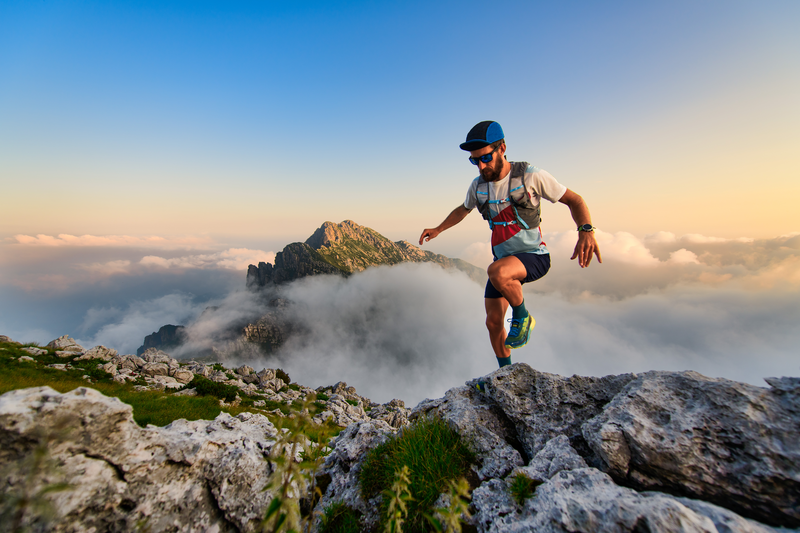
95% of researchers rate our articles as excellent or good
Learn more about the work of our research integrity team to safeguard the quality of each article we publish.
Find out more
ORIGINAL RESEARCH article
Front. Microbiol. , 28 November 2022
Sec. Food Microbiology
Volume 13 - 2022 | https://doi.org/10.3389/fmicb.2022.1022054
Introduction: The rare earth gadolinium (Gd) is widely used in industry and medicine, which has been treated as an emerging pollutant in environment. The increasing pollution of Gd has potential hazards to living organisms. Thus it is essential to investigate the toxicity and action mechanism of Gd in biological system.
Methods: In this study, the global effect and activation mechanism of Gd on yeast were investigated by genome-scale screening.
Results and discussion: Our results show that 45 gene deletion strains are sensitive to Gd and 10 gene deletion strains are Gd resistant from the diploid gene deletion strain library of Saccharomyces cerevisiae. The result of localization analysis shows that most of these genes are involved in cell metabolism, cell cycle, transcription, translation, protein synthesis, protein folding, and cell transport. The result of functional analysis shows that four genes (CNB1, CRZ1, VCX1, and GDT1) are involved in the calcium signaling pathway, and four genes (PHO84, PHO86, PHO2, and PHO4) are involved in phosphorus metabolism. For Gd3+ has the similar ion radius with Ca2+ and easily binds to the phosphate radical, it affects Ca2+ signaling pathway and phosphorus metabolism. The genes ARF1, ARL1, ARL3, SYS1, COG5, COG6, YPT6, VPS9, SSO2, MRL1, AKL1, and TRS85 participate in vesicle transport and protein sorting. Thus, Gd accumulation affects the function of proteins related to vesicle transport, which may result in the failure of Gd transport out of cells. In addition, the intracellular Gd content in the 45 sensitive deletion strains is higher than that in the wild type yeast under Gd stress. It suggests that the sensitivity of yeast deletion strains is related to the excessive intracellular Gd accumulation.
Rare earth elements (REEs) include 17 chemical elements (lanthanum-luteum, scandium, and yttrium) (Gwenzi et al., 2018). Depending on the physical and chemical properties, REEs can be classified as light and heavy rare earths. Gadolinium (Gd) is one of heavy rare earth elements, and it is mostly present in the form of compounds. The concentration of Gd in the soil environment is 1.6 ~ 7.1 mg/kg in China, and it is 0.2 ~ 36 mg/kg worldwide (Ramos et al., 2016). The concentration of Gd is 0.36 ~ 26.9 ng/l in marine systems, 0.347 ~ 80 μg/l in freshwater environments, and it reached 409.4 ng/l in a submarine outfall (Trapasso et al., 2021).
At present, Gd has been widely used in magnetic refrigeration, medical treatment, and nuclear energy (Gwenzi et al., 2018; Ben Salem and Barrat, 2021; Itoh et al., 2021). Therefore, the wide application of Gd has made it become a new emerging contaminant of aquatic environment (Rogowska et al., 2018). In the medical field, Gd contrast is widely used in magnetic resonance imaging (MRI) to improve the imaging signal and enhance magnetic resonance angiography (MRA) (Kim et al., 2018; Yon et al., 2019; Sakol et al., 2020). However, it is also observed that Gd-containing contrast has side effects to a small number of people (Raczeck et al., 2019). In addition, the toxicity of Gd on rice seedlings shows that the higher concentration of Gd inhibits the growth of rice and Gd can be accumulated in rice (Zhang et al., 2019a).
Microorganisms are widely used for preventing heavy metal pollution. Various biosorption materials, such as yeasts, bacteria and algae, have been used to remove metal ions in water environment. Biosorption is an ideal biological materials for treating wastewaters contaminated by heavy metals (Veglio and Beolchini, 1997; Gaur et al., 2014; Razzak et al., 2022). In the beverage and food industry, yeast Saccharomyces cerevisiae is easily to cultivate at a large scale and possesses various advantages as a metal biosorbent (Kapoor and Viraraghavan, 1995; Sagar Jena et al., 2022). Moreover, S. cerevisiae is a simple, easily operable and flexible unicellular eukaryotic organism, and it is one of the most widely used eukaryotic model organisms in laboratory (Orr-Weaver et al., 1983; Baudin et al., 1993; Wach et al., 1994; Nelissen et al., 1997). As a eukaryotic model organism, S. cerevisiae is extensively used to investigate the mechanism of metal ion transport (Eide, 2000; Revel et al., 2022).
In addition, the genome-wide information of yeast has been acquired with the completion of genome sequencing. Compared to the other model organisms, yeast reproduces quickly and can reproduce 1 generation in 1.5 ~ 2 h (Baudin et al., 1993). In addition, the growth of yeast can be divided into haploid and diploid, which is the advantage of yeast in the studies on gene functions. The genome of S. cerevisiae is simpler compared to Caenorhabditis elegans and human. The apart between two intercoding protein genes are approximately 6 kb in C. elegans, and it is at least 30 kb in human, while it is only 2 kb in yeast (Baudin et al., 1993).
It is known that some metal ions are essential nutritional elements for organisms. However, the over-accumulation of metal ions is toxic for organisms. Fortunately, the cellular homeostasis mechanism and the detoxification system precisely control the level and distribution of metal ions in cells. At present, the functional genomics is rapidly accelerating research on the metal ion stresses in yeast (Eide, 2001; Shao et al., 2018; Akao, 2019). If yeast cells are exposed to the extracellular environment stress, various signal transduction pathways will be stimulated in the response to stress. Previously, Du et al. found that PHO4 gene encodes a transcription factor of the myc family helix–loop–helix (bHLH) structure, and it is activated under low phosphate stress (Du et al., 2015). In addition, Yoshimoto et al. observed that the Ca2+/calcineurin signaling pathway was activated by various stress factors, such as exposure to high Ca2+ and Na+ stresses (Yoshimoto et al., 2002). Cunningham et al. found that PMC1 (Ca2+-ATPases on the plasma membrane) plays a key role in calcineurin activation through regulating Ca2+ concentration in yeast (Cunningham and Fink, 1994).
As a fully sequenced eukaryotic cell, yeast S. cerevisiae is one of the main experimental models for understanding eukaryotic systems, especially for studying the mechanism of metal ion toxicity (Horstmann and Kim, 2021; Robinson et al., 2021). At present, S. cerevisiae has been widely used as a model for studying metal ion stresses and related signal transduction pathway. Previously, various signaling pathways and ion transporters has been found in yeast under metal stresses (Cools et al., 2019; Johnston and Strobel, 2019; Zhang et al., 2019b; Kumari et al., 2021; Shi et al., 2021). Rare earth element Gd is a new emerging contaminant of environment, and Gd3+ has the similar ion radius with Ca2+ and easily binds to the phosphate radical. To fully understand the global effect and regulatory mechanism of Gd in eukaryotic cells, we screened the diploid gene deletion library of yeast under Gd stress. And, we identified 45 Gd sensitive deletion strains and 10 resistance gene deletion strains in total. The functional localization analysis was further performed to investigate the mechanism of Gd detoxification and transportation in yeast.
The diploid S. cerevisiae strains (BY4743 genetic background and KanMX4 genetic labels with G418 resistant) were purchased from Invitrogen Inc. (USA). The yeast deletion library BY4743 was created by a collaboration of eight North American and eight European laboratories composing the Saccharomyces Genome Deletion (SGD) Project. The intent of this consortium was to produce a deletion clone for each gene in the yeast genome. The library consists of 4,741 homozygous diploid clones. Yeast was grown at 30°C in the YPD medium (2% peptone, 1% yeast extract, and 2% glucose, pH 5.6).
To find a sublethal concentration of Gd to S. cerevisiae, we firstly designed the effect of 0, 1, 2, 3, and 4 mM Gd on the yeast growth, and we observed that 4 mM Gd was lethal for yeast. For the status of yeast growth was good at 3 mM Gd and 4 mM Gd was lethal for yeast, we further designed three additional Gd concentrations (3, 3.5, and 4 mM Gd) to study the effect of Gd on yeast growth. The detailed method was as follows. The experiment for screening Gd-sensitive and Gd-resistant phenotype of yeast was repeated three times.
Firstly, the single colonies of yeast were incubated in 3 ml YPD liquid medium and shaken at 200 rpm for 16 h at 30°C. The yeast solution was diluted and transferred to YPD medium containing 0, 1, 2, 3, and 4 mM Gd(NO3)3 (Aladdin Co., Ltd., Shanghai, China). The size of bacterial plaque was observed after 2 days incubation at 30°C to investigate the sublethal concentration of Gd to S. cerevisiae.
Then, according to the protocal of a previous study (Luo et al., 2016), 0, 3, 3.5, and 4 mM Gd(NO3)3 was further used for Gd toxicity preliminary screening. We used the library strains for the preliminary screening of fungi mutations that were sensitive to Gd. All strains were copied to the YPD medium containing 0 or 3.5 mM Gd by using 384 pin tool, respectively. Two days later, the tablet was photographed and analyzed the growth of each mutant. Based on the analysis of colony size, 3.5 mM Gd nitrate was used for screening Gd-sensitive mutants. Compared to the surrounding mutants, if the colony size of mutant was reduced more than 30% by Gd treatment, it was considered Gd-sensitive.
Finally, we performed the re-screening and verification of Gd-sensitive mutants by the method of continuous yeast culture dilution determination (Jiang et al., 2014). The sensitive mutants were streak cultured from the original library to the YPD liquid medium, and inoculated into YPD liquid medium for 12 h. The corresponding yeast strains from preliminary screening were cultured and purified on YPD solid plates for 48 h at 30°C to acquire the single colonies of yeast. The single colonies were inoculated into YPD liquid medium and cultured for 16 h at 30°C in an incubator with 200 rpm. After adjusting the concentration of bacteria solution, we obtained five yeast culture diluents (10−0, 10−1, 10−2, 10−3, 10−4). Then, five yeast culture diluents (10−0, 10−1, 10−2, 10−3, 10−4) were put on the YPD solid medium containing 0, 3.5 mM Gd(NO3)3, and 10.5 mM NaNO3 (Aladdin Co., Ltd., Shanghai, China), respectively. To confirm that the mutant was sensitive to Gd3+ rather than NO3−, 10.5 mM NaNO3 was used for the wild-type comparison (Luo et al., 2016). The yeasts were cultured at 30°C for 2 ~ 5 days and analyzed the growth and plaque size of each mutant. In addition, for screening Gd-resistant phenotype of yeast, 0, 3.8 mM Gd(NO3)3, and 10.5 mM NaNO3 were used according to the above method.
The intracellular Gd concentration was detected according to a previous method. (Zhao et al., 2013). For 3.5 mM Gd was lethal for various yeast strains, we used 1.75 mM Gd to detect the intracellular Gd concentration. The yeast strains were seeded into 5 ml sterilized medium and incubated at 30°C with 220 rpm shaking for 16 h. Then appropriate volume of bacterial solution was transferred to 50 ml medium containing 0 and 1.75 mM Gd, and cultured at 30°C with 220 rpm shaking for 4 h. The yeast were collected by centrifugation at 2000 rpm for 2 min at 4°C. Then, the yeasts were washed with 10 mM MgCl2 (containing 1 M sorbitol), and centrifuged at 2000 rpm for 2 min at 4°C. The yeasts were lysed in 10 ml 10 mM MgCl2. 0.3 ml bacterial solution was diluted 10 times with MgCl2, and the OD value was measured at 600 nm to adjust bacterial solution for consistent concentrations. Finally, the remaining 2.7 ml solution was added into 50 μl 6 M HNO3 and nitrated at 95°C for 60 min. After centrifugation at 2000 rpm for 2 min at 4°C, the supernatant was collected to analyze the intracellular Gd concentration with an inductively coupled plasma mass spectrometer (ICP-MS, Agilent 7,500). Six single mutant colonies were detected and the wild type BY4743 was used as control. The experiment was repeated six times.
To test the missing strain genome, each genome of yeast missing strain was used as a template for PCR. Its upstream primer is designed within 100 ~ 300 bp upstream of the mutant gene open reading box, and the downstream primer is designed at the internal sequence of the KanMX4 knockout box (about 1,200 bp from initiation codon). The primers used for testing are listed in Supplementary Table 1. The experiment was repeated three times.
The localization and function of the corresponding genes of Gd-sensitive mutants were annotated by using MIPS, the yeast genome database,1 FunSpec,2 and BioGRID.3 In addition, the protein interactome (protein–protein interactions) was further analyzed in yeast. The proteins corresponding to Gd sensitive gene deletion strains were mapped onto the interactome, and then filtered to identify the connected groups of proteins.4 The minimum required interaction score was set at high confidence 0.7.
Data was expressed as mean ± SEM. The difference among various experimental groups was analyzed with the single factor variance analysis (LSD’s test) analysis by using the statistical software SPSS (16.0). The level of significance was set at p < 0.05.
We firstly tested the sensitivity of yeast cells to gadolinium nitrate in this study, and the experiment for screening Gd-sensitive phenotype of yeast was repeated three times. After cultivating yeast cells with gadolinium nitrate medium with different concentration gradients, it was observed that yeast cells had a specific sensitivity at 3.5 mM Gd (Figures 1A,B). Therefore, we confirmed screening the diploid mutant library of S. cerevisiae with 3.5 mM Gd (Figure 1B). Finally, we identified a total of 45 mutant sensitive to Gd and 10 resistance deletion mutant strains. In addition, the genotypes of mutants were further confirmed by PCR testing, and the result indicated that the genotypes of these mutants were correct (Supplementary Figures 1, 2).
Figure 1. The toxicity of Gd and screening Gd-sensitive concentration. (A) Effect of 0, 1, 2, 3, and 4 mM Gd on yeast growth. (B) Effect of 3, 3.5, and 4 mM Gd on yeast growth. To find a sublethal concentration of Gd to S. cerevisiae, the effect of 0, 1, 2, 3, and 4 mM Gd on the yeast growth was observed, and 4 mM Gd was lethal for yeast. For 4 mM Gd was lethal for yeast, three additional Gd concentrations (3, 3.5, and 4 mM Gd) were further used to study the effect of Gd on yeast growth. The experiment was repeated three times.
Moreover, we performed the function and subcellular localization analysis of the sensitive genes by MIPS related network resources and SGD. By analyzing the functional classification, these Gd-sensitive genes are divided into the following 7 categories (Table 1; Figure 2; Supplementary Figure 3A). Group A, Metabolism (2 genes); Group B, DNA processing and cell cycle (3 genes); Group C, Transcription (4 genes); Group D, Protein synthesis, modification, folding, and destination (6 genes); Group E, Cellular transport, transport routes, and transport facilities (22 genes); Group F, Cell rescue, virulence, and defense (2 genes); Group G, Unclassified proteins (6 genes). Moreover, the sensitive genes are localized in cytoplasm (11 genes), Golgi bodies (10 genes), nuclei (9 genes), vesicles (7 genes), endoplasmic reticulum (4 genes), plasma membrane (4 genes), mitochondria (1 gene), and 5 unidentified location genes (Supplementary Figure 3A).
Figure 2. Phenotypes of Gd-sensitive gene deletion mutants. Five yeast culture diluents (10−0, 10−1, 10−2, 10−3, 10−4) were put on the YPD solid medium containing 0, 3.5 mM Gd(NO3)3, and 10.5 mM NaNO3, respectively. Cells of the wild-type BY4743 and 45 gene deletion mutants were identified from the genome-scale screen, and functional categories of the genes corresponding to the sensitive phenotypes were classified. (A) DNA processing and cell cycle (3 genes); (B) Metabolism (2 genes); (C) Cell rescue, virulence, and defense (2 genes); (D) Protein synthesis, modification, folding, and destination (6 genes); (E) Unclassified proteins (6 genes); (F) Cellular transport, transport routes, and transport facilities (22 genes); (G) Transcription (4 genes). The experiment was repeated three times.
In this study, we confirmed a total of 10 Gd resistance deletion mutant strains (Table 2; Figure 3; Supplementary Figure 3B), and the experiment for screening Gd-resistant phenotype of yeast was repeated three times. In addition, the result of PCR testing indicated that the genotypes of these mutants were correct (Supplementary Figure 2). The Gd resistance genes were involved in 4 groups, which are as follows (Supplementary Figure 3B). Group H, Metabolism (4 genes); Group I, DNA processing and cell cycle (1 gene); Group J, Protein synthesis, modification, folding, and destination (3 genes); Group K, Cellular transport, transport routes, and transport facilities (2 genes). The Gd resistance genes are localized in the cytoplasm (4 genes), nuclei (2 genes), mitochondria (1 gene), endoplasmic reticulum (2 genes), and Golgi apparatus (1 gene).
Figure 3. Phenotypes of Gd-resistant gene deletion mutants. Five yeast culture diluents (10−0, 10−1, 10−2, 10−3, 10−4) were put on the YPD solid medium containing 0, 3.8 mM Gd(NO3)3, and 10.5 mM NaNO3, respectively. Cells of the wild-type BY4743 and 10 gene deletion mutants were identified from the genome-scale screen, and functional categories of the genes corresponding to the resistant phenotypes were classified. (A) Metabolism (4 genes); (B) DNA processing and cell cycle (1 gene); (C) Protein synthesis, modification, folding, and destination (3 genes); (D) Cellular transport, transport routes, and transport facilities (2 genes). The experiment was repeated three times.
The intracellular Gd concentration was detected and the experiment was repeated six times. Compared to the wild-type yeast, 42 sensitive deletion mutants had significantly higher intracellular Gd content than that in the wild-type cells except for 3 mutants vcx1, lem3, and isa2 (Figure 4). The intracellular Gd content in 36 sensitive deletion mutants was about 1-fold of the wild-type cell (Figure 4). In addition, the intracellular Gd content in the mutants related to ssz1/ssz1, ykl118w/ykl118w, vma1/vma1, vma2/vma2, vps9/vps9 Gd sensitive gene deletion mutants was about 2-fold of the wild-type cell (Figure 4A,B). The intracellular Gd content in the mutants related to hit1/hit1 and sur1/sur1 Gd sensitive gene deletion mutants were about 3-fold, and Gd content in the mutants rps1a/rps1a was about 4-fold of the wild-type cells (Figure 4A). Nevertheless, no remarkable difference on the intracellular Gd content was observed in 6 Gd resistance gene deletion mutants compared to wild-type BY4743 (Figure 4C).
Figure 4. Intracellular Gd concentration in 45 Gd-sensitive and 15 Gd-resistant gene deletion mutants under Gd stress. (A) Intracellular Gd concentration in 23 Gd-sensitive gene deletion mutants; (B) Intracellular Gd concentration in 22 Gd-sensitive gene deletion mutants; (C) Intracellular Gd concentration in 15 Gd-resistant gene deletion mutants. Data was expressed as mean ± SEM (n = 6). The difference was analyzed with the single factor variance analysis (LSD’s test) analysis, and the level of significance is set at p < 0.05. Statistically significant differences are denoted by asterisk (*) (p < 0.05). The experiment was repeated six times.
For 55 deletion mutant strains were screened, we further performed protein function analysis on these genes (Figure 5). The result showed that these gene encode proteins were mainly related to intracellular vesicle transport, calcium signaling pathway, modification of tRNA wobble position, protein synthesis, composition of V-ATPase, and phosphorus metabolism (Figure 5). Notably, the most abundant of Gd sensitive genes (ARF1, ARL1, ARL3, SYS1, COG5, COG6, YPT6, VPS9, SSO2, MRL1, AKL1, and TRS85) were associated with the intracellular vesicle transport (Figure 5A). Some Gd sensitive genes (CNB1, MCK1, VCX1, and CRZ1) were involved in calcium signaling pathway (Figure 5B). Gd sensitive genes NCS2 and TRM9 were associated with the modification of tRNA wobble position (Figure 5C). In addition, Gd sensitive genes PRS1A and MRT4 participated in protein synthesis (Figure 5D). Gd sensitive genes VMA1 and VMA2 were the subunit A and Subunit B of V1 peripheral membrane domain of V-ATPase (Figure 5E). Gd sensitive genes (PHO84, PHO86, PHO2, and PHO4) participated in phosphorus metabolism (Figures 5F).
Figure 5. Gd toxicity modulating networks identified with proteins whose absence renders cells sensitive to Gd. (A) Gd sensitive genes (ARF1, ARL1, ARL3, SYS1, COG5, COG6, YPT6, VPS9, SSO2, MRL1, AKL1, and TRS85) were associated with the intracellular vesicle transport; (B) Gd sensitive genes (CNB1, MCK1, VCX1, and CRZ1) were involved in calcium signaling pathway; (C) Gd sensitive genes NCS2 and TRM9 were associated with the modification of tRNA wobble position; (D) Gd sensitive genes PRS1A and MRT4 participated in protein synthesis; (E) Gd sensitive genes VMA1 and VMA2 were the subunit A and Subunit B of V1 peripheral membrane domain of V-ATPase; (F) Gd sensitive genes (PHO84, PHO86, PHO2, and PHO4) participated in phosphorus metabolism. The proteins corresponding to Gd sensitive gene deletion strains were mapped onto the interactome, and then filtered to identify the connected groups of proteins (https://cn.string-db.org/). The minimum required interaction score was set at high confidence 0.7. The straight lines indicate the protein–protein interactions.
In this study, the global effect and regulatory mechanism of Gd on yeast were investigated by genome-scale screening. We firstly tested the sensitivity of yeast cells to Gd by cultivating yeast cells in the different concentration of Gd. It is observed that yeast cells have a specific sensitivity at 3.5 mM Gd. In addition, we find that the yeast mutants lacking ARF1, ARL1, ARL3, SYS1, COG5, COG6, YPT6, VPS9, SSO2, MRL1, AKL1, and TRS85 are significantly sensitive to Gd stress. These genes are involved in the process of vesicle trafficking. ARL1 is a key coding gene of the Arf/Arl/Sar family, and YPT6 is a key coding gene in the Rab family. Both of Arf/Arl/Sar family and Rab family play a key regulatory role in vesicle trafficking. In addition, ARL1 and YPT6 are present on the opposite membrane of Golgi network (TGN) and regulate vesicular trafficking between TGN and early endosomes (Rosenwald et al., 2002). Similar to the other GTP-binding proteins, the activities of ARL1 and YPT6 are positively related with GTP concentration. However, if GTP is hydrolyzed to GDP, they remain in the cytosol but are not active (Li and Warner, 1996). Ypt6p is a protein similar to human Rab6p (Yang and Rosenwald, 2016). YPT6, ARF1, and ARL1 are important regulators of vesicle trafficking on the Golgi membrane. TRS85 is a component of the transporter pellet (TRAPP) complex III. Moreover, TRAPP I and TRAPPIII, as the GEF of YPT1, can activate YPT1 through the subunit TRS85 and further participates in vesicle trafficking in the Golgi apparatus (Lynch-Day et al., 2010). ARL3 requires the repositioning of Arl1p (a GTPase that regulates vesicular transport) to Golgi. SYS1 is an integral membrane protein on Golgi, and is necessary for targeting Arf-like GTPase Arl3p to Golgi. AKL1 is a Ser-Thr protein kinase, which belongs to the Ark kinase family (containing Ark1p and Prk1p) and participates in endocytosis and actin cytoskeleton formation (Roelants et al., 2017). The absence of the above 12 genes may affect intracellular vesicle trafficking, and Gd can not be effectively transported out of the cells.
In addition, VPS9 participates in vacuolar protein sorting, and it is a transporter from the late Golgi complex to the storage vacuole precursor (PVC) (Bonangelino et al., 2002). In this study, the deletion of VPS9 gene leads to the yeast cells being sensitive to Gd. However, we find that the intracellular Gd content in the VPS9 yeast deletion strain is 2-fold higher than that in the wild-type yeast. Therefore, it indicates that the vacuolar septum also plays a key role in decreasing the intracellular Gd toxicity in yeast cells.
It is known that Ca2+ is one of the key signaling molecules and it is involved in regulating various cell activities (Berridge et al., 2003). Calcineurin (CaN), as a serine/threonine protein phosphatase, participates in various cellular metabolism processes and Ca2+-dependent signaling transduction pathways (Romano et al., 2017). In addition, CNB1 is a gene encoding the CaN regulatory subunit (CnB) (Cunningham, 2011). The activity of CaN is regulated by Rcn1, whereas Rcn1 activity is regulated by MCK1, a member of the GSK-3 kinase protein family (Hilioti et al., 2004). If yeast is exposed to the external environmental stress, the Ca2+/CaN signaling pathway will be activated. In the normal growing environment, there is adequate Ca2+ concentration in the cytoplasm and CaN can be activated. Thus, cells lacking CNB1, MCK1, VCX1, and CRZ1 gene can grow normally in YPD medium. For the radius of Gd3+ is similar to Ca2+, Gd3+ can compete for binding with the site of Ca2+, which will reduce the intracellular Ca2+ levels and affect the Ca2+-dependent signaling transduction pathway. However, in a certain range of Ca2+ levels, it does not decrease yeast cell viability.
Moreover, CaN dephosphorylates the transcription factor Crz1 in the cytoplasm, and Crz1 will rapidly transfer from cytoplasm to nucleus (Bodvard et al., 2013). Crz1 further induces the target genes, including the calcium pump genes PMC1 in the vacuolar membrane and PMR1 in the membrane of ER and Golgi (Xu et al., 2019; Yan et al., 2020). These two calcium pumps (PMC1 and PMR1) and the Ca2+/H+ exchange protein Vcx1 on the vacuole membrane cooperate to control Ca2+ concentration within the normal physiological concentration range in the cytoplasm (Miseta et al., 1999), which allows the cells to grow normally. However, the yeast cells lacking CRZ1 and VCX1 genes are difficult to survive under the Gd stress environment. In addition, Gdt1 is a Ca2+/H+ exchanger on the membrane of vacuole and Golgi, which is involved in regulating Ca2+ transmembrane transport. Gdt1 also plays a key role in maintaining the dynamic intracellular Ca2+ balance (Colinet et al., 2017; Dulary et al., 2018; Thines et al., 2020).
Gd sensitive genes NCS2 and TRM9 are associated with the modification of tRNA wobble position. Protein NCS2 is required for uridine thiolation at the wobble position of tRNA and plays function in protein urmylation (Noma et al., 2009). And, NCS2 has a role in regulating urmylation, invasive, and pseudohyphal growth. Protein TRM9 is a tRNA methyltransferase and it catalyzes modification of wobble bases in tRNA anticodons (Patil et al., 2012). It is involved in avoiding the deletion mutation in the translational infidelity, including amino acid misincorporation and frameshifting. In addition, Gd sensitive genes PRS1A and MRT4 participated in protein synthesis (Zuk et al., 1999). In our study, Gd sensitive genes NCS2, TRM9, PRS1A and MRT4 were identified in yeast. It shows that the yeast mutants of NCS2, TRM9, PRS1A and MRT4 display the activation of Gd stress responses. The stress of Gd may affect the modification of tRNA wobble position and protein synthesis in yeast.
The H+-ATPase on the vacuolar membrane is composed of two complexes, including V1 and V0. V1 consists of eight subunits, which are responsible for ATP hydrolysis (Jefferies et al., 2008). Moreover, V0 consists of six subunits responsible for H+ transport. The absence of genes VMA2 and VMA1, which encode the A and B subunits of V1 complex, makes the yeast cells sensitive to Gd. In addition, the intracellular Gd content was also significantly higher in the VMA2 and VMA1 gene deletion strains than that in the control.
Phosphorus is an essential and massive element for cell growth. Maintaining the dynamic balance of intracellular phosphorus is essential for cell survival. Once the phosphorus levels are unbalanced, it will affect cell differentiation and proliferation, disrupt cell metabolism, and seriously deform cytoskeletal morphology (Dick et al., 2011). In response to the dynamic phosphate levels in environment, yeast has evolved a signaling pathway (PHO pathway) to real-time monitor the intracellular phosphorus metabolism. In this study, we observed that the cells lacking PHO84, PHO86, PHO2, and PHO4 genes were abnormally sensitive to Gd stress. The result is similar to the effect of reduced intracellular phosphorus levels or the formation of inactive phosphate precipitation (Mouillon and Persson, 2006; Wykoff et al., 2007; Dick et al., 2011). Moreover, in the five unidentified location genes, the yml122c mutant does not have a death ratio as much as the other 5 mutants. Five genes are highly sensitive to the Cd exposure while yml122c is resistant, which indicates this gene may have a crucial role in cell death mechanisms. Previously, the result shows that yml122c is also one member of the high-affinity PHO pathway (Haas, 2012). Thus, yml122c also plays a role in regulating phosphate levels. In addition, our result shows that four deletion strains also had higher intracellular Gd content than the wild-type yeast. Previously, we found that Gd and phosphate salts form an insoluble inactive state in rice seedlings (Zhang et al., 2019a). Therefore, Gd and phosphate salts may form an insoluble inactive state in yeast, and the intracellular absence of available phosphorus finally results in the cellular phosphorus deficiency stress.
In addition, LEM3 is a membrane protein of the plasma membrane and ER involved in phospholipid translocation. ISA2 is a protein required for maturation of mitochondrial [4Fe-4S] proteins. HIT1 is a protein involved in box C/D snoRNP assembly, and LOA1 isa lysophosphatidic acid acyltransferase. TRK1 is a component of the Trk1p-Trk2p potassium transport system, and GDA1 is a guanosine diphosphatase in the Golgi lumen. HIR2 is a subunit of HIR nucleosome assembly complex involved in regulation of histone gene transcription. SAC7 is a Rho1p GTPase activating protein (GAP), and SIF2 is a WD40 repeat-containing subunit of Set3C histone deacetylase complex. Moreover, TPS1 is a synthase subunit of trehalose-6-phosphate synthase/phosphatase complex. However, the detailed function of these proteins needs to be further studied in future for there are less information on these proteins.
In summary, the global effect and regulatory mechanism of Gd on yeast were investigated by genome-scale screening. Our result shows that 45 gene deletion strains are sensitive to Gd and 10 gene deletion strains are Gd-resistant from the diploid gene deletion strain library of S. cerevisiae. The localization analysis shows that most of these genes are involved in cell metabolism, cell cycle, transcription, translation, protein synthesis and folding, cell transport, etc. The result of functional analysis shows that four genes (CNB1, CRZ1, VCX1, and GDT1) are involved in the calcium signaling pathway, and four genes (PHO84, PHO86, PHO2, and PHO4) are involved in phosphorus metabolism. For Gd3+ has the similar ion radius with Ca2+ and easily binds to the phosphate radical, it further affects the Ca2+ signaling pathway and phosphorus metabolism. The genes ARF1, ARL1, ARL3, SYS1, COG5, COG6, YPT6, VPS9, SSO2, MRL1, AKL1, and TRS85 participate in protein sorting and vesicle transport. In addition, the intracellular Gd content in the 45 sensitive deletion strains is higher than that in the wild type yeast under Gd stress. It suggests that the sensitivity of yeast deletion strains may be related to the excessive intracellular Gd accumulation.
The original contributions presented in the study are included in the article/Supplementary material, further inquiries can be directed to the corresponding author.
XW: conceptualization, supervision. YL, KL, and JD: methodology. YC and CZ: writing–original draft preparation. YC, CZ, and YF: investigation. YC and XW: writing–reviewing and editing. All authors contributed to the article and approved the submitted version.
This study was supported by National Natural Science Foundation of China (30900071).
The authors declare that the research was conducted in the absence of any commercial or financial relationships that could be construed as a potential conflict of interest.
All claims expressed in this article are solely those of the authors and do not necessarily represent those of their affiliated organizations, or those of the publisher, the editors and the reviewers. Any product that may be evaluated in this article, or claim that may be made by its manufacturer, is not guaranteed or endorsed by the publisher.
The Supplementary material for this article can be found online at: https://www.frontiersin.org/articles/10.3389/fmicb.2022.1022054/full#supplementary-material
Akao, T. (2019). Progress in the genomics and genome-wide study of sake yeast. Biosci. Biotechnol. Biochem. 83, 1463–1472. doi: 10.1080/09168451.2019.1588098
Baudin, A., Ozier-Kalogeropoulos, O., Denouel, A., Lacroute, F., and Cullin, C. (1993). A simple and efficient method for direct gene deletion in Saccharomyces cerevisiae. Nucleic Acids Res. 21, 3329–3330. doi: 10.1093/nar/21.14.3329
Ben Salem, D., and Barrat, J. A. (2021). Determination of rare earth elements in gadolinium-based contrast agents by ICP-MS. Talanta 221:121589. doi: 10.1016/j.talanta.2020.121589
Berridge, M. J., Bootman, M. D., and Roderick, H. L. (2003). Calcium signalling: dynamics, homeostasis and remodelling. Nat. Rev. Mol. Cell Biol. 4, 517–529. doi: 10.1038/nrm1155
Bodvard, K., Jörhov, A., Blomberg, A., Molin, M., and Käll, M. (2013). The yeast transcription factor Crz1 is activated by light in a Ca2+/calcineurin-dependent and PKA-independent manner. PLoS One 8:e53404. doi: 10.1371/journal.pone.0053404
Bonangelino, C. J., Chavez, E. M., and Bonifacino, J. S. (2002). Genomic screen for vacuolar protein sorting genes in Saccharomyces cerevisiae. Mol. Biol. Cell 13, 2486–2501. doi: 10.1091/mbc.02-01-0005
Colinet, A. S., Thines, L., Deschamps, A., Flémal, G., Demaegd, D., and Morsomme, P. (2017). Acidic and uncharged polar residues in the consensus motifs of the yeast Ca2+ transporter Gdt1p are required for calcium transport. Cell. Microbiol. 19:e12729. doi: 10.1111/cmi.12729
Cools, M., Rompf, M., Mayer, A., and André, B. (2019). Measuring the activity of plasma membrane and vacuolar transporters in yeast. Methods Mol. Biol. 2049, 247–261. doi: 10.1007/978-1-4939-9736-7_15
Cunningham, K. W. (2011). Acidic calcium stores of Saccharomyces cerevisiae. Cell Calcium 50, 129–138. doi: 10.1016/j.ceca.2011.01.010
Cunningham, K. W., and Fink, G. R. (1994). Calcineurin-dependent growth control in Saccharomyces cerevisiae mutants lacking PMC1, a homolog of plasma membrane Ca2+ ATPases. J. Cell Biol. 124, 351–363. doi: 10.1083/jcb.124.3.351
Dick, C. F., Dos-Santos, A. L., and Meyer-Fernandes, J. R. (2011). Inorganic phosphate as an important regulator of phosphatases. Enzyme. Res. 2011:103980. doi: 10.4061/2011/103980
Du, J., Cao, C., and Jiang, L. (2015). Genome-scale genetic screen of lead ion-sensitive gene deletion mutations in Saccharomyces cerevisiae. Gene 563, 155–159. doi: 10.1016/j.gene.2015.03.018
Dulary, E., Yu, S. Y., Houdou, M., de Bettignies, G., Decool, V., Potelle, S., et al. (2018). Investigating the function of Gdt1p in yeast Golgi glycosylation. Biochim. Biophys. Acta Gen. Subj. 1862, 394–402. doi: 10.1016/j.bbagen.2017.11.006
Eide, D. J. (2000). Metal ion transport in eukaryotic microorganisms: insights from Saccharomyces cerevisiae. Adv. Microb. Physiol. 43, 1–38. doi: 10.1016/s0065-2911(00)43001-8
Eide, D. J. (2001). Functional genomics and metal metabolism. Genome Biol. 2:REVIEWS1028. doi: 10.1186/gb-2001-2-10-reviews1028
Gaur, N., Flora, G., Yadav, M., and Tiwari, A. (2014). A review with recent advancements on bioremediation-based abolition of heavy metals. Environ Sci Process Impacts 16, 180–193. doi: 10.1039/c3em00491k
Gwenzi, W., Mangori, L., Danha, C., Chaukura, N., Dunjana, N., and Sanganyado, E. (2018). Sources, behaviour, and environmental and human health risks of high-technology rare earth elements as emerging contaminants. Sci. Total Environ. 636, 299–313. doi: 10.1016/j.scitotenv.2018.04.235
Haas, K. C. Z. (2012). Understanding the genetic requirements for Saccharomyces cerevisiae to survive at low temperatures. [dissertation/master’s thesis]. Minnesota: University of Minnesota
Hilioti, Z., Gallagher, D. A., Low-Nam, S. T., Ramaswamy, P., Gajer, P., Kingsbury, T. J., et al. (2004). GSK-3 kinases enhance calcineurin signaling by phosphorylation of RCNs. Genes Dev. 18, 35–47. doi: 10.1101/gad.1159204
Horstmann, C., and Kim, K. (2021). Comparing Transcriptome profiles of saccharomyces Cerevisiae cells exposed to cadmium Selenide/zinc sulfide and indium phosphide/zinc sulfide. Genes (Basel) 12:428. doi: 10.3390/genes12030428
Itoh, A., Yaida, A., and Zhu, Y. (2021). Potential anthropogenic pollution of high-technology metals with a focus on rare earth elements in environmental water. Anal. Sci. 37, 131–143. doi: 10.2116/analsci.20SAR16
Jefferies, K. C., Cipriano, D. J., and Forgac, M. (2008). Function, structure and regulation of the vacuolar (H+)-ATPases. Arch. Biochem. Biophys. 476, 33–42. doi: 10.1016/j.abb.2008.03.025
Jiang, L., Cao, C., Zhang, L., Lin, W., Xia, J., Xu, H., et al. (2014). Cadmium-induced activation of high osmolarity glycerol pathway through its Sln 1 branch is dependent on the MAP kinase kinase kinase Ssk2, but not its paralog Ssk22, in budding yeast. FEMS Yeast Res. 14, 1263–1272. doi: 10.1111/1567-1364.12220
Johnston, N. R., and Strobel, S. A. (2019). Nitrate and phosphate transporters rescue fluoride toxicity in yeast. Chem. Res. Toxicol. 32, 2305–2319. doi: 10.1021/acs.chemrestox.9b00315
Kapoor, A., and Viraraghavan, T. (1995). Fungal biosorption — an alternative treatment option for heavy metal bearing wastewaters: a review. Bioresour. Technol. 53, 195–206. doi: 10.1016/0960-8524(95)00072-M
Kim, H. K., Lee, G. H., and Chang, Y. (2018). Gadolinium as an MRI contrast agent. Future Med. Chem. 10, 639–661. doi: 10.4155/fmc-2017-0215
Kumari, S., Kumar, M., Gaur, N. A., and Prasad, R. (2021). Multiple roles of ABC transporters in yeast. Fungal Genet. Biol. 150:103550. doi: 10.1016/j.fgb.2021.103550
Li, B., and Warner, J. R. (1996). Mutation of the Rab6 homologue of Saccharomyces cerevisiae, YPT6, inhibits both early Golgi function and ribosome biosynthesis. J. Biol. Chem. 271, 16813–16819. doi: 10.1074/jbc.271.28.16813
Luo, C., Cao, C., and Jiang, L. (2016). The endosomal sorting complex required for transport (ESCRT) is required for the sensitivity of yeast cells to nickel ions in Saccharomyces cerevisiae. FEMS Yeast Res. 16:fow028. doi: 10.1093/femsyr/fow028
Lynch-Day, M. A., Bhandari, D., Menon, S., Huang, J., Cai, H., Bartholomew, C. R., et al. (2010). Trs85 directs a Ypt1 GEF, TRAPPIII, to the phagophore to promote autophagy. Proc. Natl. Acad. Sci. U. S. A. 107, 7811–7816. doi: 10.1073/pnas.1000063107
Miseta, A., Kellermayer, R., Aiello, D. P., Fu, L., and Bedwell, D. M. (1999). The vacuolar Ca2+/H+ exchanger Vcx1p/Hum1p tightly controls cytosolic Ca2+ levels in S. cerevisiae. FEBS Lett. 451, 132–136. doi: 10.1016/s0014-5793(99)00519-0
Mouillon, J. M., and Persson, B. L. (2006). New aspects on phosphate sensing and signalling in Saccharomyces cerevisiae. FEMS Yeast Res. 6, 171–176. doi: 10.1111/j.1567-1364.2006.00036.x
Nelissen, B., De Wachter, R., and Goffeau, A. (1997). Classification of all putative permeases and other membrane plurispanners of the major facilitator superfamily encoded by the complete genome of Saccharomyces cerevisiae. FEMS Microbiol. Rev. 21, 113–134. doi: 10.1111/j.1574-6976.1997.tb00347.x
Noma, A., Sakaguchi, Y., and Suzuki, T. (2009). Mechanistic characterization of the sulfur-relay system for eukaryotic 2-thiouridine biogenesis at tRNA wobble positions. Nucleic Acids Res. 37, 1335–1352. doi: 10.1093/nar/gkn1023
Orr-Weaver, T. L., Szostak, J. W., and Rothstein, R. J. (1983). Genetic applications of yeast transformation with linear and gapped plasmids. Methods Enzymol. 101, 228–245. doi: 10.1016/0076-6879(83)01017-4
Patil, A., Chan, C. T., Dyavaiah, M., Rooney, J. P., Dedon, P. C., and Begley, T. J. (2012). Translational infidelity-induced protein stress results from a deficiency in Trm9-catalyzed tRNA modifications. RNA Biol. 9, 990–1001. doi: 10.4161/rna.20531
Raczeck, P., Fries, P., Bücker, A., and Schneider, G. (2019). Gadolinium deposition-“gadolinium deposition disease”. Radiologe 59, 435–443. doi: 10.1007/s00117-019-0522-9
Ramos, S. J., Dinali, G. S., Oliveira, C., Martins, G. C., Moreira, C. G., Siqueira, J. O., et al. (2016). Rare earth elements in the soil environment. Curr. Pollut. Rep. 2, 28–50. doi: 10.1007/s40726-016-0026-4
Razzak, S. A., Faruque, M. O., Alsheikh, Z., Alsheikhmohamad, L., Alkuroud, D., Alfayez, A., et al. (2022). A comprehensive review on conventional and biological-driven heavy metals removal from industrial wastewater. Environ. Adv. 7:100168. doi: 10.1016/j.envadv.2022.100168
Revel, B., Catty, P., Ravanel, S., Bourguignon, J., and Alban, C. (2022). High-affinity iron and calcium transport pathways are involved in U(VI) uptake in the budding yeast Saccharomyces cerevisiae. J. Hazard. Mater. 422:126894. doi: 10.1016/j.jhazmat.2021.126894
Robinson, J. R., Isikhuemhen, O. S., and Anike, F. N. (2021). Fungal-metal interactions: a review of toxicity and homeostasis. J. Fungi (Basel). 7:225. doi: 10.3390/jof7030225
Roelants, F. M., Leskoske, K. L., Pedersen, R. T. A., Muir, A., Liu, J. M., Finnigan, G. C., et al. (2017). TOR complex 2-regulated protein kinase Fpk1 stimulates endocytosis via inhibition of Ark1/Prk1-related protein kinase Akl1 in Saccharomyces cerevisiae. Mol. Cell. Biol. 37, e00627–e00616. doi: 10.1128/mcb.00627-16
Rogowska, J., Olkowska, E., Ratajczyk, W., and Wolska, L. (2018). Gadolinium as a new emerging contaminant of aquatic environments. Environ. Toxicol. Chem. 37, 1523–1534. doi: 10.1002/etc.4116
Romano, D. R., Pharris, M. C., Patel, N. M., and Kinzer-Ursem, T. L. (2017). Competitive tuning: Competition's role in setting the frequency-dependence of Ca2+-dependent proteins. PLoS Comput. Biol. 13:e1005820. doi: 10.1371/journal.pcbi.1005820
Rosenwald, A. G., Rhodes, M. A., Van Valkenburgh, H., Palanivel, V., Chapman, G., Boman, A., et al. (2002). ARL1 and membrane traffic in Saccharomyces cerevisiae. Yeast 19, 1039–1056. doi: 10.1002/yea.897
Sagar Jena, P., Pradhan, A., Prakash Nanda, S., Kishore Dash, A., and Naik, B. (2022). Biosorption of heavy metals from wastewater using Saccharomyces cerevisiae as a biosorbent: a mini review. Mater. Today. Proc. 67, 1140–1146. doi: 10.1016/j.matpr.2022.07.306
Sakol, N., Egawa, A., and Fujiwara, T. (2020). Gadolinium complexes as contrast agent for cellular NMR spectroscopy. Int. J. Mol. Sci. 21:4042. doi: 10.3390/ijms21114042
Shao, Y., Lu, N., Wu, Z., Cai, C., Wang, S., Zhang, L. L., et al. (2018). Creating a functional single-chromosome yeast. Nature 560, 331–335. doi: 10.1038/s41586-018-0382-x
Shi, H., Jiang, Y., Yang, Y., Peng, Y., and Li, C. (2021). Copper metabolism in Saccharomyces cerevisiae: an update. Biometals 34, 3–14. doi: 10.1007/s10534-020-00264-y
Thines, L., Stribny, J., and Morsomme, P. (2020). From the uncharacterized protein family 0016 to the GDT1 family: molecular insights into a newly-characterized family of cation secondary transporters. Microb. Cell. 7, 202–214. doi: 10.15698/mic2020.08.725
Trapasso, G., Chiesa, S., Freitas, R., and Pereira, E. (2021). What do we know about the ecotoxicological implications of the rare earth element gadolinium in aquatic ecosystems? Sci. Total Environ. 781:146273. doi: 10.1016/j.scitotenv.2021.146273
Veglio, F., and Beolchini, F. (1997). Removal of metals by biosorption: a review. Hydrometallurgy 44, 301–316. doi: 10.1016/S0304-386X(96)00059-X
Wach, A., Brachat, A., Pöhlmann, R., and Philippsen, P. (1994). New heterologous modules for classical or PCR-based gene disruptions in Saccharomyces cerevisiae. Yeast 10, 1793–1808. doi: 10.1002/yea.320101310
Wykoff, D. D., Rizvi, A. H., Raser, J. M., Margolin, B., and O'Shea, E. K. (2007). Positive feedback regulates switching of phosphate transporters in S. cerevisiae. Mol. Cell 27, 1005–1013. doi: 10.1016/j.molcel.2007.07.022
Xu, H., Fang, T., Yan, H., and Jiang, L. (2019). The protein kinase Cmk 2 negatively regulates the calcium/calcineurin signalling pathway and expression of calcium pump genes PMR1 and PMC1 in budding yeast. Cell Commun. Signal 17:7. doi: 10.1186/s12964-019-0320-z
Yan, H., Fang, T., Xu, H., and Jiang, L. (2020). The pH-sensing Rim101 pathway positively regulates the transcriptional expression of the calcium pump gene PMR1 to affect calcium sensitivity in budding yeast. Biochem. Biophys. Res. Commun. 532, 453–458. doi: 10.1016/j.bbrc.2020.08.083
Yang, S., and Rosenwald, A. G. (2016). Autophagy in Saccharomyces cerevisiae requires the monomeric GTP-binding proteins, Arl1 and Ypt6. Autophagy 12, 1721–1737. doi: 10.1080/15548627.2016.1196316
Yon, M., Billotey, C., and Marty, J. D. (2019). Gadolinium-based contrast agents: from gadolinium complexes to colloidal systems. Int. J. Pharm. 569:118577. doi: 10.1016/j.ijpharm.2019.118577
Yoshimoto, H., Saltsman, K., Gasch, A. P., Li, H. X., Ogawa, N., Botstein, D., et al. (2002). Genome-wide analysis of gene expression regulated by the calcineurin/Crz1p signaling pathway in Saccharomyces cerevisiae. J. Biol. Chem. 277, 31079–31088. doi: 10.1074/jbc.M202718200
Zhang, F., Bian, J., Chen, X., Huang, J., Smith, N., Lu, W., et al. (2019b). Roles for intracellular cation transporters in respiratory growth of yeast. Metallomics 11, 1667–1678. doi: 10.1039/c9mt00145j
Zhang, C., Liu, D., Shi, K., Liu, C., Lyu, K., Chen, J., et al. (2019a). Gadolinium accumulation, distribution, chemical forms, and influence on the growth of rice seedlings. Ecotoxicol. Environ. Saf. 179, 265–271. doi: 10.1016/j.ecoenv.2019.04.074
Zhao, Y., Du, J., Zhao, G., and Jiang, L. (2013). Activation of calcineurin is mainly responsible for the calcium sensitivity of gene deletion mutations in the genome of budding yeast. Genomics 101, 49–56. doi: 10.1016/j.ygeno.2012.09.005
Keywords: global effect, gadolinium, sensitive genes, genome-scale screening, yeast
Citation: Cao Y, Zhang C, Fang Y, Liu Y, Lyu K, Ding J and Wang X (2022) Investigation the global effect of rare earth gadolinium on the budding Saccharomyces cerevisiae by genome-scale screening. Front. Microbiol. 13:1022054. doi: 10.3389/fmicb.2022.1022054
Received: 18 August 2022; Accepted: 11 November 2022;
Published: 28 November 2022.
Edited by:
Lifang Feng, Zhejiang Gongshang University, ChinaReviewed by:
Hao Li, Beijing University of Chemical Technology, ChinaCopyright © 2022 Cao, Zhang, Fang, Liu, Lyu, Ding and Wang. This is an open-access article distributed under the terms of the Creative Commons Attribution License (CC BY). The use, distribution or reproduction in other forums is permitted, provided the original author(s) and the copyright owner(s) are credited and that the original publication in this journal is cited, in accordance with accepted academic practice. No use, distribution or reproduction is permitted which does not comply with these terms.
*Correspondence: Xue Wang, eHVld2FuZ0BzZHV0LmVkdS5jbg==
†These authors have contributed equally to this work
Disclaimer: All claims expressed in this article are solely those of the authors and do not necessarily represent those of their affiliated organizations, or those of the publisher, the editors and the reviewers. Any product that may be evaluated in this article or claim that may be made by its manufacturer is not guaranteed or endorsed by the publisher.
Research integrity at Frontiers
Learn more about the work of our research integrity team to safeguard the quality of each article we publish.