- 1Section for Experimental Biomedicine, Department of Production Animal Clinical Sciences, Faculty of Veterinary Medicine, Norwegian University of Life Sciences, Ås, Norway
- 2State Key Laboratory of Biocontrol, Guangdong Key Laboratory of Pharmaceutical Functional Genes, School of Life Sciences, Southern Marine Science and Engineering Guangdong Laboratory (Zhuhai), Sun Yat-sen University, Higher Education Mega Center, Guangzhou, China
- 3Department of Paraclinical Sciences, Faculty of Veterinary Medicine, Norwegian University of Life Sciences, Ås, Norway
- 4Faculty of Biosciences and Aquaculture, Nord University, Bodø, Norway
Aeromonas media is a Gram-negative bacterium ubiquitously found in aquatic environments. It is a foodborne pathogen associated with diarrhea in humans and skin ulceration in fish. In this study, we used whole genome sequencing to profile all antimicrobial resistance (AMR) and virulence genes found in A. media strain SD/21–15 isolated from marine sediments in Denmark. To gain a better understanding of virulence and AMR genes found in several A. media strains, we included 24 whole genomes retrieved from the public databanks whose isolates originate from different host species and environmental samples from Asia, Europe, and North America. We also compared the virulence genes of strain SD/21–15 with A. hydrophila, A. veronii, and A. salmonicida reference strains. We detected Msh pili, tap IV pili, and lateral flagella genes responsible for expression of motility and adherence proteins in all isolates. We also found hylA, hylIII, and TSH hemolysin genes in all isolates responsible for virulence in all isolates while the aerA gene was not detected in all A. media isolates but was present in A. hydrophila, A. veronii, and A. salmonicida reference strains. In addition, we detected LuxS and mshA-Q responsible for quorum sensing and biofilm formation as well as the ferric uptake regulator (Fur), heme and siderophore genes responsible for iron acquisition in all A. media isolates. As for the secretory systems, we found all genes that form the T2SS in all isolates while only the vgrG1, vrgG3, hcp, and ats genes that form parts of the T6SS were detected in some isolates. Presence of blaMOX-9 and blaOXA-427 β-lactamases as well as crp and mcr genes in all isolates is suggestive that these genes were intrinsically encoded in the genomes of all A. media isolates. Finally, the presence of various transposases, integrases, recombinases, virulence, and AMR genes in the plasmids examined in this study is suggestive that A. media has the potential to transfer virulence and AMR genes to other bacteria. Overall, we anticipate these data will pave way for further studies on virulence mechanisms and the role of A. media in the spread of AMR genes.
Introduction
Aeromonas media was first reported as a new species by Allen et al. (1983) who isolated the bacterium from River Avon in Hampshire, England. Since then, it has been reported from sewage, sludge, lakes, rivers, and drinking water (Singh, 2000; Picao et al., 2008; Picão et al., 2008; Figueira et al., 2011; Pablos et al., 2011). In humans, A. media has mostly been isolated from diarrhea patients (Singh, 2000) while in fish it has been linked to skin ulcerations (Lü et al., 2016). In fish, it has been isolated from Koi carp (Cyprinus carpio; Lü et al., 2016), catfish (Clarias batrachus; Singh, 2000), bluntnose bream (Megalobrama amblycephala), eel (Anguilla anguilla; Yi et al., 2013), southern black bream (Acanthopagrus butcheri; Zhou et al., 2013), and crucian carp (Carassius carassius; Hu et al., 2012). In shellfish, it has been isolated from oysters (Crassostrea rhizophorea; Evangelista-Barreto et al., 2006), snails (Roger et al., 2012; Talagrand-Reboul et al., 2017), Yesso scallop (Patinopecten yessoensis; De Silva et al., 2019), shrimps (Litopenaeus vannamei; De Silva et al., 2018), cockles (Tegillarca granosa; Dahanayake et al., 2020), and clam (Ruditapes philippinarum; Dahanayake et al., 2019). In Norwegian markets, it has been isolated from retail foods such as sushi, oysters, and scallops (Hoel et al., 2017; Lee et al., 2021), while in Korean markets it has also been isolated from frozen shrimps, clams, and Yesso scallop (De Silva et al., 2018, 2019; Dahanayake et al., 2019, 2020). It has also been isolated from chilled chicken in China (Wang et al., 2017; Shao et al., 2022), turkey in Germany (Shen et al., 2018), and pork and pig slaughter house in Portugal (Fontes et al., 2011). These studies show that A. media can be transmitted to humans through food, drinking water and the environment.
Although A. media has been linked to diarrhea in humans and skin ulcerations in fish (Lü et al., 2016), there is limited information describing the profile of virulence factors found in A. media. It is unknown whether A. media shares a similar composition of virulence genes with other Aeromonas spp. like A. hydrophila, A. veronii, and A. salmonicida. As pointed out by Guerra et al. (2007) and Bhowmick and Bhattacharjee (2018) Aeromonas virulence is multifactorial involving various factors like endotoxins, enterotoxins, cytotoxins, hemolysins, proteases, and adhesins. However, A. hydrophila, A. veronii, A. caviae, and A. sobria are considered as major pathogens in the genus Aeromonas because they account for the largest proportion of the aeromonads isolated from clinical cases unlike A. media, which is considered a minor pathogen because of fewer cases isolated from human and animal diseases. Thus, there have been more virulence factor studies done for the major Aeromonas pathogens than for minor pathogenic species like A. media (Piotrowska and Popowska, 2015; Rasmussen-Ivey et al., 2016; Romero et al., 2016; Gauthier et al., 2017; Talagrand-Reboul et al., 2017). However, the increasing number of cases linked to human and animal infections reported in recent years coupled with increasing isolations from retail ready-to-eat foods (Fontes et al., 2011; Wang et al., 2017; Shen et al., 2018; De Silva et al., 2019; Shao et al., 2022) indicates that A. media is emerging as an important environmental and foodborne pathogen with significant public health implications. Thus, there is need to elucidate the virulence factors of A. media isolated from different aquatic environments and host species with the view of developing effective control measures.
Antimicrobial resistance (AMR) has emerged to be an important global public health threat classified as among the top 10 global priorities by World Health Organization (2021). Multidrug resistant Aeromonas spp. have been isolated from different aquatic environments, animals, and retail foods (Stratev and Odeyemi, 2016; Teodoro et al., 2022). Also, Aeromonas spp. have been shown to carry plasmids encoding AMR and virulence genes (Tomás, 2012). Although previous studies reported the presence of AMR and virulence genes from Aeromonas spp. that included A. media isolated from ready-to-eat foods in Norway, the major limitation with these studies was that they used primers that targeted only a few selected genes, which did not give a global overview of all AMR genes present in bacteria genomes. Thus, in the present study, we used whole genome sequencing (WGS) to characterize all virulence and AMR genes present in A. media isolated from marine sediments collected from the Øresund Bay in Denmark. To gain a wide overview of the virulence and AMR genes found in A. media strains isolated from different geographical areas, we compared our isolate (strain SD/21–15) with genomes of 24 other isolates from Europe, North America, and South America retrieved from the National Center for Biotechnology Information (NCBI). We also compared our isolate with whole genome sequences of A. hydrophila, A. veronii, and A. salmonicida reference strains to determine the difference in the composition of virulence genes between A. media strain SD/21–15 and other Aeromonas spp. Our findings show that WGS is a reliable tool able to profile all AMR and virulence genes found in bacteria genomes unlike PCR based assays that only identify a few selected genes based on the primers used in the assay. Thus, we found a high similarity in the profile of AMR and virulence genes found in strain SD/21–15 with other A. media strains isolated from different host species and geographical areas in the world. Our findings show that A. media harbors several intrinsic AMR genes that could be transmissible to other bacteria species and it also harbors several virulence genes that could be responsible for its pathogenicity in different host species. We anticipate that data generated in this study will shed new insights on the role of A. media in the spread AMR genes and that it will pave way for studies aimed at elucidating the virulence mechanisms of A. media in different susceptible hosts.
Materials and methods
Characterization of bacteria using MALDI-TOF and sequences of The 16S rRNA gene
The A. media isolate designated as strain SD/21–15, originally isolated from marine sediments collected from Øresund in Denmark in 1992 (Andersen and Sandaa, 1994), was retrieved from the −80°C freezer and cultured in tryptose soy broth (TSB) followed by incubation at 10°C for 5–7 days. The isolate was previous classified as Aeromonas spp. (Andersen and Sandaa, 1994). The bacteria initially grown in TSB was later cultured on blood agar plates by incubation at 10°C for 5–7 days for individual colony purity followed by characterization using the Matrix-Assisted Laser Desorption/Ionization-Time Of Flight (MALDI-TOF) mass spectrometry (MS; Singhal et al., 2015). The purified bacteria confirmed by MALDI-TOF were used for DNA extraction using the DNA extraction kit based on the manufacturer’s protocol (Qiagen, Germany). Species identification and confirmation was carried out by PCR amplification of the 16S rRNA gene using the universal primers 27F and 1492R (Kuncham et al., 2017).
Testing of antimicrobial resistance using disk diffusion assay
The antibiotic disk experiment was carried out based on the Clinical and Laboratory Standards Institute (CLSI; Cockerill et al., 2012) guidelines to determine the susceptibility or resistance of bacteria to antibiotic treatment (Kahlmeter et al., 2006). The A. media isolate from Øresund in Denmark (Andersen and Sandaa, 1994) was tested for antibiotic resistance using the Kirby-Bauer disk diffusion assay (Joseph et al., 2011) using commercially available antibiotic discs (Neo-SensitabsTM, Rosco). Antibiotics used in the disk diffusion test were Ciprofloxacin (CIPR—5 μg), Erythromycin (Ery—15 μg), Gentamycin (GEN—10 μg), Ampicillin (AMP—10 μg), Cefoxitin (CFO—30 μg), Cephalothin (CEP—30 μg), Nitrofurantoin (NI—300 μg), Penicillin (PEN—10 μg), Tetracycline (TET—30 μg), Trimethoprim (TRIM—5 μg), Colistin (CO—150 μg), Sulfonamide (SULFA—240 μg), Amoxicillin (AMOXY—30 μg), Rifampicin (RIF—5 μg). The bacteria cultured overnight was diluted to 0.5 McFarland at a concentration of 108 CFU/ml and was spread on the surface of the Muller Hinton agar using sterile cotton swabs (Saffari et al., 2016). The antibiotics discs were put on the plate containing the bacterial lawn. This was followed by incubation at 10°C for 5–7 days. Afterward, antibiotic susceptibility and resistance was measured based on the manufacturer’s instruction (Neo-SensitabsTM, Rosco).
Bacterial genomic DNA extraction and quality control analysis
Genomic DNA (gDNA) was extracted from A. media strain SD/21–15 isolate using the MagAttract® HMW DNA kit based on manufacturer’s protocols (Qiagen, Germany) (Becker et al., 2016). A concentration of 2 × 109 CFU/ml freshly grown A. media strain SD/21–15 was centrifuged in 2 ml Eppendorf tubes, and pellets were resuspended in 180 μl ATL buffer followed by adding 20 μl Proteinase K to each tube. This was followed by incubation at 56°C in an Eppendorf thermomixer for 30 min. Afterward, 4 μl RNase was added to each tube followed by pulse vortexing and adding 15 μl of MagAttract Suspension G and 280 μl Buffer MB to each vial (Tarumoto et al., 2017). Next, the suspension from each tube was transferred onto a MagAttract holder followed by mixing for 60 s on an Eppendorf thermomixer. Magnetic beads containing gDNA were separated on the MagAttract magnetic rack for 60 s. Supernatants were removed without disturbing the beads and were washed twice using MW1 and PE buffer (Becker et al., 2016; Tarumoto et al., 2017). Thereafter, the remaining suspension from each vial was removed by rinsing the beads with 1 ml distilled water twice. The gDNA was harvested by eluting in 100 μl buffer EB while the purity of the gDNA was assessed using the NanoDrop (Thermo fisher, United States) followed by gel electrophoresis using 1% agarose. The harvested gDNA was quantified using the Qubit double-stranded DNA (dsDNA) high-CHS kit based on the manufacturer’s instructions (Life Technologies Inc., Carlsbad, CA, United States; Guan et al., 2020).
Library preparation and sequencing
The sequence library for A. media strain SD/21–15 was prepared using the paired end DNA libraries using the Nextera DNA Flex Tagmentation (Illumina Inc. San Diego, CA, United States; Gaio et al., 2021) while the Illumina library was quantified using the Qubit® DNA HS Assay Kit in a Qubit fluorometer (Thermo Fisher Scientific, Waltham, MA, United States). Agilent HS DNA Kit (Agilent Technologies, CA, United States) based on the Agilent 2,100 Bioanalyzer System was used to check the size of library fragments. Illumina MiSeq (Illumina Inc., United States) was used for sequencing using V3 reagent kits using paired-end read length of 2 × 300 bp as previously described (Kaspersen et al., 2020). Bioinformatic analysis was done using the online Galaxy platform1 version 21.05. Quality of both forward and reverse raw reads was analyzed using the FastQC Version 0.11.9 software (Bioinformatics, 2011). Adapters and low-quality reads from paired end sequences were removed using Trimmometric version 0.38.1 (Bolger et al., 2014). Afterward, the resulting paired-end sequence reads were de novo assembled into contigs using A5-miseq assembler (Coil et al., 2015). Quality read sequence contigs with 33–91 k-mers were assembled using SPAdes v. 3.12.0 (Bankevich et al., 2012). Genome annotation was made using the prokaryotic genome annotation pipeline (PGAP; Tatusova et al., 2016) from NCBI while annotation was done using Prokka (Seemann, 2014).
Prediction of average nucleotide identity and virulence genes
In addition to the A. media strain SD/21–15 whole genome sequence (WGS), we retrieved 24 WGSs of A. media isolates from the NCBI database obtained from different host species and environmental samples from Asia, Europe, and North America (Table 1). It is noteworthy that although A. media strain SD/21–15 was isolated in 1992 when it was classified as Aeromonas spp. (Andersen and Sandaa, 1994), the 24 genomes retrieved from the NCBI database covered the period 2013–2022 because there were no whole genome sequences of A. media prior to 2013 found in the NCBI database. The Galaxy platform using abricate v. 1.0.1 was used to identify genes of the virulence factors of pathogenic bacteria (VFDB; Seemann, 2014, 2020; Chen et al., 2005) of which the threshold for virulence-gene identification using the VFDB was set at 80%. On the other hand, the Average Nucleotide Identity (ANI) of all 25 A. media genomes was analyzed using the online Galaxy Europe2 using FastANI v. 1.3. Aeromonas media strain MC64 from the Chinese hospital (CP047962.1) was used as a reference to calculate the ANI of all the 25 A. media genomes (Table 1). The threshold for FastANI was set at 90% based on pairwise sequence mapping (Jain et al., 2018) while heatmap based on calculated ANI for all 25 A. media genomes were generated using the package heatmap in the R studio v 4.0.4 statistical software with online Orion NMBU software.3
Prediction of antimicrobial resistance genes and mobile genetic elements
A total of 25 A. media whole genome sequences were used for identification of AMR genes, plasmids and transposons. Staramr version 0.7.2 (Tran et al., 2021) and ABRicate version 1.0.1 (Seemann, 2014, 2020) were used for identification of antibiotic resistance genes in the Comprehensive Antimicrobial Resistance Database (CARD) software (Alcock et al., 2020) of which the CARD identification threshold for AMR-genes was set at 80%. Identification of plasmids in bacterial genomes was done using Plasmidfinder v 2.0 (Ullah et al., 2020) with the threshold for plasmid identification set at 80%. Proksee software4 was used to generate circular maps of all 25 A. media genomes and plasmids online.
Results
Whole genome sequencing and phylogenetic analysis
The A. media genomes retrieved from the NCBI databank were from Asia, Europe, and North America with the majority coming from Asia (Table 1). Thus, we did not find whole genome sequences of A. media isolates from Africa, Central, and South America in the public database. The genome size varied between 4.5 and 5.2 Mb while the GC content varied between 59 and 62.5% for all isolates (Table 1). The number of genes detected varied between 3,934 and 5,101 while the number of proteins varied between 3,535 and 4,704. Apart from the archived strain SD/21–15 obtained from marine sediments in Øresund in Denmark in 1992, all A. media strains used were isolated from the period 2013 to 2022 indicating that they were isolated in the last decade. Other details that include strain names, country of origin, and accession numbers are shown in Table 1. The circular map showing all A. media genomes used shows that strain SD/21–15 had a complete genome comparable with other A. media isolates obtained from different host species and the environment (Figure 1). Equally, phylogenetic analysis showed high similarity (>94%) of strain SD/21–15 with other A. media isolates from different host species and environments.
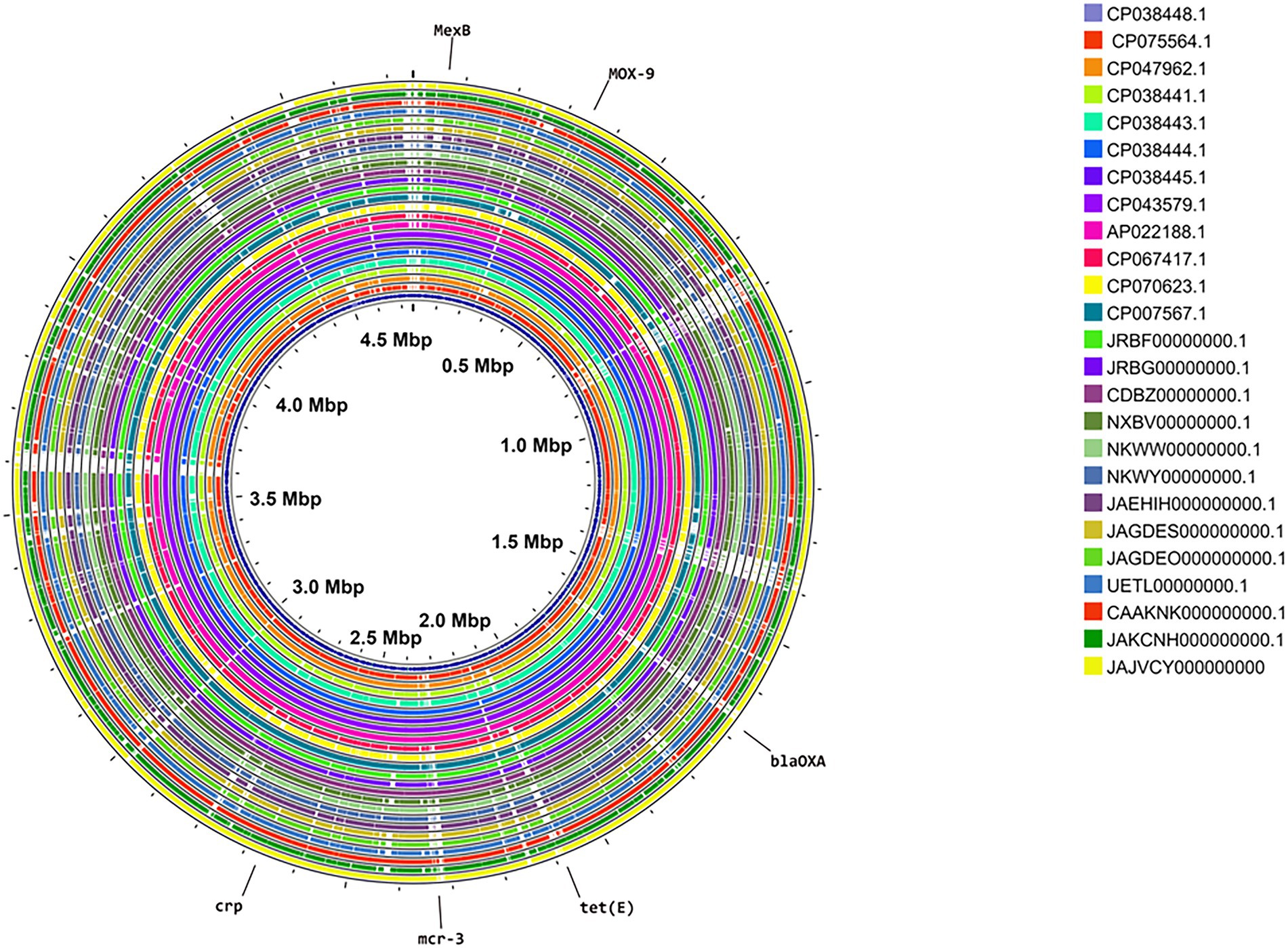
Figure 1. Circular map showing a comparison of the genome of Aeromonas media strain SD/21–15 together with genomes retrieved from the National Center for Biotechnology Information (NCBI) public databank obtained from different host species and environmental samples from different geographical areas in the world (See Table 1). Note strain SD/21–15 (JAJVCY000000000; outermost) shows a complete circular map similar with other 25 A. media strains. Figure 1 was created in Proksee (https://proksee.ca/).
Average nucleotide identity and heatmap
The ANI phylogenetic analysis showed high similarity (>94%) of all A. media isolates despite emanating from different host species and geographical areas (Figure 2). The ANI of A. media strain SD/21–15 was >97% similar with the Chinese hospital strain MC64 (CP047962.1) used as a reference. The ANI phylogenetic tree clustered all 25 isolates into two groups, of which group-I comprised of 17 isolates with >97% similarities that included isolates from Denmark (SD/21–15), Japan (ARB13, ARB20, and WP7-W18-ESBL-02), China (T0.1–19, R1-26, and E31), United States (CECT 4232), Spain (ATCC 33907), and United Kingdom (KLG6; Figure 2). On the other hand, group-II comprised seven isolates with >93% similarities consisting of isolates from United States (BAQ071013-132 and BAQ071013-115), Spain (D180), Portugal (INSAq193), Thailand (Colony414), and China (CN17A0010 and Z1-6).
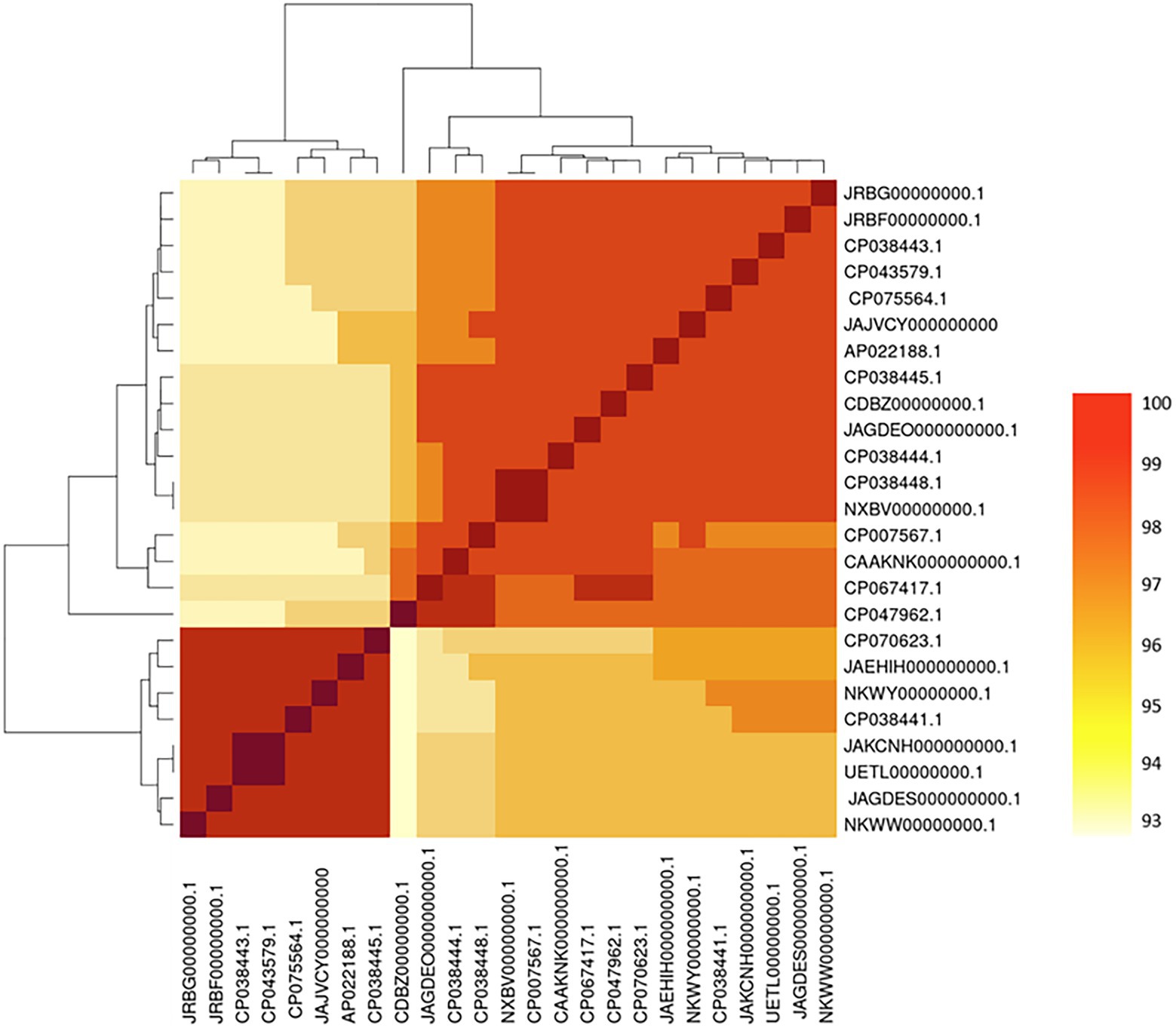
Figure 2. Heatmap/cladogram combined with phylogenetic comparison of Aeromonas media strain SD/21–15 isolated from an aquatic environment in Denmark with other strains retrieved from the National Center for Biotechnology Information (NCBI) public databank isolated from different host species and environments in the world. Note the high similarity among all 25 A. media strains with homology varying between 93 and 100%.
Virulence factors
The virulence factors examined comprised of six elements, namely; (i) adherence and motility, (ii) immune evasion, (iii) secretions system, (iv) toxins, (v) iron acquisition, and (vi) biofilm formation together with quorum sensing (Figure 3; Table 2).
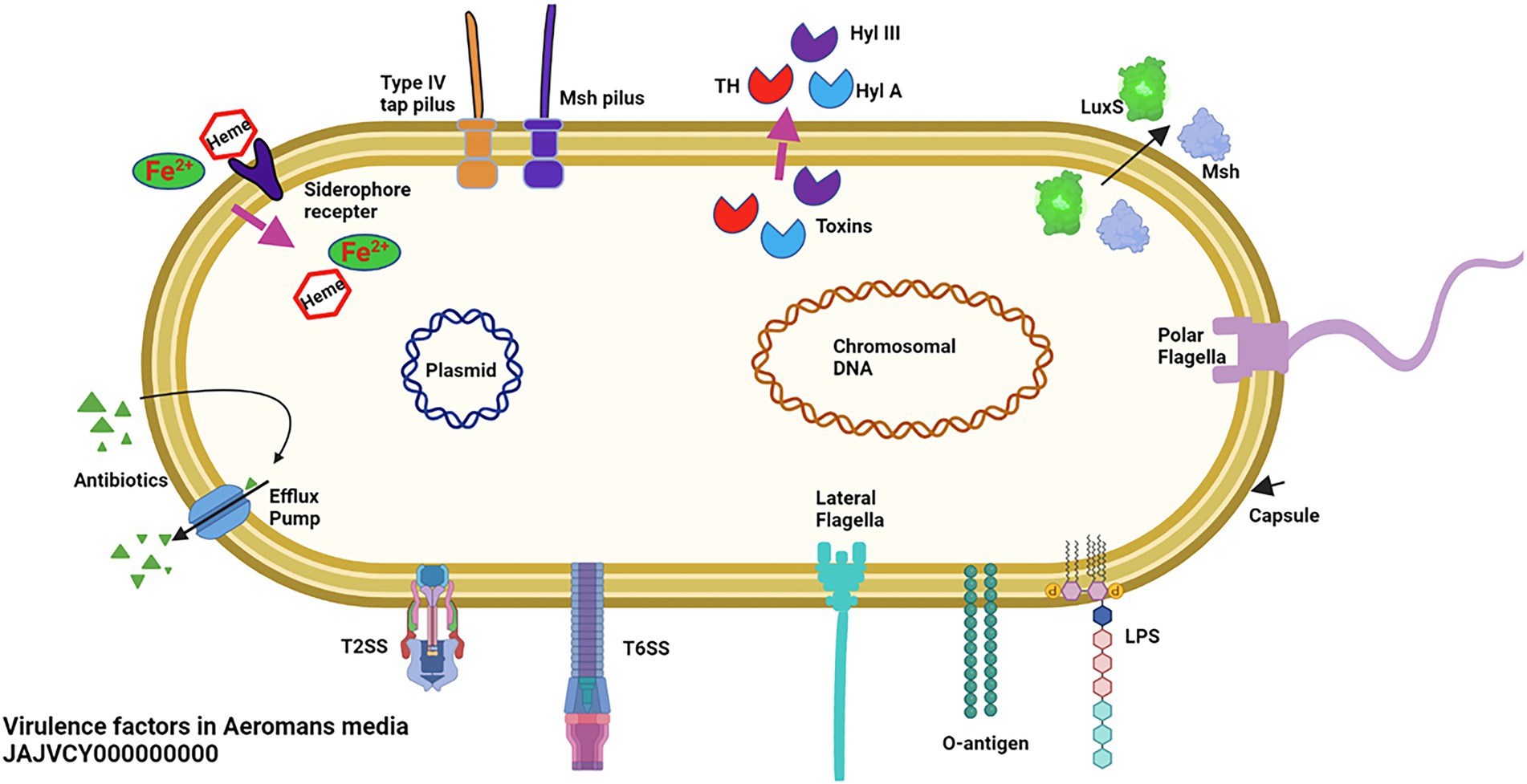
Figure 3. Schematic diagram of virulence genes on Aeromonas media included investigated showing (i) adherence proteins consisting of the Msh and type tap IV pili as well as the polar and lateral flagella, (ii) iron acquisition components comprising of the ferric uptake regulator (Fur), heme, and siderophore proteins, (iii) secretion system consisting of T2SS and T6SS, (iv) hemolysins consisting of hemolysin A (hylA), hemolysin III (hylIII), and thermostable heat (TH) protein, (v) biofilm and quorum sensing components consisting of S-ribosylhomocysteinase (LuxS) and MQS, (iv) Immune proteins consisting of capsule, lipopolysaccharide (LPS), and somatic O-antigen. In addition, schematic diagram shows A. media components associated with antimicrobial resistance (AMR) components consisting of (vii) efflux pumps and (viii) plasmid. Figure 3 was created in BioRender.com (https://biorender.com/).
Adherence, motility proteins, and immune evasion genes
The adherence and motility genes detected were classified into four groups namely the (i) Msh pilus, (ii) Tap type IV pili, (iii) polar flagellar, and (iv) lateral flagella (Table 2). The Msh pilus, polar flagellar, and Tap type IV pili genes were detected in all 25 A. media strains including strain SD/21–15 while the lateral flagella genes were only found in strains BAQ071013-132 and BAQ071013-1115 isolated from perch in the United States as well as strain D180 isolated from fish in Spain (Table 2). Comparison of strain SD/21–15 with other Aeromonas spp. showed that it had all genes that form the Flp type IV and polar flagella proteins similar with the A. hydrophila (ATCC 7966), A. veronii (B565), and A. salmonicida (A449) reference strains (Supplementary Table S1). However, it only had 15 genes that form the Tap type IV pili unlike A. veronii (B565), A. hydrophila (ATCC 7966), and A. salmonicida (A449) reference strains that had 20, 22, and 23 proteins, respectively. Our findings also show that A. media SD/21–15 strain did not have type I fimbriae genes found in A. veronii (B565), A. hydrophila (ATCC 7966), and A. salmonicida (A449) reference strains. In addition, genes that form the lateral flagella were only detected in A. salmonicida (A449) but not in A. media strain SD21/01–15 and the other Aeromonas reference strains (Supplementary Table S1). On the contrary, the polar flagella genes were detected in all four Aeromonas spp. examined although A. hydrophila (ATCC 7966) and A. media strain SD/21–15 had more genes that form the polar flagella than A. veronii (B565) and A. salmonicida (A449) reference strains (Supplementary Table S1).
Capsule and immune evasion genes
Our findings show that only eight of the 25 isolates examined had capsules and those included the strains SD/21–15, ARB13, CN17A0010, D180, ATCC 33907, Z1-6, KLG6, and INSAq193 (Table 2). A comparison of strain SD/21–15 with other Aeromonas spp. showed that only strain SD/21–15 had a capsule, and no capsule genes were detected in the genomes of A. hydrophila (ATCC 7966), A. veronii (B565), and A. salmonicida (A449; Supplementary Table S1). Other immune evasion genes detected in A. media strain SD/21–15 include the nitrate reductase (narH), antiphagocytosis capsule (wzb), serum resistance LPS (rfb), and stress adaptation catalase peroxidase (karG) genes (Supplementary Table S1).
Secretion system
Although we investigated the presence of all secretory systems, our findings show that only the type II secretory system (T2SS) was detected in all 25 A. media (Table 2). Comparative analysis showed that strain SD/21–15 had 14 of the 15 T2SS genes ranging from exeA to exeM with the exception of exeN while A. hydrophila (ATCC 7966), A. veronii (B565), and A. salmonicida (A449) had all 15 genes from exeA to exeN (Supplementary Table S1). On the contrary, the type III secretory system (T3SS) was not detected in all 25 A. media genomes, and it was not detected in A. hydrophila (ATCC 7966), A. veronii (B565), and A. salmonicida (A449) reference strains. As for the type VI secretory system (T6SS), only three isolates had the genes vrG1, vgrG3, hcp, and ats genes in their genomes while most strains only had two of these genes detected (Table 2). Comparison of strain SD/21–15 with other Aeromonas spp. showed that only A. hydrophila (ATCC 7966) had all 25 genes that form the T6SS while A. salmonicida (A449) had 14 and A. veronii (B565) had none (Supplementary Table S1).
Hemolysin and other toxin genes
All A. media isolates had hemolysin genes namely hemolysin HlyA (hlyA), hemolysin III (hlyIII), and thermostable hemolysin (TSH) genes (Table 2). On the contrary, the aerolysin gene was not detected in all 25 A. media strains while the RTX toxin genes were only detected from Strain NXB, T0.1–19, and Z1-6 from chicken meat, sludge, and humans in China (Tables 1, 2), respectively. Comparison of strain SD/21–15 with other Aeromonas spp. showed that the aerolysin AerA/cytotoxic enterotoxin aerA/act gene was present in A. hydrophila (ATCC 7966), A. veronii (B565), and A. salmonicida (A449) reference strains but not in strain SD/21–15. Other toxin genes, such as the heat stable cytotoxic enterotoxin (ast) and the repeat toxins (RTX; rtxA, rtxB, rtxC, rtxD, rtxE, and rtxH) genes only found in A. hydrophila (ATCC 7966) were not detected in strain SD/21–15. It also lacked the extracellular hemolysin (ahh1) gene found in A. hydrophila (ATCC 7966) and A. salmonicida (A449). On the other hand, hlyA, hlyIII, and TH toxin genes found in strain SD/21–15 were also present in A. hydrophila (ATCC 7966), A. veronii (B565), and A. salmonicida (A449) reference strains.
Iron acquisition, biofilm formation, and quorum sensing genes
The biofilm and quorum sensing luxS and mshA-Q genes were present in the genomes of all A. media isolates examined (Table 2). Similarly, the iron acquisition genes consisting of the gene of ferric uptake regulator (fur), siderophore synthesis, and heme uptake genes were present in all A. media isolates (Table 2).
Antimicrobial resistance
Phenotype characterization using the disk diffusion test
The A. media strain SD/21–15 showed multidrug resistance (MDR) to more than five antibiotics that included AMP-10 and PEN-10. They also showed resistance to CFO-30, CEP-30, TET-30, and AMOXY-30. It showed intermediate resistance for CIPR-5, ERY-15, and RIF-5 but was susceptible to Gentamycin GEN-10, NI-300, SULFA-, and TRIM-5 (Table 3) on the disk diffusion test.
Antimicrobial resistance genes
Whole genome sequence analysis showed that all 25 A. media isolates had multiple AMR genes encoded in their genomes (Table 4). Only blaKPC-1 and blaTEM-1 were detected among class A β-lactamases, of which blaKPC-1 was found in strains MC64 and E31 that were isolated from a hospital and water in China while blaTEM-1 was found in strains MC64, E31 and INSAq193 isolated from hospital, water and fish from China and Portugal, respectively. The only gene identified in the class B metallo-β-lactamases (MBL) was cphA7 found in strains R1-18 and R1-26 isolated from sludge and biofilm reactors in China, respectively. The class C β-lactamase group was dominated by blaMOX-9 found in all 25 A. media isolates while blaCMY-8b was only detected in strain SD/21–15. Equally, class D was dominated by blaOXA-427 found in all 25 A. media strains while blaOXA-1 was only found in strain R50-22 and blaOXA-10 in strain WP7-W18-ESBL-02. Outside the β-lactamase, the dominant AMR genes detected were CRP and MCR that were present in all 25 A. media isolates followed by MCR-3 and MCR-3.6 that were detected in eight, and sul1 from five isolates. Other AMR genes detected from different A. media strains are shown in Table 4.
Multidrug resistance proteins
Our findings show that different genes encoding multidrug resistance proteins were detected from each of the 25 A. media isolates. Among these mexB was detected in 24 of the total 25 A. media isolates while tetE was detected in 11 and Mcr3 in seven of the 25 isolates (Table 4). Other multidrug efflux pump proteins detected included vatF, catB, mphA, mphE, msrE, arr-3, and ugd tet(E) (Table 4).
Mobile genetic elements
Components of the mobile genetic elements (MBE) identified consisted of the transposases, integrases, recombinases, and plasmids (Tables 5, 6; Figure 5). Our findings show that strain D/21–15 had more transposons detected than the other strains (Table 5). Although the Tn3 family of transposons was detected in several strains, the insertion sequence (IS) class of transposons was the most dominant in all A. media isolates. Although integrase was detected in all 25 isolates only six isolates had all four components of the recombinases comprising of the recombinase family protein, tyrosine recombinase XerC, recombinase RecA, and site-specific tyrosine recombinase XerD while most strains only had the tyrosine recombinase XerC and site specific tyrosine recombinase XerD (Table 5). Finally, only 10 isolates had plasmids of which strains MC64, R25-3, R50-22, and E31 had two plasmids each while strains TR3_1, SD/21–15, T0.1–19, T5-8, INSAq193, and WS had only one plasmid each (Table 6). We generated circular maps from six out of the 10 plasmids detected to determine whether they encoded AMR genes, transposons, and integrases. The circular map of strain SD/21–15 plasmid has no AMR genes, transposases, or integrase encoded in its genome (Figure 4A). However, the circular maps of strains TR3_1, R50-22, MC64, PE31A, and T5-1 plasmids encoded AMR genes, transposases, virulence factors, efflux pumps, recombinases, and other genes (Figures 4B–F).
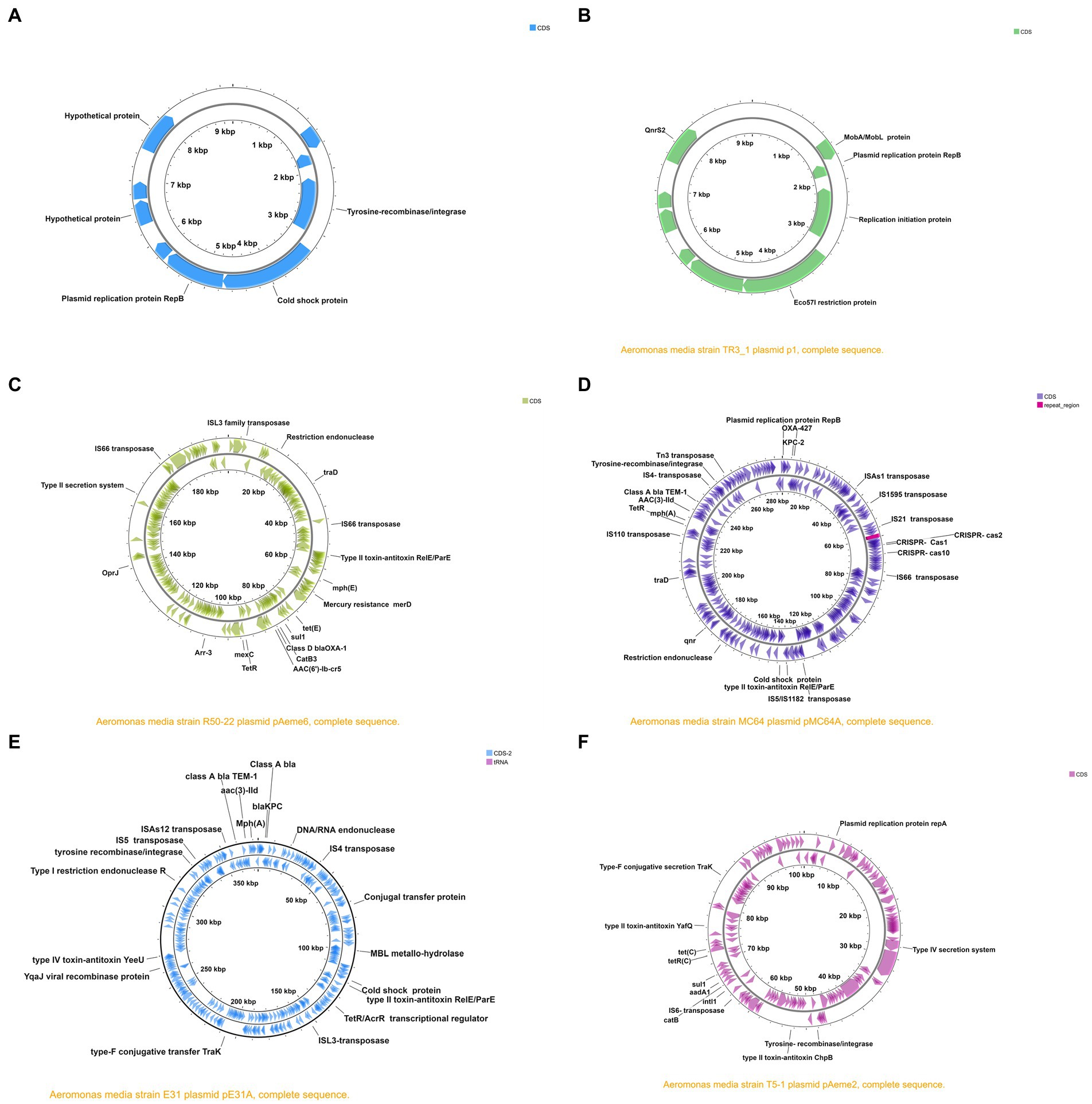
Figure 4. Circular maps of plasmids (i) pSD/21–15 isolated from Denmark (A), (ii) pTR3_1 waste water treatment from United States (B), (iii) pR50_22 from a sludge in China (C), (iv) pMC64 from a hospital in China (D), (v) pE31 from water in China (E), and (vi) pT5-1 from a sludge in China (F). Note that the location of virulence factors, antimicrobial resistance (AMR) genes, transposases, efflux pumps, secretion system, and other genes are shown in the circular map for each the plasmid.
Discussion
In this study, we have shown that strain SD/21–15 has several virulence and AMR genes similar to those found in other Aeromonas spp. Although we did not find whole genome sequences of A. media from Africa, Central, and South America in the public databanks, the 25 strains used in this study show a wide geographical distribution covering North America, Europe, and Asia. The absence of whole genome sequences of A. media from Africa and South America in the NCBI database is unknown whether this is due to lack of studies or resources for WGS of A. media in these continents. In terms of host distribution, the 25 isolates used covered a wide range of hosts from humans, fish, and chickens while environmental samples were from rivers, sludge, water treatment facility, hospital, biofilm reactors, and marine environments. As for the time span covered, the isolates used covered the period 2013–2022, with the exception of strain SD/21–15 isolated in 1992, because we did not find whole genome sequences of A. media deposited in the NCBI database prior to 2013. Note that strain SD/21–15 was initially classified as Aeromonas spp. using morphological, motility, and biochemical tests in 1992 (Andersen and Sandaa, 1994) but the WGS carried out in the present study classified the isolate as A. media. Thus, it is likely that several other isolates previously classified as Aeromonas spp. using morphological and biochemical tests could classified as A. media using WGS. Nonetheless, our comparison of AMR and virulence genes for A. media in the present study is based on a collection of genomes from a broad geographical distribution and wide host species using recent data. The similarity of AMR genes detected in strain SD/21–15 from marine sediments isolated in 1992 with recent isolates covering the period 2013 to 2022 is suggestive that A. media could be a hidden environmental risk carrying several intrinsic AMR genes as a source of transmission to other bacteria. The diverse host range and environmental source is suggestive that A. media, like other aeromonads, bridges the gap between the environment, aquaculture, animals and humans in the transmission of AMR genes.
The adherence of bacteria to host cells using pili and flagella is a crucial pathogenicity step in early stages of bacterial infection. The presence of genes that form Msh pili, tap type IV pili, and polar flagella proteins in all 25 isolates is suggestive that these proteins could be important for the adherence of A. media to host cells. This finding shows that A. media shares similar adherence proteins with other Aeromonas spp. where these proteins are used for intestinal adherence, colonization and biofilm formation (Canals et al., 2006; Hadi et al., 2012). However, only three isolates had the lateral flagella genes suggesting that this protein might not be obligatory for the adherence and biofilm formation in A. media. Other genes detected include luxS needed for biofilm formation and quorum sensing (Kozlova et al., 2008) and mshQ required for mannose-sensitive hemagglutinin pilus biosynthesis (Qin et al., 2014). Thus, detection of luxS and mshQ in all 25 isolates is suggestive that these proteins could be vital for biofilm and quorum sensing in A. media.
Previous studies reported presence of four (T2SS, T3SS, T4SS, and T6SS) secretory systems in Aeromonas spp. out of the six characterized in Gram negative bacteria (Beaz-Hidalgo and Figueras, 2013). However, only the T2SS and T6SS were detected in the A. media isolates examined in this study. Detection of all T2SS genes in all isolates may indicate that it might be required for A. media virulence. In other Aeromonas spp., T2SS has been linked with the presence of various proteins such as amylases, DNases, proteases, and the aerolysin-related cytotoxic enterotoxin Act shown to cause diarrhea (Xu et al., 1998; Sandkvist, 2001; Sha et al., 2002; Galindo et al., 2004). On the other hand, the T6SS uses the glycine repeat G (VrgG) and hemolysin-coregulated (Hcp) genes as part of the pore-forming protein to inject toxins into host cells (Bingle et al., 2008). Our findings show that only 3/25 isolates had the genes for all four proteins (VgR1, VgrG1, VgrG3, and Hcp) characterized to be crucial for T6SS virulence in Aeromonas spp. (Suarez et al., 2008, 2010). Interestingly, Rasmussen-Ivey et al. (2016) pointed out that T6SS is not obligatory for Aeromonas virulence as shown that not all hypervirulent A. hydrophila strains causing diseases in fish possess the T6SS. Similarly, it is likely that T6SS is not obligatory for the virulence of A. media given that most isolates used in this study did not have all T6SS genes. However, there is need for in vivo studies to validate these observations.
We detected three hemolysin genes namely hlyA, hlyIII, and TSH in all 25 isolates suggesting that these genes might be important for A. media virulence. Previous studies have shown that hlyA, hlyIII, and TH are pore forming cytotoxic enterotoxins found in different bacteria species including Aeromonas spp. that cause membrane damage and fluid accumulation in host cells leading to diarrhea (Agger et al., 1985; Kozaki et al., 1987; Honda et al., 1992; Baida and Kuzmin, 1996; Stanley et al., 1998; Chopra and Houston, 1999; Abrami et al., 2000; DelVecchio et al., 2002; Wang et al., 2003; Maté et al., 2014; Abdel-Fattah et al., 2017). Thus, it is likely that the diarrhea reported in humans infected by A. media might be caused by the hemolysin genes. However, we did not find the aerA gene in all 25 A. media isolates and yet it was present in other Aeromonas spp. examined (Hirono and Aoki, 1991; Wang et al., 2003). Wong et al. (1998) and Heuzenroeder et al. (1999) showed that a combination of the hlyA(+)aerA(+) double mutant significantly reduced the virulence of A. hydrophila in mice. They observed that cytotoxicity to buffalo green monkey kidney cells and hemolysis on horse blood agar were eliminated only in the double and not in the single mutants of A. hydrophila, A. veronii, and A. caviae. They also showed that only the double mutant eliminated the β-hemolysis on horse blood agar and cytotoxic activities on buffalo green monkey and Vero cells. Inactivation of the double mutant completely attenuated the virulence of A. hydrophila in mice (Heuzenroeder et al., 1999). In this study, all A. media isolates only had hlyA but not aerA. So, it is unknown whether the absence of aerA renders A. media isolates less pathogenic than other Aeromonas spp. that have the hlyA(+)-aerA(+) combination.
Iron is a vital cofactor used for various metabolic processes for the survival of bacteria in infected hosts (Ratledge and Dover, 2000; Wandersman and Delepelaire, 2004; Maltz et al., 2015). Thus, different bacteria species have devised various mechanisms for getting iron from their hosts (Byers, 1987; Calderwood and Mekalanos, 1987; Litwin and Calderwood, 1993; Morton et al., 2007, 2009). So, the uptake of iron from host cells is considered a virulence factor because of the damage impacted on the host due to iron deprivation. Common molecules used by Gram negative bacteria for iron uptake include the ferric uptake regulator (fur), siderophores, and heme (Barghouthi et al., 1989a,b; Litwin and Calderwood, 1993; Morton et al., 2007). Ebanks et al. (2013) showed that fur knockout mutants reduced the pathogenicity of A. salmonicida while Najimi et al. (2008a) showed that mutation in the hemin-binding protein caused a drastic reduction in the pathogenicity of A. salmonicida due to reduced heme uptake as a source of iron. In another study, Najimi et al. (2008b) showed that mutations in genes used for catecholate siderophore production reduced the pathogenicity of A. salmonicida. Thus, the detection of the genes encoding fur, siderophore, and heme in all 25 isolates is suggestive that these genes could be crucial for iron acquisition in A. media being similar to observations seen in other Aeromonas spp. (Byers, 1987; Najimi et al., 2008b; Ebanks et al., 2013).
A recent study by Ebmeyer et al. (2019) reported Aeromonas spp. as the origin of several clinically significant β-lactamases such as the CMY-1/MOX-family that include blaAmpC, blaMOX-1, blaMOX-2, and blaMOX-9. Thus, the detection of blaMOX-9 in all 25 isolates from different host species and geographical areas in the world corroborates with Ebmeyer (2021) who reported A. media as the origin of blaMOX-9. In 2017, Bogaerts et al. (2017) reported that blaOXA-427 from Enterobacteriaceae was closely related to isolates from A. media, A. hydrophila, and A. sobria as a novel emerging carbapenem-hydrolysing class D β-lactamase (CHDL) from patients in a Belgian hospital. They showed that blaOXA-427 hydrolyzed imipenem and conferred resistance to extended-spectrum cephalosporins, penicillin and carbapenems when expressed in Escherichia coli. Its presence in all 25 isolates emanating from North America, Europe, and Asia is suggestive that blaOXA-427 could be highly prevalent in A. media strains across the world posing the danger of being the source of blaOXA-427 transmission to humans and animals. Its higher presence among Aeromonas spp. than other bacterial species, support observations made by Bogaerts et al. (2017) who pointed out that CHDLs are restricted to a few bacterial genera. Detection of CRP and MCR genes in all 25 A. media shows that the presence of these genes in A. media extends across several continents while the presence of blaKPC-1 in A. media isolates is a significant finding given that infections caused by blaKPC-1 producing bacteria are extremely difficult to treat because of their multidrug resistance linked to high mortalities in humans (Sacha et al., 2009). The presence of MexB in all isolates is suggestive that this efflux pump could be important for transportation of genes like blaMOX-9, blaOXA-427, crp, and mcr genes found in all A. media isolates.
Although the plasmid of strain SD/21–15 had no AMR-genes in its genome, other A. media isolates had plasmids having various AMR genes that included blaKPC-1, blaOXA-427, sul1, blaOXA-1, and qnr genes. In additions, the detected plasmids had several transposases, such as Tn3, ISAs1, IS1595, and IS4 known to carry various AMR-gene cassettes (Dziewit et al., 2012; Baquero et al., 2013; Carvalho et al., 2021). The plasmids also encoded various efflux pump proteins, such as tet(E), merD, mexC, OprJ, mph(E), (Chopra, 2002), and mph(A) known to play significant roles in drug trafficking across cell membranes (Dayao et al., 2016; Kim et al., 2017; Yang et al., 2021). The presence of type II toxin-antitoxin RelEParE and T2SS is indicative that the plasmids also carry virulence genes. The presence of proteins such as the conjugal transfer protein TraF points to the presence of proteins that facilitate gene transfer between bacteria species. Other researchers (Majumdar et al., 2006; Preena et al., 2021) have noted that plasmids of aeromonads can be cured after sub-culturing, and depending on the history of the isolates after primary isolation, we may not have sequenced all plasmids of the original isolates in this study. Altogether, these observations show that A. media strains isolated from different geographical and host species in the world carry various multidrug efflux pump proteins, transposons, AMR, and virulence genes. However, this is need for in vivo studies using approaches such as mutagenesis, cloning, and purification of virulent genes identified in this study in order to determine their virulence mechanisms in different host species. Such studies would shed more insight on genes that are crucial for the pathogenicity of A. media.
Conclusion
In this study, we have shown that A. media strain SD/21–15 isolated from marine sediments in Denmark shares several virulence genes such as adherence proteins, hemolysins, secretion system, iron acquisition, biofilm formation and quorum sensing genes with other A. media strains isolated from different host species and geographical areas in the world. We have also shown that strain SD/21–15 shares several genes like hemolysins, adherence proteins, and T2SS with other Aeromonas spp. although it lacks the cytotoxic aerA gene. The presence of blaMOX-9, blaOXA-427, crp, and mcr genes in all 25 isolates is indicative that these AMR genes are highly prevalent in A. media isolates found in different ecosystems. The presence of transposases, integrase, recombinases, virulence, and AMR genes in the plasmids is indicative that the A. media strains examined in this study had the potential to transmit virulence and AMR genes to other bacteria. In summary, our findings shed new insights on virulence genes and the role of A. media in the spread of AMR genes.
Data availability statement
The datasets presented in this study can be found in online repositories. The link to the repository can be found below: https://www.ncbi.nlm.nih.gov/nuccore/JAJVCY000000000.1.
Author contributions
SD, HS, and HM: conceptualization, methodology, supervision, data curation, bioinformatics analysis, and mobilizing resources. SD, EA-W, BP, ØE, HS, and HM: manuscript preparation, editing, and submission. All authors contributed to the article and approved the submitted version.
Funding
This study was financed by the Research Council of Norway under the FIFOSA-21 Project Grant Number 320692.
Acknowledgments
The authors are thankful to Erik Hjerde based at the Arctic university of Norway and ELIXIR Norway for guidance on Bioinformatics analysis, and also thankful to Aud Kari Fauske, Sofie Persdatter Sangnæs and Solveig B. Wiig based at the Norwegian University of Life Sciences (NMBU) for technical support. We also thank researchers Simen F. Nørstebø, PhD scholar Lisa M. Ånestad and Eiril Soltvedt for laboratory assistance.
Conflict of interest
The authors declare that the research was conducted in the absence of any commercial or financial relationships that could be construed as a potential conflict of interest.
Publisher’s note
All claims expressed in this article are solely those of the authors and do not necessarily represent those of their affiliated organizations, or those of the publisher, the editors and the reviewers. Any product that may be evaluated in this article, or claim that may be made by its manufacturer, is not guaranteed or endorsed by the publisher.
Supplementary material
The Supplementary material for this article can be found online at: https://www.frontiersin.org/articles/10.3389/fmicb.2022.1022639/full#supplementary-material
Footnotes
References
Abdel-Fattah, G. M., Hafez, E. E., Zaki, M. E., and Darwesh, N. M. (2017). Cloning and expression of alpha hemolysin toxin gene of Staphylococcus aureus against human cancer tissue. Int. J. Appl. Sci. Biotechnol. 5, 22–29. doi: 10.3126/ijasbt.v5i1.17000
Abrami, L., Fivaz, M., and Van Der Goot, F. G. (2000). Adventures of a pore-forming toxin at the target cell surface. Trends Microbiol. 8, 168–172. doi: 10.1016/S0966-842X(00)01722-4
Agger, W. A., McCormick, J., and Gurwith, M. J. (1985). Clinical and microbiological features of Aeromonas hydrophila-associated diarrhea. J. Clin. Microbiol. 21, 909–913. doi: 10.1128/jcm.21.6.909-913.1985
Alcock, B. P., Raphenya, A. R., Lau, T. T., Tsang, K. K., Bouchard, M., Edalatmand, A., et al. (2020). Nguyen A-LV, Cheng AA, Liu S: CARD 2020: antibiotic resistome surveillance with the comprehensive antibiotic resistance database. Nucleic Acids Res. 48, D517–D525. doi: 10.1093/nar/gkz935
Allen, D., Austin, B., and Colwell, R. (1983). Aeromonas media, a new species isolated from river water. Int. J. Syst. Evol. Microbiol. 33, 599–604.
Andersen, S. R., and Sandaa, R.-A. (1994). Distribution of tetracycline resistance determinants among gram-negative bacteria isolated from polluted and unpolluted marine sediments. Appl. Environ. Microbiol. 60, 908–912. doi: 10.1128/aem.60.3.908-912.1994
Baida, G. E., and Kuzmin, N. P. (1996). Mechanism of action of hemolysin III from Bacillus cereus. Biochim. Biophys. Acta 1284, 122–124. doi: 10.1016/S0005-2736(96)00168-X
Bankevich, A., Nurk, S., Antipov, D., Gurevich, A. A., Dvorkin, M., Kulikov, A. S., et al. (2012). SPAdes: a new genome assembly algorithm and its applications to single-cell sequencing. J. Comput. Biol. 19, 455–477. doi: 10.1089/cmb.2012.0021
Baquero, F., Tedim, A. P., and Coque, T. M. (2013). Antibiotic resistance shaping multi-level population biology of bacteria. Front. Microbiol. 4:15.
Barghouthi, S., Young, R., Arceneaux, J., and Byers, B. (1989a). Physiological control of amonabactin biosynthesis inAeromonas hydrophila. Biol. Met. 2, 155–160. doi: 10.1007/BF01142554
Barghouthi, S., Young, R., Olson, M., Arceneaux, J., Clem, L., and Byers, B. (1989b). Amonabactin, a novel tryptophan-or phenylalanine-containing phenolate siderophore in Aeromonas hydrophila. J. Bacteriol. 171, 1811–1816. doi: 10.1128/jb.171.4.1811-1816.1989
Beaz-Hidalgo, R., and Figueras, M. (2013). A eromonas spp. whole genomes and virulence factors implicated in fish disease. J. Fish Dis. 36, 371–388. doi: 10.1111/jfd.12025
Becker, L., Steglich, M., Fuchs, S., Werner, G., and Nübel, U. (2016). Comparison of six commercial kits to extract bacterial chromosome and plasmid DNA for MiSeq sequencing. Sci. Rep. 6, 1–5.
Bhowmick, U. D., and Bhattacharjee, S. (2018). Bacteriological, clinical and virulence aspects of Aeromonas-associated diseases in humans. Pol. J. Microbiol. 67, 137–150. doi: 10.21307/pjm-2018-020
Bingle, L. E., Bailey, C. M., and Pallen, M. J. (2008). Type VI secretion: a beginner's guide. Curr. Opin. Microbiol. 11, 3–8. doi: 10.1016/j.mib.2008.01.006
Bioinformatics (2011). FastQC: A Quality Control Tool for High Throughput Sequence Data. Cambridge, UK: Babraham Institute
Bogaerts, P., Naas, T., Saegeman, V., Bonnin, R. A., Schuermans, A., Evrard, S., et al. (2017). OXA-427, a new plasmid-borne carbapenem-hydrolysing class D β-lactamase in Enterobacteriaceae. J. Antimicrob. Chemother. 72, 2469–2477. doi: 10.1093/jac/dkx184
Bolger, A. M., Lohse, M., and Usadel, B. (2014). Trimmomatic: a flexible trimmer for Illumina sequence data. Bioinformatics 30, 2114–2120. doi: 10.1093/bioinformatics/btu170
Byers, B. R. (1987). “Iron, siderophores, and virulence in Aeromonas hydrophila,” in Iron Transport in Microbes, Plants and Animals. eds. B. H. Igewski and V. L. Clark (Academic Press, Inc.), 111–116.
Calderwood, S. B., and Mekalanos, J. J. (1987). Iron regulation of Shiga-like toxin expression in Escherichia coli is mediated by the fur locus. J. Bacteriol. 169, 4759–4764. doi: 10.1128/jb.169.10.4759-4764.1987
Canals, R., Altarriba, M., Vilches, S., Horsburgh, G., Shaw, J. G., Tomás, J. M., et al. (2006). Analysis of the lateral flagellar gene system of Aeromonas hydrophila AH-3. J. Bacteriol. 188, 852–862. doi: 10.1128/JB.188.3.852-862.2006
Carvalho, R., Aburjaile, F., Canario, M., Nascimento, A. M., Chartone-Souza, E., De Jesus, L., et al. (2021). Genomic characterization of multidrug-resistant Escherichia coli BH100 sub-strains. Front. Microbiol. 11:549254. doi: 10.3389/fmicb.2020.549254
Chen, L., Yang, J., Yu, J., Yao, Z., Sun, L., Shen, Y., et al. (2005). VFDB: a reference database for bacterial virulence factors. Nucleic Acids Res. 33, D325–D328. doi: 10.1093/nar/gki008
Chopra, I. (2002). New developments in tetracycline antibiotics: glycylcyclines and tetracycline efflux pump inhibitors. Drug Resist. Updat. 5, 119–125. doi: 10.1016/S1368-7646(02)00051-1
Chopra, A. K., and Houston, C. W. (1999). Enterotoxins in Aeromonas-associated gastroenteritis. Microbes Infect. 1, 1129–1137. doi: 10.1016/S1286-4579(99)00202-6
Cockerill, F. R., Wikler, M., Bush, K., Dudley, M., Eliopoulos, G., and Hardy, D. (2012). Clinical and laboratory standards institute. Performance standards for antimicrobial susceptibility testing: twenty-second informational supplement
Coil, D., Jospin, G., and Darling, A. E. (2015). A5-miseq: an updated pipeline to assemble microbial genomes from Illumina MiSeq data. Bioinformatics 31, 587–589. doi: 10.1093/bioinformatics/btu661
Dahanayake, P., Hossain, S., Wickramanayake, M., and Heo, G. J. (2019). Antibiotic and heavy metal resistance genes in Aeromonas spp. isolated from marketed Manila clam (Ruditapes philippinarum) in Korea. J. Appl. Microbiol. 127, 941–952. doi: 10.1111/jam.14355
Dahanayake, P., Hossain, S., Wickramanayake, M., and Heo, G. J. (2020). Prevalence of virulence and antimicrobial resistance genes in Aeromonas species isolated from marketed cockles (Tegillarca granosa) in Korea. Lett. Appl. Microbiol. 71, 94–101. doi: 10.1111/lam.13261
Dayao, D., Gibson, J., Blackall, P., and Turni, C. (2016). Antimicrobial resistance genes in Actinobacillus pleuropneumoniae, Haemophilus parasuis and Pasteurella multocida isolated from Australian pigs. Aust. Vet. J. 94, 227–231. doi: 10.1111/avj.12458
De Silva, B., Hossain, S., Dahanayake, P., and Heo, G. J. (2019). Aeromonas spp. from marketed yesso scallop (Patinopecten yessoensis): molecular characterization, phylogenetic analysis, virulence properties and antimicrobial susceptibility. J. Appl. Microbiol. 126, 288–299. doi: 10.1111/jam.14106
De Silva, B., Hossain, S., Wimalasena, S., Pathirana, H., and Heo, G. J. (2018). Putative virulence traits and antibiogram profile of Aeromonas spp. isolated from frozen white-leg shrimp (Litopenaeus vannamei) marketed in Korea. J. Food Saf. 38:e12470. doi: 10.1111/jfs.12470
DelVecchio, V. G., Kapatral, V., Redkar, R. J., Patra, G., Mujer, C., Los, T., et al. (2002). The genome sequence of the facultative intracellular pathogen Brucella melitensis. Proc. Natl. Acad. Sci. 99, 443–448. doi: 10.1073/pnas.221575398
Dziewit, L., Baj, J., Szuplewska, M., Maj, A., Tabin, M., Czyzkowska, A., et al. (2012). Insights into the transposable mobilome of Paracoccus spp.(Alphaproteobacteria). PLoS One 7:e32277. doi: 10.1371/journal.pone.0032277
Ebanks, R. O., Goguen, M., Knickle, L., Dacanay, A., Leslie, A., Ross, N. W., et al. (2013). Analysis of a ferric uptake regulator (fur) knockout mutant in Aeromonas salmonicida subsp. salmonicida. Vet. Microbiol. 162, 831–841. doi: 10.1016/j.vetmic.2012.10.038
Ebmeyer, S., Kristiansson, E., and Larsson, D. J. (2019). CMY-1/MOX-family AmpC β-lactamases MOX-1, MOX-2 and MOX-9 were mobilized independently from three aeromonas species. J. Antimicrob. Chemother. 74, 1202–1206. doi: 10.1093/jac/dkz025
Evangelista-Barreto, N. S., Vieira, R. H., Carvalho, F. C. T., Torres, R. C., Sant'Anna, E. S., Rodrigues, D. P., et al. (2006). Aeromonas spp. isolated from oysters (Crassostrea rhizophorea) from a natural oyster bed, Ceará, Brazil. Rev. Inst. Med. Trop. Sao Paulo 48, 129–133. doi: 10.1590/S0036-46652006000300003
Figueira, V., Vaz-Moreira, I., Silva, M., and Manaia, C. M. (2011). Diversity and antibiotic resistance of Aeromonas spp. in drinking and waste water treatment plants. Water Res. 45, 5599–5611. doi: 10.1016/j.watres.2011.08.021
Fontes, M., Saavedra, M., Martins, C., and Martínez-Murcia, A. (2011). Phylogenetic identification of Aeromonas from pigs slaughtered for consumption in slaughterhouses at the north of Portugal. Int. J. Food Microbiol. 146, 118–122. doi: 10.1016/j.ijfoodmicro.2011.02.010
Gaio, D, Anantanawat, K, To, J, Liu, M, Monahan, L, and Darling, A.E. (2021). Hackflex: low cost Illumina Nextera flex sequencing library construction. BioRxiv [Preprint]. doi: 10.1099/mgen.0.000744
Galindo, C. L., Fadl, A. A., Sha, J., Gutierrez, C., Popov, V. L., Boldogh, I., et al. (2004). Aeromonas hydrophila cytotoxic enterotoxin activates mitogen-activated protein kinases and induces apoptosis in murine macrophages and human intestinal epithelial cells. J. Biol. Chem. 279, 37597–37612. doi: 10.1074/jbc.M404641200
Gauthier, J., Vincent, A. T., Charette, S. J., and Derome, N. (2017). Strong genomic and phenotypic heterogeneity in the Aeromonas sobria species complex. Front. Microbiol. 8:2434. doi: 10.3389/fmicb.2017.02434
Guan, G., He, X., Chen, J., Bin, L., and Tang, X. (2020). Identifying the mechanisms underlying the protective effect of tetramethylpyrazine against cisplatin-induced in vitro ototoxicity in HEI-OC1 auditory cells using gene expression profiling. Mol. Med. Rep. 22, 5053–5068. doi: 10.3892/mmr.2020.11631
Guerra, I. M., Fadanelli, R., Figueiró, M., Schreiner, F., Delamare, A. P. L., Wollheim, C., et al. (2007). Aeromonas associated diarrhoeal disease in South Brazil: prevalence, virulence factors and antimicrobial resistance. Braz. J. Microbiol. 38, 638–643. doi: 10.1590/S1517-83822007000400011
Hadi, N., Yang, Q., Barnett, T. C., Tabei, S. M. B., Kirov, S. M., and Shaw, J. G. (2012). Bundle-forming pilus locus of Aeromonas veronii bv. Infect. Immun. 80, 1351–1360. doi: 10.1128/IAI.06304-11
Heuzenroeder, M. W., Wong, C. Y., and Flower, R. L. (1999). Distribution of two hemolytic toxin genes in clinical and environmental isolates of Aeromonas spp.: correlation with virulence in a suckling mouse model. FEMS Microbiol. Lett. 174, 131–136. doi: 10.1111/j.1574-6968.1999.tb13559.x
Hirono, I., and Aoki, T. (1991). Nucleotide sequence and expression of an extracellular hemolysin gene of Aeromonas hydrophila. Microb. Pathog. 11, 189–197. doi: 10.1016/0882-4010(91)90049-G
Hoel, S., Vadstein, O., and Jakobsen, A. N. (2017). Species distribution and prevalence of putative virulence factors in mesophilic Aeromonas spp. isolated from fresh retail sushi. Front. Microbiol. 8:931. doi: 10.3389/fmicb.2017.00931
Honda, T., Ni, Y., Miwatani, T., Adachi, T., and Kim, J. (1992). The thermostable direct hemolysin of Vibrio parahaemolyticus is a pore-forming toxin. Can. J. Microbiol. 38, 1175–1180. doi: 10.1139/m92-192
Hu, M., Wang, N., Pan, Z., Lu, C., and Liu, Y. (2012). Identity and virulence properties of Aeromonas isolates from diseased fish, healthy controls and water environment in China. Lett. Appl. Microbiol. 55, 224–233. doi: 10.1111/j.1472-765X.2012.03281.x
Jain, C., Rodriguez-R, L. M., Phillippy, A. M., Konstantinidis, K. T., and Aluru, S. (2018). High throughput ANI analysis of 90K prokaryotic genomes reveals clear species boundaries. Nat. Commun. 9, 1–8. doi: 10.1038/s41467-018-07641-9
Joseph, N. M., Sistla, S., Dutta, T. K., Badhe, A. S., Rasitha, D., and Parija, S. C. (2011). Reliability of Kirby-Bauer disk diffusion method for detecting meropenem resistance among non-fermenting gram-negative bacilli. Indian J. Pathol. Microbiol. 54, 556–560. doi: 10.4103/0377-4929.85092
Kahlmeter, G., Brown, D., Goldstein, F., MacGowan, A., Mouton, J., Odenholt, I., et al. (2006). European Committee on Antimicrobial Susceptibility Testing (EUCAST) Technical Notes on Antimicrobial Susceptibility Testing, vol. 12. Switzerland: Wiley Online Library, 501–503.
Kaspersen, H., Fiskebeck, E. Z., Sekse, C., Slettemeås, J. S., Urdahl, A. M., Norström, M., et al. (2020). Comparative genome analyses of wild type-and quinolone resistant Escherichia coli indicate dissemination of QREC in the Norwegian broiler breeding pyramid. Front. Microbiol. 11:938. doi: 10.3389/fmicb.2020.00938
Kim, J., Shin, B., Park, C., and Park, W. (2017). Indole-induced activities of β-lactamase and efflux pump confer ampicillin resistance in pseudomonas putida KT2440. Front. Microbiol. 8:433. doi: 10.3389/fmicb.2017.00433
Kozaki, S., Kurokawa, A., Asao, T., Kato, K., Uemura, T., and Sakaguchi, G. (1987). Enzyme-linked immunosorbent assay for Aeromonas hydrophila hemolysins. FEMS Microbiol. Lett. 41, 147–151. doi: 10.1111/j.1574-6968.1987.tb02186.x
Kozlova, E. V., Popov, V. L., Sha, J., Foltz, S. M., Erova, T. E., Agar, S. L., et al. (2008). Mutation in the S-ribosylhomocysteinase (luxS) gene involved in quorum sensing affects biofilm formation and virulence in a clinical isolate of Aeromonas hydrophila. Microb. Pathog. 45, 343–354. doi: 10.1016/j.micpath.2008.08.007
Kuncham, R., Sivaprakasam, T., Kumar, R. P., Sreenath, P., Nayak, R., Thayumanavan, T., et al. (2017). Bacterial fauna associating with chironomid larvae from lakes of Bengaluru city, India-a 16S rRNA gene based identification. Genomics Data 12, 44–48. doi: 10.1016/j.gdata.2017.03.001
Lee, H. J., Hoel, S., Lunestad, B. T., Lerfall, J., and Jakobsen, A. (2021). Aeromonas spp. isolated from ready-to-eat seafood on the Norwegian market: prevalence, putative virulence factors and antimicrobial resistance. J. Appl. Microbiol. 130, 1380–1393. doi: 10.1111/jam.14865
Litwin, C. M., and Calderwood, S. (1993). Role of iron in regulation of virulence genes. Clin. Microbiol. Rev. 6, 137–149. doi: 10.1128/CMR.6.2.137
Lü, A., Hu, X., Li, L., Sun, J., Song, Y., Pei, C., et al. (2016). Isolation, identification and antimicrobial susceptibility of pathogenic Aeromonas media isolated from diseased koi carp (Cyprinus carpio koi). Iran. J. Fish. Sci. 15, 760–774.
Majumdar, T., Ghosh, S., Pal, J., and Mazumder, S. (2006). Possible role of a plasmid in the pathogenesis of a fish disease caused by Aeromonas hydrophila. Aquaculture 256, 95–104. doi: 10.1016/j.aquaculture.2006.02.042
Maltz, M., Levarge, B. L., and Graf, J. (2015). Identification of iron and heme utilization genes in Aeromonas and their role in the colonization of the leech digestive tract. Front. Microbiol. 6:763. doi: 10.3389/fmicb.2015.00763
Maté, S. M., Vázquez, R. F., Herlax, V. S., Millone, M. A. D., Fanani, M. L., Maggio, B., et al. (2014). Bakás LS: boundary region between coexisting lipid phases as initial binding sites for Escherichia coli alpha-hemolysin: a real-time study. Biochim. Biophys. Acta 1838, 1832–1841. doi: 10.1016/j.bbamem.2014.02.022
Morton, D. J., Seale, T. W., Bakaletz, L. O., Jurcisek, J. A., Smith, A., VanWagoner, T. M., et al. (2009). The heme-binding protein (HbpA) of Haemophilus influenzae as a virulence determinant. Int. J. Med. Microbiol. 299, 479–488. doi: 10.1016/j.ijmm.2009.03.004
Morton, D. J., Seale, T. W., Madore, L. L., VanWagoner, T. M., Whitby, P. W., and Stull, T. L. (2007). The haem–haemopexin utilization gene cluster (hxuCBA) as a virulence factor of Haemophilus influenzae. Microbiology 153, 215–224. doi: 10.1099/mic.0.2006/000190-0
Najimi, M., Lemos, M. L., and Osorio, C. R. (2008a). Identification of heme uptake genes in the fish pathogen Aeromonas salmonicida subsp. salmonicida. Arch. Microbiol. 190, 439–449. doi: 10.1007/s00203-008-0391-5
Najimi, M., Lemos, M. L., and Osorio, C. R. (2008b). Identification of siderophore biosynthesis genes essential for growth of Aeromonas salmonicida under iron limitation conditions. Appl. Environ. Microbiol. 74, 2341–2348. doi: 10.1128/AEM.02728-07
Pablos, M., Huys, G., Cnockaert, M., Rodríguez-Calleja, J., Otero, A., Santos, J., et al. (2011). Identification and epidemiological relationships of Aeromonas isolates from patients with diarrhea, drinking water and foods. Int. J. Food Microbiol. 147, 203–210. doi: 10.1016/j.ijfoodmicro.2011.04.006
Picão, R. C., Poirel, L., Demarta, A., Petrini, O., Corvaglia, A. R., and Nordmann, P. (2008). Expanded-spectrum β-lactamase PER-1 in an environmental Aeromonas media isolate from Switzerland. Antimicrob. Agents Chemother. 52, 3461–3462. doi: 10.1128/AAC.00770-08
Picao, R. C., Poirel, L., Demarta, A., Silva, C. S. F., Corvaglia, A. R., Petrini, O., et al. (2008). Plasmid-mediated quinolone resistance in Aeromonas allosaccharophila recovered from a Swiss lake. J. Antimicrob. Chemother. 62, 948–950. doi: 10.1093/jac/dkn341
Piotrowska, M., and Popowska, M. (2015). Insight into the mobilome of Aeromonas strains. Front. Microbiol. 6:494. doi: 10.3389/fmicb.2015.00494
Preena, P. G., Dharmaratnam, A., Rejish Kumar, J. V., and Swaminathan, T. R. (2021). Plasmid-mediated antimicrobial resistance in motile aeromonads from diseased Nile tilapia (Oreochromis niloticus). Aquac. Res. 52, 237–248. doi: 10.1111/are.14886
Qin, Y., Yan, Q., Mao, X., Chen, Z., and Su, Y. (2014). Role of MshQ in MSHA pili biosynthesis and biofilm formation of Aeromonas hydrophila. Genet. Mol. Res. 13, 8982–8996. doi: 10.4238/2014.October.31.13
Rasmussen-Ivey, C. R., Figueras, M. J., McGarey, D., and Liles, M. R. (2016). Virulence factors of Aeromonas hydrophila: in the wake of reclassification. Front. Microbiol. 7:1337. doi: 10.3389/fmicb.2016.01337
Rasmussen-Ivey, C. R., Hossain, M. J., Odom, S. E., Terhune, J. S., Hemstreet, W. G., Shoemaker, C. A., et al. (2016). Classification of a hypervirulent Aeromonas hydrophila pathotype responsible for epidemic outbreaks in warm-water fishes. Front. Microbiol. 7:1615. doi: 10.3389/fmicb.2016.01615
Ratledge, C., and Dover, L. G. (2000). Iron metabolism in pathogenic bacteria. Annu. Rev. Microbiol. 54, 881–941. doi: 10.1146/annurev.micro.54.1.881
Roger, F., Lamy, B., Jumas-Bilak, E., Kodjo, A., and Marchandin, H. (2012). Ribosomal multi-operon diversity: An original perspective on the genus Aeromonas.
Romero, A., Saraceni, P. R., Merino, S., Figueras, A., Tomás, J. M., and Novoa, B. (2016). The animal model determines the results of Aeromonas virulence factors. Front. Microbiol. 7:1574. doi: 10.3389/fmicb.2016.01574
Sacha, P., Ostas, A., Jaworowska, J., Wieczorek, P., Ojdana, D., Ratajczak, J., et al. (2009). The KPC type beta-lactamases: new enzymes that confer resistance to carbapenems in gram-negative bacilli. Folia Histochem. Cytobiol. 47, 537–543. doi: 10.2478/v10042-009-0079-y
Saffari, N., Salmanzadeh-Ahrabi, S., Abdi-Ali, A., and Rezaei-Hemami, M. (2016). A comparison of antibiotic disks from different sources on Quicolor and Mueller-Hinton agar media in evaluation of antibacterial susceptibility testing. Iran. J. Microbiol. 8, 307–311.
Sandkvist, M. (2001). Type II secretion and pathogenesis. Infect. Immun. 69, 3523–3535. doi: 10.1128/IAI.69.6.3523-3535.2001
Seemann, T. (2014). Prokka: rapid prokaryotic genome annotation. Bioinformatics 30, 2068–2069. doi: 10.1093/bioinformatics/btu153
Sha, J., Kozlova, E., and Chopra, A. (2002). Role of various enterotoxins in Aeromonas hydrophila-induced gastroenteritis: generation of enterotoxin gene-deficient mutants and evaluation of their enterotoxic activity. Infect. Immun. 70, 1924–1935. doi: 10.1128/IAI.70.4.1924-1935.2002
Shao, L., Tian, Y., Chen, S., Xu, X., and Wang, H. (2022). Characterization of the spoilage heterogeneity of Aeromonas isolated from chilled chicken meat: in vitro and in situ. LWT 162:113470. doi: 10.1016/j.lwt.2022.113470
Shen, Y., Xu, C., Sun, Q., Schwarz, S., Ou, Y., Yang, L., et al. (2018). Prevalence and genetic analysis of mcr-3-positive Aeromonas species from humans, retail meat, and environmental water samples. Antimicrob. Agents Chemother. 62, e00404–e00418. doi: 10.1128/AAC.00404-18
Singh, D. (2000). A putative heat-labile enterotoxin expressed by strains of Aeromonas media. J. Med. Microbiol. 49, 685–689. doi: 10.1099/0022-1317-49-8-685
Singhal, N., Kumar, M., Kanaujia, P. K., and Virdi, J. S. (2015). MALDI-TOF mass spectrometry: an emerging technology for microbial identification and diagnosis. Front. Microbiol. 6:791. doi: 10.3389/fmicb.2015.00791
Stanley, P., Koronakis, V., and Hughes, C. (1998). Acylation of Escherichia coli hemolysin: a unique protein lipidation mechanism underlying toxin function. Microbiol. Mol. Biol. Rev. 62, 309–333. doi: 10.1128/MMBR.62.2.309-333.1998
Stratev, D., and Odeyemi, O. A. (2016). Antimicrobial resistance of Aeromonas hydrophila isolated from different food sources: a mini-review. J. Infect. Public Health 9, 535–544. doi: 10.1016/j.jiph.2015.10.006
Suarez, G., Sierra, J., Erova, T., Sha, J., Horneman, A., and Chopra, A. (2010). A type VI secretion system effector protein, VgrG1, from Aeromonas hydrophila that induces host cell toxicity by ADP ribosylation of actin. J. Bacteriol. 192, 155–168. doi: 10.1128/JB.01260-09
Suarez, G., Sierra, J. C., Sha, J., Wang, S., Erova, T. E., Fadl, A. A., et al. (2008). Molecular characterization of a functional type VI secretion system from a clinical isolate of Aeromonas hydrophila. Microb. Pathog. 44, 344–361. doi: 10.1016/j.micpath.2007.10.005
Talagrand-Reboul, E., Roger, F., Kimper, J.-L., Colston, S. M., Graf, J., Latif-Eugenín, F., et al. (2017). Delineation of taxonomic species within complex of species: Aeromonas media and related species as a test case. Front. Microbiol. 8:621. doi: 10.3389/fmicb.2017.00621
Tarumoto, N., Sakai, J., Sujino, K., Yamaguchi, T., Ohta, M., Yamagishi, J., et al. (2017). Use of the Oxford Nanopore MinION sequencer for MLST genotyping of vancomycin-resistant enterococci. J. Hosp. Infect. 96, 296–298. doi: 10.1016/j.jhin.2017.02.020
Tatusova, T., DiCuccio, M., Badretdin, A., Chetvernin, V., Nawrocki, E. P., Zaslavsky, L., et al. (2016). NCBI prokaryotic genome annotation pipeline. Nucleic Acids Res. 44, 6614–6624. doi: 10.1093/nar/gkw569
Teodoro, J. R., Carvalho, G. G., Queiroz, M. M., Levy, C. E., and Kabuki, D. Y. (2022). Incidence, evaluation of detection and identification methods, and antimicrobial resistance of Aeromonas spp. in ready-to-eat foods. Int. J. Food Microbiol. 379:109862. doi: 10.1016/j.ijfoodmicro.2022.109862
Tomás, J. (2012). The main Aeromonas pathogenic factors. International Scholarly Research Notices, 2012.
Tran, T. T., Scott, A., Tien, Y.-C., Murray, R., Boerlin, P., Pearl, D. L., et al. (2021). On-farm anaerobic digestion of dairy manure reduces the abundance of antibiotic resistance-associated gene targets, and the potential for plasmid transfer. Appl. Environ. Microbiol. 87, 02980–02920. doi: 10.1128/AEM.02980-20
Ullah, S. R., Majid, M., and Andleeb, S. (2020). Draft genome sequence of an extensively drug-resistant neonatal Klebsiella pneumoniae isolate harbouring multiple plasmids contributing to antibiotic resistance. J. Glob. Antimicrob. Resist. 23, 100–101. doi: 10.1016/j.jgar.2020.08.008
Wandersman, C., and Delepelaire, P. (2004). Bacterial iron sources: from siderophores to hemophores. Annu. Rev. Microbiol. 58, 611–647. doi: 10.1146/annurev.micro.58.030603.123811
Wang, G., Clark, C. G., Liu, C., Pucknell, C., Munro, C. K., Kruk, T. M., et al. (2003). Detection and characterization of the hemolysin genes in Aeromonas hydrophila and Aeromonas sobria by multiplex PCR. J. Clin. Microbiol. 41, 1048–1054. doi: 10.1128/JCM.41.3.1048-1054.2003
Wang, G.-y., Wang, H.-h., Han, Y.-w., Xing, T., Ye, K.-P., Xu, X.-I., et al. (2017). evaluation of the spoilage potential of bacteria isolated from chilled chicken in vitro and in situ. Food Microbiol. 63, 139–146. doi: 10.1016/j.fm.2016.11.015
Wong, C. Y., Heuzenroeder, M. W., and Flower, R. L. (1998). Inactivation of two haemolytic toxin genes in Aeromonas hydrophila attenuates virulence in a suckling mouse model. Microbiology 144, 291–298. doi: 10.1099/00221287-144-2-291
World Health Organization (2021). Antimicrobial resistance. Available at: https://www.who.int/news-room/fact-sheets/detail/antimicrobial-resistance
Xu, X.-J., Ferguson, M., Popov, V., Houston, C., Peterson, J., and Chopra, A. (1998). Role of a cytotoxic enterotoxin in Aeromonas-mediated infections: development of transposon and isogenic mutants. Infect. Immun. 66, 3501–3509. doi: 10.1128/IAI.66.8.3501-3509.1998
Yang, X., Ye, L., Chan, E. W.-C., Zhang, R., and Chen, S. (2021). Characterization of an IncFIB/IncHI1B plasmid encoding efflux pump TMexCD1-TOprJ1 in a clinical tigecycline-and carbapenem-resistant Klebsiella pneumoniae strain. Antimicrob. Agents Chemother. 65, e02340–e02420. doi: 10.1128/AAC.02340-20
Yi, S.-W., You, M.-J., Cho, H.-S., Lee, C.-S., Kwon, J.-K., and Shin, G.-W. (2013). Molecular characterization of Aeromonas species isolated from farmed eels (Anguilla japonica). Vet. Microbiol. 164, 195–200. doi: 10.1016/j.vetmic.2013.02.006
Keywords: Aeromonas media, antimicrobial resistance, virulence, plasmid, intrinsic–extrinsic, whole genome sequencing
Citation: Dubey S, Ager-Wick E, Peng B, Evensen Ø, Sørum H and Munang’andu HM (2022) Characterization of virulence and antimicrobial resistance genes of Aeromonas media strain SD/21–15 from marine sediments in comparison with other Aeromonas spp. Front. Microbiol. 13:1022639. doi: 10.3389/fmicb.2022.1022639
Edited by:
Zhi Ruan, Zhejiang University, ChinaReviewed by:
Chamara De Silva Benthotage, Southern Cross University, AustraliaLelia Chambel, University of Lisbon, Portugal
Copyright © 2022 Dubey, Ager-Wick, Peng, Evensen, Sørum and Munang’andu. This is an open-access article distributed under the terms of the Creative Commons Attribution License (CC BY). The use, distribution or reproduction in other forums is permitted, provided the original author(s) and the copyright owner(s) are credited and that the original publication in this journal is cited, in accordance with accepted academic practice. No use, distribution or reproduction is permitted which does not comply with these terms.
*Correspondence: Hetron Mweemba Munang’andu, hetron.m.munangandu@nord.no