- 1Department of Education Management, Our Lady of Fatima University, Valenzuela, Philippines
- 2School of Physical Education and Sports Science, South China Normal University, Guangzhou, China
- 3School of Physical Education, Yan’an University, Yan’an, China
- 4The First Middle School of Tongliao City, Tongliao, China
- 5Collaborative Innovation Center for Child Nutrition and Health Development, Chongqing Engineering Research Center of Functional Food, Chongqing Engineering Laboratory for Research and Development of Functional Food Chongqing University of Education, Chongqing, China
Objectives: Aging is a process that involves comprehensive physiological changes throughout the body, and improvements in the exercise capacity of individuals may delay aging and relieve fatigue. Probiotics are subject to ongoing research to investigate their antioxidant properties. The purpose of this study was to investigate the effect of the probiotic Lactobacillus plantarum KSFY01 (L. plantarum KSFY01) on exercise tolerance in mice induced into a state of accelerated physiological aging by oxidative stress.
Methods: A mouse model of accelerated aging was established using D-galactose to induce oxidative stress. The bacteria L. plantarum KSFY01 was isolated from fermented yak yogurt. The effect of L. plantarum KSFY01 on the improvement of exercise capacity in aging-accelerated mice was evaluated by measuring their running time until exhaustion, histopathological sections, related biochemical indicators, and underlying gene expression.
Results: The oral administration of L. plantarum KSFY01 prolonged the running time of mice and reduced their creatine kinase (CK), alanine aminotransferase (ALT), and aspartate aminotransferasem (AST) levels. From this study, we observed that L. plantarum KSFY01 significantly improved the exercise capacity of mice and alleviated liver damage. Treatment with L. plantarum KSFY01 reduced the blood urea nitrogen (BUN), lactic acid (LD) accumulation, and lactate dehydrogenase (LDH) elevations produced by the accelerated aging state, and also reversed the changes in muscle glycogen (MG). Overall, L. plantarum KSFY01 could effectively improve metabolite accumulation, thereby relieving fatigue in exercised mice. The results of the antioxidant indices in vivo showed that L. plantarum KSFY01 intervention increased the activity of antioxidant enzymes, decreased the level of malondialdehyde (MDA), and restored the balance between the oxidative and antioxidant systems in fatigued mice. By investigating the underlying molecular mechanism, our results showed that L. plantarum KSFY01 intervention significantly reversed the decline in the expression levels of nuclear factor-erythroid 2 related factor 2 (Nrf2) signaling pathway-related factors and improved the body’s antioxidant capacity. We determined that the underlying molecular mechanism responsible for the antioxidant effect of L. plantarum KSFY01 mainly involves the activation of the Nrf2 pathway. The effect of L. plantarum KSFY01 was dose-dependent, and the expression level of Nrf2 increased with increasing dosage of the probiotic.
Conclusion: This study demonstrated that the probiotic L. plantarum KSFY01 exerts antioxidant effects and improved the athletic ability of mice. These findings are of significance to the development and utilization of probiotic resources.
1. Introduction
Aging is a process that involves comprehensive physiological changes throughout the body, and is one that all biological individuals must experience (Wang W. et al., 2022; Wang L. et al., 2022). At present, the aging population is expanding, and it is estimated that the global population over the age of 60 will reach 2.1 billion by 2050 (Leeson, 2018). Aging reduces the metabolic rate, imbalances the antioxidant system, and results in an excess of free radicals due to their reduced clearance, thereby resulting in their accumulation in the body (Adetuyi et al., 2022). Simultaneously, the content of lipid peroxide increases in the plasma and tissues, and the anti-fatigue ability of the body also gradually decreases (Zheng et al., 2017). Studies have found that telomere length is preserved in healthy elderly people who practise vigorous aerobic exercise, being positively correlated with the maximum aerobic exercise capacity, and thereby indicating that a high capacity for exercise may contribute to delaying the process of aging (LaRocca et al., 2010). People who regularly exercise have slower heart rates, lower blood pressure, and lower cholesterol levels (Myers et al., 2007). These indicators directly reflect the association between exercise capacity and aging. The decline in organ and muscular function as well as the increase in fatigue caused by aging all contribute to the decline of exercise capacity. To counter this, maintaining an exercise regime can help improve bodily functions, alleviate fatigue, and maintain the overall vitality of the body. The process of aging interacts with the exercise capacity of an individual, whereby bodily health is not only the embodiment of a high capacity for exercise, but can also promote continuous exercise in a positive feedback loop, so as to further delay aging (Padilha et al., 2021).
Under normal physiological conditions, the aerobic metabolism of organisms continuously produces oxygen free radicals (ROS) which cause damage to cells. When the burden of damage caused by these ROS is greater than the repair capacity of the body, bodily aging results (Liu et al., 2022). During the aging process, the activities of antioxidant enzymes are significantly reduced. This causes the continuous accumulation of free radicals in the body, which consequently affects normal metabolism, increases bodily fatigue, and reduces the capacity for exercise, thereby ultimately resulting in the decline of the quality of life for the elderly (Tan et al., 2000). This trajectory illustrates a close relationship between oxidative stress, aging, and exercise capacity. Enhancing exercise function by bolstering the antioxidant processes within the body represents an effective way to delay aging. Slowing the process of aging, in turn, strengthens the vitality of the organs and muscles, thus promoting athletic function. This strategy comprises one effective method which can be used to delay physical fatigue; namely, by supplementing exogenous antioxidants to the body, thereby preventing the oxidation of easily-oxidized substrates in cells, inhibiting lipid peroxidation, and directly eliminating oxygen free radicals to reduce oxidative stress in the body (Kerksick and Willoughby, 2005; McLeay et al., 2017).
The development and utilization of probiotic lactic acid bacteria (LAB) comprises an active area of research in the field of food bioengineering. Numerous studies have been conducted on the prebiotic function of LAB to show that many employ antioxidant functions, though there exist differences in these functions between different strains. Zhang et al. (2021) Found that Lactobacillus fermentum HFY03 isolated from yak yogurt could significantly improve the running duration of mice, reduce their contents of urea nitrogen and lactic acid, and increase their levels of catalase and superoxide dismutase in the liver tissue, thereby indicating that L. fermentum HFY03 exerts an antifatigue effect through improving the antioxidant capacity of mice that run until exhaustion. Some studies have also found that the use of LAB that employ antioxidant functions could improve the antioxidant properties of fermentation-based products. Zhou et al. (2021) used Lactobacillus plantarum HFY09 to ferment soymilk and found that the resulting fermented soymilk could effectively alleviate the accelerated aging of mice induced by D-galactose (Zhou et al., 2021). There are many studies existing on probiotics, but at present, there are few reports existing on their impacts on exercise capacity. In this study, a new lactic acid bacterium, Lactobacillus plantarum KSFY01 (L. plantarum KSFY01), was isolated from traditional fermented yak yogurt. It possessed high resistance to the conditions of pH 3.0, artificial gastric juice, and 0.3% bile salt. The results showed that L. plantarum KSFY01 was mostly unaffected by artificial gastric juice, and its survival rate was 86.26%. The growth rate in 0.3% bile salt was 54.24%. This study then constructed a mouse model of accelerated aging by the injection of D-galactose injection to investigate the effect of L. plantarum KSFY01 on the antioxidant levels and anti-fatigue response of mice. The results are intended to provide a reference for the application of L. plantarum in functional foods and for the development of health products.
2. Materials and methods
2.1. Experimental microorganism strain
This study isolated a L. plantarum strain from yak yogurt obtained from Kashgar, Xinjiang, and named it L. plantarum KSFY01. The bacteria were stored frozen at-80°C and subsequently inoculated into sterilized MRS medium for resuscitation. The resuscitation conditions involved 37°C, culturing for 18–24 h, and the bacterial activation spanning two generations. The second generation of bacterial suspension was then taken and centrifuged at 5000 rpm/min for 10 min. The upper culture medium was discarded, while that which remained was added with the same volume of 0.9% physiological saline to make up the bacterial suspension. Using the gradient dilution method, the L. plantarum KSFY01 bacterial solution was diluted to 1.0 × 1010 and 1.0 × 109 colony-forming units (CFU).
2.2. Animal experiment
Fifty 6-week-old male Kunming mice were purchased from the animal experiment center of Chongqing Medical University. Before the formal experiment commenced, the mice were placed under a 12-h light/dark cycle for 1 week of adaptive feeding with free access to food and water. At the end of the one-week adaptation period, the mice were equally divided into 5 groups (with 10 mice each): the normal group, model group, vitamin C group (Vit C), KSFY01 high-dose group (KSFY01-H), and KSFY01 low-dose group (KSFY01-L). The duration of the experimental period was 10 weeks (Figure 1). After the start of the experiment, the mice in all groups except the normal group were injected with D-galactose solution (120 mg/kg) intraperitoneally every day for 6 weeks, while the mice in the normal group were intraperitoneally injected with the same amount of saline. From the 7th week onwards, the mice in the normal group and the model group were gavaged with distilled water (0.02 ml/kg) every day; the mice in the Vit C group were gavaged with 200 mg/kg of vitamin C every day; the mice in the KSFY01-H group were gavaged every day with L. plantarum KSFY01 bacterial suspension at a dose of 1.0 × 1010 CFU/kg; mice in the KSFY01-L group were administered the L. plantarum KSFY01 bacterial suspension at a dose of 1.0 × 109 CFU/kg for 4 weeks. After 10 weeks, the mice were subjected to the running exhaustion test, whereby the time to exhaustion of each group of mice was recorded. Then, the mice were immediately sacrificed, had their blood was sampled from the eyeballs, and their liver and kidney tissues were promptly collected for subsequent experiments.
2.3. Running test
After 10 weeks, the mice were subjected to the running exhaustion test. The running wheel was set to 20 rpm/min, and the mice were forced to run on the wheel (YH-CS, Wuhan Yihong Technology Co., Ltd., Wuhan, Hubei, China). When the mice stopped running, electric shocks were performed 5 consecutive times until the mice did not run, thereby indicating exhaustion, and the running time was recorded.
2.4. Biochemical and energy metabolism indices
Mice eyeball blood was collected into 1.5 ml centrifuge tubes, placed in a 4°C refrigerator for 30 min, and then centrifuged (3,000 rpm at 4°C for 10 min). An appropriate amount of serum was then taken and analyzed with biochemical kits (Nanjing Jiancheng Bioengineering Institute, Nanjing, China) to determine the contents of blood urea nitrogen (BUN), serum free fatty acid (NEFA), lactic acid (LA), lactate dehydrogenase (LDH), catalase (CAT), glutathione (GSH), malondialdehyde (MDA) levels, and muscle glycogen (MG). The enzyme-linked immunosorbent assay (ELISA; Shanghai Enzyme Link Biotechnology Co., Ltd., Shanghai, China) was used to determine the activities of aspartate aminotransferase (AST), alanine aminotransferase (ALT), and creatine kinase (CK) in the mouse serum.
2.5. Histopathological analysis
Immediately after the mice were dissected, portions of the mouse liver and kidney tissues were taken and placed in a 10% neutral formalin solution for fixation. The tissue samples were embedded, cut into 5–10 μm slices, and then stained with hematoxylin–eosin (H&E) dye (Li et al., 2021a). The pathological changes in the tissue were then observed under a light microscope.
2.6. Real-time quantitative PCR detection
The mRNA expression of SOD1, SOD2, CAT, interleukin-1β (IL-1β), tumor necrosis factor α (TNF-α), interleukin-10 (IL-10), heme oxygenase-1 (HO-1), nuclear factor-erythroid 2 related factor 2 (Nrf2), γ-glutamylcysteine synthetase (γ-GCS), and NAD(P)H dehydrogenase [quinone] 1 (NQO-1) were measured using real-time quantitative PCR (RT-qPCR; Wang et al., 2021). The extraction of mouse RNA from the liver and muscle tissue was conducted as follows. Mouse liver tissue and muscle tissue were removed from storage at −80°C, and 100 mg of each was then weighed into a homogenization tube, added with 1 ml of TRIzol reagent, and homogenized using beads for extraction. A nucleic acid analyzer was then used to measure the concentration of the extracted RNA to ensure that the purity value was between 1.8 and 2.0. The RNA was then reverse-transcribed into cDNA using a kit (Thermo Fisher Scientific, Inc., Waltham, MA, United States) according to the manufacturer’s instructions, after which the resulting cDNA was aliquotted and stored at −80°C for later use. The reverse-transcribed cDNA was then used as a template whereby it was added with fluorescent dye and placed into a fluorescence quantitative PCR instrument to carry out the reaction. The volume used for the RT-PCR reaction system was 20 μl, and the reaction conditions were as follows: preheating at 95°C for 60 s, 40 cycles of 95°C for 15 s, 55°C for 30 s, 72°C for 35 s, and then ultimately tested at 95°C for 30 s and 55°C for 35 s. Each reaction was performed four times (Long et al., 2020). In the experiment, the β-actin gene was used as the internal reference gene, and the relative expression level of the target mRNA was determined by the 2−ΔΔCt relative quantitative method (Li et al., 2021b). The respective primer sequences of the internal reference gene and target gene used in this study are shown in Table 1.
2.7. Statistical analysis
All data are expressed as the mean ± standard deviation and plotted using GraphPad Prism (version 7.00) software. SPSS software (SPSS 22.0, SPSS Inc.) was used for statistical analysis of the data, and ANOVA and Duncan’s test were used to evaluate the significance of the differences for each parameter of the samples (p < 0.05).
3. Results
3.1. Effects of Lactobacillus plantarum KSFY01 on exercise endurance In accelerated-aging mice
It can be seen from Figure 2 that the running time of the mice in the normal group was the greatest among all the groups, while the running time in the aging model group was the shortest. Compared with the aging model group, both L. plantarum KSFY01 and vitamin C could significantly (p < 0.05) prolong the running time of aging mice, and the L. plantarum KSFY01 high-dose group had the greatest prolongation effect, which was significantly better than that of either the low-dose L. plantarum KSFY01 group or the Vit C group. These experimental results show that L. plantarum KSFY01 can improve the exercise endurance of mice, prolong the exercise time of mice, and relieve their exercise-induced fatigue.
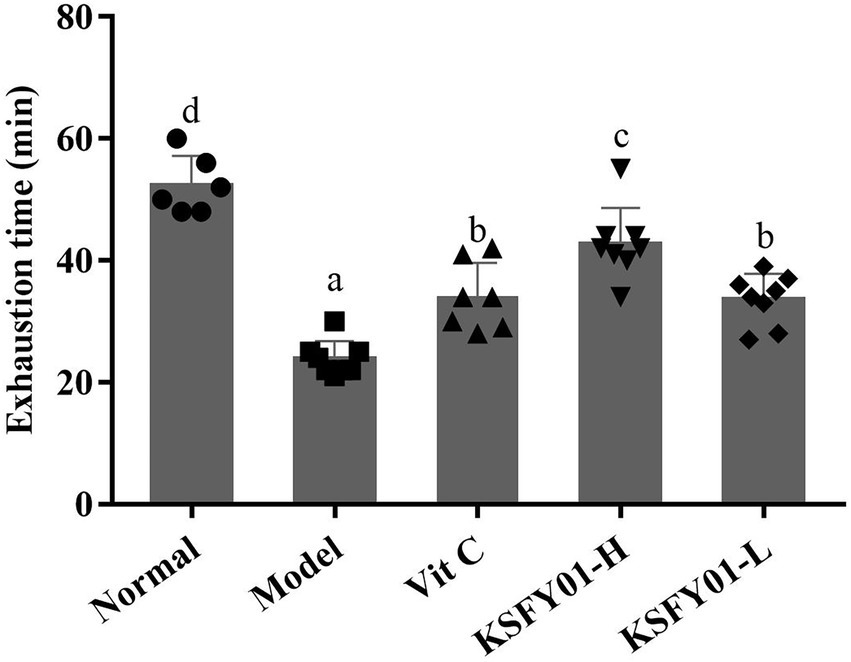
Figure 2. Running exhaustion time of mouse in each group. a–dValues in the same column with different letter superscripts indicate significant difference (p < 0.05). Vit C, mice treated with vitamin C (200 mg/kg); KSFY01-H, mice treated with L. plantarum KSFY01 (1 × 1010 CFU/kg); KSFY01-L, mice treated with L. plantarum KSFY01 (1 × 109 CFU/kg).
3.2. Energy metabolism in mice
As shown in Table 2, the serum levels of BUN, NEFA, and LD in the normal group were significantly (p < 0.05) lower than those in other groups, while the LDH and MG levels were significantly (p < 0.05) higher than those in other groups. Conversely, the above indicators in the serum of mice in the aging model group showed the opposite trend to that of the normal group. Compared with the aging model group, L. plantarum KSFY01 and vitamin C each reduced the levels of BUN, NEFA, and LD in the serum of aging mice, while having increased the levels of LDH and MG. These experimental results show that L. plantarum KSFY01 can reduce the BUN produced by mice following exercise, increase the activity of LDH, reduce the content of LD, and relieve the exercise-induced fatigue of mice.
3.3. Sports injuries in mice
It can be seen from Table 3 that the ALT, AST, and CK contents of the vitamin C group, L. plantarum KSFY01 high dose group, and L. plantarum KSFY01 low dose groups lay between the results of the model group and the normal group. Both the L. plantarum KSFY01 bacterial suspension and vitamin C had good alleviating effects by decreasing the high ALT, AST, and CK contents induced by D-galactose. The effects of the high-dose L. plantarum KSFY01 group and the vitamin C group were better than that of the low-dose L. plantarum KSFY01 group.
3.4. Serum oxidation level of mice
The serum levels of CAT, GSH, and MDA were compared between groups, as shown in Table 4. Compared with the normal group, CAT enzyme activity in the model group was significantly decreased (p < 0.05), and the GSH content was also significantly decreased. After either vitamin C or L. plantarum KSFY01 intervention, the CAT enzyme activity and GSH level were both increased; among these, the high dose of L. plantarum KSFY01 was more effective than either vitamin C or the low dose of L. plantarum KSFY01. Compared with the model group, vitamin C and L. plantarum KSFY01 significantly reduced the MDA content (p < 0.05); among these, high-dose L. plantarum KSFY01 had the greatest effect.
3.5. Pathological evaluation
The morphology of liver tissue under the microscope in mice is shown in Figure 3. The liver lobules of the mice in the normal group exhibited a clear structure and no obvious changes in the intact liver cells, and there were no phenomena such as granular degeneration, vacuolar degeneration, hepatocyte enlargement, or necrosis. In the D-galactose-induced aging model group, the structure of the hepatic lobules of the mice was destroyed, the arrangement of hepatocytes was disordered, many vacuoles of different sizes appeared in the encapsulation, and there was inflammatory infiltration accompanied by cell necrosis. Both vitamin C and L. plantarum KSFY01 could reduce hepatocyte damage in aging mice. After the action of KSFY01-H, the structure of hepatic lobules was mainly intact, and the degeneration of hepatocytes was significantly improved. There were still vacuoles of different sizes in the liver tissue of mice observed in both the Vit C group and KSFY01-L group.
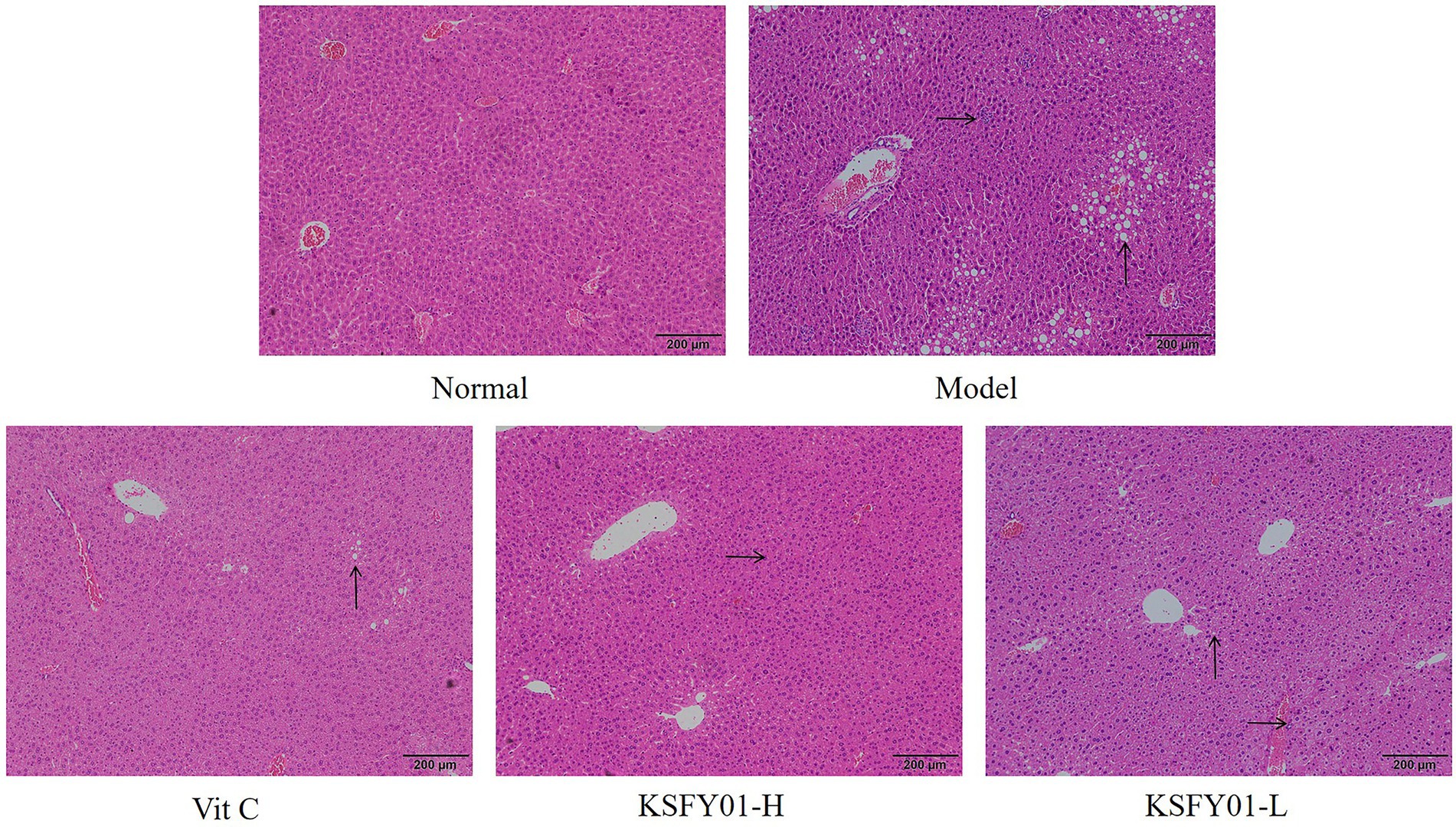
Figure 3. H&E pathological observation of liver tissue in aging mouse (magnification, ×100). Vit C, mice treated with vitamin C (200 mg/kg); KSFY01-H, mice treated with L. plantarum KSFY01 (1 × 1010 CFU/kg); KSFY01-L, mice treated with L. plantarum KSFY01 (1 × 109 CFU/kg). → indicate adipocyte; ↑ indicate inflammatory cell in liver.
The morphology of kidney tissue under the microscope of mice is shown in Figure 4, and the glomerular vascular loops in the normal group were thin and clear. The number of endothelial and mesangial cells was normal. The surrounding renal tubules were also normal. The glomeruli in the kidney tissue of the mice in the aging model group were irregular in shape, some were ruptured or swollen, and there was inflammatory cell infiltration observed between the tissues. Both vitamin C and L. plantarum KSFY01 could reduce kidney tissue damage caused by aging, and the infiltration of inflammatory cells decreased to varying degrees.
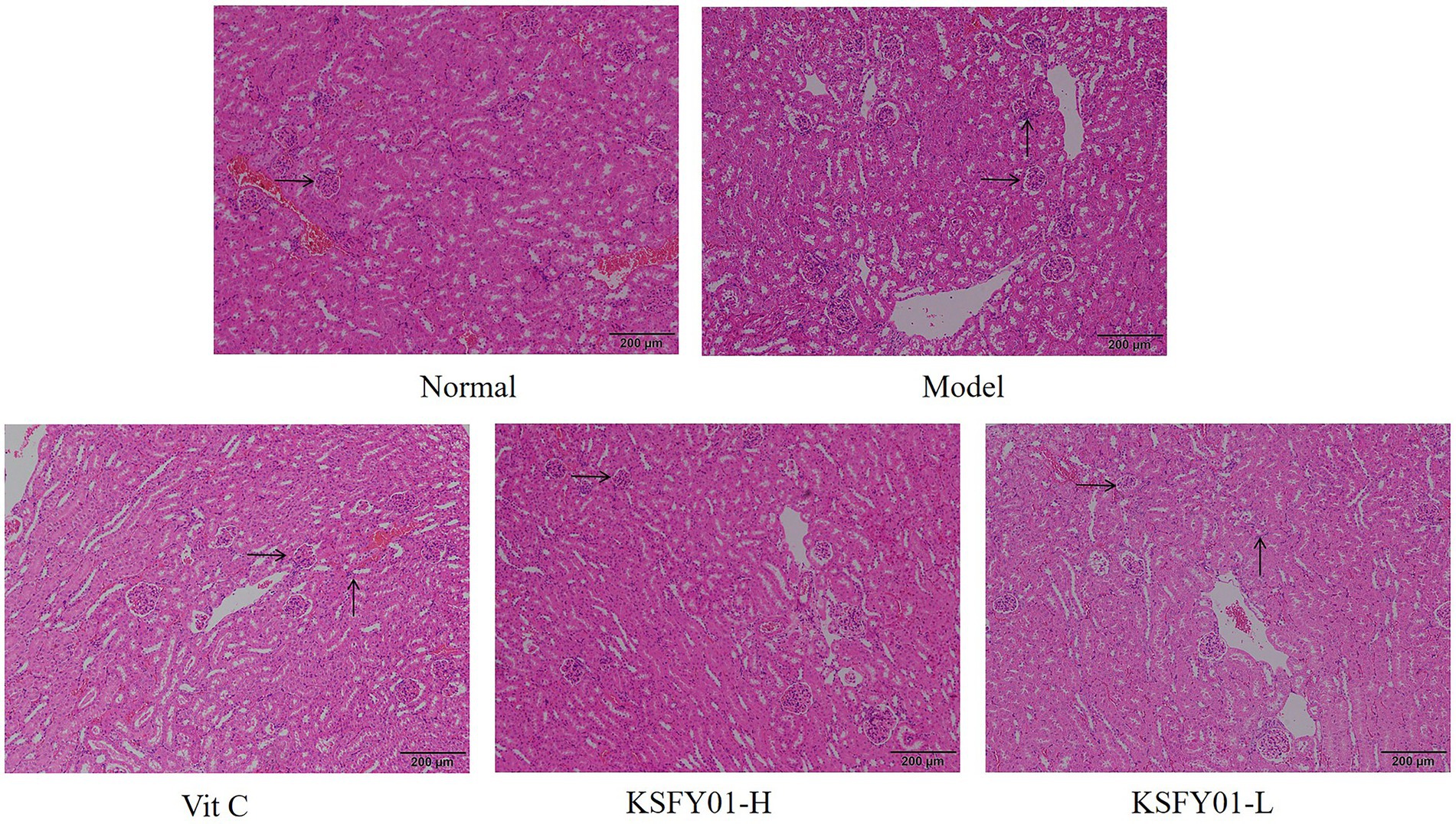
Figure 4. H&E pathological observation of kidney tissue in aging mouse (magnification ×100). Vit C, mice treated with vitamin C (200 mg/kg); KSFY01-H, mice treated with L. plantarum KSFY01 (1 × 1010 CFU/kg); KSFY01-L, mice treated with L. plantarum KSFY01 (1 × 109 CFU/kg). → indicate glomerulus; ↑ indicate inflammatory cell in kidney.
3.6. Expression of mRNA in liver tissue
As shown in Figure 5, the expression of IL-1β and TNF-α mRNA in the liver tissue of the normal mice was the lowest, while the expression of IL-10, SOD1, SOD2, CAT, HO-1, Nrf2, γ-GCS, and NQO1 were the greatest. The model group showed the opposite trend. Vitamin C and L. plantarum KSFY01 could each downregulate IL-1β and TNF-α expression while upregulating IL-10, SOD1, SOD2, CAT, HO-1, Nrf2, γ-GCS, and NQO1 expression. The high-dose L. plantarum KSFY01 group had the strongest ability to cause these expression changes and gave the most similar values to those of the normal mice.
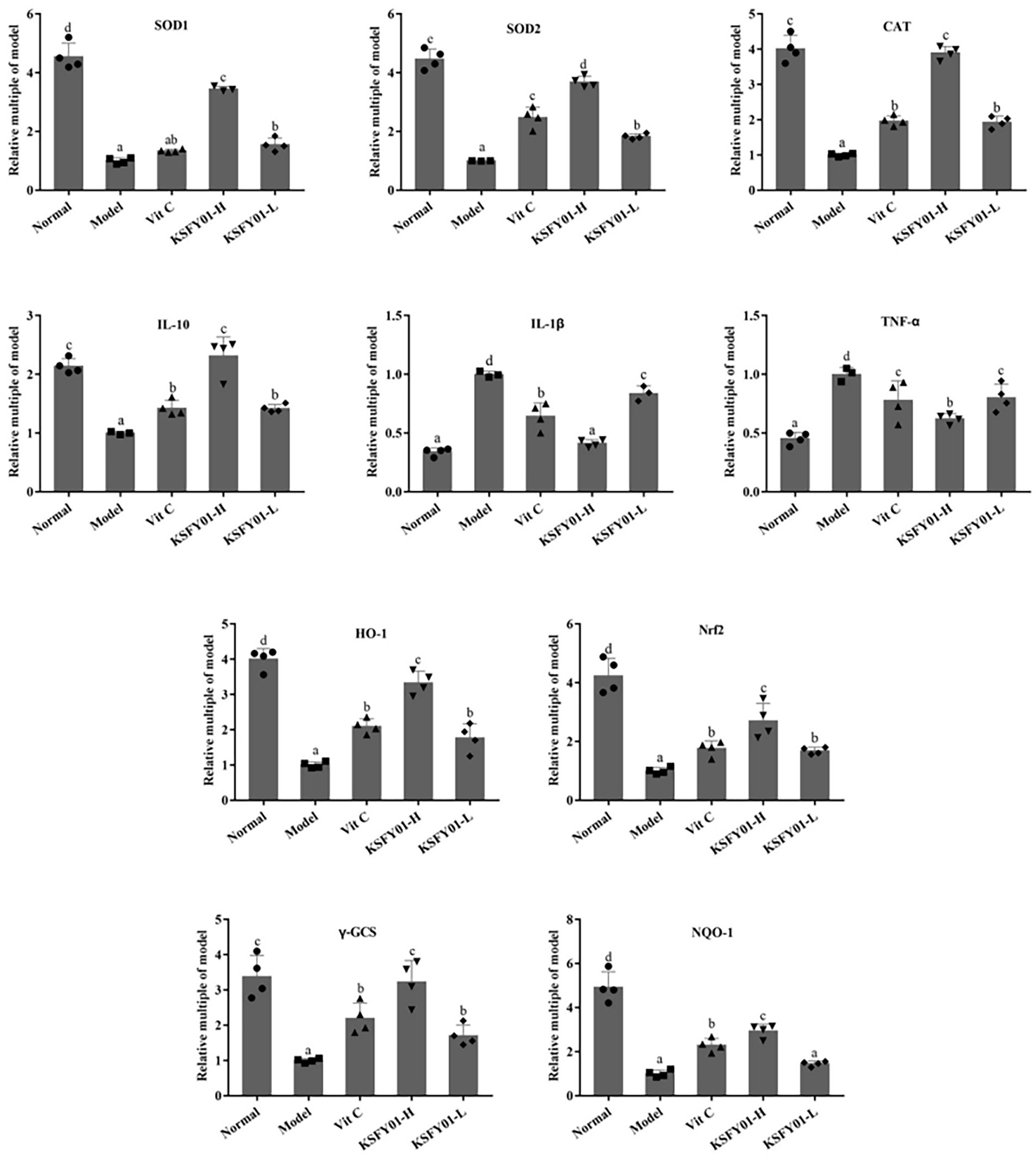
Figure 5. The SOD1, SOD2, CAT, IL-1β, IL-10, TNF-α, HO-1, Nrf2, γ-GCS, and NQO-1 mRNA expressions in mouse liver tissue. a–eValues in the same column with different letter superscripts indicate significant difference (p < 0.05). Vit C, mice treated with vitamin C (200 mg/kg); KSFY01-H, mice treated with L. plantarum KSFY01 (1 × 1010 CFU/kg); KSFY01-L, mice treated with L. plantarum KSFY01 (1 × 109 CFU/kg).
3.7. Expression of mRNA in mouse skeletal muscle tissue
We also analyzed Nrf2 pathway-related genes in mouse muscle tissue. IL-10, IL-1β, TNF-α, SOD1, SOD2, CAT, HO-1, Nrf2, γ-GCS, and NQO1 are oxidative genes involved in this pathway. These genes become abnormally expressed when the expression of Nrf2 changes. As shown in Figure 6, compared with the normal group, the expression levels of IL-10, Cu/Zn-SOD, Mn-SOD, CAT, HO-1, Nrf2, γ-GCS, and NQO1 mRNA in the muscle tissue of the model group were significantly decreased (p < 0.05). Conversely, the expression of IL-1β and TNF-α mRNA increased significantly (p < 0.05). Compared with the model group, the vitamin C and L. plantarum KSFY01 interventions each greatly improved the expression of these genes (p < 0.05) to the degree that they more resembled the normal group. The regulatory effect of L. plantarum KSFY01 was enhanced in a dose-dependent manner. The regulatory effect of high dose L. plantarum KSFY01 was greater than that of low dose L. plantarum KSFY01. These results indicate that L. plantarum KSFY01 has a positive effect on aging-induced muscle damage in mice.
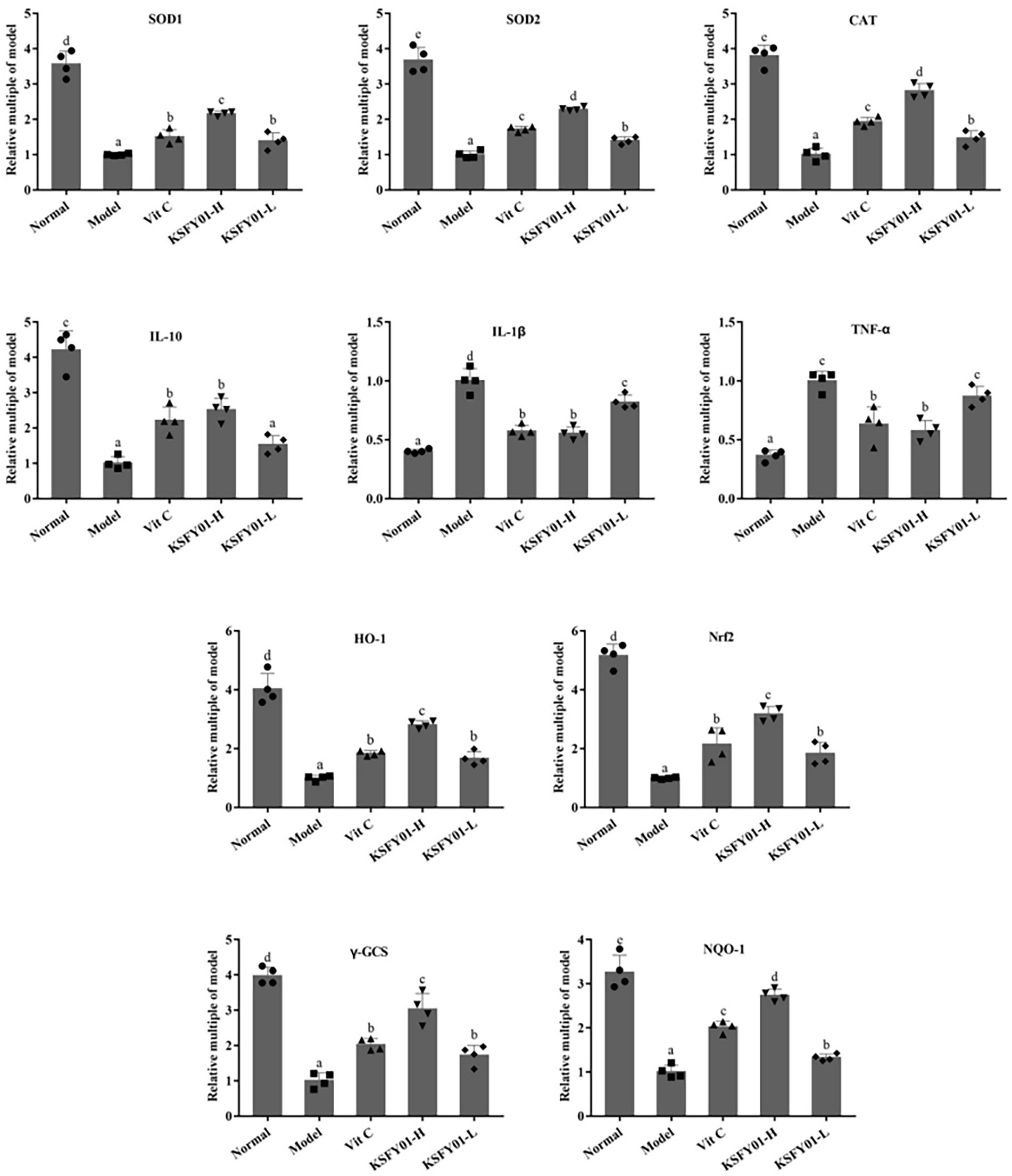
Figure 6. The SOD1, SOD2, CAT, IL-1β, IL-10, TNF-α, HO-1, Nrf2, γ-GCS, and NQO-1 mRNA expressions in mouse skeletal muscle tissue. a–eValues in the same column with different letter superscripts indicate significant difference (p < 0.05). Vit C, mice treated with vitamin C (200 mg/kg); KSFY01-H, mice treated with L. plantarum KSFY01 (1 × 1010 CFU/kg); KSFY01-L, mice treated with L. plantarum KSFY01 (1 × 109 CFU/kg).
4. Discussion
With increasing age, the generation of endogenous free radicals increases in parallel, while homeostasis, stress resilience, and the function of the endogenous antioxidant system all gradually decline. With the abnormal and excessive accumulation of free radicals in the body, the balance between the oxidation and antioxidant systems in the body breaks down, thereby damaging organelles such as the mitochondria and cell membranes. These changes result in cellular dysfunction, in turn affecting the metabolism and accelerating the aging process (Qian et al., 2018). As people age, the conduction velocity of their motor and sensory nerves gradually decreases, and this decline in the function of the nervous system causes the weakening of motor ability, which is accompanied by the frequent occurrence of fatigue (Wu et al., 2022). Exercise can help clear free radicals and thereby allow normal cells to maintain the integrity of mitochondrial structure and function; simultaneously, being in good physical condition can facilitate the body to maintain exercise capacity (Vargas-Mendoza et al., 2021). Improvements in exercise capacity represent the most direct manifestation of bodily resistance to fatigue, and also serve as a key manifestation of resistance to aging (Zhong et al., 2022). Studies have confirmed that some LAB possess good antioxidant and anti-aging effects (Suo et al., 2018). This study also established a mouse aging model by the intraperitoneal injection of D-galactose. This was done to observe the effect of L. plantarum KSFY01 on endurance running in mice in an attempt to verify the intervention effect of L. plantarum KSFY01 on exercise capacity in the aging state. The experimental results also confirmed that L. plantarum KSFY01 could prolong the endurance running time of mice, enhance their exercise capacity, and relieve exercise-induced fatigue.
Long-term continuous exercise causes a substantial degree of bodily fatigue. In this study, we designed a high-intensity exercise treadmill experiment to induce exercise fatigue in mice. The depletion of hepatic glycogen and the accumulation of metabolic byproducts are each thought to contribute to bodily fatigue (Wang et al., 2015; Yi et al., 2021; Zhu et al., 2021). Physical exercise initiates with increases in aerobic muscle activity. If the exercise is intense, anaerobic metabolism will be employed, thereby resulting in the accumulation of LA. Increasing LA then reduces the pH value of both tissues and blood, consequently affecting the physiological and biochemical processes occurring in the body, and ultimately affecting bodily function (Peng et al., 2021). In this way, LA is an important indicator for measuring the level of fatigue. The normal function of LDH in cells is to catalyze the interconversion between pyruvate and lactate (Majid, 2022). BUN is a by-product of metabolic proteins that is produced by the body after excessive glycogen consumption, and it represents a sensitive parameter related to fatigue (Yang et al., 2022). The lower the physical endurance of an individual, the greater the increase in BUN levels during physical exertion (Lee et al., 2021). Therefore, serum levels of LA, LDH, and BUN can indicate both the speed and extent of fatigue that has developed within the body. In addition, the physical exhaustion of mice during long-term high-intensity exercise is related to changes in MG content. After a period of exercise, glycogen in the body is decomposed into lactic acid, and energy is then released to allow muscle activity. The greater the MG reserve, the better the endurance of the body, and the longer the exercise duration (Kim et al., 2020). In this study, compared with the model group, vitamin C and L. plantarum KSFY01 interventions each significantly attenuated the accumulation of these metabolites and regulated blood sugar levels. L. plantarum KSFY01 also reduced muscle damage by regulating LDH levels. This indicates that L. plantarum KSFY01 can relieve fatigue by effectively reducing the accumulation of metabolic byproducts, improving muscle damage, and enhancing energy storage capacity.
There is increasing evidence that high-intensity and acute exercise can each cause damage to hepatocytes by reducing blood flow to the liver and portal vein, which often leads to hepatocyte hypoxia, and ultimately, liver necrosis (Kinoshita et al., 2003). This injury is often accompanied by elevated levels of CK, AST, and ALT (Sangita et al., 2018). In this study, we also found that exercise fatigue caused a significant increase in the liver function indicators of serum CK, ALT, and AST, thereby indicating liver dysfunction. This outcome could be reversed by either the oral administration of vitamin C or L. plantarum KSFY01.
The liver is the central organ of oxidative stress reaction, which can significantly reflect the degree of oxidation of the body, and is also an organ easy to produce free radicals and lipid peroxides. The increase of free radicals caused by aging can be clearly shown in the pathological changes of liver tissue. In addition, with the aging of the body, the kidney gradually shrinks, and the secretion of prostaglandins decreases, leading to vasoconstriction and reduced blood flow. As aging continues, the vascular contraction frequency of liver and kidney decreases, and the blood supply of various organs decreases, which directly reduces the exercise ability (Aschner et al., 2021). In this study, pathological section observation also confirmed that aging led to liver and kidney tissue damage, L. plantarum KSFY01 can effectively reduce the decline and damage of liver and kidney tissues, and one of the important roles it plays may be to improve motor function.
During exercise, many free radicals (such as hydroxyl radicals and superoxide anion radicals) are generated, and this phenomenon is especially pronounced in the aged state (Mariusz and Sławomir, 2013). CAT, GSH, etc. are all important antioxidants and free radical scavengers in the human body. Their existence can maintain the normal oxidative stress homeostasis by removing excess free radicals. The excessive accumulation of ROS can cause oxidative stress and attack biological macromolecules, such as lipids, proteins, and nucleic acids, to form lipid peroxidation products, like MDA (Kheradmand et al., 2009; Li et al., 2022). MDA further damages the structure of the cell membrane, resulting in cell swelling and necrosis. After the intervention of either vitamin C or L. plantarum KSFY01, the content of MDA in the blood of mice was decreased, while the contents of CAT and GSH were increased. Therefore, our results suggest that L. plantarum KSFY01 has potent antioxidant activity and can be used to inhibit aging, whereby it exerts the effect of enhancing exercise endurance and performance.
In terms of inflammatory response, with the growth of age, the function of the immune system gradually declines, which induces some diseases that seriously affect tissues and organs, and aggravates the aging of various systems of the body. TNF-α and IL-1β can activate T cells and enhance the immune response of the body. The results showed that in the model group, proinflammatory factor levels (TNF-α and IL-1β) were the highest, while anti-inflammatory factor levels (IL-10) were the lowest. In the normal group, a completely opposite trend was noted. After treatment with KSFY01, the expression of TNF-α and IL-1β was inhibited, while the expression of IL-10 was enhanced, indicating that the L. plantarum KSFY01 inhibits the production of inflammatory factors.
To elucidate the molecular mechanism by which L. plantarum KSFY01 affects fatigue and fatigue-related organ dysfunction in the aging state, we measured the expression of oxidative stress-related signaling genes in the liver and muscle tissue of mice. Many studies have shown that the Nrf2 signaling pathway serves as an extremely important endogenous defense system in the body (Sunil et al., 2021). Nrf2 is the most critical in the response to oxidative stress in cell. The protein primarily interacts with antioxidant response elements (AREs) to induce the expression of encoded antioxidant proteins and phase II detoxification enzymes (Johnson et al., 2008), thereby increasing cellular resistance against harmful stimuli within cells to play an important protective role. This confers anti-tumor, anti-stress, anti-apoptotic, anti-inflammatory, and neuroprotective effects (Vriend and Reiter, 2015; Zhang et al., 2022). Downstream antioxidant enzymes that are regulated by the Nrf2 signaling pathway include γ-GCS, SOD, CAT, and CSH-Px (Na and Surh, 2005), while the downstream phase II detoxification enzymes that are regulated by the Nrf2 signaling pathway include HO-1 and NQO1 (Keum, 2012). Oxidative stress generates a large amount of ROS to cause peroxidative damage, while the increase of ROS in mitochondria can lead to progressive lipid oxidation, generate more lipid peroxides, and induce tumor necrosis factor TNF-α (Wang W. et al., 2022; Wang L. et al., 2022). TNF-α not only damages the function of the mitochondrial respiratory chain and affects the electron transfer in the respiratory chain, but also opens the permeability transition pore of mitochondria, depletes cytochrome C in mitochondria, and then triggers the apoptosis and necrosis of liver cells (Wang W. et al., 2022; Wang L. et al., 2022). In this study, we used RT-PCR to analyze whether vitamin C and L. plantarum KSFY01 could affect the expression of these genes. The results showed that high-intensity exercise decreased the mRNA expression levels of Nrf2, NQO1, γ-GCS HO-1, SOD1, SOD2, CAT, and IL-10 in both liver and muscle tissue. Conversely, vitamin C and L. plantarum KSFY01 significantly increased the expression of these genes, and the intervention of L. plantarum KSFY01 was more significant. The intervention effect of L. plantarum KSFY01 was dose-dependent, and the intervention effect of high-dose L. plantarum KSFY01FDB was better than that of low-dose L. plantarum KSFY01. These findings suggest that the Nrf2 pathway is the underlying molecular mechanism by which L. plantarum KSFY01 exerts its antioxidant and anti-fatigue effects.
5. Conclusion
In this study, we established a mouse aging model to explore the antioxidant effect of L. plantarum KSFY01 and its effect on improving the ability of mice to run. The experimental results show that L. plantarum KSFY01 could alleviate exercise-induced fatigue and improve the exercise capacity of aging mice by improving their metabolite accumulation, glycogen storage, muscle and liver damage, and levels of oxidative stress. These results provide a reference for the future development of food-derived antioxidants for anti-fatigue effects, and for improving the motor function of the elderly. While these findings are promising, this study only examined the effect of LP-KFY04 in an animal model, so further experimentation is required to determine if a similar effect can be achieved in humans. In addition to fatigue, many diseases are related to oxidative stress, such as aging, diabetes and nonalcoholic fatty liver. In future research, other effects of L. plantarum KSFY01 can be further explored.
Data availability statement
The raw data supporting the conclusions of this article will be made available by the authors, without undue reservation.
Ethics statement
The animal study was reviewed and approved by the Ethics Committee of Chongqing Collaborative Innovation Center for Functional Food.
Author contributions
QC performed the majority of the experiments and wrote the manuscript. CL, YZ, and SW contributed to the data analysis. FL designed and supervised the study and checked the final manuscript. All authors contributed to the article and approved the submitted version.
Funding
This research was funded by the Science and Technology Project of Chongqing (cstc2021jcyj-msxmX0408), the Science and Technology Project of Chongqing Education Commission (KJQN202001614).
Conflict of interest
The authors declare that the research was conducted in the absence of any commercial or financial relationships that could be construed as a potential conflict of interest.
Publisher’s note
All claims expressed in this article are solely those of the authors and do not necessarily represent those of their affiliated organizations, or those of the publisher, the editors and the reviewers. Any product that may be evaluated in this article, or claim that may be made by its manufacturer, is not guaranteed or endorsed by the publisher.
References
Adetuyi, B. O., Adebayo, P. F., Olajide, P. A., Atanda, O. O., and Oloke, J. K. (2022). Involvement of free radicals in the ageing of cutaneous membrane. World News Nat. Sci. 43, 11–37.
Aschner, M., Nguyen, T. T., Sinitskii, A. I., Santamaría, A., Bornhorst, J., Ajsuvakova, O. P., et al. (2021). Isolevuglandins (isoLGs) as toxic lipid peroxidation byproducts and their pathogenetic role in human diseases. Free Radic. Biol. Med. 162, 266–273. doi: 10.1016/j.freeradbiomed.2020.10.024
Johnson, J. A., Johnson, D. A., Kraft, A. D., Calkins, M. J., Jakel, R. J., Vargas, M. R., et al. (2008). The Nrf2-ARE pathway: an indicator and modulator of oxidative stress in neurodegeneration. Ann. N. Y. Acad. Sci. 1147, 61–69. doi: 10.1196/annals.1427.036
Kerksick, C., and Willoughby, D. (2005). The antioxidant role of glutathione and N-acetyl-cysteine supplements and exercise-induced oxidative stress. J. Int. Soc. Sports Nutr. 2, 38–44. doi: 10.1186/1550-2783-2-2-38
Keum, Y. S. (2012). Regulation of Nrf2-mediated phase ii detoxification and anti-oxidant genes. Biomol. Ther. 20, 144–151. doi: 10.4062/biomolther.2012.20.2.144
Kheradmand, A., Alirezaei, M., Asadian, P., Rafiei Alavi, E., and Joorabi, S. (2009). Antioxidant enzyme activity and MDA level in the rat testis following chronic administration of ghrelin. Andrologia 41, 335–340. doi: 10.1111/j.1439-0272.2009.00932.x
Kim, J. H., Cho, H. D., Won, Y. S., Hong, S. M., Moon, K. D., and Seo, K. I. (2020). Anti-fatigue effect of Prunus mume vinegar in high-intensity exercised rats. Nutrients 12:1205. doi: 10.3390/nu12051205
Kinoshita, S., Yano, H., and Tsuji, E. (2003). An increase in damaged hepatocytes in rats after high intensity exercise. Acta Physiol. Scand. 178, 225–230. doi: 10.1046/j.1365-201X.2003.01135.x
LaRocca, T. J., Seals, D. R., and Pierce, G. L. (2010). Leukocyte telomere length is preserved with aging in endurance exercise-trained adults and related to maximal aerobic capacity. Mech. Ageing Dev. 131, 165–167. doi: 10.1016/j.mad.2009.12.009
Lee, M. C., Hsu, Y. J., Ho, H. H., Kuo, Y. W., Lin, W. Y., Tsai, S. Y., et al. (2021). Effectiveness of human-origin Lactobacillus plantarum PL-02 in improving muscle mass, exercise performance and anti-fatigue. Sci. Rep. 11, 19469–19412. doi: 10.1038/s41598-021-98958-x
Leeson, G. W. (2018). The growth, ageing and urbanisation of our world. J. Popul. Ageing 11, 107–115. doi: 10.1007/s12062-018-9225-7
Li, F., Huang, H., Wu, Y., Lu, Z., Zhou, X., Tan, F., et al. (2021a). Lactobacillus fermentum HFY06 attenuates d-galactose-induced oxidative stress and inflammation in male Kunming mice. Food Funct. 12, 12479–12489. doi: 10.1039/d1fo00982f
Li, F., Huang, H., Zhang, Y., Chen, H., Zhou, X., He, Y., et al. (2022). Effect of Lactobacillus fermentum HFY06 combined with arabinoxylan on reducing lipid accumulation in mice fed with high-fat diet. Oxidative Med. Cell. Longev. 2022, 1–15. doi: 10.1155/2022/1068845
Li, F., Huang, H., Zhu, F., Zhou, X., Yang, Z., and Zhao, X. (2021b). A mixture of Lactobacillus fermentum hfy06 and arabinoxylan ameliorates dextran sulfate sodium-induced acute ulcerative colitis in mice. J. Inflamm. Res. 14, 6575–6585. doi: 10.2147/JIR.S344695
Liu, X., Ma, Y., Ma, H., and Yang, W. (2022). Butanediol induces brown blotch (rhizoctonia solani) resistance in creeping bentgrass by enhancing the anti-oxidation of reactive oxygen species and sucrose metabolism. Australas. Plant Pathol. 51, 281–294. doi: 10.1007/s13313-022-00853-0
Long, X., Zeng, X., Tan, F., Yi, R., Pan, Y., Zhou, X., et al. (2020). Lactobacillus plantarum KFY04 prevents obesity in mice through the PPAR pathway and alleviates oxidative damage and inflammation. Food Funct. 11, 5460–5472. doi: 10.1039/D0FO00519C
Majid, A. (2022). Antioxidant effects of nootkatone on enhancing physical endurance in mice subjected to treadmill exercise. Int. J. Pharm. Sci. Res. 13, 2081–2088. doi: 10.13040/IJPSR.0975-8232.13(5).2081-88
Mariusz, G., and Sławomir, K. (2013). A study of free radical chemistry: their role and pathophysiological significance. Acta Biochim. Pol. 60, 1–16. doi: 10.18388/abp.2013_1944
McLeay, Y., Stannard, S., Houltham, S., and Starck, C. (2017). Dietary thiols in exercise: oxidative stress defence, exercise performance, and adaptation. J. Int. Soc. Sports Nutr. 14, 1–8. doi: 10.1186/s12970-017-0168-9
Myers, J., Tan, S. Y., Abella, J., Aleti, V., and Froelicher, V. F. (2007). Comparison of the chronotropic response to exercise and heart rate recovery in predicting cardiovascular mortality. Eur. J. Prev. Cardiol. 14, 215–221. doi: 10.1097/HJR.0b013e328088cb92
Na, H. K., and Surh, Y. J. (2005). EGCG upregulates phase-2 detoxifying and antioxidant enzymes via the Nrf2 signaling pathway in human breast epithelial cells. Cancer Res. 65:367.
Padilha, C. S., Figueiredo, C., Minuzzi, L. G., Chimin, P., Deminice, R., Krüger, K., et al. (2021). Immunometabolic responses according to physical fitness status and lifelong exercise during aging: new roads for exercise immunology. Ageing Res. Rev. 68:101341. doi: 10.1016/j.arr.2021.101341
Peng, F., Yin, H., Du, B., Niu, K., Ren, X., and Yang, Y. (2021). Anti-fatigue activity of purified flavonoids prepared from chestnut (Castanea mollissima) flower. J. Funct. Foods 79:104365. doi: 10.1016/j.jff.2021.104365
Qian, Y., Zhang, J., Zhou, X., Yi, R., Mu, J., Long, X., et al. (2018). Lactobacillus plantarum CQPC11 isolated from Sichuan pickled cabbages antagonizes D-galactose-induced oxidation and aging in mice. Molecules 23, 1–17. doi: 10.3390/molecules23113026
Sangita, P., Biswajit, C., Sreya, C., and Amit, B. (2018). High-intensity exercise induced oxidative stress and skeletal muscle damage in postpubertal boys and girls: a comparative study. J. Strength Cond. Res. 32, 1045–1052. doi: 10.1519/JSC.0000000000002167
Sunil, C., Zheng, X., Yang, Z., Cui, K., Su, Y., and Xu, B. (2021). Antifatigue effects of Hechong (Tylorrhynchus heterochaetus) through modulation of Nrf2/ARE-mediated antioxidant signaling pathway. Food Chem. Toxicol. 157:112589. doi: 10.1016/j.fct.2021.112589
Suo, H., Liu, S., Li, J., Ding, Y., Wang, H., Zhang, Y., et al. (2018). Lactobacillus paracasei ssp. paracasei YBJ01 reduced D-galactose–induced oxidation in male Kuming mice. J. Dairy Sci. 101, 10664–10674. doi: 10.3168/jds.2018-14758
Tan, D. X., Manchester, L. C., Reiter, R. J., Qi, W. B., Karbownik, M., and Calvoa, J. R. (2000). Significance of melatonin in antioxidative defense system: reactions and products. Neurosignals 9, 137–159. doi: 10.1159/000014635
Vargas-Mendoza, N., Angeles-Valencia, M., Morales-González, Á., Madrigal-Santillán, E. O., Morales-Martínez, M., Madrigal-Bujaidar, E., et al. (2021). Oxidative stress, mitochondrial function and adaptation to exercise: new perspectives in nutrition. Life 11, 1–24. doi: 10.3390/life11111269
Vriend, J., and Reiter, R. J. (2015). The Keap1-Nrf2-antioxidant response element pathway: a review of its regulation by melatonin and the proteasome. Mol. Cell. Endocrinol. 401, 213–220. doi: 10.1016/j.mce.2014.12.013
Wang, Y., Liu, J., Cong, X., and Yu, Z. (2015). Anti-fatigue activities of polysaccharides extracted from Hericium erinaceus. Exp. Ther. Med. 9, 483–487. doi: 10.3892/etm.2014.2139
Wang, W., Liu, F., Xu, C., Liu, Z., Ma, J., Gu, L., et al. (2021). Lactobacillus plantarum 69-2 combined with galacto-oligosaccharides alleviates d-galactose-induced aging by regulating the AMPK/SIRT1 signaling pathway and gut microbiota in mice. J. Agric. Food Chem. 69, 2745–2757. doi: 10.1021/acs.jafc.0c06730
Wang, W., Xu, C., Zhou, X., Zhang, L., Gu, L., Liu, Z., et al. (2022). Lactobacillus plantarum combined with galactooligosaccharides supplement: a neuroprotective regimen against neurodegeneration and memory impairment by regulating short-chain fatty acids and the c-Jun N-terminal kinase Signaling pathway in mice. J. Agric. Food Chem. 70, 8619–8630. doi: 10.1021/acs.jafc.2c01950
Wang, L., Zhao, Z., Zhao, L., Zhao, Y., Yang, G., Wang, C., et al. (2022). Lactobacillus plantarum DP189 reduces α-SYN aggravation in MPTP-induced Parkinson’s disease mice via regulating oxidative damage, inflammation, and gut microbiota disorder. J. Agric. Food Chem. 70, 1163–1173. doi: 10.1021/acs.jafc.1c07711
Wu, J., Chen, H., Wang, D., and Zhao, X. (2022). Effect of Clerodendranthus spicatus (Thunb.) C. Y. Wu on the exercise ability of D-galactose-induced oxidative aging mice. Food Science and Technology (Brazil) 42, 1–12. doi: 10.1590/fst.09822
Yang, C., Yang, J., Tan, L., Tang, P., Pen, T., Gao, T., et al. (2022). A novel formula comprising wolfberry, figs, white lentils, raspberries, and maca (WFWRM) induced antifatigue effects in a forced exercise mouse model. Evid. Based Complement. Alternat. Med. 2022, 1–12. doi: 10.1155/2022/3784580
Yi, R., Feng, M., Chen, Q., Long, X., Park, K. Y., and Zhao, X. (2021). The effect of Lactobacillus plantarum CQPC02 on fatigue and biochemical oxidation levels in a mouse model of physical exhaustion. Front. Nutr. 8:641544. doi: 10.3389/fnut.2021.641544
Zhang, J., Chen, L., Zhang, L., Chen, Q., Tan, F., and Zhao, X. (2021). Effect of Lactobacillus fermentum HFY03 on the antifatigue and antioxidation ability of running exhausted mice. Oxidative Med. Cell. Longev. 2021, 1–11. doi: 10.1155/2021/8013681
Zhang, X., Zhang, Y., Zhou, M., Xie, Y., Dong, X., Bai, F., et al. (2022). DPHC from Alpinia officinarum ameliorates oxidative stress and insulin resistance via activation of Nrf2/ARE pathway in db/db mice and high glucose-treated HepG2 cells. Front. Pharmacol. 12:792977. doi: 10.3389/fphar.2021.792977
Zheng, Z., Yang, X., Liu, J., Qian, P., Hao, L., Wang, Z., et al. (2017). Effects of wheat peptide supplementation on anti-fatigue and immunoregulation during incremental swimming exercise in rats. RSC Adv. 7, 43345–43355. doi: 10.1039/C7RA07860A
Zhong, H., Shi, J., Zhang, J., Wang, Q., Zhang, Y., Yu, P., et al. (2022). Soft-shelled turtle peptide supplementation modifies energy metabolism and oxidative stress, enhances exercise endurance, and decreases physical fatigue in mice. Foods 11:600. doi: 10.3390/foods11040600
Zhou, X., Sun, H., Tan, F., Yi, R., Zhou, C., Deng, Y., et al. (2021). Anti-aging effect of Lactobacillus plantarum HFY09-fermented soymilk on D-galactose-induced oxidative aging in mice through modulation of the Nrf2 signaling pathway. J. Funct. Foods 78:104386. doi: 10.1016/j.jff.2021.104386
Keywords: Lactobacillus plantarum, D-galactose, oxidative, exercise, mRNA
Citation: Chen Q, Liu C, Zhang Y, Wang S and Li F (2022) Effect of Lactobacillus plantarum KSFY01 on the exercise capacity of D-galactose-induced oxidative stress-aged mice. Front. Microbiol. 13:1030833. doi: 10.3389/fmicb.2022.1030833
Edited by:
Mariana Monteiro, Federal University of Rio de Janeiro, BrazilReviewed by:
Salam A. Ibrahim, North Carolina Agricultural and Technical State University, United StatesJuncai Hou, Northeast Agricultural University, China
Copyright © 2022 Chen, Liu, Zhang, Wang and Li. This is an open-access article distributed under the terms of the Creative Commons Attribution License (CC BY). The use, distribution or reproduction in other forums is permitted, provided the original author(s) and the copyright owner(s) are credited and that the original publication in this journal is cited, in accordance with accepted academic practice. No use, distribution or reproduction is permitted which does not comply with these terms.
*Correspondence: Fang Li, ✉ bGlmYW5nQGNxdWUuZWR1LmNu