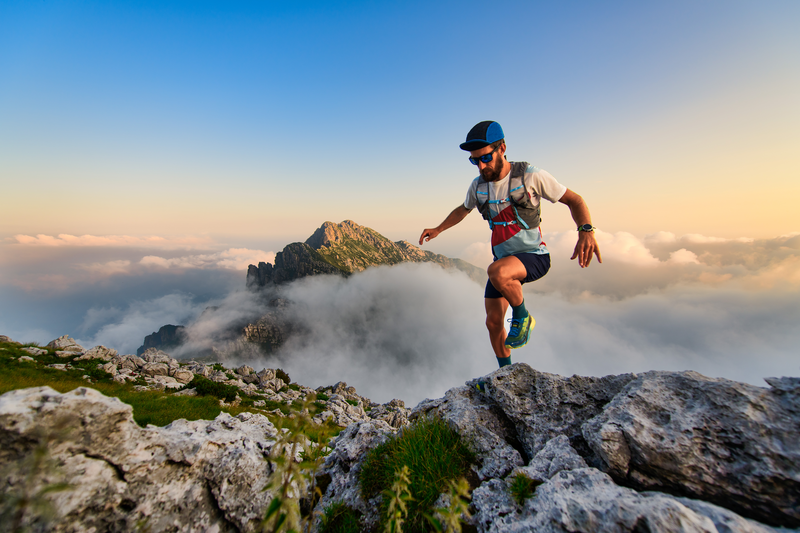
95% of researchers rate our articles as excellent or good
Learn more about the work of our research integrity team to safeguard the quality of each article we publish.
Find out more
ORIGINAL RESEARCH article
Front. Microbiol. , 23 December 2022
Sec. Microbiotechnology
Volume 13 - 2022 | https://doi.org/10.3389/fmicb.2022.1015613
This article is part of the Research Topic Microbial Nanotechnology: A New Frontier in Microbiology View all 6 articles
Heavy metal accumulation and pathogenic bacteria cause adverse effects on aquaculture. The active surface of selenium (Se) nanoparticles can mitigate these effects. The present study used Se-resistant Bacillus subtilis AS12 to fabricate biological Se nanoparticles (Bio-SeNPs). The double-edged Bio-SeNPs were tested for their ability to reduce the harmful effects of heavy metals and bacterial load in Nile tilapia (Oreochromis niloticus) and their respective influences on fish growth, behavior, and health. The Bio-SeNPs have a spherical shape with an average size of 77 nm and high flavonoids and phenolic content (0.7 and 1.9 g g−1 quercetin and gallic acid equivalents, respectively), resulting in considerable antioxidant and antibacterial activity. The Bio-SeNPs (3–5 μg ml−1) in the current study resolved two serious issues facing the aquaculture industry, firstly, the population of pathogenic bacteria, especially Aeromonas hydrophilia, which was reduced by 28–45% in fish organs. Secondly, heavy metals (Cd and Hg) at two levels (1 and 2 μg ml−1) were reduced by 50–87% and 57–73% in response to Bio-SeNPs (3–5 μg ml−1). Thus, liver function parameters were reduced, and inner immunity was enhanced. The application of Bio-SeNPs (3–5 μg ml−1) improved fish gut health, growth, and behavior, resulting in fish higher weight gain by 36–52% and a 40% specific growth rate, compared to controls. Furthermore, feeding and arousal times increased by 20–22% and 28–53%, respectively, while aggression time decreased by 78% compared to the control by the same treatment. In conclusion, Bio-SeNPs can mitigate the accumulation of heavy metals and reduce the bacterial load in a concentration-dependent manner, either in the fish media or fish organs.
Fish and fish products are important sources of amino acids and micronutrients for health and a well-balanced diet. Rising demands for animal protein are usually met with an increase in intensive farming and production, which contributes to negative environmental effects. However, aquaculture faces increased challenges due to exotic and endemic epizootic diseases and the shifting climate (Abu-Elala et al., 2020).
Many fish farms use agricultural drainage water, which is usually full of fertilizers, pesticides, and toxic heavy metals such as cadmium (Cd), mercuric (Hg), lead (Pb), copper (Cu), and zinc (Zn; Omar et al., 2012). Bosch et al. (2016) found that fish products usually have several heavy elements, especially Hg and other pollutants. For example, Cd is both non-biodegradable and non-digestible and is consequently directly integrated into the food chain (Bosch et al., 2016). Hg tightly binds to proteins within fish tissues, where it hyper concentrates, often as “methylmercury,” a highly toxic organic compound. Cd and Hg induce free radicals in fish, damaging antioxidative defenses and increasing lipid peroxidation (Negahdari et al., 2021).
Also, Cd and Hg are toxic to the immune and nervous systems, genes, kidneys, and cells and cause cancer in other organisms, including humans (El Nemr et al., 2016). There are ongoing studies in search of novel antioxidants and antimicrobial agents with the potential to provide fish with the required nutrients to simultaneously improve growth performance while also promoting their defense and immunological systems (Dawood et al., 2019).
Selenium (Se) is a fundamental element in enzymatic and non-enzymatic systems that protects the cellular membrane against oxidative damage (Talas et al., 2008). Additionally, it is a vital micronutrient in fish food, promoting growth and fertility (Longbaf Dezfouli et al., 2019). Despite Se′s physiological, biochemical, and immunological benefits for fish, it is a highly toxic element (Mechlaoui et al., 2019). Nanotechnology offers novel solutions for making selenium safe and bioavailable for aquatic organisms in the form of selenium nanoparticles (SeNPs) because of the small size of Se in nanoparticles (Shaalan et al., 2020; El-Adawy et al., 2021; Lang et al., 2021).
SeNPs have shown outstanding bioavailability and low toxicity levels (Menon et al., 2018). They can exert envisioned activity at much lower concentrations than organic or inorganic Se compounds. Further, SeNPs exhibit biological activities that promote optimum substitutions for various seleno-compounds (Yanhua et al., 2016). Including SeNPs in fish diets releases safe Se, which acts as a nutritional additive and promotes growth performance (Ren et al., 2021).
There are many routes to produce SeNPs, including chemical and physical methods. SeNPs have a low cytotoxicity level, as the nanoparticles destroy cancerous cells by releasing more reactive oxygen species (ROS) into cancer cells rather than normal cells. Therefore, it is being investigated for integration into nanomedicine applications for accelerating wound healing (Kumar et al., 2015; Skalickova et al., 2017).
However, these methods have many problems, i.e., toxic chemicals and high energy consumption. Therefore, nanoparticles have emerged as eco-friendly and green synthesis methods (Salem, 2022a). High pressure or temperature is not required for the green synthesis of NPs, and the use of toxic and hazardous substances and the addition of external reducing, stabilizing, or capping agents are avoided (Salem and Fouda, 2021).
Several studies used bacteria for biological synthesis of nanoparticles, i.e., Lactobacillus paracasei HM1 (El-Saadony et al., 2021a), Bacillus subtilis AL43 (Abdel-Moneim et al., 2022), and Lactobacillus acidophilus ML14 (El-Saadony et al., 2021b). Additionally, fungi such as Aspergillus oryzae (Mosallam et al., 2018) and Mariannaea sp. were also used (Zhang et al., 2019).
This “green” (biological) synthesis of SeNPs ensures its safe use in many biomedical applications, with no side effects (Alagesan and Venugopal, 2019). Also, it was used in water and wastewater treatment, carbon nanotubes, metal oxides (Salem et al., 2022a), and in agriculture as nano-fertilizers (El-Saadony et al., 2021e). SeNPs exhibited antioxidant, antimicrobial, antiviral, and anti-tumor activities (Hariharan and Dharmaraj, 2020). They also have insecticidal activity (Salem et al., 2021; Hashem et al., 2022) and have antifouling against Pseudomonas aeruginosa, Staphylococcus aureus, and Candida albicans (Abu-Elghait et al., 2021; Salem et al., 2022b).
SeNPs are protective agents against heavy metals such as Chromium (Cr), Cd, and Hg, as well as chemotherapeutic agents with adverse effects through the generation of ROS. SeNPs may activate caspase-3 and enhance poly (ADP-ribose) polymerase-1 (PARP) cleavage, leading to mitochondria-mediated apoptosis through stabilizing DNA and neutralizing ROS production (Kiełczykowska et al., 2018; Khurana et al., 2019; Sonkusre, 2020).
The SeNPs, produced from Na2SeO3 or seleno-methionine, have been reported to promote efficient growth performance and antioxidant defense systems of Cyprinus carpio based on their bioavailability (Saffari et al., 2018). SeNPs enhance physiological parameters (lysozyme activity, hemoglobin concentration, red blood cells (RBC) counts, and hematocrit value). Besides, promoting biochemical parameters in fish organs growth hormone level in serum, total proteins in tissue, and peroxidase and glutathione activities in the liver and muscles (Khan et al., 2016).
Dawood et al. (2019) confirmed that SeNPs promoted the immune system in Red Sea Bream by increasing skin mucus, serum protein, and reducing salinity tolerance by increasing the populations and activities of T-cells and NK cells (Avery and Hoffmann, 2018). Simultaneously, Se was reported to have substantial antioxidant activity (Saffari et al., 2017).
No studies yet, have investigated the effects of double-edged Bio-SeNPs synthesized by bacteria to simultaneously reduce the harmful effects of heavy metals and microbial load on Nile tilapia (Oreochromis niloticus) and to delineate their influences on fish growth, behavior, and health. In continuation with the authors’ previous research on green nanotechnologies (El-Saadony et al., 2021c), the current study attempts to introduce new green nanotechnology; in addition, the optimum conditions for producing Bio-SeNPs and the transformation of Bio-SeNPs was chemically and biologically evidenced, then characterized. Furthermore, we demonstrated their antioxidant and antibacterial properties. Consequently, Bio-SeNPs were included in Nile tilapia media to counteract heavy metal and microbial accumulation in fish. Then light was casted on fish growth and immunological effects of dietary Bio-SeNPs. The prospective role of Bio-SeNPs in mitigating oxidative status and the adverse effects of toxic Hg and Cd, and the microbial contamination on Nile tilapia was also studied.
Soil samples were collected in sterile bags from soils polluted with industrial wastewater near the Ismailia Canal (30°0.11′02.054” N, 31°0.27′87.466″ E), Ismailia, Egypt.
All bacteriological media, Plate Count Agar (PCA), nutrient broth (NB), Luria-Bertani (LB), enrichment medium (EM), plate count broth (PCB), and tryptic soy broth (TSB), Mueller Hinton agar (MHA), Mueller Hinton broth (MHB) were purchased from Lab M Limited, Lancashire, United Kingdom. All other chemicals; NaCl, sodium selenite (Na2SeO3), HCl, potassium iodine, starch indicator 1,1-diphenyl-2-picrylhydrazyl (DPPH), ethanol, 2,2-azino-bis-3-ethylbenzothiazoline-6-sulfonic acid (ABTS), peptone, and Tret-Butyl hydroquinone (TBHQ) were of analytical grade and obtained from Sigma-Aldrich Chemie GmbH, Taufkirchen, Germany.
The bacterial isolates, Staphylococcus aureus, Bacillus cereus, Listeria monocytogenes, Escherichia coli, Aeromonas hydrophilia, and Klebsiella pneumonia were obtained from the Agricultural Microbiology Department, Faculty of Agriculture, Zagazig University, Zagazig, Egypt.
Luria-Bertani (LB) broth [prepared by dissolving 10 g tryptone, 5 g yeast extract, and 5 g sodium chloride in 1000 ml distilled water (pH 7.5)]. Enrichment medium (EM) broth (was prepared by dissolving 0.5 g sodium nitrate, 5 g sodium chloride, 0.1 g ammonium chloride, 2.7 g di-potassium hydrogen phosphate, 3 g tryptone, 1 g beef extract, 0.5 g yeast extract, and 3 g glucose in 1000 ml distilled water).
For selecting Se-tolerant bacterial isolates, the collected soil samples were stored at 4°C until transport to the laboratories of the Agricultural Microbiology Department, Faculty of Agriculture, Zagazig University, Egypt, for immediate processing. The soil samples were used to isolate selenium-resistant bacteria. Twenty-five grams of soil samples were homogenized in of sterile saline peptone water (1 g l−1 peptone and 8.5 g l−1 NaCl) for 10 min at 25°C to prepare a 10−1 dilution.
One ml of the previous dilution was added to a 9 ml sterile saline peptone water tube to obtain a 10−2 dilution. Further serial dilution to 10−7. Aliquots (100 μl) of each dilution was spread across the surface of Plate Count Agar (PCA) plates supplemented with 1, 2, 3, 4, 5, and 6 mM Na2SeO3 in sterilized plastic petri dishes (90 mm diameter) using sterilized L-shaped spreaders. For each dilution, three plates were used for each sample. The plates were incubated for 1 day at 30°C; the red colonies were then selected based on their efficiency in reducing sodium selenite (Srivastava and Kowshik, 2016).
Selenium-tolerant bacteria were identified by microscopic examination. The microscopic images of bacteria were compared with the morphological and biochemical tests defined in the Bergy manual. Further identification was made by MALDI-TOF spectroscopy (Bruker Daltonics, Bremen, Germany). Phylogenetic analysis was performed using the neighbor-joining method (Saitou and Nei, 1987). The MEGA-X software was used to reconstruct a neighbor-joining tree (Tamura et al., 2004; Kumar et al., 2018) using data imported from the GenBank database.1
An aliquot (100 μl) of the selected bacterial suspension (108 CFU) was inoculated in 100 ml of Luria-Bertani (LB) broth and incubated in a shaking incubator at 30°C and 180 rpm for 48 h. It was then centrifuged at 8,000 rpm for 20 min. The supernatant was discarded, and the bacterial pellets were homogenized in 100 ml of the Enrichment medium (EM) broth supplemented with 5.0 mM of Na2SeO3. The mixture was then incubated at 30°C for 3 days in a shaking incubator of 180 rpm. The conversion in the flask color (EM medium containing Na2SeO3 and the tested bacterium) from bright yellow to red at the end of the incubation period confirms the transformation of Na2SeO3 to Bio-SeNPs by the selected isolate. The flask that contains only Na2SeO3 and the flask that contains only bacterial pellets without Na2SeO3 (5 mM) were kept under the same conditions. The Bio-SeNPs were extracted from the bacterial pellets through autoclaving until the explosion of the bacterial membrane; the resulting solution containing Bio-SeNPs was centrifuged at 15,000 rpm for 30 min, the supernatant was subsequently obtained, and the exploded cells were suspended (Fesharaki et al., 2010).
The biosynthesis of Bio-SeNPs were optimized individually using several parameters, namely: (i) various mediums, including NB, LB, EM, PCB, and TSB; (ii) reaction time on different days (1, 2, 3, 4, and 5 days); (iii) temperature (15, 20, 25, 30, and 35°C); (iv) pH (5, 6, 7, 8, and 9); and (v) and agitation speed (100, 140, 180, 220, and 260 rpm). The particle size of the Bio-SeNPs was estimated using the dynamic light scattering (DLS) technique.
A specific amount (1.9 g) of Na2SeO3 was homogenized in 1,000 ml distilled water to prepare a standard stock solution. The HCl (2 N) solutions and potassium iodine 2% were prepared as a starch indicator. Additionally, numerous concentrations of stock solutions were prepared. An aliquot (100 μl) of stock solution was placed in test tubes before adding 1 ml of HCl and 1 ml of potassium iodine 2% and homogenizing until a brown-yellow color appeared. The Se content was calculated by applying the absorbance in a standard curve equation y = 0.6633x + 0.0015 to acquire the amount in Bio-SeNPs. The same conditions were applied, in parallel, to Na2SeO3 and Bio-SeNPs. The starch indicator was added to all tubes. The obtained color was read at 644 nm using a microtiter plate reader (BioTek Elx808, United States).
Bio-SeNPs were characterized according to Abdel-Moneim et al. (2022) and El-Saadony et al. (2021a). The shape and average size of Bio-SeNPs were assessed by transmission electron microscopy images (TEM; JEOL 1010, Japan). The absorption spectra of the synthesized Bio-SeNPs were carried out by UV–visible spectrophotometer in the range of 200–1,000 nm (Mettler-Toledo LLC., Columbus, OH, USA). The exact size and surface charge of Bio-SeNPs were estimated using a Zeta sizer analyzer and the Zeta potential, respectively (Nano Z2 Malven, Malvern Hills, United Kingdom). The active components in Bio-SeNPs were detected by Fourier transform-infrared (FTIR) spectroscopy (Bruker Tensor 37, Kaller Germany) at a wavelength range of 500–3,500 cm−1.
The radical scavenging activity of Bio-SeNPs at different concentrations (0.5, 1, 2, 3, 4, and 5 μg ml−1) was estimated according to Bhakya et al. (2016), with some modificationss. A volume (100 μl) of each concentration was mixed with an equal volume of DPPH in ethanol and placed in the microtiter plate. Absorbance was read after 30 min, at 517 nm, using a microtiter plate reader (BioTek Elx808, United States). The absorbance was then applied using Eq. (1) as follows:
The antioxidant activity of Bio-SeNPs was estimated following the methods outlined by Gil et al. (2002), with slight modifications. Briefly, 100 μl of Bio-SeNPs concentrations (0.5, 1, 2, 3, 4, and 5 μg ml−1) were homogenized in an equal volume of 0.1 mM ABTS, in a microtiter plate. The absorbance was subsequently estimated after 30 min at 745 nm, using a microtiter plate reader (BioTek Elx808, United States).
TBHQ and ABTS were used as controls. The % inhibition of radicals was calculated using Eq. (2).
The antibacterial activity of Bio-SeNPs was estimated using the disc diffusion method (Saad et al., 2021). In brief, 100 μl of bacterial inoculum (1×108 CFU ml−1) was spread over the surface of an MHA plate. Sterilized paper discs (6 mm) were prepared and saturated with Bio-SeNPs concentrations of (0.5, 1, 2, 3, 4, and 5 μg ml−1), then placed at the sides of MHA plates. Discs saturated with sterilized distilled water served as the control. All plates were incubated at 37°C for 1 day. The inhibition zones around the discs were measured and recorded (mm).
The control was a tube containing 9 ml of Mueller Hinton Broth (MHB) inoculated with the bacterial inoculum. A 500 μl of each Bio-SeNPs level was mixed in 9 ml of MHB tubes previously inoculated with 500 μl of the bacterial inoculum. All tubes were incubated for 24 h at 37°C. The minimum inhibitory concentration (MIC) and the minimum bactericidal concentration (MBC) of Bio-SeNPs were estimated following Saad et al. (2021). The MIC was recorded as the lowest concentration of Bio-SeNPs, inhabiting bacterial growth. The MBC of Bio-SeNPs was determined by spreading 100 μl of MIC tubes over new MHA plates while observing the bacterial growth after a day of incubation at 37°C.
This trial was carried out at the Fish Research Unit, Faculty of Veterinary, Cairo University, Egypt. The total duration of this trial was 10 weeks. All procedures in the study followed international ethical standards. The research involved no human participants. All experimental groups were fed on a basal diet.
A group of 120 healthy (The fish was judged healthy from its overall normal morphology, feeding behavior, active breathing, active movement, brightness, and clear eyes) Nile tilapia (Oreochromis niloticus) fish with an initial weight (60 g) were purchased from the Fish Research Central Lab., Ministry of Agriculture, Egypt. Fish were acclimated in glass aquaria containing dechlorinated water for 2 weeks. Water parameters were checked weekly. The quality of water General water parameters were monitored and stabilized; the levels of sodium, potassium, magnesium, and calcium in the water were adjusted to 0.4, 0.05, 0.6, and 0.8 mmol L-1, respectively. At a temperature of 25.4 ± 0.3°C, a dissolved oxygen level of 7.30 ± 0.13 mg/l, a pH of 7.90 ± 0.06, a total hardness level of 132 ± 4.4 mg CaCO3/L, ammonia (NH3) from 0.03 to 0.038 mg/l, total salinity of 23.41 ppt, total ammonia nitrogen 0.004 ± 0.001 g/l, and a photoperiod regime (12:12 h light: dark).
During acclimatization and experimentation, all groups were fed on a basal diet (20% soybean meal, corn 23.0%, fish meal 15.0%, alfalfa hay 14.0%, wheat bran 13.0%, corn gluten meal 11.5%, sunflower oil 1, 1% vitamin mixture, 0.5% mineral mixture, and 1% carboxymethyl cellulose). The approximate analysis of the diet was 30.32% crude protein, 3.53% crude fibers, 1.79% lysine, 0.74% methionine, 0.97% calcium, and 0.86% available phosphorus, with a 9.91% energy expenditure, and a total of 2,905.93 Kcal/Kg of digestible energy.
A group of 120 healthy Nile tilapia fish with an initial weight of 60 g was divided into three main groups; the first group was kept in freshwater, while the second and third groups were subjected to heavy metal-contaminated water (Cd and Hg), respectively. Each group was further subdivided into four subgroups (Z, A, B, and C), each receiving Bio-SNP at 0, 3, 4, and 5 μg ml−1. The subgroups for group 1 were 1Z, 1A, 1B, and 1C (Scheme 1). This nomination was consistent for all three groups, with the first digit referring to the group and the second letter to the subgroup. The control in the first group (1Z) was incubated in tap water and received neither heavy metals nor Bio-SeNPs. In contrast, the controls of the second and third groups (2Z and 3Z) received heavy metals at 0.5 + 0.5 Hg mg/L and 1.0 + 1.0 Cd + Hg mg/L, respectively, but without any treatment (Scheme 1). Experimental fish were treated as previously described for the experimental period, a total of 10 weeks (Section “Antioxidant activity”).
The doses of Cd and Hg were inferior to the maximum permissible limit approved by the FDA. The fish were exposed to a semi-static water system through a daily siphoning of 60% in the morning and 40% in the evening, then replaced with fresh water the following day. Levels of the heavy metals and Bio-SeNPs were re-adjusted to the starting values.
Fish were marked with short plastic strips applied to the dorsal fin. The observation technique followed a focal sample procedure for 15 s intervals during 1 h videotaping using a software observer (Phillips et al., 2018). The observed behavior pattern was recorded for all tested behavioral patterns 3 h weekly. Additionally, the mean time and frequency of each behavior were recorded.
Fish behavior was estimated according to the methods described by Neetha et al. (2022). Feeding behavior was recorded based on methods described by Alonso and Valle-Torres (2018). Foraging behavior was defined by fish searching for food while “grazing” within the aquarium. Surfacing behavior was distinguished by how often and how long the fish rose to the surface to breathe. The swimming behavior of fish included rapid and slow movement in the water without any other activities (Bégout Anras et al., 2004). The resting behavior was estimated when fish were not active in the aquaria and without an open eye (Sekiguchi and Kohshima, 2003). Body care behavior includes the time and frequency of lateral body shaking as either rapid movements or scratching of the body against any object (Coppedge et al., 1997; Sekiguchi and Kohshima, 2003).
Aggressive behavior was defined as fish vigorously chasing another group of fish (Molano et al., 2013). Agonistic behavior was considered fin tugging, i.e., biting another fish’s fin and butting the genital papilla. In addition, arousal behavior included movement activity without actual swimming or surfacing behavior.
Five fish per aquarium were collected, washed, and weighed for the final body composition at 20°C. Performance traits, including weight gain (WG), percentage specific growth rate (SGR) per day, total feed intake (TFI), feed conversion ratio (FCR), and percentage survival rate (SR), were calculated according to Eqs. 3–7.
Initial weight (W1) and final weight (W2), as well as acclimation days (T) were recorded during the feeding duration (70 days).
Three fish per aquarium were dissected, and their skin, muscle, intestine, and gills were removed and kept at −20°C for microbial analysis. Another three fish per aquarium were collected for detecting Cd and Hg content, then the liver, intestine, gills, and kidney were extracted and kept in formalin (10%) until reaching the laboratory. The water samples were collected daily from the aquarium, then homogenized to take a representative sample for analysis.
For the biochemical study, three fish per aquarium were collected. Then, 3 ml of caudal vein blood samples were taken using a heparinized needle and kept in sterile Eppendorf tubes at 4°C. All tubes were centrifuged (Sigma 3-30 K, Germany) at 10,000 rpm for 5 min at 4°C to collect sera. All blood biochemical parameters were measured using kits from Bio-Diagnostic Co. (Dokki, Cairo, Egypt) as described by Al-Deriny et al. (2020) and Dawood et al. (2020). All parameters were estimated at a respected wavelength using a microtiter plate reader (BioTek Elx808, United States).
A nitro blue tetrazolium (NBT) reagent was used to determine respiratory system failure. The levels of blood glucose (GLU), and liver biomarkers, i.e., alanine aminotransferase (ALT), and aspartate aminotransferase (AST), were measured. Additionally, the activities of lysozyme (LYZ), testosterone, progesterone, follicle-stimulating hormone (FSH), growth hormone (GH), and cortisol were evaluated in sera.
Frozen fish samples were thawed and dissected with stainless steel instruments to separate fish organs, liver, kidneys, gills, and muscles. A 5 g of fish organs were taken and digested with concentrated nitric acid for all organs except the gills with a nitric and perchloric acid (4:1) mixture at a temperature of 100°C until reaching a transparent solution.
Cd and Hg were estimated using an atomic absorption Spectrophotometer (Model AAS GPC A932, Version 1.1). The obtained solution was diluted to a known volume with deionized distilled water. Analytical results of fish organs indicated a suitable performance of heavy metal determination within the range of certified values, with a 95–111% recovery for the metals studied (Murtala et al., 2012).
Whole fish (i.e., body including skins, gills, intestines, and muscles) were collected with water samples from aquaria at weekly intervals at 1, 4, 8, and 10 weeks to determine the total bacterial count and Aeromonas count. A total of 10 g of fish samples were homogenized in 90 ml of sterilized peptone buffer. This solution was decimally diluted up to 10−7.
Similarly, 10 ml of water representative sample was mixed with 90 ml of a sterilized peptone buffer and serially diluted up to 10−7. The bacterial count was expressed as log CFU ml−1 for water and fish samples. The total bacterial count was enumerated on a plate count agar (PCA) previously supplemented with 100 μl of each dilution (fish and water samples), then incubated at 37°C for 1 day (Saad et al., 2021), while the Aeromonas count was calculated on an Aeromonas agar medium after 1 day of incubation at 37°C (Sheir et al., 2020). The Aeromonas spp., colonies were distinguished by dark green with black-centered colonies.
An ANOVA test was used to distinguish the significant differences between sample means at a probability level (p ≤ 0.05). The LSD test determined significant differences between means. All statistical calculations were performed with SPSS 20 for Windows.
A total of 35 bacterial isolates were isolated from soil samples using PCA plates supplemented with sodium selenite (1 mM), 5 of which survived at a higher concentration (4 mM), with codes AS12, AS26, AS37, AS43, and AS66. One of them, AS12, could tolerate 5 mM sodium selenite and was considered a Se-resistant bacterium.
No colonies occurred at sodium selenite (6 mM). Based on the morphological, physiological, and biochemical tests in Bergey’s Manual, this isolate was aerobic, gram-positive, motile, long-rod, and spore-forming under a light microscope, which revealed that this bacterium is similar to the Bacillus species. Subsequently, the isolate was nominated as Bacillus subtilis AS12 based on MALDI TOF Mass Spectrometry.
Maximum similarity (99%) of the obtained isolate (Bacillus subtitles AS12) was established by the MALDI-TOF score to Bacillus subtilis DSM 5552 DSM. B. subtilis is an assemblage of closely-related species that cannot be easily differentiated based on phenotypic characteristics. In recent years, it has been disclosed that the identification of the 16S rRNA using gene-based phylogenetic analysis seems impossible because of the highly conserved nature of the gene.
As an alternative approach, the phylogenetic analysis of protein-coding loci can be used to identify and characterize the Bacillus species. MALDI-TOF MS identified the B. subtilis isolate as DSM 5552 DSM, with a score of 2.13 and NCBI identifier 1,432. According to GenBank Database BLAST results, the B. subtilis DSM 5552 DSM was registered based on the small acid-soluble spore protein gamma-type (sspE) gene as a complementary identification method of bacteria.
Our phylogenetic analysis was performed using the neighbor-joining method based on existing data of the sspE gene. Results revealed that the B. subtilis DSM 5552 DSM isolate is closely related to newly-isolated bacteria (Bacillus subtilis AS12), as shown in Supplementary Figure S1.
Figure 1 shows the optimal conditions for Bio-SeNPs fabrication by Bacillus subtilis AS12. The SeNPs with small size and high yield were obtained when the bacteria were inoculated on EM medium, and its supernatant was mixed with Na2SeO3 at pH 7. The mixture was incubated at 30°C for 2 days in a shaking incubator with an agitation speed of 180 rpm in the dark condition. These optimum conditions achieved SeNPs sizes ranging from 60 to 81 nm.
Figure 1. Optimization of the physiochemical parameters for fabricating by Bacillus subtilis AS12. Data are presented as means ± SE.
Figure 2 shows the qualitative and quantitative colorimetric tests that evidenced the reduction of Na2SeO3 (Se+4) to the elemental Se form (Se0) in the Bio-SeNPs. The Tube-Series (No. 1) shows the control tubes (A) and (B) with transparent solutions of sodium selenite and sodium selenate. On the other hand, tubes (C) and (D), which contained the substrate sodium selenite and were incubated with the supernatant of Bacillus subtilis AS12 for 2 and 3 days, respectively, produced brick-red dispersions, primarily referring to elemental Se.
Figure 2. Qualitative identification of Selenium status in bio-selenium nanoparticles [BioSeNPs], obtained after 2d and 3d incubation with bacterial supernatant (C and D), as compared to two different controls:) Sodium selenite (A) and Sodium selenate (B). I= The original selenium solutions. II= (I) + KI. III= (II) + Starch, IV= Microtiter plate to determine I2 content equal Se content.
Therefore, it can be concluded that an incubation time between 2 and 3 days were sufficient to reduce sodium selenite into elemental Se under the metabolic action of Bacillus subtilis AS12. It was further evidenced by adding potassium iodide (KI) in an acidic medium to the tubes in series I, producing series II. The tubes in series (No. 2) showed that the control tubes (A and B) released I2, which could turn iodide into iodine because the Se was in its most oxidizing form. In contrast, tubes C and D had the uncharged form of Se. They did not have any sodium selenite leftover or any form of Se available to oxidize.
Thus, the total original amount of this Se form selenite (Se+4) may have transformed into elemental Se (Se0), which cannot oxidize iodide into iodine. However, since all the tubes in series II were brown, which might originate from iodine or the elemental Se, it was necessary to distinguish them. This distinguishing was done by interacting them with the starch solution to series II, resulting in tube series III. Tubes A and B of the series (No. 3) produced a blue color, confirming the release of iodine. At the same time, tubes C and D did not change color, confirming that no iodine was released and that the brick red color is solely due to the presence of the formed elemental Se (Se0) produced under the action of Bacillus subtilis AS12.
The inability of the Bio-Se-nanoparticles (tubes C and D) to oxidize KI into I2 implies that Se has changed its oxidation state from the original high values (selenite+4) into metallic Se (oxidation state equals zero). Alternately, the quantitative assessment of Se content in the nanoparticles recorded 4.83 mg/L, indicating the complete transformation of sodium selenite to Se0 in Bio-SeNPs under the action of Bacillus subtilis AS12.
The color change from colorless to red when adding Bacillus subtilis AS12 pellets to the EM supplemented with Na2SeO3 solution indicates the biotransformation of Na2SeO3 into Bio-SeNPs. The UV–VIS spectroscopy, revealing the product’s absorption spectrum at 280 nm (Figure 3A), further confirms this transformation observation.
Figure 3. Characterization of biosynthesized selenium nanoparticles (BioSeNPs) synthesized by Bacillus subtilis AS12. (A) U.V. absorbance at 280 nm; (B) the size of 25–67 nm and its spherical shape in a transmission electron micropscopic image; (C) average size of 77.78 nm by Zeta sizer; (D) surface net negative charge by Zeta potential (−26.4); and (E) Fourier transform-infrared (FTIR) spectroscopy.
The TEM image (Figure 3B) of the produced Bio-SeNPs revealed a spherical shape with an average size in the range of 25–85 nm. SEM image of Bio-SeNPs showed in Supplementary Figure S2C. The FTIR of Bacillus subtilis AS12 pellets detected 9 bands (Supplementary Figure S3), while 15 bands between 3,420.38 and 440.51 cm−1 appeared in the FTIR spectrum of Bio-SeNPs (Figure 3E). These bands refer to the presence of various functional groups surrounding the SeNPs, e.g., alcohol, primary and secondary amines, amides, and phenols. The strong, broad peak at 3,420.38 cm−1 refers to the O–H and N–H groups, while the peak at 2,896.72 cm−1 refers to the CH group. Bands appearing at 2,423.78 and 2,355.48 cm−1 refer to the ester group, while bands at 1,639.09 and 1,384.23 cm−1 indicate alkenes and aromatics, respectively. The peak at 1,078.60 cm−1 indicated the presence of SO compounds, while the peaks at the range of 941.88–538.18 cm−1 referred to aromatic C–H, C﹦C, and halide compounds.
Dynamic Light Scattering (DLS) is a technique used to study the behavior of nanoparticles in suspension in terms of size and charge. Figure 3C,D shows the DLS analysis of the stable Bio-SeNPs, indicating an exact size of 77.47 nm (Figure 4C), with a net negative charge of −26.33 mV (Figure 3D). These results confirm that the biotransformation of Bio-SeNPs was mono-dispersed.
Figure 4. Radical scavenging activity of biosynthesized selenium nanoparticles (BioSeNPs) at different concentrations (0.5–5 μg ml−1) against ABTS and DPPH radicals after 30 min. of incubation, as compared to tret-butyl hydroquinone (TBHQ 5 μg ml−1). Data are presented as means ± SE. Bold uppercase letters indicate the significant differences between concentrations as compared to TBHQ, while lowercase letters indicate significant differences between DPPH and ABTS subjected to SeNPs and TBHQ.
XPS image confirming the production of elemental selenium founded in Supplementary Figure S2A XRD image in Supplementary Figure S2B revealed that Bio-SeNPs were in crystalline nature as evidenced by the peaks at 2θ value of 30° with stronger intensity of peaks for plane (101).
Figure 4 shows that Bio-SeNPs exhibited considerable ABTS+ and DPPḢ scavenging activity comparable to the synthetic antioxidant TBHQ (5 μg ml−1). The SC50 against DPPḢ and ABTS+ was 0.5 μg ml−1 and 1 μg ml−1, respectively. Antioxidant activity was significantly increased in a concentration-dependent manner. The Bio-SeNPs (5 μg ml−1) scavenged 91 and 94% of DPPḢ and ABTS+ compared to 95 and 97% in the case of TBHQ, respectively.
The phenolic and flavonoid content in Bio-SeNPs is presented in Figures 5A,B. The antioxidant activity of nanoparticles depended on the polyphenol content, which significantly increased concentration-dependent. The Bio-SeNPs (5 μg ml−1) have a total phenolic and flavonoids of 1.9 g/g and 0.7 g/g GAE and QE, respectively.
Figure 5. (A) Total phenolic content expressed as gallic acid equivalent (GAE) in biosynthesized selenium nanoparticles (BioSeNPs) suspension, fabricated by Bacillus subtilis AS12. (B) Flavonoids content expressed as Quercetin quercetin equivalent in BioSeNPs suspension fabricated by Bacillus subtilis AS12.
The inhibition zone diameters (IZDs) in Table 1 and Figure 6 show that Bio-SeNPs can kill bacteria. The IDZs increased significantly (p ≤ 0.05) with increasing Bio-SeNPs concentrations in the 0.5–5 μg ml−1 range. The IDZs ranged from 9 to 30 mm. Staphylococcus aureus and Klebsiella pneumoniae were the most sensitive G+ and G− bacteria to Bio-SeNPs (5 μg ml−1) with 30 mm and 24 mm, respectively.
Table 1. The inhibition zones diameters (IZDs) of the biosynthesized selenium nanoparticles (BioSeNPs) with minimum inhibitory concentration (MIC) and minimum bactericidal concentration (MBC) levels against certain Gram positive (G+) and Gram negative (G-) pathogenic bacteria.
Figure 6. Antibacterial activity of biosynthesized selenium nanoparticles (BioSeNPs) suspensions (0.5–5.0 μg ml−1) fabricated by Bacillus subtilis AS12 against pathogenic Gram-positive bacteria (G+ bacteria) and Gram-negative bacteria (G− bacteria).
However, Listeria monocytogenes and Aeromonas hydrophilia were the most resistant G+ and G− bacteria to Bio-SeNPs (5 μg ml−1), recoding lower inhibition zones of 25 mm and 21 mm, respectively. The plates’ images in Figure 6 supported the results in Table 1. Generally, all tested bacteria were inhibited by Bio-SeNPs MIC in the range of (0.25–0.45 μg ml−1), and completely killed by Bio-SeNPs MBC (0.45–0.85 μg ml−1).
Adding the Bio-SeNPs to normal living fish, i.e., without subjection to heavy metal (group 1), improved the growth performance of fish (Table 2), meaning they exhibited weight gain and specific growth rate increases with all levels of nanoparticles. The treated fish with Bio-SeNPs levels (3–5 μg ml−1) gained weight in the 36–52% range, with a specific growth rate (SGR) of 40% higher than the control. At the same time, FCR was reduced, inferring improved food conversion. Therefore, using these Bio-SeNPs for normal living fish can enhance their growth performance. In parallel, survival in the Bio-SeNPs-treated fish was not negatively affected, supporting these nanoparticles’ safe and beneficial use.
Table 2. Growth performance of Nile tilapia (Oreochromis niloticus) fish subjected to heavy metals, mercury (Hg) and cadmium (Cd), and treated with biosynthesized selenium nanoparticles (BioSeNPs) during the 10-week experimental period.
Alternatively, exposing heavy metals, i.e., Hg and Cd mixtures at two levels (0.5 and 1 μg ml−1) in Nile tilapia (Oreochromis niloticus) media significantly influenced (p ≤ 0.05) the growth performance parameters. Weight gain improved (p ≤ 0.05) ≈ 0.0299 by ~8% and ~15%, and SGR was (p ≤ 0.05) ≈ 0.0302 increased by only 18 and 4%. However, the survival rate was decreased (p ≤ 0.05) ≈ 0.0385 by 9 and 10% in fish subjected to the first and second levels of heavy metals, respectively. FCR values fluctuated slightly with heavy metal additions in the two doses.
On the other hand, the water application of Bio-SeNPs at three levels (3, 4, and 5 μg ml−1) revealed greater positive effects on the growth performance of Nile tilapia in a concentration-dependent manner, consequently mitigating the negative effects of heavy metals. Weight gain in the second group (2A, 2B, and 2C) increased in the 22–30% range as compared to the respective control (2Z), and saw an ~8–20% increase in weight gain in the third group (3A, 3B, and 3C) compared to 3Z by the addition of Bio-SeNPs. Similarly, SGR was enhanced in treated fish in group 2 by 2–19%, compared to a 15% relative increase in group 3 in response to the 3 doses of the Bio SeNPs. Here, the survival rate improved by ~3–4% in the 2 groups compared to their respective non-treated controls.
Table 3 presents the serum biochemical parameters of Nile tilapia (Oreochromis niloticus) fish both subjected and not subjected to the Hg and Cd mixture, at two levels in the water, 0.5 + 0.5 μg ml−1 and 1 + 1 μg ml−1, respectively. The Bio-SeNPs significantly reduced liver function marker levels (ALT and AST) in the heavy metal-free water and enhanced the innate immune responses, GH level, the male hormone, and XX hormones, but reduced both the F hormone P4 level and cortisol levels.
Table 3. Serum biochemical parameters, immune activity and hormonal levels of Nile tilapia (Oreochromis niloticus) fish subjected to heavy metals, mercury (Hg) and cadmium (Cd), and treated with the biosynthesized selenium nanoparticles (BioSeNPs) during the 10-week experimental period.
Considerable biological changes occurred from the subjection of fish to heavy metals. Glucose levels increased by 16 and 21% in fish subjected to the 2 heavy metals (2Z and 3Z) levels over the untreated control (1Z). Heavy metals adversely affected liver functions, raising ALT and AST enzymes by 38 and 45% and by 14 and 15% in 2Z and 3Z over the control (1Z), respectively. The innate immunity, responses, and growth hormones were moderately reduced by fish subjected to heavy metals in a heavy metal concentration manner. Cortisol levels in fish exposed to heavy metals (2Z and 3Z) went up by 108 and 165%, respectively, compared to the control group (1Z) fish. These changes were proportional to the concentration of the metals.
Alternately, treating fish subjected to heavy metals with Bio-SeNPs at three graded levels (3, 4, and 5 μg ml−1) efficiently reversed the heavy metals’ harmful effects in a concentration-dependent manner. The second concentration of Bio-SeNPs (4 μg ml−1) can mostly eliminate the adverse effects of heavy metals exposed at a medium dose (0.5 μg ml−1 and 0.5 μg ml−1 of Cd and Hg), while the highest concentration of Bio-SeNPs (5 μg ml−1) was required to eliminate adverse effects originating from high heavy metal doses (1 μg ml−1 and 1 μg ml−1 of Cd and Hg). Therefore, applying Bio-SeNPs in the rising environment can efficiently and effectively alleviate the adverse effects of heavy metals on the serum biochemical parameters of fish.
Table 4 shows the behavioral patterns of fish exposed to heavy metals throughout a 10-week experiment. Initially, the sole presence of Bio-SeNPs in the water enhanced the feeding time and frequency in a concentration-dependent manner, with the maximum relative increases amounting to 60 and 66% in feeding time and frequency for 5 μg ml−1 over the untreated fish.
Table 4. Behavioral patterns of Nile tilapia (Oreochromis niloticus) fish subjected to the heavy metals, mercury (Hg) and cadmium (Cd), and treated with biosynthesized selenium nanoparticles (BioSeNPs) during the 10 week experimental period.
Bio-SeNPs treatments led to a moderate increase in swimming time and frequency but only a slight increase in resting time and frequency. Moderate decreases accompanied these increases in both foraging time and frequency. While surfacing time was not affected, Bio-SeNPs moderately reduced the surfacing frequency. Arousal time was increased while aggregation time was greatly reduced, with a maximum reduction of 78% being the highest amount of Bio-SeNPs (5 μg ml−1).
A different trend was observed from the exposure to heavy metals (Cd + Hg). The feeding time decreased by ~13 and 35%, with the 2 levels of heavy metals, 0.5, and 1 mg L−1, respectively, while the feeding frequency was slightly increased (7 and 12%, respectively). Surfacing time increased by ~14–16% while surfacing frequency increased by ~5% to the high level of heavy metal exposure. Foraging time slightly fluctuated, but the foraging frequency was moderately increased by exposure to heavy metals.
Both swimming time and frequency were slightly reduced while resting time and frequency were only moderately reduced by exposure to heavy metals. Both levels of heavy metal exposure slightly increased arousal time and frequency. In contrast, aggression time was reduced by 29 and 53% at the first and second levels of heavy metal exposure, respectively.
Water application of Bio-SeNPs mitigated the adverse effects of Cd + Hg on the behavioral patterns of fish. For example, the decreasing effect of heavy metals on feeding time was encountered by an increasing effect in the 28–44% range in the group exposed to 0.5 + 0.5 μg ml−1 (Cd + Hg), and in the 42–53% range in the group exposed to 1 + 1 μg ml−1 (Cd + Hg), in response to (3–5 μg ml−1), respectively. The increase in foraging frequency induced by heavy metals was reversed by considerable reductions in the 6–37% and the 18–29% range in groups 2 and 3, respectively. Surfacing behavior decreased in the 10–23% range in group 2 and the 8–19% range in group 3 when treated with Bio-SeNPs (3–5 μg ml−1) compared to the untreated control.
The decreases in swimming time and resting time caused by exposure to heavy metals were considerably reversed when treated with Bio-SeNPs (3–5 μg ml−1). The observed increases in arousal time and frequencies in fish exposed to heavy metals were further enhanced by Bio-SeNPs (3–5 μg ml−1) in groups 2 and 3, with ranges of 3–13% and 1–11%, respectively. The reductions in the aggression time noticed when fish were exposed to heavy metals were also further reduced by treatment with Bio-SeNPs (3–5 μg ml−1).
Table 5 presents the accumulation of Hg and Cd (μg/g) in fish organs, liver, intestines, gills, and kidney after 10 weeks of exposure to heavy metals and treatment with Bio-SeNPs concentrations of 3, 4, and 5 μg ml−1. Control fish in the free heavy metal water exhibited low levels of heavy metals. The presence of Bio-SeNPs further eliminated heavy metals to undetectable levels in fish organs. Subjecting fish to heavy metals in water resulted in massive levels of heavy metal accumulation in fish organs. Cadmium accumulation in exposed fish was 25–69 times the amount observed in organs of untreated fish (0.5 μg ml−1 Cd). At the same time, Hg accumulation was in the 38–128 times range of fish subjected to the first level of heavy metals in water (0.5 μg ml−1 Hg). When doubling the heavy metal exposure level (1 μg ml−1 for both Cd and Hg), the range of heavy metal accumulation in fish organs excelled to 32–170 times for Cd and 88–280 times for Hg.
Table 5. Content of heavy metals, mercury (Hg) and cadmium (Cd) in Nile tilapia (Oreochromis niloticus) fish organs (liver, intestine, gills and kidney) at the end of experiment (10 weeks), and the reducing effect of biosynthesized selenium nanoparticles (BioSeNPs).
Treating the polluted water with Bio-SeNPs (3, 4, and 5 μg ml−1) mitigated the accumulation of heavy metals in fish organs in a concentration-dependent manner. Treating the contaminated media with Bio-SeNPs at 5 μg/ml significantly reduced the heavy metal accumulation in group 2 (low level of heavy metal exposure) by 50–87% compared to untreated fish. Simultaneously, reducing the heavy metal accumulation by 57–73% in case of high levels of heavy metal exposure (group 3).
The levels of contaminating bacteria (total bacterial count and Aeromonas count) in the fish water media during the 10 weeks are shown in Table 6. Uncontaminated media contained a total bacterial count increasing from 5.4 Log CFU ml−1 in the first week to 6 Log CFU ml−1 in the tenth week. The total Aeromonas count increased from 2.9–3.9 Log CFU ml−1 for the same period. Including Bio-SeNPs (5 μg ml−1) in the uncontaminated water media at different concentrations (group 1) reduced the bacterial load in a concentration-dependent manner. Maximum reductions amounted to 27–35% and 36–42% in response to the highest level of Bio-SeNPs.
Table 6. Total bacterial and Aeromonas counts in fish farming water during the 10-weeks experimental period.
In heavy metal-contaminated water media, total bacterial counts were slightly reduced by ~4–7% and 8–12%, at the low and high levels of applied heavy metals, respectively, against relative increases in Aeromonas counts of 5–12% and 13–18%, respectively. Adding Bio-SeNPs (5 μg ml−1) to heavy metal-contaminated media further reduced the levels of bacterial counts (Table 6).
Bacteria levels in the media were reflected in the bacterial counts within fish organs, particularly those within direct contact with water, i.e., the skin, the gills, and the intestine (Table 7). These organs recorded high levels of total bacterial counts in the 7.5–8.2 Log CFU ml−1 range and 3.1–4.7 Log CFU ml−1 range of Aeromonas count. The addition of Bio-SeNPs (3–5 μg ml−1) reduced these levels by ~28–42% in both total bacterial and total Aeromonas counts.
Table 7. Total bacterial and Aeromonas counts in Nile talipa (Oreochromis niloticus) fish organs (skin, muscle, intestine and gills) after 10 weeks of treatment.
Exposure to high levels of heavy metals (Cd + Hg) significantly decreased the total bacterial count in fish organs within a 9–14% range and total Aeromonas within a 6–16% range over the respective controls. Combining Bio-SeNPs application with heavy metals further reduced the bacterial counts.
In this study, a selenium (Se)-resistant bacteria, Bacillus subtilis AS12, was isolated from the soil and identified by morphological, physiological, and biochemical tests following Bergey’s Manual (Wang et al., 2007). It was considered safe (GRAS) for this study. Further identification by MALDI-TOF qualified the isolate as B. subtilis DSM 5552 DSM. The high identity of 99.77–99.25% between B. subtilis DSM 5552 DSM and the other B. subtilis species showed that phylogenetic analysis based on MALDI-TOF MS results and NCBI identifiers could be a reliable, complementary, and alternative way to identify and classify B. subtilis. This observation can avoid the complicated differentiation of closely related B. subtilis based on 16S rRNA (Chun and Bae, 2000; Palmisano et al., 2001; Gatson et al., 2006).
To date, this is the first study chemically evidencing the complete transformation of Na₂SeO₃ (Se+4) into Bio-SeNPs (Se0) with Bacillus subtilis AS12, resulting in the conversion of all Se+4 to elemental Se, as quantitatively assessed at 4.83 mg/l in the final nanoparticles. The bacterial suspension has alkaloids, tannin, cinnamic acid, phenolic acid, monoterpenes, and secondary metabolites that can bind to the metallic core and help make Se nanoparticles with stable shapes and sizes (Javed et al., 2020).
Bacillus species have been reported as nanoparticle producers, namely, B. mycoides (Cremonini et al., 2016) and B. pumilus sp. BAB-3706 (Prasad et al., 2015), B. licheniformis (Sonkusre, 2020), and B. amyloliquefaciens SRB04 (Ashengroph and Hosseini, 2021). The Bio-SeNPs, produced by Bacillus subtilis AS12 in this study, were spherical, at 67 nm in diameter, with a − 26.33-mV negative charge, and rich in various bioactive natural compounds. The obtained results similar to other work with different species of Bacillus. For example, Pouri et al. (2017) synthesized spherical SeNPs with a size of 36 nm by Bacillus cereus; and Abdallah et al. (2019) fabricated spherical SeNPs, at 60–125 nm diameter and −30.6 negative charge by Bacillus tropicus Ism 2 (MK332444), isolated from soil polluted with industrial wastewater.
The prepared Bio-SeNPs showed considerable antioxidant activity to scavenge the ROS, which damages the DNA and protein. The scavenging power of Bio-SeNPs may be due to the wide surface area and the released Se. The potential action of the associated phenolic and flavonoid content derived from the components of the bacterial media, LB, and supernatant acted as reducing agents, which is required for nanoparticle production. Yeast extract is one of the medium components, which may be the source of several phenolic compounds (Vieira et al., 2016; El-Saadony et al., 2021d). In a similar study, L. acidophilus ML14 fabricates different Se nanoparticles (El-Saadony et al., 2021b), and (Abdel-Moneim et al., 2022) found that Bacillus subtilis AL43 exhibits antioxidant activity. These components may also contribute to the observed antibacterial activity of the NPs following Abdel-Shafi et al. (2019a).
Generally, all tested pathogenic bacteria were inhibited by Bio-SeNPs concentrations in the range of 250–450 ng ml−1 and entirely killed by 450–850 ng ml−1. These effective concentrations are vastly lower than previously reported native- or chemically-modified natural proteins, including soybean glycinin, chickpea protein (Sitohy et al., 2012; Osman et al., 2018), or esterified proteins (Sitohy and Osman, 2010). However, several nanoparticles, such as silver NP showing antibacterial activities are probably associated with harmful side effects (Huang et al., 2019; Li et al., 2020). Unlike other metals, Se is an essential element that can be administered as a green nanoparticle. Also, the antibacterial activity of the Bio-SeNPs decreased with their size. For example, SeNPs with 81 nm were more effective against S. aureus than SeNPs with a size of 124 nm (Huang et al., 2019).
Based on these results, it can be assumed that the small size of the Bio-SeNPs may explain why they are so effective as antibacterial. The low MIC values observed with Bio-SeNPs (250–450 ng ml−1) match and excel the values reported for Penicillium chrysogenum-mediated SeNPs 3.950–0.245 μg ml−1 (El-Sayyad et al., 2020), probably due to the different composition of each preparation.
The possible antimicrobial potential of Bio-SeNPs may occur through damaging the plasma membrane, which affects its functionality and integrity, or through altering the deoxyribonucleic acid replication, protein synthesis process, and food metabolism cycle (Mosallam et al., 2018; El-Gazzar and Ismail, 2020). In contrast to the positively charged antimicrobial basic proteins (Abdel-Shafi et al., 2016), nanoparticles carry negative charges on their outer surfaces. The positive charges were the main driving force for these basic proteins, which can distort the bacterial membranes through chemical electrostatic interactions with the negative charges on the cell membranes (Sitohy et al., 2011, 2013).
Accordingly, it is unlikely that the negatively charged NPs (−26.33 mV) will act in the same chemical way with negatively charged cell membranes. As a result, the possible interaction of Bio-SeNPs may go through physical and not chemical interactions. The potential interaction may start with repulsion between the similar negative charges of both NPs and the cell membrane, generating a random mechanical movement of the NPs in all directions and causing the collision of nanoparticles with other bacterial cells. This potential collision between the same NPs based on similar negative charges may double the collisional motions with bacterial cells, leading to accelerated cellular damage. Further, explain the strong antibacterial activity of NPs.
As rigid and strict structures generally characterize heavy metal nanoparticles (Khan et al., 2019), their collision with bacterial cells may be quite destructive to the bacterial cells. The collision force may depend on the rigidity of NPs and the speed of their random movement, which may depend on the NPs’ size. Thus, the medium size of Bio-SeNPs (~67 nm) may guarantee the high speed, thereby supporting its effectiveness. Thus, the nanoparticles’ speed and rigidity may contribute to the effectiveness of the physical antimicrobial action of NPs (Hashem et al., 2021; Elakraa et al., 2022; Hashem and Salem, 2022; Salem, 2022b).
The spherical shape of Bio-SeNPs may allow a multitude of simultaneous interactions with different bacterial cells, thus contributing to the effectiveness of the NPs and explaining the very low MIC values against different pathogenic bacteria. Once the outer cell membrane is distorted, the NPs can interact indiscriminately with any positively-charged cellular component, assuring the death of the entire bacteria. This physical mechanism of the NPs’ action may explain the relatively lower MIC values of Bio-SeNPs (250–450 ng ml−1) than the antimicrobial proteins 50–200 μg ml−1 (Abdel-Shafi et al., 2019b; Saad et al., 2021). This MIC level is also much lower than other synthetic NPs (Sitohy et al., 2021), showing that this green nanoparticle preparation is a better antimicrobial agent.
Several techniques remedy the presence of heavy metals in wastewater, e.g., electrolysis, adsorption, coagulation, flocculation, and membrane filtration (Chen et al., 2013; Zeng et al., 2015). However, the effectiveness and efficiency of these techniques are still low. Biogenic SeNPs have attracted attention in the last few decades and have been recommended as effective adsorbents for removing heavy metals from contaminated media (Nancharaiah and Lens, 2015). Additionally, Qi et al. (2021) reported that SeNPs scavenged the ROS induced by cadmium (Cd) contamination by inhibiting the activities of NADPH and glycolate oxidases. In addition, it has been reported that SeNPs can eliminate elemental mercury (Hg) from groundwater (Wang et al., 2018).
Selenium is essential to produce selenoproteins in the glutathione cycle. Consequently, the deficiency of Se in aquafeed causes malnutrition and loss in weight gain in various fish species (Kumar et al., 2020; Wang et al., 2021). Furthermore, biogenic SeNPs exhibited considerable antimicrobial potential against several pathogenic microorganisms in various fish (Khiralla and El-Deeb, 2015; Korde et al., 2020).
Bio-SeNPs in this study significantly enhanced growth performance in Nile tilapia fish by enhancing feed efficiency and increasing nutrient availability for optimal metabolic functions (Swain et al., 2019; Abd El-Kader et al., 2020; Dawood, 2021). Furthermore, the addition of Se enhances the gastrointestinal absorption capacity by enhancing protein utilization and improving the activity of digestive enzymes for properly utilizing food (Iqbal et al., 2020; Adineh et al., 2021), indicating the role of SeNPs as a growth promoter and safe additive for aquaculture without adverse side effects. Furthermore, the positive effect of SeNPs on the upregulation of the Insulin-like growth factor 1 (IGF-1) gene expression, enhancing the ghrelin hormone, and improving feed utilization can improve growth performance (Abd El-Kader et al., 2020; Jahanbakhshi et al., 2021).
In this regard, Çiçek et al. (2021) found that adding SeNPs to the diets of common carp, Nile tilapia, European seabass, red seabream, and yellowfin seabream led to a significant improvement in growth performance. Furthermore, Kumar et al. (2018) reported improving growth performance traits, e.g., WG, SGR, and PER values in striped catfish grown under high Pb concentration and high-temperature stress of 34°C, particularly when fish were delivered the SeNPs diet (1 mg/kg). Moreover, Rathore et al. (2021) found that the inclusion of SeNPs (1 mg/kg) in Nile tilapia fish challenged with A. hydrophila considerably enhanced the values of growth traits, WG, SGR, ADG, and PER and reduced FCR.
Several parameters in fish media may induce oxidative stress, specifically low water quality, overcrowding, heavy metals, pathogenic microorganisms, and malnutrition (Dawood et al., 2020). ROS production causes lipid peroxidation by attacking the lipid in the immune cells, ending with the impairment of the cell DNA and, thereby, the tissue’s death (Zhang et al., 2020; Gupta and Verma, 2022).
SeNPs were reported to keep blood parameters at normal levels in Nile tilapia challenged by S. iniae infection, making the fish healthier and more robust (Neamat-Allah et al., 2019). In addition, the inclusion of 1 mg/kg SeNPs for a 28-day diet reversed the decreases in RBC, WBC, Hb, Ht, MCV, MCH, and MCHC in Caspian roach exposed to 0.5 mg/L malathion (Zahmatkesh et al., 2020).
Several studies reported significant decreases in serum AST, ALT, and ALP levels in Nile tilapia, common carp, Caspian roach, and grass carp fed SeNPs (Yu et al., 2020; Zahmatkesh et al., 2020). The obtained results confirmed the protective action of Bio-SeNPs on liver performance and other tissue organs in fish.
The antioxidant potential of Se released from SeNPs may be linked to the synthesis of selenoproteins, which are essential in building and for the activity of GPx (Rotruck et al., 1973; Kumar et al., 2020). Further, selenoproteins are connected to a good immunity status of catfish, enabling resistance against various stressors.
In the current study, Bio-SeNPs have been revealed to have a special role in improving intestinal health and achieving maximum feed utilization, reflecting growth performance. Furthermore, the antibacterial potential of Bio-SeNPs may reduce pathogens present in fish intestines, increasing the efficiency of beneficial bacteria to digest the nutrients favoring good feeding behavior and avoiding bad ones. The promoting action of Bio-SeNPs on fish growth performance and feeding behavior can be partially attributed to the activation effect of Se on the digestive enzymes and improving the efficiency of intestinal villi (Dawood et al., 2021). Intestinal morphometry, villi length, and goblet cell number in Nile tilapia fish were reported to be enhanced by SeNPs (Ghazi et al., 2022).
Selenoproteins and GPx transport Se to the testis, forming an essential role in spermatogenesis (Imai et al., 2009). Additionally, Se has a role in male reproduction, incorporated in the mitochondrial capsule protein, developing spermatozoa motility and function (Boitani and Puglisi, 2008; Moslemi and Tavanbakhsh, 2011). Therefore, it is a potential solution for male sterility (Shi et al., 2010). Several studies have reported that the inclusion of SeNPs (0.3 mg/kg body weight) significantly improved Se content in the testis, the activity of semen GPx, and ATPase in male boar goats. Additionally, Shi et al. (2010) ensured the effect of SeNPs in protecting the membrane efficiency and the tight arrangement of the midpiece of the mitochondria. Hence, SeNPs appear more effective in enhancing male reproduction than the single Se element.
Hozyen et al. (2020) observed the adverse effects of deltamethrin (DLM) in male rats on sperm characteristics, testosterone, and antioxidant biomarkers, as well as behavioral and histopathological alterations. The Bio-SeNPs-treated groups improved semen parameters, antioxidant status, and sexual performance. Based on these observations, it can be concluded that Bio-SeNPs in aquafeed can enhance feeding and sexual behaviors, leading to increased fish yield. Therefore, SeNPs may represent an effective treatment for reducing the detrimental effects on male fertility, leading to enhanced reproductive performance.
The accumulation of heavy metals and pathogenic microbes in fish organs is a severe problem, adversely affecting growth performance and productivity. The accumulation of heavy metals in fish organs causes oxidative stress by producing ROS, which damages cells and causes a low survival rate. Additionally, pathogenic bacteria accumulate in the gut and interfere with beneficial microorganisms, causing malnutrition, which affects growth performance. In this study, Bio-SeNPs synthesized by Bacillus subtilis AS12 isolate were proven with maximum efficiency as a remedy for heavy metal treatment and pathogen accumulation in fish organs and media. The unique characteristics of the obtained Bio-SeNPs, especially the negative charge and bioactive compounds’ coat, provide their antioxidant and antibacterial activity. Fish pathogens and heavy metal accumulation in fish media can be mitigated by including Bio-SeNPs (3–5 μg ml−1) in fish media, where they can improve the growth performance and survival rate of fish grown in regular or heavy metal-stressed media. Also, it maintained liver function at normal levels, enhancing the innate immune responses, GH, male hormones, and XX hormone levels. It is recommended to use Bio-SeNPs (3–5 μg ml−1) in polluted water as an efficient remedy to mitigate the accumulation of heavy metals and pathogens in fish organs.
The raw data supporting the conclusions of this article will be made available by the authors, without undue reservation.
The animal study has been reviewed and approved by ZU-IACUC committee. was performed in accordance with the guidelines of the Egyptian Research Ethics Committee and the guidelines specified in the Guide for the Care and Use of Laboratory Animals (2022). Ethical code number ZU-IACUC/2/F/394/2022. Written informed consent was obtained from the owners for the participation of their animals in this study.
AS, MS, MS-A, KE-T, and ME-S, conceived and designed the experiments, analyzed the data, drafted the manuscript, and wrote and edited the final manuscript. All authors contributed to the article and approved the submitted version.
The authors declare that the research was conducted in the absence of any commercial or financial relationships that could be construed as a potential conflict of interest.
All claims expressed in this article are solely those of the authors and do not necessarily represent those of their affiliated organizations, or those of the publisher, the editors and the reviewers. Any product that may be evaluated in this article, or claim that may be made by its manufacturer, is not guaranteed or endorsed by the publisher.
The Supplementary material for this article can be found online at: https://www.frontiersin.org/articles/10.3389/fmicb.2022.1015613/full#supplementary-material
Abd El-Kader, M. F., Fath El-Bab, A. F., Abd-Elghany, M. F., Abdel-Warith, A. W. A., Younis, E. M., and Dawood, M. A. (2020). Selenium nanoparticles act potentially on the growth performance, hemato-biochemical indices, antioxidative, and immune-related genes of European Seabass (Dicentrarchus labrax). Biol. Trace Elem. Res. 199, 3126–3134. doi: 10.1007/s12011-020-02431-1
Abdallah, O. A., EL-Baghdady, K. Z., Khalil, M. M., El Borhamy, M. I., and Meligi, G. (2019). Biosynthesis, characterization and cytotoxicity of selenium nanoparticles using Bacillus tropicus Ism 2. Egypt. Acad. J. Biolog. Sci. 11, 47–57. doi: 10.21608/EAJBSG.2019.55269
Abdel-Moneim, A. M. E., El-Saadony, M. T., Shehata, A. M., Saad, A. M., Aldhumri, S. A., Ouda, S. M., et al. (2022). Antioxidant and antimicrobial activities of Spirulina platensis extracts and biogenic selenium nanoparticles against selected pathogenic bacteria and fungi. Saudi J. Biol. Sci. 29, 1197–1209. doi: 10.1016/j.sjbs.2021.09.046
Abdel-Shafi, S., Al-Mohammadi, A. R., Osman, A., Enan, G., Abdel-Hameid, S., and Sitohy, M. (2019b). Characterization and antibacterial activity of 7S and 11S globulins isolated from cowpea seed protein. Molecules 24:1082. doi: 10.3390/molecules24061082
Abdel-Shafi, S., Al-Mohammadi, A. R., Sitohy, M., Mosa, B., Ismaiel, A., Enan, G., et al. (2019a). Antimicrobial activity and chemical constitution of the crude, phenolic-rich extracts of Hibiscus sabdariffa, Brassica oleracea, and Beta vulgaris. Molecules 24:4280. doi: 10.3390/molecules24234280
Abdel-Shafi, S., Osman, A., Enan, G., El-Nemer, M., and Sitohy, M. (2016). Antibacterial activity of methylated egg white proteins against pathogenic G+ and G− bacteria matching antibiotics. Springerplus 5:983. doi: 10.1186/s40064-016-2625-3
Abu-Elala, N. M., Younis, N. A., AbuBakr, H. O., Ragaa, N. M., Borges, L. L., and Bonato, M. A. (2020). Influence of dietary fermented Saccharomyces cerevisiae on growth performance, oxidative stress parameters, and immune response of cultured Oreochromis niloticus. Fish Physiol. Biochem. 46, 533–545. doi: 10.1007/s10695-019-00711-9
Abu-Elghait, M., Hasanin, M., Hashem, A. H., and Salem, S. S. (2021). Ecofriendly novel synthesis of tertiary composite based on cellulose and myco-synthesized selenium nanoparticles: characterization, antibiofilm and biocompatibility. Int. J. Biol. Macromol. 175, 294–303. doi: 10.1016/j.ijbiomac.2021.02.040
Adineh, H., Naderi, M., Nazer, A., Yousefi, M., and Ahmadifar, E. (2021). Interactive effects of stocking density and dietary supplementation with Nano selenium and garlic extract on growth, feed utilization, digestive enzymes, stress responses, and antioxidant capacity of grass carp, Ctenopharyngodon idella. J. World Aquacult. Soc. 52, 789–804. doi: 10.1111/jwas.12747
Alagesan, V., and Venugopal, S. (2019). Green synthesis of selenium nanoparticle using leaves extract of Withania somnifera and its biological applications and photocatalytic activities. BioNanosci. 9, 105–116. doi: 10.1007/s12668-018-0566-8
Al-Deriny, S. H., Dawood, M. A., Elbialy, Z. I., El-Tras, W. F., and Mohamed, R. A. (2020). Selenium nanoparticles and spirulina alleviate growth performance, hemato-biochemical, immune-related genes, and heat shock protein in Nile tilapia (Oreochromis niloticus). Biol. Trace Elem. Res. 198, 661–668. doi: 10.1007/s12011-020-02096-w
Alonso, Á., and Valle-Torres, G. (2018). Feeding behavior of an aquatic snail as a simple endpoint to assess the exposure to cadmium. Bull. Environ. Contam. Toxicol. 100, 82–88. doi: 10.1007/s00128-017-2230-3
Ashengroph, M., and Hosseini, S. R. (2021). A newly isolated Bacillus amyloliquefaciens SRB04 for the synthesis of selenium nanoparticles with potential antibacterial properties. Int. Microbiol. 24, 103–114. doi: 10.1007/s10123-020-00147-9
Avery, J. C., and Hoffmann, P. R. (2018). Selenium, selenoproteins, and immunity. Nutrients 10:1203. doi: 10.3390/nu10091203
Bégout Anras, M. L., Lagardere, J. P., and Covès, D. (2004). Swimming activity of seabass: comparing patterns obtained in natural environment and in re-circulating tanks under high density. J. Fish Biol. 65, 314–315. doi: 10.1111/j.0022-1112.2004.0559b.x
Bhakya, S., Muthukrishnan, S., Sukumaran, M., and Muthukumar, M. (2016). Biogenic synthesis of silver nanoparticles and their antioxidant and antibacterial activity. Appl. Nanosci. 6, 755–766. doi: 10.1007/s13204-015-0473-z
Boitani, C., and Puglisi, R. (2008). Selenium, a key element in spermatogenesis and male fertility. Adv. Exp. Med. Biol. 636, 65–73. doi: 10.1007/978-0-387-09597-4_4
Bosch, A. C., O'Neill, B., Sigge, G. O., Kerwath, S. E., and Hoffman, L. C. (2016). Heavy metals in marine fish meat and consumer health: a review. J. Sci. Food Agric. 96, 32–48. doi: 10.1002/jsfa.7360
Chen, G., Liu, Y., Wang, R., Zhang, J., and Owens, G. (2013). Cadmium adsorption by willow root: the role of cell walls and their subfractions. Environ. Sci. Pollut. Res. Int. 20, 5665–5672. doi: 10.1007/s11356-013-1506-3
Chun, J., and Bae, K. S. (2000). Phylogenetic analysis of Bacillus subtilis and related taxa based on partial gyrA gene sequences. Antonie Van Leeuwenhoek 78, 123–127. doi: 10.1023/A:1026555830014
Çiçek, S., and Özoğul, F. A. T. İ. H. (2021). Effects of selenium nanoparticles on growth performance, hematological, serum biochemical parameters, and antioxidant status in fish. Anim. Feed Sci.Technol. 281:115099. doi: 10.1016/j.anifeedsci.2021.115099
Coppedge, B. R., Carter, T. S., Shaw, J. H., and Hamilton, R. G. (1997). Agonistic behaviour associated with orphan bison (Bison bison L.) calves released into a mixed resident population. Appl. Anim. Behav. Sci. 55, 1–10. doi: 10.1016/S0168-1591(97)00035-X
Cremonini, E., Zonaro, E., Donini, M., Lampis, S., Boaretti, M., Dusi, S., et al. (2016). Biogenic selenium nanoparticles: characterization, antimicrobial activity and effects on human dendritic cells and fibroblasts. Microb. Biotechnol. 9, 758–771. doi: 10.1111/1751-7915.12374
Dawood, M. A. (2021). Nutritional immunity of fish intestines: important insights for sustainable aquaculture. Rev. Aquac. 13, 642–663. doi: 10.1111/raq.12492
Dawood, M. A. O., El Basuini, M. F., Zaineldin, A. I., Yilmaz, S., Hasan, M., Ahmadifar, E., et al. (2021). Antiparasitic and antibacterial functionality of essential oils: an alternative approach for sustainable aquaculture. Pathogens 10:185. doi: 10.3390/pathogens10020185
Dawood, M. A. O., Koshio, S., Zaineldin, A. I., Van Doan, H., Moustafa, E. M., Abdel-Daim, M. M., et al. (2019). Dietary supplementation of selenium nanoparticles modulated systemic and mucosal immune status and stress resistance of red sea bream (Pagrus major). Fish Physiol. Biochem. 45, 219–230. doi: 10.1007/s10695-018-0556-3
Dawood, M. A. O., Zommara, M., Eweedah, N. M., Helal, A. I., and Aboel-Darag, M. A. (2020). The potential role of nano-selenium and vitamin C on the performances of Nile tilapia (Oreochromis niloticus). Environ. Sci. Pollut. Res. 27, 9843–9852. doi: 10.1007/s11356-020-07651-5
El Nemr, A., El-Said, G. F., Ragab, S., Khaled, A., and El-Sikaily, A. (2016). The distribution, contamination and risk assessment of heavy metals in sediment and shellfish from the Red Sea coast, Egypt. Chemosphere 165, 369–380. doi: 10.1016/j.chemosphere.2016.09.048
El-Adawy, M. M., Eissa, A. E., Shaalan, M., Ahmed, A. A., Younis, N. A., Ismail, M. M., et al. (2021). Green synthesis and physical properties of gum Arabic-silver nanoparticles and its antibacterial efficacy against fish bacterial pathogens. Aquac. Res. 52, 1247–1254. doi: 10.1111/are.14983
Elakraa, A. A., Salem, S. S., El-Sayyad, G. S., and Attia, M. S. (2022). Cefotaxime incorporated bimetallic silver-selenium nanoparticles: promising antimicrobial synergism, antibiofilm activity, and bacterial membrane leakage reaction mechanism. RSC Adv. 12, 26603–26619. doi: 10.1039/D2RA04717A
El-Gazzar, N., and Ismail, A. M. (2020). The potential use of titanium, silver and selenium nanoparticles in controlling leaf blight of tomato caused by Alternaria alternata. Biocatal. Agric. Biotechnol. 27:101708. doi: 10.1016/j.bcab.2020.101708
El-Saadony, M. T., Alkhatib, F. M., Alzahrani, S. O., Shafi, M. E., Abdel-Hamid, S. E., Taha, T. F., et al. (2021c). Impact of mycogenic zinc nanoparticles on performance, behavior, immune response, and microbial load in Oreochromis niloticus. Saudi J. Biol. Sci. 28, 4592–4604. doi: 10.1016/j.sjbs.2021.04.066
El-Saadony, M. T., ALmoshadak, A. S., Shafi, M. E., Albaqami, N. M., Saad, A. M., El-Tahan, A. M., et al. (2021e). Vital roles of sustainable nano-fertilizers in improving plant quality and quantity-an updated review. Saudi J. Biol. Sci. 28, 7349–7359. doi: 10.1016/j.sjbs.2021.08.032
El-Saadony, M. T., Saad, A. M., Najjar, A. A., Alzahrani, S. O., Alkhatib, F. M., Shafi, M. E., et al. (2021b). The use of biological selenium nanoparticles to suppress Triticum aestivum L. crown and root rot diseases induced by Fusarium species and improve yield under drought and heat stress. Saudi J. Biol. Sci. 28, 4461–4471. doi: 10.1016/j.sjbs.2021.04.043
El-Saadony, M. T., Saad, A. M., Taha, T. F., Najjar, A. A., Zabermawi, N. M., Nader, M. M., et al. (2021a). Selenium nanoparticles from lactobacillus paracasei capable of antagonizing animal pathogenic fungi as a new source from human breast milk. Saudi J. Biol. Sci. 28, 6782–6794. doi: 10.1016/j.sjbs.2021.07.059
El-Saadony, M. T., Sitohy, M. Z., Ramadan, M. F., and Saad, A. M. (2021d). Green nanotechnology for preserving and enriching yogurt with biologically available iron (II). Innov. Food Sci. Emerg. Technol. 69:102645. doi: 10.1016/j.ifset.2021.102645
El-Sayyad, G. S., El-Bastawisy, H. S., Gobara, M., and El-Batal, A. I. (2020). Gentamicin-assisted mycogenic selenium nanoparticles synthesized under gamma irradiation for robust reluctance of resistant urinary tract infection-causing pathogens. Biol. Trace Elem. Res. 195, 323–342. doi: 10.1007/s12011-019-01842-z
Fesharaki, P. J., Nazari, P., Shakibaie, M., Rezaie, S., Banoee, M., Abdollahi, M., et al. (2010). Biosynthesis of selenium nanoparticles using Klebsiella pneumoniae and their recovery by a simple sterilization process. Braz. J. Microbiol. 41, 461–466. doi: 10.1590/S1517-83822010000200002
Gatson, J. W., Benz, B. F., Chandrasekaran, C., Satomi, M., Venkateswaran, K., and Hart, M. E. (2006). Bacillus tequilensis sp. nov., isolated from a 2000-year-old Mexican shaft-tomb, is closely related to Bacillus subtilis. Int. J. Syst. Evol. Microbiol. 56, 1475–1484. doi: 10.1099/ijs.0.63946-0
Ghazi, S., Diab, A. M., Khalafalla, M. M., and Mohamed, R. A. (2022). Synergistic effects of selenium and zinc oxide nanoparticles on growth performance, hemato-biochemical profile, immune and oxidative stress responses, and intestinal morphometry of Nile tilapia (Oreochromis niloticus). Biol. Trace Elem. Res. 200, 364–374. doi: 10.1007/s12011-021-02631-3
Gil, M. I., Tomás-Barberán, F. A., Pierce, B. H., and Kader, A. A. (2002). Antioxidant capacities, phenolic compounds, carotenoids, and vitamin C contents of nectarine, peach, and plum cultivars from California. J. Agric. Food Chem. 50, 4976–4982. doi: 10.1021/jf020136b
Gupta, P., and Verma, S. K. (2022). Evaluation of genotoxicity induced by herbicide pendimethalin in fresh water fish Clarias batrachus (Linn.) and possible role of oxidative stress in induced DNA damage. Drug Chem. Toxicol. 45, 750–759. doi: 10.1080/01480545.2020.1774603
Hariharan, S., and Dharmaraj, S. (2020). Selenium and selenoproteins: It’s role in regulation of inflammation. Inflammopharmacology 28, 667–695. doi: 10.1007/s10787-020-00690-x
Hashem, A. H., Khalil, A. M. A., Reyad, A. M., and Salem, S. S. (2021). Biomedical applications of mycosynthesized selenium nanoparticles using Penicillium expansum ATTC 36200. Biol. Trace Elem. Res. 199, 3998–4008. doi: 10.1007/s12011-020-02506-z
Hashem, A. H., and Salem, S. S. (2022). Green and ecofriendly biosynthesis of selenium nanoparticles using Urtica dioica (stinging nettle) leaf extract: antimicrobial and anticancer activity. Biotechnol. J. 17:2100432. doi: 10.1002/biot.202100432
Hashem, A. H., Selim, T. A., Alruhaili, M. H., Selim, S., Alkhalifah, D. H. M., Al Jaouni, S. K., et al. (2022). Unveiling antimicrobial and insecticidal activities of biosynthesized selenium nanoparticles using prickly pear peel waste. J. Funct. Biomater. 13:112. doi: 10.3390/jfb13030112
Hozyen, H. F., Khalil, H. M., Ghandour, R. A., Al-Mokaddem, A. K., Amer, M. S., and Azouz, R. A. (2020). Nano selenium protects against deltamethrin-induced reproductive toxicity in male rats. Toxicol. Appl. Pharmacol. 408:115274. doi: 10.1016/j.taap.2020.115274
Huang, T., Holden, J. A., Heath, D. E., O'Brien-Simpson, N. M., and O'Connor, A. J. (2019). Engineering highly effective antimicrobial selenium nanoparticles through control of particle size. Nanoscale 11, 14937–14951. doi: 10.1039/c9nr04424h
Imai, H., Hakkaku, N., Iwamoto, R., Suzuki, J., Suzuki, T., Tajima, Y., et al. (2009). Depletion of selenoprotein GPx4 in spermatocytes causes male infertility in mice. J. Biol. Chem. 284, 32522–32532. doi: 10.1074/jbc.M109.016139
Iqbal, S., Atique, U., Mahboob, S., Haider, M. S., Iqbal, H. S., Al-Ghanim, K. A., et al. (2020). Effect of supplemental selenium in fish feed boosts growth and gut enzyme activity in juvenile tilapia (Oreochromis niloticus). J. King Saud Univ. Sci. 32, 2610–2616. doi: 10.1016/j.jksus.2020.05.001
Jahanbakhshi, A., Pourmozaffar, S., Adeshina, I., Mahmoudi, R., Erfanifar, E., and Ajdari, A. (2021). Selenium nanoparticle and selenomethionine as feed additives: effects on growth performance, hepatic enzymes’ activity, mucosal immune parameters, liver histology, and appetite-related gene transcript in goldfish (Carassius auratus). Fish Physiol. Biochem. 47, 639–652. doi: 10.1007/s10695-021-00937-6
Javed, B., Raja, N. I., Nadhman, A., and Mashwani, Z. U. R. (2020). Understanding the potential of bio-fabricated non-oxidative silver nanoparticles to eradicate Leishmania and plant bacterial pathogens. Appl. Nanosci. 10, 2057–2067. doi: 10.1007/s13204-020-01355-5
Khan, I., Saeed, K., and Khan, I. (2019). Nanoparticles: properties, applications and toxicities. Arab. J. Chem. 12, 908–931. doi: 10.1016/j.arabjc.2017.05.011
Khan, K. U., Zuberi, A., Nazir, S., Fernandes, J. B. K., Jamil, Z., and Sarwar, H. (2016). Effects of dietary selenium nanoparticles on physiological and biochemical aspects of juvenile tor putitora. Turk. J. Zool. 40, 704–712. doi: 10.3906/zoo-1510-5
Khiralla, G. M., and El-Deeb, B. A. (2015). Antimicrobial and antibiofilm effects of selenium nanoparticles on some foodborne pathogens. LWT Food Sci. Technol. 63, 1001–1007. doi: 10.1016/j.lwt.2015.03.086
Khurana, A., Tekula, S., Saifi, M. A., Venkatesh, P., and Godugu, C. (2019). Therapeutic applications of selenium nanoparticles. Biomed. Pharmacother. 111, 802–812. doi: 10.1016/j.biopha.2018.12.14
Kiełczykowska, M., Kocot, J., Paździor, M., and Musik, I. (2018). Selenium - a fascinating antioxidant of protective properties. Adv. Clin. Exp. Med. 27, 245–255. doi: 10.17219/acem/68826
Korde, P., Ghotekar, S., Pagar, T., Pansambal, S., Oza, R., and Mane, D. (2020). Plant extract assisted eco-benevolent synthesis of selenium nanoparticles-a review on plant parts involved, characterization and their recent applications. J. Chem. Rev. 2, 157–168. doi: 10.22034/JCR.2020.106601
Kumar, N., Gupta, S. K., Chandan, N. K., Bhushan, S., Singh, D. K., Kumar, P., et al. (2020). Mitigation potential of selenium nanoparticles and riboflavin against arsenic and elevated temperature stress in Pangasianodon hypophthalmus. Sci. Rep. 10, 17883–17817. doi: 10.1038/s41598-020-74911-2
Kumar, N., Krishnani, K. K., Gupta, S. K., Sharma, R., Baitha, R., Singh, D. K., et al. (2018). Immuno-protective role of biologically synthesized dietary selenium nanoparticles against multiple stressors in Pangasinodon hypophthalmus. Fish Shellfish Immunol. 78, 289–298. doi: 10.1016/j.fsi.2018.04.051
Kumar, S., Tomar, M. S., and Acharya, A. (2015). Carboxylic group-induced synthesis and characterization of selenium nanoparticles and its anti-tumor potential on Dalton's lymphoma cells. Colloids Surf. B: Biointerfaces 126, 546–552. doi: 10.1016/j.colsurfb.2015.01.009
Lang, C., Mission, E. G., Fuaad, A. A. H. A., and Shaalan, M. (2021). Nanoparticle tools to improve and advance precision practices in the Agrifoods sector towards sustainability-a review. J. Clean. Prod. 293:126063. doi: 10.1016/j.jclepro.2021.126063
Li, X., Huang, T., Heath, D. E., O’Brien-Simpson, N. M., and O’Connor, A. J. (2020). Antimicrobial nanoparticle coatings for medical implants: design challenges and prospects. Biointerphases 15:060801. doi: 10.1116/6.0000625
Longbaf Dezfouli, M., Ghaedtaheri, A., Keyvanshokooh, S., Salati, A. P., Mousavi, S. M., and Pasha-Zanoosi, H. (2019). Combined or individual effects of dietary magnesium and selenium nanoparticles on growth performance, immunity, blood biochemistry and antioxidant status of Asian seabass (Lates calcarifer) reared in freshwater. Aquac. Nutr. 25, 1422–1430. doi: 10.1111/anu.12962
Mechlaoui, M., Dominguez, D., Robaina, L., Geraert, P.-A., Kaushik, S., Saleh, R., et al. (2019). Effects of different dietary selenium sources on growth performance, liver and muscle composition, antioxidant status, stress response and expression of related genes in gilthead seabream (Sparus aurata). Aquaculture 507, 251–259. doi: 10.1016/j.aquaculture.2019.04.037
Menon, S., Ks, S. D., Santhiya, R., Rajeshkumar, S., and Kumar, V. (2018). Selenium nanoparticles: a potent chemotherapeutic agent and an elucidation of its mechanism. Colloids Surf. B: Biointerfaces 170, 280–292. doi: 10.1016/j.colsurfb.2018.06.006
Molano, A., Jones, S. M., Brown, J. L., and Aber, J. L. (2013). Selection and socialization of aggressive and prosocial behavior: the moderating role of social-cognitive processes. J. Res. Adolesc. 23, 424–436. doi: 10.1111/jora.12034
Mosallam, F. M., El-Sayyad, G. S., Fathy, R. M., and El-Batal, A. I. (2018). Biomolecules-mediated synthesis of selenium nanoparticles using Aspergillus oryzae fermented Lupin extract and gamma radiation for hindering the growth of some multidrug-resistant bacteria and pathogenic fungi. Microb. Pathog. 122, 108–116. doi: 10.1016/j.micpath.2018.06.013
Moslemi, M. K., and Tavanbakhsh, S. (2011). Selenium–vitamin E supplementation in infertile men: effects on semen parameters and pregnancy rate. Int. J. Gen. Med. 4, 99–104. doi: 10.2147/ijgm.s16275
Murtala, B. A., Abdul, W. O., and Akinyemi, A. A. (2012). Bioaccumulation of heavy metals in fish (Hydrocynus forskahlii, Hyperopisus bebe occidentalis and Clarias gariepinus) organs in downstream Ogun coastal water, Nigeria. TJST 4, 119–133. doi: 10.5539/jas.v4n11p51
Nancharaiah, Y. V., and Lens, P. N. (2015). Selenium biomineralization for biotechnological applications. Trends Biotechnol. 33, 323–330. doi: 10.1016/j.tibtech.2015.03.004
Neamat-Allah, A. N., Mahmoud, E. A., and Abd El Hakim, Y. (2019). Efficacy of dietary Nano-selenium on growth, immune response, antioxidant, transcriptomic profile and resistance of Nile tilapia, Oreochromis niloticus against Streptococcus iniae infection. Fish Shellfish Immunol. 94, 280–287. doi: 10.1016/j.fsi.2019.09.019
Neetha, R., Nikhil, J., and Chitra, K. C. (2022). A comparative study on the sensitivity of selected freshwater fishes against triclosan exposure. Drug. Chem. Toxicol. 1–14. doi: 10.1080/01480545.2022.2131813
Negahdari, S., Sabaghan, M., Pirhadi, M., Alikord, M., Sadighara, P., Darvishi, M., et al. (2021). Potential harmful effects of heavy metals as a toxic and carcinogenic agent in marine food-an overview. Egypt. J. Vet. Sci. 52, 379–385. doi: 10.21608/ejvs.2021.83716.1245
Omar, W. A., Zaghloul, K. H., Abdel-Khalek, A. A., and Abo-Hegab, S. (2012). Genotoxic effects of metal pollution in two fish species, Oreochromis niloticus and Mugil cephalus, from highly degraded aquatic habitats. Mutat. Res. 746, 7–14. doi: 10.1016/j.mrgentox.2012.01.013
Osman, A., Goda, H. A., and Sitohy, M. (2018). Storage stability of minced beef supplemented with chickpea legumin at 4 C as a potential substitute for nisin. LWT Food Sci. Technol. 93, 434–441. doi: 10.1016/j.lwt.2018.03.071
Palmisano, G., Palumbo, G., and Pennisi, S. (2001). Design procedure for two-stage CMOS transconductance operational amplifiers: a tutorial. Analog Integr. Circ. Sig. Process 27, 179–189. doi: 10.1023/A:1011229119071
Phillips, C. J., Farrugia, C., Lin, C. H., Mancera, K., and Doneley, B. (2018). The effect providing space in excess of standards on the behaviour of budgerigars in aviaries. Appl. Anim. Behav. Sci. 199, 89–93. doi: 10.1016/j.applanim.2017.10.015
Pouri, S., Motamedi, H., Honary, S., and Kazeminezhad, I. (2017). Biological synthesis of selenium nanoparticles and evaluation of their bioavailability. Braz. Arch. Biol. Technol. 60. doi: 10.1590/1678-4324-2017160452
Prasad, K. S., Vaghasiya, J. V., Soni, S. S., Patel, J., Patel, R., Kumari, M., et al. (2015). Microbial selenium nanoparticles (SeNPs) and their application as a sensitive hydrogen peroxide biosensor. Appl. Biochem. Biotechnol. 177, 1386–1393. doi: 10.1007/s12010-015-1814-9
Qi, W. Y., Li, Q., Chen, H., Liu, J., Xing, S. F., Xu, M., et al. (2021). Selenium nanoparticles ameliorate Brassica napus L. cadmium toxicity by inhibiting the respiratory burst and scavenging reactive oxygen species. J. Hazard. Mater. 417:125900. doi: 10.1016/j.jhazmat.2021.125900
Rathore, S. S., Murthy, H. S., Mamun, M. A. A., Nasren, S., Rakesh, K., Kumar, B. T. N., et al. (2021). Nano-selenium supplementation to ameliorate nutrition physiology, immune response, antioxidant system and disease resistance against Aeromonas hydrophila in monosex Nile tilapia (Oreochromis niloticus). Biol. Trace Elem. Res. 199, 3073–3088. doi: 10.1007/s12011-020-02416-0
Ren, L., Wu, Z., Ma, Y., Jian, W., Xiong, H., and Zhou, L. (2021). Preparation and growth-promoting effect of selenium nanoparticles capped by polysaccharide-protein complexes on tilapia. J. Sci. Food Agric. 101, 476–485. doi: 10.1002/jsfa.10656
Rotruck, J. T., Pope, A. L., Ganther, H. E., Swanson, A. B., Hafeman, D. G., and Hoekstra, W. (1973). Selenium: biochemical role as a component of glutathione peroxidase. Science 179, 588–590. doi: 10.1126/science.179.4073.588
Saad, A. M., Sitohy, M. Z., Ahmed, A. I., Rabie, N. A., Amin, S. A., Aboelenin, S. M., et al. (2021). Biochemical and functional characterization of kidney bean protein alcalase-hydrolysates and their preservative action on stored chicken meat. Molecules 26:4690. doi: 10.3390/molecules26154690
Saffari, S., Keyvanshokooh, S., Zakeri, M., Johari, S. A., and Pasha-Zanoosi, H. J. A. N. (2017). Effects of different dietary selenium sources (sodium selenite, selenomethionine and nanoselenium) on growth performance, muscle composition, blood enzymes and antioxidant status of common carp (Cyprinus carpio). Aquac. Nutr. 23, 611–617. doi: 10.1111/anu.12428
Saffari, S., Keyvanshokooh, S., Zakeri, M., Johari, S. A., Pasha-Zanoosi, H., and Mozanzadeh, M. T. (2018). Effects of dietary organic, inorganic, and nanoparticulate selenium sources on growth, hemato-immunological, and serum biochemical parameters of common carp (Cyprinus carpio). Fish Physiol. Biochem. 44, 1087–1097. doi: 10.1007/s10695-018-0496-y
Saitou, N., and Nei, M. (1987). The neighbor-joining method: a new method for reconstructing phylogenetic trees. Mol. Biol. Evol. 4, 406–425. doi: 10.1093/oxfordjournals.molbev.a040454
Salem, S. S. (2022a). Bio-fabrication of selenium nanoparticles using Baker’s yeast extract and its antimicrobial efficacy on food borne pathogens. Appl. Biochem. Biotechnol. 194, 1898–1910. doi: 10.1007/s12010-022-03809-8
Salem, S. S. (2022b). Baker’s yeast-mediated silver nanoparticles: characterisation and antimicrobial biogenic tool for suppressing pathogenic microbes. BioNanoSci. 1-10, 1–10. doi: 10.1007/s12668-022-01026-5
Salem, S. S., Badawy, M. S. E., Al-Askar, A. A., Arishi, A. A., Elkady, F. M., and Hashem, A. H. (2022b). Green biosynthesis of selenium nanoparticles using orange peel waste: characterization, antibacterial and antibiofilm activities against multidrug-resistant bacteria. Life 12:893. doi: 10.3390/life12060893
Salem, S. S., and Fouda, A. (2021). Green synthesis of metallic nanoparticles and their prospective biotechnological applications: an overview. Biol. Trace Elem. Res. 199, 344–370. doi: 10.1007/s12011-020-02138-3
Salem, S. S., Fouda, M. M., Fouda, A., Awad, M. A., Al-Olayan, E. M., Allam, A. A., et al. (2021). Antibacterial, cytotoxicity and larvicidal activity of green synthesized selenium nanoparticles using Penicillium corylophilum. J. Clust. Sci. 32, 351–361. doi: 10.1007/s10876-020-01794-8
Salem, S. S., Hammad, E. N., Mohamed, A. A., and El-Dougdoug, W. (2022a). A comprehensive review of nanomaterials: types, synthesis, characterization, and applications. Biointerface Res. Appl. Chem. 13:41. doi: 10.33263/BRIAC131.041
Sekiguchi, Y., and Kohshima, S. (2003). Resting behaviors of captive bottlenose dolphins (Tursiops truncatus). Physiol. Behav. 79, 643–653. doi: 10.1016/s0031-9384(03)00119-7
Shaalan, M., Sellyei, B., El-Matbouli, M., and Székely, C. (2020). Efficacy of silver nanoparticles to control flavobacteriosis caused by Flavobacterium johnsoniae in common carp Cyprinus carpio. Dis. Aquat. Org. 137, 175–183. doi: 10.3354/dao03439
Sheir, S. H., Ibrahim, H., Hassan, M. A., and Shawky, N. A. (2020). Prevalence of Aeromonas spp and their virulence factors isolated from frozen chicken meat products. BVMJ 39, 47–51. doi: 10.21608/bvmj.2020.37742.1237
Shi, L. G., Yang, R. J., Yue, W. B., Xun, W. J., Zhang, C. X., Ren, Y. S., et al. (2010). Effect of elemental nano-selenium on semen quality, glutathione peroxidase activity, and testis ultrastructure in male Boer goats. Anim. Reprod. Sci. 118, 248–254. doi: 10.1016/j.anireprosci.2009.10.003
Sitohy, M., Al-Mohammadi, A. R., Osman, A., Abdel-Shafi, S., El-Gazzar, N., Hamdi, S., et al. (2021). Silver-protein Nanocomposites as antimicrobial agents. Nano 11:3006. doi: 10.3390/nano11113006
Sitohy, M., Mahgoub, S., and Osman, A. (2011). Controlling psychrotrophic bacteria in raw buffalo milk preserved at 4 C with esterified legume proteins. LWT 44, 1697–1702. doi: 10.1016/j.lwt.2011.03.008
Sitohy, M. Z., Mahgoub, S. A., and Osman, A. O. (2012). In vitro and in situ antimicrobial action and mechanism of glycinin and its basic subunit. Int. J. Food Microbiol. 154, 19–29. doi: 10.1016/j.ijfoodmicro.2011.12.004
Sitohy, M., Mahgoub, S., Osman, A., El-Masry, R., and Al-Gaby, A. (2013). Extent and mode of action of cationic legume proteins against Listeria monocytogenes and Salmonella Enteritidis. Probiotics Antimicrob. Proteins 5, 195–205. doi: 10.1007/s12602-013-9134-2
Sitohy, M., and Osman, A. (2010). Antimicrobial activity of native and esterified legume proteins against gram-negative and gram-positive bacteria. Food Chem. 120, 66–73. doi: 10.1016/j.foodchem.2009.09.071
Skalickova, S., Milosavljevic, V., Cihalova, K., Horky, P., Richtera, L., and Adam, V. (2017). Selenium nanoparticles as a nutritional supplement. Nutrition 33, 83–90. doi: 10.1016/j.nut.2016.05.001
Sonkusre, P. (2020). Specificity of biogenic selenium nanoparticles for prostate cancer therapy with reduced risk of toxicity: an in vitro and in vivo study. Front. Oncol. 9:1541. doi: 10.3389/fonc.2019.01541
Srivastava, P., and Kowshik, M. (2016). Anti-neoplastic selenium nanoparticles from Idiomarina sp. PR58-8. Enzym. Microb. Technol. 95, 192–200. doi: 10.1016/j.enzmictec.2016.08.002
Swain, P., Das, R., Das, A., Padhi, S. K., Das, K. C., and Mishra, S. S. (2019). Effects of dietary zinc oxide and selenium nanoparticles on growth performance, immune responses and enzyme activity in rohu, Labeo rohita (Hamilton). Aquac. Nutr. 25, 486–494. doi: 10.1111/anu.12874
Talas, Z. S., Orun, I., Ozdemir, I., Erdogan, K., Alkan, A., and Yılmaz, I. (2008). Antioxidative role of selenium against the toxic effect of heavy metals (cd+ 2, Cr+ 3) on liver of rainbow trout (Oncorhynchus mykiss Walbaum 1792). Fish Physiol. Biochem. 34, 217–222. doi: 10.1007/s10695-007-9179-9
Tamura, K., Nei, M., and Kumar, S. (2004). Prospects for inferring very large phylogenies by using the neighbor-joining method. Proc. Natl. Acad. Sci. U.S.A. 101, 11030–11030. doi: 10.1073/pnas.0404206101
Vieira, E. F., Carvalho, J., Pinto, E., Cunha, S., Almeida, A. A., and Ferreira, I. M. (2016). Nutritive value, antioxidant activity and phenolic compounds profile of brewer’s spent yeast extract. J. Food Compos. Anal. 52, 44–51. doi: 10.1016/j.jfca.2016.07.006
Wang, Q., Garrity, G. M., Tiedje, J. M., and Cole, J. R. (2007). Naive Bayesian classifier for rapid assignment of rRNA sequences into the new bacterial taxonomy. Appl. Environ. Microbiol. 73, 5261–5267. doi: 10.1128/AEM.00062-07
Wang, L., Wang, L., Zhang, D., Li, S., Yin, J., Xu, Z., et al. (2021). Effect of dietary selenium on postprandial protein deposition in the muscle of juvenile rainbow trout (Oncorhynchus mykiss). Br. J. Nutr. 125, 721–731. doi: 10.1017/S000711452000313X
Wang, X., Zhang, D., Qian, H., Liang, Y., Pan, X., and Gadd, G. M. (2018). Interactions between biogenic selenium nanoparticles and goethite colloids and consequence for remediation of elemental mercury contaminated groundwater. Sci. Total Environ. 613-614, 672–678. doi: 10.1016/j.scitotenv.2017.09.113
Yanhua, W., Hao, H., Li, Y., and Zhang, S. (2016). Selenium-substituted hydroxyapatite nanoparticles and their in vivo antitumor effect on hepatocellular carcinoma. Colloids Surf. B: Biointerfaces 140, 297–306. doi: 10.1016/j.colsurfb.2015.12.056
Yu, H., Zhang, C., Zhang, X., Wang, C., Li, P., Liu, G., et al. (2020). Dietary nano-selenium enhances antioxidant capacity and hypoxia tolerance of grass carp Ctenopharyngodon idella fed with high-fat diet. Aquac. Nutr. 26, 545–557. doi: 10.1111/anu.13016
Zahmatkesh, A., Karimzadeh, K., and Faridnia, M. (2020). Effect of dietary selenium nanoparticles and chitosan oligosaccharide on biochemical parameters of Caspian roach (Rutilus caspicus) under malathion stress. Casp. J. Environ. Sci. 18, 59–71. doi: 10.22124/cjes.2020.3979
Zeng, L., Chen, Y., Zhang, Q., Guo, X., Peng, Y., Xiao, H., et al. (2015). Adsorption of cd (II), cu (II) and Ni (II) ions by cross-linking chitosan/rectorite nano-hybrid composite microspheres. Carbohydr. Polym. 130, 333–343. doi: 10.1016/j.carbpol.2015.05.015
Zhang, M., Yin, X., Li, M., Wang, R., Qian, Y., and Hong, M. (2020). Effect of nitrite exposure on haematological status, oxidative stress, immune response and apoptosis in yellow catfish (Pelteobagrus fulvidraco). Comp. Biochem. Physiol. C Toxicol. Pharmacol. 238:108867. doi: 10.1016/j.cbpc.2020.108867
Keywords: Bacillus, bacterial load, behavior, Bio-SeNPs, fish, growth, heavy metals, performance
Citation: Saad AM, Sitohy MZ, Sultan-Alolama MI, El-Tarabily KA and El-Saadony MT (2022) Green nanotechnology for controlling bacterial load and heavy metal accumulation in Nile tilapia fish using biological selenium nanoparticles biosynthesized by Bacillus subtilis AS12. Front. Microbiol. 13:1015613. doi: 10.3389/fmicb.2022.1015613
Received: 09 August 2022; Accepted: 31 October 2022;
Published: 23 December 2022.
Edited by:
Randhir Makkar, Guild BioSciences, United StatesCopyright © 2022 Saad, Sitohy, Sultan-Alolama, El-Tarabily and El-Saadony. This is an open-access article distributed under the terms of the Creative Commons Attribution License (CC BY). The use, distribution or reproduction in other forums is permitted, provided the original author(s) and the copyright owner(s) are credited and that the original publication in this journal is cited, in accordance with accepted academic practice. No use, distribution or reproduction is permitted which does not comply with these terms.
*Correspondence: Khaled A. El-Tarabily, a3RhcmFiaWx5QHVhZXUuYWMuYWU=
Disclaimer: All claims expressed in this article are solely those of the authors and do not necessarily represent those of their affiliated organizations, or those of the publisher, the editors and the reviewers. Any product that may be evaluated in this article or claim that may be made by its manufacturer is not guaranteed or endorsed by the publisher.
Research integrity at Frontiers
Learn more about the work of our research integrity team to safeguard the quality of each article we publish.