- 1Department of Plant Protection, Shandong Agricultural University, Tai’an, Shandong, China
- 2Shandong Laboratory of Advanced Agricultural Sciences at Weifang, Peking University Institute of Advanced Agricultural Sciences, Weifang, Shandong, China
The use of polysaccharides to induce the systemic immune response of plants for disease resistance has become an effective plant protection measure. Sharp eyespot wheat and crown rot wheat are serious diseases of wheat. In this study, the control effects of hexaconazole and lentinan (LNT) seed dressing of the two wheat diseases were evaluated by field experiments, and the effects of the seed dressing on plant growth, soil enzyme activity, and community diversity in the wheat rhizosphere were discussed. The results showed that the combined seed dressing of hexaconazole at 0.5 a.i. g·100 kg−1 and LNT at 4 a.i. g·100 kg−1 could significantly improve the control effect of the two wheat diseases. The combined treatment of hexaconazole and LNT had little effect on wheat soil enzyme activities. Different seed dressing treatments changed the fungal community structure in the wheat rhizosphere soil, and the combination of LNT and hexaconazole reduced the relative abundance of Rhizoctonia, Cladosporium, Fusarium, Bipolaris, and Gibberella in wheat planting soils. These findings suggested that the combined seed dressing of hexaconazole and LNT could effectively control soilborne diseases of wheat, concurrently could change in rhizosphere fungal community, and reduce in potential soilborne pathogens.
Introduction
Wheat is an important food crop worldwide (Adrees et al., 2020). Soilborne fungal diseases cost the wheat industry billions of dollars each year (Zhang et al., 2021a). Wheat sharp eyespot is a globally prevalent soilborne disease caused by Rhizoctonia cerealis (Hamada et al., 2011), which can occur throughout the growth period of wheat, infecting leaf sheaths and stems and affecting the transport of nutrients and water, causing wheat lodging and dieback (Lemańczyk and Kwaśna, 2013). Wheat crown rot is an important soilborne disease caused by the infection of various pathogens such as Fusarium pseudograminearum and F. graminearum. Due to different regions, the dominant pathogens are also different (Kazan and Gardiner, 2018; Deng et al., 2020). Wheat crown rot has spread rapidly in China in the past decade, which, in severe cases, can lead to plant death, withering, and white ears (Deng et al., 2020). Soilborne diseases are usually difficult to control, and the combined infection of various soilborne fungal diseases will aggravate the occurrence of wheat diseases. In recent years, with the development of global climate change, straw turnover practices, mechanized cross-regional operations, and other labor-saving farming systems, the incidence and distribution of wheat sharp eyespot and wheat crown rot have increased significantly, seriously threatening wheat yield and quality (Hamada et al., 2011).
Although soilborne diseases can be prevented through crop rotation, selection of disease-resistant varieties, and solar disinfection, chemical control is still the most rapid and effective means when diseases break out (Wang et al., 2022). Commonly used fungicides for wheat sharp eyespot are difenoconazole and tebuconazole. However, due to the long-term single usage of chemical pesticides, R. cerealis has exhibited decreased sensitivity to a variety of chemical agents (Qi et al., 2014). Stem and foliar spray for wheat sharp eyespot control is time-consuming and is not environmentally friendly. Seed treatment with systemic fungicides can provide integrated control of wheat seedling and foliar diseases, improve the pesticide utilization rate, and reduce the required dosage and thus has become the focus of wheat disease management in recent years. Almasudy et al. (2015) reported that “ipconazole + metalaxyl + N-methyl-2-pyrrolidone” seed dressing treatment can significantly reduce the incidence of sharp eyespot. Ren et al. (2019) found that 6% difenoconazole-fludioxonil FSC can effectively control wheat sharp eyespot. At present, there is no registered medicine for the prevention and control of wheat crown rot in China, but many studies have shown that chemical seed dressing can effectively prevent and control the occurrence of wheat crown rot. Moya-Elizondo and Jacobsen (2016) showed that difenoconazole-mefenoxam fungicide seed treatment could reduce the severity of wheat crown rot by 29–50%, and Wang et al. (2022) reported that wheat associated with seed dressing treatment with pyraclostrobin suspension seed coating could achieve a 50.33% control effect.
Polysaccharides are complex carbohydrates with biological regulatory functions. These compounds not only provide energy for plant growth but also act as signal substances to regulate physiological processes and enhance plant disease resistance. Fungal polysaccharides have exhibited broad application prospects due to their lack of toxicity, easy degradation, environmental friendliness, and ability to induce plant disease resistance. Studies have shown that LNT can induce plant disease resistance and has an inhibitory effect on diseases. Li et al. (2013) reported that LNT showed a direct inhibitory efficiency of only 5.08% against Phytophthora infestans but exhibited a control efficiency of 83.00% in vivo. The use of polysaccharides to induce the systemic immune response of plants for disease resistance has become an effective plant protection measure.
Soilborne diseases, in addition to chemical control, can also be prevented by adjusting soil physical and chemical properties and improving soil microbial community composition. Soil microbes play key roles in ecosystems, especially fungi (Cai et al., 2021). Beneficial fungi in soil microorganisms can promote the growth and development of plants (Jia et al., 2018). Similarly, some soilborne fungi can survive in soil or diseased debris and infect plants in the form of mycelium and spores cause disease. Wheat sharp eyespot and wheat crown rot are soilborne diseases caused by pathogenic fungi, which accumulate continuously in the soil and seriously endanger the production of wheat. The complexity and diversity of microorganisms in the rhizosphere are critical for maintaining soil ecosystem homeostasis (Winter et al., 2014). Therefore, understanding the relationship between the diversity of the soil fungal community and the occurrence of wheat soilborne diseases before and after chemical seed dressing can control wheat soilborne fungal diseases by changing the nature and composition of the soil community, which provides a theoretical basis for disease control.
In a previous study, seed treatment with LNT promoted wheat germination and seedling growth in the variety Jimai 22, and the effects were dose dependent. Mechanistic analysis showed that LNT increased the transcription of genes related to alternative oxidase (AOX), β-1, 3-glucanase (GLU), and the salicylic acid signaling pathway and disease resistance, especially AOX (Zhang et al., 2017a). On this basis, we intend to systematically analyze the field control effect against two wheat diseases and the rhizosphere soil fungal community effect of LNT and hexaconazole seed treatment to provide a scientific basis for its safe and rational application.
Materials and methods
Test material and seed treatment
Seeds of Jimai 22 were provided by the Agricultural College, Shandong Agricultural University. Hexaconazole (95%) was provided by Shandong United Pesticide Industry Co. Ltd., China. Prepare 1 × 104 mg/l with acetone and store at 4°C. The LNT with a polysaccharide content of 91% was extracted and purified in the laboratory in the early stage (Zhang et al., 2017a).
The preparation of the seed coating agent adopts the wet sand grinding ultra-fine pulverization method (Marín et al., 2016), and the other components such as the original drug, dispersant, thickener, physical stabilizer, and appropriate amount of water were mixed uniformly in a certain proportion. After pre-dispersing with a high shear emulsifier (Guangzhou Yike Laboratory Technology Co., Ltd.), the slurry was moved into a sand mill, sanded under cooling conditions, a film-forming agent was added, and a laser particle size analyzer (Jinan Runzhi Technology Co., Ltd.) after testing that the particle size of the sample is qualified, add rose essence, grind for 0.5 h, then adjust and grind the remaining water for another 0.5 h, and filter to obtain the finished product (Ren et al., 2019).
In all experiments, the coating was applied at a dose of 100 ml per 100 kg of seeds. There were 4 treatments for wheat seeds: (1) LNT (8 a.i. g·100 kg−1); (2) hexaconazole (1 a.i. g·100 kg−1) (3) (4 a.i. g·100 kg−1) LNT + (0.5 a.i. g·100 kg−1) hexaconazole, and (4) sterile water treatment (control).
Site description and experimental design
From 2018 to 2020, a two-year field experiment was conducted on the plot of the South Campus of Shandong Agricultural University (117°13′E, 36°20′N). This area has a warm temperate semi-humid continental monsoon climate, with rain and heat in the same season, moderate rainfall, the annual average temperature is 13.2°C, and the annual average annual precipitation is 683.2 mm. Wheat is grown all year round in this area, which suffers from severe wheat sharp eyespot and wheat crown rot. It belongs to fertile brown soil. The experiment was arranged in random blocks, and each treatment was repeated 3 times. The plot area was 3.75 m2 and the row spacing was 0.25 m. The occurrence of wheat sharp eyespot and wheat crown rot at the overwintering, elongation, and grain filling stages was studied. All cultivation and management conditions in the experimental area were uniform. Wheat sharp eyespot disease incidences and indexes were graded from 0 to 7 as follows (Peng et al., 2014): grade 0: no disease; grade 1: the leaf sheath is affected but the stem is not affected; grade 3: the leaf sheath is affected and invades the stem, but the stem lesions are less than 1/2 of the stem; grade 5: the stem lesions are more than 1/2, but not lodging or breaking; and grade 7: lodging and withering.
Grading standard of wheat crown rot at the emergence stage and jointing stage is as follows (Erginbas-Orakci et al., 2016): grade 0: no browning symptoms on the whole plant; grade 1: browning of the coleoptile or ground stem; grade 2: plant coleoptiles and stems turn brown; grade 3: the first leaf sheath turns brown; grade 4: the second leaf sheath turns brown; and grade 5: the third leaf sheath turns brown or the whole stem turns brown and soft.
Grain-filling stage grading standard is as follows: grade 0: no browning symptoms on the innermost leaf sheath and the whole stem; grade 1: obvious browning on the innermost leaf sheath in the aerial part, and no browning and rot at the first stem node; grade 3: above ground part of the first stem node has browning and rot phenomenon; grade 5: the second stem node of the aerial part has browning and rot phenomenon; grade 7: the third stem node of the aerial part has browning and rot phenomenon; and grade 9: The lesions exceed the third stem node, with white spikes or no spikes due to disease.
Disease severity was calculated as follows (Peng et al., 2014; Zhang et al., 2017a):
The control efficiency of each treatment was calculated as follows:
After harvest, wheat grain number per ear and thousand seed weight were determined.
Greenhouse emergence test and sample description
The soil used in this test was collected from the south campus of Shandong Agricultural University (117°13′E, 36°20′N) in China. The plow layer soil (5–15 cm) was collected randomly and passed through a 1-mm sieve for planting the experimental wheat. Seeds of Jimai 22 were treated as described for seed treatment. The original brown soil, brown soil treated with water only, and brown soil sown with wheat seeds treated with water only were used as controls. The plastic pots were filled with soil with uniform nutrients and particles and a water content of about 60%, a plastic pot sown with 10 treated seeds was considered a replicate, and each treatment was repeated 3 times, and cultivated in the greenhouse of Shandong Agricultural University. The growth status of crops was recorded at 7, 14, and 21 days after emergence, and the plant height among the treatments was counted. Twenty-one days after total germination in the control, the wheat rhizosphere soil was collected and divided into two parts. One subsample was stored at 4°C for the soil enzyme activity test, and the other subsample was stored in dry ice for high-throughput sequencing of fungal community diversity.
Determination of soil enzyme activity
The activities of two soil enzymes were tested with standard methods. Soil catalase (EC 1.11.1.6, CAT) activity was determined with the KMnO4 titrimetric method, and urease (EC 3.5.1.5, URE) activity was determined with the colorimetric method according to Yu et al. (2019).
DNA extraction, amplification, and sequencing
Total DNA of soil samples was extracted with the E.Z.N.A. ®Stool DNA Kit (D4015, Omega, Inc., United States). Primers fITS7 (5’-GTGARTCATCGAATCTTTG-3′) and ITS4 (5’-TCCTCCGCTTATTGATATGC-3′) were used to amplify the ITS2 fragment tagged with specific barcodes at the 5′ ends (Karlsson et al., 2014). The PCR mixture consisted of 25 ng of template DNA, 12.5 μl of PCR Premix, 2.5 μl of each primer, and ddH2O to a volume of 25 μl. The PCR conditions to amplify the eukaryotic ITS fragments consisted of an initial denaturation at 98°C for 30 s; 35 cycles of denaturation at 98°C for 10 s, annealing at 54°C/52°C for 30 s, and extension at 72°C for 45 s; and then final extension at 72°C for 10 min. After recovery and purification of PCR products by gel electrophoresis, AMPure XT beads and Qubit were used for verification and quantification, respectively. By size and quantity assessment, a library of amplicons required for subsequent sequencing was prepared. The qualified libraries were sequenced on a 250PE MiSeq platform.
Data analysis and bioinformatics analysis
SPSS 22.0 was used for statistical analysis of the data, and Duncan’s new multiple range method was used for multiple comparisons between treatments. Paired-end reads were assigned to samples according to their barcode. After eliminating barcodes and primer sequences and merging paired-end reads, raw tags were filtered to obtain clean tags. Chimeric sequences were filtered using Verseach software (v2.3.4). For ease of analysis, sequences are usually divided into different OTUs according to a 97% similarity threshold, and each OTU is treated as a microbial species (Blaxter et al., 2005). That is to say, if the similarity is less than 97%, it can be regarded as different species, and if the similarity is less than 93% ~ 95%, it can be regarded as belonging to different genera.
Representative sequences were assigned taxonomic data with the RDP (Ribosomal Database Project) classifier. The phylogenetic relationship of different dominant OTUs in different groups was analyzed by PyNAST with the multiple sequence alignment method. OTU abundance information was normalized corresponding to the sample with the fewest sequences. Alpha diversity indexes, calculated with QIIME (Version 1.8.0), were used to represent species diversity, including Chao1, Shannon, Simpson, and observed species indices. Beta diversity analysis was used to evaluate differences of samples in species complexity. Beta diversity was calculated by principal co-ordinates analysis (PCoA) and cluster analysis by QIIME software (Version 1.8.0). Redundancy analysis (RDA) of soil colonies and soil properties using CANOCO4.5.
Results
Field control efficiency of hexaconazole, LNT, and their combination against wheat sharp eyespot and wheat crown rot
In the field test, seed dressing with LNT alone exhibited lower efficiency of control against wheat sharp eyespot and wheat crown rot (Tables 1, 2). During overwintering, the control effect of 8 a.i. g·100 kg−1 LNT on Jimai 22 was lower than 40%. Seed dressing with hexaconazole showed excellent control efficiency against two wheat diseases in the earlier stages of wheat growth. During overwintering, the control effects of hexaconazole at 1 a.i. g·100 kg−1 against sharp eyespot were 81.5% and 76.9%, respectively and the control effects on wheat crown rot were 75.7% and 81.4%, respectively. However, the effects decreased with time during the grain filling stage; the control effects on the two diseases were 38.8%, 35.6%, 30.5%, and 29.8%, respectively. From the field test results, the combination of LNT with a dosage of hexaconazole showed high and long-lasting control efficiency. During the wintering period, the control effects of 4 a.i. g·100 kg−1 LNT and 0.5 a.i. g·100 kg−1 hexaconazole on Jimai 22 were all above 70% and still above 40% at the grain filling stage, which were significantly higher than those of hexaconazole at 1 a.i. g·100 kg−1.
The number of grains per panicle of Jimai 22 was not significantly different among the different treatments. When Jimai 22 was used in combination with 0.5 a.i. g·100 kg−1 of hexaconazole and 4 a.i. g·100 kg−1 of LNT, the thousand-kernel weights were 45.9 g, 45.6 g, 45.1 g, and 45.4 g, respectively, which represented significant increases compared with the control.
Effects of different seed dressing treatments on the growth of wheat seedlings
From the measured plant height data (Table 3), 8 a.i. g·100 kg−1 LNT seed dressing treatment promoted the growth of Jimai 22. The plant height was averaged at 7, 14, and 21 days after the seeds fully emerged, which was significantly higher than the control. Compared with other treatments, the seed plant height of the 1 a.i. g·100 kg−1 hexaconazole seed dressing treatment was significantly different and lower than that of other treatments. Judging from the photos of potted plants in the greenhouse and the roots of wheat seedlings (Figures 1A,B), hexaconazole has a regulating effect on the growth of wheat, showing that the root system is developed, the aerial part is short and strong, and the leaf color becomes darker, which has a certain effect of strengthening seedlings. The small dose of hexaconazole combined with LNT relieved the inhibitory effect of hexaconazole and promoted the growth of wheat seedlings.
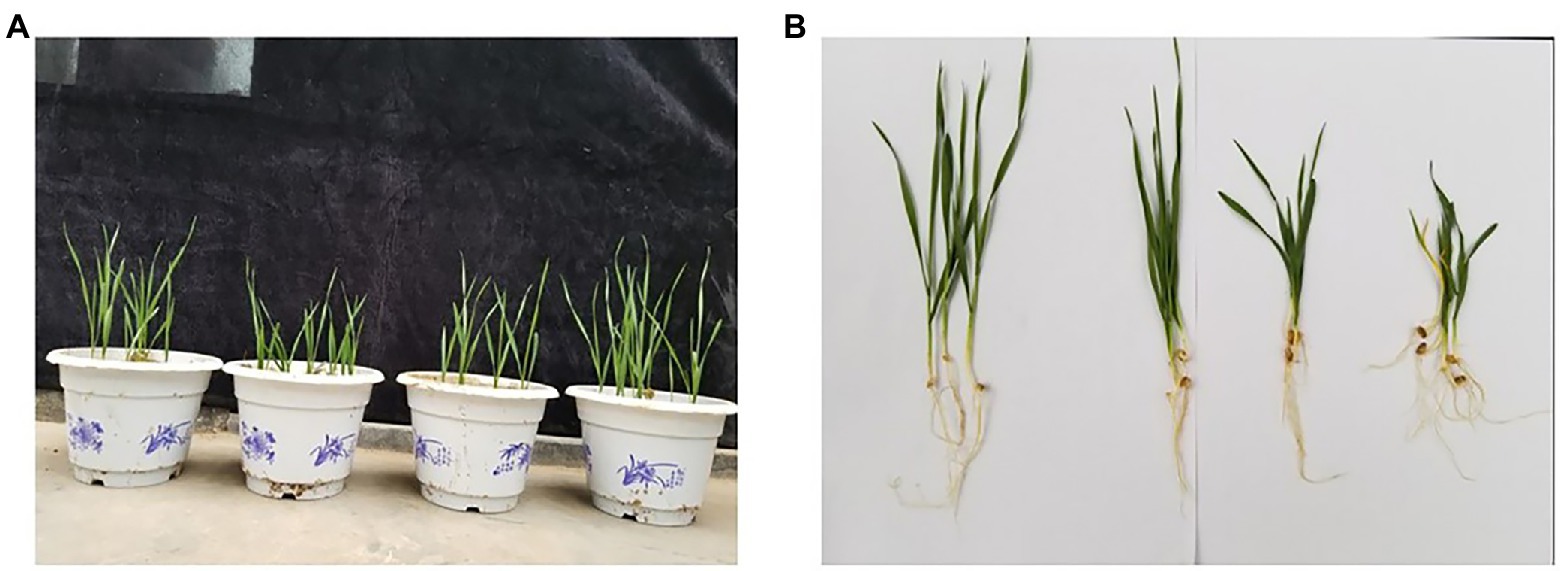
Figure 1. Greenhouse pot experiments on the growth of Jimai 22 with different seed dressing treatments. (A) Effects of different treatments on the growth of Jimai 22. Processing from left to right: CK, Hexaconazole, ḤLNT, LNT. (B) Effects of different seed dressing treatments on the root system of Jimai 22 The treatments from left to right are: LNT, CK, ḤLNT, Hexaconazole.
Effect of hexaconazole and LNT seed dressing on wheat soil enzyme activity
Seed dressing treatment affected the enzymatic activity of wheat rhizosphere soil (Figure 2). Brown original soil (BO) exhibited the lowest CAT and URE activities, followed by brown soil sowed with wheat seeds dressed with hexaconazole (BH), indicating an inhibitory effect of this chemical fungicide on soil enzyme activity. Brown soil sowed with wheat seeds dressed with LNT (BL) showed the highest CAT and URE activities, followed by brown soil sowed with wheat seeds dressed with water (BCK), indicating that LNT has little effect on soil enzyme activities. The soil enzyme activities of hexaconazole and LNT treatments (BHL) were higher than those of hexaconazole-treated seeds in brown soil (BH). A moderate effect on soil enzyme activities was observed in brown soil sown with wheat seeds dressed with hexaconazole and LNT (BHL).
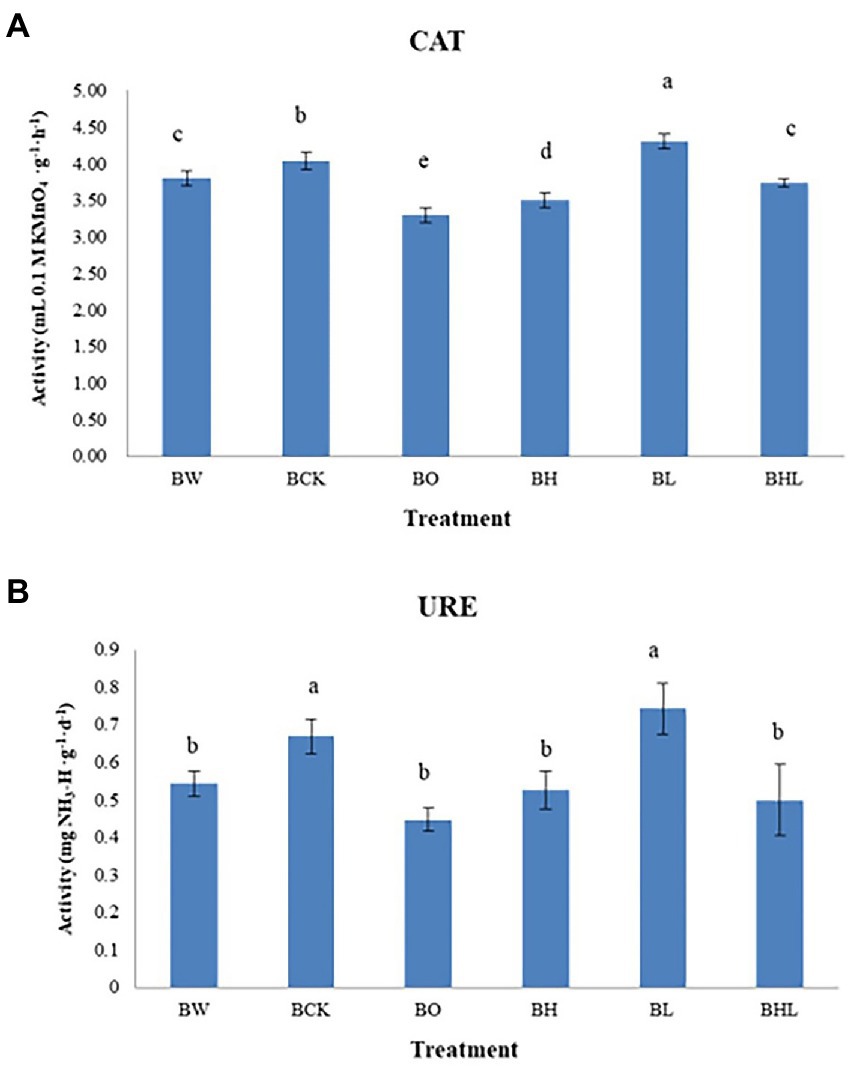
Figure 2. Soil enzyme activities in the different treatments. (A) Effects of different treatments on CAT activity in soil. (B) Effects of different treatments on soil URE activity. BCK – brown soil sowed with wheat seeds dressed with water only; BH – brown soil sowed with wheat seeds dressed with hexaconazole; BHL – brown soil sowed with wheat seeds dressed with hexaconazole and LNT; BL – brown soil sowed with wheat seeds dressed with LNT; BO – brown original soil; BW – brown soil with water only. Different lowercases in the same column indicate significantly different at p < 0.05 level.
Alpha diversity of the soil fungal community under by different wheat seed treatments
The dilution curve of soil can be used to assess whether the measurement is up to standard. As shown in Figure 3, with increasing sequencing data, all dilution curves increased first and then leveled off, which means that the sequencing depth was sufficient for subsequent data analysis.
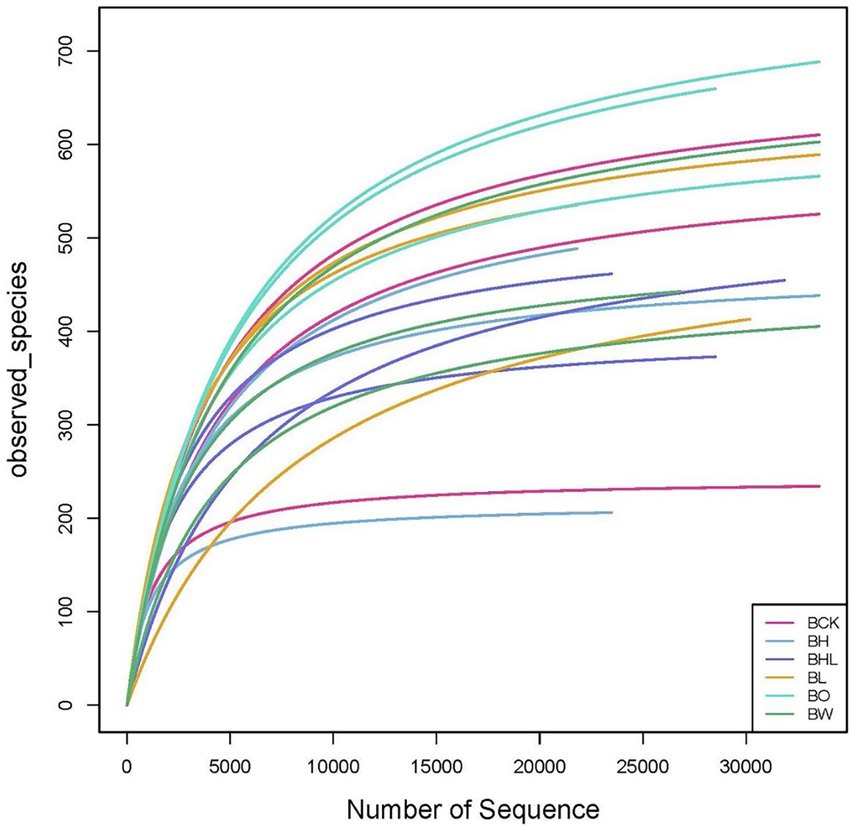
Figure 3. Dilution curve of soil ITS rDNA after wheat seed dressing. Divide OUT at the 97% similarity level and make a sparse curve. The curve of each sample tends to be flat, indicating that the sampling is reasonable. BCK – brown soil sowed with wheat seeds dressed with water only; BH – brown soil sowed with wheat seeds dressed with hexaconazole; BHL – brown soil sowed with wheat seeds dressed with hexaconazole and LNT; BL – brown soil sowed with wheat seeds dressed with LNT; BO – brown original soil; BW – brown soil with water only.
As shown in Table 4, the hexaconazole-containing seed dressing treatments (BH) showed lower observed species and Chao1 indices. These values were lowest in BH, with the lowest observed species and Chao1 indices of 380.3 and 452.0, respectively, indicating the decreasing effect of hexaconazole on soil fungal number and diversity. In the LNT treatment (BL), there was no significant difference in the number of observed species and Chao1 index compared with the brown soil original soil (BO), and the relative abundance was higher. The observed species and Chao1 index of LNT and hexaconazole combined seed dressing (BHL) were 421.3 and 496.9, respectively, lower than those of the other treatments, which had a greater impact on the number and diversity of fungi in the soil.
β-Diversity of the soil fungal community in different wheat seed treatments
PCoA of soil fungi in different treatments was performed based on the Weighted UniFrac distance matrix (Figure 4). The two axes explained 51.57% of the variance, the first axis PCo1 explained 32.79% of the variance, and the second axis PCo2 explained 18.78% of the variance. From the results, different wheat seed treatments changed the structure of the wheat rhizosphere soil fungal community. The distribution of BL and BHL samples was relatively discrete, and the samples were distributed farther, indicating that the LNT treatment and the combined treatment of hexaconazole and LNT had a greater impact on the soil fungal community structure.
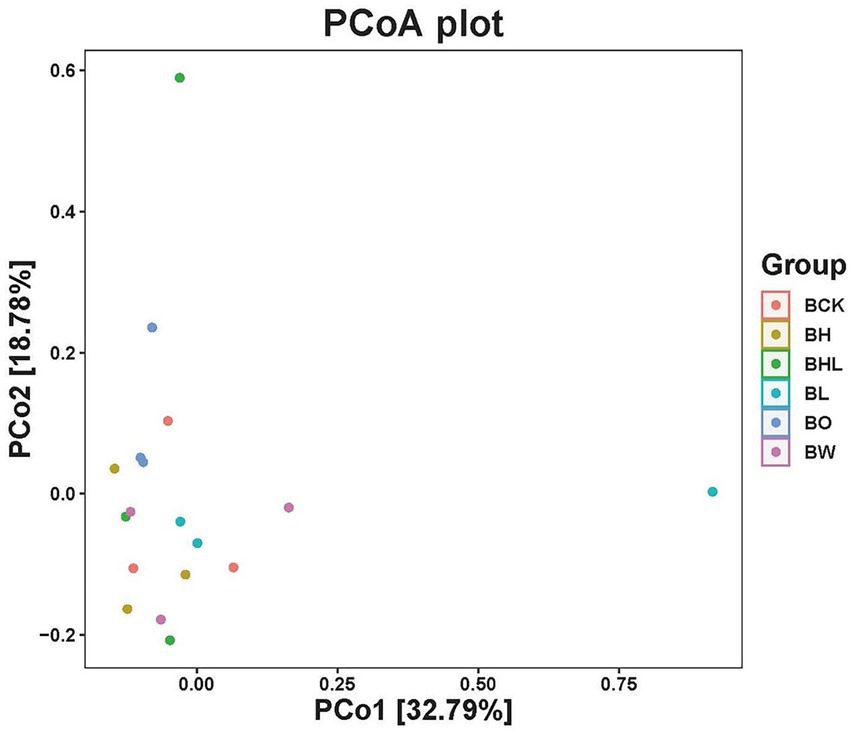
Figure 4. PCoA analysis of soil fungi by different seed treatments. BCK – brown soil sowed with wheat seeds dressed with water only; BH – brown soil sowed with wheat seeds dressed with hexaconazole; BHL – brown soil sowed with wheat seeds dressed with hexaconazole and LNT; BL – brown soil sowed with wheat seeds dressed with LNT; BO – brown original soil; BW – brown soil with water only.
Effects of different seed dressing treatments on the abundance of fungal taxa in soil
Figure 5 shows the similarity and species relative abundance of soil samples for different treatments. Figure 5 shows that the species compositions of BW and BCK and of BO and BHL were similar. Among the 20 most abundant species in the soil, the dominant species among the treatments was Ascomycota. Compared with the control (BCK, BO and BW), hexaconazole-containing seed dressing (BH and BHL) decreased the relative abundances of Ascomycota, Alternaria, and Davidiella. BHL exhibited higher relative abundances of Mycosphaerella, while BL exhibited higher relative abundances of Waitea and Dothideomycetes and lower relative abundances of Alternaria and Gibberella.
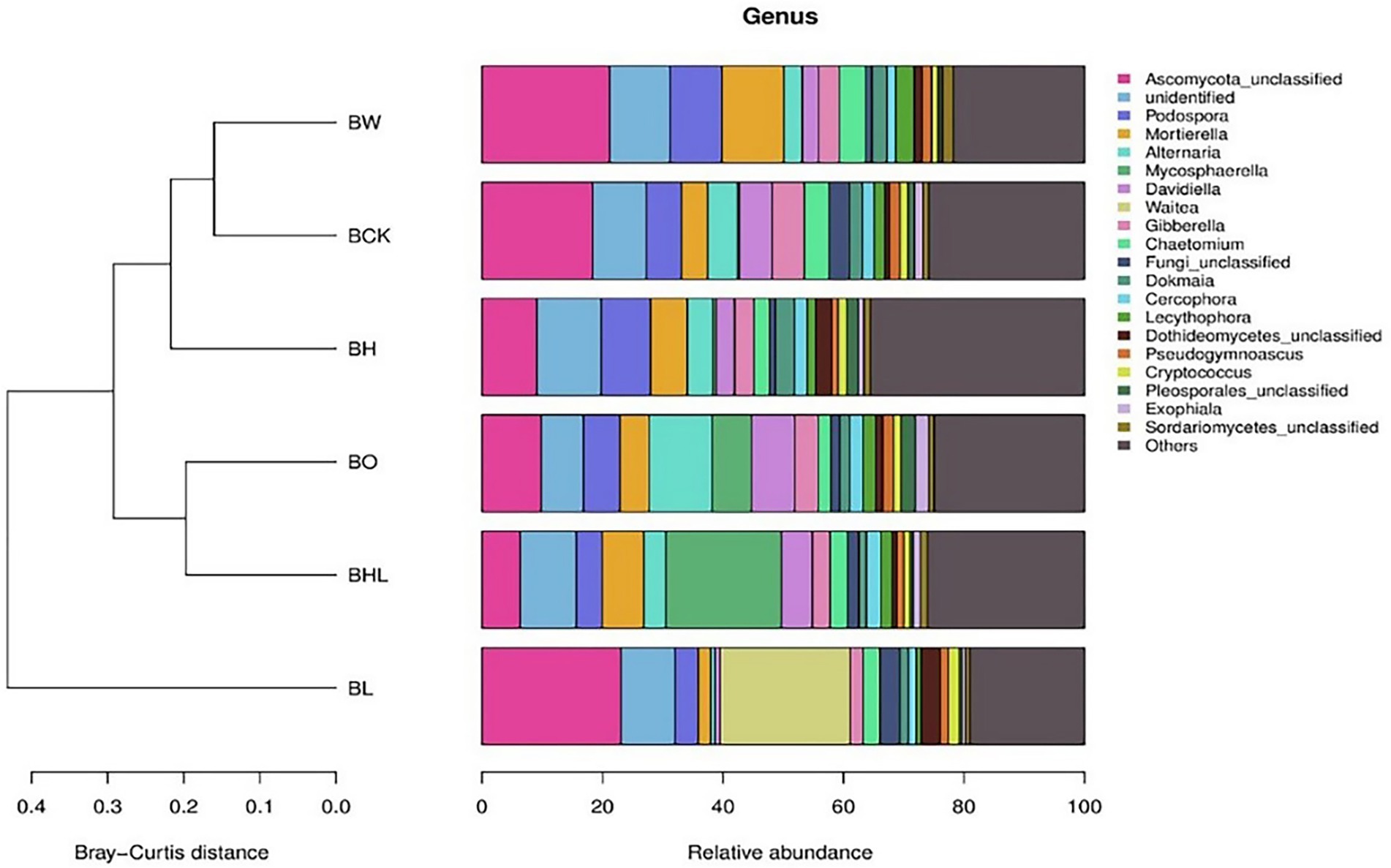
Figure 5. Relative abundance of fungal genera in brown soil in the different treatments. The legend shows the names of the 20 most abundant fungal genera, other genera are classified as “Other.” BCK – brown soil sowed with wheat seeds dressed with water only; BH – brown soil sowed with wheat seeds dressed with hexaconazole; BHL – brown soil sowed with wheat seeds dressed with hexaconazole and LNT; BL – brown soil sowed with wheat seeds dressed with LNT; BO – brown original soil; BW – brown soil with water only.
The main soilborne fungal genera of wheat were screened from the relative abundance of fungi at the genus level and made into Figure 6 according to their relative abundance. As shown in Figure 6, the relative abundances of Bipolaris, Rhizoctonia, Cladosporium, Fusarium, and Gibberella in BCK were 0.000%, 0.020%, 0.027%, 0.136%, 0.020%, and 5.333% respectively, close to those in BO and BW. Compared with BCK, BL showed the lowest relative abundances of the above soilborne pathogens, followed by BHL. BH exhibited higher relative abundances of Bipolaris and Rhizoctonia. Compared with BW, the relative abundance of Fusarium increased in BL, and the relative abundance of Rhizoctonia decreased. Both Fusarium and Rhizoctonia were significantly reduced in the BHL treatment compared with the BL and BW treatments.
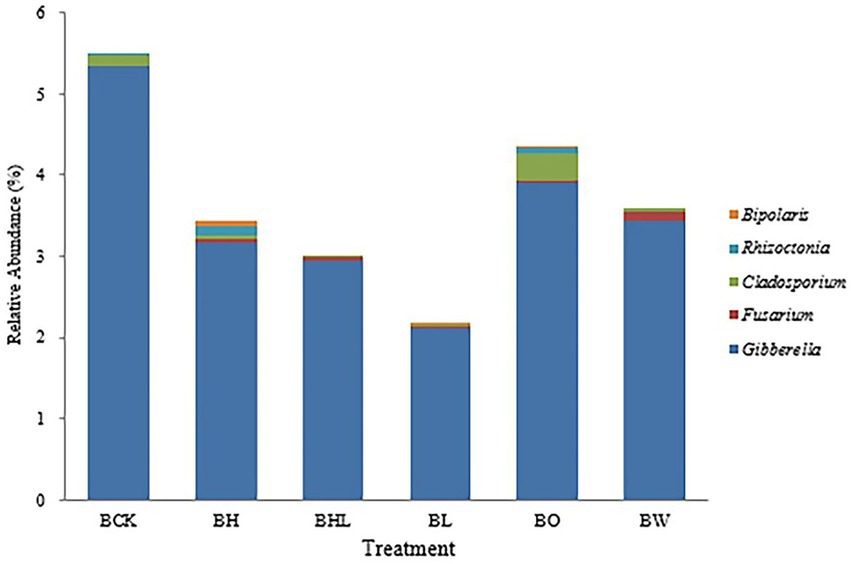
Figure 6. Relative abundances of the main soilborne fungi in the different treatments. Screening of relative abundances of major soilborne fungal genera in wheat at the fungal genus level. BCK – brown soil sowed with wheat seeds dressed with water only; BH – brown soil sowed with wheat seeds dressed with hexaconazole; BHL – brown soil sowed with wheat seeds dressed with hexaconazole and LNT; BL – brown soil sowed with wheat seeds dressed with LNT; BO – brown original soil; BW – brown soil with water only.
Effects of different wheat seed treatments on soil properties
The chemical properties of the soil by different treatments are shown in Table 5. The seen that the seed dressing treatments affected the pH, AK, AP, OM, and available N in the soil. LNT seed dressing treatment increased pH, AP, OM, and available N in soil compared with other seed dressing treatments.
RDA explained the correlation between soil fungal communities and soil properties across treatments (Figure 7). The first axis of RDA (RDA1) explained 8.81% of the variance, and the second axis (RDA2) explained 7.92% of the variance. The closer the distance between points is, the more similar the community structure between samples. Arrows represent environmental factors, and an angle between each factor is less than 90° is positive correlation; otherwise, it is a negative correlation. AK, AP, OM, and available N were positively correlated. The length of the line connecting the arrow and the origin represents the degree of correlation between an environmental factor and the community distribution. The longer the line is, the greater the effect of the factor. The fungal community was greatly affected by pH, AP, and OM in brown soil. Moreover, the fungal communities of soil sown with wheat seeds dressed with hexaconazole-containing agents (BH and BHL) were similar to those of soil sown with non-treated wheat seeds (BCK) and with water only (BW).
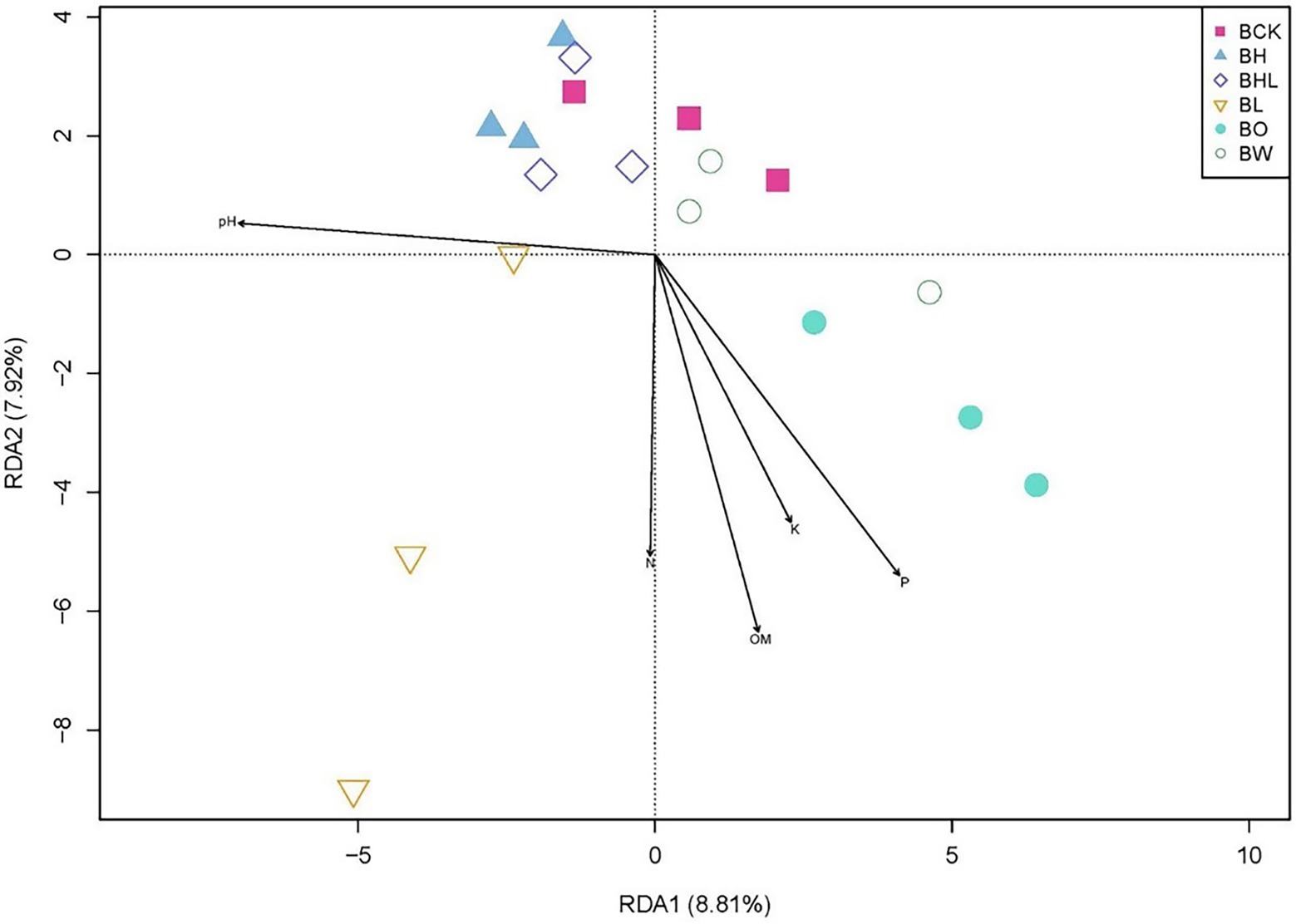
Figure 7. RDA analysis of soil fungal community based on seed dressing. Soil factors include P (available phosphorus), K (available potassium), N (available nitrogen), OM (organic matter), and pH. BCK – brown soil sowed with wheat seeds dressed with water only; BH – brown soil sowed with wheat seeds dressed with hexaconazole; BHL – brown soil sowed with wheat seeds dressed with hexaconazole and LNT; BL – brown soil sowed with wheat seeds dressed with LNT; BO – brown original soil; BW – brown soil with water only.
Discussion
Effects of hexaconazole and LNT seed dressing on the growth of wheat seedlings and their control effects on two wheat diseases
Biological inducer can induce resistance to pathogenic bacteria by activating the plant’s own immune response (Walters et al., 2013). It takes a certain amount of time for biological inducer to induce plant disease resistance. Chemical control has the advantages of good effect and quick effect. The combination of fungicides and biological inducer can increase plant disease control efficiency. Baider and Cohen (2003) reported that the mixed application of mancozeb and the plant activator β-aminobutyric acid at a ratio of 1:5 had a higher control effect on cucumber downy mildew and tomato late blight than the single treatment. In addition, conventional fungicides can reduce the pathogenicity of fungi, thus making it easier for plants to induce resistance against fungi and reducing the amount and frequency of chemical fungicide applications (Oostendorp et al., 2001). Zhang et al. (2021b) found that compared with Trichoderma asperellum SC012 and hymexazol alone, combined application can not only reduce the dosage of chemical fungicides, but also have a better control effect on cowpea fusarium wilt.
In a previous greenhouse experiment, the combined application of LNT at 4 a.i. g·100 kg−1 seed and hexaconazole at 0.5 a.i. g·100 kg−1 seed showed a control efficiency of 75.2% against wheat sharp eyespot, which was significantly higher than that achieved with a single application (Jiang et al., 2018). From the field test results of this study, the combination of small doses of hexaconazole and LNT has a good long-term control effect on wheat sharp eyespot and wheat crown rot. In the overwintering stage, the control effect of 4 a.i. g·100 kg−1 LNT and 0.5 a.i. g·100 kg−1 hexaconazole on Jimai 22 was above 70%, and it was still above 40% in the grain filling stage, which was significantly higher than that 1 a.i. g·100 kg−1 of hexaconazole. The combination of a lower dosage of hexaconazole and LNT could significantly increase the wheat thousand seed weight. Seeds dressed with a combination of hexaconazole at 0.5 a.i. g·100 kg−1 and LNT at 4 a.i. g·100 kg−1 showed thousand seed weights of 45.9 g, 45.6 g, 45.1 g and 45.1 g for Jimai 22, which were significantly higher than those of the LNT single treatment. This may be because the combination of hexaconazole and LNT provides a better control effect on wheat disease during the wheat grain-filling period, which is conducive to ensuring the transport of wheat nutrients.
Studies have shown that some polysaccharides can not only regulate plant immunity, but also promote plant growth, such as chitosan can induce resistance and promote plant growth (Pichyangkura and Chadchawan, 2015). From the data of the greenhouse test, 8 a.i. g·100 kg−1 LNT seed dressing had a promoting effect on the growth of Jimai 22. The seed plant height of 1 a.i. g·100 kg−1 hexaconazole seed dressing treatment was significantly lower than that of other treatments. Hexaconazole is a triazole fungicide and has a growth-regulating effect on plants. Fletcher and Hofstra (1985) pointed out that triazolone seed dressing treatment of seeds has a significant inhibitory effect on plant growth. Domestic studies have also shown that triazole fungicides can dwarf broad bean seedlings and have the effect of strengthening seedlings (Duan et al., 2009). This is generally consistent with the greenhouse results in this study. Hexaconazole treatment has a regulatory effect on the growth of wheat. The combination of hexaconazole and LNT reduced the application dosage of hexaconazole and alleviated the inhibitory effect of high concentrations of triazole fungicides on wheat seedling growth. This is of great significance for safe wheat production.
Effect of hexaconazole and LNT seed dressing on soil enzyme activities
Soil enzymes participate in the cycle of soil ecosystems, and their activities can be affected by many chemical and physical properties. Soil enzyme activity can directly reflect soil quality and fertility (Huang et al., 2020). Liu et al. (2020) reported that the opposite pattern of soil urease activities exhibited with heavy metal concentrations. Yu et al. (2019) reported that soil CAT activities were more sensitive to land use conversion than URE. In this study, wheat seed dressing exhibited similar effects on soil CAT and URE activities. The chemical fungicide hexaconazole showed some inhibitory effect, whereas the polysaccharide inducer LNT had little effect on soil enzyme activity. The soil enzyme activity of the hexaconazole and LNT (BHL) treatments was higher than that of the brown soil treated with hexaconazole. It may be that the combination of LNT and hexaconazole relieved the inhibitory effect of hexaconazole on soil enzyme activity, and the LNT treatment (BL) and hexaconazole (BH) treatment of wheat seeds had certain effects on wheat soil enzyme activities.
Effects of different seed dressing treatments on the abundance of fungal taxa in soil
Soil microorganisms have received increasing attention because of their potential for optimizing plant nutrient utilization and disturbing the pathogenesis of soilborne diseases (Chang et al., 2017). Chemical fungicides applied for disease control interrupt the soil microbial activity and community structure (Fang et al., 2016). Zhang et al. (2017b) reported that repeated application of iprodione could significantly increase the relative abundance of Proteobacteria and decrease those of Chloroflexi and Acidobacteria in soil. In this study, seed dressing with LNT, hexaconazole, and their combination all influenced the fungal community diversity of wheat rhizosphere soil. Hexaconazole-containing seed dressing (BH and BHL) decreased the relative abundances of Ascomycota, Alternaria, and Davidiella, and BL exhibited lower relative abundances of Alternaria and Gibberella.
Rhizoctonia, Cladosporium, Fusarium, Bipolaris, and Gibberella are the main seed- and soilborne pathogens of wheat and can cause sharp eyespot, blight, wilt, rot, and scab diseases (Ridout and Newcombe, 2016; De Mol et al., 2018). The existence of these pathogenic bacteria creates conditions for the occurrence of wheat diseases. Cai et al. (2021) reported that fertilizer application increased the abundance of beneficial fungi in cassava rhizosphere soil and decreased the abundance of pathogenic bacteria, thereby promoting cassava growth and yield. In this study, high-throughput sequencing analysis revealed a significant inhibitory effect of the combination of LNT and hexaconazole on the relative abundances of Rhizoctonia, Cladosporium, Fusarium, Bipolaris, and Gibberella, changes the structure of soil fungal community, and reduces the relative abundance of pathogenic fungi. It is helpful for scientific prevention and control of wheat soilborne diseases.
Effects of different wheat seed treatments on soil community diversity and soil properties
Microbial diversity indices are important indicators of the ecological function of soil (Wang et al., 2009). In this study, BH showed the lowest observed species and Chao1, consistent with its greater effect on soil fungal number and diversity. Hexaconazole is a triazole fungicide and is persistent in soil, but the mobility of hexaconazole is not strong. In the study by Singh (2005), hexaconazole was only moderately mobile in sandy loam soils with low organic matter content. Due to the low mobility of hexaconazole, the range of soil communities affected is also limited. Compared with other treatments, the observed species and Chao1 index of the LNT and hexaconazole combined seed dressing treatment (BHL) were lower, which had a greater impact on the number and diversity of fungi in the soil.
Soil characteristics, such as pH and nutrient content, can greatly affect the soil microbial community structure (Stefanowicz et al., 2020). Murphy et al. (2011) reported that the amount of soil organic matter is strongly linked to the size and structure of the soil microbial community. Similar to beta diversity, RDA can be used to reflect the diversity relationships among different samples. In this study, RDA showed that the soil fungal community was greatly affected by pH, available P, and organic matter.
Conclusion
Seed treatment with LNT combined with a low dosage of hexaconazole could significantly improve the control efficiency of wheat sharp eyespot and wheat crown rot, prolong the control period, reduce the use of hexaconazole, improve the utilization rate of pesticides, and increase wheat thousand seed weight. Low-dose LNT combined with hexaconazole had little effect on the activities of soil CAT and URE. The combined seed dressing treatment (BHL) of LNT and hexaconazole had a greater effect on the number and diversity of fungi in the soil. Different seed dressing treatments changed the structure of the wheat rhizosphere soil fungal community, and the combination treatment could significantly decrease the relative abundances of the soilborne pathogens Rhizoctonia, Cladosporium, Fusarium, Bipolaris, and Gibberella, which reduced the incidence of soilborne diseases. In addition, the fungal community structure in wheat brown soil was related to pH, available phosphorus, and organic matter. The occurrence of soilborne diseases of crops is closely related to the physical and chemical properties of the soil. The combined seed dressing of hexaconazole and LNT can reduce the relative abundance of pathogenic fungi in soil and change the community structure of wheat rhizosphere soil fungi, which is beneficial to the prevention and control of soilborne diseases such as wheat sharp eyespot and wheat crown rot, so as to promote the yield increase of wheat.
Data availability statement
The datasets generated for this study could be found in NCBI, BioProject: PRJNA862277.
Author contributions
XY performed the experiments, analyzed data, and wrote the manuscript. ZZ and YY collected pathogen isolates and conducted the experiments. KW revised the manuscript. YC analyzed data and revised the manuscript. HW designed the experiments, supervised the project, and wrote the manuscript. All authors have read and agreed to the published version of the manuscript.
Funding
This work was supported by the National Natural Science Foundation of China (32102259), the Special Fund for Agro-Scientific Research in the Public Interest of China (no. 201503130), and the Grants from Taishan Scholar Foundation of Shandong Province (tsqn202103162).
Conflict of interest
The authors declare that the research was conducted in the absence of any commercial or financial relationships that could be construed as a potential conflict of interest.
Publisher’s note
All claims expressed in this article are solely those of the authors and do not necessarily represent those of their affiliated organizations, or those of the publisher, the editors and the reviewers. Any product that may be evaluated in this article, or claim that may be made by its manufacturer, is not guaranteed or endorsed by the publisher.
References
Adrees, M., Khan, Z. S., Ali, S., Hafeez, M., Khalid, S., Rehman, M. Z., et al. (2020). Simultaneous mitigation of cadmium and drought stress in wheat by soil application of iron nanoparticles. Chemosphere 238:124681. doi: 10.1016/j.chemosphere.2019.124681
Almasudy, A. M., You, M. P., and Barbetti, M. J. (2015). Influence of fungicidal seed treatments and soil type on severity of root disease caused by Rhizoctonia solani AG-8 on wheat. Crop. Prot. 75, 40–45. doi: 10.1016/j.cropro.2015.05.007
Baider, A., and Cohen, Y. (2003). Synergistic interaction between BABA and mancozeb in controlling Phytophthora infestans in potato and tomato and Pseudoperonospora cubensis in cucumber. Phytoparasitica 31, 399–409. doi: 10.1007/bf02979812
Blaxter, M., Mann, J., Chapman, T., Thomas, F., and Abebe, E. (2005). Defining operational taxonomic units using DNA barcode data. Philos. Trans. R. Soc. Lond. B. Biol. Sci. 360, 1935–1943. doi: 10.1098/rstb.2005.1725
Cai, J., Zhang, J., Ding, Y., Yu, S., Lin, H., Yuan, Z., et al. (2021). Different fertilizers applied alter fungal community structure in rhizospheric soil of cassava (Manihot esculenta Crantz) and increase crop yield. Front. Microbiol. 12:663781. doi: 10.3389/fmicb.2021.663781
Chang, H. X., Haudenshield, J. S., Bowen, C. R., and Hartman, G. L. (2017). Metagenome-wide association study and machine learning prediction of bulk soil microbiome and crop productivity. Front. Microbiol. 8:519. doi: 10.3389/fmicb.2017.00519
De Mol, F., Winter, M., and Gerowitt, B. (2018). Weather determines the occurrence of wheat stem base diseases in biogas cropping systems. Crop Prot. 114, 1–11. doi: 10.1016/j.cropro.2018.07.017
Deng, Y. Y., Li, W., Zhang, P., Sun, H. Y., Zhang, X. X., Zhang, A. X., et al. (2020). Fusarium pseudograminearum as an emerging pathogen of crown rot of wheat in eastern China. Plant Pathol. 69, 240–248. doi: 10.1111/ppa.13122
Duan, H. G., Zhao, J. M., Xiang, L. H., Tian, C. H., and Yang, H. B. (2009). Effect of triadimefon on the growth of vicia faba seedlings. J. Anhui Agric. Sci. 37, 4945–4946. doi: 10.13989/j.cnki.0517-6611.2009.11.092
Erginbas-Orakci, G., Poole, G., Nicol, J. M., Paulitz, T., Dababat, A. A., and Campbell, K. (2016). Assessment of inoculation methods to identify resistance to fusarium crown rot in wheat. J. Plant Dis. Protect. 123, 19–27. doi: 10.1007/s41348-016-0001-8
Fang, H., Han, L. X., Cui, Y. L., Xue, Y. F., Cai, L., and Yu, Y. L. (2016). Changes in soil microbial community structure and function associated with degradation and resistance of carbendazim and chlortetracycline during repeated treatments. Sci. Total Environ. 572, 1203–1212. doi: 10.1016/j.scitotenv.2016.08.038
Fletcher, R. A., and Hofstra, G. (1985). Triadimefon a plant multi-protectant. Plant Cell Physiol. 39, 255–265. doi: 10.1016/0303-7207(85)90069-3
Hamada, M. S., Yin, Y., Chen, H., and Ma, Z. (2011). The escalating threat of Rhizoctonia cerealis, the causal agent of sharp eyespot in wheat. Pest Manag. Sci. 67, 1411–1419. doi: 10.1002/ps.2236
Huang, G. Y., You, J. W., Zhou, X. P., Ren, C., and Islam, M. S. (2020). Effects of low molecular weight organic acids on cu accumulation by castor bean and soil enzyme activities. Ecotox. Environ. Safe. 203:110983. doi: 10.1016/j.ecoenv.2020.110983
Jia, Z., Jiang, L., Hong, L., Jing, H., Zhe, C., Yuanjun, N., et al. (2018). Manipulation of the rhizosphere microbial community through application of a new bio-organic fertilizer improves watermelon quality and health. PLoS One 13:e0192967. doi: 10.1371/journal.pone.0192967
Jiang, L. L., Zhang, Z. X., Wang, H. Y., Qiao, K., Wang, K. Y., and Wang, D. (2018). Screening of efficient fungicides against sharp eyespot and root rot of wheat and control effect of lentinan combined with fungicides on wheat diseases. J. Triticeae Crops. 38, 113–118. doi: 10.7606/j.issn.1009-1041.2018.01.16
Karlsson, I., Friberg, H., Steinberg, C., and Persson, P. (2014). Fungicide effects on fungal community composition in the wheat phyllosphere. PLoS One 9:e111786. doi: 10.1371/journal.pone.0111786
Kazan, K., and Gardiner, D. M. (2018). Fusarium crown rot caused by Fusarium pseudograminearum in cereal crops: recent progress and future prospects. Mol. Plant Pathol. 19, 1547–1562. doi: 10.1111/mpp.12639
Lemańczyk, G., and Kwaśna, H. (2013). Effects of sharp eyespot (Rhizoctonia cerealis) on yield and grain quality of winter wheat. Eur. J. Plant Pathol. 135, 187–200. doi: 10.1007/s10658-012-0077
Li, S. B., Shi, Y. X., Liu, J. L., Xie, X. W., and Li, B. J. (2013). Preliminary effects of 4 resistance inducers on tomato resistance to late blight. Chin. Veg. 12, 49–54.
Liu, K. H., Li, C. M., Tang, S. Q., Shang, G. D., and Yu, F. M. (2020). Heavy metal concentration, potential ecological risk assessment and enzyme activity in soils affected by a lead-zinc tailing spill in Guangxi, China. Chemosphere 251:126415. doi: 10.1016/j.chemosphere.2020.126415
Marín, A., Cháfer, M., Atarés, L., Chiralt, A., Torres, R., Usall, J., et al. (2016). Effect of different coating-forming agents on the efficacy of the biocontrol agent candida sake CPA-1 for control of botrytis cinerea on grapes. Biol. Control 96, 108–119. doi: 10.1016/j.biocontrol.2016.02.012
Moya-Elizondo, E. A., and Jacobsen, B. J. (2016). Integrated management of fusarium crown rot of wheat using fungicide seed treatment, cultivar resistance, and induction of systemic acquired resistance (Sar). Biol. Control 92, 153–163. doi: 10.1016/j.biocontrol.2015.10.006
Murphy, D. V., Cookson, W. R., Braimbridge, M., Marschner, P., Jones, D. L., Stockdale, E. A., et al. (2011). Relationships between soil organic matter and the soil microbial biomass (size, functional diversity, and community structure) in crop and pasture systems in a semi-arid environment. Soil Res. 49, 582–594. doi: 10.1071/sr11203
Oostendorp, M., Kunz, W., Dietrich, B., and Staub, T. (2001). Induced disease resistance in plants by chemicals. Eur. J. Plant Pathol. 107, 19–28. doi: 10.1023/a:1008760518772
Peng, D., Li, S., Chen, C., and Zhou, M. (2014). Combined application of Bacillus subtilis NJ-18 with fungicides for control of sharp eyespot of wheat. Biol. Control 70, 28–34. doi: 10.1016/j.biocontrol.2013.11.013
Pichyangkura, R., and Chadchawan, S. (2015). Biostimulant activity of chitosan in horticulture. Sci. Hortic. 196, 49–65. doi: 10.1016/j.scienta.2015.09.031
Qi, Y. Z., Li, H. Y., Su, Y., and Zhen, W. C. (2014). Sensitivity to trifluzamide and main biological characteristics of resistant mutants of Rhizoctonia cerealis. Chin. J. Pestic. Sci. 16, 271–280. doi: 10.3969/j.issn.1008-7303.2014.03.05
Ren, X. X., Chen, C., Ye, Z. H., Su, X. Y., Xiao, J. J., Liao, M., et al. (2019). Development and application of seed coating agent for the control of major soil-borne diseases infecting wheat. Agronomy 9:413. doi: 10.3390/agronomy9080413
Ridout, M., and Newcombe, G. (2016). Disease suppression in winter wheat from novel symbiosis with forest fungi. Fungal Ecol. 20, 40–48. doi: 10.1016/j.funeco.2015.10.005
Singh, N. (2005). Mobility of four triazole fungicides in two Indian soils. Pest Manag. Sci. 61, 191–196. doi: 10.1002/ps.973
Stefanowicz, A. M., Kapusta, P., Zubek, S., Stanek, M., and Woch, M. W. (2020). Soil organic matter prevails over heavy metal pollution and vegetation as a factor shaping soil microbial communities at historical Zn-Pb mining sites. Chemosphere 240:124922. doi: 10.1016/j.chemosphere.2019.124922
Walters, D. R., Ratsep, J., and Havis, N. D. (2013). Controlling crop diseases using induced resistance: challenges for the future. J. Exp. Bot. 64, 1263–1280. doi: 10.1093/jxb/ert026
Wang, Y. S., Huang, Y. J., Chen, W. C., and Yen, J. H. (2009). Effect of carbendazim and pencycuron on soil bacterial community. J. Hazard. Mater. 172, 84–91. doi: 10.1016/j.jhazmat.2009.06.142
Wang, L. L., Xu, J. Q., Zhu, K., Sun, Y., Zheng, W., and Hou, Y. (2022). Inhibitory effect of kresoxim-methyl on Fusarum pseudograminearum and control effect on crown rot of wheat. Acta Phytopathol. Sin. 52, 434–442. doi: 10.13926/j.cnki.apps.000758
Winter, M., Mol, F. D., and Tiedemann, A. V. (2014). Cropping systems with maize and oilseed rape for energy production may reduce the risk of stem base diseases in wheat. Field. Crop. Res. 156, 249–257. doi: 10.1016/j.fcr.2013.10.009
Yu, P. J., Tang, X. G., Zhang, A. C., Fan, G. H., and Liu, S. W. (2019). Responses of soil specific enzyme activities to short-term land use conversions in a salt-affected region, northeastern China. Sci. Total Environ. 687, 939–945. doi: 10.1016/j.scitotenv.2019.06.171
Zhang, X. J., Wang, H. Y., Que, Y. W., Yu, D. Z., and Wang, H. (2021a). The influence of rhizosphere soil fungal diversity and complex community structure on wheat root rot disease. PeerJ 9:e12601. doi: 10.7717/peerj.12601
Zhang, Z. X., Wang, H. Y., Wang, K. Y., Jiang, L. L., and Wang, D. (2017a). Use of lentinan to control sharp eyespot of wheat, and the mechanism involved. J. Agric. Food Chem. 65, 10891–10898. doi: 10.1021/acs.jafc.7b04665
Zhang, C., Wang, W., Xue, M., Liu, Z., Zhang, Q., Hou, J., et al. (2021b). The combination of a biocontrol agent Trichoderma asperellum SC012 and hymexazol reduces the effective fungicide dose to control fusarium wilt in cowpea. J. Fungi 7:685. doi: 10.3390/jof7090685
Keywords: seed dressing, wheat sharp eyespot, wheat crown rot, control efficiency, fungal community
Citation: Yang X, Zhang Z, Yuan Y, Wang K, Chen Y and Wang H (2022) Control efficiency of hexaconazole-lentinan against wheat sharp eyespot and wheat crown rot and the associated effects on rhizosphere soil fungal community. Front. Microbiol. 13:1014969. doi: 10.3389/fmicb.2022.1014969
Edited by:
Bin Huang, Tobacco Research Institute (CAAS), ChinaReviewed by:
Feng Wei, Cotton Research Institute (CAAS), ChinaFenglong Wang, Tobacco Research Institute (CAAS), China
Copyright © 2022 Yang, Zhang, Yuan, Wang, Chen and Wang. This is an open-access article distributed under the terms of the Creative Commons Attribution License (CC BY). The use, distribution or reproduction in other forums is permitted, provided the original author(s) and the copyright owner(s) are credited and that the original publication in this journal is cited, in accordance with accepted academic practice. No use, distribution or reproduction is permitted which does not comply with these terms.
*Correspondence: Hongyan Wang, c2RhdXdoeUAxNjMuY29t; Yuan Chen, eXVhbi5jaGVuQHBrdS1pYWFzLmVkdS5jbg==