibit antibacterial properties against some pathogenic bacteria linked to gastrointestinal illnesses. Some of these components are constitutive, exist in healthy plants in their physiologically active forms, and exhibit chemotherapeutic or chemoprophylactic characteristics against a variety of enteric infectious illnesses (Godstime and Akpotu, 2014).
The proteins in legume seeds as beans (Pharsalus species) and peas (Pisum sativum) are about 20%, whereas in lupin (Lupinus species) and soybean (Glycine max) are up to 40% (Đorpevic’, 2009). The globulins predominate as storage proteins and typically account for 50–90 percent of the protein content in legume seeds. Some of these proteins were recognized to have important pharmacological and therapeutic values (Scarafoni et al., 2007).
Four out of five persons turn to conventional medicine for their healthcare necessities (Kebede et al., 2021). However, medicinal plants are equipped with a variety of bioactive chemicals to combat multidrug resistance (Kebede et al., 2021). Beans proteins such as lectins, arcelins, and protease and amylase inhibitors were proven to have antimicrobial properties (Ma et al., 2009). Roy et al. (2020) documented that dark red kidney bean protein hydrolyzates may be used as auspicious antimicrobial and antioxidant candidates. Previous studies described the significance of 7S and 11S globulins from different sources as antimicrobial agents (Sitohy et al., 2012; Osman et al., 2013; Mahgoub et al., 2013, 2016; Abbas et al., 2020; Atallah et al., 2021). To further enrich the library of natural antibacterial agents, the objective of the work was to investigate the antibacterial activities of 11S globulins, 7S globulins, and phenolic-rich extracts isolated from red kidney bean seeds against MDR Enterobacterales isolated from animal and human sources.
Materials and methods
Sampling
From August 2020 to June 2021, 110 samples were collected from both animal (n = 80) and human (n = 30) sources. Animal samples, including raw milk (n = 25), milk products (n = 17), fresh meat (n = 12), and meat products (n = 26), were drawn randomly from various supermarkets in Fakous City, Sharkia Governorate, Egypt. In addition, human stools (n = 30) were obtained from attending patients at various laboratories and hospitals in Fakous City, Sharkia Governorate. The samples were put into sterile containers aseptically, stored in an ice bag, and transferred immediately to the bacteriology laboratory for their examination upon arrival. The declaration of Helsinki, the World Medical Association’s Code of Ethics, was followed during the study’s execution. The patients provided informed written consent to take part in this investigation.
Isolation and identification of Enterobacterales
A loopful from each supplemented broth was grown on an agar medium that was selective for all predictable bacteria; MacConkey‘s agar (Oxoid, Cambridge, UK) for coliforms, Eosin Methylene Blue agar (EMB; Oxoid, Cambridge, UK) for E. coli, HiCrome Klebsiella selective agar (Himedia, Thane West, India) for Klebsiella species and MacConkey‘s agar without salt (Oxoid, Cambridge, UK) for Proteus species. To isolate Salmonella, one gram of each sample was inoculated into 9 mL of buffered peptone water (BPW; Oxoid, Cambridge, UK), followed by incubation for 18 h at 37°C. One-tenth mL of the BPW was injected into 10 mL of Rappaport Vassiliadis soy broth (RV; Oxoid, Cambridge, UK), then incubation was done for 24 h at 42°C. Selective plating was applied using Xylose Lysine Deoxycholate agar (XLD; Sigma-Aldrich, St. Louis, USA). Gram stain was used to identify a suspected colony of each bacterium, then conventional biochemical assays (Faddin, 2000) were performed to identify each bacterial species. Biochemically identified E. coli isolates were serotyped by the rapid agglutination approach using specific antisera (Denka Seiken Co., Tokyo, Japan). Molecular confirmation of Gram-negative isolates was done by polymerase chain reaction (PCR) utilizing the species-specific primer pairs indicated in Supplementary Table 1 (Brisse and Verhoef, 2001; Murugkar et al., 2003; Kumar et al., 2008; Turton et al., 2010; Bi et al., 2013; Litty et al., 2013; Zhang et al., 2013; Halimi et al., 2014). PCR amplimers were visualized by a U.V. transilluminator (Sigma-Aldrich, St. Louis, USA) after electrophoresis for 30 min on a 2% agarose gel incorporated with 0.5 μg/mL ethidium bromide.
Antimicrobial susceptibility testing of Enterobacterales
The antimicrobial susceptibilities of Enterobacterales against 17 antimicrobial agents were detected by adopting the Clinical and Laboratory Standards Institute [CLSI] recommendations (Clinical and Laboratory Standards Institute [CLSI], 2020). The examined antimicrobials (Oxoid, Cambridge, UK) were penicillins [amoxicillin (25 μg), amoxicillin-clavulanic acid (20/10 μg), ampicillin (10 μg), ampicillin-sulbactam (10/10 mg)], cephalosporins [cefoxitin (30 μg), ceftriaxone (30 μg), cefepime (30 μg)], carbapenems [imipenem (10 μg)], fluoroquinolones [ciprofloxacin (5 μg)], aminoglycosides [gentamicin (10 μg), amikacin (30 μg)], sulfonamides [trimethoprim-sulfamethoxazole (1.25/23.75 μg)], macrolides [erythromycin (15 μg)], tetracyclines [doxycycline (30 μg)], chloramphenicol [chloramphenicol (30 μg)], monobactam [aztreonam (10 μg)], and glycylcycline [tigecycline (15 μg)]. The multiple antibiotic resistance (MAR) indices were assessed according to Tambekar et al. (2006), and the MDR isolates were recorded following Magiorakos et al. (2012).
Red kidney bean (Phaseolus vulgaris L.) seeds
Red kidney bean seeds were obtained from a local outlet in Zagazig City, Sharkia Governorate, Egypt. The seeds were manually cleaned, ground, and defatted using a Soxhlet apparatus (Prosperity Biotech Co., Ltd., Shandong, China) with hexane for 6–8 h. The solvent was vaporized using a rotary evaporator (VWR International GmbH, Darmstadt, Germany), and the defatted dried meal was preserved at 4°C until analysis.
Preparation and characterization of phenolic-rich kidney bean extract
The phenolic-rich red kidney bean extract (PRKE) was prepared as designated elsewhere (Abdel-Shafi et al., 2019a; Omar et al., 2020). Briefly, defatted red kidney bean flour (100 g) was extracted for 2 h in darkness at room temperature with 70% v/v aqueous methanol (1 L). Centrifugation of the sample was done for 15 min at 10,000 × g to recover the supernatant. Removal of the methanol from the extract was performed by vacuum evaporation in a BüCHI-water bath B-480 evaporator (Buchi, Switzerland) at 45°C followed by a freeze-dryer lyophilization (Thermo-electron Corporation–Heto power dry LL 300 Freeze drier, Bossgoo, China).
The total phenolic contents (TPCs) of the PRKE (1,000 μg/mL) were calculated by the Folin–Ciocalteu assay (Singleton et al., 1999). To create a standard curve, various concentrations of gallic acid (10–2000 g/mL) were dissolved in distilled water. The gallic acid calibration equation was as follows: y = 0.001x + 0.0563 (R2 = 0.9792), where y and x stand for the gallic acid absorbance and concentration expressed in μg/mL, respectively. The reaction mixture [1 mL from the standard solution or extract + 3 mL Folin-Ciocalteu diluted with distilled water (1:10, V/V) + 2 mL Na2Co3 7.5%] was agitated for one min, then kept at room temperature for 30 min in the darkness. The mixture absorbance was determined at 765 nm using a JANEWAY spectrophotometer (6405 UV/Vis, Missouri, UK). Gallic acid equivalents (GAE) per gram of extract were used to measure the sample’s ability to reduce the Folin-Ciocalteu reagent.
Total flavonoids of the PRKE (1,000 μg/mL) were approximated following Ordonez et al. (2006) a protocol as described previously (Abdel-Shafi et al., 2019b). Quercetin was dissolved in ethyl alcohol at various concentrations (10–1000 μg/mL) to get a standard curve. Total flavonoid contents (TFCs) are indicated as quercetin equivalent (QE). The later was computed using the subsequent calibration curve: y = 0.0012x + 0.008 (R2 = 0.944), where y means the absorbance and x refers to the concentration of quercetin in μg/mL. The reaction mixture involved 500 μL from the standard solution or the extract plus 1,000 μL aliquot of 20 g/L AlCl3 ethanol. The absorbance at 420 nm was recorded using a spectrophotometer (JENWAY, Missouri, UK).
High-performance liquid chromatography (HPLC)-(Agilent 1100) analysis is comprised of two liquid chromatography (LC) pumps, a C18 column (5 μm particle size; 125 mm × 4.60 mm), and a UV/Vis detector. The Agilent ChemStation Phenolic acids could analyze the chromatograms, which were then separated by engaging a gradient mobile phase of two solvents; methanol (solvent A) and acetic acid in water (solvent B; 1:25). Solvent B was used at 100% concentration to start the gradient program and remained there for the first 3 min. Thereafter, 50% eluent A was added for the next 5 min. The eluent A`s concentration was then raised to 80% for the following 2 min, then decreased to 50% once more for the subsequent 5 min, then detection of the wavelength was set at 250 nm.
Red kidney bean protein and its subunits isolation and characterization
Defatted red kidney bean flour (5%) dispersions in water were accustomed to pH 9 at room temperature with 0.1 N NaOH, centrifuged for 15 min at 2,000 × g after being agitated for an hour. To improve the yield, repeated isolation and centrifugation operations on the residue were performed. To precipitate the protein, the extracts were mixed and the pH was accustomed to 4.5 using 1N HCl. Thereafter, the proteins were extracted by centrifugation for 15 min at 2,000 × g. Decantation of the supernatant was done. After washing in distilled water, the crude protein was dispersed in a bit of water with a pH of 7.5, dialyzed overnight, and lyophilized (Johnson and Brekke, 1983). With minor changes, the 7S and 11S globulin subunits were extracted from the lupine seed‘s defatted powder using the procedures reported earlier (Abdel-Shafi et al., 2019a).
Sodium dodecyl sulfate-polyacrylamide gel electrophoresis
The defatted red kidney bean flour and crude protein isolate were melted in 1 mL of 10% SDS, 100 μL β-mercaptoethanol, and 20 mg of kidney bean protein. The mixture was then vortexed occasionally for 15 min. To separate the extract, centrifugation was done at 10,000 × g for 5 min. Twenty μL of extracted proteins were mixed with 20 μL of SDS-loading sample buffer (4% SDS, 3% β-mercaptoethanol, 20% glycerol, Tris-HCl 50 mM pH 6.8, and bromophenol blue traces), then heated for five min at 96°C. Aliquots of 10 μL of protein per lane were electrophoresed and analyzed by sodium dodecyl sulfate-polyacrylamide gel electrophoresis (SDS-PAGE) (Laemmli, 1970).
Urea-polyacrylamide gel electrophoresis
Lyophilized red kidney bean protein, 7S, and 11S globulins from red kidney bean seeds (10 mg/mL) were dispersed in a 6.8 pH buffer comprising bromophenol blue traces, 50% glycerol, and 0.25 M Tris-base. Centrifugation of samples (15,000 × g for 5 min) was applied at 20°C. Urea-PAGE analysis of the supernatants (10 μL of protein per lane) was performed using stacking (3%) and resolving (12%) gels (Evans and Williams, 1980).
The pH-solubility curve
Lyophilized red kidney bean protein, 7S, and 11S globulins from red kidney bean seeds were tested for pH-solubility curves in the pH 2–10 range (Chobert et al., 1991). Since the pH corresponds to the least solubility of proteins, the isoelectric points were calculated.
Fourier transform infrared spectroscopy
The potassium bromide (KBr) pellet method was followed to prepare the protein samples (Souillac et al., 2002). An Fourier transform infrared (FTIR) spectrometer (Thermo Scientific, Waltham, MA, USA) was used to measure infrared spectra at a temperature of 25°C. Thereafter, 256 interfero grams were obtained for each spectrum with a resolution of 4 cm–1 with 64 scans and a 2 cm–1 interval from the 4,000 to 400 cm–1 regions. The system was incessantly purged with dry air. Savitsky-Golay derivative function soft was used to get second derivation spectra. The areas under the bands associated with a particular substructure were manually computed from the infrared second derivative amide spectra to determine their relative amounts.
Antibacterial activity of biologically active compounds isolated from red kidney bean seeds
The antibacterial activities of red kidney beans were evaluated against MDR Enterobacterales. The agar well diffusion assay was performed (Valgas et al., 2007), and the bacterial isolates with inhibition zones diameters less than or equal to 8 mm were deemed susceptible (Choi et al., 2016). The broth microdilution technique was adopted to determine the minimum inhibitory concentrations (MICs) of the studied antimicrobials (Rankin, 2005).
Statistical analysis
All the study experiments were performed in triplicates. Data were edited in Microsoft Excel (Microsoft Corporation, Redmond, WA, USA). Fisher exact test was applied to detect the significant differences in microbial presence in different animal and human sources. Kruskal–Wallis Test was used to compare between the newly tested antimicrobial agents per concentrate (25, 50, 75, and 100%). Figures were fitted by the Graph-Pad Prism software 5.0 (Graph Pad, USA). A p-value of <0.05 is considered statistically different.
Results
Bacteriological analysis and PCR identification of Enterobacterales
In this study, the bacteriological analysis revealed that Enterobacterales isolates were detected in 48 out of 110 examined samples from animal and human sources with a percentage of 43.63%. Microscopical examination of characteristic colonies stained with Gram stain revealed Gram-negative, medium-sized bacilli, non-spore-forming and arranged single, pairs, and in groups. Klebsiella isolates showed mucoid colonies with purple magenta color on the Klebsiella selective agar, large mucoid pink to purple colonies on EMB, and pink mucoid colonies (rapid lactose fermenters) on MacConkey’s agar media. Green metallic sheen colonies on EMB media were characteristic of E. coli and Citrobacter freundii, which were identified using a citrate test that exhibited a negative reaction with E. coli. On XLD agar media, slight transparent reddish-colored colonies with a black center were characteristic of Salmonella or Proteus species. Both showed red slant, yellow butt with H2S production on TSI agar, which were differentiated using a urease test; Salmonella species is urease negative (yellow color), while Proteus is a rapid urease producer (pink color). The O-H serotyping of cultural and biochemically positive E. coli (n = 23) revealed 7 serotypes including O26:H11 (n = 5), O111:H2 (n = 3) O51:H49, O146:H21 (n = 2 each) and O28:H28 (n = 1) for human isolates, O146:H21 (n = 3) and O26:H11, O86:H21 (n = 2 each) for milk isolates and O26:H11 (n = 2) and O55:H7 (n = 1) for meat isolates.
Suspected Enterobacterales isolates were further confirmed by PCR. Twenty-three isolates were positive for the uidA gene giving characteristic bands at 530 bp to be assured as E. coli (Supplementary Figures 1A,B). Seventeen isolates were identified as K. pneumoniae following the PCR-based genus (gyrA) and species-specific identification (K. pneumoniae 16S-23ITS) genes giving characteristic bands at 441 and 130 bp, respectively (Supplementary Figures 2A,B). Whilst, six isolates had the ureR gene supposing them as Proteus mirabilis at characteristic bands of 101 bp (Supplementary Figures 3A,B). Eleven Enterobacterales isolates had the genus-specific invA gene of Salmonella species (248 bp product size), ten of them were identified as Salmonella Typhimurium (fliC gene; 613 bp amplicon), and one was Salmonella Enteritidis (sefC gene; 1104 bp) (Supplementary Figures 4A–C).
Occurrence of Enterobacterales in animal and human sources
As documented in Table 1, the occurrence of Enterobacterales was 43.6% (48/110), which significantly differed between animal (31/80, 38.75%) and human (17/30, 56.67%) sources. A relatively high occurrence rate of Enterobacterales was reported in raw milk (15/25, 60%) followed by fresh meat (7/12, 58.3%), milk products (5/17, 29.4%), and meat products (4/26, 15.3%).
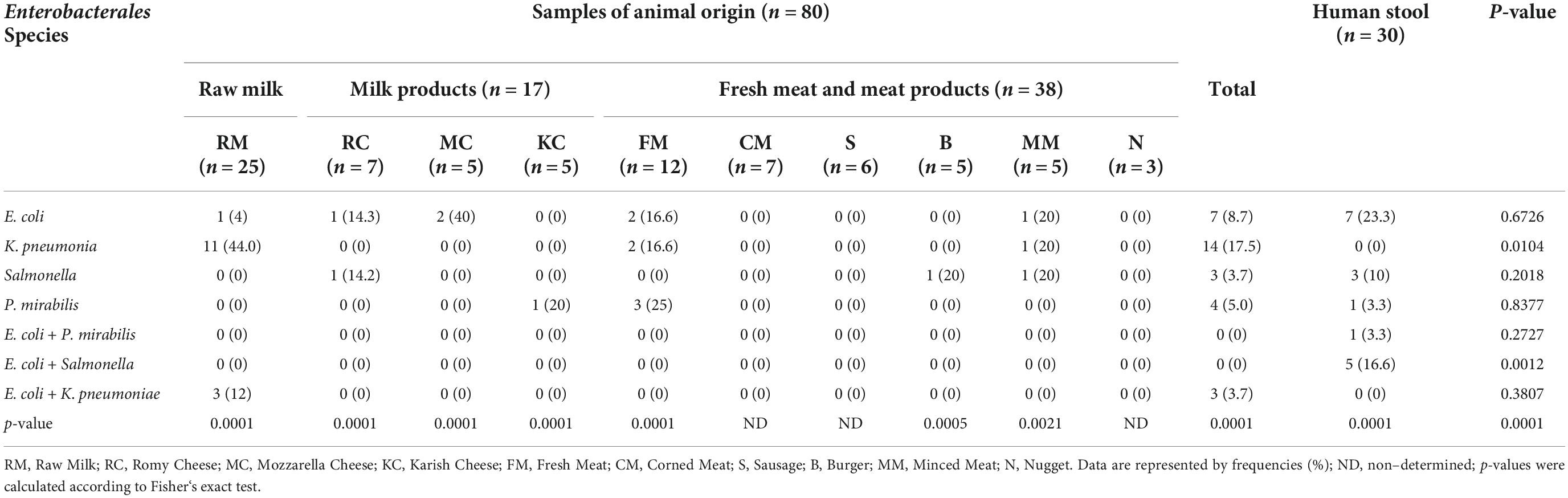
Table 1. Occurrence of Enterobacterales in examined milk and milk products, meat and meat products, and human stools.
Worthy of note, the highest prevalence of Enterobacterales was detected for each of E. coli and K. pneumoniae (14/48, 29.17%), followed by Salmonella (6/48, 12.5), and P. mirabilis (5/48, 10.42) species. Mixed bacterial contamination was reported for E. coli + Salmonella (5/48, 10.42%), E. coli + K. pneumoniae (3/48, 6.25%), and E. coli + P. mirabilis (1/48, 2.08%). Statistical analysis revealed significant differences (P < 0.05) in the occurrence of K. pneumoniae or mixed infection of E. coli with Salmonella in animal and human samples. However, the existence of various Enterobacterales among each animal and human sources showed highly significant variations (P = 0.0001).
Antibiogram of Enterobacterales isolates
Antimicrobial susceptibilities of 57 Enterobacterales isolates to 17 antimicrobials of diverse classes are summarized in Tables 2, 3, and Figure 1. The results declared that all Enterobacterales isolates of animal and human origins showed resistance to amoxicillin-clavulanate (100%), followed by ampicillin (75.44%), erythromycin (71.93%), cefoxitin (70.18%), amoxicillin (66.66%), ceftriaxone (64.91%), and trimethoprim/sulfamethoxazole (56.14). However, a moderate sensitivity level was observed for chloramphenicol (47.37%), followed by ciprofloxacin and tigecycline (40.35 and 42.11%, respectively). Enterobacterales showed a high sensitivity level for imipenem (91.23%). MAR indices of most Enterobacterales isolates were higher than 0.2 (0.235–1.0), except one showed a MAR index of 0.176. Interestingly, 97.92% of Enterobacterales isolates were categorized as MDR. Statistical analysis showed significant variations in the antimicrobial resistance among Enterobacterales species for amoxicillin, ampicillin-sulbactam, cefepime, tigecycline, aztreonam, and doxycycline (P < 0.05).
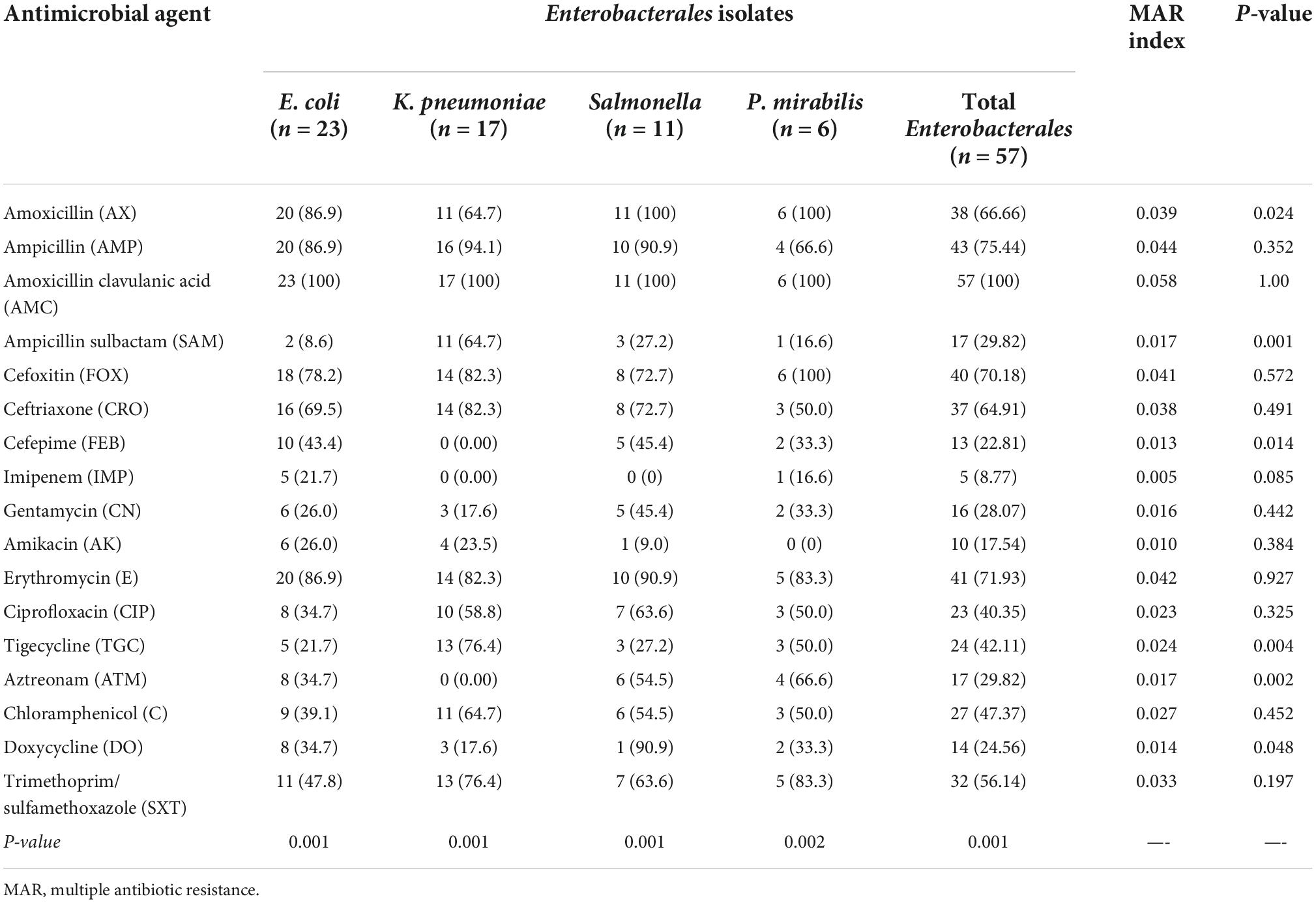
Table 2. Antimicrobial resistance of Enterobacterales isolates (n = 57) recovered from human and animal origins.
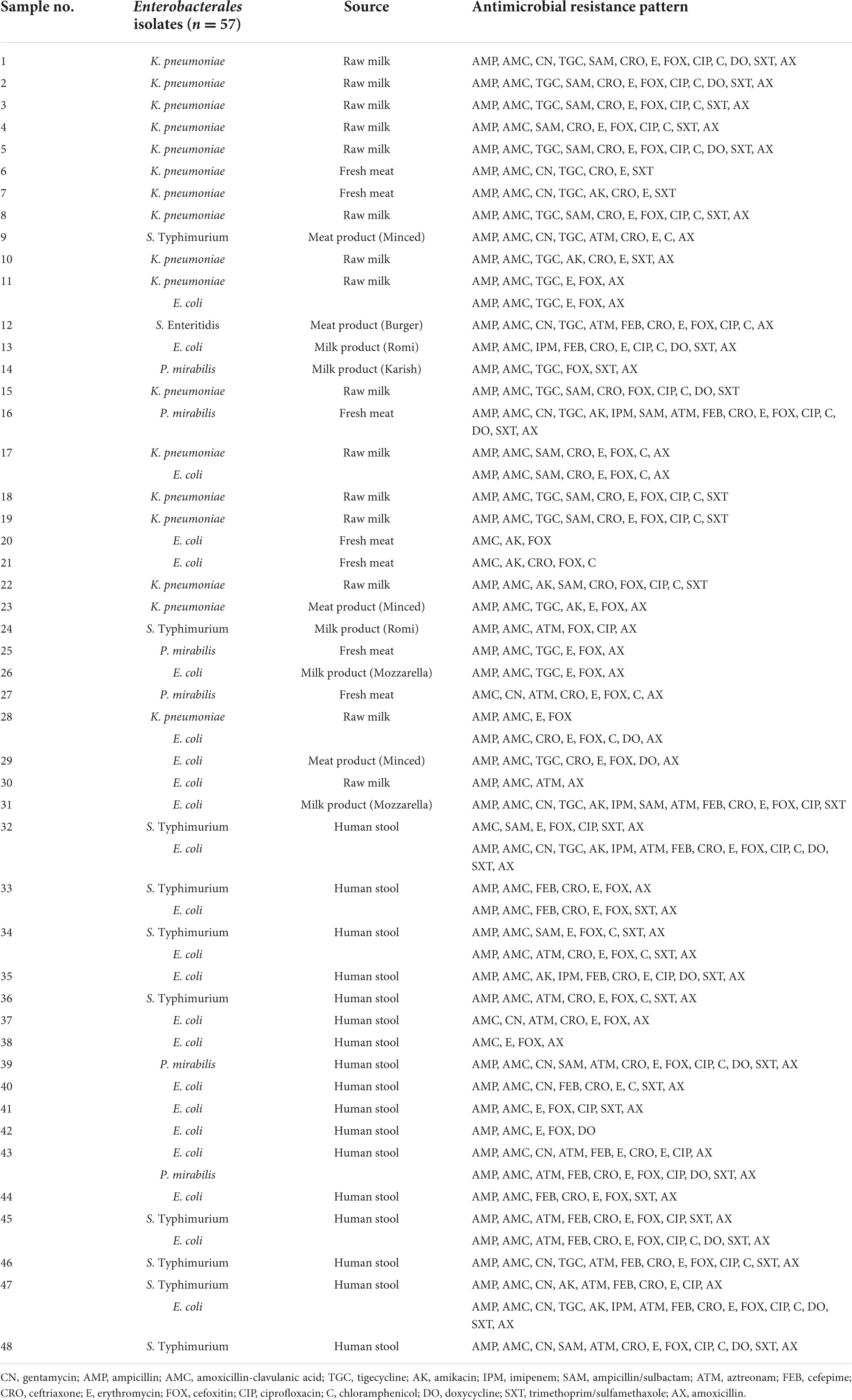
Table 3. Antimicrobial resistance patterns of 57 Enterobacterales isolates recovered from 48 samples of animal and human origins.
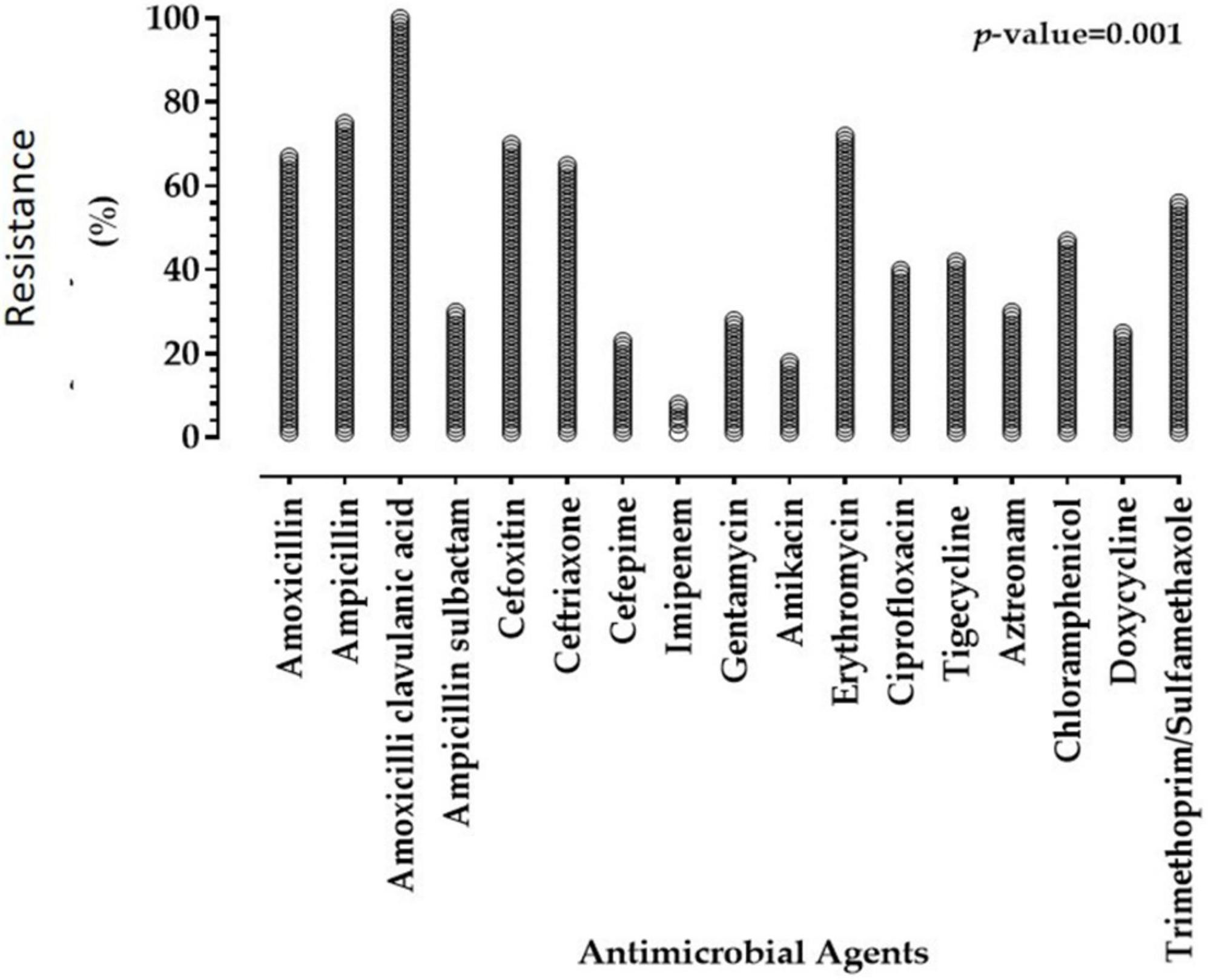
Figure 1. Resistance of Enterobacterales isolates of animal and human origins to antimicrobial agents.
Characterization of red kidney bean seeds phenolic-rich extract
The TPCs and TFCs of the methanolic extract obtained from red kidney bean seeds flour were determined. The TPCs of the extract were 53 ± 2 mg GAE g–1 extract. Meanwhile, the TFCs of the extract were 26 ± 1 mg QE g–1 extract.
A representative HPLC phenolic compounds analysis chromatogram of red kidney bean methanolic extract is presented in Figure 2. Seven peaks of retention times at 3, 4, 5.1, 6.9, 8, 9, and 10.5 min dominated the chromatogram from the separation of red kidney bean methanolic extract. Table 4 listed the phenolic compounds contents (1–7) in the red kidney bean methanolic extract. The highest content is catechol (17.63 μg/mL) followed by caffeic (14.55 μg/mL), pyrogallol (13.12 μg/mL), coumaric (10.60 μg/mL), protocatechulic (6.33 μg/mL), chlorogenic (6.12 μg/mL), and syringenic (5.41 μg/mL).
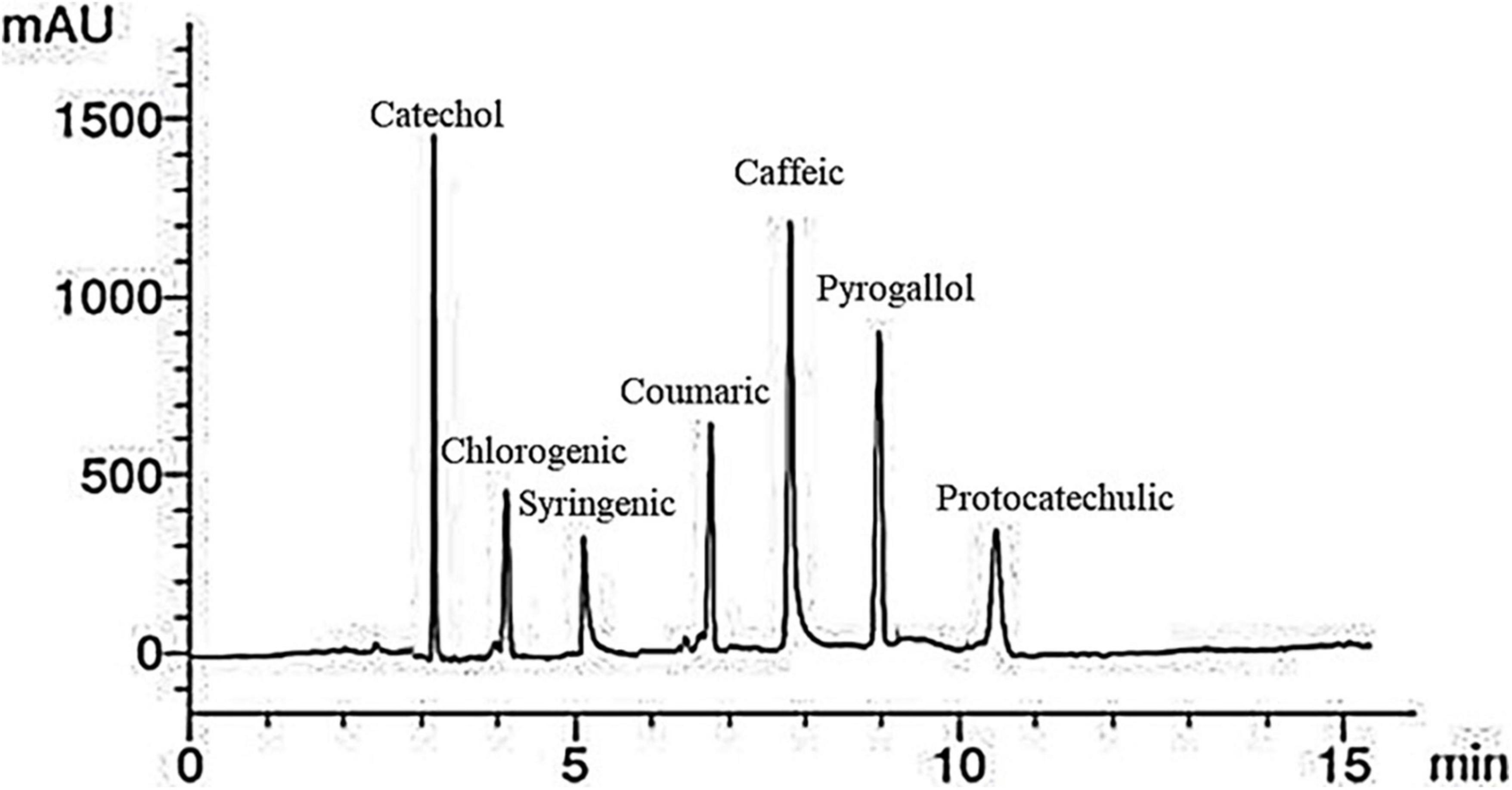
Figure 2. High-performance liquid chromatography-chromatogram of major phenolic compounds in red kidney bean seeds. mAU, milli-absorbance unit.
An illustrative chromatogram of the HPLC flavonoid composites analysis of red kidney bean methanolic extract is publicized in Figure 3. Six peaks with retention times at 3, 5.3, 7, 8, 10, and 12.01 min dominated the chromatogram from the separation of red kidney bean methanolic extract. Table 5 demonstrates the phenolic compound contents (1–6) in the red kidney bean methanolic extract. The highest content is hesperidin (11.37 μg/mL) followed by catechin (10.41 μg/mL), quercetin (9.12 μg/mL), naringin (6.56 μg/mL), kaempferol (4.24 μg/mL), and rutin (1.56 μg/mL).
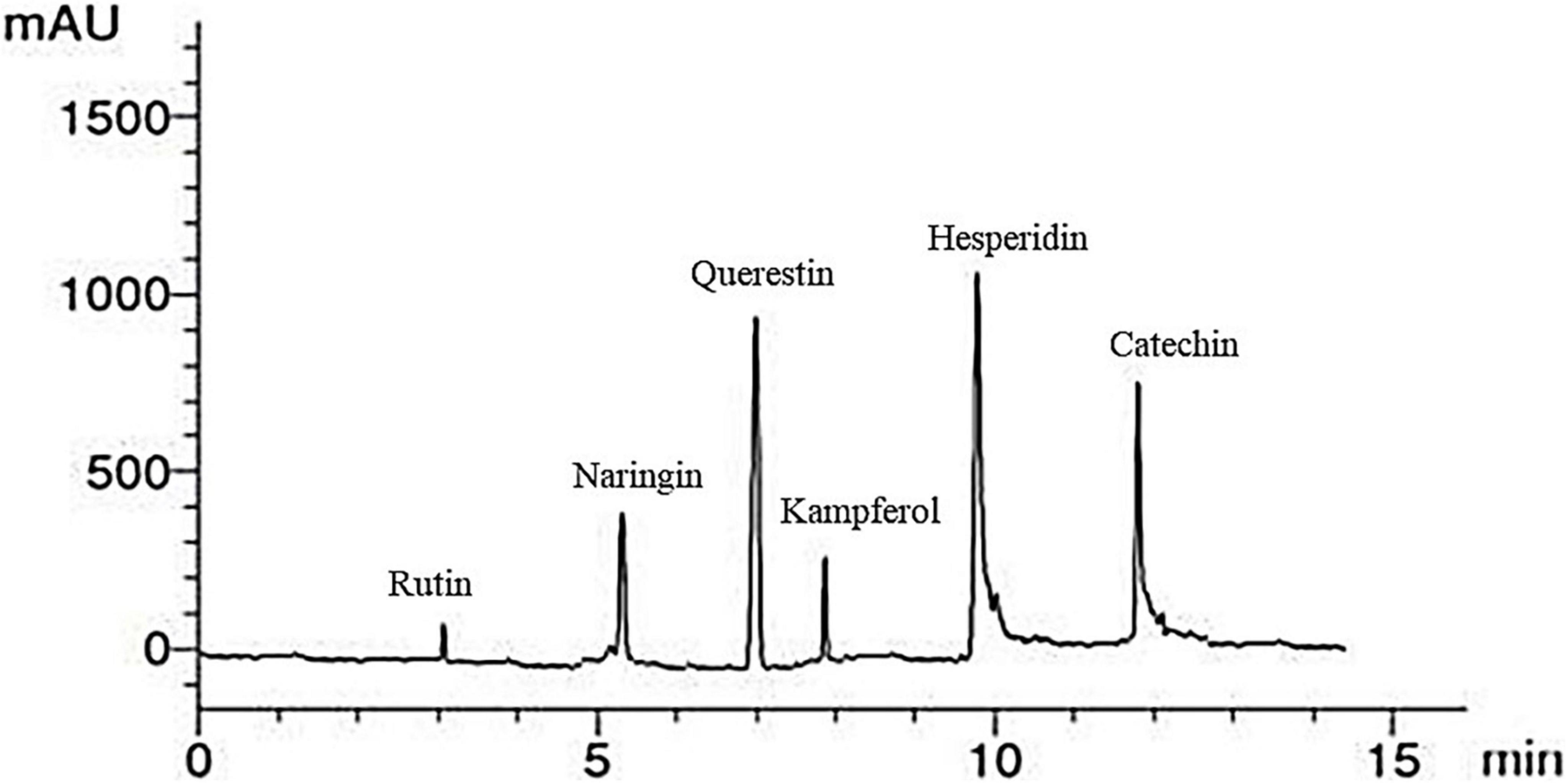
Figure 3. High-performance liquid chromatography-chromatogram of major flavonoid compounds in red kidney bean seeds. mAU, milli-absorbance unit.
Protein characterization
Sodium dodecyl sulfate-polyacrylamide gel electrophoresis patterns of defatted red kidney bean seeds and red kidney beans protein isolate (KPI) are presented in Figure 4A. Both involved two major portions: 7S (vicilin) and 11S (legumin) globulins. The bands matching legumin are 19 (basic subunits) and 22 (acidic subunits) kDa. The bands conforming to vicilin are about 35, 33, and 28 kDa, indicating α/, α, and β subunits, respectively.
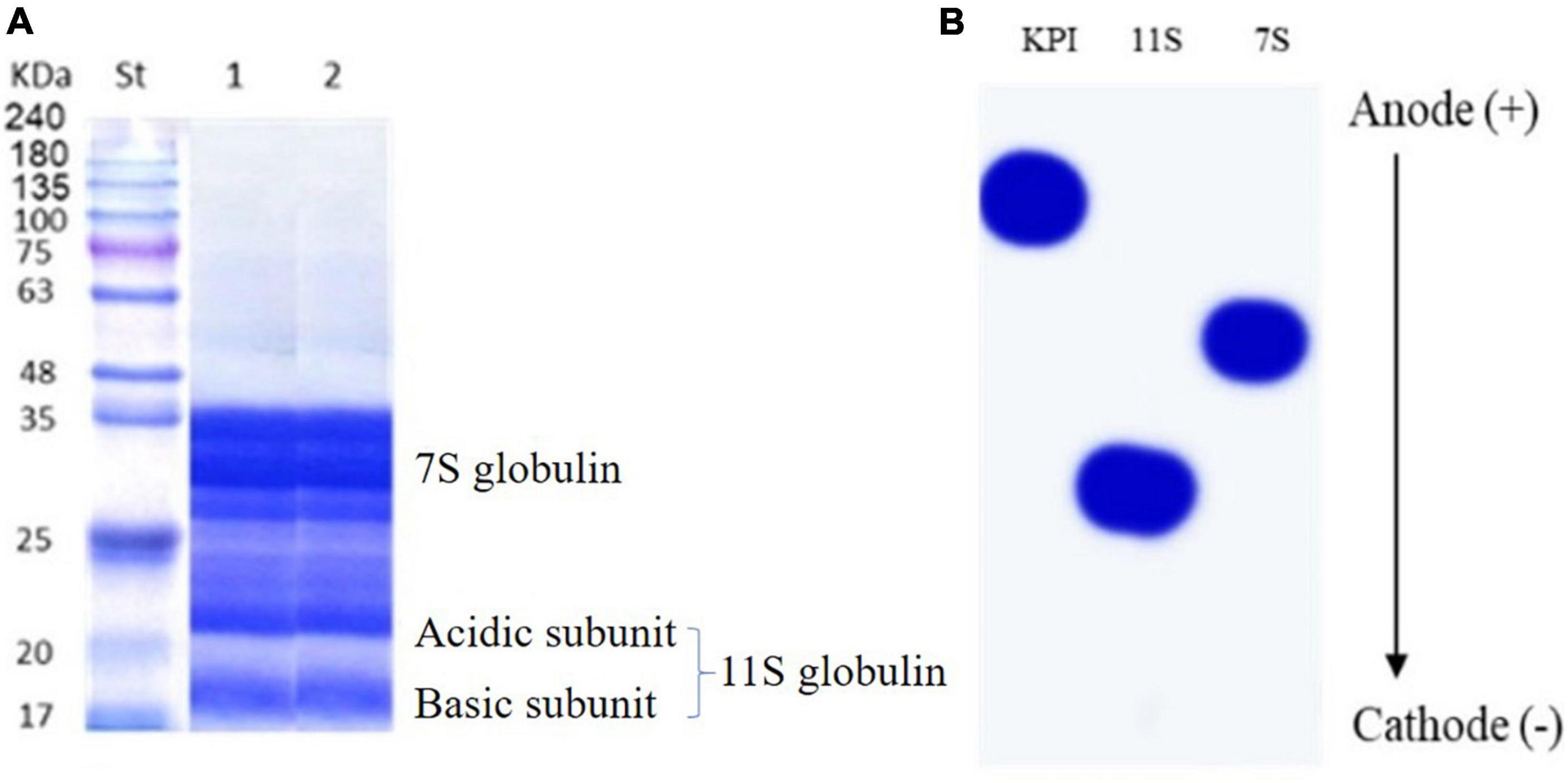
Figure 4. (A) SDS-PAGE of defatted red kidney bean seeds (lane 1) and red kidney bean protein isolate (lane 2) compared to protein marker (St). (B) Urea-PAGE red kidney bean protein isolate (KPI), 11S globulin, and 7S globulin.
Figure 4B shows the urea-PAGE patterns for KPI, 7S, and 11S globulins. The passage in urea-PAGE of 7S and 11S globulins in the cathode direction was substantially faster than their corresponding KPI, representing more positive charges.
The pH solubility profile of KPI, 7S globulin (7S), and 11S globulin (11S) are presented in Figure 5. KPI, 7S, and 11S globulins have broad solubility minima at pH 4.5, 5.5, and 7.5, respectively (Figure 5).
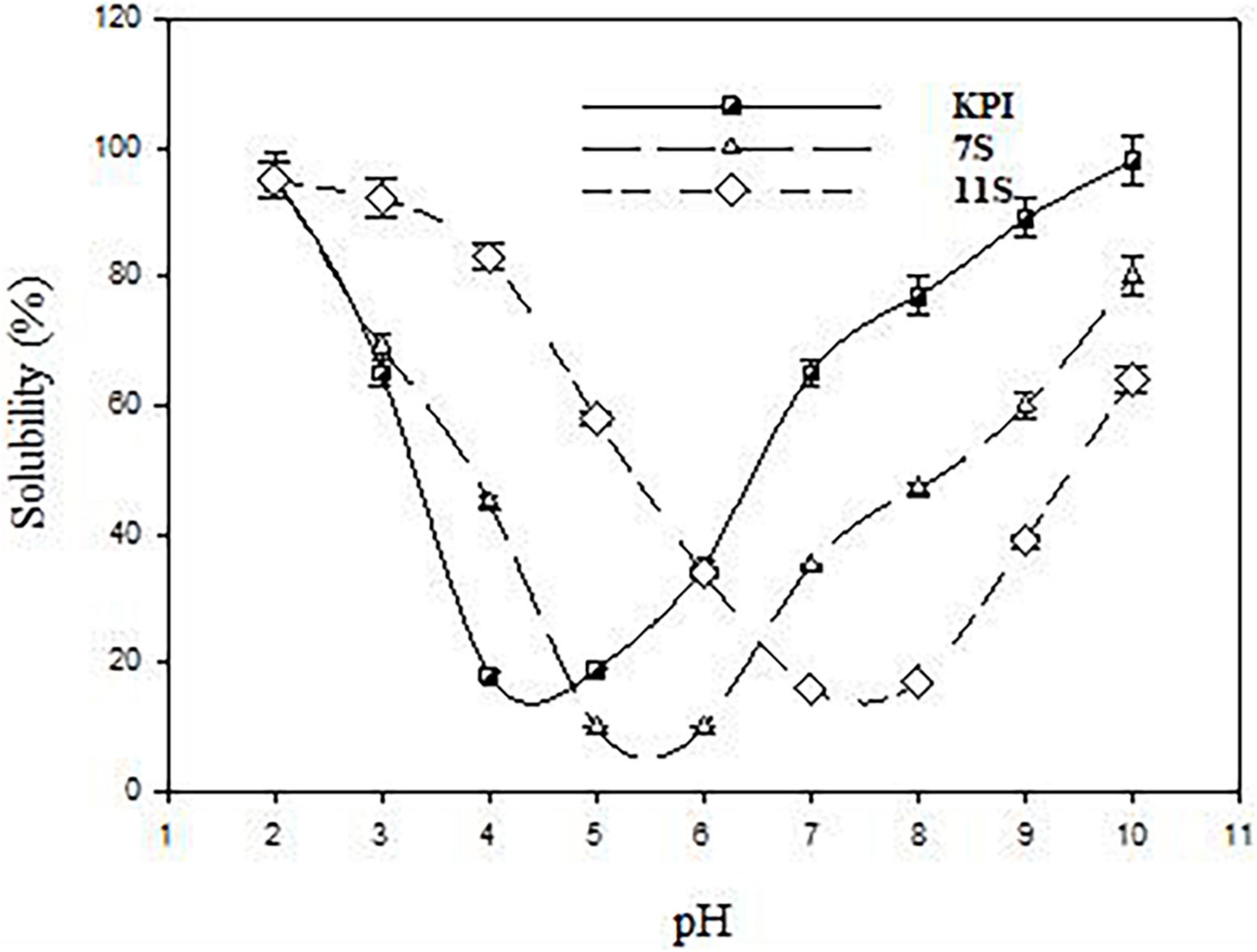
Figure 5. pH solubility profile of red kidney bean protein isolate (KPI), 7S globulin (7S), and 11S globulin (11S).
Fourier transform infrared spectroscopy different spectra of KPI, 7S, and 11S globulins are presented in Figure 6. The amide-I region in the proteins IR spectra (1700–1600 cm–1) is best discernible from their secondary structure including their α-helix, β-sheet, turns, coils, etc. Thus, the amide-I area was assessed to estimate the proportion of various secondary structures in KPI, 7S, and 11S globulins. The primary amide I peak was observed at wave numbers 1636, 1634, and 1620 cm–1 for KPI, 7S, and 11S globulin, respectively.
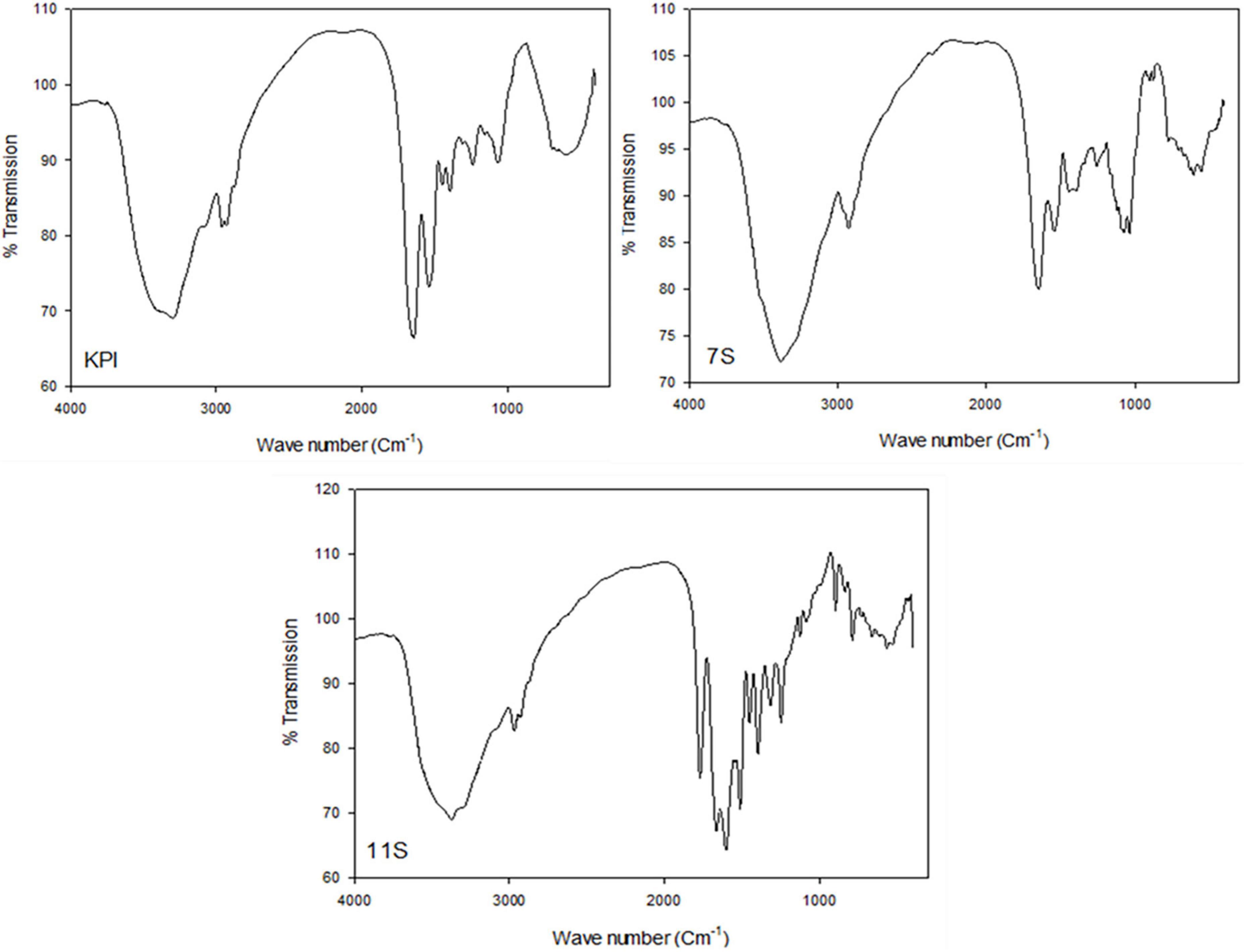
Figure 6. FTIR different spectra of red kidney bean protein isolate (KPI), 7S globulin (7S) and 11S globulin (11S).
Antimicrobial susceptibilities of Phaseolus vulgaris against multidrug-resistant Enterobacterales
Phaseolus vulgaris extracts were examined against MDR Enterobacterales (n = 13) of high MAR indices (0.647–0.941) (Table 6). Our results revealed that the highest activity was reported for protein components in all concentrations (53.85%; inhibition zone diameter mean 17.14 ± 1.51 – 28.14 ± 1.48; MIC value = 0.125–2 μg/mL) followed by 30.77% for each of 7S globulin [inhibition zone diameter mean 16.75 ± 1.72 – 27.75 ± 1.59; MIC value = 0.5–2 μg/mL and 11S globulin (inhibition zone diameter mean 13.67 ± 1.76 – 24.33 ± 1.85; MIC value = 0.5-2 μg/mL)] and finally methanol (15.38%; inhibition zone diameter mean 10.50 ± 0.20 – 21.50 ± 0.20; MIC value = 2 μg/mL). Statistically, significant differences (P < 0.05) were reported between zone diameters of different concentrations of all antimicrobial agents for P. mirabilis isolates. Whereas, significant variations were observed for all antimicrobial agents except 11S globulin in tested K. pneumoniae isolates. Regarding E. coli, significant differences were noted only between different concentrations of 11S globulin and methanolic extract of RKP, while the antibacterial activity of the total protein components and 11S globulin differed statistically in examined Salmonella species. The MIC results of all antimicrobials differed statistically (P < 0.05) for tested Enterobacterales species except for P. mirabilis (P > 0.05).
Discussion
Antimicrobial-resistant bacteria denote a significant concern influencing veterinary medicine and public health (Adams et al., 2018). It is crucial to evaluate the efficacy of antimicrobial stewardship programs as well as practices of infection prevention and control, especially in critically diseased patients (Alkofide et al., 2020). This study was designed to explore the antimicrobial resistance of Enterobacterales recovered from animal and human origins as well as alternative approaches for resistance mitigation.
Herein, Enterobacterales were recovered from 38.75% of analyzed samples from animal sources. Although milk is high-quality nutritional food, it is considered a medium for the growth, multiplication, and transfer of many microbes to humans (Donkor et al., 2007).
The percentage of Enterobacterales in raw milk was 60%, which was almost similar to what was conveyed previously (36%) (Tartor et al., 2021), higher than that reported by El-Mokadem et al. (2020) (14.28%) but lower than that obtained by a previous study (Sobeiha et al., 2020) in Egypt (84%). However, the percentage of Enterobacterales in milk products was 29.4%, whereas Canizalez-Roman et al. (2013) reported a nearly similar existence of diarrheic E. coli in dairy products (28.4%). Bacterial contamination of milk and its products could be retrieved from the udder and/or milk handling equipment (Oliver et al., 2005). E. coli recovery from milk indicates fecal contamination and the existence of toxigenic and/or enteropathogenic bacteria, both of which pose public health risks (Ali and Abdelgadir, 2011). These results suggest a need for improvement of milking operations and good manufacturing practices (Costanzo et al., 2020).
A higher probability of microbial contamination of meat samples may be from the intestinal tract or certain animal illnesses resulting in gastrointestinal ruptures that lead to cross-contamination (Gwida et al., 2014). Coliforms can be found in a diversity of environments, including water, soil, and vegetation and are commonly used as an indicator of the hygienic feature of foods. They can be found profusely in the feces of warm-blooded animals. Their existence could indicate other pathogenic microorganisms of fecal origin (Zhao et al., 2001). This is a substantial public health issue around the world, with significant economic implications in developed countries (Abdelmalek et al., 2019). In the present work, the existence of Enterobacterales in meat and its products was 25.5%. This aligns with previous research (Enayat et al., 2012; Elhawary et al., 2016) in which Enterobacterales isolates were detected in meat samples with percentages of 67 and 54%, respectively. However, Abd El-Aziz et al. (2018) reported Enterobacterales in 80.33% of examined meat samples. The variations in the results obtained by different investigators may be due to differences in handling, manufacturing practices, and the time of exposure.
In the current study, Enterobacterales isolates were recovered from human stools with a percentage of 56.6%, with a predominance of E. coli (40%) either single or mixed with Salmonella species, whereas no Klebsiella species were isolated. Previously, a lower prevalence of Enterobacterales (10.8%) among patients diagnosed with gastrointestinal infection was recorded (Rustam et al., 2006). Meanwhile, 22.7% of human stools were positive for K. pneumonia lately in Egypt (Elmonir et al., 2021). However, Salmonella infections were previously reported in human stools; 4% (Abd El-Aziz et al., 2021) and 44% (Kasumba et al., 2021). A negative stool culture may be attributed to the reason that Enterobacterales isolates were not present in sufficient numbers to be detected. Moreover, Tarr et al. (2005) documented that enterohemorrhagic E. coli are difficult to be identified in human stools in late infection and the recovery rate decreased to 33.3% in stools collected more than 6 days after the outset of diarrhea.
Infections occurred by antibiotic-resistant Gram-negatives, mainly those developed in hospitals, pose serious threat to global public health (Exner et al., 2017; Elmowalid et al., 2018; Abd El-Aziz et al., 2020; Elmonir et al., 2021). Antimicrobial resistance represents a global crisis that endangers the efficacy of antibiotics and consequently the efficiency of treatment provided. The mortality rate associated with drug resistance is substantial, adding to the burden of infectious diseases (Livermore, 2009; De Kraker et al., 2011).
In this study, among 57 tested Enterobacterales isolates from both human and animal sources, antimicrobial resistance was mostly observed for amoxicillin-clavulanate (100%), ampicillin (75.44%), erythromycin (71.93%), cefoxitin (70.18%), amoxicillin (66.66%), ceftriaxone (64.91%), and trimethoprim/sulfamethoxazole (56.14%). However, a moderate sensitivity level was observed for chloramphenicol (47.37%), followed by ciprofloxacin and tigecycline (40.35 and 42.11%, respectively). In previous reports, high resistance levels were detected for the abovementioned antimicrobial agents (Abd El-Aziz and Gharib, 2015; Elmonir et al., 2021; Kotb et al., 2022).
Kidney beans‘ health advantages are attributable to their particular phytochemicals and nutrients. Kidney beans are rich in protein, dietary fiber, minerals, carbohydrates, and phytochemicals, containing phenolic compounds (Kan et al., 2017). Bean phenolic antioxidants have been demonstrated to affect biomarkers linked to health and help to prevent chronic diseases (Redan et al., 2013). The anti-inflammatory, antioxidant, anti-atherosclerotic, anticancer, antihypertensive, and antiaging properties of phenolic chemicals found in kidney beans have been demonstrated (García-Lafuente et al., 2014). Polyphenolic chemicals generated from plant tissue have been shown to offer health benefits in several studies (Aboghoniem et al., 2022; Darwesh et al., 2022). This study proved that red kidney bean polyphenols had antibacterial properties against Enterobacterales. The highest activity was reported for protein components in all concentrations (53.85%; inhibition zone diameter mean 17.14 ± 1.51–28.14 ± 1.48; MIC value = 0.125–2 μg/mL) followed by 7S globulin and 11S globulin (30.77% each) and finally methanol (15.38%). These findings demonstrate new visions into the antimicrobial potential of red kidney beans and propose an auspicious therapeutic action against MDR Enterobacterales, while no available data document this investigation.
Conclusion
This is a comprehensive study on the antibacterial action of red kidney beans (Phaseolus vulgaris L.) against Enterobacterales isolated from human and animal sources. The overall occurrence rate of Enterobacterales significantly differed between animal and human sources. Antimicrobial susceptibility results declared that Enterobacterales isolates were highly resistant to different broad-spectrum antibiotics. The protein component of red kidney bean seeds was the most effective extract, followed by 7S and 11S globulin, exhibiting their potential as promising alternatives for improving future dosing strategies against Enterobacterales as a first report.
Data availability statement
The datasets presented in this study can be found in online repositories. The names of the repository/repositories and accession number(s) can be found in the article/Supplementary material.
Ethics statement
Ethical approval was not provided for this study on human participants because the study was conducted following the Ethics of the World Medical Association (Declaration of Helsinki). Written informed consent was obtained from the patients for participation in this study. We collected human stool samples for the study. The patients/participants provided their written informed consent to participate in this study.
Author contributions
AE and NKA contributed similarly to the study’s design and conception. WH, DHMA, DE-H, and EE conceived the work and contributed to the design. AE, NKA, EE, WH, DHMA, DE-H, and AO participated in the data interpretation. AE, NKA, and AO typed the draft of the manuscript. All authors revised the manuscript and approved the last version.
Acknowledgments
Many thanks to the Faculty of Veterinary Medicine, Zagazig University, Egypt for supporting the research’s authors. We also acknowledge the support from Princess Nourah bint Abdulrahman University Researchers Supporting Project number (PNURSP2022R15), Princess Nourah Bint Abdul Rahman University, Riyadh, Saudi Arabia.
Conflict of interest
The authors declare that the research was conducted in the absence of any commercial or financial relationships that could be construed as a potential conflict of interest.
Publisher’s note
All claims expressed in this article are solely those of the authors and do not necessarily represent those of their affiliated organizations, or those of the publisher, the editors and the reviewers. Any product that may be evaluated in this article, or claim that may be made by its manufacturer, is not guaranteed or endorsed by the publisher.
Supplementary material
The Supplementary Material for this article can be found online at: https://www.frontiersin.org/articles/10.3389/fmicb.2022.1035586/full#supplementary-material
References
Abbas, E., Osman, A., and Sitohy, M. Z. (2020). Biochemical control of Alternaria tenuissima infecting post-harvest fig fruit by chickpea vicilin. J. Sci. Food Agric. 100, 2889–2897. doi: 10.1002/jsfa.10314
Abd El-Aziz, N. K., Ammar, A. M., Hamdy, M. M., Gobouri, A. A., Azab, E., and Sewid, A. H. (2020). First report of aacC5-aadA7Δ4 gene cassette array and phage tail tape measure protein on class 1 integrons of Campylobacter species isolated from animal and human sources in Egypt. Animals 10:2067. doi: 10.3390/ani10112067
Abd El-Aziz, N. K., and Gharib, A. A. (2015). Coexistence of plasmid-mediated quinolone resistance determinants and AmpC-Beta-lactamases in Escherichia coli strains in Egypt. Cell. Mol. Biol. 61, 29–35. doi: 10.14715/cmb/2015.61.5.5
Abd El-Aziz, N. K., Tartor, Y. H., Gharib, A. A., and Ammar, A. M. (2018). Propidium monoazide quantitative real-time polymerase chain reaction for enumeration of some viable but non culturable foodborne bacteria in meat and meat products. Foodborne pathog. Dis. 15, 226–234. doi: 10.1089/fpd.2017.2356
Abd El-Aziz, N. K., Tartor, Y. H., Gharieb, R. M. A., Erfan, A. M., Khalifa, E., Said, M. A., et al. (2021). Extensive drug-resistant Salmonella enterica isolated from poultry and humans: Prevalence and molecular determinants behind the co-resistance to ciprofloxacin and tigecycline. Front. Microbiol. 12:738784. doi: 10.3389/fmicb.2021.738784
Abdelmalek, S. H., Kadry, M., Elshafiee, E. A., Hamed, W., Moussa, I. M., Al-Maary, K. S., et al. (2019). Occurrence of Salmonella infection and antimicrobial susceptibility for local Salmonella isolates from different sources in a cross-sectional study. Int. J. Food Saf. 8:8525. doi: 10.4081/ijfs.2019.8525
Abdel-Shafi, S., Al-Mohammadi, A. R., Osman, A., Enan, G., Abdel-Hameid, S., and Sitohy, M. (2019a). Characterization and antibacterial activity of 7s and 11s globulins isolated from cowpea seed protein. Molecules 24:1082. doi: 10.3390/molecules24061082
Abdel-Shafi, S., Al-Mohammadi, A. R., Sitohy, M., Mosa, B., Ismaiel, A., Enan, G., et al. (2019b). Antimicrobial activity and chemical constitution of the crude, phenolic-rich extracts of Hibiscus sabdariffa, Brassica oleracea and Beta vulgaris. Molecules 24:4280. doi: 10.3390/molecules24234280
Aboghoniem, A. M., Youssef, M. A., Darwesh, O. M., Gaballah, S. T., and Rabie, S. T. (2022). Microwave-assisted preparation of fatty acid esters based eco-friendly plasticizers for biologically-active thiazole-functionalized PVC. J. Vinyl. Addit. Technol. 2022, 1–16. doi: 10.1002/vnl.21931
Adams, R. J., Kim, S. S., Mollenkopf, D. F., Mathys, D. A., Schuenemann, G. M., Daniels, J. B., et al. (2018). Antimicrobial-resistant Enterobacteriaceae recovered from companion animal and livestock environments. Zoonoses Public Health 65, 519–527. doi: 10.1111/zph.12462
Ali, A. A., and Abdelgadir, W. (2011). Incidence of Escherichia coli in raw cow’s milk in Khartoum State. Br. J. Dairy Sci. 2, 23–26.
Alkofide, H., Alhammad, A. M., Alruwaili, A., Aldemerdash, A., Almangour, T. A., Alsuwayegh, A., et al. (2020). Multidrug-resistant and extensively drug-resistant Enterobacteriaceae: Prevalence, treatments, and outcomes - A Retrospective Cohort Study. Infec. Drug Resist. 13, 4653–4662. doi: 10.2147/IDR.S283488
Atallah, O. O., Osman, A., Ali, M. A., and Sitohy, M. Z. (2021). Soybean β -conglycinin and catfish cutaneous mucous p22 glycoproteins deteriorate sporangial cell walls of Pseudoperonospora cubensis and suppress cucumber downy mildew. Pest. Manag. Sci. 77, 3313–3324. doi: 10.1002/ps.6375
Bakal, S. N., Bereswill, S., and Heimesaat, M. M. (2017). Finding novel antibiotic substances from medicinal plants-antimicrobial properties of Nigella sativa directed against multidrug-resistant bacteria. Eur. J. Microbiol. Immunol. 7, 92–98. doi: 10.1556/1886.2017.00001
Bi, S., Tang, S., Wu, X., and Chen, S. (2013). Quantitative detection of Proteus species by real-time polymerase chain reaction using SYBR Green. Ann. Microbiol. 63, 1205–1208. doi: 10.1007/s13213-012-0519-3
Brisse, S., and Verhoef, J. (2001). Phylogenetic diversity of Klebsiella pneumoniae and Klebsiella oxytoca clinical isolates revealed by randomly amplified polymorphic DNA, gyrA and parC gene sequencing and automated ribotyping. Int. J. Syst. Evol. Microbiol. 51, 915–924. doi: 10.1099/00207713-51-3-915
Canizalez-Roman, A., Gonzalez-Nuñez’s, E., Vidal, J. E., Flores-Villaseñor, H., and León-Sicairos, N. (2013). Prevalence and antibiotic resistance profiles of diarrheagenic Escherichia coli strains isolated from food items in Northwestern Mexico. Int. J. Food Microbiol. 164, 36–45. doi: 10.1016/j.ijfoodmicro.2013.03.020
Chobert, J. M., Touati, A., Bertrand-Harb, C., Dalgalarrondo, M., Nicolas, M.-G., and Haertle, T. (1991). In vitro proteolysis and functional properties of reductively alkylated β-casein derivatives. J. Dairy Res. 58, 285–298. doi: 10.1017/s0022029900029861
Choi, O., Choi, S. K., Kim, J., Park, C. G., and Kim, J. (2016). In vitro antibacterial activity and major bioactive components of Cinnamomum verum essential oils against cariogenic bacteria. Streptococcus mutans and Streptococcus sobrinus. Asian Pac. J. Trop Biomed. 6, 308–314. doi: 10.1016/j.apjtb.2016.01.007
Clinical and Laboratory Standards Institute [CLSI] (2020). Performance standards for antimicrobial susceptibility testing, 30th Edn. Wayne, PA: Clinical and Laboratory Standards Institute.
Costanzo, N., Ceniti, C. A., Santoro, A., Clausi, M. T., and Casalinuovo, F. (2020). Foodborne pathogen assessment in raw milk cheeses. Int. J. Food Sci. 2020:3616713. doi: 10.1155/2020/3616713
Darwesh, O. M., Mahmoud, R. H., Abdo, S. M., and Marrez, D. A. (2022). Isolation of Haematococcus lacustris as source of novel anti-multi-antibiotic resistant microbes agents; fractionation and identification of bioactive compounds. Biotechnol Rep (Amst) 35:e00753. doi: 10.1016/j.btre.2022/e00753
De Kraker, M. E., Davey, P. G., and Grundmann, H. (2011). Group, B.S. mortality and hospital stay associated with resistant Staphylococcus aureus and Escherichia coli bacteremia: Estimating the burden of antibiotic resistance in Europe. PLoS Med. 8:e1001104. doi: 10.1371/journal.pmed.1001104
Donkor, E. S., Aning, K. G., and Quaye, J. (2007). Bacterial contaminations of informally marketed raw milk in Ghana. Ghana Med. J. 41, 58–61. doi: 10.4314/gmj.v41i2.55302
Duffy, G., Cagney, C., Crowley, H., and Sheridan, J. J. (2003). A nationwide surveillance study on E. coli 0157:H7 and Enterobacteriaceae in Irish minced beef products. Nat. Food Centre Res. Rep. 63, 1–18.
Elhawary, S. F., Hassanein, R., Agban, M. N., and Elsayh, K. I. (2016). Effect of thyme extract on some Enterobacteriaceae isolated from some meat products in Assuit City. Int. Clin. Pathol. J. 3:69. doi: 10.15406/icpjl.2016.03.00069
El-Mokadem, E. A., El-Leboudy, A. A., and Amer, A. A. (2020). Occurrence of Enterobacteriaceae in dairy farm milk. Alex. J. Vet. Sci. 64, 66–71. doi: 10.5455/ajvs.254389
Elmonir, W., Abd El-Aziz, N. K., Tartor, Y. H., Moustafa, S. M., Abo Remela, E. M., Eissa, R., et al. (2021). Emergence of colistin and carbapenem resistance in extended-spectrum β-lactamase producing Klebsiella pneumoniae isolated from chickens and humans in Egypt. Biology 10:373. doi: 10.3390/biology10050373
Elmowalid, G. A., Ahmad, A. A. M., Hassan, M. N., Abd El-Aziz, N. K., Abdelwahab, A. M., and Elwan, S. I. (2018). Molecular detection of new SHV β-lactamase variants in clinical Escherichia coli and Klebsiella pneumoniae isolates from Egypt. Comp. Immunol. Microbiol. Infect. Dis. 60, 35–41. doi: 10.1016/j.cimid.2018.09.013
Enayat, K., Mansour, A., Nasrin, B., Mohammad, T., and Hashemzadeh, M. (2012). Antibiotic resistance pattern in bacterial isolates obtained from frozen food samples of animal origin in Sanandaj and Ahvaz. J. Bacteriol. Res. 4, 38–41. doi: 10.5897/JBR12.012
Evans, R. W., and Williams, J. (1980). The electrophoresis of transferrins in urea/polyacrylamide gels. Biochem. J. 189, 541–546.
Exner, M., Bhattacharya, S., Christiansen, B., Gebel, J., Goroncy-Bermes, P., Hartemann, P., et al. (2017). Antibiotic resistance: What is so special about multidrug-resistant gram-negative bacteria? GMS Hyg. Infect. Cont. 12:Doc05. doi: 10.3205/dgkh000290
Faddin, J. F. M. (2000). Biochemical tests for identification of medical bacteria. Philadelphia, PA: Lippincott Williams &Wilkins.
Food and Drug Administration (2007). Food and Drug Administration Amendments Act (FDAAA) of 2007. Available online at: https://www.fda.gov/regulatory-information/selected-amendments-fdc-act/food-and-drug-administration-amendments-act-fdaaa-2007. (accessed on June 10, 2022).
García-Lafuente, A., Moro, C., Manchón, N., Gonzalo-Ruiz, A., Villares, A., Guillamón, E., et al. (2014). In vitro anti-inflammatory activity of phenolic rich extracts from white and red common beans. Food Chem. 161, 216–223. doi: 10.1016/j.foodchem.2014.04.004
Godstime, C. O., and Akpotu, M. O. (2014). A review on the phytochemical and anti-hyperglycaemic properties of the fractionated Anacardium occidentale L. Leaves, seeds and stem barks extracts. IOSR J. Pharmacy 04, 27–32.
Gwida, M., Hotzel, H., Geue, L., and Tomaso, H. (2014). Occurrence of Enterobacteriaceae in raw meat and in human samples from Egyptian retail sellers. Int. Sch. Res. Notices 2014:565671. doi: 10.1155/2014/565671
Halimi, H. A., Seifi, H. A., and Rad, M. (2014). Bovine salmonellosis in northeast of Iran: Frequency, genetic fingerprinting and antimicrobial resistance patterns of Salmonella spp. Asian Pac. J. Trop. Biomed. 4, 1–7. doi: 10.1016/S2221-1691(14)60199-4
Johnson, E. A., and Brekke, C. (1983). Functional properties of acylated pea protein isolates. J. Food Sci. 48, 722–725. doi: 10.1111/j.1365-2621.1983.tb14883.x
Kan, L., Nie, S., Hu, J., Wang, S., Cui, S. W., Li, Y., et al. (2017). Nutrients, phytochemicals and antioxidant activities of 26 kidney bean cultivars. Food Chem. Toxicol. 108, 467–477. doi: 10.1016/j.fct.2016.09.007
Kasumba, I. N., Pulford, C. V., Perez-Sepulveda, B. M., Sen, S., Sayed, N., Permala-Booth, J., et al. (2021). Characteristics of Salmonella recovered from stools of children enrolled in the global enteric multicenter study. Clin. Infect. Dis. 73, 631–641. doi: 10.1093/cid/ciab051
Kebede, T., Gadisa, E., and Tufa, A. (2021). Antimicrobial activities evaluation and phytochemical screening of some selected medicinal plants: An alternative in the treatment of multidrug-resistant microbes. PLoS One 16:e0249253. doi: 10.1371/journal.pone.0249253
Khalid, K. A., Darwesh, O. M., and Ahmed, A. M. A. (2021). Peel essential oils of citrus types and their antimicrobial activities in response to various growth locations. J. Essent. Oil Bear. Plants 24, 480–499. doi: 10.1080/0972060X.2021.1941278
Kotb, A. M. E., Abd El-Aziz, N. K., Elariny, E. Y. T., Yahya, R., Alkhalifah, D. H. M., and Ahmed, R. M. (2022). Synergistic antibacterial potential of 6-pentyl-α-pyrone lactone and zinc oxide nanoparticles against multidrug-resistant Enterobacterales isolated from urinary tract infections in humans. Antibiotics 11:440. doi: 10.3390/antibiotics11040440
Kumar, K., Saklaini, A. C., Singh, S., and Singh, V. P. (2008). “Proceeding of VIIth annual conference of Indian association of veterinary public health specialists (IAVPHS),” in Evaluation of specificity for invA gene PCR for detection of Salmonella spp, (Meghalaya).
Laemmli, U. K. (1970). Cleavage of structural proteins during the assembly of the head of bacteriophage T4. Nature 227, 680–685.
Litty, B., Prakash, R., Harishchandra, S., and Harsh, V. (2013). Optimization and evaluation of a multiplex PCR for simultaneous detection of prominent foodborne pathogens of Enterobacteriaceae. Ann. Microbiol. 63, 1591–1599. doi: 10.1007/s13213-013-0622-0
Livermore, D. M. (2009). Has the era of untreatable infections arrived? J. Antimicrob. Chemother. 64, i29–i36. doi: 10.1093/jac/dkp255
Ma, D. Z., Wang, H. X., and Ng, T. B. (2009). A peptide with potent antifungal and antiproliferative activities from Nepalese large red beans. Peptides 30, 2089–2094. doi: 10.1016/j.peptides.2009.08.017
Magiorakos, A., Srinivasan, A., Carey, R. B., Carmeli, Y., Falagas, M. E., Giske, C. G., et al. (2012). Multidrug-resistant, extensively drug-resistant and pandrug-resistant bacteria: An international expert proposal for interim standard definitions for acquired resistance. Clin. Microbiol. Infect. 18:268281. doi: 10.1111/j.1469-0691.2011.03570.x
Mahgoub, S. A., Sitohy, M. Z., and Osman, A. O. (2013). Counteracting recontamination of pasteurized milk by methylated soybean protein. Food Bioproc. Technol. 6, 101–109. doi: 10.1007/s11947-011-0653-0
Mahgoub, S. A., Osman, A. O., and Sitohy, M. Z. (2016). Impeding Bacillus spore germination in vitro and in milk by soy glycinin during long cold storage. J. Gen. Appl. Microbiol. 62, 52–59. doi: 10.2323/jgam.62.52
Mohammed, M. A., Khalid, I. S., Elsaid, A. E., Asmaa, M. A., and Tomohiro, J. (2014). Occurrence, serotypes and virulence genes of non-O157 Shiga toxinproducing Escherichia coli in fresh beef, ground beef, and beef burger. J. Food Control 37, 182–187. doi: 10.1016/j.foodcont.2013.09.035
Murugkar, H. V., Rahman, H., and Dutta, P. K. (2003). Distribution of virulence genes in Salmonella serovars isolated from man and animals. Indian J. Med. Res. 117, 66–70.
Oliver, S. P., Jayarao, B. M., and Almeida, R. A. (2005). Foodborne pathogens in milk and the dairy farm environment: Food safety and public health implications. Foodborne Pathog. Dis. 2, 115–129. doi: 10.1089/fpd.2005.2.115
Omar, A. E., Al-Khalaifah, H. S., Mohamed, W. A., Gharib, H. S., Osman, A., Al-Gabri, N. A., et al. (2020). Effects of phenolic-rich onion (Allium cepa L.) extract on the growth performance, behavior, intestinal histology, amino acid digestibility, antioxidant activity, and the immune status of broiler chickens. Front. Vet. Sci. 7:728. doi: 10.3389/fvets.2020.582612
Ordonez, A., Gomez, J., and Vattuone, M. (2006). Antioxidant activities of sechium edule (Jacq.) swartz extracts. Food Chem. 97, 452–458. doi: 10.1016/j.foodchem.2005.05.024
Osman, A. O., Mahgoub, S. A., and Sitohy, M. Z. (2013). Preservative action of 11S (glycinin) and 7S (β-conglycinin) soy globulin on bovine raw milk stored either at 4 or 25°C. J. Dairy Res. 80, 174-183. doi: 10.1017/S0022029913000095.
Rankin, I. D. (2005). “MIC testing. Manual of antimicrobial susceptibility testing,” in American society for microbiology, (Washington, DC: Pan American Health Organization), 53–62.
Redan, B. W., Vinson, J. A., and Coco, M. G. Jr. (2013). Effect of thermal processing on free and total phenolics in nine varieties of common beans. Int. J. Food Sci. Nutr. 64, 243–247. doi: 10.3109/09637486.2012.710892
Roy, M., Sarker, A., Azad, A., Shaheb, R., and Hoque, M. (2020). Evaluation of antioxidant and antimicrobial properties of dark red kidney bean (Phaseolus vulgaris) protein hydrolysates. J. Food Meas. Charact. 14, 303–313. doi: 10.1007/s11694-019-00292-4
Rustam, S., Saba, N. U., Qayyum, M., Islam, B. U., and Qazilbash, A. A. (2006). Enterobacteriaceae: Etiological agents of diarrhea. J. Med. Sci. (Faisalabad) 6, 149–154. doi: 10.3923/jms.2006.149.154
Sabry, E., Mohamed, H. A., Ewies, E. F., Kariuki, B. M., Darwesh, O. M., and Bekheit, M. S. (2022). Microwave-assisted synthesis of novel sulfonamide-based compounds bearing α-aminophosphonate and their antimicrobial properties. J. Mol. Struct. 1266:133553. doi: 10.1016/j.molstruc.2022.133553
Scarafoni, A., Magni, C., and Duranti, M. (2007). Molecular nutraceutics as a mean to investigate the positive effects of legume seed proteins on human health. Trends Food Sci. Technol. 18, 454–463. doi: 10.1016/j.tifs.2007.04.002
Severgnini, M., Cremonesi, P., Consolandi, C., De Bellis, G., and Castiglioni, B. (2011). Advances in DNA microarray technology for the detection of foodborne pathogens. Food Bioprocess Technol. 4, 936–953. doi: 10.1007/s11947-010-0430-5
Singleton, V. L., Orthofer, R., and Lamuela-Raventós, R. M. (1999). [14] Analysis of total phenols and other oxidation substrates and antioxidants by means of folin-ciocalteu reagent, Methods in enzymology. Amsterdam: Elsevier, 152–178.
Sitohy, M. Z., Mahgoub, S. A., and Osman, A. O. (2012). In vitro and in situ antimicrobial action and mechanism of glycinin and its basic subunit. Int. J. Food Microbiol. 154, 19-29. doi: 10.1016/j.ijfoodmicro.2011.12.004
Sobeiha, A. M., Al-Hawaryb, I. I., Khalifac, E. M., and Ebied, N. A. (2020). Prevalence of Enterobacteriaceae in raw milk and some dairy products. Kafr. Vet. Med. J. 18, 9-13. doi: 10.21608/KVMJ.2020.39992.1009
Souillac, P. O., Middaugh, C. R., and Rytting, J. H. (2002). Investigation of protein/carbohydrate interactions in the dried state. 2. Diffuse reflectance FTIR studies. Int. J. Pharm. 235, 207-218. doi: 10.1016/s0378-5173(01)00987-5
Tambekar, D. H., Dhanorkar, D. V., Gulhane, S. R., Khandelwal, V. K., and Dudhane, M. N. (2006). Antibacterial susceptibility of some urinary tract pathogens to commonly used antibiotics. Afr. J. Biotechnol. 5, 1562–1565.
Tarr, P. I., Gordon, C. A., and Chandler, W. L. (2005). Shiga-toxin-producing Escherichia coli and haemolytic uraemic syndrome. Lancet 365, 1073-1086.
Tartor, Y. H., Abd El-Aziz, N. K., Gharieb, R. M. A., El Damaty, H. M., Enany, S., Soliman, E. A., et al. (2021). Whole-genome sequencing of Gram-negative bacteria isolated from bovine mastitis and raw milk: The first emergence of colistin mcr-10 and fosfomycin fosA5 resistance genes in Klebsiella pneumoniae in Middle East. Front. Microbiol. 12:770813. doi: 10.3389/fmicb.2021.770813
Terreni, M., Taccani, M., and Pregnolato, M. (2021). New antibiotics for multidrug-resistant bacterial strains: Latest research developments and future perspectives. Molecules 26:2671. doi: 10.3390/molecules26092671
Turton, J. F., Perry, P., Elgohari, S., and Hampton, C. V. (2010). PCR characterization and typing of Klebsiella pneumoniae using capsular type-specific, variable number tandem repeat and virulence gene targets. J. Med. Microbiol. 59, 541–547. doi: 10.1099/jmm.0.015198-0
Valgas, C., Machado de Souza, S., Smânia, E. F. A., and Smânia, A. J. R. (2007). Screening methods to determine antibacterial activity of natural products. Braz. J. Microbiol. 38, 369–380. doi: 10.1590/S1517-83822007000200034
Zhang, W., Niu, Z., Yin, K., Liu, P., and Chen, L. (2013). Quick identification and quantification of Proteus mirabilis by polymerase chain reaction (PCR) assays. Ann. Microbiol. 63, 683–689. doi: 10.1007/s13213-012-0520-x
Zhao, C., Ge, B., De Villena, J., Sudler, R., Yeh, E., Zhao, S., et al. (2001). Prevalence of Campylobacter spp., Escherichia coli and Salmonella Serovars in retail chicken, Turkey, pork, and beef from the greater Washington. Appl. Environ. Microbiol. 67, 5431–5436. doi: 10.1128/AEM.67.12.5431-5436.2001
Keywords: Enterobacterales, red kidney beans, multidrug-resistant, seed storage proteins, 11S globulin, 7S globulin
Citation: Ebrahim AE, Abd El-Aziz NK, Elariny EYT, Shindia A, Osman A, Hozzein WN, Alkhalifah DHM and El-Hossary D (2022) Antibacterial activity of bioactive compounds extracted from red kidney bean (Phaseolus vulgaris L.) seeds against multidrug-resistant Enterobacterales. Front. Microbiol. 13:1035586. doi: 10.3389/fmicb.2022.1035586
Received: 02 September 2022; Accepted: 17 October 2022;
Published: 07 November 2022.
Edited by:
Helal F. Hetta, Assiut University, EgyptReviewed by:
Semanti Ray, Lerner Research Institute, Cleveland Clinic, United StatesOsama M. Darwesh, National Research Centre, Egypt
Copyright © 2022 Ebrahim, Abd El-Aziz, Elariny, Shindia, Osman, Hozzein, Alkhalifah and El-Hossary. This is an open-access article distributed under the terms of the Creative Commons Attribution License (CC BY). The use, distribution or reproduction in other forums is permitted, provided the original author(s) and the copyright owner(s) are credited and that the original publication in this journal is cited, in accordance with accepted academic practice. No use, distribution or reproduction is permitted which does not comply with these terms.
*Correspondence: Norhan K. Abd El-Aziz, norhan_vet@hotmail.com; NKMahmoud@vet.zu.edu.eg
†These authors have contributed equally to this work and share first authorship