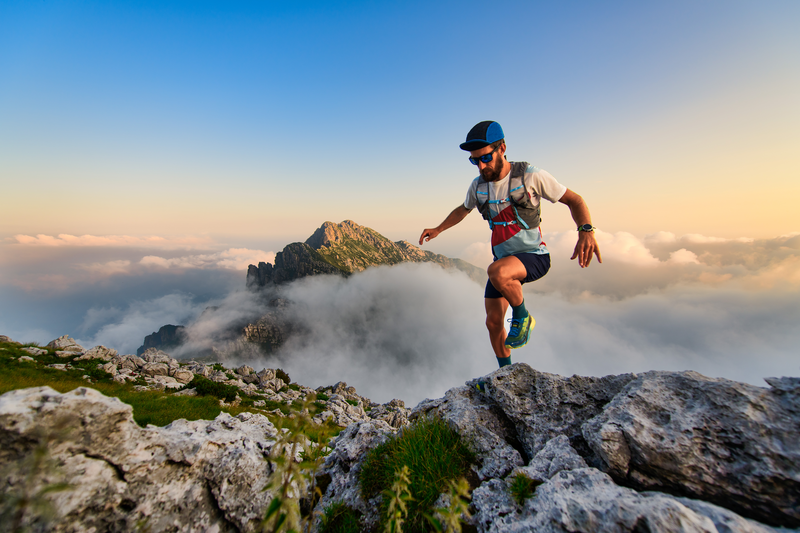
95% of researchers rate our articles as excellent or good
Learn more about the work of our research integrity team to safeguard the quality of each article we publish.
Find out more
ORIGINAL RESEARCH article
Front. Microbiol. , 30 September 2022
Sec. Microbial Physiology and Metabolism
Volume 13 - 2022 | https://doi.org/10.3389/fmicb.2022.1018008
This article is part of the Research Topic Biological Trace Element, Vitamins and Gut Microbiome View all 6 articles
Functional constipation is a common functional gastrointestinal disease. In our previous study, we found that the gut microbiota structure was disordered and the level of phenylalanine (Phe) in serum was decreased in constipated women. We conducted the present study to elucidate the role of Phe in remodeling the composition of gut microbiota and the relationship between gut microbiota and serum metabolites. Here, we demonstrated that Phe treatment significantly enhanced intestinal motility, suppressed inflammatory responses, and prevented intestinal barrier damage in rats with loperamide (Lop)-induced constipation. By metagenomic sequencing, the disbalanced gut microbial profile was analyzed in constipated rats. Phe treatment reversed changes in the abundance of several gut bacteria at the phylum, genus, and species levels. Further, we observed distinct metabolic patterns in constipated rats through targeted metabolomics and identified constipation-related gut microbial species linked to changes in circulating neurotransmitter metabolites. The abundances of species s_Lactobacillus murinus, s_Enterococcus italicus, s_Lactobacillus animalis, s_Lactobacillus apodemi, s_Enterococcus faecalis, and s_Lactobacillus backii were positively correlated with L-asparagine, L-Glutamic acid, Putrescine, and Spermidine levels. The abundances of s_Lactobacillus johnsonii and s_Butyricimonas virosa were negatively correlated with L-asparagine, L-Glutamic acid, Putrescine, and Spermidine levels. Taken together, our findings suggest that Phe can ameliorate the development of Lop-induced constipation in rats by remodeling the gut microbial community structure and changing metabolite levels.
Functional gastrointestinal diseases, also termed disorders of gut–brain interaction, are a common diagnosis in gastroenterology (Black et al., 2020). They frequently occur in combination with morphological and physiological abnormalities, including motility disturbance, visceral hypersensitivity, altered mucosal and immune function, altered gut microbiota, and altered central nervous system processing (Drossman, 2016). They lead to higher global healthcare costs (Tack et al., 2019) and reduce health-related quality of life (Wong and Drossman, 2010). According to the newly revised Rome IV standard, chronic functional constipation was defined as a disorder of intestinal brain function interaction (Drossman and Hasler, 2016). Moreover, a number of clinical studies have shown that anxiety and depression were present in patients with functional constipation (Albiani et al., 2013; Bouchoucha et al., 2014). Although the etiology of functional constipation is still unclear, the currently accepted theory is the “microbe-gut-brain axis.” Therefore, it is particularly important to explore the relationship between gut microbiota and neurotransmitters in functional constipation.
The diet, central nervous system, gut microbiota, and substances derived from the gut microbiota form a complex bidirectional network known as the brain-gut-microbiome axis, which is influenced by natural and social environments (Martin et al., 2018). If any level of this axis were disturbed, the homeostasis of the body would be disturbed, which may lead to the development of undesirable diseases, such as gastrointestinal and hepatic diseases, metabolic diseases, cardiovascular diseases, immune-related diseases, oncologic diseases, and neurologic and psychiatric diseases (Gomaa, 2020). An increasing body of proof from humans and animals suggests that there is a powerful correlation between gut microbiota and constipation based on the brain–gut–microbiome axis (Park et al., 2013; Jacobs et al., 2021; Zhang et al., 2021). Microbial treatment can alleviate the clinical symptoms of patients with functional constipation, including defecation frequency and stool consistence (Choi and Chang, 2015; Ge et al., 2016). Once we consider that the gut microbiota and their metabolites serve as a “transfer station” on this axis, it is crucial to investigate the explicit microbial mechanisms involved in disease development.
The abundance of g_Bacteroides was found to be significantly increased in constipation patients of reproductive age by 16S RNA sequencing (Li et al., 2020), but Khalif et al., (2005), who used culturing methods (mean age 42.2 years), found that the abundance of g_Bacteroides was decreased in patients with chronic constipation. Kim et al. used the real-time polymerase chain reaction (PCR) method and found that the abundance of g_Bacteroides was decreased in patients with functional constipation (mean age, 35 years; Kim et al., 2015). This discrepancy may be due to the influence of confounding factors such as age, gender, and detection methods, which lead to inconsistent or even contradictory research results. Therefore, it is necessary to consider relevant confounding factors and adopt a multi-omics research strategy to draw more reliable conclusions.
Phenylalanine (Phe) is a precursor to catecholamines (such as dopamine, norepinephrine, and epinephrine) and is essential for the biosynthesis of these neurotransmitters (Fernstrorn and Fernstrom, 2007). Multiple studies have found that Phe metabolism disorder was related to dementia, which may be due to the influence on the balance of neurotransmitters in the brain (Wissmann et al., 2013; Liu P. et al., 2021). In addition, gray matter and white matter changes were observed in the brain of IBS patients, including somatosensory systems such as thalamus and basal ganglia (Mayer et al., 2015). Furthermore, a study on aging and constipation found that both constipated and aging rats exhibited constipation and aging phenotypes, and through fecal metabolomics, it was found that there were differences in the metabolic profiles between the two groups, in which the level of Phe was increased in the constipation group, but it was decreased in the aging group (Liu X. et al., 2021). In our previous study, it was shown that Phe levels decreased in female patients of reproductive age with constipation, and the Phe metabolic pathway was abnormal (Liu et al., 2022). Thus, Phe may play an essential character in the pathophysiological process of chronic constipation, but the molecular mechanism is still unclear.
Therefore, in this study, we used female rats with loperamide (Lop)-induced constipation and intervened with Phe. We used metagenomics and metabolomics technology to analyze the role of Phe in the pathophysiological process of constipation and the potential mechanism.
Lop, L-Phe, active charcoal, and carboxymethylcellulose were purchased from Sigma-Aldrich (St. Louis, MO, United States). Hematoxylin and eosin staining kit was purchased from Beyotime (Shanghai, China).
Female 8-week-old Sprague Dawley rats were purchased from Beijing Vital River Laboratory Animal Technology (Beijing, China). Before the experiment, the animals were housed in a room at a temperature of 20–22°C and relative humidity of 60–70% under a 12/12 h light/dark cycle. The animals were randomly divided into three groups, with 15 animals in each group: the control (Ctrl) group, the Lop group, and the Lop + Phe group. As shown in Figure 1A, the Ctrl group was administered saline solution, and the Lop group was conducted 10 mg/kg Lop solution for 14 days. The Lop + Phe group was conducted 10 mg/kg Lop solution in the first week, and followed by the gavage with 10 mg/kg Lop and 25 mg/kg Phe solution in combination in the second week. All solutions were administered by gavage once a day for 14 days. All rats were killed at the end of treatment. The animal experiments were approved by the Animal Experiment Committee of the China Institute for Radiation Protection and were carried out according to the animal experiment guidelines of the China Institute for Radiation Protection.
Figure 1. Effects of Phe on defecation-related parameters and colonic tissue injury in constipated rats. (A) Experimental process. (B) Weight gain (n = 15). (C) Number of feces (n = 5). (D) Weight of wet feces (n = 5). (E) Weight of dry feces (n = 5). (F) Fecal water content (n = 5). (G) Gastrointestinal transit rate (n = 3). (H) Colonic staining using hematoxylin and eosin (n = 3). *p < 0.05 compared to Ctrl group; #p < 0.05 compared to LOP group.
Body weight was assessed weekly throughout the experimental period. In order to take differences in baseline weight into account, we calculated the weight increase in each group. After 2 weeks of Lop induction, fresh fecal pellets were gathered from each rat within 24 h. The collected fecal pellets were removed moisture in a forced ventilation oven at 60°C until the weight was constant. The fecal water content was estimated depending on the following formula:
Fecal water content (%) = [(wet weight − dry weight)/wet weight] × 100%.
Intestinal motility was estimated on the basis of the method described by Li et al (Li et al., 2015). Briefly, at the end of the 2-week intervention, the rats (n = 5 per group) were fasted for 12 h. Carbon solution was processed by dissolving 10% charcoal powder in 0.5% carboxymethylcellulose solution; the rats were administered the prepared carbon solution by gavage, and 30 min later, the rats were killed. The whole intestine of each rat was gathered to evaluate the distance of carbon movement. The formula below was used to calculate the transit ratio of intestinal movement:
Intestinal transit ratio (%) = distance of carbon powder movement/full length of small intestine × 100%.
Fresh colon tissues were fixed with 4% paraformaldehyde, dehydrated, paraffin-embedded, cut into 5-μm-thick slices, and stained with a hematoxylin and eosin staining kit (Beyotime, Shanghai, China). The histomorphological changes were observed by using a Zeiss (Germany) microscope.
The rat gut microbiome was investigated using a metagenomic shotgun sequencing approach as described previously (Zhang et al., 2020). Genome DNA was extracted from 22 fecal samples as previously described. Agarose gel electrophoresis was employed to assess the integrity and purity of DNA. Certified DNA samples were randomly broken into 350 bp fragments with a Covaris ultrasonic breaker, and the entire library was established through end repair, A-tail addition, adapter ligation, purification, and amplification. After the library was constructed, for purpose of ensuring the quality of the library, the insert size of the library was determined using an Agilent 2,100, and the appropriate concentration of the library was detected by qRT-PCR. Then, the metagenomic sequencing was performed on an Illumina PE150 sequencing platform at Shanghai Biotree Biomedical Technology (Shanghai, China) according to the manufacturer’s instructions. The raw data obtained by sequencing were subjected to quality control to acquire pure data. Pure data were assembled by metagenome, and MetaGeneMark is a common software used for gene prediction. Analyzed data were compared with the MicroNR library to get the species annotation information of UniGene. Kyoto Encyclopedia of Genes and Genomes (KEGG) metabolic pathway function annotation and abundance analysis was carried out, and cluster analysis, principal component analysis (PCA), and analysis of similarities (ANOSIM) were conducted based on species abundance.
A total of 30 serum samples from three groups were subjected to targeted metabolomic analysis. Each individual sample, 20 μl was transferred to a sterile Eppendorf tube. Then add 80 μl of extract solvent (acetonitrile with 0.1 percent formic acid, pre-cooled at −20°) to the above sample, the samples were fully vortexed for 30 s and sonicated in an ice water bath for 15 min, followed by subsiding at −40°C overnight and centrifugation at 12,000 rpm at 4°C for 15 min. Next, 80 μl of the supernatant was collected and transferred to a new Eppendorf tube, followed by addition of 40 μl carbonate solution with a concentration of 100 mM and 40 μl benzoyl chloride acetonitrile solution with a concentration of 2% and incubation for 30 min. After adding 10 μl of internal standard to the samples, which were centrifuged at 12,000 rpm for 15 min at 4°C. Next, 40 μl of supernatant was added to 20 μl H2O, and then, the samples were removed to an auto-sampler vial for ultra high performance liquid chromatography coupled with mass spectrometry (UHPLC–MS/MS) analyses.
The UHPLC–MS/MS analyses were conducted as previously described (Wong et al., 2016). The UHPLC separations were performed using an ExionLC system equipped with a Waters ACQUITY UPLC HSS T3 (100 mm × 2.1 mm, 1.8 μm). Mobile phase A was formic acid (concentration 0.1%) and ammonium acetate in water (concentration 1 mM), and mobile phase B was acetonitrile. We set the column temperature at 40°C. We set the auto-sampler temperature at 4°C, and each injection volume was 1 μl. We used an AB Sciex QTrap 6,500+ mass spectrometer for analytical development. The classic ion source parameters were set as follows: ion spray voltage: +5,000 V, curtain gas: 35 psi, temperature: 400°C, ion source gas 1 pressure: 60 psi, ion source gas 2 pressure: 60 psi. Multiple reaction monitoring data were processed using Skyline software.
Spearman correlation was analyzed to assess the association between gut microbiota and differentially expressed metabolites. The top 20 species between the Ctrl and Lop groups and 21 differentially expressed metabolites were also analyzed.
Defecation-related parameters are expressed as mean values ± standard deviation. If the data conformed to the normal distribution, a one-way ANOVA was used, followed by Bonferroni post-hoc test for multiple comparisons. Different abundances at the phylum, genus, and species levels and functional models among three groups were examined by the Kruskal–Wallis H-test. p values below 0.05 were considered to represent a significant difference.
To explore the effect of Phe on constipation, rats were pretreated with Lop for 7 days, followed by Phe for another 7 days (Figure 1A). The results showed that Phe intervention significantly inhibited the reduction of body weight of constipated rats (p < 0.05, Lop + Phe vs. Lop group; Figure 1B). In addition, the number of feces, wet feces weight, fecal water content, and gastrointestinal transit ratio were dramatically reduced in the Lop group compared with the Ctrl group (p < 0.05), but these trends were reversed by Phe intervention (p < 0.05, Lop + Phe vs. Lop group; Figures 1C–G). There was no distinction in fecal dry weight between the Lop + Phe and Lop groups (Figure 1E), and we speculated that the decrease in fecal water content was associated with wet weight. To further investigate the effect of Phe on constipation in rats, hematoxylin and eosin staining was used to evaluate the alterations of colon morphology, gut barrier, and inflammatory state. Representative hematoxylin–eosin-stained sections of the colon were shown in Figure 1H. In Lop-treated rats, crypt depth and crypt surface were disrupted, inflammatory cell infiltration was evident, and the thickness of muscle layers was decreased. However, Phe partially restored these changes.
To further investigate the relationship between Phe-relieving constipation-related parameters and changes in gut microbial composition and to reveal changes in gut microbial profile and function at the species level, metagenomic sequencing was applied to 7, 8, and 7 fecal samples of the Ctrl, Lop, and Lop + Phe groups, respectively. As shown in Figure 2A, the Ctrl group contained 1,290,097 specific genes, the Lop group contained 1,327,678 specific genes, and the Lop + Phe group contained 1,245,340 specific genes. The Shannon index and the Chao1 index were used to evaluate the alpha-diversity of gut microbiota between groups. As shown in Figures 2B,C, consistent with the specific gene results, the two indices were the highest in the Lop group and were lowest after Phe intervention, but this difference was not statistically significant. PCA at the genus level revealed a significant difference in microbial composition between the Ctrl and Lop groups, but the difference was reduced after Phe intervention (Figure 2D). At the genus level, the relative abundances of g_Lactobacillus were 0.335, 0.164, and 0.174 in the Ctrl group, Lop group, and Lop + Phe group, respectively (p < 0.05). Among the top 35 species in terms of relative abundance, the Lactobacillus intestinalis, Lactobacillus sp. ASF360, Phascolarctobacterium succinatutens, and Marvinbryantia formatexigens were significantly higher in the Lop group than in the Ctrl group, and the relative abundances of Enterococcus italicus, Lactobacillus animalis, and Lactobacillus murinus were dramatically lower in the Lop group than in the Ctrl group. However, the changes of these species showed opposite changes after Phe intervention (Kruskal–Wallis H test, Figure 2E).
Figure 2. Phe reverses dysbiosis of the gut microbiota in constipated rats, as revealed by metagenomics sequencing data. (A) Venn diagram depicting the number of shared (overlap) and unique (non-overlap) genes among the Ctrl, Lop, and Lop + Phe groups. (B,C) Alpha-diversity index for the three groups at the species level. Shannon index (B) and Chao index (C). (D) Beta diversity as determined using PCA plots. (E) Heatmap comparison between the three groups at the species level. *p < 0.05, Kruskal–Wallis H test.
For functional comparison of bacterial genes, we examined gut microbial function in the three groups. A total of 3,863,115 genes were identified in our study. Based on KEGG modules, PCA revealed that the functions of gut microbiota were different among the three groups (Figure 3A). Meanwhile, there were differences in KEGG pathways at level 1 (Figure 3B), level 2 (Figure 3C), and level 3 (Figure 3D), among the three groups. In carbohydrate metabolism, the tricarboxylic acid (TCA) cycle and pyruvate metabolism showed an increasing trend in the metabolic pathways of the Lop group, and the levels decreased after Phe administration. Amino sugar and nucleotide sugar metabolism, propanoate metabolism, galactose metabolism, fructose and mannose metabolism, the starch metabolic pathways, sucrose metabolism, and glycolysis/gluconeogenesis were decreased in the Lop group, and the levels were increased after administration of Phe. These results present a valuable molecular basis for revealing the intestinal dysfunction in constipated patients.
Figure 3. Microbial gene function prediction by KEGG analysis among the three groups. (A) PCA based on the Bray–Curtis distance of the KEGG model between the three groups. (B–D) Mean abundance of differentially enriched KEGG pathways among the three groups at level 1, level 2, and level 3. *p < 0.05, Kruskal–Wallis H test.
Overall, microbiome-related gene analysis showed that the Lop group exhibited dysfunction in multiple KEGG pathways compared to the Ctrl group, and partial improvement was observed after Phe intervention. Biodegradation of carbohydrates, amino acids, lipids, nucleotides, and xenobiotics was disrupted in the Lop group compared to the Ctrl group. The analysis further showed that the alleviation of constipation symptoms in the Lop + Phe group may partly be related to the effects of Phe on the gut microbiota and metabolites.
Microbial-derived metabolites affect the host through multiple pathways. An increasing body of evidence shows that some metabolites of gut microbiota could enter the blood and have an important impact on the host’s physiology and behavior. We previously found through untargeted metabolomics that there were differences in various neurotransmitters in the constipation group compared with the Ctrl group. Therefore, we performed targeted metabolomic analysis of neurotransmitters in 10 Ctrl, 10 Lop, and 10 Lop + Phe serum samples by UHPLC–MS/MS and explored potential relationships between gut microbiota and metabolites. The serum samples from different groups were separated according to orthogonal partial least squares discriminant analysis (OPLS-DA; Figures 4A,C). Moreover, volcano plots and KEGG cluster heatmap results of metabolites also showed differences in metabolism between groups (Figures 4B,D,E), which suggested that there were different metabolic patterns among the three groups.
Figure 4. Partial reversal of metabolic disturbances by Phe in constipated rats, as revealed by targeting metabolomic data. (A,C) OPLS-DA. (B,D) Volcano plot of differential metabolites between the Ctrl and Lop groups (B) and between the Lop and Lop + Phe groups (D). (E) Heatmap of the 21 differentially abundant metabolites between the three groups. (F) Bubble plot of metabolic pathways of differentially abundant metabolites among the three groups.
Specifically, there were differences in 21 of the 42 neurotransmitters detected by targeted metabolomics in the Lop group compared to the Ctrl group, among which Acetylcholine (Ach) levels increased 3.155 times, and the remaining 20 neurotransmitter species showed a decreasing trend (p < 0.05; Table 1). After Phe intervention, the increase in Ach levels in the Lop group was partially inhibited, and the decrease in the levels of 18 other metabolites (all except for glycine (Gly) and methionine (Met)) was partially inhibited (Table 2). Further analysis revealed that the levels of L-asparagine (Asn), L-Cysteine (Cys), 3,4-Dihydroxyphenylacetic acid (DOPAC), Epinephrine (E), 4-Aminobutyric acid (GABA), L-glutamine (Gln), L-histidine (His), lysine (Lys), Putrescine (Put), serine (Ser), and Spermidine (Spd) recovered to more than 70% of the Ctrl levels after Phe intervention. In total, 24 KEGG pathways were significantly different among the three groups (Figure 4F). Among them, the top 5 enriched pathways included aminoacyl-tRNA biosynthesis, glutathione metabolism, arginine and proline metabolism, beta-alanine metabolism, and alanine, aspartate (Asp), and glutamate metabolism.
To explore potential relationships between changes in gut microbiota and metabolites, a correlation matrix was generated using Spearman correlation. As shown in Figure 5, the abundance of species such as s_Lactobacillus murinus, s_Enterococcus italicus, s_Lactobacillus animalis, s_Lactobacillus apodemi, s_Enterococcus faecalis, and s_Lactobacillus backii, which belong to the same order as o_Lactobacillales, was positively correlated with Asn, Glu (L-Glutamic acid), Put, and Spd levels. The abundances of s_Lactobacillus johnsonii and s_Butyricimonas virosa, which belong to the same order as o_Lactobacillales and o_Bacteroidales, respectively, were negatively correlated with Asn, Glu, Put, and Spd levels.
Figure 5. Spearman correlations between metabolites and the gut microbial communities at the species level. *p < 0.05.
In recent years, gut health has received attention, and constipation is one of the most common gastrointestinal diseases (Mugie et al., 2011). Many studies have shown that there is intestinal flora disturbances in patients with constipation, but the results are inconsistent (Ohkusa et al., 2019). This may be due to the interference of constipation by multiple confounding factors such as gender, age, hormone levels, and detection methods. Therefore, it is necessary to control for confounding factors and adopt a multi-omics research strategy to draw more reliable conclusions. Phe is involved in the metabolism of various neurotransmitters, and it acts as a key mediator of host–microbe interactions and participates in host physiological activities (Krautkramer et al., 2021). Our previous study found that the serum Phe level was decreased in women of childbearing age with constipation (Liu et al., 2022). In the present study, metagenomics and targeted metabolomics were combined to explore the changes in gut microbiota and metabolism in constipated rats on the basis of controlling for the gender and age of mice.
As shown in our results, there were differences in the gut microbiota profiles among the three groups. In the present study, Phe reduced the abundance of several harmful bacteria and enriched the abundance of multiple beneficial bacteria at the species level. We found that g_Lactobacillus abundance was significantly reduced in constipated rats, which was consistent with the results of Khalif et al., (2005) earlier study in adult patients with functional constipation. However, Zoppi et al., (1998) found that g_Lactobacillus abundance was significantly elevated in constipated children, which may be connected with the different composition of gut microbiota at different ages, complicating gut microbiota research. Overall, g_Lactobacillus was involved in the development of constipation. Furthermore, this study found Lactobacillus intestinalis and Lactobacillus sp. ASF360, which belong to the g_Lactobacillus, were significantly higher in the Lop group than in the Ctrl group, the abundances of L. animalis and L. murinus were lower in the Lop group than in the Ctrl group, but these changes were reversed by Phe intervention. In addition, a randomized double-blind clinical trial confirmed that Lactobacillus reuteri DSM 17938 was effectived in children with functional constipation (Kubota et al., 2020). However, Lactobacillus reuteri was used to treat acute diarrhea in young children (Shornikova et al., 1997). These suggest that some specific Lactobacillus species may perform a key role in the pathophysiology of functional constipation.
Food proteins are hydrolyzed in the gastrointestinal tract to produce Phe, which are sensed and handled by the host and gut flora (Liu et al., 2020). Phenylalanine is mainly catabolized by Phe hydroxylase to generate tyrosine (Tyr) in the liver, which is then involved in the synthesis of neurotransmitters such as dopamine and norepinephrine, adrenal hormones, or melanin. Altered Phe and Tyr microbial metabolism is known to play a role in inflammatory bowel disease (Santoru et al., 2017). Untargeted metabolomic studies have shown that increased fecal phenylethylamine (a metabolite of phenylalanine) levels distinguish Crohn’s disease patients from controls (Jacobs et al., 2016; Santoru et al., 2017). However, in our previous study, it was found that the serum Phe level was significantly reduced in Constipated Women of Reproductive Age, and the Phe metabolic pathway was abnormal (Liu et al., 2022), suggesting that it may have different roles in different intestinal diseases, which means that the roles of gut microbiota and metabolites in intestinal diseases are complex. Combined analysis of multi-omics will allow us to better understand the mechanism of action of microbial metabolites in intestinal diseases.
Functional constipation is defined as a disorder of intestinal brain function interaction (Grundy et al., 2006), and is accompanied by anxiety and depression in IBD patients who are mainly constipated (Fond et al., 2014). Therefore, more attention should be paid to the neuropsychiatric research of constipation patients. In this study, 21 levels of 42 neurotransmitters were found to be different through targeted neurotransmitter metabolomics technology. The levels of DOPAC and E, which are related to Phe metabolism, were decreased in the Lop group than in the Ctrl group, but DOPAC returned to near-normal levels after Phe intervention, which may be the reason why we did not find any difference in Phe levels among the three groups in our study. In addition, a previous study found that there was a redox imbalance in patients with functional constipation (Hajji et al., 2020). In this study, we found that the levels of the redox-related metabolites Cys and Glutathione (GSH) were significantly decreased in the Lop group (Table 1). GSH levels were significantly increased after Phe intervention (Table 2). In addition, four neurotransmitters Asn, Glu, Put, and Spd attracted our attention, and they showed obvious positive/negative correlation with differential bacteria.
The abundances of s_Lactobacillus murinus, s_Enterococcus italicus, s_Lactobacillus animalis, s_Lactobacillus apodemi, s_Enterococcus faecalis, and s_Lactobacillus backii were positively correlated with Asn, Glu, Put, and Spd levels. The abundances of s_Lactobacillus johnsonii and s_Butyricimonas virosa were negatively correlated with Asn, Glu, Put, and Spd levels. A recent study has shown that Asp was important for amino acid homeostasis and was closely related to tumor progression through mTORC1 activity and mitochondrial respiration (Hettmer et al., 2015; Krall et al., 2021). In the present study, we found that the Asp level was significantly decreased in constipated rats and that it recovered to 70% of the normal level after Phe intervention, suggesting that Phe may affect Asp levels and hence cell energy metabolism and proliferation. Glu is the main excitatory neurotransmitter in mammals, which can be metabolized into Gln (Niciu et al., 2012). Gln has been shown to promote intestinal cell proliferation, regulate tight junction proteins, inhibit pro-inflammatory signals, and protect cells from apoptosis and cell stress in normal and pathological processes.A study has shown that Put and Spd derived from gut microbiota can be used as substrates to promote the functional hypusine modification of eIF5A (Hyp-eIF5A). Hyp-eIF5A can promote colonic epithelial cell proliferation, regulate macrophages, and maintain intestinal mucosal homeostasis (Nakamura et al., 2021). In addition, spermidine or L-arginine (Arg) exerts beneficial effects on the intestinal immunity by promoting the differentiation of T cells (Carriche et al., 2021). Epidemiological data show that the increase of dietary Spd intake is related to a decrease in the prevalence of colorectal cancer. In addition to colorectal cancer, spermidine can also regulate colitis (Vargas et al., 2015; Gobert et al., 2022). Spermine oxidase (SMOX) can promote spermidine production. Gobert et al. found that deletion of SMOX in mice aggravated dextran sulfate sodium (DSS)-induced colitis and azoxymethane (AOM) /DSS-induced colon tumorigenesis, increased α-defensin expression, and induced gut dysbiosis, while spermidine supplementation reversed these changes (Gobert et al., 2022). In the present study, it was found that the levels of Put and Spd were lower in the Lop group than the Ctrl group and that they were negatively correlated with the abundances of s_Lactobacillus johnsonii and s_Butyricimonas virosa. Put and Spd may play a key role in functional constipation by regulating intestinal inflammation and intestinal mucosa, which provides a direction for future research.
The present study demonstrated the beneficial effects of Phe on Lop-induced constipation in rats, as indicated by increased fecal water content, higher intestinal motility, reduced intestinal inflammation, and higher integrity of the intestinal mucosal barrier. In addition, Phe reshaped the gut microbiota and metabolite profiles. Our study demonstrates the potential application of Phe in the treatment of constipation.\.
The datasets presented in this study can be found in online repositories. The names of the repository/repositories and accession number(s) can be found below: NCBI PRJNA870741 and Metabolights database (MTBLS5717).
The animal study was reviewed and approved by Animal Experiment Committee of the China Institute for Radiation Protection.
XD and XSu: coordinated the project and conceived the study. CY: participated in the design and execution of the project and prepared and revised the manuscript. XB, TH, XX, XSh, XZ, and TW: collected samples. MZ: performed the statistical analysis. All authors contributed to the article and approved the submitted version.
The study was funded by the Shanxi Province “136” Revitalization Medical Project Construction Fund, and the Chinese Nutrition Society Nutrition Science Foundation-ZD Tizhi and Health Fund (CNS-ZD2020-59).
We thank Biotree (www.biotree.com.cn) for metagenomic sequencing, UHPLC–MS/MS, and bioinformatics analyses. We also thank LetPub company for its language editing service (www.letpub.com). We are very grateful to the China Institute of Radiation Protection for animal experimental support.
The authors declare that the research was conducted in the absence of any commercial or financial relationships that could be construed as a potential conflict of interest.
All claims expressed in this article are solely those of the authors and do not necessarily represent those of their affiliated organizations, or those of the publisher, the editors and the reviewers. Any product that may be evaluated in this article, or claim that may be made by its manufacturer, is not guaranteed or endorsed by the publisher.
Albiani, J. J., Hart, S. L., Katz, L., Berian, J., Del Rosario, A., Lee, J., et al. (2013). Impact of depression and anxiety on the quality of life of constipated patients. J. Clin. Psychol. Med. Settings 20, 123–132. doi: 10.1007/s10880-012-9306-3
Black, C. J., Drossman, D. A., Talley, N. J., Ruddy, J., and Ford, A. C. (2020). Functional gastrointestinal disorders: advances in understanding and management. Lancet 396, 1664–1674. doi: 10.1016/S0140-6736(20)32115-2
Bouchoucha, M., Hejnar, M., Devroede, G., Boubaya, M., Bon, C., and Benamouzig, R. (2014). Patients with irritable bowel syndrome and constipation are more depressed than patients with functional constipation. Dig. Liver Dis. 46, 213–218. doi: 10.1016/j.dld.2013.10.009
Carriche, G. M., Almeida, L., Stüve, P., Velasquez, L., Dhillon-LaBrooy, A., Roy, U., et al. (2021). Regulating T-cell differentiation through the polyamine spermidine. J. Allergy Clin. Immunol. 147, 335–348.e11. doi: 10.1016/j.jaci.2020.04.037
Choi, C. H., and Chang, S. K. (2015). Alteration of gut microbiota and efficacy of probiotics in functional constipation. J. Neurogastroenterol. Motil. 21, 004–007. doi: 10.5056/jnm14142
Drossman, D. A. (2016). Functional gastrointestinal disorders: history, pathophysiology, clinical features and Rome IV. Gastroenterology 150, 1262–1279.e2. doi: 10.1053/j.gastro.2016.02.032
Drossman, D. A., and Hasler, W. L. (2016). Rome IV-functional GI disorders: disorders of gut-brain interaction. Gastroenterology 150, 1257–1261. doi: 10.1053/j.gastro.2016.03.035
Fernstrorn, J. D., and Fernstrom, M. H. (2007). Tyrosine, phenylalanine, and catecholamine synthesis and function in the brain. J. Nutr. 137, 1539S–1547S. doi: 10.1093/jn/137.6.1539S
Fond, G., Loundou, A., Hamdani, N., Boukouaci, W., Dargel, A., Oliveira, J., et al. (2014). Anxiety and depression comorbidities in irritable bowel syndrome (IBS): a systematic review and meta-analysis. Eur. Arch. Psychiatry Clin. Neurosci. 264, 651–660. doi: 10.1007/s00406-014-0502-z
Ge, X., Tian, H., Ding, C., Gu, L., Wei, Y., Gong, J., et al. (2016). Fecal microbiota transplantation in combination with soluble dietary fiber for treatment of slow transit constipation. Arch. Med. Res. 47, 236–242. doi: 10.1016/j.arcmed.2016.06.005
Gobert, A. P., Latour, Y. L., Asim, M., Barry, D. P., Allaman, M. M., Finley, J. L., et al. (2022). Protective role of Spermidine in colitis and colon carcinogenesis. Gastroenterology 162, 813–827.e8. doi: 10.1053/j.gastro.2021.11.005
Gomaa, E. Z. (2020). Human gut microbiota/microbiome in health and diseases: a review. Antonie Van Leeuwenhoek 113, 2019–2040. doi: 10.1007/s10482-020-01474-7
Grundy, D., Al-Chaer, E. D., Aziz, Q., Collins, S. M., Ke, M., Taché, Y., et al. (2006). Fundamentals of neurogastroenterology: basic science. Gastroenterology 130, 1391–1411. doi: 10.1053/j.gastro.2005.11.060
Hajji, N., Wannes, D., Jabri, M.-A., Rtibi, K., Tounsi, H., Abdellaoui, A., et al. (2020). Purgative/laxative actions of Globularia alypum aqueous extract on gastrointestinal-physiological function and against loperamide-induced constipation coupled to oxidative stress and inflammation in rats. Neurogastroenterol. Motil. 32:e13858. doi: 10.1111/nmo.13858
Hettmer, S., Schinzel, A. C., Tchessalova, D., Schneider, M., Parker, C. L., Bronson, R. T., et al. (2015). Functional genomic screening reveals asparagine dependence as a metabolic vulnerability in sarcoma. elife 4:e09536. doi: 10.7554/eLife.09436
Jacobs, J. P., Goudarzi, M., Singh, N., Tong, M., McHardy, I. H., Ruegger, P., et al. (2016). A disease-associated microbial and metabolomics state in relatives of pediatric inflammatory bowel disease patients. Cell. Mol. Gastroenterol. Hepatol. 2, 750–766. doi: 10.1016/j.jcmgh.2016.06.004
Jacobs, J. P., Gupta, A., Bhatt, R. R., Brawer, J., Gao, K., Tillisch, K., et al. (2021). Cognitive behavioral therapy for irritable bowel syndrome induces bidirectional alterations in the brain-gut-microbiome axis associated with gastrointestinal symptom improvement. Microbiome 9:236. doi: 10.1186/s40168-021-01188-6
Khalif, I. L., Quigley, E. M. M., Konovitch, E. A., and Maximova, I. D. (2005). Alterations in the colonic flora and intestinal permeability and evidence of immune activation in chronic constipation. Dig. Liver Dis. 37, 838–849. doi: 10.1016/j.dld.2005.06.008
Kim, S.-E., Choi, S. C., Park, K. S., Park, M. I., Shin, J. E., Lee, T. H., et al. (2015). Change of fecal Flora and Effectiveness of the short-term VSL#3 probiotic treatment in patients with functional constipation. J. Neurogastroenterol. Motil. 21, 111–120. doi: 10.5056/jnm14048
Krall, A. S., Mullen, P. J., Surjono, F., Momcilovic, M., Schmid, E. W., Halbrook, C. J., et al. (2021). Asparagine couples mitochondrial respiration to ATF4 activity and tumor growth. Cell Metab. 33, 1013–1026.e6. doi: 10.1016/j.cmet.2021.02.001
Krautkramer, K. A., Fan, J., and Bäckhed, F. (2021). Gut microbial metabolites as multi-kingdom intermediates. Nat. Rev. Microbiol. 19, 77–94. doi: 10.1038/s41579-020-0438-4
Kubota, M., Ito, K., Tomimoto, K., Kanazaki, M., Tsukiyama, K., Kubota, A., et al. (2020). Lactobacillus reuteri DSM 17938 and magnesium oxide in children with functional chronic constipation: a double-blind and randomized clinical trial. Nutrients 12:225. doi: 10.3390/nu12010225
Li, H., Chen, J., Ren, X., Yang, C., Liu, S., Bai, X., et al. (2020). Gut microbiota composition changes in constipated women of reproductive age. Front. Cell. Infect. Microbiol. 10:557515. doi: 10.3389/fcimb.2020.557515
Li, C., Nie, S.-P., Zhu, K.-X., Xiong, T., Li, C., Gong, J., et al. (2015). Effect of lactobacillus plantarum NCU116 on loperamide-induced constipation in mice. Int. J. Food Sci. Nutr. 66, 533–538. doi: 10.3109/09637486.2015.1024204
Liu, Y., Hou, Y., Wang, G., Zheng, X., and Hao, H. (2020). Gut microbial metabolites of aromatic amino acids as signals in host-microbe interplay. Trends Endocrinol. Metab. 31, 818–834. doi: 10.1016/j.tem.2020.02.012
Liu, S., Yang, C., Li, H., Bai, X., Hu, T., Xue, X., et al. (2022). Alteration of serum metabolites in women of reproductive age with chronic constipation. Med. Sci. Monit. 28:e934117. doi: 10.12659/MSM.934117
Liu, P., Yang, Q., Yu, N., Cao, Y., Wang, X., Wang, Z., et al. (2021). Phenylalanine metabolism is Dysregulated in human hippocampus with Alzheimer's disease related pathological changes. J. Alzheimers Dis. 83, 609–622. doi: 10.3233/JAD-210461
Liu, X., Zhao, D., Zhao, S., Li, Z., Wang, Y., and Qin, X. (2021). Deciphering the correlations between aging and constipation by metabolomics and network pharmacology. Aging 13, 3798–3818. doi: 10.18632/aging.202340
Martin, C. R., Osadchiy, V., Kalani, A., and Mayer, E. A. (2018). The brain-gut-microbiome Axis. Cell. Mol. Gastroenterol. Hepatol. 6, 133–148. doi: 10.1016/j.jcmgh.2018.04.003
Mayer, E. A., Gupta, A., Kilpatrick, L. A., and Hong, J.-Y. (2015). Imaging brain mechanisms in chronic visceral pain. Pain Suppl. 156, S50–S63. doi: 10.1097/j.pain.0000000000000106
Mugie, S. M., Benninga, M. A., and Di Lorenzo, C. (2011). Epidemiology of constipation in children and adults: a systematic review. Best Pract. Res. Clin. Gastroenterol. 25, 3–18. doi: 10.1016/j.bpg.2010.12.010
Nakamura, A., Kurihara, S., Takahashi, D., Ohashi, W., Nakamura, Y., Kimura, S., et al. (2021). Symbiotic polyamine metabolism regulates epithelial proliferation and macrophage differentiation in the colon. Nat. Commun. 12:2105. doi: 10.1038/s41467-021-22212-1
Niciu, M. J., Kelmendi, B., and Sanacora, G. (2012). Overview of glutamatergic neurotransmission in the nervous system. Pharmacol. Biochem. Behav. 100, 656–664. doi: 10.1016/j.pbb.2011.08.008
Ohkusa, T., Koido, S., Nishikawa, Y., and Sato, N. (2019). Gut microbiota and chronic constipation: a review and update. Front. Med. 6:19. doi: 10.3389/fmed.2019.00019
Park, A. J., Collins, J., Blennerhassett, P. A., Ghia, J. E., Verdu, E. F., Bercik, P., et al. (2013). Altered colonic function and microbiota profile in a mouse model of chronic depression. Neurogastroenterol. Motil. 25, 733–e575. doi: 10.1111/nmo.12153
Santoru, M. L., Piras, C., Murgia, A., Palmas, V., Camboni, T., Liggi, S., et al. (2017). Cross sectional evaluation of the gut-microbiome metabolome axis in an Italian cohort of IBD patients. Sci. Rep. 7:9523. doi: 10.1038/s41598-017-10034-5
Shornikova, A. V., Casas, I. A., Isolauri, E., Mykkänen, H., and Vesikari, T. (1997). Lactobacillus reuteri as a therapeutic agent in acute diarrhea in young children. J. Pediatr. Gastroenterol. Nutr. 24, 399–404. doi: 10.1097/00005176-199704000-00008
Tack, J., Stanghellini, V., Mearin, F., Yiannakou, Y., Layer, P., Coffin, B., et al. (2019). Economic burden of moderate to severe irritable bowel syndrome with constipation in six European countries. BMC Gastroenterol. 19:69. doi: 10.1186/s12876-019-0985-1
Vargas, A. J., Ashbeck, E. L., Wertheim, B. C., Wallace, R. B., Neuhouser, M. L., Thomson, C. A., et al. (2015). Dietary polyamine intake and colorectal cancer risk in postmenopausal women. Am. J. Clin. Nutr. 102, 411–419. doi: 10.3945/ajcn.114.103895
Wissmann, P., Geisler, S., Leblhuber, F., and Fuchs, D. (2013). Immune activation in patients with Alzheimer's disease is associated with high serum phenylalanine concentrations. J. Neurol. Sci. 329, 29–33. doi: 10.1016/j.jns.2013.03.007
Wong, R. K. M., and Drossman, D. A. (2010). Quality of life measures in irritable bowel syndrome. Expert Rev. Gastroenterol. Hepatol. 4, 277–284. doi: 10.1586/egh.10.19
Wong, J.-M. T., Malec, P. A., Mabrouk, O. S., Ro, J., Dus, M., and Kennedy, R. T. (2016). Benzoyl chloride derivatization with liquid chromatography-mass spectrometry for targeted metabolomics of neurochemicals in biological samples. J. Chromatogr. A 1446, 78–90. doi: 10.1016/j.chroma.2016.04.006
Zhang, Y., Gu, Y., Ren, H., Wang, S., Zhong, H., Zhao, X., et al. (2020). Gut microbiome-related effects of berberine and probiotics on type 2 diabetes (the PREMOTE study). Nat. Commun. 11:5015. doi: 10.1038/s41467-020-18414-8
Zhang, S., Wang, R., Li, D., Zhao, L., and Zhu, L. (2021). Role of gut microbiota in functional constipation. Gastroenterol Rep (Oxf). 9, 392–401. doi: 10.1093/gastro/goab035
Keywords: phenylalanine, constipation, gut microbiota, metagenomics, metabolomics, neurotransmitter
Citation: Yang C, Bai X, Hu T, Xue X, Su X, Zhang X, Wu T, Zhang M, Shen X and Dong X (2022) Integrated metagenomics and targeted-metabolomics analysis of the effects of phenylalanine on loperamide-induced constipation in rats. Front. Microbiol. 13:1018008. doi: 10.3389/fmicb.2022.1018008
Received: 12 August 2022; Accepted: 12 September 2022;
Published: 30 September 2022.
Edited by:
Khaled Abouelezz Fouad Mohammed, Assiut University, EgyptCopyright © 2022 Yang, Bai, Hu, Xue, Su, Zhang, Wu, Zhang, Shen and Dong. This is an open-access article distributed under the terms of the Creative Commons Attribution License (CC BY). The use, distribution or reproduction in other forums is permitted, provided the original author(s) and the copyright owner(s) are credited and that the original publication in this journal is cited, in accordance with accepted academic practice. No use, distribution or reproduction is permitted which does not comply with these terms.
*Correspondence: Xiaobing Shen, eGIuc2hlbkBzZXUuZWR1LmNu; Xiushan Dong, ZG9uZ3hpdXNoYW4yMDEyQDE2My5jb20=
Disclaimer: All claims expressed in this article are solely those of the authors and do not necessarily represent those of their affiliated organizations, or those of the publisher, the editors and the reviewers. Any product that may be evaluated in this article or claim that may be made by its manufacturer is not guaranteed or endorsed by the publisher.
Research integrity at Frontiers
Learn more about the work of our research integrity team to safeguard the quality of each article we publish.