- 1School of Hydraulic and Environmental Engineering, Changsha University of Science and Technology, Changsha, China
- 2Key Laboratory of Dongting Lake Aquatic Eco-Environmental Control and Restoration of Hunan Province, Changsha, China
- 3Changjiang River Scientific Research Institute, Wuhan, China
As an important environmental protection measure, the Poplar Ecological Retreat (PER) project aims to restore the ecology of the Dongting Lake (DL, China’s second largest freshwater lake) wetland. And its ecological impact is yet to be revealed. This study selected soil bacterial community structure (BCS) as an indicator of ecological restoration to explore the ecological impact of PER project on DL wetland. Soil samples were collected from reed area (RA, where poplar had never been planted, as the end point of ecological restoration for comparison in this study), poplar planting area (PA), poplar retreat for 1-year area (PR1A) and poplar retreat for 2 years area (PR2A), then their soil properties and BCS were measured. The results showed that the PER project caused significant changes in soil properties, such as the soil organic matter (SOM) and moisture, and an increase in the diversity and richness index of soil BCS. The Shannon-wiener index of RA, PA, PR1A and PR2A were 3.3, 2.63, 2.75 and 2.87, respectively. The number of operational taxonomic units (OTUs) changed similarly to the Shannon-wiener index. The Pearson correlation analysis and redundancy analysis (RDA) showed that the poplar retreat time, SOM and moisture content were the main factors leading to the increase of BCS diversity. All of these indicated that after the implementation of the PER project, the ecology of the lake area showed a trend of gradual recovery.
Introduction
Monoculture of large-scale fast-growing tree plantations is considered to be one of the threats to biodiversity (Kindlmann et al., 2017; Egoh et al., 2020). Generally, they are superior to native species in competition for water, light and nutrients (Li et al., 2014b; Toledo et al., 2020), which may lead to transformation or even disappearance of natural ecosystems (Gardner et al., 2015; Sica et al., 2016). However,the use of fast-growing plantations is still widespread around the world to develop economies, meet landscape and other needs (Fashing et al., 2012). These plantations not only alter the structure, composition, and function of the natural ecosystem (Giejsztowt et al., 2019; Wang et al., 2020a), and would also affect the physicochemical and biological properties of the soil in various ways, such as altering the understory plant diversity (Li et al., 2014b), root and litter composition (Xu et al., 2014), depleting soil nutrients and water (Ferre et al., 2014), and intercepting and filtering surface runoff (Crampe et al., 2021). With the increasing attention on environmental issues in the country, it is of great significance to make further study on the impact of plantation forests on the ecosystem.
Dongting Lake (DL) includes three important international wetlands and four nature reserves (Ren et al., 2020). It provides water and soil conservation, carbon sequestration and other services (Sun et al., 2017; Deng et al., 2018), and plays an important role in sustaining regional ecological balance, regulating water resources of the Yangtze River system, and protecting biodiversity and rare species resources (Wang et al., 2020b; Hu et al., 2021). In the past century, however, the anthropogenic impacts and inconsequent land use have resulted in high level of ecological degradation in DL wetland (Sun et al., 2018; Wang et al., 2021). Poplar (Populus deltoides) as a common tree species for human afforestation, is a Populus genus of Salicaceae with moist-loving. It has a fast growth rate, strong adaptability and considerable economic benefits. In the 1970s, poplar trees began to be planted in Dongting Lake. With the rapid growth of planting area, poplar has become one of the important tree species for the construction of short-period industrial raw material forest in DL wetland (Li et al., 2014b). However, the single planting of large-scale fast-growing poplar plantation has led to various environmental problems, such as serious damage to the native vegetation of wetlands, decline of groundwater level, sharp changes in landscape pattern etc. (Li et al., 2014a; Sun et al., 2017; Lu et al., 2020). Coupled with the planting method of digging trenches and raising ridges, the service function of the wetland ecosystem was further destroyed (Du et al., 2011; Qu et al., 2022). These problems have caused the government to pay attention to the ecological restoration of the DL wetland (Jing et al., 2016). In order to alleviate a number of environmental problems caused by large-scale poplar planting, the “Poplar Ecological Retreat” (PER) project was launched in 2017 (Deng et al., 2022). About 6.5 million poplar trees had been retreated by the end of 2018, and all poplars in the core area of DL Nature Reserve had been removed (Bai et al., 2018; Hu et al., 2020). As a response to the governance, the wetland coverage and landscape pattern has transformed; and the regional ecological characteristics and functions, such as biodiversity, climate regulation, have also changed (Gong et al., 2019).
Several studies have been conducted on the PER project in the DL area. For example, Hu et al. (2020) founded that PER project improved the wetland water yield by changing landscape pattern; Qu et al. (2022) reported that habitat suitability of Anatidae and Ardeidae migratory birds could be significantly improved by PER project; The implementation of the PER project, the density of soil seed banks and the number of species increased significantly, and above-ground plant diversity gradually recovered (Deng et al., 2022). The PER project has considerably optimized the landscape pattern of DL wetland and promoted the improvement of the ecological environment in this area (Lu et al., 2020). Overall, these studies contributed to a comprehensive understanding of the ecological restoration of Dongting Lake wetland after the implementation of the PER project. Nevertheless, we know little about the PER project on soil biological characteristics, particularly the influence of the bacterial community structure (BCS).
Microorganisms have a great significance in soil structure formation, decomposition of SOM and minerals (Harris, 2009), maintaining system material transformation and energy flow (Hu et al., 2013), nitrogen fixation (Hartman et al., 2008), regulating growth of plants (Poret-Peterson et al., 2007), soil-borne diseases control (Wu et al., 2015) and other aspects. As the most active part of soil, microorganisms are extremely sensitive to human activities and external environmental changes compared with other large organisms, so as an evaluation index of soil quality has been widely recognized (Hargreaves et al., 2003; Card and Quideau, 2010; Wright and Wimberly, 2013). These means that the PER project will inevitably induce changes in soil microorganisms.
In this study, the soil BCS was applied as an indicator to investigate the ecological responses of the DL wetland to different time series of the implementation of the PER project. The purposes of this study were: (1) to analyze the response of the BCS and soil properties to different time series of the implementation of PER project in DL wetland; (2) to increase our knowledge of the relationship among human activities, soil properties and BCS in DL wetland; and (3) to provide scientific statistics and theoretical support for ecological restoration and environmental research in DL wetland.
Study area and methods
Study sites
DL wetland (27°39′-29°51’N, 111°19′-113°34′E), located on the southern bank of Jingjiang River in the middle reaches of the Yangtze River (Dai et al., 2016). It receives water from the Yangtze River and its four tributaries (Xiangjiang, Zijiang, Yuanjiang and Lishui), then flows into the Yangtze River (Du et al., 2011). Its natural lake area is 2,625 km2, and the main Lake District is connected by floodways and numerous river networks (Ding and Li, 2011). This region has abundant rainfall and heat. The area of the lake and water level will change with the monsoon-driven precipitation, presented as the rainy season (May–October) with high area and water level and dry season (November – following April) with low area and water level (Wu et al., 2017). High water levels in the rainy season may cause poplars to be partially submerged, making it difficult to implement the PER project, so that forestry workers need to cut down poplar trees and transport them out during the dry season. Our sampling time was also scheduled for the dry season. In addition, the ravines originally dug need to be leveled for subsequent ecological restoration. Through the interpretation and comparison of remote sensing satellite images, the selected research area is located in the west DL. The specific location confirmed by field investigation is illustrated in Figure 1.
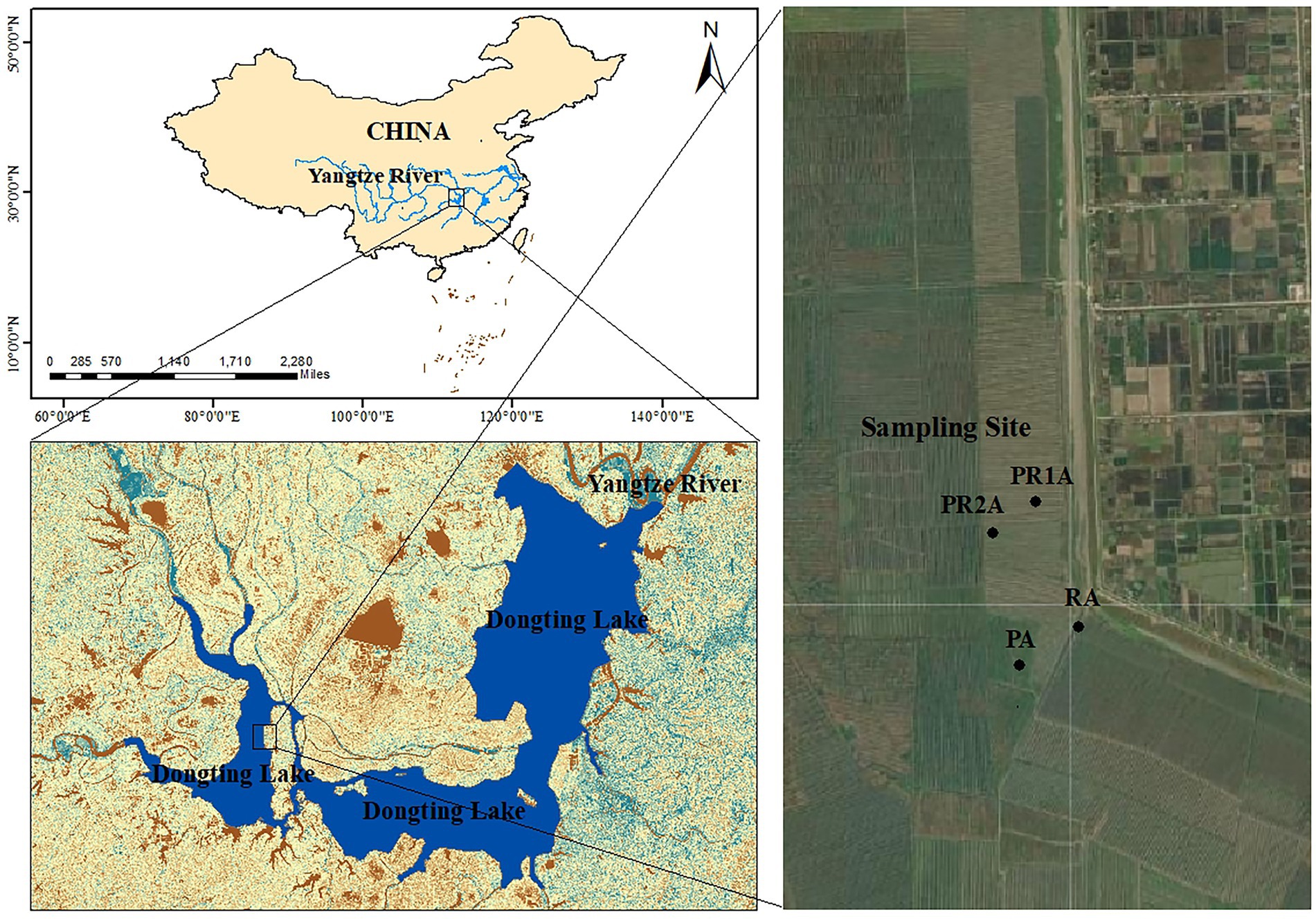
Figure 1. Locations of Dongting Lake and sample points of the reed area (RA), poplar planting area (PA), poplar retreat for 1-year area (PR1A) and poplar retreat for 2 years area (PR2A).
Samples collection and soil properties determination
Soil samples at depths of 5 to 15 cm (Inacio et al., 2019) from 4 areas of DL wetland were collected in December 2020 and March 2021. Three parallel soil samples from each area were collected as the visible plant root matter and impurities were removed, and placed in plastic bags. Utilize a vehicle refrigerator to transport the samples to the laboratory. Samples for determination of soil properties were stored in a laboratory-specific refrigerator at 4°C, whereas samples for high-throughput sequencing were stored in an ultra-low temperature refrigerator at −50°C.
Soil organic matter (SOM) was measured by the combustion loss method, which means burning at 550°C for 4 h (Ghabbour et al., 2014). The quality method was used to determine the soil moisture, that is, the mass loss during overnight stoving at 105°C (Wu et al., 2015). Soil pH value was measured by portable pH meter (The ratio of dry soil to water is 1:2.5) (Damianova et al., 2016). The ammonium nitrogen was examined by indiophenol blue spectrophotometry (extracted with potassium chloride solution). The nitrate nitrogen was measured by phenol disulfonic acid spectrophotometry (Yan and Fu, 2021).
High-throughput sequencing
Soil samples for molecular genome analysis were collected in March 2021. Extract DNA from 0.2 g of soil from three parallel samples in each region using the MagaBio Soil Genomic DNA Purification Kit. After that, Thermo NanoDrop One was used to detect the purity and concentration of the DNA, without dilution. The variable V4 region of the 16S rDNA coding genes was amplified by the bacterial universal primers GC 515F/806R. The PCR mixture containing 50 ng of template DNA, 2 × Premix Taq PCR MasterMix (TaKaRa Biotechnology Co., Dalian, China) 25 μl, 1 μl (10 μM) of each primer (-F and -R), then Nuclease-free water was injected to make the final volume 50 μl. The PCR amplification process was executed as follows: 30 cycles of denaturation were carried out after the initial denaturation (94°C, 5 min), including denaturation (94°C, 30 s), annealing (52°C, 30 s), extension (72°C, 30 s), and single elongation (72°C, 10 min), ended in a temperature of 4°C finally. Agarose gel (1%) electrophoresis was applied to detect the concentration and fragment length of PCR products, and the samples with the main band length between the normal range (290–310 bp) can be used for further experiments. After the PCR product concentration was compared by the GeneTools Analysis Software (SynGene, V.03.05.0,) we computed the volume needed for each sample in accordance with the equal quality principle, then mixed the PCR product. The PCR mixed products were recycled by Gel Extraction Kit (Omega, USA), and the target DNA fragments were eluted by the TE buffer solution. Follow the standard process to build the library, then the amplified sublibrary was sequenced by using Illumina Nova 6,000 platform of Magigene Biotechnology Co., Ltd. (Guangzhou, China).
Bioinformatic analyses
Fastp (V.0.14.1) was used to execute window clipping of raw reads data on both ends (Kong, 2011), and effective clean tags were obtained. In accordance with the primer information of the head and tail of the sequence, the primer was deleted by cutadapt software, and the quality-controlled paired-end clean reads was gained. For dual-end sequencing data, usearch-Fastq_mergepairs (V.10, the preset minimum length of overlap is 16 bp, and the splicing sequence of the overlap area allows the maximum error to be 5 bp) was used to filter disconformed tags, then obtain the original stitching sequences (Raw Tags) on the basis of the overlap relationship among PE reads. Use the UCHIME algorithm in QIIME (V.2020.11.0) pipeline to remove chimeric sequences (Leray and Knowlton, 2016). Bacterial sequences were categorized into operational taxonomic units (OTUs) at a threshold value of 97% comparability by utilizing UPARSE (Edgar, 2013). Usearch-sintax (V.10.0.240) was used to compare the representative sequences of each OTU with UNITE (Abarenkov et al., 2010) and RDP (Cole et al., 2009) databases to obtain species annotation information (the default confidence threshold was set at 0.8), so as to understand the species origin of all sequences.
Data processing and analysis
The alpha diversity of bacterial community was measured by Chao1 index and Shannon-wiener diversity index. After testing, all the data conform to the normal distribution, and the variance of each sample population is equal, so the mean values of physicochemical parameters of reed area (RA), poplar planting area (PA), poplar retreat for 1-year area (PR1A) and poplar retreat for 2 years area (PR2A) were compared with one-way analysis of variance (ANOVA), while the post-hoc Tukey’s test was applied to evaluate significant differences in the data (p < 0.05). Using Pearson correlation analysis to examine the single factor correlation between the parameters. The pluralistic relationships among the soil properties, the poplar retreat time and the BCS were confirmed by Canoco (V.5.0, Centre for Biometry, Wageningen, the Netherlands). Detrended correspondence analysis (DCA) showed that the length of the first sorting axis of DCA was 1.98, which indicated the linear response of species. Consequently, the default setting is used for redundancy analysis (RDA) to coordinate the soil characteristics and BCS. All analyses were performed by using SPSS (V.26) and R language.
Results
Responses of soil properties
Soil property parameters for each area are listed in Table 1. Several properties of soil samples from different regions have significant differences, especially SOM (F = 28.220, p < 0.001) and moisture (F = 20.861, p < 0.001). The SOM and moisture in PA were significantly lower than those in RA in December 2020 and March 2021. Compared with PA, the SOM and moisture in PR1A and PR2A increased, but still lower than that in RA. The pH, and the contents of nitrate nitrogen and ammonium nitrogen showed no significant differences among RA, PA, PR1A and PR2A.
Comparing the soil properties of each sample in December 2020 and March 2021, we found that there was no significant change in soil moisture, SOM, ammonium nitrogen, nitrate nitrogen and pH in RA and PA. The SOM and moisture increased in PR1A and PR2A from December 2020 to March 2021. The Pearson correlation coefficients among the factors considered listed in Table 2. The moisture of all soil samples was significantly positively correlated with SOM.
Response of bacterial community structure
After quality filtration, the 4 areas were sequenced to produce a total of 671,698 high-quality 16SrRNA gene sequences, then they were classified into 11,413 different OTUs. The shared bacterial OTUs among four areas was 3,072, which accounted for 39.8, 58.0, 49.6 and 47.3% of the total OTUs from RA, PA, PR1A and PR2A sites, respectively (Supplementary Figure S1). The top 15 most abundant taxa of the communities have no difference between regions at the phylum level (Supplementary Figure S2), while the Proteobacteria, Actinobacteria, Bacteroides and Chloroflexi were the dominant phyla. The relative abundance of Bacteroidetes in samples of PR2A (12.3%) was higher than PA (p < 0.001) and PR1A (p < 0.001). In the heat map, the top 30 most abundant taxa corresponding to 4 regions confirms the differences in bacterial community composition (Supplementary Figure S3).
As shown in Figure 2, the chao1 index performed a significant difference (F = 8.655, p = 0.007), as the average value dropped from 5010.6 in RA to 3730.0 in PA, then rose to 4115.4 and 4156.5 in PR1A and PR2A. A significant difference (F = 7.947, p = 0.009) was also shown in Shannon-wiener index, as the average value decreased from 3.3 in RA to 2.63 in PA, then rose to 2.75 and 2.87 in PR1A and PR2A, respectively. The results of Chao1 and Shannon-Wiener index indicated that there were significant differences in BCS among soil samples from RA, PA, PR1A and PR2A. Overall, the BCS of RA performed more abundant and diverse than that of PA. 2 years after the implementation of the PER project, the BCS diversity has gradually increased, but it was still lower than that in RA.
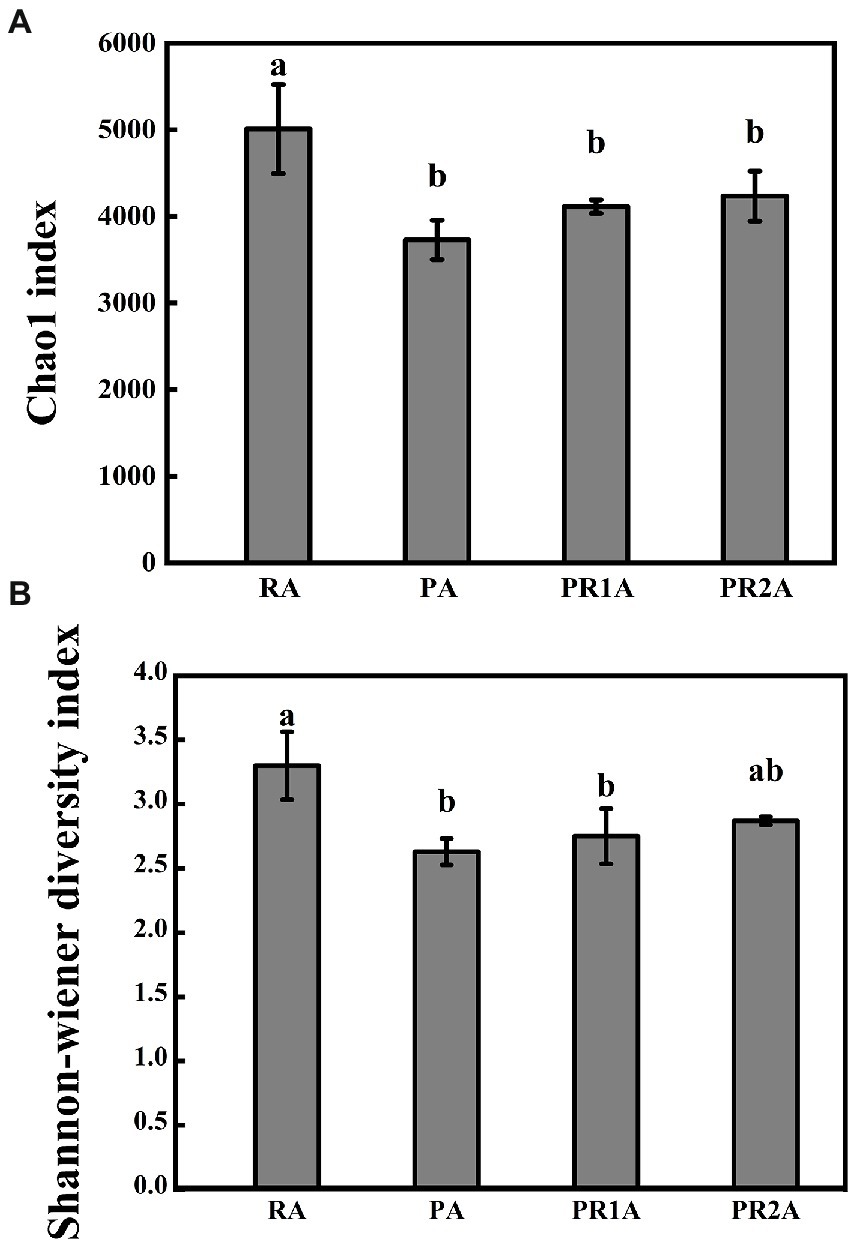
Figure 2. Chao1 index (A) and Shannon-Wiener diversity index (B) of the reed area (RA), poplar planting area (PA), poplar retreat for 1-year area (PR1A) and poplar retreat for 2 years area (PR2A) in March 2021. The vertical line above the histogram correspond to standard errors, while significant differences (p < 0.05) represented by different letters.
RDA biplots of the BCS and soil characteristics are illustrated in Figure 3. And Table 3 revealed that all soil properties parameters could explain 78.5% of the species variation. The poplar retreat time, the SOM and the moisture exerted extremely significant impacts on soil BCS. The order of the influence of these factors on BCS was: poplar retreat time > SOM > moisture > nitrate nitrogen > pH > ammonia nitrogen.
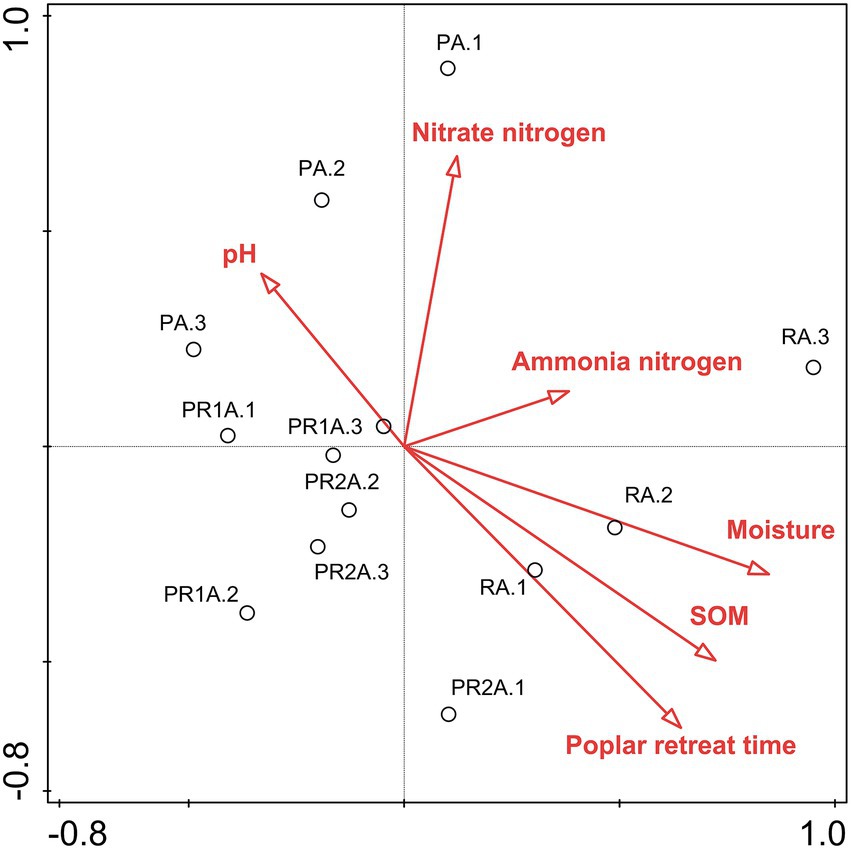
Figure 3. Two-dimensional ranking map of bacterial community structure and environmental factors based on Redundancy analysis. Samples are shown as circles; environmental factors are indicated by straight lines and hollow arrows. SOM: soil organic matter.
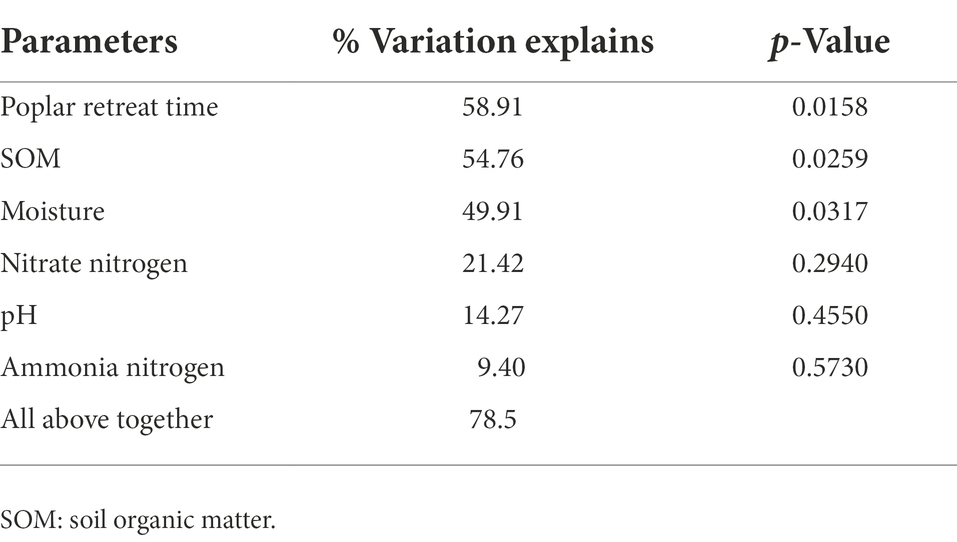
Table 3. Monte Carlo experiment to test the influence of poplar retreat time and soil properties on the results.
Discussion
Response of soil properties
The results showed that, taking RA as control, the PER project had a significant influence on the soil properties. Reed has better carbon storage capacity than poplar, which may lead to lower SOM in PA than that in RA (Nocentini and Monti, 2017). Previous studies have shown that, compared with poplar, reed has a higher underground biomass development, more prosperous root system, and is more difficult to decompose than poplar litter (Monti and Zatta, 2009; Chimento and Amaducci, 2015), which makes more biological residues in the root system of reed and resulting in higher SOM. Moreover, understory plants were significantly correlated with the SOM (Abebe et al., 2020), the lack of understory plants would lead to low carbon storage in plantations (Lyu et al., 2021). The litter accumulated under the poplar plantation will cause direct physical obstacles to the germination of understory plants (Ellsworth et al., 2004; Yu et al., 2021a), and will also directly or indirectly affect the survival and renewal of understory plants by affecting the microenvironment such as light, temperature, and humidity, thereby reducing the SOM content (Graae and Heskjær, 1997; Esteso-Martínez and Gil-Pelegrín, 2004; Bo et al., 2018). Other studies have reported that poplar plantations could lower the water table and dry out the soil (Wilske et al., 2009; Boothroyd-Roberts et al., 2013), which is consistent with our research results. As shown in Table 1, the soil moisture in PA was significantly lower than that in RA. It can be speculated that the leaf area index of woody plants is usually higher than herbaceous plants, so that the stomatal conductance and transpiration rate of poplar are higher than reed (Wilske et al., 2009). While plant transpiration is considered to be the main mechanism of soil water efflux (Huang et al., 2011), which ultimately leads to the difference in soil moisture between PA and RA. Some studies have also shown that fast-growing plantations also have pumping effects in other ecosystems (Hernández-Santana et al., 2008; Tan et al., 2011). For example, Boothroyd-Roberts et al. (2013) found that due to transpiration, water content in plantation is lower than that in wasteland and secondary forest; Wilske et al. (2009) also reported that poplar dries the wetland soil by absorbing groundwater and transpiration. In this study, the SOM and moisture contents of samples in each region were in the order of PA < PR1A < PR2A < RA. This is because after the implementation of the PER project, the soil moisture by plantation transpiration loss no longer, and the growth of plants also have better environment conditions such as illumination, temperature, which cause the SOM and moisture to gradually increase in the process of natural restoration of wetlands (Figure 1).
Previous studies have reported that wildlife can also affect the properties of soil (Elhottová et al., 2012). Poplar blocked the landing of migratory birds, crowding out the habitat of wintering migratory birds (Qu et al., 2022). The poplar planting method of digging trenches and lifting ridges made the ground ravine crisscross, which leads to the destruction of fish spawning and migration channels (Li et al., 2020). As mentioned above, a lack of understory plants could also lead to a decrease in herbivores (Veblen et al., 2016). All of these destroyed the diversity of wildlife. After the implementation of the project, the living space of migratory birds, fish and wildlife was restored and the breeding conditions were better, resulting in an increase in their diversity and abundance, which would lead to changes in soil properties, such as SOM and moisture. This is because wildlife can import unstable carbon to the soil through the deposition of excrement, provoke new underground biomass and increase SOM (Bardgett et al., 1998; Olsen et al., 2011); they also change the rate of infiltration and drainage of soil water by compacting the soil and digging holes (Olsen et al., 2011). In a word, increased wildlife also improved SOM and water content in the soil.
Besides, Table 2 shows that there is a significant correlation between SOM and moisture, which consistent with the results obtained by Wu et al. (2015). This is because SOM improved the water holding capacity by ameliorating the colloid condition and improving the porosity of the soil (Douterelo et al., 2009). The higher the amount of SOM, the more stable and homogeneous the aggregate structure of the soil, and the more organic colloids are carried, thus adsorb and store more water (Dai et al., 2020). While soil moisture can affect plant uptake of mineral substance and alter the growth of plants by changing the concentration of soil mineral element ions, and ultimately the soil nutrient status (Kajiura et al., 2012); and can also affect SOM decomposition and carbon storage by altering soil redox conditions (Chen et al., 2018; Yu et al., 2021b). For example, Wu et al. (2013) and Qu et al. (2021) reported that SOM content would increase with soil moisture in a certain range; while Boothroyd-Roberts et al. (2013) and Lal (2020) founded that the recovery of SOM would also contribute to the increase of water content.
Response of bacterial community structure
In this study, soil samples from areas that had never been planted with poplar (RA) showed higher BCS richness and diversity than the samples from areas that had been planted with poplar. After poplar retreat, the diversity of soil BCS increased gradually in the process of natural restoration of wetland. The PER project affects soil microbial community by changing soil properties. As mentioned above, PER projects affect soil properties by improving understory plant survival and regeneration conditions, reducing transpiration, restoring wildlife living spaces. Soil microorganism was extremely sensitive to the changes in soil properties, such as moisture and SOM. For example, water as a resource, solvent and transport medium can directly affect the structure of microbial communities (Schimel, 2018); and can also drive the growth and change of microbial communities by affecting the activity of various enzymes in the soil (Brockett et al., 2012; Zhang et al., 2021). In this study, the shortage of water caused by the planting of poplars resulted in a decrease in soil microbial abundance in soil samples of PA, which was attributed to limited dispersal of extracellular enzymes and nutrients in the soil due to water scarcity, thus inhibiting microbial growth (Wu et al., 2013; Yang et al., 2017). He et al. (2020) also reported that water deficiency would lead to a decrease in microbial abundance. After the implementation of the PER project, the restoration of wildlife populations and plant diversity injected more organic matter into the soil, resulting in an increase in the diversity of microbial community structures, as the SOM is an essential substrate for microbial survival (Marshman and Marshall, 1981; Yu et al., 2022). These soil characteristics can regulate nutrients and anaerobic or aerobic processes in the soil to affect microbial metabolism, and then change the community structure of microorganisms (Gutknecht et al., 2006; Card and Quideau, 2010). This study concluded that the SOM and the moisture imposed highly significant impacts on the soil BCS. Other studies also founded that the soil microbial diversity and structure could be affected by soil elements, such as SOM and moisture (Tang et al., 2011; Qu et al., 2021).
Noteworthy, taking the soil in the RA as the end point of the restoration for the control, the properties of the soil and the BCS diversity showed a gradual recovery trend after the implementation of the PER project. Though this change is not significant within 2 years, it can be speculated that with the extension of ecological restoration time, this change will further expand and reach a significant level.
After ecological restoration measures have achieved good results, how to further maintain the stability of the ecosystem is also an important topic. Material circulation is important for maintaining the stability of ecosystems. Studies have shown that the changes in soil BCS would directly affect the material circulation of the ecosystem (Jia et al., 2022; Zhu et al., 2022), so the changes of BCS caused by the PER project will also have an impact on the material circulation of DL wetland. Nevertheless, how the PER project will affect the material circulation of the DL ecosystem and what significance it has for maintaining the stability of the DL wetland ecosystem need to be further studied.
Conclusion
The soil properties and BCS before and after the implementation of the PER project in DL wetlands was effectively estimated by means of high-throughput sequencing and methods of correlation and significance analysis. The study led to the following conclusions: the PER project has significantly changed the soil properties, especially the SOM and the moisture; there were also significant differences in BCS, the Chao1 index of PA, PR1A, PR2A and RA were 3730.0, 4115.4, 4156.5 and 5010.6, respectively. And the change trend of Shannon index is similar to Chao1 index, both of them increase steadily after the implementation of the project. The correlation analysis and redundancy analysis indicated that the difference in poplar retreat time, SOM and moisture content were the key influence of soil microbial community structure. The PER project would be the root cause for these changes.
The improvement of BCS diversity and richness indicates the effectiveness of PER project in ecological restoration of the DL wetland, suggesting that the PER project is a scientific demonstration for restoring the ecological environment of the DL wetland. In addition, these results also increase our knowledge of the relationship between human activities and environmental change in DL wetland, and provide scientific data and theoretical support for the ecological restoration of Dongting Lake wetland.
Data availability statement
The datasets presented in this study can be found in online repositories. The names of the repository/repositories and accession number(s) can be found at: NCBI – PRJNA878642, SAMN30734537.
Author contributions
HW: ideas, experimental resources, administrative support, funding support, writing review, academic supervision, and manuscript revision. SX: experimental design and implementation, material and equipment purchase, field research, data collection and analysis, and manuscript writing. JD and JC: concept, method, data auditing, and manuscript revision. YX: administrative support, funding support, and academic supervision. XQ and GW: software, field research, and manuscript revision. RY: field research, experiments, and manuscript revision. All authors contributed to the article and approved the submitted version.
Funding
This research was financially supported by the National Natural Science Foundation of China (52270195, 51809011, and U21A2010), the Natural Science Foundation of Hunan Province (2021JJ40601), and the Scientific Research Project of the Education Department of Hunan province (20B005).
Conflict of interest
The authors declare that the research was conducted in the absence of any commercial or financial relationships that could be construed as a potential conflict of interest.
Publisher’s note
All claims expressed in this article are solely those of the authors and do not necessarily represent those of their affiliated organizations, or those of the publisher, the editors and the reviewers. Any product that may be evaluated in this article, or claim that may be made by its manufacturer, is not guaranteed or endorsed by the publisher.
Supplementary material
The Supplementary material for this article can be found online at: https://www.frontiersin.org/articles/10.3389/fmicb.2022.1026872/full#supplementary-material
References
Abarenkov, K., Nilsson, R. H., Larsson, K. H., Alexander, I. J., Eberhardt, U., Erland, S., et al. (2010). The UNITE database for molecular identification of fungi - recent updates and future perspectives. New Phytol. 186, 281–285. doi: 10.1111/j.1469-8137.2009.03160.x
Abebe, G., Tsunekawa, A., Haregeweyn, N., Takeshi, T., Wondie, M., Adgo, E., et al. (2020). Effects of land use and topographic position on Soil organic carbon and Total nitrogen stocks in different agro-ecosystems of the upper Blue Nile Basin. Sustainability 12. doi: 10.3390/su12062425
Bai, Y., Wong, C., Jiang, B., Hughes, A. C., Min, W., and Wang, Q. (2018). Developing China's ecological redline policy using ecosystem services assessments for land use planning. Nat. Commun. 9:3034. doi: 10.1038/s41467-018-05306-1
Bardgett, R. D., Wardle, D. A., and Yeates, G. W. (1998). Linking above-ground and below-ground interactions: how plant responses to foliar herbivory influence soil organisms. Soil Biol. Biochem. 30, 1867–1878. doi: 10.1016/S0038-0717(98)00069-8
Bo, H. J., Wen, C. Y., Song, L. J., Yue, Y. T., and Nie, L. S. (2018). Fine-root responses of Populus tomentosa forests to stand density. Forests 9. doi: 10.3390/f9090562
Boothroyd-Roberts, K., Gagnon, D., and Truax, B. (2013). Can hybrid poplar plantations accelerate the restoration of forest understory attributes on abandoned fields? For. Ecol. Manag. 287, 77–89. doi: 10.1016/j.foreco.2012.09.021
Brockett, B. F. T., Prescott, C. E., and Grayston, S. J. (2012). Soil moisture is the major factor influencing microbial community structure and enzyme activities across seven biogeoclimatic zones in western Canada. Soil Biol. Biochem. 44, 9–20. doi: 10.1016/j.soilbio.2011.09.003
Card, S. M., and Quideau, S. A. (2010). Microbial community structure in restored riparian soils of the Canadian prairie pothole region. Soil Biol. Biochem. 42, 1463–1471. doi: 10.1016/j.soilbio.2010.05.010
Chen, H., Zou, J., Cui, J., Ming, N., and Fang, C. (2018). Wetland drying increases the temperature sensitivity of soil respiration. Soil Biol. Biochem. 120, 24–27. doi: 10.1016/j.soilbio.2018.01.035
Chimento, C., and Amaducci, S. (2015). Characterization of fine root system and potential contribution to soil organic carbon of six perennial bioenergy crops. Biomass Bioenergy 83, 116–122. doi: 10.1016/j.biombioe.2015.09.008
Cole, J. R., Wang, Q., Cardenas, E., Fish, J., Chai, B., Farris, R. J., et al. (2009). The Ribosomal Database Project: improved alignments and new tools for rRNA analysis. Nucleic Acids Res. 37, D141–D145. doi: 10.1093/nar/gkn879
Crampe, E. A., Segura, C., and Jones, J. A. (2021). Fifty years of runoff response to conversion of old-growth forest to planted forest in the H. J. Andrews Forest, Oregon, USA. Hydrol. Process. 35:e14168.
Dai, J., Wu, H., Zhang, C., Zeng, G., Liang, J., Guo, S., et al. (2016). Responses of soil microbial biomass and bacterial community structure to closed-off management (an ecological natural restoration measures): a case study of Dongting Lake wetland, middle China. J. Biosci. Bioeng. 122, 345–350. doi: 10.1016/j.jbiosc.2016.03.001
Dai, Y. Y., Zhuang, J., and Chen, X. J. (2020). Synergistic effects of unsaturated flow and soil organic matter on retention and transport of PPCPs in soils. Environ. Res. 191:110135. doi: 10.1016/j.envres.2020.110135
Damianova, A., Tsolova, Z., and Geleva, E. (2016). Tritium and chemical content of water in the area with Balkan endemic nephropathy in Bulgaria. Comptes Rendus De L Academie Bulgare Des Sciences 69, 1129–1136.
Deng, Z., Li, Y., Xie, Y., Peng, C., Chen, X., Li, F., et al. (2018). Hydrologic and edaphic controls on soil carbon emission in Dongting Lake Floodplain, China. J. Geophys. Res. Biogeo. 123, 3088–3097. doi: 10.1029/2018JG004515
Deng, N., Tian, Y. X., Song, Q. A., Tian, F., Ma, F. F., Luo, X. W., et al. (2022). Characteristics of microbial structure of typical plant communities in south Dongting Lake poplar clearing area. J. Ecol. Rural Environ. 38, 915–924.
Ding, X. W., and Li, X. F. (2011). Monitoring of the water-area variations of Lake Dongting in China with ENVISAT ASAR images. Int. J. Appl. Earth Observat. 13, 894–901. doi: 10.1016/j.jag.2011.06.009
Douterelo, I., Goulder, R., and Lillie, M. (2009). Response of the microbial community to water table variation and nutrient addition and its implications for in situ preservation of organic archaeological remains in wetland soils. Int. Biodeterior. Biodegradation 63, 795–805. doi: 10.1016/j.ibiod.2009.06.010
Du, Y., Xue, H.-P., Wu, S.-J., Ling, F., Xiao, F., and Wei, X.-H. (2011). Lake area changes in the middle Yangtze region of China over the 20th century. J. Environ. Manag. 92, 1248–1255. doi: 10.1016/j.jenvman.2010.12.007
Edgar, R. C. (2013). UPARSE: highly accurate OTU sequences from microbial amplicon reads. Nat. Methods 10, 996–998. doi: 10.1038/nmeth.2604
Egoh, B. N., Ntshotsho, P., Maoela, M. A., Blanchard, R., Ayompe, L. M., and Rahlao, S. (2020). Setting the scene for achievable post-2020 convention on biological diversity targets: a review of the impacts of invasive alien species on ecosystem services in Africa. J. Environ. Manag. 261, 110171–110171.110178. doi: 10.1016/j.jenvman.2020.110171
Elhottová, D., Koubová, A., Šimek, M., Cajthaml, T., Jirout, J., Esperschuetz, J., et al. (2012). Changes in soil microbial communities as affected by intensive cattle husbandry. Appl. Soil Ecol. 58, 56–65. doi: 10.1016/j.apsoil.2012.03.009
Ellsworth, J. W., Harrington, R. A., and Fownes, J. H. (2004). Seedling emergence, growth, and allocation of Oriental bittersweet: effects of seed input, seed bank, and forest floor litter. For. Ecol. Manag. 190, 255–264. doi: 10.1016/j.foreco.2003.10.015
Esteso-Martínez, J., and Gil-Pelegrín, E. (2004). Frost resistance of seeds in Mediterranean oaks and the role of litter in the thermal protection of acorns. Ann. For. Sci. 61, 481–486.
Fashing, P. J., Nguyen, N., Luteshi, P., Opondo, W., Cash, J. F., and Cords, M. (2012). Evaluating the suitability of planted forests for African forest monkeys: a case study from Kakamega forest, Kenya. Am. J. Primatol. 74, 77–90. doi: 10.1002/ajp.21012
Ferre, C., Comolli, R., Leip, A., and Seufert, G. (2014). Forest conversion to poplar plantation in a Lombardy floodplain (Italy): effects on soil organic carbon stock. Biogeosciences 11, 6483–6493. doi: 10.5194/bg-11-6483-2014
Gardner, R. C., Barchiesi, S., Beltrame, C., Finlayson, C., Galewski, T., Harrison, I., et al. (2015). State of the World's Wetlands and Their Services to People: A Compilation of Recent Analyses. New York, NY: Social Science Electronic Publishing.
Ghabbour, E. A., Davies, G., Cuozzo, N. P., and Miller, R. O. (2014). Optimized conditions for determination of total soil organic matter in diverse samples by mass loss on ignition. J. Plant Nutr. Soil Sci. 177, 914–919. doi: 10.1002/jpln.201400326
Giejsztowt,, Classen, A. T., and Deslippe, J. R. (2019). Climate change and invasion may synergistically affect native plant reproduction. Ecology 101:e02913.
Gong, J., Xie, Y., Cao, E., Huang, Q., and Li, H. (2019). Integration of InVEST-habitat quality model with landscape pattern indexes to assess mountain plant biodiversity change: a case study of Bailongjiang watershed in Gansu Province. J. Geogr. Sci. 29, 1193–1210. doi: 10.1007/s11442-019-1653-7
Graae, B. J., and Heskjær, V. S. (1997). A comparison of understorey vegetation between untouched and managed deciduous forest in Denmark. For. Ecol. Manag. 96, 111–123. doi: 10.1016/S0378-1127(97)00046-7
Gutknecht, J. L. M., Goodman, R. M., and Balser, T. C. (2006). Linking soil process and microbial ecology in freshwater wetland ecosystems. Plant Soil 289, 17–34.
Hargreaves, P. R., Brookes, P. C., Ross, G. J. S., and Poulton, P. R. (2003). Evaluating soil microbial biomass carbon as an indicator of long-term environmental change. Soil Biol. Biochem. 35, 401–407. doi: 10.1016/S0038-0717(02)00291-2
Harris, J. (2009). Soil microbial communities and restoration ecology: Facilitators or followers? Science 325, 573–574.
Hartman, W. H., Richardson, C. J., Vilgalys, R., and Bruland, G. L. (2008). Environmental and anthropogenic controls over bacterial communities in wetland soils. Proc. Natl. Acad. Sci. U. S. A. 105, 17842–17847. doi: 10.1073/pnas.0808254105
He, H., Liu, Y. X., Hu, Y., Zhang, M. Q., Wang, G. D., and Shen, W. B. (2020). Soil microbial community and its interaction with soil carbon dynamics following a wetland drying process in mu us Sandy Land. Int. J. Environ. Res. Public Health 17. doi: 10.3390/ijerph17124199
Hernández-Santana, V., David, T. S., and Martínez-Fernández, J. (2008). Environmental and plant-based controls of water use in a Mediterranean oak stand. For. Ecol. Manag. 255, 3707–3715. doi: 10.1016/j.foreco.2008.03.004
Hu, W., Li, G., Gao, Z., Jia, G., Wang, Z., and Li, Y. (2020). Assessment of the impact of the Poplar Ecological Retreat Project on water conservation in the Dongting Lake wetland region using the InVEST model. Sci. Total Environ. 733:139423. doi: 10.1016/j.scitotenv.2020.139423
Hu, W., Li, G., and Li, Z. (2021). Spatial and temporal evolution characteristics of the water conservation function and its driving factors in regional lake wetlands-Two types of homogeneous lakes as examples. Ecol. Indic. 130, 108069–101160. doi: 10.1016/j.ecolind.2021.108069
Hu, G. J., Li, J. B., and Zeng, G. M. (2013). Recent development in the treatment of oily sludge from petroleum industry: a review. J. Hazard. Mater. 261, 470–490. doi: 10.1016/j.jhazmat.2013.07.069
Huang, Y., Li, X., Zhang, Z., He, C., Zhao, P., You, Y., et al. (2011). Seasonal changes in Cyclobalanopsis glauca transpiration and canopy stomatal conductance and their dependence on subterranean water and climatic factors in rocky karst terrain. J. Hydrol. 402, 135–143. doi: 10.1016/j.jhydrol.2011.03.013
Inacio, M. L., Rusinque, L. C., Camacho, M. J., and Nobrega, F. (2019). First report of Mesocriconema xenoplax (Nematoda: Criconematidae) from turfgrass in Portugal and in Europe. J. Nematol. 51, 1–6. doi: 10.21307/jofnem-2019-035
Jia, T., Liang, X. X., Guo, T. Y., Wu, T. H., and Chai, B. F. (2022). Bacterial community succession and influencing factors for Imperata cylindrica litter decomposition in a copper tailings area of China. Sci. Total Environ. 815:152908. doi: 10.1016/j.scitotenv.2021.152908
Jing, L., Lyu, C., Zhou, Y., Zuo, A. J., and Lei, G. C. (2016). Spatio-temporal characteristics of the expansion of poplar plantation in West Dongting Lake wetland, China. J. Appl. Ecol. 27, 2039–2047.
Kajiura, M., Tokida, T., and Seki, K. (2012). Effects of moisture conditions on potential soil water repellency in a tropical forest regenerated after fire. Geoderma 181-182, 30–35. doi: 10.1016/j.geoderma.2012.02.028
Kindlmann, P., Honěk, A., and M, Z. (2017). Spreading of alien species and diversity of communities. BioControl 62, 397–407. doi: 10.1007/s10526-017-9787-y
Kong, Y. (2011). Btrim: a fast, lightweight adapter and quality trimming program for next-generation sequencing technologies. Genomics 98, 152–153. doi: 10.1016/j.ygeno.2011.05.009
Lal, R. (2020). Soil organic matter and water retention. Agron. J. 112, 3265–3277. doi: 10.1002/agj2.20282
Leray, M., and Knowlton, N. (2016). Visualizing patterns of marine eukaryotic diversity from metabarcoding data using QIIME. Methods Mol. Biol. 1452, 219–235.
Li, Y., Chen, X. S., Xie, Y. H., Li, X., Li, F., and Hou, Z. Y. (2014b). Effects of young poplar plantations on understory plant diversity in the Dongting Lake wetlands, China. Sci. Rep. 4:8. doi: 10.1038/srep06339
Li, Y., Qin, H., Xie, Y., Wang, W., Chen, X., and Zhang, C. (2014a). Physiological mechanism for the reduction in soil water in poplar (Populus deltoides) plantations in Dongting Lake wetlands. Wetl. Ecol. Manag. 22, 25–33. doi: 10.1007/s11273-013-9319-1
Li, B., Tan, W. Z., Wen, L., Zhao, X. F., Peng, B. F., Yang, J. F., et al. (2020). Anthropogenic habitat alternation significantly decreases alpha- and beta-diversity of benthopelagic metacommunity in a large floodplain lake. Hydrobiologia 847, 293–307. doi: 10.1007/s10750-019-04091-2
Lu, H. W., Hu, W. M., She, J. Y., Zeng, W., and Song, Y. B. (2020). Study on the influence of Ecological Poplar Withdrawal on the landscape pattern of Dongting Lake wetland. J. Nanjing For. Univ. 44, 171–178.
Lyu, Q., Shen, Y., Li, X. W., Chen, G., Li, D. H., and Fan, C. A. (2021). Early effects of crop tree management on undergrowth plant diversity and soil physicochemical properties in a Pinus massoniana plantation. Peerj 9:e11852.
Marshman, N. A., and Marshall, K. C. (1981). Bacterial growth on proteins in the presence of clay minerals. Soil Biol. Biochem. 13, 127–134. doi: 10.1016/0038-0717(81)90008-0
Monti, A., and Zatta, A. (2009). Root distribution and soil moisture retrieval in perennial and annual energy crops in Northern Italy. Agric. Ecosyst. Environ. 132, 252–259. doi: 10.1016/j.agee.2009.04.007
Nocentini, A., and Monti, A. (2017). Land-use change from poplar to switchgrass and giant reed increases soil organic carbon. Agron. Sustain. Dev. 37:23. doi: 10.1007/s13593-017-0435-9
Olsen, Y. S., Dausse, A., Garbutt, A., Ford, H., Thomas, D. N., and Jones, D. L. (2011). Cattle grazing drives nitrogen and carbon cycling in a temperate salt marsh. Soil Biol. Biochem. 43, 531–541. doi: 10.1016/j.soilbio.2010.11.018
Poret-Peterson, A. T., Ji, B., Engelhaupt, E., and Gulledge, J. (2007). Soil microbial biomass along a hydrologic gradient in a subsiding coastal bottomland forest: implications for future subsidence and sea-level rise. Soil Biol. Biochem. 39, 641–645. doi: 10.1016/j.soilbio.2006.09.016
Qu, X. Y., Du, C. Y., Wu, H. P., Xiong, Y., Yu, G. L., Wang, J. S., et al. (2022). Effects of poplar ecological retreat on habitat suitability for migratory birds in China's Dongting Lake Wetland. Front. Environ. Sci. 9:793005. doi: 10.3389/fenvs.2021.793005
Qu, W., Han, G., Wang, J., Li, J., Zhao, M., He, W., et al. (2021). Short-term effects of soil moisture on soil organic carbon decomposition in a coastal wetland of the Yellow River Delta. Hydrobiologia 848, 3259–3271. doi: 10.1007/s10750-020-04422-8
Ren, B., Xie, Y. H., and Li, Y. Z. (2020). Understory floristic diversity in poplar plantations in the Dongting Lake Wetlands, China. Wetlands 40, 1549–1560. doi: 10.1007/s13157-019-01255-7
Schimel, J. P. (2018). Life in dry soils: effects of drought on soil microbial communities and processes. Ann. Rev. Ecol. Evolut. Systemat. 49, 409–432.
Sica, Y. V., Quintana, R. D., Radeloff, V. C., and Gavier-Pizarro, G. I. (2016). Wetland loss due to land use change in the Lower Paraná River Delta, Argentina. Sci. Total Environ. 568, 967–978. doi: 10.1016/j.scitotenv.2016.04.200
Sun, X. F., Gao, L., Ren, H., Ye, Y. Q., Li, A., Stafford-Smith, M., et al. (2018). China's progress towards sustainable land development and ecological civilization. Landsc. Ecol. 33, 1647–1653. doi: 10.1007/s10980-018-0706-0
Sun, Z., Lotz, T., and Chang, N.-B. (2017). Assessing the long-term effects of land use changes on runoff patterns and food production in a large lake watershed with policy implications. J. Environ. Manag. 204, 92–101. doi: 10.1016/j.jenvman.2017.08.043
Tan, Z., Zhang, Y., Song, Q., Liu, W., Deng, X., Tang, J., et al. (2011). Rubber plantations act as water pumps in tropical China. Geophys. Res. Lett. 38:24406.
Tang, Y., Wang, L., Jia, J., Li, Y., Zhang, W., Wang, H., et al. (2011). Response of soil microbial respiration of tidal wetlands in the Yangtze River estuary to different artificial disturbances. Ecol. Eng. 37, 1638–1646. doi: 10.1016/j.ecoleng.2011.06.004
Toledo, F. H., McIntosh, T., Knothe, C., and Aubrey, D. P. (2020). EucalyptusAre unlikely to escape plantations and invade surrounding forests managed with prescribed fire in southeastern US. Forests 11. doi: 10.3390/f11060694
Veblen, K. E., Porensky, L. M., Riginos, C., and Young, T. P. (2016). Are cattle surrogate wildlife? Savanna plant community composition explained by total herbivory more than herbivore type. Ecol. Appl. 26, 1610–1623. doi: 10.1890/15-1367.1
Wang, L., Li, Z., Wang, D., Chen, J., Liu, Y., Nie, X., et al. (2021). Unbalanced social-ecological development within the Dongting Lake basin: inspiration from evaluation of ecological restoration projects. J. Clean. Prod. 315:128161. doi: 10.1016/j.jclepro.2021.128161
Wang, C., Wei, M., Wang, S., Wu, B., and Cheng, H. (2020a). Erigeron annuus (L.) Pers. and Solidago canadensis L. antagonistically affect community stability and community invasibility under the co-invasion condition. Sci. Total Environ. 716:137128. doi: 10.1016/j.scitotenv.2020.137128
Wang, C., Wei, M., Wang, S., Wu, B., and Du, D. (2020b). Cadmium influences the litter decomposition of Solidago canadensis L. and soil N-fixing bacterial communities. Chemosphere 246:125717. doi: 10.1016/j.chemosphere.2019.125717
Wilske, B., Lu, N., Wei, L., Chen, S., Zha, T., Liu, C., et al. (2009). Poplar plantation has the potential to alter the water balance in semiarid Inner Mongolia. J. Environ. Manag. 90, 2762–2770. doi: 10.1016/j.jenvman.2009.03.004
Wright, C. K., and Wimberly, M. C. (2013). Recent land use change in the Western Corn Belt threatens grasslands and wetlands. Proc. Natl. Acad. Sci. U. S. A. 110, 4134–4139. doi: 10.1073/pnas.1215404110
Wu, G., Ren, G., Wang, D., Shi, Z., and Warrington, D. (2013). Above- and below-ground response to soil water change in an alpine wetland ecosystem on the Qinghai-Tibetan Plateau, China. J. Hydrol. 476, 120–127. doi: 10.1016/j.jhydrol.2012.10.031
Wu, H., Zeng, G., Liang, J., Chen, J., Xu, J., Dai, J., et al. (2017). Responses of landscape pattern of China’s two largest freshwater lakes to early dry season after the impoundment of Three-Gorges Dam. Int. J. Appl. Earth Obs. Geoinf. 56, 36–43. doi: 10.1016/j.jag.2016.11.006
Wu, H., Zeng, G., Liang, J., Guo, S., Dai, J., Lu, L., et al. (2015). Effect of early dry season induced by the Three Gorges Dam on the soil microbial biomass and bacterial community structure in the Dongting Lake wetland 53, 129–136.
Xu, M., Zhang, J., Liu, G. B., and Yamanaka, N. (2014). Soil properties in natural grassland, Caragana korshinskii planted shrubland, and Robinia pseudoacacia planted forest in gullies on the hilly Loess Plateau, China. Catena 119, 116–124. doi: 10.1016/j.catena.2014.03.016
Yan, J., and Fu, E. (2021). Improvement of phenol disulfonic acid method for determination of soil nitrate nitrogen. Contempor. Chem. Indust. 62:2.
Yang, G., Wang, M., Chen, H., Liu, L. F., Wu, N., Zhu, D., et al. (2017). Responses of CO2 emission and pore water DOC concentration to soil warming and water table drawdown in Zoige Peatlands. Atmos. Environ. 152, 323–329. doi: 10.1016/j.atmosenv.2016.12.051
Yu, Z., Rabiee, H., and Guo, J. (2021a). Synergistic effect of sulfidated nano zerovalent iron and persulfate on inactivating antibiotic resistant bacteria and antibiotic resistance genes. Water Res. 198:117141. doi: 10.1016/j.watres.2021.117141
Yu, Z. G., Wang, Y., Henderson, I. R., and Guo, J. H. (2022). Artificial sweeteners stimulate horizontal transfer of extracellular antibiotic resistance genes through natural transformation. ISME J. 16, 543–554. doi: 10.1038/s41396-021-01095-6
Yu, Z., Wang, Y., Lu, J., Bond, P. L., and Guo, J. H. (2021b). Nonnutritive sweeteners can promote the dissemination of antibiotic resistance through conjugative gene transfer. ISME J. 15, 2117–2130. doi: 10.1038/s41396-021-00909-x
Zhang, H. J., Sun, L. W., Li, Y., Zhang, W. L., Niu, L. H., and Wang, L. F. (2021). The bacterial community structure and N-cycling gene abundance in response to dam construction in a riparian zone. Environ. Res. 194:110717. doi: 10.1016/j.envres.2021.110717
Keywords: poplar ecological retreat, soil properties, bacterial community structure, Dongting Lake, ecological restoration
Citation: Wu H, Xiao S, Dai J, Xiong Y, Cao J, Qu X, Wang G and Yang R (2022) Effect of poplar ecological retreat project on soil bacterial community structure in Dongting Lake wetland. Front. Microbiol. 13:1026872. doi: 10.3389/fmicb.2022.1026872
Edited by:
Kai Xue, University of Chinese Academy of Sciences, ChinaCopyright © 2022 Wu, Xiao, Dai, Xiong, Cao, Qu, Wang and Yang. This is an open-access article distributed under the terms of the Creative Commons Attribution License (CC BY). The use, distribution or reproduction in other forums is permitted, provided the original author(s) and the copyright owner(s) are credited and that the original publication in this journal is cited, in accordance with accepted academic practice. No use, distribution or reproduction is permitted which does not comply with these terms.
*Correspondence: Haipeng Wu, d3VoYWlwZW5nMDcwMUAxMjYuY29t