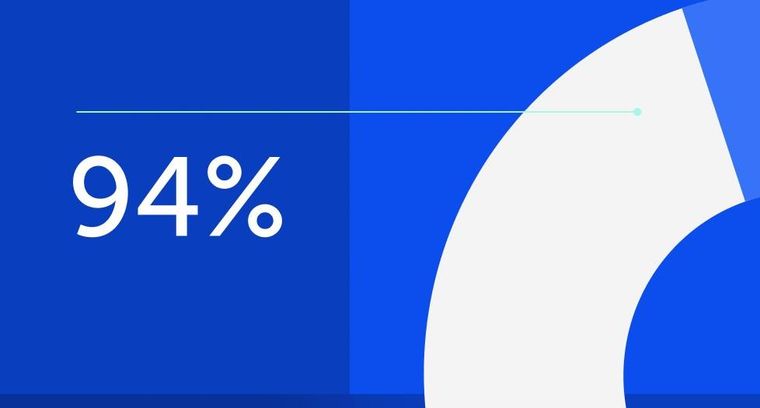
94% of researchers rate our articles as excellent or good
Learn more about the work of our research integrity team to safeguard the quality of each article we publish.
Find out more
ORIGINAL RESEARCH article
Front. Microbiol., 15 September 2022
Sec. Antimicrobials, Resistance and Chemotherapy
Volume 13 - 2022 | https://doi.org/10.3389/fmicb.2022.1010148
This article is part of the Research TopicCommunity Series in Antimicrobial Peptides: Molecular Design, Structure Function Relationship and Biosynthesis OptimizationView all 22 articles
Bovine mastitis caused by Streptococcus dysgalactiae (S. dysgalactiae) is usually treated with antibiotics, which may potentially increase drug resistance as the abuse. NZ2114, a variant of fungal defensin plectasin, displayed a potent antibacterial activity against S. dysgalactiae. The inhibition/eradication effect of the antimicrobial peptide NZ2114 on the early/mature biofilm of S. dysgalactiae CVCC 3938 was evaluated, as well as the elimination of bacteria in mature biofilms. In this study, NZ2114 displayed potent antibacterial activity against S. dysgalactiae CVCC 3938 and three clinical isolated S. dysgalactiae strains (0.11-0.45 μM). The early biofilm inhibition of S. dysgalactiae CVCC 3938 was 55.5–85.9% after treatment with NZ2114 at concentrations of 1–16 × MIC, which was better than that of vancomycin at the same concentration. The mature biofilm eradication rate was up to 92.7–97.6% with the increasing concentration (2–16 × MIC) of NZ2114, and the eradication rate did not change significantly with further increase of NZ2114 concentration, while the biofilm eradication rate of vancomycin-treated group at the same concentration remained at 92.5%. NZ2114 reduced the number of persister bacteria in biofilm. Scanning electron microscopy (SEM) and confocal laser scanning microscopy (CLSM) further demonstrated that NZ2114 could effectively reduce the biofilm thickness and bacterial number of S. dysgalactiae CVCC 3938. In vivo therapeutic effect of NZ2114 on murine mastitis model showed that NZ2114 was better than vancomycin in alleviating mammary gland inflammation by regulating cytokines production, inhibiting bacterial proliferation, and reducing the number of mammary gland bacteria. These data suggested that NZ2114 is a potential peptide candidate for the treatment of mastitis.
Bovine mastitis is an inflammatory disease caused by variety of pathogenic microorganisms with invasion of mammary gland tissue and long-term infection. The disease reduces milk production, augments breast necrosis rate, and even leads to death of dairy cows, which seriously threatens the health of dairy cows and affects the development of the dairy industry (Melchior et al., 2006). Streptococcus dysgalactiae (S. dysgalactiae) is one of the major Streptococcus species that cause mastitis (Ma et al., 2021), it is considered to be environmental pathogenic bacteria, which is parasitic in the mouth, vagina and skin of livestock, and also distributed in the environment. This pathogen can infect livestock, causing abscesses, endocarditis and mastitis. In China, the S. dysgalactiae is the top three strains among the main pathogenic bacteria leading to bovine mastitis, which can reach 21.35% in Jilin province (Hao et al., 2016). In addition, this bacterium also infects humans under special situations, causing bacteremia, endocarditis, meningitis, sepsis, arthritis, respiratory and skin infections and other diseases in patients (Rantala, 2014; Rantala and Tuohinen, 2014; Rutherford et al., 2014).
Biofilm is an aggregate in which microorganisms grow organically. Bacteria irreversibly adhere to the surface of inert or active entities, multiply, differentiate, and secrete some polysaccharide matrix, which wraps the bacterial community to form aggregate membrane. It had been suggested that the bacterial biofilms of Staphylococcus aureus (S. aureus), Escherichia coli and Streptococcus were highly resistant to antibiotics and host defense (Costerton et al., 1999; Olson et al., 2002; Parastan et al., 2020). Gomes et al., study showed that the formation of biofilm of S. dysgalactiae is an important virulence mechanism of the pathogenic causing bovine mastitis (Gomes et al., 2016). Previous studies showed that 46.7% of isolates displayed moderate or strong biofilm-forming abilities among 246 clinical S. dysgalactiae (Ma et al., 2017). Two biofilm formation related genes, eno and napr, were detected in 76% and 86% of S. dysgalactiae (n = 41), respectively (Kaczorek et al., 2017). The presence of biofilms in the mammary gland has led to a decrease in the effectiveness of antibiotic therapy, there are 10 to 1000 times more resistant to the effects of antibiotics, resulting in persistent infections of pathogenic bacteria, which increases the difficulty of treatment and prolongs the time of medication. In addition, some studies had reported that subinhibitory concentrations of some antibiotics could induce biofilm formation (Costa et al., 2012). Therefore, the removal of S. dysgalactiae biofilm has an important clinical significance.
Antimicrobial peptides (AMPs) are a class of small molecule peptides with high-efficiency antibacterial activity in innate immune system in living organism. The multifactorial antimicrobial mechanisms of AMPs derived from intracellular compatibility, high sensitivity to early warning and low resistance from multiple targets. AMPs could cover the merits of antibiotics in disease treatment and vaccines in disease prevention, and avoid their shortcomings, such as high resistance and high variation in pathogens, and high residue in animals. AMPs, antibiotics, and vaccines could complement each other, and build an iron triangle of animal health care together to halt the development of drug resistance and reduce the residues in tissues (Teng et al., 2014; Zhang et al., 2014; Hao et al., 2017, 2022; Li Z. et al., 2017; Yang et al., 2017, 2019; Wang et al., 2018; Liu et al., 2021; Zheng et al., 2021). Therefore, it has been highly expected that AMPs could exert a positive contribution to support green husbandry in terms of a new health concept One World and One Health. However, most AMPs stop at the assessment into clinical application due to their poor druggability, only a few AMPs show a promising future in drug development. It was reported that NZ2114 is the derived peptide of the first fungal defensin plectasin which targets specially Lipid II in cell wall of gram-positive bacteria, with three amino acid sites mutated (D9N, M13L, and Q14R). NZ2114 exhibited potent antimicrobial activity, with the minimum inhibitory concentration (MIC) values of 0.057–0.454 μmol/L for methicillin-resistant S. aureus (Chen et al., 2017). When NZ2114 was combined with hybrid catheter material, it displayed significant inhibition effect of methicillin-resistant S. aureus biofilms (Klein et al., 2017). NZ2114 also exhibited effective activity against Streptococcus (Pillar et al., 2008). In our previous study, NZ2114 had potent antimicrobial activity against Streptococcus suis (S. suis) with MIC values of 0.03–0.06 μmol/L. The in vitro time-kinetic results showed that NZ2114 was 99.9% bactericidal against S. suis CVCC 606 within 4 h at 4 × MIC concentration. In addition, the antimicrobial peptide NZ2114 had a good protective effect on mouse peritonitis infected by S. suis. The dose of 0.2 mg/kg NZ2114 treatment could result in a 100% survival rate of mouse, which effectively reduced the bacterial load in mice organs, regulated the release of inflammatory factors, alleviated the histopathological damage of organs and thus effectively protected mice against S. suis type 2 strains infection (Jiao et al., 2017). Therefore, NZ2114 is a potential antimicrobial agent to inhibit and eradicate S. dysgalactiae and its biofilms.
Based on the previous studies of plectasin-derived peptides in treatment of Streptococcus infection, this study is focus on exploring the inhibition/eradication effect of NZ2114 on S. dysgalactiae biofilm in vitro and the therapeutic effect of mouse mastitis in vivo.
Streptococcus dysgalactiae CVCC 3938 was purchased from the China Veterinary Culture Collection Center (CVCC) (Beijing, China). The tested strains of S. dysgalactiae CAU-FRI 1-3 isolated from bovine mastitis were obtained from China Agricultural University. The four-day post-parturient SPF ICR female mice were purchased from Vital River Laboratories (Beijing, China), kept in a sterile environment and supplied with sterile water and feed. The antimicrobial peptide NZ2114 was prepared by recombinant expression of Pichia pastoris X-33 with purity > 95% in Feed Research Institute, Chinese Academy of Agricultural Sciences (Zhang et al., 2014), the physicochemical properties of NZ2114 were shown in Table 1. All other chemical reagents used were of analytical grade.
The MIC values of NZ2114 were measured by the microbroth dilution method (Wiegand et al., 2008). In brief, mid-log phase S. dysgalactiae CVCC 3938 cells were diluted to a suspension of 1 × 105 CFU/mL with Tryptic Soy Broth medium (TSB, Qingdao Hope BioTechnology Co., Ltd.). NZ2114 was twofold diluted to final concentrations of 0.625–1280 μg/mL. The different concentrations of peptide (10 μL) and cell suspensions (90 μL) were added into a 96-well plate and incubated at 37°C for 16–18 h. The MIC value was defined as the lowest concentration at which there was no visible bacterial growth. All assays were performed in triplicate.
S. dysgalactiae CVCC 3938 is a strong biofilm-producing strain according to the previous study (Zhang et al., 2021). The growth of biofilms was measured with a 3-(4,5-dimethylthiazole-2-yl)-2,5-diphenyl tetrazolium bromide (MTT) (Sigma) (Pires et al., 2011). In brief, mid-log phase S. dysgalactiae CVCC 3938 cells were diluted to a suspension of 1 × 108 CFU/mL with TSB medium, 200 μL suspension and 100 μL MTT solution (0.5 mg/mL) were added into 96-well plates and incubated at 37°C for 72 h in the dark for further biofilm metabolism test. After incubation, MTT was displaced by the 150 μL DMSO dissolving solution and the optical density (OD) was determined at 570 nm using a microtiter plate reader (Cui et al., 2016).
The PBS suspension of mid-log phase S. dysgalactiae CVCC 3938 (1 × 108 CFU/mL) was mixed with different final concentrations of NZ2114 or vancomycin (1–16 × MIC) and then incubated in 96-well plates at 37°C for 24 h. Untreated bacterial solution was the control group, and TSB was the blank control group. After incubation, the cells were washed with PBS for 3 times to remove floating cells and the effect of NZ2114 on early biofilm formation of S. dysgalactiae CVCC 3938 was assessed by the crystal violet staining. The inhibitory effect of NZ2114 on biofilms was determined according to the previous study (Yang et al., 2019).
Mid-log phase S. dysgalactiae CVCC 3938 was diluted to a suspension of 1 × 108 CFU/mL with TSB medium and 200 μL bacterial suspension was incubated at 37°C for 24 h in 96-well plates. The supernatant was gently removed and 200 μL of NZ2114 or vancomycin at final concentrations of 1–16 × MIC were added and continued to incubate for 24 h. Untreated bacterial solution was the control group, and TSB was the blank control group. After incubation, the plates were washed with PBS for 3 times to remove the planktonic cells. The effect of NZ2114 on the eradication of mature biofilms of S. dysgalactiae CVCC 3938 was evaluated by crystal violet staining and the absorbance was measured as described above. All assays were performed in triplicate (Yang et al., 2019).
A volume of 200 μL mid-log phage S. dysgalactiae CVCC 3938 suspension (1 × 108 CFU/mL in TSB medium) was added into 96-well plates and incubated at 37°C for 24 h. The plates were washed gently to remove the planktonic bacteria and incubated with NZ2114 or vancomycin at 37°C for 2 h. The untreated bacteria and free medium were used as control group and blank control group. After ultrasound, the attached bacteria were removed and colony count (Yang et al., 2019).
In order to obtain persister bacteria from mature biofilms, a volume of 200 μL S. dysgalactiae CVCC 3938 bacterial suspension (1 × 108 CFU/mL) was added into 96-well plates and incubated at 37°C for 24 h. After removed the planktonic bacteria, the plates were incubated with 100 × MIC vancomycin at 37°C for 24 h. After washed with PBS, the plates were treated with 16 × MIC NZ2114 or vancomycin at 37°C for 24 h for combatting the bacteria encapsulated in biofilm and then cultured in fresh PBS were used as a control. After ultrasound, the attached bacteria were removed and counted in colony forming units (Yang et al., 2019).
According to the previous study, the biofilm of S. dysgalactiae CVCC 3938 was analyzed by SEM with some modifications. A volume of 360 μL S. dysgalactiae CVCC 3938 bacterial suspension (1 × 108 CFU/mL) and 40 μL 160 × MIC NZ2114 or antibiotics were added into 24-well plates with sterile guide sheets and co-incubated at 37°C for 24 h. After incubation, the plates were washed 3 times with PBS to remove planktonic bacteria and the biofilm was fixed with 2.5% glutaraldehyde at 4°C for 24 h. The cells of biofilms were dehydrated with ethanol series (50, 70, 85, 95, and 100%) for three times with 15 min/time, dried by CO2, sputtered with platinum, and observed using a QUANTA200 SEM (FEI, Philips, Netherlands) (Zhang et al., 2021).
To further study the inhibition and elimination effect of NZ2114 on biofilm and internal bacteria, biofilms were observed by CLSM. The sample pretreatment was consistent with SEM. After removed the planktonic bacteria, the biofilms were dyed with PI and SYTO9 (LIVE/DEAD BacLight Bacterial Viability Kit, ThermoFisher) for 15 min. Finally, the slides were washed with PBS and observed by Zeiss LSM880 confocal microscope (CLSM). The excitation/emission for these dyes are 480/500 nm for SYTO 9 stain and 490/635 nm for propidium iodide (Zhang et al., 2021).
In order to study the therapeutic effect of NZ2114 against S. dysgalactiae CVCC 3938 on animals, a murine mastitis model was constructed. Specific pathogen free (SPF) ICR female mice at 4-day postpartum were selected. During the experiment, the mice were placed in an animal room with a temperature-controlled light-dark cycle (light: 8:00–20:00), supplied feed and water freely. The murine mastitis model was established as previous study with minor modifications (Li L. B. et al., 2017).
Briefly, the pups were separated from their lactating female mice 2 h before the experiment, and lactation was resumed 1 h before bacteria injection, so that the milk could be sucked up as much as possible. The mice were anesthetized with isoflurane through respiratory inhalation and placed them under the dissecting microscope, adjusting the focus so that the mouse nipples are clearly visible under the microscope. After disinfection with 75% alcohol, the nipple was gently fixed with sterile ophthalmic forceps in the left hand, and 100 μL bacterial suspension (1 × 105 CFU/mL S. dysgalactiae CVCC 3938) or PBS was injected into the 4th pair of mammary glands through the milk ducts with a 100 μL microsyringe in the right hand.
In this study, a total of 16 lactating ICR mice were randomly divided into 4 groups: (1) Blank control group (Uninfected group); (2) Negative control group (Uninfected group); (3) NZ2114 treatment group; (4) Vancomycin treatment group. After challenge with S. aureus for 3 h, the dose of 100 μg/mammary gland NZ2114 and vancomycin was treated (dissolved in 100 μL PBS), and the blank control group was given the same volume of PBS. Mice were euthanized by cervical dislocation at 24 h, 3 day, 7 day after challenge, and the fourth pair of mammary glands were collected aseptically and weighed for bacterial counting.
ELISA kits were used to determine IL-1β, IL-6, IL-10, TNF-α and MCP-1 indexes, and the remaining mammary tissues of each group were treated with 10% paraformaldehyde and stained with hematoxylin and eosin (HE) for histopathological analysis. All mouse experiments and animal welfare were performed in accordance with the protocol approved by the Animal Care and Use Committee-Ethical Committee for Experimental Animals (AEC-CAAS-20090609) of the Feed Research Institute, Chinese Academy of Agricultural Sciences.
All data were analyzed by GraphPad Prism 8 (GraphPad Software, United States), and the results were given as means ± standard deviations (SDs). Statistical significance of groups was analyzed using the one-way ANOVA and Tukey multiple comparison.
As shown in Table 2, NZ2114 displayed a potent antibacterial activity against S. dysgalactiae. The MICs of NZ2114 against S. dysgalactiae CVCC3938 was 0.11 μM, which was significantly superior to vancomycin (0.67 μM). For the clinical isolates S. dysgalactiae CAU-FRI 2,3, NZ2114 displayed a stronger antibacterial capacity with 0.23 μM than vancomycin (0.67 and 0.34 μM). Only for S. dysgalactiae CAU-FRI 1, NZ2114 showed an inferior activity (0.45 μM) compared with vancomycin (0.34 μM).
The growth curve of S. dysgalactiae CVCC 3938 reflected the biofilm formation pattern. As shown in Figure 1, the absorbance value of S. dysgalactiae CVCC 3938 biofilm grew rapidly (OD570 0.08–1.21) and the biofilm metabolism was vigorous in the first 4 h. At 12–20 h, the absorbance value tended to be stable (OD570 0.36–0.40), and at 24–72 h, the absorbance values gradually decreased (OD570 0.08–0.25).
The inhibitory effect of NZ2114 on the initial stage of biofilm formation was shown in Figure 2A, NZ2114 inhibited the initial biofilm of S. dysgalactiae CVCC 3938 by 77.9%–85.9% at concentrations of 1–8 × MIC, which was better than that of vancomycin (53.2–62.6%) at the same concentration. The results indicated that NZ2114 could effectively inhibit the initial biofilm formation of S. dysgalactiae at a certain concentration range.
Figure 2. Effects of NZ2114 on S. dysgalactiae biofilms and bacteria in biofilms. (A) Inhibition effect of NZ2114 on biofilms formation. (B) Eradication effect of NZ2114 on mature biofilms. (C) Bactericidal activity of NZ2114 against the established biofilms. (D) Bactericidal activity of NZ2114 against persister.
As shown in Figure 2B, NZ2114 had a certain destructive effect on the mature biofilm, the biofilm eradication rates were 92.7–97.6% with increasing concentrations of NZ2114 at 2–16 × MIC, and the eradication rates did not change significantly when the concentration continued to increase, maintaining at about 96.7%, while the biofilm eradication rate of vancomycin-treated group was about 92.5% at the same concentration. Therefore, NZ2114 had the potential to eradicate mature biofilm.
As shown in Figure 2C, both NZ2114 and vancomycin reduced the number of bacteria encased in the biofilm. After treatment with 1–2 × MIC NZ2114, the colony number of S. dysgalactiae CVCC 3938 was rapidly decreased by 2.27–2.92 lg, but during 2–64 × MIC the number of colony remained stable, 128 × MIC NZ2114 completely killed the S. dysgalactiae CVCC 3938. The colony number of S. dysgalactiae CVCC 3938 was reduced by 2.04 lg after treated with 1 × MIC vancomycin, and until the concentration was 512 × MIC, vancomycin could completely eliminate the S. dysgalactiae CVCC 3938. Therefore, the killing efficiency of NZ2114 on bacteria in biofilm was significantly better than that of vancomycin.
Through external application of antibiotics, bacteria were artificially induced biofilm to produce persister bacteria. A high dose of vancomycin (100 × MIC) was first added to a 96-well plate in which a mature biofilm of S. dysgalactiae CVCC 3938 had been formed. As shown in the Figure 2D, there still existed 6.8 × 104 CFU/mL of persister bacteria encapsulated in the biofilm after treatment with 100 × MIC vancomycin for 24 h. After co-incubation with 16 × MIC NZ2114 for 24 h, the persister bacteria eradication rate of NZ2114 was 99.9%, significant difference with the untreated group (P < 0.001), while 16 × MIC vancomycin was less effective in inhibiting the persister bacteria. The number of viable bacteria in the biofilm with 16 × MIC vancomycin treatment decreased from 6.8 × 104 to 1.4 × 103 CFU/mL after 24 h, which was only one order of magnitude lower.
As shown in Figure 3A, the biofilm of the untreated control group was intact, and the whole biofilms were patchy and irregularly covered on the surface of the carrier, forming an irregular multi-layer structure. S. dysgalactiae CVCC 3938 cells were joined together by a sticky extracellular product secreted by the bacterium, which was encapsulated in the extracellular secretion. The vancomycin treatment group showed a decrease in the number of extracellular polymers and slight deformation of bacteria in the biofilm. After treatment with NZ2114, the biofilm became thin and loose, and the bacterial cells in the biofilm were deformed or ruptured.
Figure 3. Effects of NZ2114 on S. dysgalactiae biofilms by SEM and CLSM observation. (A) Biofilms observation by SEM. (B) Biofilms observation by CLSM. Live cells are stained in green by SYTO9 and dead cells are stained in red by PI. CK: the untreated S. dysgalactiae biofilms. Van: vancomycin.
In order to further confirm the inhibition and eradication effect of NZ2114 on biofilm and internal bacteria, the treated S. dysgalactiae CVCC 3938 were observed by CLSM. As shown in the Figure 3B, the untreatment group formed biofilm of S. dysgalactiae CVCC 3938 with a thickness of 18.82 μm and more than 90% were live cells (stained with green color) in the field of view, after treatment with NZ2114, bacterial biofilm became significantly thinner (a thickness of 10.48 μm) and the number of dead bacteria increased (stained with red color), which was better than that of the vancomycin-treated group (a thickness of 15.16 μm). These results indicated that NZ2114 was superior to vancomycin in the inhibition and elimination of S. dysgalactiae CVCC 3938 biofilm and its internal bacteria.
Compared with the blank control group, the female mice infected with S. dysgalactiae CVCC 3938 for 24 h showed signs of swelling, erythema and hemorrhage with mammary tissue (Figure 4A). The acinar morphology was changed and acinar wall was thickened. In addition, levels of TNF-α, IL-1β, IL-6 and MCP-1 in mammary tissue were significantly increased (Figure 4B). The murine model in mice was successfully established.
Figure 4. Establishment of murine mastitis model. (A) Pathological changes of mammary glands in mouse. (B) The change of inflammatory cytokine. A probability value of < 0.05 was considered significant. (*) Indicates the significance between uninfected and untreated groups. **p < 0.01; ****p < 0.0001. The results are given as the means ± SDs (n = 3).
As shown in Figure 5, after challenge with S. dysgalactiae CVCC 3938 for 24 h, the bacterial number of untreated group was approximately 3.1 × 107 CFU/gland. After treatment with NZ2114, the bacterial numbers in the mammary glands decreased significantly by 5.64 lg (from 7.33 to 1.87 lg, P < 0.01), 5.16 lg (from 5.16 to 0 lg, P < 0.01), and 1.69 lg (from 3.31 to 1.62 lg) at 1 d, 3 d and 7 d, respectively. For vancomycin treatment group, the bacterial load in the mammary glands decreased by 1.73 lg (from 7.33 to 5.96 lg), 2.16 lg (from 5.16 to 3 lg, P < 0.001), and 3.06 lg (from 3.31 to 0.25 lg, P < 0.01) at 1 d, 3 d and 7 d, respectively. Those results showed that the in vivo treatment effect of NZ2114 was better than that of vancomycin treatment group.
Figure 5. Effects of NZ2114 on bacterial loads in mammary tissue of S. dysgalactiae infected mice. (A) Treatment for 24 h; (B) treatment for 3 day; (C) treatment for 7 day. A probability value of < 0.05 was considered significant. (*) Indicates the significance between untreated and each of treatment groups. **p < 0.01; ***p < 0.001. The results are given as the means ± SDs (n = 3).
To investigate the regulation effect of NZ2114 on immune factor of mammary gland in mice, the levels of inflammatory factors (TNF-α, IL-6 and IL-1β), anti-inflammatory factors (IL-10) and chemokines (MCP-1) in the mammary gland of mice were measured. As shown in Figures 6A–E, the levels of IL-1β, IL-6, IL-10, TNF-α and MCP-1 were 1.86–1.99, 19.05–19.57, 1.79–2.17, 6.48–7.13 and 4.35–5.88 pg/mg, respectively, after treatment with NZ2114. The levels of IL-1β, IL-6, TNF-α and MCP-1 in NZ2114 treatment group were significantly lower than those in negative control group and the regulatory effect of NZ2114 was comparable to those of the vancomycin treatment group.
Figure 6. The protective effect of NZ2114 on the mammary gland of mice at the immune level. Mammary tissues were collected and the levels of (A) TNF-α, (B) IL-1β, (C) IL-6, (D) IL-10, and (E) MCP-1 were detected by using an ELISA kit after 24 h treatment, respectively. The analyses were measured by one-way ANOVA, with Duncan’s multiple comparisons test. All assays were performed in triplicate. The analyses were measured by one-way ANOVA, with Duncan’s multiple comparisons test. A probability value of <0.05 was considered significant. (*) Indicates the significance between control and each of treatment groups. **p < 0.01; ***p < 0.001; ****p < 0.0001. The results are given as the means ± SDs (n = 3).
As shown in Figures 7A–D, the inflammatory response of mammary tissue in NZ2114 and vancomycin treatment group were significantly improved, the infiltration of macrophages and neutrophils in the glandular follicles were significantly reduced, and the milk clot was reduced. Among them, NZ2114 treatment group was comparable to the normal level. The results indicated that NZ2114 had protective immune effects on mammary glands of mice.
Figure 7. Histological evaluation of breast tissues. (A) Uninfected group; (B) Infected and untreated group; (C) NZ2114 treated group; (D) Vancomycin treated group.
Bovine mastitis is easy to relapse and difficult to cure, and S. dysgalactiae is one of the main pathogen. In this study, plectasin derived peptide NZ2114 displayed potent activity against S. dysgalactiae (Table 2). Therefore, the anti-biofilm and in vivo effects of NZ2114 on S. dysgalactiae were studied.
NZ2114 was demonstrated to inhibit the formation of early biofilm and eradicate mature biofilm of S. dysgalactiae CVCC 3938, which was significantly superior to vancomycin (Figures 2A,B). SEM and CLSM further demonstrated that NZ2114 could effectively reduce the biofilm thickness and bacterial colony of S. dysgalactiae CVCC 3938 (Figure 3). In addition, after treatment with antibiotics or attack by immune system of host, the bacteria could form small colonies of persister bacteria within the biofilm and remain in the host for a long time. Lewis et al., proposed that persister bacteria may play a role in biofilm tolerance and demonstrated that the presence of persister bacteria does prevent effective treatment of bacterial infections (Levin and Rozen, 2006). In this study, NZ2114 showed a potent role in the eradication of persister in early biofilm and mature biofilm, which was significantly better than that of vancomycin (Figures 2C,D). At the early stage of biofilm development, the adhesion ability of bacteria to the surface of natural polymer was increased under the action of cell wall associated adhesins. Therefore, the bacteria elimination effect during early biofilm showed that NZ2114 can inhibit the biofilm formation at the source. While for the mature biofilm, the elimination of biofilm plays an important role, then the persister bacteria in the biofilm could be dissociated, and the planktonic bacteria are further killed. NZ2114 not only had a more potent antimicrobial activity than its parent peptide plectasin in vitro and in vivo (Mygind et al., 2005; Andes et al., 2009; Brinch et al., 2009), but also more efficient bactericidal and biofilm removal effect than the other fungal defensin peptide P2 against S. dysgalactiae (Zhang et al., 2021), which may be related to the increased charge of NZ2114 (NZ2114 with 3 positive charges, plectasin and P2 with 1 positive charge). Recent studies have highlighted the potential use of AMPs to prevent biofilm formation or eradicate established biofilms (Rajasekaran et al., 2017; De Breij et al., 2018; Mwangi et al., 2019). However, there are also related researches of AMPs and compounds that only destroyed biofilms but did not kill bacteria. For example, De La Fuente et al. screened and obtained a strong anti-biofilm cationic nine-peptide 1037, which can inhibit the formation of biofilm at the concentration of 1/30 × MIC (>50% reduction in cell biomass). It disables the ability of bacteria to swim and aggregate and inhibit the expression of genes related to biofilm formation after interacting with bacteria, but the killing ability is relatively weak (de la Fuente-Núñez et al., 2012). Lee et al. had confirmed that extracts of various medicinal plants exhibited anti-biofilm activity against S. aureus without inhibiting the growth of cells in planktonic culture (Lee et al., 2013).
During lactation, the bacteria can easily pass through the milk ducts to the mammary gland. When a cow’s mammary gland is infected or damaged, the osmotic pressure balance between the cow’s milk and blood is disrupted, causing various cells and proteins in the blood to enter the milk pool or vesicles, which leads to changes in milk composition, κ-casein and lactoglobulin levels were reduced, whey protein, pH, and somatic cell count (SCC) were increased, respectively. This phenomenon significantly affects the technological quality of cows’ milk (Carroll et al., 1963; Pecka-Kiełb et al., 2016). What’s more, a large collection of white blood cells will block the ducts of the breast, resulting in the inability to discharge milk, a decrease in milk production, and even the cessation of lactation and loss of breast function. Because of the complex environment in the udder of dairy cows, in vivo testing is an essential part of evaluating new drugs for the treatment of mastitis. Several researchers have reported that the 4th pair of mouse mammary gland have been used as an infection model to test the therapeutic effects of gut microbiota, probiotics and phage (Wang et al., 2017; Hu et al., 2020; Ngassam-Tchamba et al., 2020). Therefore, a model of mastitis induced by S. dysgalactiae in mice was established in this work, which showed the typical symptoms of mastitis at the 4th pair of mouse mammary tissue as previously reported (Xi et al., 2020), the swelling, congestion and hemorrhage mammary tissue and the increased inflammatory cytokines. Compared with the vancomycin treatment group, NZ2114 displayed significant decrease of bacteria and immunomodulatory effects against S. dysgalactiae-induced mastitis, and the effect of NZ2114 was superior to that of vancomycin. However, the bacterial loads in mammary tissue were increased after treatment with NZ2114 for 7 days compared to treatment for 3 days, the reason may be related to the only once treatment after the challenge with S. dysgalacetiae within 7 days. Previous studies had proved that NZ2114 has more rapid bactericidal properties in vitro (Zhang et al., 2014) and intracellular (Wang et al., 2019) than vancomycin. Therefore, the treatment of mastitis in vivo with NZ2114 achieved a rapid bactericidal effect in the short term (1 d: from 7.33 to 1.87 lg, 3 d: from 7.33 lg to 0), but the concentration of NZ2114 in the breast was lower than the MIC value due to the absence of secondary administration, and the surviving S. dysgalacetiae was able to proliferate again. This result laterally proves that the antimicrobial peptide NZ2114 is not easily residual in the body, and the dosing interval needs to grope according to the in vivo pharmacokinetics. Vancomycin has the minimal colony count on day 7 in vivo, indicating that vancomycin is slow to take effect and has a long residual time in the body, and a 2–7 days milk abandonment period is needed after antibiotic treatment. NZ2114 could kill the two main pathogens S. dysgalactiae and S. aureus of mastitis, which is expected to be the best candidate for the treatment of bovine mastitis. And, it is reported that the industrial scale of 20 and 30 m3 special for AMPs fermentation and relative purification platform system were established in 2019 and 2021 in China for the first time, respectively (Gao and Wang, 2022), and a total amount of 49 Kg AMP products with a purity higher than 92% were harvested, which would be great helpful to evaluation and clinical trials on AMPs during drug development in next works.
S. dysgalactiae can not only form biofilm but also invade and survive within mammary epithelial cells, and persist for long periods, which can avoid the bactericidal action of antibiotics, resulting in persistent infection (Calvinho and Oliver, 1998). Streptococcus also can induce autophagy to increase inflammatory reactions of bovine mammary epithelial cells (Chen et al., 2022; Qi et al., 2022). Because of the low intracellular accumulation and special intracellular environmental effects, it is difficult for the antibiotics to play a good bactericidal effect in intracellular. In this study, NZ2114 not only killed bacteria in vitro, but also completely eliminated S. dysgalactiae in mammary gland tissue, which may be related to the intracellular bactericidal efficacy of NZ2114. The previous studies have shown that NZ2114 was internalized in the bovine mammary epithelial cells via clathrin-mediated endocytosis and micropinocytosis, and maintained potent intracellular antibacterial activities (Wang et al., 2018). NZ2114 has dual effects of anti-biofilm and anti-intracellular bacteria, which may explain the main reason that NZ2114 could effectively cure murine mastitis.
In general, in vitro and in vivo antibacterial and antibiofilm effects of NZ2114 on S. dysgalactiae CVCC 3938 were systemically studied for the first time. NZ2114 revealed the inhibition/eradication effect on the early/mature biofilm of S. dysgalactiae and vancomycin-resistant persister encapsulated the biofilm. Furthermore, NZ2114 is an excellent drug for the treatment of S. dysgalactiae-induced mastitis. These results indicated NZ2114 is a potential effective drug for clinical treatment of S. dysgalactiae infection.
The original contributions presented in this study are included in the article/supplementary material, further inquiries can be directed to the corresponding authors.
All animal procedures used in this study were approved by the Animal Care and Use Committee of Feed Research Institute, Chinese Academy of Agricultural Sciences (Permit Number: 20150309) and were performed in accordance with ARRIVE (Animals in Research: Reporting in vivo Experiments) guidelines.
QZ, NY, RM, DT, HF, and JW conceived and designed experiments. NY and QZ carried out all the experiments. NY, QZ, DT, and JW contributed in writing. JW and HF contributed in funding acquisition. YH and XM contributed to the materials and reagents. RM contributed in modifying figures. All authors contributed to the article and approved the submitted version.
This study was funded by the National Natural Science Foundation of China (Grant No. 31872393), the Key Projects of Alternatives to Antibiotic for Animal (Grant No. CAAS-ZDRW202111), and Feed (Grant No. CAAS-ZDXT2018008). Usages of the Agricultural Science and Technology Innovation Program (ASTIP) and Tianjin Science and Technology Planning Project (18YFZCNC01130).
We acknowledge Chunli Li and Tong Zhao from the Core Facility at the Institute of Microbiology at the Chinese Academy of Sciences (CAS) for their technical support with SEM, TEM, and flow cytometer analysis and Dan Zhang from the Core Facility at the Center of Biomedical Analysis at Tsinghua University for her CLSM analysis.
The authors declare that the research was conducted in the absence of any commercial or financial relationships that could be construed as a potential conflict of interest.
All claims expressed in this article are solely those of the authors and do not necessarily represent those of their affiliated organizations, or those of the publisher, the editors and the reviewers. Any product that may be evaluated in this article, or claim that may be made by its manufacturer, is not guaranteed or endorsed by the publisher.
Andes, D., Craig, W., Nielsen, L. A., and Kristensen, H. H. (2009). In vivo pharmacodynamic characterization of a novel plectasin antibiotic, NZ2114, in a murine infection model. Antimicrob. Agents Chemother. 53, 3003–3009. doi: 10.1128/AAC.01584-08
Brinch, K. S., Sandberg, A., Baudoux, P., Françoise, B., and Kristensen, H. H. (2009). Plectasin shows intracellular activity against Staphylococcus aureus in human THP-1 monocytes and in a mouse peritonitis model. Antimicrob. Agents Chemother. 53, 4801–4808. doi: 10.1128/AAC.00685-09
Calvinho, L. F., and Oliver, S. P. (1998). Invasion and persistence of Streptococcus dysgalactiae within bovine mammary epithelial cells. J. Dairy Sci. 81, 678–686. doi: 10.3168/jds.S0022-0302(98)75623-1
Carroll, E. J., Schalm, O. W., and Lasmanis, J. (1963). Experimental coliform (Aerobacter aerogenes) mastitis: Distribution of whey proteins during the early acute phase1. J. Dairy Sci. 46, 1236–1242. doi: 10.3168/jds.S0022-0302(63)89251-6
Chen, H. X., Mao, R. Y., Teng, D., Wang, X. M., Hao, Y., Feng, X. J., et al. (2017). Design and pharmacodynamics of recombinant NZ2114 histidine mutants with improved activity against methicillin-resistant Staphylococcus aureus. AMB Express 7:46. doi: 10.1186/s13568-017-0345-x
Chen, P., Yang, J. Y., Wu, N. W., Han, B., Kastelic, J. P., and Gao, J. (2022). Streptococcus lutetiensis induces autophagy via oxidative stress in bovine mammary epithelial cells. Oxid. Med. Cell Longev. 7:2549772. doi: 10.1155/2022/2549772
Costa, J. C. M., Espeschit, I., Pieri, F. A., Benjamin, L. A., and Moreira, M. A. S. (2012). Increased production of biofilms by Escherichia coli in the presence of enrofloxacin. Vet. Microbiol. 160, 488–490. doi: 10.1016/j.vetmic.2012.05.036
Costerton, J. W., Stewart, P. S., and Greenberg, E. P. (1999). Bacterial biofilms: A common cause of persistent infections. Science 284, 1318–1322. doi: 10.1126/science.284.5418.1318
Cui, H., Li, W., Li, C., Vittayapadung, S., and Lin, L. (2016). Liposome containing cinnamon oil with antibacterial activity against methicillin-resistant Staphylococcus aureus biofilm. Biofouling 32, 215–225. doi: 10.1080/08927014.2015.1134516
De Breij, A., Riool, M., Cordfunke, R. A., Malanovic, N., De Boer, L., Koning, R. I., et al. (2018). The antimicrobial peptide SAAP-148 combats drug-resistant bacteria and biofilms. Sci. Transl. Med. 10:eaan4044. doi: 10.1126/scitranslmed.aan4044
de la Fuente-Núñez, C., Korolik, V., Bains, M., Nguyen, U., Breidenstein, E. B., Horsman, S., et al. (2012). Inhibition of bacterial biofilm formation and swarming motility by a small synthetic cationic peptide. Antimicrob. Agents Chemother. 56, 2696–2704. doi: 10.1128/AAC.00064-12
Gao, G., and Wang, J.H. (2022) Research topic on antimicrobial peptides under frontiers in microbiology. Available online at: https://www.caas.cn/xwzx/kyjz/320564.html (accessed April 29, 2022).
Gomes, F., Saavedra, M. J., and Henriques, M. (2016). Bovine mastitis disease/pathogenicity: Evidence of the potential role of microbial biofilms. Pathog. Dis. 74, 1–7. doi: 10.1093/femspd/ftw006
Hao, J. F., Li, J. J., Zhang, Y. H., and Yin, B. S. (2016). Isolation, identification and drug sensitivity test of pathogenic bactcria causing dairy cow mastitis in Jilin province. Prog. Veter. Med. 37, 126–129.
Hao, Y., Wang, J. H., de la Fuente-Nunez, C., and Franco, O. L. (2022). Editorial: Antimicrobial peptides: Molecular design, structure-function relationship, and biosynthesis optimization. Front. Microbiol. 13:888540. doi: 10.3389/fmicb.2022.888540
Hao, Y., Yang, N., Wang, X., Teng, D., Mao, R., Wang, X., et al. (2017). Killing of Staphylococcus aureus and Salmonella enteritidis and neutralization of lipopolysaccharide by 17-residue bovine lactoferricins: Improved activity of Trp/Ala-containing molecules. Sci. Rep. 7:44278. doi: 10.1038/srep44278
Hu, X., Guo, J., Zhao, C., Jiang, P., Maimai, T., Li, Y. L., et al. (2020). The gut microbiota contributes to the development of Staphylococcus aureus-induced mastitis in mice. ISME J. 14, 1897–1910. doi: 10.1038/s41396-020-0651-1
Jiao, J., Mao, R., Teng, D., Wang, X. M., Hao, Y., Yang, N., et al. (2017). In vitro and in vivo antibacterial effect of NZ2114 against Streptococcus suis type 2 infection in mice peritonitis models. AMB Express 7:46. doi: 10.1186/s13568-017-0347-8
Kaczorek, E., Maaczewska, J., Wojcik, R., and Siwicki, A. K. (2017). Biofilm production and other virulence factors in Streptococcus spp. isolated from clinical cases of bovine mastitis in Poland. BMC Vet. Res. 13:398. doi: 10.1186/s12917-017-1322-y
Klein, K., Grønnemose, R. B., Alm, M., Brinch, K. S., Kolmos, H. J., and Andersen, T. E. (2017). Controlled release of plectasin NZ2114 from a hybrid silicone-hydrogel material for inhibition of Staphylococcus aureus biofilm. Antimicrob. Agents Chemother. 61, e00604–e00617. doi: 10.1128/AAC.00604-17
Lee, J. H., Park, J. H., Cho, H. S., Joo, S. W., Cho, M. H., and Lee, J. (2013). Anti-biofilm activities of quercetin and tannic acid against Staphylococcus aureus. Biofouling 29, 491–499. doi: 10.1080/08927014.2013.788692
Levin, B., and Rozen, D. (2006). Opinion-non-inherited antibiotic resistance. Nat. Rev. Microbiol. 4, 556–562. doi: 10.1016/j.scriptamat.2005.11.003
Li, L. B., Wan, L. L., Ga, Y. Q., Wang, J. H., and Zhao, X. (2017). Effective antimicrobial activity of plectasin-derived antimicrobial peptides against Staphylococcus aureus infection in mammary glands. Front. Microbiol. 8:2386. doi: 10.3389/fmicb.2017.02386
Li, Z., Mao, R., Teng, D., Hao, Y., Chen, H., Wang, X., et al. (2017). Antibacterial and immunomodulatory activities of insect defensins-DLP2 and DLP4 against multidrug-resistant Staphylococcus aureus. Sci. Rep. 7:12124. doi: 10.1038/s41598-017-10839-4
Liu, H., Yang, N., Teng, D., Mao, R., Hao, Y., Ma, X., et al. (2021). Fatty acid modified-antimicrobial peptide analogues with potent antimicrobial activity and topical therapeutic efficacy against Staphylococcus hyicus. Appl. Microbiol. Biotechnol. 105, 5845–5859. doi: 10.1007/s00253-021-11454-0
Ma, J., Wang, L., Fan, Z., Liu, S., Wang, X., Wang, R., et al. (2021). Immunogenicity of multi-epitope vaccines composed of epitopes from Streptococcus dysgalactiae GapC. Res. Vet. Sci. 136, 422–429. doi: 10.1016/j.rvsc.2020.12.019
Ma, J. S., Chen, S. Y., and Lo, H. H. (2017). Biofilm formation of beta-hemolytic group G Streptococcus dysgalactiae subspecies equisimilis isolates and its association with emm polymorphism. APMIS 125, 1027–1032. doi: 10.1111/apm.12746
Melchior, M. B., Vaarkamp, H., and Fink-Gremmels, J. (2006). Biofilms: A role in recurrent mastitis infections? Vet. J. 171, 398–407. doi: 10.1016/j.tvjl.2005.01.006
Mwangi, J., Yin, Y., Wang, G., Yang, M., Li, Y., Zhang, Z., et al. (2019). The antimicrobial peptide ZY4 combats multidrug-resistant Pseudomonas aeruginosa and Acinetobacter baumannii infection. Proc. Natl. Acad. Sci. U. S. A. 116, 26516–26522. doi: 10.1073/pnas.1909585117
Mygind, P. H., Fischer, R. L., Schnorr, K. M., Hansen, M. T., Snksen, C. P., Ludvigsen, S., et al. (2005). Plectasin is a peptide antibiotic with therapeutic potential from a saprophytic fungus. Nature 437, 975–980. doi: 10.5281/zenodo.31541
Ngassam-Tchamba, C., Duprez, J. N., Fergestad, M., Visscher, A. D., and Thiry, D. (2020). In vitro and in vivo assessment of phage therapy against Staphylococcus aureus causing bovine mastitis. J. Glob. Antimicrob. Resist. 22, 762–770. doi: 10.1016/j.jgar.2020.06.020
Olson, M. E., Ceri, H., Morck, D. W., Buret, A. G., and Read, R. R. (2002). Biofilm bacteria: Formation and comparative susceptibility to antibiotics. Can. J. Vet. Res. 66, 86–92.
Parastan, R., Kargar, M., Solhjoo, K., and Kafilzadeh, F. (2020). Staphylococcus aureus biofilms: Structures, antibiotic resistance, inhibition, and vaccines. Gene Rep. 20:100739. doi: 10.1016/j.genrep.2020.100739
Pecka-Kiełb, E., Vasil, M., Zachwieja, A., Zawadzki, W., Elečko, J., Zigo, F., et al. (2016). An effect of mammary gland infection caused by Streptococcus uberis on composition and physicochemical changes of cows’ milk. Pol. J. Vet. Sci. 19, 49–55. doi: 10.1515/pjvs-2016-0007
Pillar, C., Sandvang, D., Sahm, D., Draghi, D., Kristensen, H., Torres, M., et al. (2008). “Activity of NZ2114 against Staphylococcal and Streptococcal isolates, including resistant phenotypes,” in Infectious Diseases Society of America 2008 Annual Meeting, (Washington, DC: American Society for Microbiology). doi: 10.1080/10042857.2009.10684907
Pires, R. H., Montanari, L. B., Martins, C. H., Zaia, J. E., Almeida, A. M., Matsumoto, M. T., et al. (2011). Anticandidal efficacy of cinnamon oil against planktonic and biofilm cultures of Candida parapsilosis and Candida orthopsilosis. Mycopathologia 172, 453–464. doi: 10.1007/s11046-011-9448-0
Qi, M. Z., Geng, H., Geng, N., Cui, Y. K., Qi, C. X., Cheng, G. D., et al. (2022). Streptococcus agalactiae-induced autophagy of bovine mammary epithelial cell via PI3K/AKT/mTOR pathway. J. Dairy Res. 89, 178–184. doi: 10.1017/S0022029922000243
Rajasekaran, G., Kim, E. Y., and Shin, S. Y. (2017). LL-37-derived membrane-active FK-13 analogs possessing cell selectivity, anti-biofilm activity and synergy with chloramphenicol and anti-inflammatory activity. Biochim. Biophys. Acta 1859, 722–733. doi: 10.1016/j.bbamem.2017.01.037
Rantala, S. (2014). Streptococcus dysgalactiae subsp. equisimilis bacteremia: An emerging infection. Eur. J. Clin. Microbiol. 33, 1303–1310.
Rantala, S., and Tuohinen, S. (2014). Two cases of cardiac device-related endocarditis due to Streptococcus dysgalactiae subsp. equisimilis (group C or G streptococci). BMC Infect. Dis. 14:174. doi: 10.1186/1471-2334-14-174
Rutherford, S. J., Rycroft, A. N., and Ridler, A. L. (2014). Sources of Streptococcus dysgalactiae in English and Welsh sheep flocks affected by infectious arthritis (joint ill). Vet. Rec. 14:174. doi: 10.1136/vr.101753
Teng, D., Wang, X. M., Xi, D., Mao, R. Y., Zhang, Y., Guan, Q. F., et al. (2014). A dual mechanism involved in membrane and nucleic acid disruption of avbd103b, a new avian defensin from the king penguin, against Salmonella enteritidis CVCC3377. Appl. Microbiol. Biotechnol. 98, 8313–8325. doi: 10.1007/s00253-014-5898-x
Wang, L., Si, W., Xue, H., and Zhao, X. (2017). A fibronectin-binding protein (FbpA) of Weissella cibaria inhibits colonization and infection of Staphylococcus aureus in mammary glands. Cell. Microbiol. 19:e12731. doi: 10.1111/cmi.12731
Wang, X., Teng, D., Wang, X., Hao, Y., Chen, H., Mao, R. Y., et al. (2019). Internalization, distribution, and activity of peptide H2 against the intracellular multidrug-resistant bovine mastitis-causing bacterium. Sci. Rep. 9:7968. doi: 10.1038/s41598-019-44459-x
Wang, X., Wang, X. M., Teng, D., Mao, R. Y., Hao, Y., Yang, N., et al. (2018). Increased intracellular activity of MP1102 and NZ2114 against Staphylococcus aureus in vitro and in vivo. Sci. Rep. 8:4204. doi: 10.1038/s41598-018-22245-5
Wiegand, I., Hilpert, K., and Hancock, R. E. W. (2008). Agar and broth dilution methods to determine the minimal inhibitory concentration (MIC) of antimicrobial substances. Nat. Protoc. 3, 163–175. doi: 10.1038/nprot.2007.521
Xi, H., He, D., Li, D., Liu, S., Wang, G., Ji, Y., et al. (2020). Bacteriophage protects against Aerococcus viridans infection in a murine mastitis model. Front. Vet. Sci. 7:588. doi: 10.3389/fvets.2020.00588
Yang, N., Liu, X., Teng, D., Li, Z., Wang, X., Mao, R. Y., et al. (2017). Antibacterial and detoxifying activity of NZ17074 analogues with multi-layers of selective antimicrobial actions against Escherichia coli and Salmonella enteritidis. Sci. Rep. 7:3392. doi: 10.1038/s41598-017-03664-2
Yang, N., Teng, D., Mao, R. Y., Hao, Y., Wang, X., Wang, Z. L., et al. (2019). A recombinant fungal defensin-like peptide-P2 combats multidrug-resistant Staphylococcus aureus and biofilms. Appl. Microbiol. Biotechnol. 103, 5193–5213. doi: 10.1007/s00253-019-09785-0
Zhang, Q. J., Yang, N., Mao, R. Y., Hao, Y., Ma, X. X., Teng, D., et al. (2021). A recombinant fungal defensin-like peptide-P2 combats Streptococcus dysgalactiae and biofilms. Appl. Microbiol. Biotechnol. 105, 1489–1504. doi: 10.1007/s00253-021-11135-y
Zhang, Y., Teng, D., Mao, R., Wang, X. M., Xi, D., Hu, X. Y., et al. (2014). High expression of a plectasin-derived peptide NZ2114 in Pichia pastoris and its pharmacodynamics, postantibiotic and synergy against Staphylococcus aureus. Appl. Microbiol. Biotechnol. 98, 681–694. doi: 10.1007/s00253-013-4881-2
Keywords: Streptococcus dysgalactiae, antimicrobial peptide, NZ2114, bovine mastitis, biofilms
Citation: Yang N, Zhang Q, Mao R, Hao Y, Ma X, Teng D, Fan H and Wang J (2022) Effect of NZ2114 against Streptococcus dysgalactiae biofilms and its application in murine mastitis model. Front. Microbiol. 13:1010148. doi: 10.3389/fmicb.2022.1010148
Received: 02 August 2022; Accepted: 30 August 2022;
Published: 15 September 2022.
Edited by:
Chang-Wei Lei, Sichuan University, ChinaReviewed by:
Lijie Tang, Northeast Agricultural University, ChinaCopyright © 2022 Yang, Zhang, Mao, Hao, Ma, Teng, Fan and Wang. This is an open-access article distributed under the terms of the Creative Commons Attribution License (CC BY). The use, distribution or reproduction in other forums is permitted, provided the original author(s) and the copyright owner(s) are credited and that the original publication in this journal is cited, in accordance with accepted academic practice. No use, distribution or reproduction is permitted which does not comply with these terms.
*Correspondence: Da Teng, dGVuZ2RhQGNhYXMuY24=; Huan Fan, ZmFuaHVhbjEzMjEyMTA3MjMxQDEyNi5jb20=; Jianhua Wang, d2FuZ2ppYW5odWFAY2Fhcy5jbg==, d2FuZ2ppYW5odWEucGVraW5nQHFxLmNvbQ==; orcid.org/0000-0002-4048-6055
†These authors have contributed equally to this work
Disclaimer: All claims expressed in this article are solely those of the authors and do not necessarily represent those of their affiliated organizations, or those of the publisher, the editors and the reviewers. Any product that may be evaluated in this article or claim that may be made by its manufacturer is not guaranteed or endorsed by the publisher.
Research integrity at Frontiers
Learn more about the work of our research integrity team to safeguard the quality of each article we publish.