- 1State Key Laboratory of Horticultural Crop Germplasm Resources Creation and Utilization of Ministry of Agriculture and Rural Affairs, Institute of Horticulture Research, Anhui Academy of Agricultural Sciences, Hefei, China
- 2College of Life Science, Anhui Agricultural University, Hefei, China
- 3Provincial Resource Database of Wood Rot Edible Mushrooms in Anhui Province, Hefei, China
Biodelignification is widely regarded as a low-efficiency process because it is usually slow and difficult to control. To improve its efficiency and understand its mechanism, the present study analyzed the delignification characteristics of Pleurotus ostreatus grown on a cotton stalk medium. The results demonstrated that all strains of P. ostreatus can selectively degrade the cotton stalk lignin. When cultured in a cotton stalk medium for 60 days, P. ostreatus degraded lignin primarily during its mycelium growth with up to 54.04% lignin degradation and produced laccase and manganese dependent peroxidase with high activity levels at the peaks of 70.17 U/ml and 62.39 U/ml, respectively, but no detectable lignin peroxidase. The results of nuclear magnetic resonance spectroscopy and Fourier transform infrared spectroscopy analyses of significant changes in lignin structure revealed that syringyl (S) lignin units were more degraded than guaiacyl (G) lignin units, with a significantly elevated G/S ratio. The Gas Chromatography-Mass Spectrometer analysis of low-molecular-weight compounds revealed that the delignification resulted in the formation of alcohols, organic acids, benzodiazepines, and alkanes. Identified benzodiazepines implied the degradation of G and S units of lignin. These findings will help to improve the efficiency of biodelignification and expand our understanding of its mechanism.
Introduction
Cotton stalks are a by-product of cotton planting process, and approximately 40 million tons of cotton stalks are produced annually throughout the cotton growing areas in China (Li and Zhang, 2016; Wang and Tuohedi, 2020). Cotton stalks typically contain 45% cellulose, 20% hemicellulose, 21% lignin, and a low percentage of ash (Akpinar et al., 2011; Li et al., 2014; Keshav et al., 2021). Cotton stalks are less expensive than other lignocellulosic materials and are therefore a highly desirable lignocellulosic material. However, lignocellulosic material contains lignin, a highly irregular and insoluble polymer that is covalently linked with hemicellulose and cellulose, making it difficult to be directly utilized in the biological transformation process (Xing et al., 2018; Li et al., 2021).
Compared with numerous physical and chemical delignification methods, biodelignification has several advantages such as mild reaction conditions, high product yields, less energy consumption, and low toxicity (Li and Zhang, 2014). White-rot fungi are the most effective microorganisms for removing lignin from lignocellulosic materials because they secrete lignin-degrading enzymes that catalyze the mineralization of lignin into CO2 (Ahmad et al., 2016; Rouches et al., 2016; Wang et al., 2019a). The ability to produce ligninolytic enzymes of two fungal cultures Pleurotus ostreatus PAU03 and Phanerochaete chrysosporium MTCC 787 were investigated, P. ostreatus and Ph. chrysosporium consortium pretreated wheat straw, rice straw, corn straw and corn cob with 80.3, 73.4, 76.6, and 73% delignification, respectively (Gill et al., 2022). Currently, P. ostreatus is the second largest cultivated mushroom in the world, second only to Agaricus bisporus, because of its ease of growth on various lignocellulosic materials, such as wood, sawdust, and wheat straw (Fernandez-Fueyo et al., 2016). Some researchers have discovered that certain varieties of P. ostreatus could selectively remove lignin from lignocellulose, and they believed that these varieties could be used for delignification in the conversion of lignocellulosic materials into value-added bioproducts such as ruminant feed and bioethanol (Dong et al., 2013; Salame et al., 2014; Suryadi et al., 2022).
There is, nonetheless, some controversies exist surrounding using Pleurotus spp. for delignification. Some Pleurotus spp. preferentially degrade cellulose in lignocellulosic materials, whereas others degrade more lignin than cellulose or hemicellulose in rice straws and wood bits, and still some others degrade both lignin and cellulose in bagasse in equal proportions (Javad et al., 2007; Dong et al., 2013). Therefore, whether P. ostreatus strains selectively degrade cotton stalk lignocellulose is unclear and requires further investigation. Pleurotus spp. are thought to degrade lignin with laccases (LaCs) and manganese dependent peroxidases (MnPs) that they produce (Knop et al., 2014; Xiao et al., 2017; Wang et al., 2019b). However, whether they can produce lignin peroxidases (LiPs) is debatable and has yet to be proven (Asgher et al., 2016). Moreover, the degradability of lignin by using white-rot fungi depends on its content, composition, and structure. Ceriporiopsis subvermispora preferentially degrades the syringyl (S) unit of lignin in genetically-modified poplar (Populus simoni × Populus Nigra) (S, 83.3%), as opposed to the guaiacyl (G) lignin unit of wild poplar (G, 66.7%) (Skyba et al., 2013). Certain P. ostreatus strains preferentially degrade the S unit of sugarcane lignin (a type of monocotyledon lignin) (Dong et al., 2013); consequently, whether they can degrade cotton lignin (a kind of dicotyledon lignin) in the same manner requires further investigation.
To investigate whether P. ostreatus degrades cotton stalk lignin and what is their role in degrading cotton stalk lignin, growth tests of P. ostreatus on cotton straw medium were conducted and the strains with high selectivity factor and strong ability to degrade lignin were selected as well as the ligninolytic characteristics of the strains inoculated in cotton stalk medium were analyzed. At various stages following inoculation, the content of cotton stalk lignin (also known as Klason lignin) was assayed with the sulfuric acid hydrolysis method, whereas the structural changes in lignin polymer and its degradation products were characterized with Fourier transform infrared spectroscopy (FTIR), nuclear magnetic resonance spectroscopy (1H-NMR), and Gas Chromatography-Mass Spectrometer (GC–MS). The findings have guiding significance in improving the efficiency of lignin removal through biodegradation, promoting straw-based cultivation of edible fungi and lignocellulose recycling, providing information about the structural changes in lignin and its degradation products, and helping to expound the mechanism of lignin degradation by using white-rot fungi in nature.
Materials and methods
Fungal strains and inoculation
Eight commercial Pleurotus ostreatus strains, as shown in Table 1, were used in the experiment. These strains were preserved on potato dextrose agar (PDA, boiled juice of 200 g L−1 potato, 20.0 g L−1 glucose, and 15.0 g L−1 agar added to solid medium) plates at 4°C. To prepare the inoculate, the mycelium agar plugs with 5 mm in diameter inoculated in 250 ml tissue bottles with cotton stalk solid medium (5 g dry cotton stalk powder with 60 mesh sieves added 22 ml special culture solution, which contains ammonium tartrate 22.0 g L−1, KH2PO4 20 g L−1, MgSO4·7H2O 13.8 g L−1, CaCl21.0 g L−1, NaCl 0.6 g L−1, MnSO4·H2O 0.35 g L−1, FeSO4·7H2O 60 mg L−1, CoCl2·6H2O 110 mg L−1, ZnSO4·7H2O 60 mg L−1, CuSO4·5H2O 95 mg L−1, AlK(SO4)2·12H2O 6 mg L−1, H3BO3 6 mg L−1, Na2MoO4·2H2O 6 mg L−1, and VB1 100 mg L−1), were cut along the edge of the actively growing colonies, which had been cultivated on PDA plates for 7 days at 25°C.
Determination of cotton stalks degradation
Add 50 ml of neutral detergent to 0.5 g of sample, followed by holding at 100°C for 1 h, filter and wash the sample with acetone aqueous solution, then add 50 ml of 2 M hydrochloric acid solution, hold at 100°C for 50 min, filter the filtrate to determine the hemicellulose content using lichen phenol colorimetric method. The residue was washed with water to neutral and washed twice with acetone, dried at 60°C, then placed in a 50 ml beaker, added 5 ml of 72% H2SO4, hydrolyzed at 20°C for 3 h, then added 45 ml of distilled water, room temperature overnight, the filtered filtrate was used to determine the cellulose content by a colorimetric method using anthrone reagent. The residue was then washed with water to pH 6.5 and the lignin content was obtained by weight of the residue dried at 80°C minus the weight of the ash after 550°C ashing.
Lignin (or cellulose, or hemicellulose) degrading rate (%) = [total lignin (or total cellulose, or total hemicellulose) degraded/total lignin (or total cellulose, or total hemicellulose) contained in the original sample] × 100; selectivity factor = (lignin degradation rate/cellulose degradation rate) × 100.
Measurement of lignin degrading enzyme activity
The samples are removed every 5 days, added to 15 ml of purified water in batches, and extracted overnight under 4°C. Thereafter, vibrating extraction is performed at 200 r/min for 1 h, followed by refrigerated centrifugation at 12,000 r/min for 10 min. Finally, the supernatant was obtained as the enzyme solution. The LaC activity was determined at 25°C by 2,2-azinobis-(3-ethylbenzthiazoline-6-sulfonate) (ABTS) as substrate. The assay mixture contained 2 mM ABTS and 0.1 M sodium-citrate buffer, pH 3,0. Oxidation of ABTS was followed by absorbance increase at 420 nm (ɛ = 36,000 M−1 cm−1) for 1 min. One unit activity is defined as the amount of enzyme that transformed 1 μmol of substrate per min (Ya et al., 2013). Using hydrogen peroxide oxidation, the performance of MnP activity was examined (Lueangjaroenkit et al., 2019). The reaction system was 20 mmol L−1 tartrate-sodium tartrate buffer (pH 4.5) 0.8 ml, 2 mmol L−1 MnSO4 solution 0.05 ml, appropriate amount of enzyme solution, 2 mmol L−1 H2O2 0.05 ml, and the change of absorbance value at 238 nm was measured per time unit. The absorbance value at 238 nm was measured and the change in the absorption spectrum of 0.01 was equal to one unit of enzyme activity. The activity of LiP activity was tested by oxidising veratryl alcohol. The reaction system was 1.85 ml of 0.24 mol L−1 sodium tartrate buffer (pH 3.0), 0.1 ml of 24 mmol L−1 quinacrine and an appropriate amount of enzyme solution. After preheating to 37°C, 0.05 ml of 6 mmol L−1 H2O2 was added to start the reaction, and the absorbance value was measured at 310 nm, and the change in optical density at 310 nm per minute was used as one LiP activity unit (Chandrasekaran et al., 2014). Throughout all the test, sterile water was used to maintain the blanks.
Fourier transform infrared spectroscopy analysis
FTIR analysis was conducted to determine the functional groups that were converted during SCB degradation. The homogeneous and representative wafer samples obtained using KBr Pellets. The micro-FTIR spectra of local areas of the wafer specimen were measured using a NICOLET iN10 MX spectrometer (Thermos Nicolet Corporation, Madison, WI); connected to a Nicolet NicPlan IR microscope and an MCT detector. The spectral range was taken from 4,000 to 650 cm−1 at a resolution of 4 cm−1.
Solid state 1H-NMR analysis
Lignin was extracted from the uncultured substrates and the substrates that were cultured with P. ostreatus after 30 and 60 days, respectively. A total of 20 mg of lignin samples was weighed and dissolved in 2 ml of pyridine: acetic anhydride (1:1) mixture. Nitrogen was charged into the reaction bulb, which was placed in the dark at room temperature for 72 h. After the reaction was completed, the reactant was dripped into diethyl ether until precipitate was formed, after which the solution was centrifuged to separate the precipitate. The precipitate was then washed with diethyl ether six to eight times to remove the pyridine odor from the precipitate. Finally, the completely acetylated lignin was obtained. The acetyl-treated lignin samples were dissolved in 0.5 ml of DMSO, with tetramethylsilane as the internal standard. Finally, the 1H-NMR assay was conducted with a Bruker-400 superconducting NMR spectrometer (Bruker, Switzerland) at a frequency of 400 MHz.
GC–MS analysis of lignin-degrading products
A total of 0.1 g of stalk powders was weighed and added to 1.4 ml of cooled methyl alcohol. The container was then exposed to ultrasonic waves for 30 min and centrifuged for 10 min to remove the precipitate. Add equal volume of trichloromethane to the absorbed supernatant, vortex it for 1 min, and then centrifuge it at high speed. Thereafter, 1 ml of the supernatant is blow-dried with nitrogen. The sample without powders was used as the blank control. The cotton stalk powders that were not used to culture P. ostreatus served as the experimental control, whereas the cotton stalk powders that were used to culture P. ostreatus served as the experimental group. To each group, 60 μl of 20 mg mL−1 hydroxylamine hydrochloride-pyridine solution was added. The containers were then heated under 100°C for 2 h. Then, 60 μl of 20 mg mL−1 BSTFA+TMCS solution was added, and the containers. The container was again placed at 100°C for 2 h. Thereafter, the mixtures were subjected to analysis with a gas chromatograph-mass spectrometer (Agilent789A). Finally, the data were retrieved from the National Institute of Standards and Technology (NITS) spectral library.
Statistical analysis
Three replicate trials were carried out for each sample, and all the experiments were repeated three times. Data were analyzed by one-way analysis of variance (ANOVA) using DPS 9.50 software, and expressed as the means ± standard deviations (SDs). Statistical significance was considered at the p < 0.05 level. All graphs were drawn with Excel 2010 software.
Results
Lignocellulose degradation and selectivity factors of Pleurotus ostreatus strains
After 15 days following inoculation, the cotton stalk lignin-degrading capability varied greatly among the strains. Suping 1 achieved the highest lignin-degrading rate (14.82%), followed by Wanping 1 (13.79%) and Heiping A (13.65%) in that order. Zaoqiu and Tianda300 achieved the lowest lignin-degrading rate (10.57 and 10.81%, respectively), as well as P17, Suping3 and Xingping400 were at intermediate levels (Figure 1A). The degradation rate of hemicellulose was high for all strains ranging from 17.9 to 27.57%, with the lowest being Suping3. Furthermore, the cotton stalk lignin-degrading rates of the strains were higher than their cellulose-degrading rates (Figure 1A). During the 15-days inoculation, P. ostreatus preferentially degraded lignin in the cotton stalks, thereby displaying lignin-specific selectivity. The lignin degradation selectivity of the strains is expressed using the selectivity factor (Figure 1B). Among six strains, Zaoqiu achieved the lowest selectivity factor (1.03), whereas Wanping 1, Heiping A, and Suping 1 achieved higher selectivity factors (>2), and Suping 1 achieved the highest selectivity factor (2.80). Therefore, Zaoqiu and Suping 1 were selected for further study because of the large disparity between their selectivity factor and lignin-degrading rate.
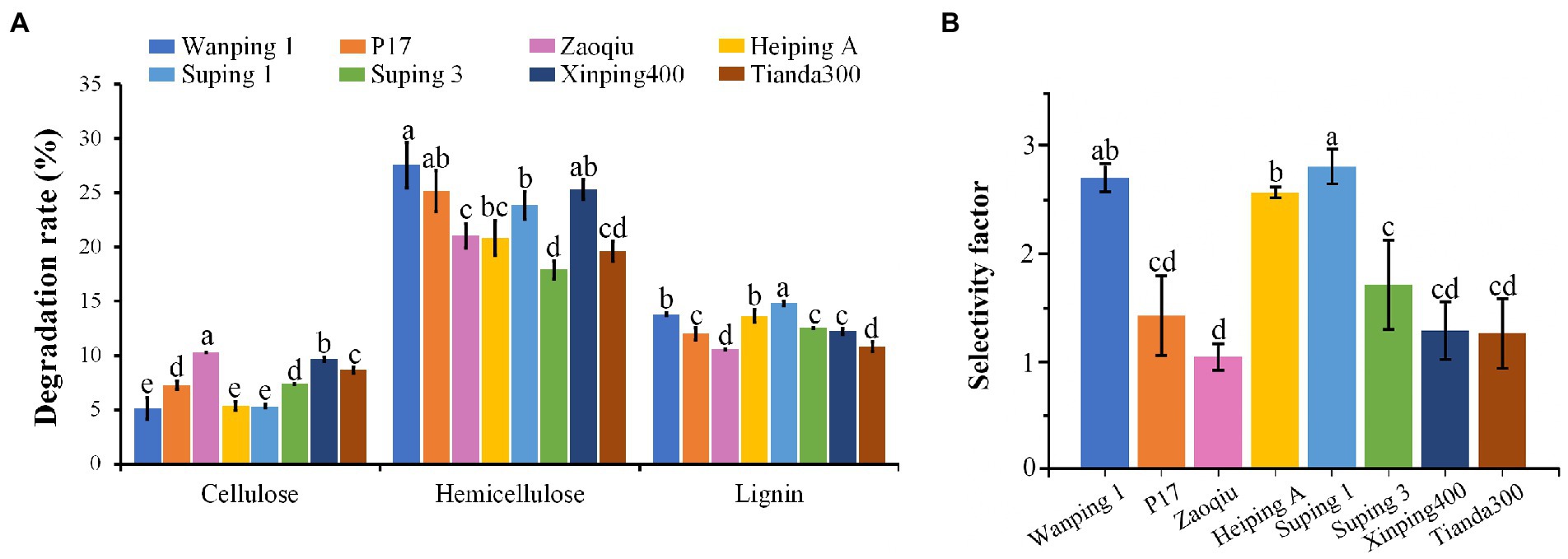
Figure 1. Degradation rates of three major components (cellulose, hemicellulose, and lignin) of cotton stalks using eight P. ostreatus strains and their selectivity factors. (A) Degradation rates of cotton stalks. (B) Selectivity factors of eight P. ostreatus strains. The different letters in the same pillars indicate significant differences according to the LSD at p ≤ 0.05 levels.
Varying lignin content in cotton stalks during degradation
The lignin content of the cotton stalks degraded using the two selected strains (Zaoqiu and Suping 1) decreased gradually as the degradation continued. Moreover, the lignin content of cotton stalks in the medium inoculated with Suping 1 strain was lower than that of Zaoqiu from 25 to 40 days of incubation, which is the most productive stage of mycelial growth and the protoplast stage of fruiting body development (Figure 2A). The results demonstrated that P. ostreatus could decompose lignin and significantly remove lignin from cotton stalks. Suping 1 demonstrated significantly greater lignin degradation capability than Zaoqiu at the stage of fruiting body development, and such a remarkable difference may be caused by the specificity of the strain.
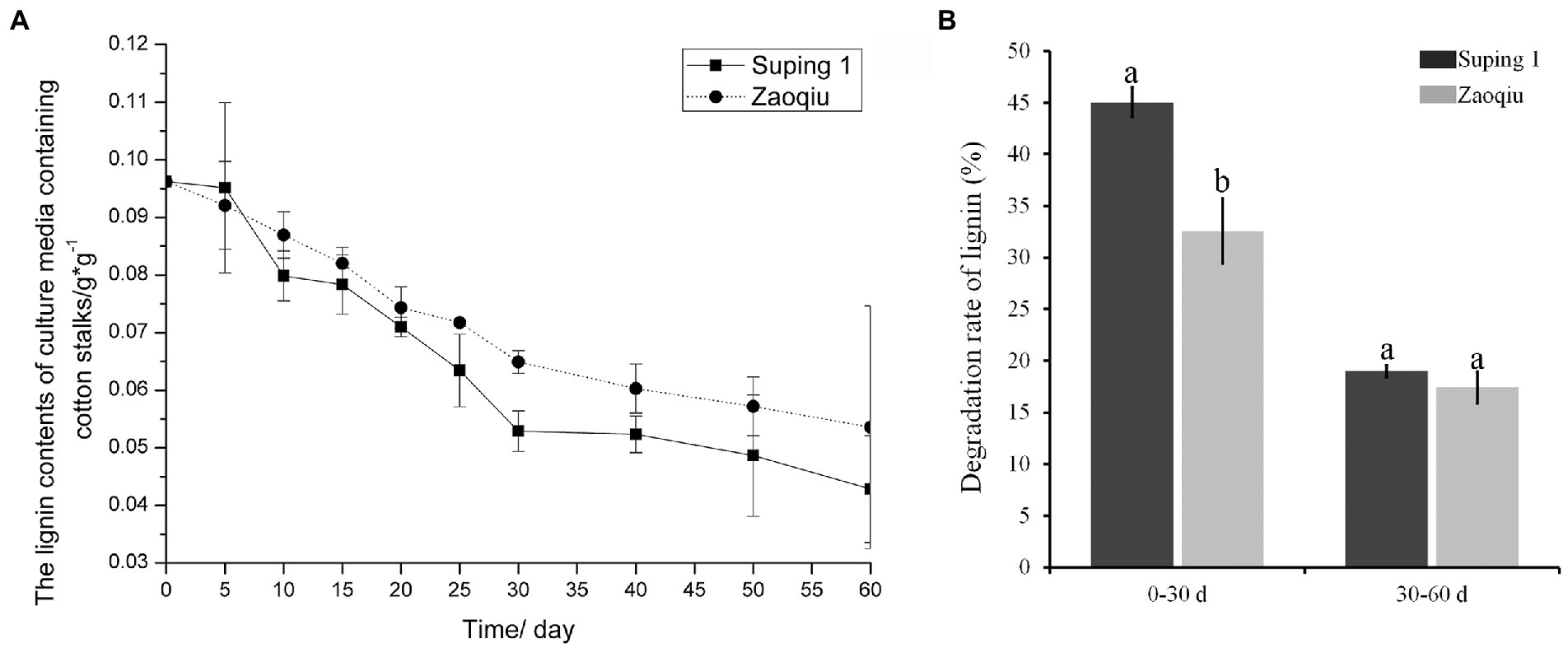
Figure 2. Dynamic monitoring of lignin content in cotton stalk medium inoculated with P. ostreatus strains (Suping 1 and Zaoqiu). (A) Changes of lignin content in cotton stalks medium after inoculation. (B) Degradation rates of cotton stalk lignin by Suping 1 and Zaoqiu at various stages. The different letters in the same pillars indicate significant differences according to the LSD at p ≤ 0.05 levels.
After the cotton stalks were degraded using the two strains for 30 days, the lignin content decreased rapidly in the first 30 days and then gradually in the following 30 days (Figure 2A). On day 30 following inoculation, the lignin-degrading rates of Suping 1 and Zaoqiu were 45.04 and 32.57%, respectively, apparently higher than those from the day 30 to day 60 (19.00 and 17.41%, respectively) (Figure 2B). These results suggest that biodegradation of lignin by P. ostreatus during growth on cotton stalks medium primarily happened in the mycelial growth stages.
Extracellular LaC and MnP activities
The LaC activity produced by Suping 1 and Zaoqiu began to increase rapidly on day 5 following inoculation, peaked at the 10th day after inoculation at 52.47 and 44.34 U mL−1, respectively, and then decreased. On day 20 following inoculation, the LaC activity levels of Suping 1 and Zaoqiu decreased to 29.41 and 31.85 U mL−1, respectively, and then LaC activity of Suping 1 and Zaoqiu started to increase again, reaching a maximum of 70.17 and 57.55 U mL−1, respectively, on day 30 after inoculation. Subsequently, the Lac activity of Suping 1 and Zaoqiu gradually decreased from 30 to 60 days of inoculation (Figure 3A). Similarly, the first peaks of MnP activity of Suping 1 and Zaoqiu was observed at day 20 and day 30 after inoculation with values of 62.39 and 35.61 U mL−1, respectively. The second MnP activity peaks of both Suping 1 and Zaoqiu was observed at the 40th day following inoculation with values of 59.59 and 40.33 U mL−1, respectively (Figure 3B). Furthermore, the MnP activity of Suping 1 was generally greater than that of Zaoqiu at 60 days after inoculation except for day 25, which may explain the stronger lignin degradation ability of Suping 1 than Zaoqiu.
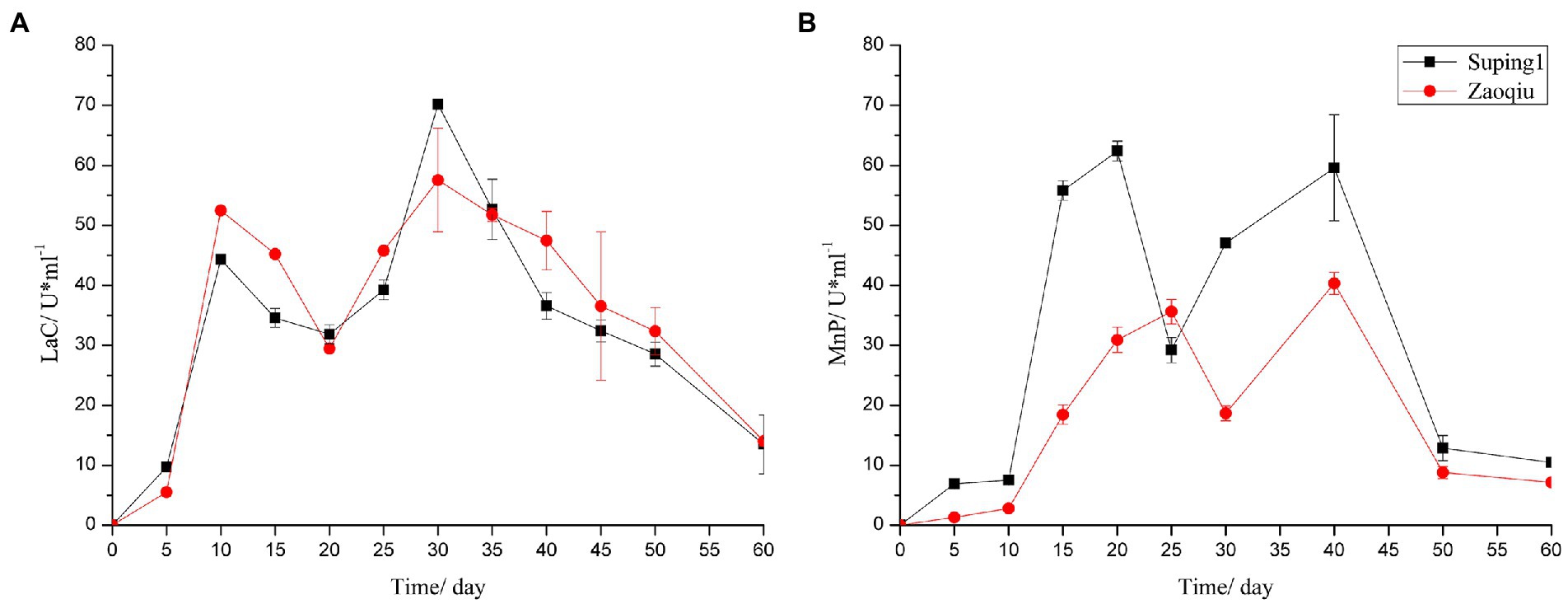
Figure 3. Ligninase enzyme activities of the cotton stalks degraded by P. ostreatus in solid-state fermentation. (A) Laccase; (B) Manganese peroxidase.
FTIR analysis
Because Suping 1 demonstrated a significantly greater lignin degradation capability than Zaoqiu, it was selected as the representative strain to study the modification of lignin structure and the small-molecular-weight metabolite produced using P. ostreatus during lignin removal. Figure 4 shows the results of an infrared spectroscopy analysis on the milled wood lignin (MWL) of the cotton stalks degraded using Suping 1.
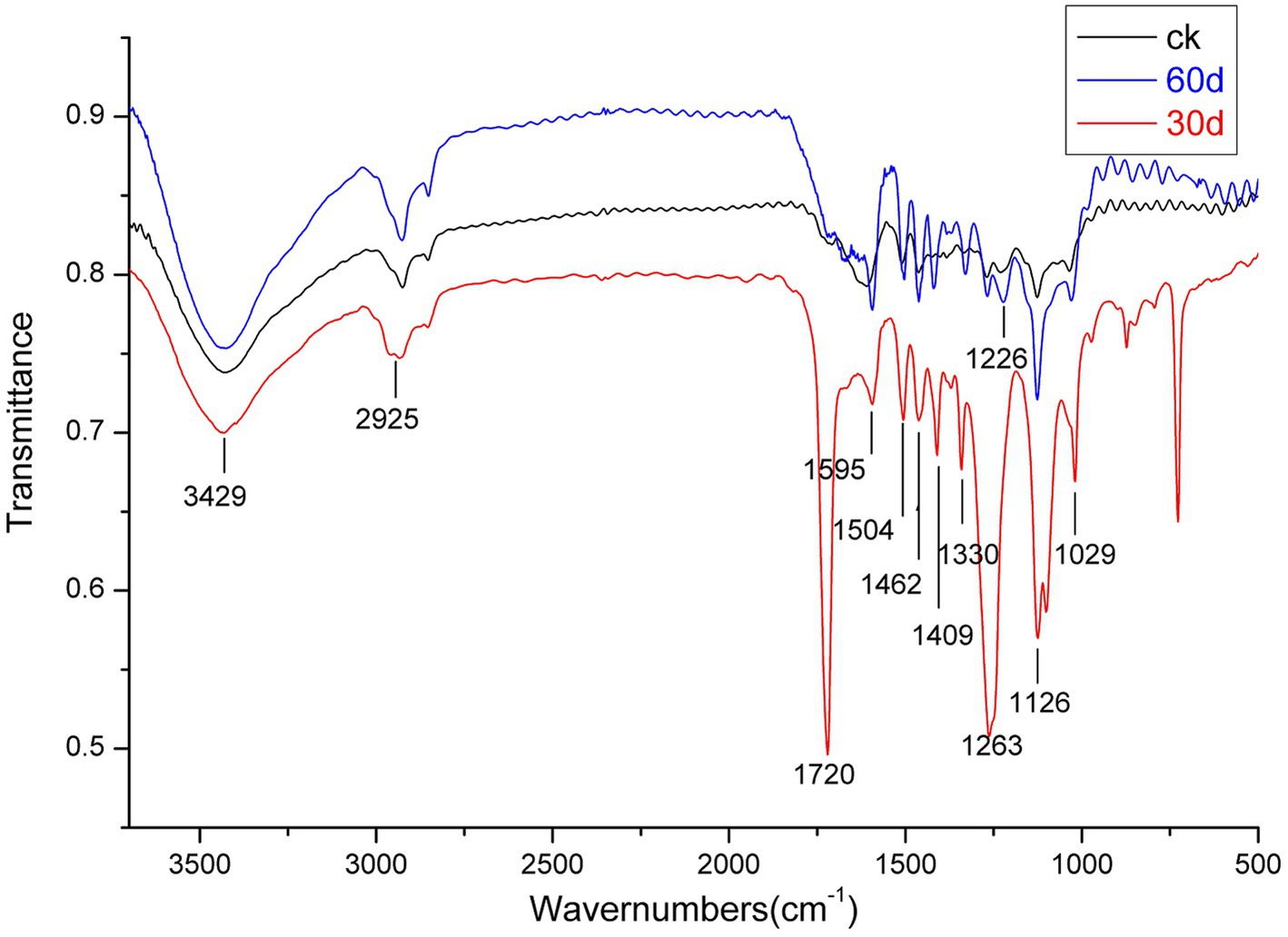
Figure 4. Infrared spectroscopy of milled wood lignin (MWL) from the cotton stalks pretreated by Suping 1 strain at various stages. ck stands for uninoculated cotton stalks medium; the red line represents 30 days pretreatment of cotton stalks medium; the blue line represents 60 days pretreatment of cotton stalks medium.
A broad and prominent peak was observed in the region between 3,425 and 3,431 cm−1, which is the stretching vibration peak of the hydrogen bond in-OH. Furthermore, a C-H stretching vibration peak was observed in the region between 2,925 and 2,933 cm−1 (Cai et al., 2010). The characteristic absorption peaks of the functional groups of lignin are primarily concentrated in the fingerprint region between 1800 and 800 cm−1. Table 2 details the representative characteristic peak in the region between 4,000 and 400 cm−1. The functional group change during lignin degradation was studied by comparing the intensities of MWL’s characteristic absorption peaks at various growth stages with those of the control. According to the method proposed by Jin et al. (2005), we calculated the intensities of the main infrared spectrum band of MWL at various growth stages, with 1,504 cm−1 as a reference (Table 2).
Within the infrared spectrum band, the absorption of methyl and methylene peaked at 2932 cm−1. This peak lowered on day 30 of the mycelium stage, compared with that of the control group, suggesting that methyl and methylene were broken during lignin degradation (Chen et al., 2015). The absorption of keto-carbonyl, acetic ester, or other esters peaked at 1720 cm−1. On day 30 following inoculation, the relative absorption intensity (A1725/A1504) of MWL was 2.03, whereas that of the control group was 0.93. These findings suggested that oxidation occurred during lignin degradation by using P. ostreatus, thus weakening the bond between lignin and hemicellulose (Dong et al., 2013).
The band near 1,263 cm−1 was the stretching vibration of the G unit and C=O stretching in lignin, which is the major structural unit of lignin, whereas the band near 1,226 cm−1 corresponds to the S unit and C-O stretching in lignin (Cai et al., 2010). The band near 1,126 cm−1 depicted the deformation vibration of the aromatic nucleus C-H of the G lignin unit on the plane. On day 30 following inoculation, the relative intensities of the absorption peaks (A1263/A1504, A1226 /A1504, and A1126/A1504) of MWL were 1.96, 1.21, and 1.62, respectively, which are higher than those in the control group (1.05, 1.04, and 1.13, respectively). During lignin degradation, the intensities of the absorption peaks at 1,263, 1,226, and 1,126 cm−1 increased, indicating that the connection bond between the lignin units broke under the action of enzymic oxidation and produced lignin monomer analogs.
According to Jin et al. (2013), the intensities of the absorption peaks at 1,263 and 1,226 cm−1 (A1263/A1226) can be used to express the relative amounts of G lignin units and S lignin units. On day 30 following inoculation, the value of A1263/A1226 was 1.62, apparently greater than that of the control group (1.00) (Table 2). Compared with the G lignin unit, the S lignin unit was preferentially degraded during lignin degradation.
1H-NMR analysis
The 1H-NMR spectrogram and results of the acetylated cotton stalk MWL at various periods are shown in Figure 5A and Table 3, respectively. Based on the 1H-NMR spectrogram for the acetylated lignin, the MWL range was distinguished, and MWL was integrated to calculate the proton ratio between functional groups and bond-type structures (Lu et al., 2008). In the 1H-NMR spectrogram for acetylated cotton stalk lignin at various degradation periods, signal peaks that appeared at the chemical shift point 7.3 × 10−6 – 6.8 × 10−6 represent the G lignin unit, whereas signal peaks that appeared at the chemical shift point 6.8 × 10−6 – 6.3 × 10−6 represent S lignin units.
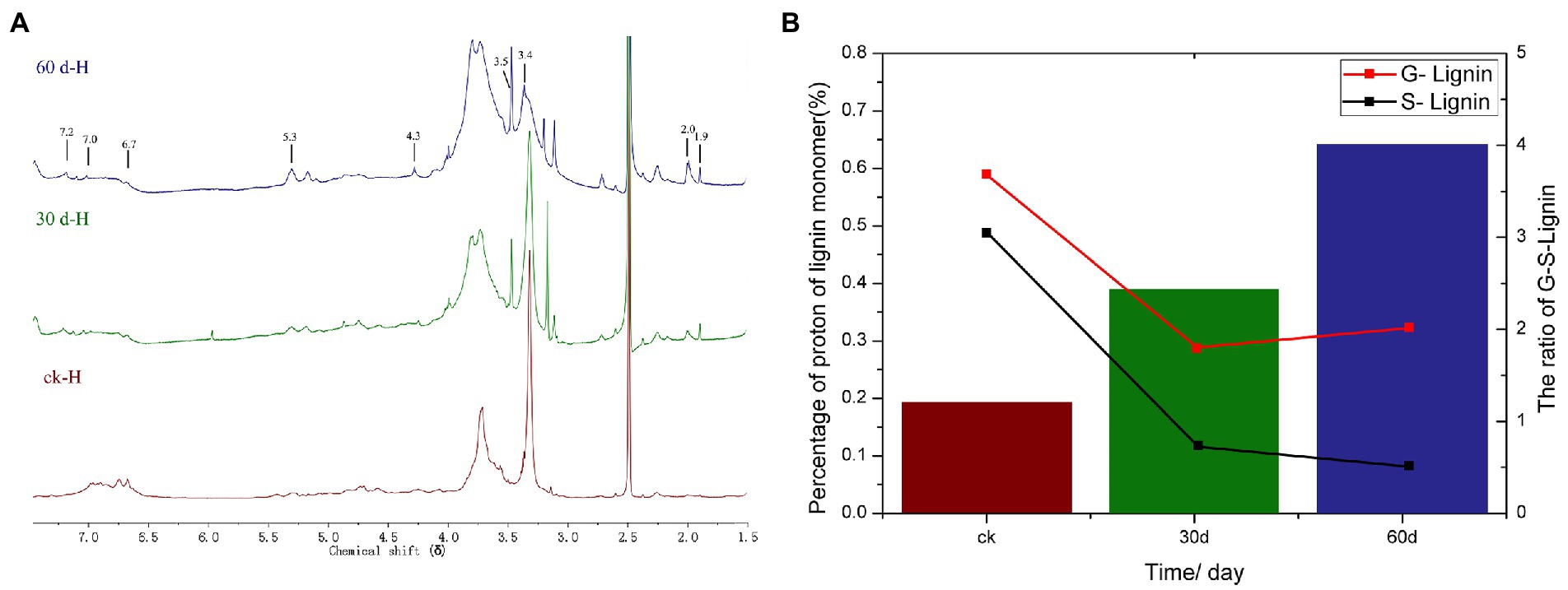
Figure 5. 1H NMR analysis of cotton stalks control and degraded by P. ostreatus for 30 and 60 days. (A) 1H-NMR spectra of acetylated MWL from the cotton stalks at various degradation stages. (B) Percentage and ratio of G lignin units and S lignin units in acetylated MWL from the cotton stalks at various degradation stages.
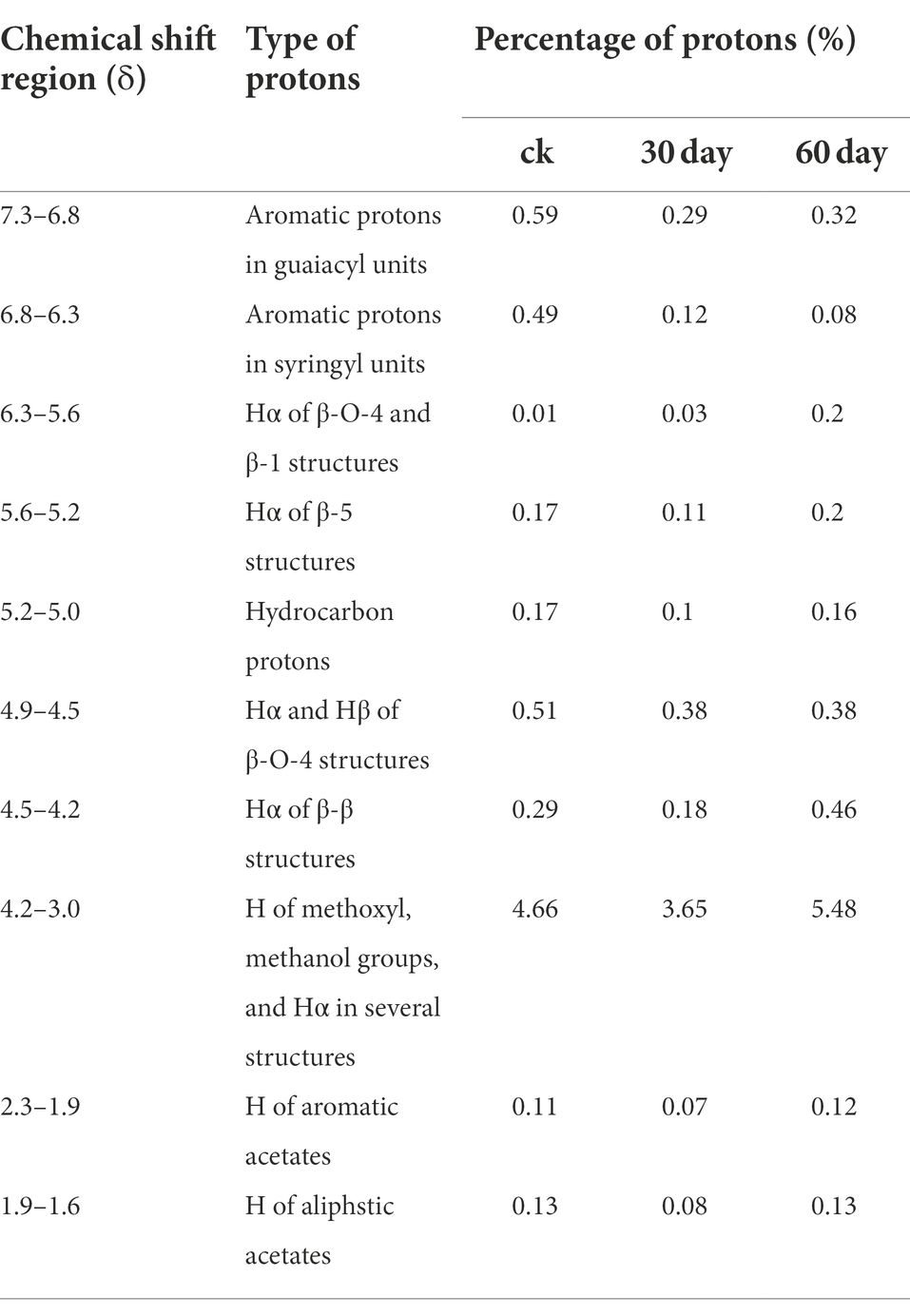
Table 3. 1H-NMR analysis of acetylated milled wood lignin from the cotton stalk at various degradation stages.
The amounts of G lignin units and S lignin units in the cotton stalk varied at various degradation periods (t), as did the G:S ratio (Table 3; Figure 5B). On day 30 of degradation, the amount of G lignin units and S lignin units significantly decreased compared with those in the control group. On day 60 of degradation, the amount of G lignin units increased slowly and the amount of S lignin units decreased more slowly than on day 30 (Figure 5B). These results suggested that P. ostreatus degraded cotton stalk lignin primarily when its mycelium grew, which is consistent with the analysis of the lignin content of cotton stalk during the degradation. On day 60 of degradation, the amount of G lignin units increased that than on day 30, suggesting that P. ostreatus converted a part of S lignin units to G lignin units (Figure 5B). Furthermore, the amount of S lignin units decreased more significantly than that of G lignin units on day 30 of degradation, and the content ratio between G lignin units and S lignin units increased (Figure 5B) during the 60 days of degradation, indicating that P. ostreatus preferentially degraded the S lignin units of the cotton stalk lignin. These results are in agreement with the infrared spectroscopy analysis.
GC–MS analysis
The low-molecular-weight compounds were identified from the supernatant of cotton stalks inoculated with P. ostreatus. Apart from the identical compounds identified from the control group, other low-molecular-weight compounds are shown in Table 4 and Figure 6. These degradation products were categorized based on their chemical structure as alcohols, organic acids, benzodiazepines, and alkanes. The compounds identified from sample supernatants at varied stages varied substantially. On day 3, 30, and 60 after the inoculation of P. ostreatus, 2 (organic acid and benzodiazepines), 14 (organic acid, benzodiazepines, and alcohol), and 4 (organic acid, benzodiazepines, and alkane) low-molecular-weight compounds were, respectively, identified from the extracts of cotton stalk samples (Figure 6).
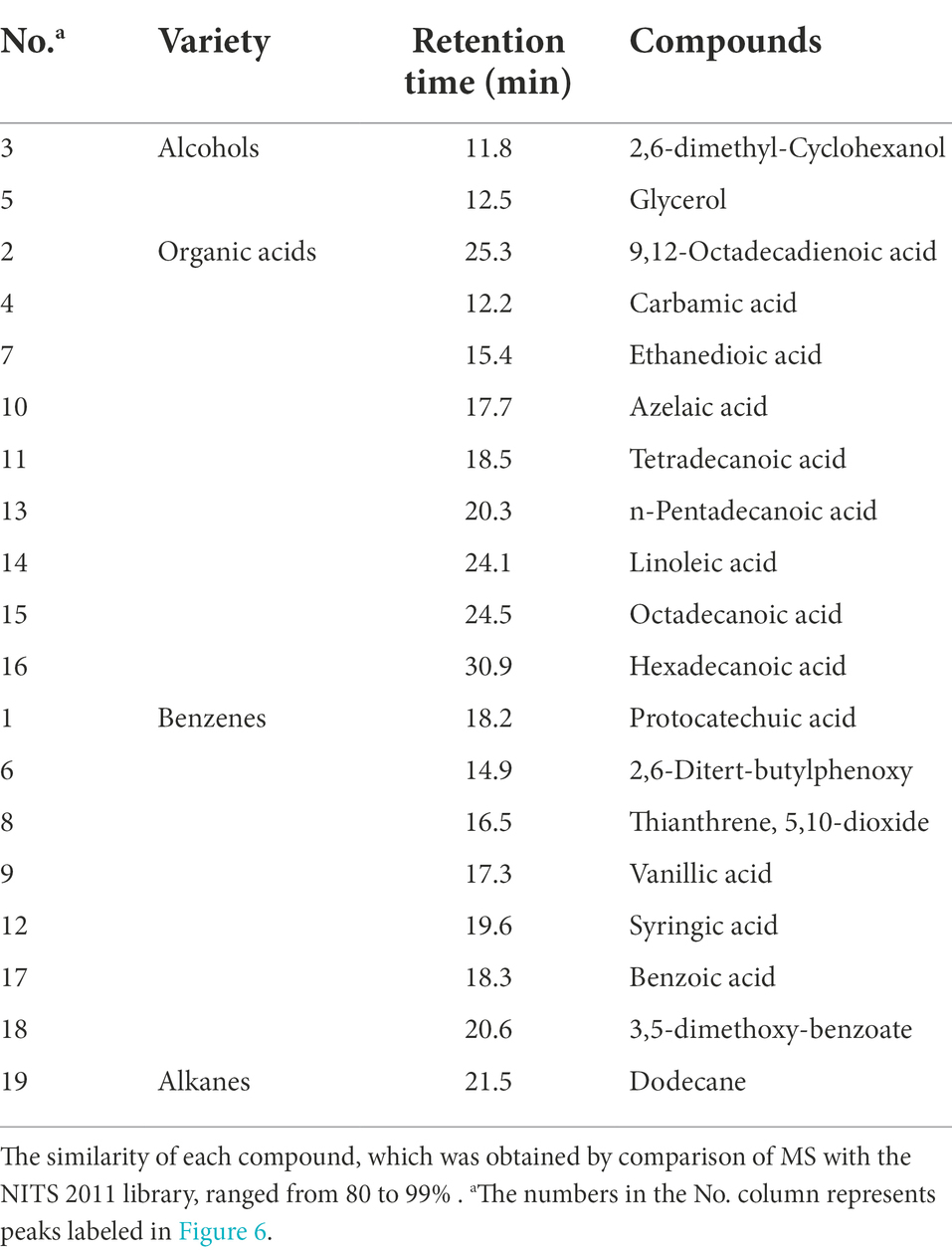
Table 4. Low-molecular-weight compounds identified as tetramethylsilane derivatives in chloroform extracts of P. ostreatus degraded cotton stalk samples at various growth stages.
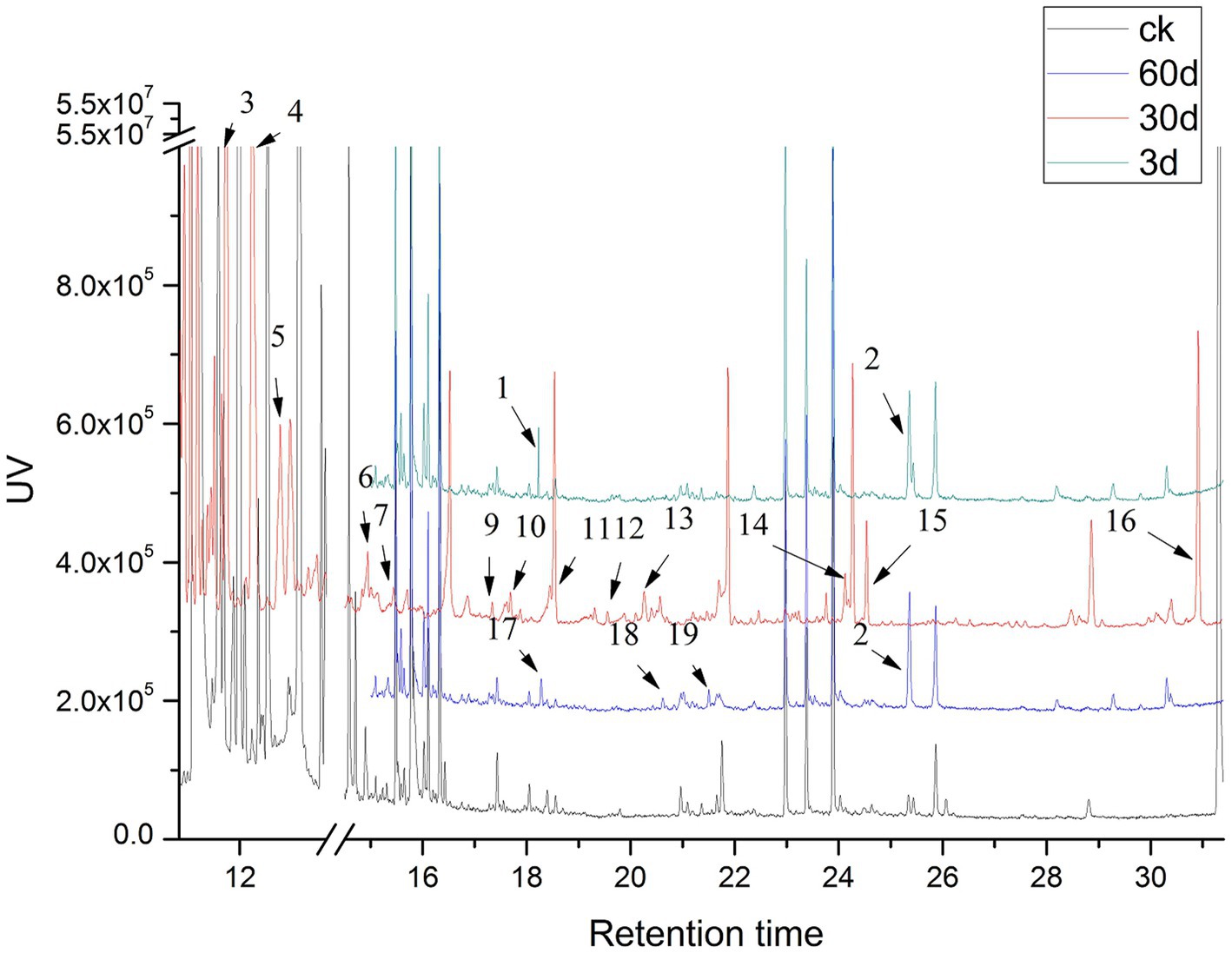
Figure 6. GC–MS analyses of lignin degradation compounds from samples collected 3, 30, and 60 days following inoculation.
In the preliminary experiment, we also detected the activity of the lignin-degrading enzyme in the cotton stalk samples on day 3 following inoculation. Therefore, we decided to collect a sample for GC–MS analysis on day 3 following inoculation. During the 3 days following inoculation, a key intermediate-protocatechuic acid-was detected in the extract of the degradation sample, but was not in the extract of the control group, suggesting that lignin degradation began on the third day following inoculation.
In addition to protocatechuic acid, vanillic acid and syringic acid were identified in the cotton stalk sample on day 30 following inoculation, and benzoic acid and 3,5-dimethoxybenzoic acid ester were identified on day 60 following inoculation. The production of vanillic acid and syringic acid was likely related to the oxidizing reaction between the two basic components of hard-wood lignin, sinapyl alcohol (G unit) and coniferyl alcohol (S unit) (Kumar et al., 2015). The general lignin-degrading mechanism is as follows (Chen et al., 2015): (1) The polyphenolic structure was formed through demethoxylation and hydroxylation followed by (2) the oxidation of the side chain of lignin monomer as well as the oxidation and falling off of the polyphenolic ring. During the cultivation of P. ostreatus on cotton stalks, aromatic compounds with benzene-ring structural units, such as vanillic acid, syringic acid, and benzoic acid, were produced. All of these substances had a structure similar to the lignin monomer, indicating that P. ostreatus was able to depolymerize lignin into a lignin monomer analog and further oxidize it to substances, such as organic acids and alkanes. That is, the production of these aromatic compounds, organic acids, and alkanes during biodegradation is consistent with the aforementioned degradation mechanism.
Discussion
The research on cotton straw delignification is intended for application in industrial production and is mainly related to physical and chemical methods (Karthyani et al., 2020; Cheng et al., 2022; Dong Z. et al., 2022). With the intensive research on white rot fungi, some strains with high production of lignin degrading enzymes were also applied for delignification production (Meehnian et al., 2016; Wittner et al., 2021; Sabogal-Otalora et al., 2022). For example, Meehnian et al. (2017) used the white rot fungi Daedalea flavida MTCC 145 and Phlebia radiata MTCC 2791 to degrade cotton straw lignin to release glucose. The aim of our study was to investigate more efficient utilization of cotton straw cellulose after efficient lignin delignification by the strains and thus to obtain a higher conversion rate. The present study revealed that all eight strains were capable of preferentially degrading lignin, albeit to varying degrees of degradation. Wanping 1, Heiping A, and Suping1 had higher selectivity factors (>2) and higher lignin-degrading rates (>13%) in 15 days. Therefore, these three strains have the potential to be grown on stalks and to recycle lignocellulose. For instance, they can be used to convert lignocellulose into ruminant feed and as a pretreatment for delignification in the biomass energy industry (Salame et al., 2014). Early studies have revealed a positive correlation between the lignin-degrading rate and the fruiting body yield (Li et al., 2014). Therefore, these three edible fungi strains can also be used to increase yield in stalk-based cultivation.
When cultured for 60 days on cotton stalk solid medium, P. ostreatus produced highly active LaC and MnP, but no LiP. The Daedalea flavida MTCC 145 strain with high LiP and Lac activity and no MnP activity had high lignin degradation ability, the Phlebia radiata MTCC 2791 strain with high MnP activity and low Lac and Lip activity had weaker lignin degradation ability than the D. flavida MTCC 145, and the Flavodon flavus MTCC168 strain with low Lac and MnP and no Lip activity had extremely weak lignin degradation ability (Meehnian et al., 2017), which was attributed to the fact that after the initial depolymerization of lignin by peroxidase (LiP and MnP), lignolytic enzymes including laccase could easily penetrate into the cotton stalk cell wall, so the biodegradation of lignin requires the cooperation of multiple enzymes (Arora et al., 2002). The complex lignin-degrading enzyme system of white-rot fungi consists of extracellular enzymes such as LaC, MnP, and LiP. However, a specific type of white-rot fungus typically produces one, two, or three types of extracellular enzymes (Torres-Farrada et al., 2017; Han et al., 2021). Phanerochaete chrysosporium produces MnP and LiP, but not LaC (Coconi-Linares et al., 2015). Pycnoporus cinnabarinus does not produce LiP and MnP in its lignin-degrading-oriented culture, but it does produce a type of LaC isozyme (Henske et al., 2018). This study also reveals that P. ostreatus can produce LaC and MnP, but not LiP, which is consistent with previous studies (Ferreira et al., 2015; Zolciak, 2019).
The degradation of cotton stalk lignin by a CDs/CuO synergistic emulsion system was investigated by Dong Z. et al., (2022), and the results showed that H-type monomers were the main products of lignin degradation in this system. However, during lignin degradation by using P. ostreatus, S-type monomers and G-type monomers were the main degradation products, and the S lignin unit was more easily degraded than the G lignin unit, and the G/S ratio exhibited an upward trend. Thus, biodegradation of cotton stalk lignin differs from chemical degradation and the type, composition, and content of lignin affect its degradation by white-rot fungi. In the study by van Kuijk et al. (2016) changes in lignin composition were a direct result of lignin degradation since it was mainly related to the mechanisms of fungal degradation and less to substrate properties. In this study, 21% of the cotton stalk lignin belongs to the dicotyledon lignin (G-S). According to infrared spectroscopy and 1H-NMR analysis, the S lignin units are more susceptible to degradation by P. ostreatus than the G lignin units. However, for the bagasse lignin (25%), a kind of monocotyledon lignin (G-S-H), its S lignin is also preferentially degraded by P. ostreatus (Dong et al., 2013), suggesting that the preferential degradation of the S lignin units by P. ostreatus is unaffected by the type, content, and composition of stalk lignin. However, other studies have suggested that the type, composition, and type of stalk lignin influence the rate of lignin degradation by white-rot fungi (Skyba et al., 2013). Hence, in the recycling of stalks, we can select an appropriate white-rot fungus according to the variation in stalk lignin to shorten the treatment cycle and improve efficiency.
In the process of the degradation of cotton stalk lignin by P. ostreatus, seven types of aromatic metabolites were produced, including protocatechuic acid, 2,6-ditertbutyl phenoxy derivative, vanillic acid, syringic acid, benzoic acid, and 3,5-dimethoxy-benzoate. The production of these aromatic metabolites is indicative of the degradation of the G units and S units of lignin. The catabolic pathway of many lignin components produces vanillin or oxidizes vanillin to produce vanillic acid, which is then demethylated and converted into protocatechuic acid. After the oxidation ring of the relevant dioxygenase (such as 3,4-dioxygenase of protocatechuic acid) of fungus breaks, protocatechuic acid is degraded. All of these dioxygenases are the key enzymes of the β-ketoadipate pathway (Bugg et al., 2011; Martins et al., 2015; Li et al., 2022). Previous studies have demonstrated that the P. ostreatus mycelium, when induced by a lignin monomer analog (such as p-hydroxybenzoic acid), produces corresponding dioxygenases (Dittmann et al., 2002; Mai et al., 2004; Xie et al., 2020). Therefore, it is speculated that P. ostreatus decomposes these aromatic intermediates (such as vanillic acid and protocatechuic acid) via the β-ketoadipate pathway.
In this study, P. ostreatus degraded lignin primarily during its mycelium growth with up to 54.04% lignin degradation. Its culture produces laccase and manganese dependent peroxidase, and no lignin peroxidase activity was detected. The results of nuclear magnetic resonance spectroscopy and Fourier transform infrared spectroscopy analyses of significant changes in lignin structure revealed that S lignin units were more degraded than G lignin units. The Gas Chromatography-Mass Spectrometer analysis of low-molecular-weight compounds revealed that the delignification resulted in the formation of alcohols, organic acids, benzodiazepines, and alkanes. These results demonstrate that S-type and G-type lignin monomers were the main products of lignin degradation by P. ostreatus, and that lignin with S-type monomers were degraded preferentially.
Data availability statement
The raw data supporting the conclusions of this article will be made available by the authors, without undue reservation.
Author contributions
GL conceptualized the idea for the study and performed most of experimental operations. YW and DY performed some of experimental operations and data analysis and led the writing of the manuscript. GL and HZ critically reviewed the data analysis and contributed substantially to the writing. PZ, GZ, and CL made key suggestions for improving the paper. All authors contributed to the article and approved the submitted version.
Funding
This work was supported by the Key Research and Development Projects of Anhui Province (201904F06020025) and the Doctoral Matching Funds Project for Agricultural Research (340000211264005000407).
Conflict of interest
The authors declare that the research was conducted in the absence of any commercial or financial relationships that could be construed as a potential conflict of interest.
Publisher’s note
All claims expressed in this article are solely those of the authors and do not necessarily represent those of their affiliated organizations, or those of the publisher, the editors and the reviewers. Any product that may be evaluated in this article, or claim that may be made by its manufacturer, is not guaranteed or endorsed by the publisher.
References
Ahmad, Z., Asgher, M., Hussain, F., and Randhawa, M. A. (2016). A novel approach to delignify lignocellulosic materials by using ligninolytic enzyme consortium. Bioresources 11, 10511–10527. doi: 10.15376/biores.11.4.10511-10527
Akpinar, O., Levent, O., Bostanci, S., Bakir, U., and Yilmaz, L. (2011). The optimization of dilute acid hydrolysis of cotton stalk in xylose production. Appl. Biochem. Biotechnol. 163, 313–325. doi: 10.1007/s12010-010-9040-y
Arora, D. S., Chander, M., and Gill, P. K. (2002). Involvement of lignin peroxidase, manganese peroxidase and laccase in degradation and selective ligninolysis of wheat straw. Int. Biodeterior. Biodegrad. 50, 115–120. doi: 10.1016/S0964-8305(02)00064-1
Asgher, M., Ijaz, A., and Bilal, M. (2016). Cost-effective lignocellulose-degrading enzyme production by Pleurotus sapidus WC 529 and their role in lignin degradation. Turkish J. Biochem. 41, 403–410. doi: 10.1515/tjb-2016-0005
Bugg, T. D. H., Ahmad, M., Hardiman, E. M., and Singh, R. (2011). The emerging role for bacteria in lignin degradation and bio-product formation. Curr. Opin. Biotechnol. 22, 394–400. doi: 10.1016/j.copbio.2010.10.009
Cai, Y., Li, G., Nie, J., Lin, Y., Nie, F., Zhang, J., et al. (2010). Study of the structure and biosynthetic pathway of lignin in stone cells of pear. Sci. Hortic. 125, 374–379. doi: 10.1016/j.scienta.2010.04.029
Chandrasekaran, G., Choi, S.-K., Lee, Y.-C., Kim, G.-J., and Shin, H.-J. (2014). Oxidative biodegradation of single-walled carbon nanotubes by partially purified lignin peroxidase from Sparassis latifolia mushroom. J. Ind. Eng. Chem. 20, 3367–3374. doi: 10.1016/j.jiec.2013.12.022
Chen, Y., Huang, J., Li, Y., Zeng, G., Zhang, J., Huang, A., et al. (2015). Study of the rice straw biodegradation in mixed culture of Trichoderma viride and Aspergillus niger by GC-MS and FTIR. Environ. Sci. Pollut. Res. 22, 9807–9815. doi: 10.1007/s11356-015-4149-8
Cheng, J., Hu, S.-C., Geng, Z.-C., and Zhu, M.-Q. (2022). Effect of structural changes of lignin during the microwave-assisted alkaline/ethanol pretreatment on cotton stalk for an effective enzymatic hydrolysis. Energy 254:124402. doi: 10.1016/j.energy.2022.124402
Coconi-Linares, N., Ortiz-Vazquez, E., Fernandez, F., Loske, A. M., and Gomez-Lim, M. A. (2015). Recombinant expression of four oxidoreductases in Phanerochaete chrysosporium improves degradation of phenolic and non-phenolic substrates. J. Biotechnol. 209, 76–84. doi: 10.1016/j.jbiotec.2015.06.401
Dittmann, J., Heyser, W., and Bucking, H. (2002). Biodegradation of aromatic compounds by white rot and ectomycorrhizal fungal species and the accumulation of chlorinated benzoic acid in ectomycorrhizal pine seedlings. Chemosphere 49, 297–306. doi: 10.1016/S0045-6535(02)00323-5
Dong, H., Ding, L., Wu, L., and Mamatjan, Y. (2022). Degradation of cotton stalk lignin by carbon dots loaded copper oxide synergistic emulsion system. Nanotechnology 33:485402. doi: 10.1088/1361-6528/ac7240
Dong, Z., Li, N., Chu, T., Ding, J., Zhang, J., and Dong, A. (2022). High-quality natural fibers from cotton stalk bark via limited alkali penetration and simultaneous accelerated temperature rise. Materials 15:422. doi: 10.3390/ma15020422
Dong, X. Q., Yang, J. S., Zhu, N., Wang, E. T., and Yuan, H. L. (2013). Sugarcane bagasse degradation and characterization of three white-rot fungi. Bioresour. Technol. 131, 443–451. doi: 10.1016/j.biortech.2012.12.182
Fernandez-Fueyo, E., Ruiz-Duenas, F. J., Lopez-Lucendo, M. F., Perez-Boada, M., Rencoret, J., Gutierrez, A., et al. (2016). A secretomic view of woody and nonwoody lignocellulose degradation by Pleurotus ostreatus. Biotechnol. Biofuels 9:49. doi: 10.1186/s13068-016-0462-9
Ferreira, P., Carro, J., Serrano, A., and Martinez, A. T. (2015). A survey of genes encoding H2O2-producing GMC oxidoreductases in 10 Polyporales genomes. Mycologia 107, 1105–1119. doi: 10.3852/15-027
Gill, M. K., Kocher, G. S., and Panesar, A. S. (2022). Evaluation of fungal consortium lignozyme for biodelignification of agricultural residues. Biofuels Bioprod. Biorefin 16:2424. doi: 10.1002/bbb.2424
Han, M.-L., Yang, J., Liu, Z.-Y., Wang, C.-R., Chen, S.-Y., Han, N., et al. (2021). Evaluation of laccase activities by three newly isolated fungal species in submerged fermentation with single or mixed lignocellulosic wastes. Front. Microbiol. 12:682679. doi: 10.3389/fmicb.2021.682679
Henske, J. K., Springer, S. D., O'malley, M. A., and Butler, A. (2018). Substrate-based differential expression analysis reveals control of biomass degrading enzymes in Pycnoporus cinnabarinus. Biochem. Eng. J. 130, 83–89. doi: 10.1016/j.bej.2017.11.015
Javad, M., Masoud, T., and Hadi, D. (2007). The effect of Pleurotus spp. fungi on chemical composition and in vitro digestibility of rice straw. Pak. J. Biol. Sci. 10, 2460–2464. doi: 10.3923/pjbs.2007.2460.2464
Jin, X.-J., Pu, J.-W., Xie, Y.-M., Takeshi, F., and Liu, X.-Y. (2005). Lignin characterization of triploid clones of Populus tomentosa Carr. J. Beijing For. Univ. 7, 52–56. doi: 10.1007/s11632-005-0022-z
Jin, Q., Yan, C., Qiu, J., Zhang, N., Lin, Y., and Cai, Y. (2013). Structural characterization and deposition of stone cell lignin in Dangshan Su pear. Sci. Hortic. 155, 123–130. doi: 10.1016/j.scienta.2013.03.020
Karthyani, S., Pandey, A., and Devendra, L. P. (2020). Delignification of cotton stalks using sodium cumene sulfonate for bioethanol production. Biofuels 11, 431–440. doi: 10.1080/17597269.2017.1370884
Keshav, P. K., Banoth, C., Kethavath, S. N., and Bhukya, B. (2021). Lignocellulosic ethanol production from cotton stalk: an overview on pretreatment, saccharification and fermentation methods for improved bioconversion process. Biomass Convers. Biorefin., 1–17. doi: 10.1007/s13399-021-01468-z
Knop, D., Ben-Ari, J., Salame, T. M., Levinson, D., Yarden, O., and Hadar, Y. (2014). Mn2+-deficiency reveals a key role for the Pleurotus ostreatus versatile peroxidase (VP4) in oxidation of aromatic compounds. Appl. Microbiol. Biotechnol. 98, 6795–6804. doi: 10.1007/s00253-014-5689-4
Kumar, M., Singh, J., Singh, M. K., Singhal, A., and Thakur, I. S. (2015). Investigating the degradation process of Kraft lignin by beta-proteobacterium, Pandoraea sp ISTKB. Environ. Sci. Pollut. Res. 22, 15690–15702. doi: 10.1007/s11356-015-4771-5
Li, T., Cui, L., Song, X., Cui, X., Wei, Y., Tang, L., et al. (2022). Wood decay fungi: an analysis of worldwide research. J. Soils Sediments 22, 1688–1702. doi: 10.1007/s11368-022-03225-9
Li, G. Q., Li, A. M., Wang, J. N., Cai, Y. P., Nie, F., and Chen, J. X. (2014). Study on the cultivation of Pleurotus ostreatus strains with cotton straw. Northern Hortic. 10, 138–142.
Li, Y., and Zhang, Q. (2014). Biodegradation of cotton stalk by three white rot fungi and the effects on composition and structure changes of cotton stalk. J. Sci Indus. Res. 73, 719–723.
Li, Y., and Zhang, Q. (2016). Effects of naturally and microbially decomposed cotton stalks on cotton seedling growth. Arch. Agron. Soil Sci. 62, 1264–1270. doi: 10.1080/03650340.2015.1135327
Li, F., Zhang, J., Ma, F., Chen, Q., Xiao, Q., Zhang, X., et al. (2021). Lytic polysaccharide monooxygenases promote oxidative cleavage of lignin and lignin-carbohydrate complexes during fungal degradation of lignocellulose. Environ. Microbiol. 23, 4547–4560. doi: 10.1111/1462-2920.15648
Lu, W., Yan, W. C., Chen, J. C., Feng, H., and Gao, P. J. (2008). Function of the iron-binding chelator produced by Coriolus versicolor in lignin biodegradation. Sci. China Ser. C 51, 214–221. doi: 10.1007/s11427-008-0033-9
Lueangjaroenkit, P., Teerapatsakul, C., Sakka, K., Sakka, M., Kimura, T., Kunitake, E., et al. (2019). Two manganese peroxidases and a laccase of Trametes polyzona KU-RNW027 with novel properties for dye and pharmaceutical product degradation in redox mediator-free system. Mycobiology 47, 217–229. doi: 10.1080/12298093.2019.1589900
Mai, C., Schormann, W., Majcherczyk, A., and Huttermann, A. (2004). Degradation of acrylic copolymers by white-rot fungi. Appl. Microbiol. Biotechnol. 65, 479–487. doi: 10.1007/s00253-004-1668-5
Martins, T. M., Hartmann, D. O., Planchon, S., Martins, I., Renaut, J., and Pereira, C. S. (2015). The old 3-oxoadipate pathway revisited: new insights in the catabolism of aromatics in the saprophytic fungus Aspergillus nidulans. Fungal Genet. Biol. 74, 32–44. doi: 10.1016/j.fgb.2014.11.002
Meehnian, H., Jana, A. K., and Jana, M. M. (2016). Effect of particle size, moisture content, and supplements on selective pretreatment of cotton stalks by Daedalea flavida and enzymatic saccharification. 3 Biotech 6:235. doi: 10.1007/s13205-016-0548-x
Meehnian, H., Jana, A. K., and Jana, M. M. (2017). Pretreatment of cotton stalks by synergistic interaction of Daedalea flavida and Phlebia radiata in co-culture for improvement in delignification and saccharification. Int. Biodeterior. Biodegradation 117, 68–77. doi: 10.1016/j.ibiod.2016.11.022
Rouches, E., Herpoel-Gimbert, I., Steyer, J. P., and Carrere, H. (2016). Improvement of anaerobic degradation by white-rot fungi pretreatment of lignocellulosic biomass: a review. Renew. Sustain. Energy Rev. 59, 179–198. doi: 10.1016/j.rser.2015.12.317
Sabogal-Otalora, A. M., Palomo-Hernandez, L. F., and Pineros-Castro, Y. (2022). Sugar production from husk coffee using combined pretreatments. Chem. Eng. Proc. 176:108966. doi: 10.1016/j.cep.2022.108966
Salame, T. M., Knop, D., Levinson, D., Mabjeesh, S. J., Yarden, O., and Hadar, Y. (2014). Inactivation of a Pleurotus ostreatus versatile peroxidase-encoding gene (mnp2) results in reduced lignin degradation. Environ. Microbiol. 16, 265–277. doi: 10.1111/1462-2920.12279
Skyba, O., Douglas, C. J., and Mansfield, S. D. (2013). Syringyl-rich lignin renders poplars more resistant to degradation by wood decay fungi. Appl. Environ. Microbiol. 79, 2560–2571. doi: 10.1128/AEM.03182-12
Suryadi, H., Judono, J. J., Putri, M. R., Eclessia, A. D., Ulhaq, J. M., Agustina, D. N., et al. (2022). Biodelignification of lignocellulose using ligninolytic enzymes from white-rot fungi. Heliyon 8:e08865. doi: 10.1016/j.heliyon.2022.e08865
Torres-Farrada, G., Manzano Leon, A. M., Rineau, F., Ledo Alonso, L. L., Sanchez-Lopez, M. I., Thijs, S., et al. (2017). Diversity of ligninolytic enzymes and their genes in strains of the genus Ganoderma: applicable for biodegradation of xenobiotic compounds? Front. Microbiol. 8:898. doi: 10.3389/fmicb.2017.00898
Van Kuijk, S. J. A., Del Rio, J. C., Rencoret, J., Gutierrez, A., Sonnenberg, A. S. M., Baars, J. J. P., et al. (2016). Selective ligninolysis of wheat straw and wood chips by the white-rot fungus Lentinula edodes and its influence on in vitro rumen degradability. J. Anim. Sci. Biotechnol. 7:55. doi: 10.1186/s40104-016-0110-z
Wang, Y., Li, G., Jiao, X., Cheng, X., Abdullah, M., Li, D., et al. (2019b). Molecular characterization and overexpression of mnp6 and vp3 from Pleurotus ostreatus revealed their involvement in biodegradation of cotton stalk lignin. Biol. Open 8:bio036483. doi: 10.1242/bio.036483
Wang, Q., and Tuohedi, N. (2020). Polyurethane foams and bio-polyols from liquefied cotton stalk agricultural waste. Sustainability 12:4214. doi: 10.3390/su12104214
Wang, F., Xu, L., Zhao, L., Ding, Z., Ma, H., and Terry, N. (2019a). Fungal laccase production from lignocellulosic agricultural wastes by solid-state fermentation: a review. Microorganisms 7:665. doi: 10.3390/microorganisms7120665
Wittner, N., Broos, W., Bauwelinck, J., Slezsak, J., Vlaeminck, S. E., and Cornet, I. (2021). Enhanced fungal delignification and enzymatic digestibility of poplar wood by combined CuSO4 and MnSO4 supplementation. Proc. Biochem. 108, 129–137. doi: 10.1016/j.procbio.2021.06.002
Xiao, Q., Ma, F., Li, Y., Yu, H., Li, C., and Zhang, X. (2017). Differential proteomic profiles of Pleurotus ostreaus in response to lignocellulosic components provide insights into divergent adaptive mechanisms. Front. Microbiol. 8:480. doi: 10.3389/fmicb.2017.00480
Xie, P., Fan, L., Huang, L., and Zhang, C. (2020). An innovative co-fungal treatment to poplar bark sawdust for delignification and polyphenol enrichment. Ind. Crop. Prod. 157:112896. doi: 10.1016/j.indcrop.2020.112896
Xing, W., Xu, G., Dong, J., Han, R., and Ni, Y. (2018). Novel dihydrogen-bonding deep eutectic solvents: pretreatment of rice straw for butanol fermentation featuring enzyme recycling and high solvent yield. Chem. Eng. J. 333, 712–720. doi: 10.1016/j.cej.2017.09.176
Ya, Y., Sakamoto, T., Honda, Y., Kagotani, Y., Izumitsu, K., Suzuki, K., et al. (2013). The white-rot fungus Pleurotus ostreatus transformant overproduced intracellular cAMP and laccase. Biosci. Biotech. Bioch. 77, 2309–2311. doi: 10.1271/bbb.130470
Keywords: Pleurotus ostreatus, cotton stalk, lignin, 1H-NMR, FTIR, GC–MS
Citation: Li G, Wang Y, Yu D, Zhu P, Zhao G, Liu C and Zhao H (2022) Ligninolytic characteristics of Pleurotus ostreatus cultivated in cotton stalk media. Front. Microbiol. 13:1035040. doi: 10.3389/fmicb.2022.1035040
Edited by:
Pankaj Chowdhary, Indian Institute of Toxicology Research (CSIR), IndiaCopyright © 2022 Li, Wang, Yu, Zhu, Zhao, Liu and Zhao. This is an open-access article distributed under the terms of the Creative Commons Attribution License (CC BY). The use, distribution or reproduction in other forums is permitted, provided the original author(s) and the copyright owner(s) are credited and that the original publication in this journal is cited, in accordance with accepted academic practice. No use, distribution or reproduction is permitted which does not comply with these terms.
*Correspondence: Hongyuan Zhao, bmt5emh5QDE2My5jb20=