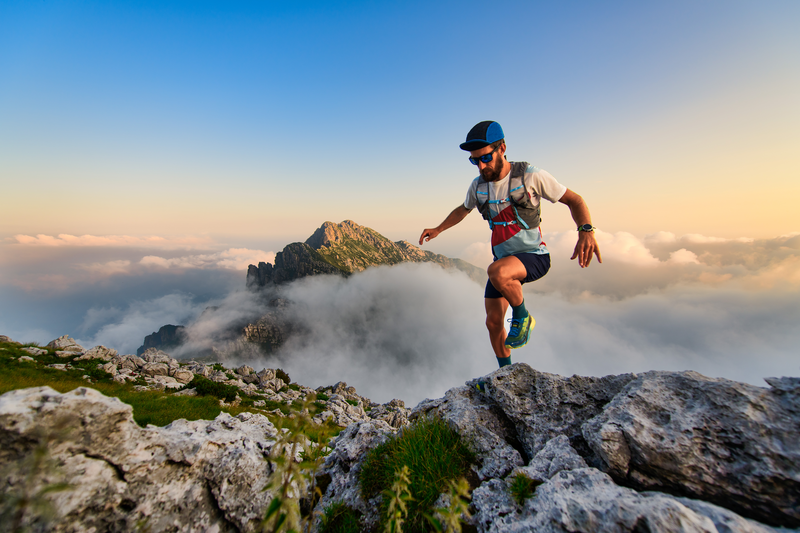
95% of researchers rate our articles as excellent or good
Learn more about the work of our research integrity team to safeguard the quality of each article we publish.
Find out more
PERSPECTIVE article
Front. Microbiol. , 13 October 2022
Sec. Extreme Microbiology
Volume 13 - 2022 | https://doi.org/10.3389/fmicb.2022.1023625
This article is part of the Research Topic Community Series-Extremophiles: Microbial Genomics and Taxogenomics, Volume II View all 16 articles
Water bodies on Mars and the icy moons of the outer solar system are now recognized as likely being associated with high levels of salt. Therefore, the study of high salinity environments and their inhabitants has become increasingly relevant for Astrobiology. Members of the archaeal class Halobacteria are the most successful microbial group living in hypersaline conditions and are recognized as key model organisms for exposure experiments. Despite this, data for the class is uneven across taxa and widely dispersed across the literature, which has made it difficult to properly assess the potential for species of Halobacteria to survive under the polyextreme conditions found beyond Earth. Here we provide an overview of published data on astrobiology-linked exposure experiments performed with members of the Halobacteria, identifying clear knowledge gaps and research opportunities.
Astrobiology is a relatively recent but increasingly relevant cross-disciplinary field of research, which focuses on the origin, distribution, and evolution of Life in the universe (Des Marais et al., 2008; Horneck et al., 2016; Hallsworth et al., 2021b). Fittingly for such a wide scope of study, astrobiology combines techniques and expertise from fields as diverse as biology, chemistry, geology, or planetary sciences (Dartnell and Burchell, 2009; Race et al., 2012; Taşkın and Aydinoglu, 2015). With the increase in frequency and broadening of scope of space exploration, including human space travel, it has become vital to better understand the survival and reproduction of microbial life under multiple extreme conditions, and their impact on space exploration missions and on human travelers who they may encounter or with whom they may cohabit (Cavicchioli, 2002; Moissl-Eichinger, 2011; Moissl-Eichinger et al., 2016; Cottin et al., 2017; Checinska Sielaff et al., 2019; Merino et al., 2019; Nickerson et al., 2022).
One of the main pillars of astrobiology is the investigation of specific terrestrial analog environments and the study of their microbial inhabitants. Such analogs exhibit characteristics found in other locations in the Solar System (Preston and Dartnell, 2014; Martins et al., 2017). Thus far, most terrestrial analog sites used for astrobiology have been selected based on conditions found on Mars (Fairén et al., 2010; Martins et al., 2017), although a few suitable analogs for the exooceans of the icy moons of the outer solar system have also been proposed (Marion et al., 2003; Kereszturi and Keszthelyi, 2013; Antunes et al., 2020).
The study of terrestrial analog sites is seen as essential for: (i) studying the limits of life (e.g., Azua-Bustos et al., 2012; DasSarma et al., 2017; Koschnitzki et al., 2021), (ii) obtaining new microbes for astrobiological exposure experiments (e.g., Musilova et al., 2015; Beblo-Vranesevic et al., 2020), (iii) analyzing long-term viability and preservation of microbes and biomolecules (e.g., Gramain et al., 2011; Leuko et al., 2017; Schreder-Gomes et al., 2022), (iv) technology development and testing for life-detection in space missions (e.g., Harris et al., 2015; García-Descalzo et al., 2019; Lantz et al., 2020; Dypvik et al., 2021; Rull et al., 2022), and (v) defining and refining planetary protection measures (e.g., Rettberg et al., 2019).
When analyzing the potential viability of microbes in other parts of our Solar System, several environmental factors need to be taken into consideration (Horneck et al., 2010; Moissl-Eichinger et al., 2016; Cottin et al., 2017). The limited information on the physical-chemical conditions present in the exooceans of the icy moons partly restrains more in-depth analyses on the survivability of microbes, despite some very interesting studies on potential microbial metabolisms (e.g., Taubner et al., 2018; Goordial, 2021; Sherwood Lollar et al., 2021). This is in stark contrast with the large amount of information already available about Mars and its apparently unwelcoming conditions. These are particularly harsh to mesophiles, combining high levels of radiation (particularly ultra-violet UVC and UVB) (Cockell et al., 2000; Vicente-Retortillo et al., 2020; Zhang et al., 2022), desiccation/low water activity (Knoll and Grotzinger, 2006; Stevenson et al., 2015b; Toner and Catling, 2018; Rivera-Valentín et al., 2020; Hallsworth et al., 2021a), high concentrations of salts and volatile oxidants induced by radiation (Quinn et al., 2013; Lasne et al., 2016; Wu et al., 2018), locations with acidic conditions (Benison and Bowen, 2006; Bibring et al., 2006; Noe Dobrea and Swayze, 2010; Ehlmann et al., 2016), solar particle events (Lillis and Brain, 2013; Ramstad et al., 2018), low temperatures, low pressures (Pätzold et al., 2016; Spiga et al., 2018), and low nutrient availability (Tomkins et al., 2019; Fackrell et al., 2021; Tarnas et al., 2021). Although the extreme setting of Mars has traditionally been seen as biocidal, this is not necessarily the case, as recently highlighted by Hallsworth (2021).
Water is essential for the existence and development of all known life forms, so the search for life in other parts of our solar system and beyond is directly linked with the existence of this vital substance. Luckily for our pursuits, water seems to be more abundant than previously thought (Nimmo and Pappalardo, 2016; Grasset et al., 2017; Greenwood et al., 2018; Schörghofer et al., 2021); there is now evidence that water deposits exist on Mars (Ojha et al., 2014; Martín-Torres et al., 2015; Stillman et al., 2017; Rivera-Valentín et al., 2020; Lauro et al., 2021; Deutsch et al., 2022) as well as on several icy moons (Spencer and Nimmo, 2013; Vance et al., 2014; Hayes, 2016; Jia et al., 2018; Genova et al., 2022). Despite several unknowns, these water bodies outside our planet appear to contain high levels of salt, including perchlorate (Navarro-González et al., 2010; Oren et al., 2014; Martín-Torres et al., 2015; Lauro et al., 2021). Therefore, the study of Earth’s wide range of high salinity environments (from salterns, to polar brine aquifers, deep-sea brines or halite and other salt deposits), as well as their microbial inhabitants, has become increasingly relevant in the context of astrobiology.
Organisms living in high salinity environments are known as halophiles (i.e., salt loving), with representatives spread across the three domains of Life. Microbes living specifically at the higher range of salinities are almost exclusively composed of members of the archaeal class Halobacteria, a phylogenetically coherent group of extreme halophiles (Edbeib et al., 2016; DasSarma and DasSarma, 2017). Among other properties, members of this class are known for their resilience and ability to thrive under multiple environmental extremes (polyextremophilic) including high salinity, high levels of radiation, vacuum, extreme cold, utilization or growth in the presence of caustic materials such as perchlorate, oxygen deprivation, low-nutrient availability, and desiccation, all of which are relevant for astrobiology and particularly important in a Martian context (e.g., Rittmann et al., 2004; McCready et al., 2005; Crowley et al., 2006; Baxter et al., 2007; Coker et al., 2007; Oren et al., 2014; Stan-Lotter and Fendrihan, 2015; Stevenson et al., 2015a; Laye et al., 2017; Sorokin et al., 2017; Thombre R. et al., 2017; Laye and DasSarma, 2018; Leuko et al., 2020; further details provided in Section “The archaeal class Halobacteria and astrobiology: Astrobiological testing,” and Table 1).
Table 1. Overview of Halobacteria tested for astrobiology-relevant features (number of species/total species, genera/total genera in each taxon).
As a result of their unique combination of adaptations and capabilities, these polyextremophilic microbes have long been hailed as models for astrobiology and flagged as priority groups for testing (DasSarma, 2006; Reid et al., 2006; Baxter et al., 2007; DasSarma et al., 2020). Accordingly, several authors have followed this research thread and data has been accumulating. However, a systematic approach for thoroughly testing Halobacteria or a good overview of its current status seems to be missing. We aimed to help to address this growing issue by collecting all available data from primary literature on exposure-based testing with strains of this group, mapping the results as reported in their original publications, and discussing the snapshot that we have recorded.
Currently, the class Halobacteria is grouped into six families and three orders (Gupta et al., 2016). The order Halobacteriales presently has the highest number of species within the group and is split into three families: Haloarculaceae, Halobacteriaceae, and Halococcaceae. Halobacteriaceae is the largest family within the Halobacteria, contrasting with the Halococcaceae which is the smallest one and comprises a single genus (and only 10 species). To date, the order containing the second-highest number of species is Haloferacales, spread across two families: Haloferacaceae, and Halorubraceae, followed by the order Natrialbales, which includes the single family Natrialbaceae.
As outlined in Table 1 and in Supplementary Figure 1, only a relatively small number of species within the class Halobacteria has been tested for their capacity to grow or survive under astrobiological- or Martian-relevant conditions, with a slightly better performance when looking at genus-based coverage. This is reflected both when looking at numbers for each class and when breaking down into intermediate taxa level such as order or family. The tested species are usually below 10% of the total number within each taxon, and between 10 and 20% for tested genera. The most noteworthy exception to these ranges can be found within the Halococcaceae, as it is composed of a single genus and has a small number of total species. These low numbers occur despite the widely recognized importance of the Halobacteria for astrobiology (e.g., Kunte et al., 2002; DasSarma, 2006; Reid et al., 2006; Baxter et al., 2007; Leuko et al., 2014; Oren, 2014a; Rettberg et al., 2019; DasSarma et al., 2020).
Another aspect worth highlighting is that most astrobiology-based studies have focused on a very narrow range of genera and species within the class Halobacteria, mostly associated with members of the order Halobacteriales, and particularly focused on the genus Halobacterium (Table 1). Indeed, the Halobacteriales represent more than half of the tested species within the class, followed by the Haloferacales (nine tested species), and by the almost unexplored Natrialbales (six species). Furthermore, within several families of the class we find a relatively common trend: tests are usually focused on a single genus (Table 1). This can be seen in the order Halobacteriales for family Haloarculaceae (genus Haloarcula) and family Halococcaceae (genus Halococcus), but also in the order Haloferacales for the family Haloferacaceae (genus Haloferax).
A better representation of diversity is found within the family Halobacteriaceae with four different genera (Halobacterium, Halovarius, Halovenus, and Natronomonas), despite the clear emphasis on Halobacterium.
Regarding the strains selected for astrobiology-related testing, most of them are type strains for their respective species (Table 1). The use of such type strains is usually recommended, as this contributes to more readily available data on the strains, and their accessibility facilitates experimental reproducibility and comparative studies (Antunes et al., 2017). One very clear and notable exception to this predominance of type strains is the use of Halobacterium sp. NRC-1. Although not a type strain, it has been deposited in microbial biological resource centers and is readily available; it has also been very extensively studied and has long been considered a model system for studies in Archaea, namely due to ease of culturing and genetic manipulation (Ng et al., 2000; McCready et al., 2005; Boubriak et al., 2008; Anderson et al., 2016; Dassarma et al., 2016). The status of this strain explains the very extensive number of astrobiological-based tests performed with it (Table 1).
Another insight of our analysis is the realization of how restricted and limited datasets on testing currently are. Indeed, most exposure experiments performed with the class Halobacteria focus exclusively on the effects of a single environmental factor (with a noticeable predominance of radiation-exposure tests; Table 1 and Supplementary Figure 2). While the testing of one variable at a time is consistent with classical experimental techniques, the low number of tests with multiple variables is limiting. This gap in our understanding of the effect of simultaneous exposure to multiple extreme conditions is particularly apparent given that astrobiology-relevant settings beyond our planet include a combination of multiple environmental extremes (e.g., Merino et al., 2019; Simões and Antunes, 2021).
Space-exposure testing provides the ideal platform for obtaining a better perspective on this, but the number of tested Halobacteria species remains low as most experiments have made use of simulated conditions only. However, it should be noted that these issues are not exclusive to Halobacteria but are rather major general limitations that apply to most microbial taxa. Several upcoming projects and recent publications have flagged members of the Halobacteria as key target organisms (Sgambati et al., 2020; Beblo-Vranesevic et al., 2022), so we can expect relevant new results in the near future.
Despite the generalized sparsity of data across the board, we have some good examples worth highlighting regarding completeness of the datasets. It should be relatively easy to complete a few datasets and cover some of the most obvious gaps. For example, testing the effects of exposure to UV currently has been performed for at least one representative of each family of the class Halobacteria except for the Halorubraceae (Table 1 and Supplementary Figure 2). Likewise, the family Haloarculaceae is currently the only one that has not been subjected to exposure to multiple combined extremes (Table 1 and Supplementary Figure 2).
Looking at the individual species/strain level (Table 1), we also have some good examples that are close to complete. Halobacterium salinarum NRC-1 has the most complete datasets, with very few gaps (12 out of 18 listed astrobiology tests). As previously mentioned, the latter is widely recognized as a model organism within the Halobacteria and an extensive amount of testing has been done with this strain. Nonetheless, regarding simulated single-factor astrobiology tests, to date there are no reported results for exposure to microgravity and several Martian chemistry and environment factors (M1 and M3–M5; Table 1 and Supplementary Figure 2). Furthermore, this strain has been included in stratospheric exposure experiments, but has not been subjected to orbital testing. Given its status as model organism (McCready et al., 2005; Boubriak et al., 2008; Dassarma et al., 2016), this strain should be flagged as a priority for future tests.
The second good example we would like to highlight regarding completeness is Halococcus morrhuae. Although currently having less complete datasets than Halobaculum salinarum NRC-1 (10 out of 18 listed astrobiology tests; Table 1), it could be flagged as a further target for future completion. This species has the advantage of having already been used in orbital exposure tests, which are arguably the most challenging from a logistic and access point of view.
Natriabales is the only order of Halobacteria that does not have a representative already subjected to orbital testing. This order has the most incomplete datasets, as it is also absent from testing for most radiation types (with the notable exception of UV), most Martian chemistry and environmental factors (apart from testing with Martian soil analogs), desiccation, microgravity, and stratospheric testing (Table 1 and Supplementary Figure 2).
Other important factors, not included on the overview table are exposure to low temperatures and low pH. Both conditions are seen as relevant from a Martian perspective but have not been directly listed as both are part of the standard characterization of new species. The number of species of Halobacteria capable of growth below 15°C or below pH 5 is notably small (currently consisting of 3 and 9 species, respectively). Nonetheless, within those, only Halorubrum lacusprofundi has been targeted for astrobiology testing.
On Earth, sites which are simultaneously acidic and highly saline are relatively rare (Mormile et al., 2009; Conner and Benison, 2013; Escudero et al., 2018), and studies on cold hypersaline sites remain largely underexplored (Oren, 2014b; Lamarche-Gagnon et al., 2015; Sapers et al., 2017). This might explain the reduced number of species within the Halobacteria that could be highlighted here. Further efforts in exploring these sites should be prioritized and could prove quite beneficial.
Looking at coverage based on the type of stressor also uncovered some unexpected areas where further work needs to be done (Supplementary Figure 2). Selected highlights of these gaps include exposure to single factors such as microgravity (missing data for Halobacteriaceae, Halorubraceae, and Natrialbaceae), simulated Martian atmosphere (with no representation for Natrialbales, Haloferacales, or Haloarculaceae) or Martian soil analogs/regolith (only tested for the Natrialbaceae). On the more complete datasets, tests looking at exposure to desiccation or to perchlorate are still needed to ensure that all families are represented.
As outlined above, we can see that there are currently clear and significant gaps in the study of the class Halobacteria under the scope of Astrobiology. More in-depth testing is needed to cover the taxonomic diversity present in this archaeal group both at higher- and lower-ranking taxonomy levels (particularly in families with single-genus testing or with few tested species). The fact that species of this class have large pangenomes, such that even closely related taxa may be functionally quite distinct (Gaba et al., 2020; de la Haba et al., 2021), further emphasizes the need for more extensive testing.
Likewise, there is a need for more completed datasets on astrobiology-relevant stressors on members of this class, both as single factors and combined, particularly making use of space-exposure experiments. A notable (and surprising) gap here is on the study of the effects of different salts. Although their relationship with salt is at the core of the definition of halophiles, studies have been almost exclusively restricted to sodium chloride (NaCl). The astrobiological relevance of studying the interaction with different salts (including perchlorates), as well as looking into chaotropic and kosmotropic effects, is clear and has been well noted (Hallsworth et al., 2007; Ball and Hallsworth, 2015; Stevenson et al., 2015b; dC Rubin et al., 2017). Yet, most species of Halobacteria still remain untested. Another noticeably neglected field here is the study of exposure to high pressure. Although technically more challenging, these settings would be very relevant in the context of the Martian subsurface or of the exooceans of the icy moons (Ueta and Sasaki, 2013; Jebbar et al., 2020; Olson et al., 2020).
From a physiological diversity perspective, new opportunities might also be opening up. Although members of the Halobacteria were typically seen as aerobic heterotrophs (Andrei et al., 2012) and reliant on the “salt-in” strategy (e.g., Kunte et al., 2002), they have consistently proven to be a lot more diverse (Müller et al., 2005; Oren, 2008; Gunde-Cimerman et al., 2018). An increasing number of species has been reported to use alternative anaerobic metabolic pathways, or even to show preference for anaerobic conditions or phototrophy (e.g., Antunes et al., 2008; Sorokin et al., 2017, 2018; Weber et al., 2017; Miralles-Robledillo et al., 2021).
There might be possible implications of this versatility in overall resilience and adaptability to astrobiology-relevant conditions. Microorganisms with “less typical” features could thus be interesting candidates for targeted future exposure studies and comparisons. An over-reliance on quickly growing or easier strains in detriment of those with higher relevance for astrobiology, such as anaerobic ones (Beblo-Vranesevic et al., 2020) or psychrotolerant/psychrophilic (Kish et al., 2012), should also be avoided despite the additional challenges and technical issues they might bring (Hamm et al., 2019; Cui and Dyall-Smith, 2021; Wang et al., 2021).
From an ecological perspective, it is relevant to note that the vast majority of tested species were not isolated from environments traditionally, or officially, flagged as terrestrial analog sites, despite some notable exceptions (e.g., Hrr. lacusprofundi). It is true that the identification of terrestrial analog sites can be seen as somewhat subjective and, in some ways, all hypersaline sites could potentially be seen as Martian analog sites (e.g., Mancinelli et al., 2004; Parro et al., 2011; Murray et al., 2012; Xiao et al., 2017). Nonetheless, it would be a useful priority to place further stress on: (i) testing members of the class Halobacteria from well-established analog sites and (ii) further identification and flagging of additional hypersaline sites as suitable terrestrial analogs. Likewise, the increase in cultivation-based studies specifically aimed toward isolating new strains and describing new species from such sites, namely polar, deep-sea, or magnesium-sulfate brines (Franzmann et al., 1988; Antunes et al., 2008; Albuquerque et al., 2012; Mou et al., 2012), would also prove beneficial and provide excellent new targets for exposure tests (DasSarma et al., 2017; Laye and DasSarma, 2018).
Furthermore, while single-species experiments are valuable, we should note that microbes do not generally live in isolation (e.g., Van Der Wielen and Heijs, 2007; Vikram et al., 2016; Abed et al., 2018; Uritskiy et al., 2021). Therefore, parallel experiments involving constructed consortia (as well as characterized natural consortia) of halophiles, could provide a useful complementary approach (e.g., Wadsworth et al., 2019; Leuko et al., 2020). Other approaches could (and should) focus on the distinction between survival and growth/multiplication in exposure experiments or the issue of long-term viability of microbes trapped in salt, a very relevant topic for halophilic research (Gramain et al., 2011; Jaakkola et al., 2014; Stan-Lotter and Fendrihan, 2015), but still under-explored from an astrobiological perspective.
Access to launching opportunities has been rather restricted in the past and has severely limited capability to perform experiments in space, but this sector is rapidly changing. The on-going rise of the space private sector, the increased use of micro-satellites, or even the recent launch of the Chinese Space Station, all provide further launch and testing opportunities that should be harnessed to address at least some of the gaps that we have identified. Perhaps there is also room for technical improvements on reducing some historical resistance toward high-salt experiments in space (due to increased risks of corrosion and clogging), allowing for, e.g., the testing of more live strains in liquid medium rather than just in lyophilized, freeze-dried form (Barravecchia et al., 2018; Rcheulishvili et al., 2020).
Finally, we highlight the increasing relevance of data and its analysis in the context of Halobacteria and astrobiology. Obviously, the selection and identification of model organisms for astrobiology should make use of a variety of data on their capabilities and characteristics. The increased relevance of the class Halobacteria, together with its significant on-going increase in the description of new species and genera, as well as in genomic and phenotypic data (e.g., DasSarma et al., 2010, 2019; Oren, 2014b; Antunes et al., 2017; Cui and Dyall-Smith, 2021; Lach et al., 2021), make it notoriously difficult to navigate this ocean of relevant yet dispersed information. Despite some efforts targeting the collection of data from this specific taxon (e.g., Pfeiffer et al., 2008; DasSarma et al., 2010; Ukani et al., 2011; Sharma et al., 2014; Loukas et al., 2018; Reimer et al., 2019), the absence of dedicated tools to quickly compare species or even to quickly identify strains with a combination of selected properties can be seen as a significant bottleneck in a range of fields of applied research with this group of archaea. Further community-wide efforts and the development of such tools should be thus seen as a key priority for researchers working with this taxon in the context of astrobiology and beyond.
The original contributions presented in this study are included in the article/Supplementary material, further inquiries can be directed to the corresponding author.
AA conceived this study. J-HW conducted the data collection and processing. J-HW and AA conducted the core analysis and interpretation of the data and wrote the manuscript. All authors contributed to the analysis and interpretation of the data, revised the manuscript, and have read and approved the final manuscript.
This work of J-HW, MS, and AA is funded by the Science and Technology Development Fund, Macao SAR, China. AA and MS are supported by Faculty Research Grants of Macau University of Science and Technology’s (FRG, AA: grant No. FRG-22-079-LPS; MS: grant No. FRG-22-RG-22-080-LPS).
The authors declare that the research was conducted in the absence of any commercial or financial relationships that could be construed as a potential conflict of interest.
All claims expressed in this article are solely those of the authors and do not necessarily represent those of their affiliated organizations, or those of the publisher, the editors and the reviewers. Any product that may be evaluated in this article, or claim that may be made by its manufacturer, is not guaranteed or endorsed by the publisher.
The Supplementary Material for this article can be found online at: https://www.frontiersin.org/articles/10.3389/fmicb.2022.1023625/full#supplementary-material
Supplementary Figure 1 | Proportion of species and genera subjected to testing of astrobiology-relevant features within the class Halobacteria and each of its orders and families (see Table 1 for full information).
Supplementary Figure 2 | Overview of total number of reports for different types of tests of astrobiological relevance performed for each family of the Halobacteria (see Table 1 for full information). R1, HZE (highly-charged and energetic particles); R2, γ-rays; R3, x-rays; R4, electron-beam irradiation; UV, ultra-violet radiation; vac, vacuum; temp, low temperature; M1, effect of ClO4– coupled with CO oxidation; M2, effect of ClO4–; M3, use of CO as carbon source; M4, simulated Martian atmosphere (95% CO2, 2.7% N2, 1.6% Ar, 0.15% O2, and 370 ppm H2O, 103 Pa); M5, Martian soil analogue; des, desiccation; G1, microgravity; G2, hypergravity; mult, multiple conditions; str, stratosphere testing; orb, orbital testing.
Abed, R. M. M., Kohls, K., Leloup, J., and de Beer, D. (2018). Abundance and diversity of aerobic heterotrophic microorganisms and their interaction with cyanobacteria in the oxic layer of an intertidal hypersaline cyanobacterial mat. FEMS Microbiol. Ecol. 94:fix183. doi: 10.1093/femsec/fix183
Abrevaya, X. C., Adamo, H. P., Cortó, N. E., and Mauas, P. J. D. (2008). The UV limits of life on extrasolar planets: An experiment with halophile Archaea bacteria at different UV doses. Bol. de la Asociacion Argent. de Astronomia La Plata Argent. 51, 3–6.
Abrevaya, X. C., Cortón, E., and Mauas, P. J. D. (2010). UV habitability and dM stars: An approach for evaluation of biological survival. Proc. Int. Astron. Union 5, 443–445. doi: 10.1017/S1743921309993073
Abrevaya, X. C., Cortón, E., and Mauas, P. J. D. (2011a). Flares and habitability. Proc. Int. Astron. Union 7, 405–409. doi: 10.1017/S1743921312005145
Abrevaya, X. C., Paulino-Lima, I. G., Galante, D., Rodrigues, F., Mauas, P. J., Cortón, E., et al. (2011b). Comparative survival analysis of Deinococcus radiodurans and the Haloarchaea Natrialba magadii and Haloferax volcanii exposed to vacuum ultraviolet irradiation. Astrobiology 11, 1034–1040. doi: 10.1089/ast.2011.0607
Albuquerque, L., Taborda, M., La Cono, V., Yakimov, M., and da Costa, M. S. (2012). Natrinema salaciae sp. nov., a halophilic Archaeon isolated from the deep, hypersaline anoxic Lake Medee in the Eastern Mediterranean Sea. Syst. Appl. Microbiol. 35, 368–373. doi: 10.1016/j.syapm.2012.06.005
Anderson, I. J., DasSarma, P., Lucas, S., Copeland, A., Lapidus, A., Del Rio, T. G., et al. (2016). Complete genome sequence of the Antarctic Halorubrum lacusprofundi type strain ACAM 34. Stand. Genom. Sci. 11:70. doi: 10.1186/s40793-016-0194-2
Andrei, A. -Ş, Banciu, H. L., and Oren, A. (2012). Living with salt: Metabolic and phylogenetic diversity of Archaea inhabiting saline ecosystems. FEMS Microbiol. Lett. 330, 1–9. doi: 10.1111/j.1574-6968.2012.02526.x
Antunes, A., Olsson-Francis, K., and McGenity, T. J. (2020). Exploring deep-sea brines as potential terrestrial analogues of oceans in the icy moons of the outer solar system. Curr. Issues Mol. Biol. 38, 123–162. doi: 10.21775/cimb.038.123
Antunes, A., Simões, M. F., Grötzinger, S. W., Eppinger, J., Bragança, J., and Bajic, V. B. (2017). “Bioprospecting Archaea: Focus on Extreme Halophiles,” in Bioprospecting: Success, Potential and Constraints, eds R. Paterson and N. Lima (Berlin: Springer International Publishing), 81–112.
Antunes, A., Taborda, M., Huber, R., Moissl, C., Nobre, M. F., and da Costa, M. S. (2008). Halorhabdus tiamatea sp. nov., a non-pigmented, extremely halophilic Archaeon from a deep-sea, hypersaline anoxic basin of the Red Sea, and emended description of the genus Halorhabdus. Int. J. Syst. Evol. Microbiol. 58, 215–220. doi: 10.1099/ijs.0.65316-0
Azua-Bustos, A., Urrejola, C., and Vicuña, R. (2012). Life at the dry edge: Microorganisms of the Atacama Desert. FEBS Lett. 586, 2939–2945. doi: 10.1016/j.febslet.2012.07.025
Baliga, N. S., Bjork, S. J., Bonneau, R., Pan, M., Iloanusi, C., Kottemann, M. C., et al. (2004). Systems level insights into the stress response to UV radiation in the halophilic Archaeon Halobacterium NRC-1. Genome Res. 14, 1025–1035. doi: 10.1101/gr.1993504
Ball, P., and Hallsworth, J. E. (2015). Water structure and chaotropicity: Their uses, abuses and biological implications. Phys. Chem. Chem. Phys. 17, 8297–8305. doi: 10.1039/C4CP04564E
Barravecchia, I., Cesari, C. D., Pyankova, O. V., Scebba, F., Mascherpa, M. C., Vecchione, A., et al. (2018). Pitting corrosion within bioreactors for space cell-culture contaminated by Paenibacillus glucanolyticus, a case report. Microgravity Sci. Technol. 30, 309–319. doi: 10.1007/s12217-018-9601-1
Baxter, B. K., Eddington, B., Riddle, M. R., Webster, T. N., and Avery, B. (2007). Great Salt Lake halophilic microorganisms as models for astrobiology: Evidence for desiccation tolerance and ultraviolet irradiation resistance. Instrum. Methods Missions Astrobiol. 6694:669415. doi: 10.1117/12.732621
Beblo-Vranesevic, K., Bohmeier, M., Schleumer, S., Rabbow, E., Perras, A. K., Moissl-Eichinger, C., et al. (2020). Impact of simulated martian conditions on (facultatively) anaerobic bacterial strains from different mars analogue sites. Curr. Issues Mol. Biol. 38, 103–122. doi: 10.21775/cimb.038.103
Beblo-Vranesevic, K., Piepjohn, J., Antunes, A., and Rettberg, P. (2022). Surviving Mars: New insights into the persistence of facultative anaerobic microbes from analogue sites. Int. J. Astrobiol. 21, 110–127. doi: 10.1017/S1473550422000064
Benison, K. C., and Bowen, B. B. (2006). Acid saline lake systems give clues about past environments and the search for life on Mars. Icarus 183, 225–229. doi: 10.1016/j.icarus.2006.02.018
Bibring, J.-P., Langevin, Y., Mustard, J. F., Poulet, F., Arvidson, R., Gendrin, A., et al. (2006). Global mineralogical and aqueous mars history derived from OMEGA/mars express data. Science 312, 400–404. doi: 10.1126/science.1122659
Boubriak, I., Ng, W. L., DasSarma, P., DasSarma, S., Crowley, D. J., and McCready, S. J. (2008). Transcriptional responses to biologically relevant doses of UV-B radiation in the model Archaeon, Halobacterium sp. NRC-1. Saline Syst. 4:13. doi: 10.1186/1746-1448-4-13
Bryanskaya, A. V., Berezhnoy, A. A., Rozanov, A. S., Peltek, S. E., and Pavlov, A. K. (2013). Adaptive capabilities of microorganisms of salt lakes of the Altai Region under conditions of early Mars. Paleontol. J. 47, 1089–1092. doi: 10.1134/S0031030113090050
Bryanskaya, A. V., Berezhnoy, A. A., Rozanov, A. S., Serdyukov, D. S., Malup, T. K., and Peltek, S. E. (2020). Survival of halophiles of Altai lakes under extreme environmental conditions: Implications for the search for Martian life. Int. J. Astrobiol. 19, 1–15. doi: 10.1017/S1473550419000077
Cavicchioli, R. (2002). Extremophiles and the Search for Extraterrestrial Life. Astrobiology 2, 281–292. doi: 10.1089/153110702762027862
Checinska Sielaff, A., Urbaniak, C., Mohan, G. B. M., Stepanov, V. G., Tran, Q., Wood, J. M., et al. (2019). Characterization of the total and viable bacterial and fungal communities associated with the International Space Station surfaces. Microbiome 7:50. doi: 10.1186/s40168-019-0666-x
Cockell, C. S., Catling, D. C., Davis, W. L., Snook, K., Kepner, R. L., Lee, P., et al. (2000). The ultraviolet environment of mars: Biological implications past, present, and future. Icarus 146, 343–359. doi: 10.1006/icar.2000.6393
Coker, J. A., DasSarma, P., Kumar, J., Müller, J. A., and DasSarma, S. (2007). Transcriptional profiling of the model Archaeon Halobacterium sp. NRC-1: Responses to changes in salinity and temperature. Saline Syst. 3:6. doi: 10.1186/1746-1448-3-6
Confalonieri, F., and Sommer, S. (2011). Bacterial and Archaeal resistance to ionizing radiation. J. Phys. 261:012005. doi: 10.1088/1742-6596/261/1/012005
Conner, A. J., and Benison, K. C. (2013). Acidophilic halophilic microorganisms in fluid inclusions in halite from lake magic, Western Australia. Astrobiology 13, 850–860. doi: 10.1089/ast.2012.0956
Cottin, H., Kotler, J. M., Billi, D., Cockell, C., Demets, R., Ehrenfreund, P., et al. (2017). Space as a tool for astrobiology: Review and recommendations for experimentations in earth orbit and beyond. Space Sci. Rev. 209, 83–181. doi: 10.1007/s11214-017-0365-5
Crowley, D. J., Boubriak, I., Berquist, B. R., Clark, M., Richard, E., Sullivan, L., et al. (2006). The uvrA, uvrB and uvrC genes are required for repair of ultraviolet light induced DNA photoproducts in Halobacterium sp. NRC-1. Saline Syst. 2:11. doi: 10.1186/1746-1448-2-11
Cui, H.-L., and Dyall-Smith, M. L. (2021). Cultivation of halophilic Archaea (class Halobacteria) from thalassohaline and athalassohaline environments. Mar. Life Sci. Technol. 3, 243–251. doi: 10.1007/s42995-020-00087-3
Dartnell, L. R., and Burchell, M. J. (2009). Survey on astrobiology research and teaching activities within the United Kingdom. Astrobiology 9, 717–730. doi: 10.1089/ast.2009.0348
DasSarma, P., Capes, M. D., and DasSarma, S. (2019). “Comparative Genomics of Halobacterium Strains From Diverse Locations,” in Microbial Diversity in the Genomic Era, eds S. Das and H. R. Dash (Cambridge: Academic Press), 285–322.
DasSarma, P., Laye, V., Harvey, J., Reid, C., Shultz, J., Yarborough, A., et al. (2017). Survival of halophilic Archaea in Earth’s cold stratosphere. Int. J. Astrobiol. 16, 321–327.
Dassarma, P., Tuel, K., Nierenberg, S. D., Phillips, T., Pecher, W. T., and Dassarma, S. (2016). Inquiry-driven teaching & learning using the Archaeal microorganism Halobacterium NRC-1. Am. Biol. Teach. 78, 7–13. doi: 10.1525/abt.2016.78.1.7
DasSarma, S. (2006). Extreme halophiles are models for astrobiology. Microbe Mag. 1, 120–126. doi: 10.1128/microbe.1.120.1
DasSarma, S., and DasSarma, P. (2017). “Halophiles,” in eLS, ed. John Wiley & Sons, Ltd (Hoboken, NJ: John Wiley & Sons, Ltd), 1–13.
DasSarma, S., DasSarma, P., Laye, V. J., and Schwieterman, E. W. (2020). Extremophilic models for astrobiology: Haloarchaeal survival strategies and pigments for remote sensing. Extremophiles 24, 31–41. doi: 10.1007/s00792-019-01126-3
DasSarma, S. L., Capes, M. D., DasSarma, P., and DasSarma, S. (2010). HaloWeb: The Haloarchaeal genomes database. Saline Syst. 6:12. doi: 10.1186/1746-1448-6-12
dC Rubin, S. S., Marín, I., Gómez, M. J., Morales, E. A., Zekker, I., San Martín-Uriz, P., et al. (2017). Prokaryotic diversity and community composition in the Salar de Uyuni, a large scale, chaotropic salt flat. Environ. Microbiol. 19, 3745–3754. doi: 10.1111/1462-2920.13876
de la Haba, R. R., Minegishi, H., Kamekura, M., Shimane, Y., and Ventosa, A. (2021). Phylogenomics of Haloarchaea: The controversy of the genera Natrinema-Haloterrigena. Front. Microbiol. 12:740909. doi: 10.3389/fmicb.2021.740909
Des Marais, D. J., Nuth, J. A., Allamandola, L. J., Boss, A. P., Farmer, J. D., Hoehler, T. M., et al. (2008). The NASA astrobiology roadmap. Astrobiology 8, 715–730. doi: 10.1089/ast.2008.0819
Deutsch, A. N., Levy, J. S., Dickson, J. L., and Head, J. W. (2022). Daily and seasonal processes shape hydrological activity and detectability of moisture in Antarctic, Mars-analog soils. Icarus 380:114990. doi: 10.1016/j.icarus.2022.114990
DeVeaux, L. C., Müller, J. A., Smith, J., Petrisko, J., Wells, D. P., and DasSarma, S. (2007). Extremely radiation-resistant mutants of a halophilic Archaeon with increased single-stranded DNA-binding protein (RPA) gene expression. Radiat. Res. 168, 507–514. doi: 10.1667/rr0935.1
Dornmayr-Pfaffenhuemer, M., Legat, A., Schwimbersky, K., Fendrihan, S., and Stan-Lotter, H. (2011). Responses of Haloarchaea to simulated microgravity. Astrobiology 11, 199–205. doi: 10.1089/ast.2010.0536
Dypvik, H., Hellevang, H., Krzesiñska, A., Sætre, C., Viennet, J.-C., Bultel, B., et al. (2021). The planetary terrestrial analogues library (PTAL) – An exclusive lithological selection of possible martian earth analogues. Planet. Space Sci. 208:105339. doi: 10.1016/j.pss.2021.105339
Edbeib, M. F., Wahab, R. A., and Huyop, F. (2016). Halophiles: Biology, adaptation, and their role in decontamination of hypersaline environments. World J. Microbiol. Biotechnol. 32:135. doi: 10.1007/s11274-016-2081-9
Ehlmann, B. L., Swayze, G. A., Milliken, R. E., Mustard, J. F., Clark, R. N., Murchie, S. L., et al. (2016). Discovery of alunite in cross crater, terra sirenum, mars: Evidence for acidic, sulfurous waters. Am. Mineral. 101, 1527–1542. doi: 10.2138/am-2016-5574
Escudero, L., Oetiker, N., Gallardo, K., Tebes-Cayo, C., Guajardo, M., Nuñez, C., et al. (2018). A thiotrophic microbial community in an acidic brine lake in Northern Chile. Antonie Leeuwenhoek 111, 1403–1419. doi: 10.1007/s10482-018-1087-8
Evans, J. J., Gygli, P. E., McCaskill, J., and DeVeaux, L. C. (2018). Divergent roles of RPA homologs of the model Archaeon Halobacterium salinarum in survival of DNA damage. Genes 9:223. doi: 10.3390/genes9040223
Fackrell, L. E., Schroeder, P. A., Thompson, A., Stockstill-Cahill, K., and Hibbitts, C. A. (2021). Development of martian regolith and bedrock simulants: Potential and limitations of martian regolith as an in-situ resource. Icarus 354:114055. doi: 10.1016/j.icarus.2020.114055
Fairén, A. G., Davila, A. F., Lim, D., Bramall, N., Bonaccorsi, R., Zavaleta, J., et al. (2010). Astrobiology through the ages of Mars: The study of terrestrial analogues to understand the habitability of Mars. Astrobiology 10, 821–843. doi: 10.1089/ast.2009.0440
Fendrihan, S., Bérces, A., Lammer, H., Musso, M., Rontó, G., Polacsek, T. K., et al. (2009). Investigating the effects of simulated martian ultraviolet radiation on Halococcus dombrowskii and other extremely halophilic Archaebacteria. Astrobiology 9, 104–112. doi: 10.1089/ast.2007.0234
Fendrihan, S., Grosbacher, M., and Stan-Lotter, H. (2010). Response of the Extremely Halophilic Halococcus Dombrowskii Strain H4 to UV Radiation and Space Conditions in the EXPOSE -ADAPT Project on the International Space Station. Munich: EGU General Assembly.
Feshangsaz, N., Semsarha, F., Tackallou, S. H., Nazmi, K., Monaghan, E. P., Riedo, A., et al. (2020). Survival of the halophilic Archaeon Halovarius luteus after desiccation, simulated martian UV radiation and vacuum in comparison to Bacillus atrophaeus. Orig. Life Evol. Biosph. 50, 157–173. doi: 10.1007/s11084-020-09597-7
Flores, N., Hoyos, S., Venegas, M., Galetoviæ, A., Zúñiga, L. M., Fábrega, F., et al. (2020). Haloterrigena sp. strain SGH1, a Bacterioruberin-rich, perchlorate-tolerant Halophilic Archaeon Isolated From halite microbial communities, atacama desert, Chile. Front. Microbiol. 11:342. doi: 10.3389/fmicb.2020.00324
Franzmann, P. D., Stackebrandt, E., Sanderson, K., Volkman, J. K., Cameron, D. E., Stevenson, P. L., et al. (1988). Halobacterium lacusprofundi sp. nov., a Halophilic bacterium isolated from deep lake, Antarctica. Syst. Appl. Microbiol. 11, 20–27. doi: 10.1016/S0723-2020(88)80044-4
Gaba, S., Kumari, A., Medema, M., and Kaushik, R. (2020). Pan-genome analysis and ancestral state reconstruction of class Halobacteria: Probability of a new super-order. Sci. Rep. 10:21205. doi: 10.1038/s41598-020-77723-6
García-Descalzo, L., Parro, V., García-Villadangos, M., Cockell, C. S., Moissl-Eichinger, C., Perras, A., et al. (2019). Microbial markers profile in anaerobic Mars analogue environments using the LDChip (Life Detector Chip) antibody microarray core of the SOLID (Signs of Life Detector) platform. Microorganisms 7:365. doi: 10.3390/microorganisms7090365
Genova, A., Smith, D. E., Canup, R., Hurford, T., Goossens, S., Mazarico, E., et al. (2022). Geodetic investigations of the mission concept MAGIC to reveal Callisto’s internal structure. Acta Astronaut. 195, 68–76. doi: 10.1016/j.actaastro.2022.02.013
Goordial, J. (2021). Cryomicrobial ecology: Still much to learn about life left out in the cold. mSystems 6:e0085221. doi: 10.1128/mSystems.00852-21
Gramain, A., Díaz, G. C., Demergasso, C., Lowenstein, T. K., and McGenity, T. J. (2011). Archaeal diversity along a subterranean salt core from the Salar Grande (Chile). Environ. Microbiol. 13, 2105–2121. doi: 10.1111/j.1462-2920.2011.02435.x
Grasset, O., Castillo-Rogez, J., Guillot, T., Fletcher, L. N., and Tosi, F. (2017). Water and volatiles in the outer solar system. Space Sci. Rev. 212, 835–875. doi: 10.1007/s11214-017-0407-z
Greenwood, J. P., Karato, S.-I., Vander Kaaden, K. E., Pahlevan, K., and Usui, T. (2018). Water and volatile inventories of mercury, venus, the moon, and Mars. Space Sci. Rev. 214:92. doi: 10.1007/s11214-018-0526-1
Gunde-Cimerman, N., Plemenitaš, A., and Oren, A. (2018). Strategies of adaptation of microorganisms of the three domains of life to high salt concentrations. FEMS Microbiol. Rev. 42, 353–375. doi: 10.1093/femsre/fuy009
Gupta, R. S., Naushad, S., Fabros, R., and Adeolu, M. (2016). A phylogenomic reappraisal of family-level divisions within the class Halobacteria: Proposal to divide the order Halobacteriales into the families Halobacteriaceae, Haloarculaceae fam. nov., and Halococcaceae fam. nov., and the order Haloferacales into the families, Haloferacaceae and Halorubraceae fam nov. Antonie Leeuwenhoek 109, 565–587. doi: 10.1007/s10482-016-0660-2
Hallsworth, J. E. (2021). Mars’ surface is not universally biocidal. Environ. Microbiol. 23, 3345–3350. doi: 10.1111/1462-2920.15494
Hallsworth, J. E., Mancinelli, R. L., Conley, C. A., Dallas, T. D., Rinaldi, T., Davila, A. F., et al. (2021b). Astrobiology of life on Earth. Environ. Microbiol. 23, 3335–3344. doi: 10.1111/1462-2920.15499
Hallsworth, J. E., Koop, T., Dallas, T. D., Zorzano, M.-P., Burkhardt, J., Golyshina, O. V., et al. (2021a). Water activity in Venus’s uninhabitable clouds and other planetary atmospheres. Nat. Astron. 5, 665–675. doi: 10.1038/s41550-021-01391-3
Hallsworth, J. E., Yakimov, M. M., Golyshin, P. N., Gillion, J. L. M., D’Auria, G., De Lima Alves, F., et al. (2007). Limits of life in MgCl2-containing environments: Chaotropicity defines the window. Environ. Microbiol. 9, 801–813. doi: 10.1111/j.1462-2920.2006.01212.x
Hamm, J. N., Erdmann, S., Eloe-Fadrosh, E. A., Angeloni, A., Zhong, L., Brownlee, C., et al. (2019). Unexpected host dependency of Antarctic Nanohaloarchaeota. Proc. Natl. Acad. Sci. U.S.A. 116, 14661–14670. doi: 10.1073/pnas.1905179116
Harris, J. K., Cousins, C. R., Gunn, M., Grindrod, P. M., Barnes, D., Crawford, I. A., et al. (2015). Remote detection of past habitability at Mars-analogue hydrothermal alteration terrains using an ExoMars Panoramic Camera emulator. Icarus 252, 284–300. doi: 10.1016/j.icarus.2015.02.004
Hayes, A. G. (2016). The Lakes and Seas of Titan. Annu. Rev. Earth Planet. Sci. 44, 57–83. doi: 10.1146/annurev-earth-060115-012247
Horneck, G. (1999). European activities in exobiology in Earth orbit: Results and perspectives. Adv. Space Res. 23, 381–386. doi: 10.1016/S0273-1177(99)00061-7
Horneck, G., Klaus, D. M., and Mancinelli, R. L. (2010). Space microbiology. Microbiol. Mol. Biol. Rev. 74, 121–156. doi: 10.1128/MMBR.00016-09
Horneck, G., Walter, N., Westall, F., Grenfell, J. L., Martin, W. F., Gomez, F., et al. (2016). AstRoMap European astrobiology roadmap. Astrobiology 16, 201–243. doi: 10.1089/ast.2015.1441
Jaakkola, S. T., Zerulla, K., Guo, Q., Liu, Y., Ma, H., Yang, C., et al. (2014). Halophilic Archaea cultivated from surface sterilized Middle-Late Eocene rock salt Are Polyploid. PLoS One 9:e110533. doi: 10.1371/journal.pone.0110533
Jebbar, M., Hickman-Lewis, K., Cavalazzi, B., Taubner, R.-S., Rittmann, S. K. M. R., and Antunes, A. (2020). Microbial diversity and biosignatures: An icy moons perspective. Space Sci. Rev. 216:10. doi: 10.1007/s11214-019-0620-z
Jia, X., Kivelson, M. G., Khurana, K. K., and Kurth, W. S. (2018). Evidence of a plume on Europa from Galileo magnetic and plasma wave signatures. Nat. Astron. 2, 459–464. doi: 10.1038/s41550-018-0450-z
Johnson, A. P., Pratt, L. M., Vishnivetskaya, T., Pfiffner, S., Bryan, R. A., Dadachova, E., et al. (2011). Extended survival of several organisms and amino acids under simulated martian surface conditions. Icarus 211, 1162–1178.
Karan, R., DasSarma, P., Balcer-Kubiczek, E., Weng, R. R., Liao, C.-C., Goodlett, D. R., et al. (2014). Bioengineering radioresistance by overproduction of RPA, a mammalian-type single-stranded DNA-binding protein, in a halophilic Archaeon. Appl. Microbiol. Biotechnol. 98, 1737–1747. doi: 10.1007/s00253-013-5368-x
Kereszturi, A., and Keszthelyi, Z. (2013). Astrobiological implications of chaos terrains on Europa to help targeting future missions. Planet. Space Sci. 77, 74–90. doi: 10.1016/j.pss.2012.08.028
King, G. M. (2015). Carbon monoxide as a metabolic energy source for extremely halophilic microbes: Implications for microbial activity in Mars regolith. Proc. Natl. Acad. Sci. U.S.A. 112, 4465–4470. doi: 10.1073/pnas.1424989112
Kish, A., Griffin, P. L., Rogers, K. L., Fogel, M. L., Hemley, R. J., and Steele, A. (2012). High-pressure tolerance in Halobacterium salinarum NRC-1 and other non-piezophilic prokaryotes. Extremophiles 16, 355–361. doi: 10.1007/s00792-011-0418-8
Kish, A., Kirkali, G., Robinson, C., Rosenblatt, R., Jaruga, P., Dizdaroglu, M., et al. (2009). Salt shield: Intracellular salts provide cellular protection against ionizing radiation in the halophilic Archaeon, Halobacterium salinarum NRC-1. Environ. Microbiol. 11, 1066–1078. doi: 10.1111/j.1462-2920.2008.01828.x
Knoll, A. H., and Grotzinger, J. (2006). Water on Mars and the prospect of martian life. Elements 2, 169–173. doi: 10.2113/gselements.2.3.169
Koike, J. (1991). Fundamental questions concerning the contamination of other planets with terrestrial micro-organisms carried by space-probes. J. Space Technol. Sci. 7, 9–14. doi: 10.11230/jsts.7.2_9
Koike, J., and Oshima, T. (1993). Planetary quarantine in the solar system. survival rates of some terrestrial organisms under simulated space conditions by proton irradiation. Acta Astronaut. 29, 629–632. doi: 10.1016/0094-5765(93)90080-G
Koike, J., Oshima, T., Kobayashi, K., and Kawasaki, Y. (1995). Studies in the search for life on Mars. Adv. Space Res. 15, 211–214. doi: 10.1016/S0273-1177(99)80086-6
Koike, J., Oshima, T., Koike, K. A., Taguchi, H., Tanaka, R., Nishimura, K., et al. (1992). Survival rates of some terrestrial microorganisms under simulated space conditions. Adv. Space Res. 12, 271–274. doi: 10.1016/0273-1177(92)90182-W
Koschnitzki, D., Moeller, R., Leuko, S., Przybyla, B., Beblo-Vranesevic, K., Wirth, R., et al. (2021). Questioning the radiation limits of life: Ignicoccus hospitalis between replication and VBNC. Arch. Microbiol. 203, 1299–1308. doi: 10.1007/s00203-020-02125-1
Kottemann, M., Kish, A., Iloanusi, C., Bjork, S., and DiRuggiero, J. (2005). Physiological responses of the halophilic Archaeon Halobacterium sp. strain NRC1 to desiccation and gamma irradiation. Extremophiles 9, 219–227. doi: 10.1007/s00792-005-0437-4
Kunte, H. J., Trüper, H. G., and Stan-Lotter, H. (2002). “Halophilic Microorganisms,” in Astrobiology: The Quest for the Conditions of Life, eds G. Horneck and C. Baumstark-Khan (Berlin: Springer), 185–200.
Lach, J., Jêcz, P., Strapagiel, D., Matera-Witkiewicz, A., and Sta̧czek, P. (2021). The methods of digging for “gold” within the salt: Characterization of Halophilic prokaryotes and identification of their valuable biological products using sequencing and genome mining tools. Genes 12:1756. doi: 10.3390/genes12111756
Lamarche-Gagnon, G., Comery, R., Greer, C. W., and Whyte, L. G. (2015). Evidence of in situ microbial activity and sulphidogenesis in perennially sub-0 °C and hypersaline sediments of a high Arctic permafrost spring. Extremophiles 19, 1–15. doi: 10.1007/s00792-014-0703-4
Lantz, C., Poulet, F., Loizeau, D., Riu, L., Pilorget, C., Carter, J., et al. (2020). Planetary Terrestrial Analogues Library project: 1. characterization of samples by near-infrared point spectrometer. Planet. Space Sci. 189:104989. doi: 10.1016/j.pss.2020.104989
Lasne, J., Noblet, A., Szopa, C., Navarro-González, R., Cabane, M., Poch, O., et al. (2016). Oxidants at the surface of Mars: A review in light of recent exploration results. Astrobiology 16, 977–996. doi: 10.1089/ast.2016.1502
Lauro, S. E., Pettinelli, E., Caprarelli, G., Guallini, L., Rossi, A. P., Mattei, E., et al. (2021). Multiple subglacial water bodies below the south pole of Mars unveiled by new MARSIS data. Nat. Astron. 5, 63–70. doi: 10.1038/s41550-020-1200-6
Laye, V. J., and DasSarma, S. (2018). An antarctic extreme halophile and its polyextremophilic enzyme: Effects of perchlorate salts. Astrobiology 18, 412–418. doi: 10.1089/ast.2017.1766
Laye, V. J., Karan, R., Kim, J.-M., Pecher, W. T., DasSarma, P., and DasSarma, S. (2017). Key amino acid residues conferring enhanced enzyme activity at cold temperatures in an Antarctic polyextremophilic β-galactosidase. Proc. Natl. Acad. Sci. U.S.A. 114, 12530–12535. doi: 10.1073/pnas.1711542114
Leuko, S., Bohmeier, M., Hanke, F., Böettger, U., Rabbow, E., Parpart, A., et al. (2017). On the stability of deinoxanthin exposed to mars conditions during a long-term space mission and implications for biomarker detection on other planets. Front. Microbiol. 8:1680. doi: 10.3389/fmicb.2017.01680
Leuko, S., Domingos, C., Parpart, A., Reitz, G., and Rettberg, P. (2015). The survival and resistance of Halobacterium salinarum NRC-1, Halococcus hamelinensis, and Halococcus morrhuae to simulated outer space solar radiation. Astrobiology 15, 987–997. doi: 10.1089/ast.2015.1310
Leuko, S., Neilan, B. A., Burns, B. P., Walter, M. R., and Rothschild, L. J. (2011). Molecular assessment of UVC radiation-induced DNA damage repair in the stromatolitic halophilic Archaeon, Halococcus hamelinensis. J. Photochem. Photobiol. B 102, 140–145. doi: 10.1016/j.jphotobiol.2010.10.002
Leuko, S., and Rettberg, P. (2017). The Effects of HZE Particles, γ and X-ray Radiation on the Survival and Genetic Integrity of Halobacterium salinarum NRC-1, Halococcus hamelinensis, and Halococcus morrhuae. Astrobiology 17, 110–117. doi: 10.1089/ast.2015.1458
Leuko, S., Rettberg, P., Pontifex, A. L., and Burns, B. P. (2014). On the response of halophilic Archaea to space conditions. Life 4, 66–76. doi: 10.3390/life4010066
Leuko, S., Stan-Lotter, H., Lamers, G., Sjöström, S., Rabbow, E., Parpart, A., et al. (2020). “Resistance of the Archaeon Halococcus morrhuae and the Biofilm-Forming Bacterium Halomonas muralis to Exposure to Low Earth Orbit for 534 Days,” in Extremophiles as Astrobiological Models, eds J. Seckbach and H. Stan-Lotter (Hoboken: Wiley-Scrivener), 221–236.
Leuko, S., Weidler, G., Radax, C., Legat, A., Kömle, N., Kargl, G., et al. (2002). “Examining the physico-chemical resistance of Halobacteria with the live-dead kit, following exposure to simulated Martian atmospheric conditions and heat,” in Proceedings of the First European Workshop on Exo-Astrobiology, ed. H. Lacoste (Paris: ESA), 473–474.
Lillis, R. J., and Brain, D. A. (2013). Nightside electron precipitation at Mars: Geographic variability and dependence on solar wind conditions. J. Geophys. Res. 118, 3546–3556. doi: 10.1002/jgra.50171
Loukas, A., Kappas, I., and Abatzopoulos, T. J. (2018). HaloDom: A new database of halophiles across all life domains. J. Biol. Res. Thessaloniki 25:2. doi: 10.1186/s40709-017-0072-0
Mancinelli, R. L. (2015). The affect of the space environment on the survival of Halorubrum chaoviator and Synechococcus (Nägeli): Data from the Space Experiment OSMO on EXPOSE-R. Int. J. Astrobiol. 14, 123–128. doi: 10.1017/S147355041400055X
Mancinelli, R. L., Fahlen, T. F., Landheim, R., and Klovstad, M. R. (2004). Brines and evaporites: Analogs for martian life. Adv. Space Res. 33, 1244–1246. doi: 10.1016/j.asr.2003.08.034
Mancinelli, R. L., White, M. R., and Rothschild, L. J. (1998). Biopan-survival I: Exposure of the osmophiles Synechococcus sp.(Nageli) and Haloarcula sp. to the space environment. Adv. Space Res. 22, 327–334. doi: 10.1016/S0273-1177(98)00189-6
Marion, G. M., Fritsen, C. H., Eicken, H., and Payne, M. C. (2003). The search for life on Europa: Limiting environmental factors, potential habitats, and earth analogues. Astrobiology 3, 785–811. doi: 10.1089/153110703322736105
Martin, E., Reinhardt, R., Baum, L., Becker, M., Shaffer, J., and Kokjohn, T. (2000). The effects of ultraviolet radiation on the moderate halophile Halomonas elongata and the extreme halophile Halobacterium salinarum. Can. J. Microbiol. 46, 180–187.
Martínez-Espinosa, R. M., Richardson, D. J., and Bonete, M. J. (2015). Characterisation of chlorate reduction in the haloarchaeon Haloferax mediterranei. Biochim. Biophys. Acta Gen. Subj. 1850, 587–594. doi: 10.1016/j.bbagen.2014.12.011
Martins, Z., Cottin, H., Kotler, J. M., Carrasco, N., Cockell, C. S., de la Torre Noetzel, R., et al. (2017). Earth as a tool for astrobiology—a european perspective. Space Sci. Rev. 209, 43–81. doi: 10.1007/s11214-017-0369-1
Martín-Torres, F. J., Zorzano, M.-P., Valentín-Serrano, P., Harri, A.-M., Genzer, M., Kemppinen, O., et al. (2015). Transient liquid water and water activity at Gale crater on Mars. Nat. Geosci. 8, 357–361. doi: 10.1038/ngeo2412
Mastascusa, V., Romano, I., Di Donato, P., Poli, A., Della Corte, V., Rotundi, A., et al. (2014). Extremophiles survival to simulated space conditions: An astrobiology model study. Orig. Life Evol. Biosph. 44, 231–237. doi: 10.1007/s11084-014-9397-y
McCready, S. (1996). The repair of ultraviolet light-induced DNA damage in the halophilic archaebacteria, Halobacterium cutirubrum, Halobacterium halobium and Haloferax volcanii. Mutat. Res. DNA Repair 364, 25–32. doi: 10.1016/0921-8777(96)00018-3
McCready, S., Müller, J. A., Boubriak, I., Berquist, B. R., Ng, W. L., and DasSarma, S. (2005). UV irradiation induces homologous recombination genes in the model Archaeon. Halobacterium sp. NRC-1. Saline Syst. 1:3. doi: 10.1186/1746-1448-1-3
McDuff, S., King, G., Neupane, S., and Myers, M. (2016). Isolation and characterization of extremely halophilic CO-oxidizing Euryarchaeota from hypersaline cinders, sediments and soils and description of a novel CO oxidizer, Haloferax namakaokahaiae Mke2. 3T, sp. nov. FEMS Microbiol. Ecol. 92:fiw028. doi: 10.1093/femsec/fiw028
Merino, N., Aronson, H. S., Bojanova, D. P., Feyhl-Buska, J., Wong, M. L., Zhang, S., et al. (2019). Living at the extremes: Extremophiles and the limits of life in a planetary context. Front. Microbiol. 10:780. doi: 10.3389/fmicb.2019.00780
Miralles-Robledillo, J. M., Bernabeu, E., Giani, M., Martínez-Serna, E., Martínez-Espinosa, R. M., and Pire, C. (2021). Distribution of denitrification among Haloarchaea: A Comprehensive Study. Microorganisms 9:1669. doi: 10.3390/microorganisms9081669
Moeller, R., Reitz, G., Douki, T., Cadet, J., Horneck, G., and Stan-Lotter, H. (2010). UV photoreactions of the extremely haloalkaliphilic Euryarchaeon Natronomonas pharaonis. FEMS Microbiol. Ecol. 73, 271–277. doi: 10.1111/j.1574-6941.2010.00893.x
Moissl-Eichinger, C. (2011). Archaea in artificial environments: Their presence in global spacecraft clean rooms and impact on planetary protection. ISME J. 5, 209–219. doi: 10.1038/ismej.2010.124
Moissl-Eichinger, C., Cockell, C., and Rettberg, P. (2016). Venturing into new realms? microorganisms in space. FEMS Microbiol. Rev. 40, 722–737. doi: 10.1093/femsre/fuw015
Mormile, M. R., Hong, B.-Y., and Benison, K. C. (2009). Molecular analysis of the microbial communities of mars analog lakes in Western Australia. Astrobiology 9, 919–930. doi: 10.1089/ast.2008.0293
Mou, Y.-Z., Qiu, X.-X., Zhao, M.-L., Cui, H.-L., Oh, D., and Dyall-Smith, M. L. (2012). Halohasta litorea gen. nov. sp. nov., and Halohasta litchfieldiae sp. nov., isolated from the Daliang aquaculture farm, China and from Deep Lake, Antarctica, respectively. Extremophiles 16, 895–901. doi: 10.1007/s00792-012-0485-5
Müller, V., Spanheimer, R., and Santos, H. (2005). Stress response by solute accumulation in Archaea. Curr. Opin. Microbiol. 8, 729–736. doi: 10.1016/j.mib.2005.10.011
Murray, A. E., Kenig, F., Fritsen, C. H., McKay, C. P., Cawley, K. M., Edwards, R., et al. (2012). Microbial life at –13 °C in the brine of an ice-sealed Antarctic lake. Proc. Natl. Acad. Sci.U.S.A. 109, 20626–20631. doi: 10.1073/pnas.1208607109
Musilova, M., Wright, G., Ward, J. M., and Dartnell, L. R. (2015). Isolation of radiation-resistant bacteria from Mars analog Antarctic Dry valleys by preselection, and the correlation between radiation and desiccation resistance. Astrobiology 15, 1076–1090. doi: 10.1089/ast.2014.1278
Myers, M. R., and King, G. (2020). Halobacterium bonnevillei sp. nov., Halobaculum saliterrae sp. nov. and Halovenus carboxidivorans sp. nov., three novel carbon monoxide-oxidizing Halobacteria from saline crusts and soils. Int. J. Syst. Evol. Microbiol. 70, 4261–4268. doi: 10.1099/ijsem.0.004282
Myers, M. R., and King, G. M. (2017). Perchlorate-coupled carbon monoxide (CO) oxidation: Evidence for a plausible microbe-mediated reaction in martian brines. Front. Microbiol. 8:2571. doi: 10.3389/fmicb.2017.02571
Myers, M. R., and King, G. M. (2019). Addendum: Perchlorate-coupled carbon monoxide (CO) Oxidation: Evidence for a plausible microbe-mediated reaction in martian brines. Front. Microbiol. 10:1158. doi: 10.3389/fmicb.2019.01158
Navarro-González, R., Vargas, E., de la Rosa, J., Raga, A. C., and McKay, C. P. (2010). Reanalysis of the viking results suggests perchlorate and organics at midlatitudes on Mars. J. Geophys. Res. 115:E12010. doi: 10.1029/2010JE003599
Ng, W. V., Kennedy, S. P., Mahairas, G. G., Berquist, B., Pan, M., Shukla, H. D., et al. (2000). Genome sequence of Halobacterium species NRC-1. Proc. Natl. Acad. Sci. U.S.A. 97, 12176–12181. doi: 10.1073/pnas.190337797
Nickerson, C. A., Medina-Colorado, A. A., Barrila, J., Poste, G., and Ott, C. M. (2022). A vision for spaceflight microbiology to enable human health and habitat sustainability. Nat. Microbiol. 7, 471–474. doi: 10.1038/s41564-021-01015-6
Nimmo, F., and Pappalardo, R. T. (2016). Ocean worlds in the outer solar system. J. Geophys. Res. 121, 1378–1399. doi: 10.1002/2016JE005081
Noe Dobrea, E. Z., and Swayze, G. (2010). “Acid Pedogenesis on Mars? Evidence for Top-Down Alteration on Mars from CRISM and HiRISE Data,” in 41st Annual Lunar and Planetary Science Conference LPI Contribution No. 1533, (Woodlands, TX), 2620.
Ojha, L., McEwen, A., Dundas, C., Byrne, S., Mattson, S., Wray, J., et al. (2014). HiRISE observations of Recurring Slope Lineae (RSL) during southern summer on Mars. Icarus 231, 365–376. doi: 10.1016/j.icarus.2013.12.021
Okeke, B. C., Giblin, T., and Frankenberger, W. T. (2002). Reduction of perchlorate and nitrate by salt tolerant bacteria. Environ. Pollut. 118, 357–363. doi: 10.1016/S0269-7491(01)00288-3
Olson, S. L., Jansen, M., and Abbot, D. S. (2020). Oceanographic considerations for exoplanet life detection. Astrophys. J. 895:19. doi: 10.3847/1538-4357/ab88c9
Oren, A. (2008). Microbial life at high salt concentrations: Phylogenetic and metabolic diversity. Saline Syst. 4:2. doi: 10.1186/1746-1448-4-2
Oren, A. (2014a). Halophilic Archaea on earth and in space: Growth and survival under extreme conditions. Philos. Trans. R. Soc. A 372:20140194. doi: 10.1098/rsta.2014.0194
Oren, A. (2014b). Taxonomy of halophilic Archaea: Current status and future challenges. Extremophiles 18, 825–834. doi: 10.1007/s00792-014-0654-9
Oren, A., Elevi Bardavid, R., and Mana, L. (2014). Perchlorate and halophilic prokaryotes: Implications for possible halophilic life on Mars. Extremophiles 18, 75–80. doi: 10.1007/s00792-013-0594-9
Parro, V., de Diego-Castilla, G., Moreno-Paz, M., Blanco, Y., Cruz-Gil, P., Rodríguez-Manfredi, J. A., et al. (2011). A microbial Oasis in the Hypersaline Atacama subsurface discovered by a life detector chip: Implications for the search for life on Mars. Astrobiology 11, 969–996. doi: 10.1089/ast.2011.0654
Pätzold, M., Häusler, B., Tyler, G. L., Andert, T., Asmar, S. W., Bird, M. K., et al. (2016). Mars express 10 years at Mars: Observations by the Mars express radio science experiment (MaRS). Planet. Space Sci. 127, 44–90. doi: 10.1016/j.pss.2016.02.013
Peeters, Z., Vos, D., Ten Kate, I., Selch, F., Van Sluis, C., Sorokin, D. Y., et al. (2010). Survival and death of the Haloarchaeon Natronorubrum strain HG-1 in a simulated martian environment. Adv. Space Res. 46, 1149–1155. doi: 10.1016/j.asr.2010.05.025
Pfeiffer, F., Broicher, A., Gillich, T., Klee, K., Mejía, J., Rampp, M., et al. (2008). Genome information management and integrated data analysis with HaloLex. Arch. Microbiol. 190, 281–299. doi: 10.1007/s00203-008-0389-z
Preston, L. J., and Dartnell, L. R. (2014). Planetary habitability: Lessons learned from terrestrial analogues. Int. J. Astrobiol. 13, 81–98. doi: 10.1017/S1473550413000396
Quinn, R. C., Martucci, H. F. H., Miller, S. R., Bryson, C. E., Grunthaner, F. J., and Grunthaner, P. J. (2013). Perchlorate radiolysis on Mars and the origin of martian soil reactivity. Astrobiology 13, 515–520. doi: 10.1089/ast.2013.0999
Race, M., Denning, K., Bertka, C. M., Dick, S. J., Harrison, A. A., Impey, C., et al. (2012). Astrobiology and society: Building an interdisciplinary research community. Astrobiology 12, 958–965. doi: 10.1089/ast.2011.0723
Ramstad, R., Barabash, S., Futaana, Y., Nilsson, H., and Holmström, M. (2018). Ion escape from Mars through time: An extrapolation of atmospheric loss based on 10 years of Mars express measurements. J. Geophys. Res. 123, 3051–3060. doi: 10.1029/2018JE005727
Rcheulishvili, N., Zhang, Y., Papukashvili, D., and Deng, Y.-L. (2020). Survey and evaluation of spacecraft-associated aluminum-degrading microbes and their rapid identification methods. Astrobiology 20, 925–934. doi: 10.1089/ast.2019.2078
Reid, I. N., Sparks, W. B., Lubow, S., McGrath, M., Livio, M., Valenti, J., et al. (2006). Terrestrial models for extraterrestrial life: Methanogens and halophiles at Martian temperatures. Int. J. Astrobiol. 5, 89–97. doi: 10.1017/S1473550406002916
Reimer, L. C., Vetcininova, A., Carbasse, J. S., Söhngen, C., Gleim, D., Ebeling, C., et al. (2019). BacDive in 2019: Bacterial phenotypic data for High-throughput biodiversity analysis. Nucleic Acids Res. 47:D631–D636. doi: 10.1093/nar/gky879
Rettberg, P., Antunes, A., Brucato, J., Cabezas, P., Collins, G., Haddaji, A., et al. (2019). Biological contamination prevention for outer solar system moons of astrobiological interest: What Do We Need to Know? Astrobiology 19, 951–974. doi: 10.1089/ast.2018.1996
Rittmann, S., Legat, A., Fendrihan, S., and Stan-Lotter, H. (2004). “Viability and morphology of Halobacterium species following desiccation - implications for contaminants on Mars,” in Proceedings of the Third European Workshop on Exo-Astrobiology, eds R. A. Harris and L. Ouwehand (Paris: ESA), 275–276.
Rivera-Valentín, E. G., Chevrier, V. F., Soto, A., and Martínez, G. (2020). Distribution and habitability of (meta)stable brines on present-day Mars. Nat. Astron. 4, 756–761. doi: 10.1038/s41550-020-1080-9
Romano, I., Camerlingo, C., Vaccari, L., Birarda, G., Poli, A., Fujimori, A., et al. (2022). Effects of ionizing radiation and long-term storage on hydrated vs. dried cell samples of Extremophilic microorganisms. Microorganisms 10:190. doi: 10.3390/microorganisms10010190
Rull, F., Veneranda, M., Manrique-Martinez, J. A., Sanz-Arranz, A., Saiz, J., Medina, J., et al. (2022). Spectroscopic study of terrestrial analogues to support rover missions to Mars – a raman-centred review. Anal. Chim. Acta 1209:339003. doi: 10.1016/j.aca.2021.339003
Sapers, H. M., Ronholm, J., Raymond-Bouchard, I., Comrey, R., Osinski, G. R., and Whyte, L. G. (2017). Biological characterization of microenvironments in a hypersaline cold spring Mars analog. Front. Microbiol. 8:2527. doi: 10.3389/fmicb.2017.02527
Schörghofer, N., Benna, M., Berezhnoy, A. A., Greenhagen, B., Jones, B. M., Li, S., et al. (2021). Water group exospheres and surface interactions on the moon. mercury, and Ceres. Space Sci. Rev. 217:74. doi: 10.1007/s11214-021-00846-3
Schreder-Gomes, S. I., Benison, K. C., and Bernau, J. A. (2022). 830-million-year-old microorganisms in primary fluid inclusions in halite. Geology 50, 918–922. doi: 10.1130/G49957.1
Sgambati, A., Deiml, M., Stettner, A., Kahrs, J., Brozek, P., Kapoun, P., et al. (2020). SPECTROModule: A modular in-situ spectroscopy platform for exobiology and space sciences. Acta Astronaut. 166, 377–390. doi: 10.1016/j.actaastro.2019.10.010
Shahmohammadi, H. R., Asgarani, E., Terato, H., Ide, H., and Yamamoto, O. (1997). Effects of 60Co gamma-rays, ultraviolet light, and mitomycin C on Halobacterium salinarium and Thiobacillus intermedius. J. Radiat. Res. 38, 37–43. doi: 10.1269/jrr.38.37
Shahmohammadi, H. R., Asgarani, E., Terato, H., Saito, T., Ohyama, Y., Gekko, K., et al. (1998). Protective roles of bacterioruberin and intracellular KCl in the resistance of Halobacterium salinarium against DNA-damaging agents. J. Radiat. Res. 39, 251–262. doi: 10.1269/jrr.39.251
Sharma, N., Farooqi, M. S., Chaturvedi, K. K., Lal, S. B., Grover, M., Rai, A., et al. (2014). The halophile protein database. Database 2014:bau114. doi: 10.1093/database/bau114
Sherwood Lollar, B., Heuer, V. B., McDermott, J., Tille, S., Warr, O., Moran, J. J., et al. (2021). A window into the abiotic carbon cycle – Acetate and formate in fracture waters in 2.7 billion year-old host rocks of the Canadian Shield. Geochim. Cosmochim. Acta 294, 295–314. doi: 10.1016/j.gca.2020.11.026
Shirsalimian, M. S., Amoozegar, M. A., Sepahy, A. A., Kalantar, S. M., and Dabbagh, R. (2017). Isolation of extremely halophilic Archaea from a saline river in the Lut Desert of Iran, moderately resistant to desiccation and gamma radiation. Microbiology 86, 403–411. doi: 10.1134/S0026261717030158
Simões, M. F., and Antunes, A. (2021). Microbial pathogenicity in space. Pathogens 10:450. doi: 10.3390/pathogens10040450
Sorokin, D. Y., Messina, E., La Cono, V., Ferrer, M., Ciordia, S., Mena, M. C., et al. (2018). Sulfur respiration in a group of facultatively Anaerobic Natrono Archaea Ubiquitous in Hypersaline Soda Lakes. Front. Microbiol. 9:2359. doi: 10.3389/fmicb.2018.02359
Sorokin, D. Y., Messina, E., Smedile, F., Roman, P., Damsté, J. S. S., Ciordia, S., et al. (2017). Discovery of anaerobic lithoheterotrophic Haloarchaea, ubiquitous in hypersaline habitats. ISME J. 11, 1245–1260. doi: 10.1038/ismej.2016.203
Spencer, J. R., and Nimmo, F. (2013). Enceladus: An active ice world in the Saturn System. Annu. Rev. Earth Planet. Sci. 41, 693–717. doi: 10.1146/annurev-earth-050212-124025
Spiga, A., Banfield, D., Teanby, N. A., Forget, F., Lucas, A., Kenda, B., et al. (2018). Atmospheric Science with InSight. Space Sci. Rev. 214:109. doi: 10.1007/s11214-018-0543-0
Stan-Lotter, H., and Fendrihan, S. (2015). Halophilic Archaea: Life with desiccation, radiation and oligotrophy over geological times. Life 5, 1487–1496. doi: 10.3390/life5031487
Stan-Lotter, H., Radax, C., Gruber, C., Legat, A., Pfaffenhuemer, M., Wieland, H., et al. (2003). Astrobiology with Haloarchaea from permo-triassic rock salt. Int. J. Astrobiol. 1, 271–284. doi: 10.1017/S1473550403001307
Stevenson, A., Cray, J. A., Williams, J. P., Santos, R., Sahay, R., Neuenkirchen, N., et al. (2015b). Is there a common water-activity limit for the three domains of life? ISME J. 9, 1333–1351. doi: 10.1038/ismej.2014.219
Stevenson, A., Burkhardt, J., Cockell, C. S., Cray, J. A., Dijksterhuis, J., Fox-Powell, M., et al. (2015a). Multiplication of microbes below 0.690 water activity: Implications for terrestrial and extraterrestrial life. Environ. Microbiol. 17, 257–277. doi: 10.1111/1462-2920.12598
Stillman, D. E., Michaels, T. I., and Grimm, R. E. (2017). Characteristics of the numerous and widespread recurring slope lineae (RSL) in Valles Marineris. Mars. Icarus 285, 195–210. doi: 10.1016/j.icarus.2016.10.025
Tarnas, J. D., Mustard, J. F., Sherwood Lollar, B., Stamenkoviæ, V., Cannon, K. M., Lorand, J. P., et al. (2021). Earth-like habitable environments in the subsurface of Mars. Astrobiology 21, 741–756. doi: 10.1089/ast.2020.2386
Taşkın, Z., and Aydinoglu, A. U. (2015). Collaborative interdisciplinary astrobiology research: A bibliometric study of the NASA Astrobiology Institute. Scientometrics 103, 1003–1022. doi: 10.1007/s11192-015-1576-8
Taubner, R.-S., Pappenreiter, P., Zwicker, J., Smrzka, D., Pruckner, C., Kolar, P., et al. (2018). Biological methane production under putative Enceladus-like conditions. Nat. Commun. 9:748. doi: 10.1038/s41467-018-02876-y
Thombre, R., Shinde, V., Dixit, J., Jagtap, S., and Vidyasagar, P. B. (2017). Response of extreme Haloarchaeon Haloarcula argentinensis RR10 to simulated microgravity in clinorotation. Biotech 7:30. doi: 10.1007/s13205-016-0596-2
Thombre, R. S., Bhalerao, A. R., Shinde, V. D., Dhar, S. K., and Shouche, Y. S. (2017). Response of haloalkaliphilic Archaeon Natronococcus jeotgali RR17 to hypergravity. Microgravity Sci. Technol. 29, 191–200. doi: 10.1007/s12217-017-9538-9
Tomkins, A. G., Genge, M. J., Tait, A. W., Alkemade, S. L., Langendam, A. D., Perry, P. P., et al. (2019). High Survivability of Micrometeorites on Mars: Sites With Enhanced Availability of Limiting Nutrients. J. Geophys. Res. 124, 1802–1818. doi: 10.1029/2019JE006005
Toner, J. D., and Catling, D. C. (2018). Chlorate brines on Mars: Implications for the occurrence of liquid water and deliquescence. Earth Planet. Sci. Lett. 497, 161–168. doi: 10.1016/j.epsl.2018.06.011
Trigui, H., Masmoudi, S., Brochier-Armanet, C., Maalej, S., and Dukan, S. (2011). Survival of extremely and moderately halophilic isolates of Tunisian solar salterns after UV-B or oxidative stress. Can. J. Microbiol. 57, 923–933. doi: 10.1139/w11-087
Ueta, S., and Sasaki, T. (2013). The Structures of Surface H2O Layers of Ice-Covered Planets with High-Pressure Ice. Astrophys. J. 775:96. doi: 10.1088/0004-637x/775/2/96
Ukani, H., Purohit, M. K., Georrge, J. J., Paul, S., and Singh, S. (2011). HaloBase: Development of database system for halophilic bacteria and Archaea with respect to proteomics, genomics & other molecular traits. J. Sci. Ind. 70, 976–981. doi: 10.5281/zenodo.4398512
Uritskiy, G., Tisza, M. J., Gelsinger, D. R., Munn, A., Taylor, J., and DiRuggiero, J. (2021). Cellular life from the three domains and viruses are transcriptionally active in a hypersaline desert community. Environ. Microbiol. 23, 3401–3417. doi: 10.1111/1462-2920.15023
Van Der Wielen, P. W. J. J., and Heijs, S. K. (2007). Sulfate-reducing prokaryotic communities in two deep hypersaline anoxic basins in the Eastern Mediterranean deep sea. Environ. Microbiol. 9, 1335–1340. doi: 10.1111/j.1462-2920.2006.01210.x
Vance, S., Bouffard, M., Choukroun, M., and Sotin, C. (2014). Ganymede×s internal structure including thermodynamics of magnesium sulfate oceans in contact with ice. Planet. Space Sci. 96, 62–70. doi: 10.1016/j.pss.2014.03.011
Vicente-Retortillo, A., Martínez, G. M., Rennó, N. O., Lemmon, M. T., de la Torre-Juárez, M., and Gómez-Elvira, J. (2020). In Situ UV Measurements by MSL/REMS: Dust deposition and angular response corrections. Space Sci. Rev. 216:97. doi: 10.1007/s11214-020-00722-6
Vikram, S., Guerrero, L. D., Makhalanyane, T. P., Le, P. T., Seely, M., and Cowan, D. A. (2016). Metagenomic analysis provides insights into functional capacity in a hyperarid desert soil niche community. Environ. Microbiol. 18, 1875–1888. doi: 10.1111/1462-2920.13088
Wadsworth, J., Rettberg, P., and Cockell, C. S. (2019). Aggregated Cell masses provide protection against space extremes and a microhabitat for hitchhiking co-inhabitants. Astrobiology 19, 995–1007. doi: 10.1089/ast.2018.1924
Wang, F., Li, M., Huang, L., and Zhang, X.-H. (2021). Cultivation of uncultured marine microorganisms. Mar. Life sci. Technol. 3, 117–120. doi: 10.1007/s42995-021-00093-z
Webb, K. M., Yu, J., Robinson, C. K., Noboru, T., Lee, Y. C., and DiRuggiero, J. (2013). Effects of intracellular Mn on the radiation resistance of the halophilic Archaeon Halobacterium salinarum. Extremophiles 17, 485–497. doi: 10.1007/s00792-013-0533-9
Weber, H. S., Habicht, K. S., and Thamdrup, B. (2017). Anaerobic Methanotrophic Archaea of the ANME-2d Cluster Are Active in a Low-sulfate, Iron-rich Freshwater Sediment. Front. Microbiol. 8:619. doi: 10.3389/fmicb.2017.00619
Weidler, G., Leuko, S., Radax, C., Kargl, G., Kömle, N., and Stan-Lotter, H. (2002). “Viability and DNA damage of Halobacteria under physical stress condition including a simulated Martian atmosphere,” in Proceedings of the First European Workshop on Exo-Astrobiology, ed. H. Lacoste (Paris: ESA), 491–492.
Weidler, G., Leunko, S., and Stan-Lotter, H. (2004). “Survival and growth of Halobacterium sp. NRC-1 following incubation at –15°C, freezing or freeze-drying, and the protective effect of cations,” in Proceedings of the Third European Workshop on Exo-Astrobiology, eds R. A. Harris and L. Ouwehand (Paris: ESA), 311–312.
Whitehead, K., Kish, A., Pan, M., Kaur, A., Reiss, D. J., King, N., et al. (2006). An integrated systems approach for understanding cellular responses to gamma radiation. Mol. Syst. Biol. 2:47. doi: 10.1038/msb4100091
Wu, Z., Wang, A., Farrell, W. M., Yan, Y., Wang, K., Houghton, J., et al. (2018). Forming perchlorates on Mars through plasma chemistry during dust events. Earth Planet. Sci. Lett. 504, 94–105. doi: 10.1016/j.epsl.2018.08.040
Xiao, L., Wang, J., Dang, Y., Cheng, Z., Huang, T., Zhao, J., et al. (2017). A new terrestrial analogue site for Mars research: The Qaidam Basin, Tibetan Plateau (NW China). Earth Sci. Rev. 164, 84–101. doi: 10.1016/j.earscirev.2016.11.003
Keywords: astrobiology, archaea, Halobacteria, extremophiles, Mars, icy moons
Citation: Wu J-H, McGenity TJ, Rettberg P, Simões MF, Li W-J and Antunes A (2022) The archaeal class Halobacteria and astrobiology: Knowledge gaps and research opportunities. Front. Microbiol. 13:1023625. doi: 10.3389/fmicb.2022.1023625
Received: 20 August 2022; Accepted: 07 September 2022;
Published: 13 October 2022.
Edited by:
Mohammad Ali Amoozegar, University of Tehran, IranReviewed by:
Ricardo Amils, Autonomous University of Madrid, SpainCopyright © 2022 Wu, McGenity, Rettberg, Simões, Li and Antunes. This is an open-access article distributed under the terms of the Creative Commons Attribution License (CC BY). The use, distribution or reproduction in other forums is permitted, provided the original author(s) and the copyright owner(s) are credited and that the original publication in this journal is cited, in accordance with accepted academic practice. No use, distribution or reproduction is permitted which does not comply with these terms.
*Correspondence: André Antunes, YWdsYW50dW5lc0BtdXN0LmVkdS5tbw==
Disclaimer: All claims expressed in this article are solely those of the authors and do not necessarily represent those of their affiliated organizations, or those of the publisher, the editors and the reviewers. Any product that may be evaluated in this article or claim that may be made by its manufacturer is not guaranteed or endorsed by the publisher.
Research integrity at Frontiers
Learn more about the work of our research integrity team to safeguard the quality of each article we publish.