- 1Division of Plant Pathology, ICAR-Indian Agricultural Research Institute, New Delhi, India
- 2Division of Entomology, ICAR-Indian Agricultural Research Institute, New Delhi, India
Beauveria bassiana is an entomopathogenic fungus that causes the white muscadine disease in insects. The majority of entomopathogenic fungi are soil and insect borne, 15 soil samples were collected from seven different locations during 2021, from January to December. Similarly, during 2022, March to December, 15 fungus-infected insect specimens were collected from five different locations hence soil and insect samples from various ecosystems were collected. As a result, 30 B. bassiana isolates from 11 different geographical areas were identified using morphological characteristics and multilocus sequence data in this investigation. The taxonomical positions of the isolates were determined using morphological characteristics and phylogenetic inferences based on three loci (Internal Transcribed Sequence, Elongation Factor-1α, and B. bassiana chitinase 1). In phylogenetic analysis of B. bassiana, the Maximum Likelihood analytical method produced distinct tree topology when compared to Neighbor-joining and minimum evolution. Three isolates viz., Bb3, Bb7 and Bb20 were found closely linked with reference isolate (KTU-24) and other showed the higher population diversity among them. The genetic distances of 30 B. bassiana isolates revealed that 15 were not closely related (D varied from 0.003 to 0.036). The pathogenicity of B. bassiana isolates from various hosts along with one commercial formulation (Beveroz) was assessed against Tetranychus truncatus under in vitro conditions by a completely randomized design (CRD) experiment. The same experiment was repeated thrice to confirm the pathogenicity of B. bassiana against T. truncatus. Later, the collected T. truncatus mortality data was converted into corrected mortality by using the Abbott formula and the values were examined using analysis of variance (ANOVA) in SPSS 23.0 software. Duncan’s Multiple Comparison Test was also done to compare the percentage mortality rates among the 30 B. bassiana isolates. The recorded results showed that the Bb6, Bb15 and Bb12 isolates caused significantly higher mortality of T. truncatus, i.e., 97.73, 96.73 and 94.50% respectively, than the other isolates. This study showed the relativeness among the B. bassiana isolates and establishes their bio-efficacy against T. truncatus, which further can be used for commercialization as bio-pesticide.
Introduction
Beauveria is the most prevalent entomopathogenic fungal genus worldwide (Robene-Soustrade et al., 2015; Chen et al., 2018). It had broad host range (Inglis et al., 2001) and can be isolated from insects, mites, and soil in all parts of the world (Boucias and Pendland, 1998). Beauveria bassiana populations are genetically and phenotypically diverse, range from sympodial to whorled clusters of short-globose to flask-shaped conidiogenous cells, which give rise to a succession of one-celled, hyaline, holoblastic conidia carried on a progressively elongated sympodial rachis, morphological differentiation. In Beauveria, conidia are the most important morphological trait for species identification. Conidia are globose, ellipsoidal, reniform to cylindrical or comma shaped and 1.7 to 5.5 μm in diameter in size. However, phenotypic traits are insufficient to identify Beauveria isolates or to monitor biocontrol agent releases in the field (Castrillo et al., 2003). As a result, a number of genetic approaches have been developed to address taxonomy and genetic variation issues in B. bassiana.
B. bassiana genetic diversity, characterization, and relatedness were successfully studied using molecular markers. Grasp population structure, gene flow, isolate type, ecology, and possible impact when utilized as insect biological control agents requires a thorough understanding of genetic diversity and intraspecies relationships. For molecular identification of B. bassiana isolates, Hegedus and Khachatourians (1996) developed a B. bassiana-specific PCR-based method. Since many years ago, the ribosomal DNA (rDNA) internal transcribed spacer (ITS) region has been recognised as the key “universal” genetic barcode for fungi. Intragenomic comparisons of high quality genomes of Hypoxylaceae (Xylariales, Ascomycota) revealed a majority of polymorphisms in the ITS regions (Stadler et al., 2020). A recent DNA sequence analysis of the ITS and the elongation factor 1-α (EF1-α) gene of B. bassiana has shown that a sampling of globally distributed species complexes combined with morphological and molecular phylogenetic analysis is an efficient strategy for assessing species diversity and the necessary first step in detailing the species evolutionary history and historical ecology (Rehner and Buckley, 2005).
The virulence of B. bassiana can be improved by understanding pathogenic pathways. B. bassiana infects the host insect by penetrating the cuticle. The insect cuticle, which is made up of a thin outer epicuticle with lipid and proteins and a thick procuticle with chitin and proteins, forms an efficient barrier protecting against invasion by eukaryotic parasites and infection by microorganisms (Moret and Moreau, 2012). By producing proteases first, followed by chitinases, and lipases, B bassiana penetrates the insect cuticle through a combination of mechanical pressure from expanding hyphae and enzymatic destruction of the proteins, chitin, and lipids that make up the cuticle (Gillespie et al., 2000). The chitinase gene has been intensively studied for a pest control agent due to its ability to dissolve chitin (Kramer et al., 1993). The entomopathogenic fungus Bbchit1 gene can greatly improve the virulence of transgenic B. bassiana against aphids (Fang et al., 2005). Beauveria species are utilized as a model system for studying fungal insect pathology and biological insect pest control (Rehner and Buckley, 2005; Imoulan et al., 2016). Spider mites are most common and important polyphagous pests of agricultural ecosystem (Sakunwarin et al., 2003; Pang et al., 2004). Nearly 1,300 species of spider mites are described worldwide, 100 of them are phytophagous and 10 of them are serious agricultural pests. Tetranychus truncatus Ehara, a spider mite species, commonly called as a cassava mite, is a significant pest of agricultural crops. It was initially reported in Japan on mulberry (Ehara, 1956). Traditional acaricides are being used to manage the Tetranychus group of spider mites, which resulted in the development of resistance to approximately 800 acaricides and 93% of those involve tetranychids (Ullah and Gotoh, 2013). In addition, high fecundity, inbreeding and a very short life cycle result in numerous generations per year, especially in warmer circumstances, which builds the selection pressure for resistance. To solve this issue, numerous studies have demonstrated that B. bassiana has insecticidal properties in the form of mycopesticides and commercial endophytic fungi, allowing us to employ it as a natural pesticide alternative (Jaber and Ownley, 2018). Several strains of B. bassiana have been developed into a commercial biopesticides of several pests (BotaniGard®ES, BotaniGard®22WP, Naturalis®TNO, and Mycotrol®). However, currently there are no registered formulations with CIB&RC (Central Insectiside Board and Registration Committee) against mites in India. Therefore, the present study was undertaken to screen a highly virulent indigenous B. bassiana strains against T. truncatus.
Commercial bio-pesticides have been recommended in India for the control of a variety of insect pests on different crops, but their use has resulted in lower pesticide efficacy or non-adaptability to Indian agro-climatic conditions. With these considerations, we present a molecular phylogenetic analysis of native B. bassiana strains from different regions. We compared and integrated reconstructed phylogenies of three nuclear loci, ITS+ EF1-α + Bbchit1 gene, to study the genetic diversity, population dynamics and co-evolutionary pattern of interaction between B. bassiana and its insect host. We also established that B. bassiana isolates which may be used to control T. truncatus and may be commercialized in future.
Materials and methods
Soil and specimen collection and fungus isolation
During 2021, from January to December, fifteen soil samples were collected from seven different locations. Similarly, during 2022, March to December, fifteen fungus-infected insect specimens were collected from five different locations (Table 1). Using the serial dilution approach, Beauveria strains were isolated from soil samples. Conidia growing on insect cadavers were transferred onto PDA (Potato Dextrose Agar) plates and grown at 25°C. The isolated fungus colonies were transferred to fresh PDA medium. The purified fungal strains were maintained on PDA slants at 4°C. All B. bassiana isolates were deposited at the Indian Type Culture Collection (ITCC) at the Indian Agricultural Research Institute, New Delhi.
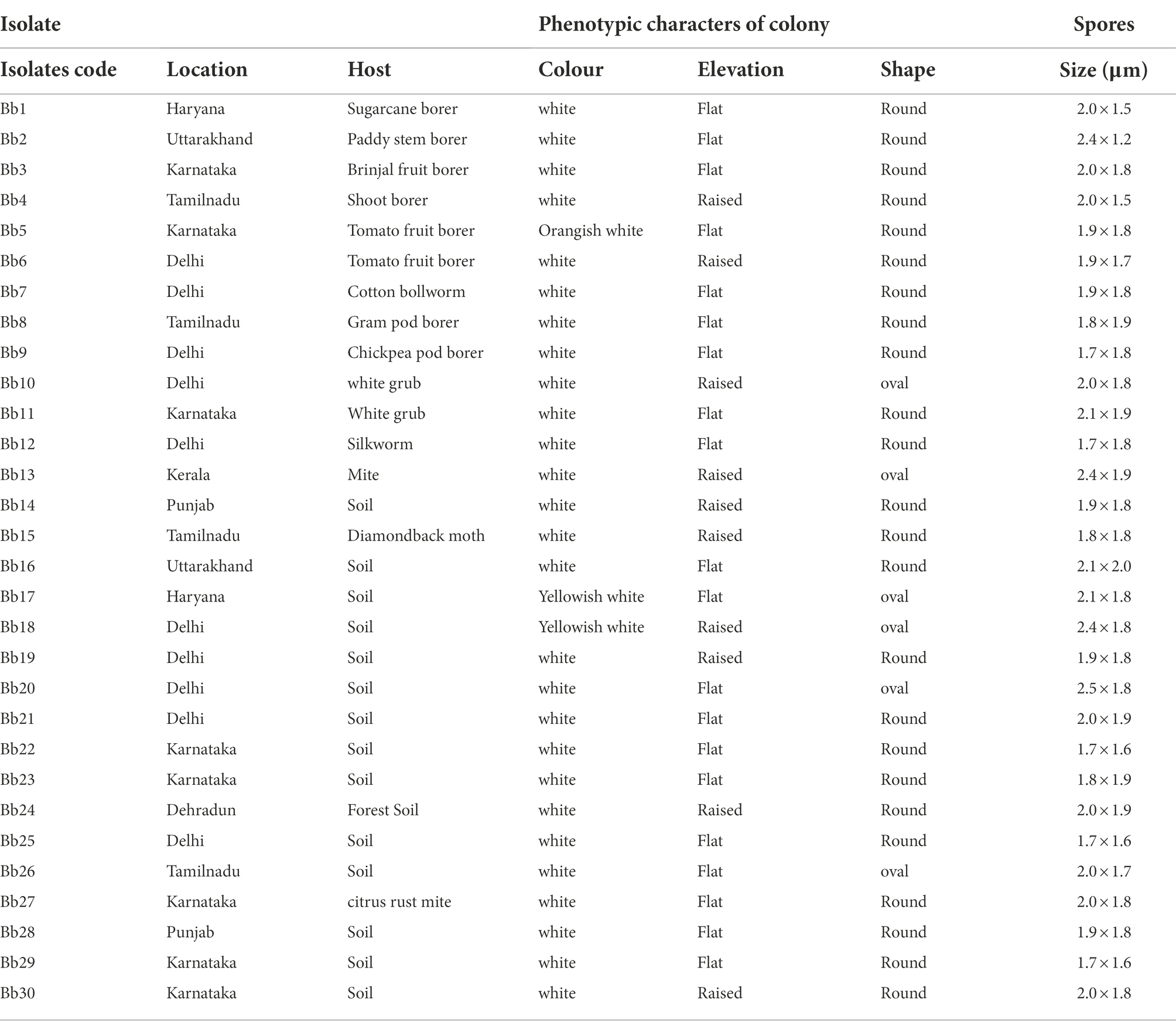
Table 1. Morphological characters of 30 isolates of B. bassiana collected from different locations at 16 DAI on Potato Dextrose Agar (PDA) medium.
Morphological observations
PDA slant cultures were transferred to PDA plates and incubated for 14 days at 25°C. Mycelia along with sporulation from the PDA cultures were placed on the clean side in a drop of water and then overlaid with a coverslip for morphological examination. A compound microscope along with camera attachment (Progres capture pro2.7-JENOPTIK) was used to make micro-morphological observations, photomicrographs and measurements. All of these isolates were identified using Humber’s identification key (Humber, 2012).
Molecular identification
At 25°C, Beauveria isolates were grown in 50 ml PD broth. Mycelial biomass was collected on filter paper and rinsed three times in sterile distilled water. Genomic DNA was isolated as described by Rehner and Buckley (2005). The quantity and purity of DNA were measured using a Thermo Scientific NanoDrop Lite Spectrophotometer (Thermo Scientific, Waltham, MA, United States). Similarly, aliquots of PCR products were electrophoresed on a 0.8% agarose gel with ethidium bromide and UV transillumination were used to verify them. Supplementary Table 1 lists the three nuclear gene, i.e., ITS, EF1-α, and Bbchit1primers used to PCR amplify from total DNA of distinct fungal isolates. All PCR reactions were performed in a final volume of 25 μl containing 12.5 μl, 2 × Taq PCR Master Mix (Thermo Scientific), 1 μl of each primer, 1 μl of genomic DNA and 9.5 μl of nuclease-free water. The targeted gene’s PCR conditions were followed exactly as reported (ITS and EF1; Rehner and Buckley, 2005; chitinase; Khemika et al., 2006). The PCR results were run on a 1.8% agarose gel and sequenced using the Sanger technique by outsourcing [Anuvanshiki (OPC) Pvt. Ltd].
Phylogenetic analysis
The combined 3-gene (ITS+EF1-α + Bbchit1) sequences were used in the phylogenetic analysis of 32 isolates, which contained 30 B. bassiana isolates (Bb1 to Bb30), 1 reference strain (B. bassiana: KTU-24) and 1 outgroup (Metarhizium anisopliae: E6). NCBI nucleotide BLAST analysis was used to compare the retrieved nucleotide sequences with GenBank database. Sequences were constructed, modified, and aligned using BioEdit. BioEdit (version 7.09) and Clustal W were used to perform cluster analyses. In the phylogenetic analysis, ambiguously aligned sites were eliminated, and gaps were treated as missing data. After that, the sequences were artificially aligned and ambiguous areas caused by insertions and deletions (indel) were removed. The gene sequences were concatenated after alignment. MEGA version 10 was used largely for maximum-likelihood (ML) phylogenetic analysis (Swofford, 2002) with the Kimura-two-parameter model, heuristic searches with nearest-neighbor interchange branch switching and 10 random taxon additions were adjusted, and bootstrapping was set to 1,000 replications. TrN + I were the best model test for estimating the data (Posada and Crandall, 1998). The robustness of ML tree topology was confirmed using Minimum Evolution (ME) and Neighbour joining (NJ) with 1,000 bootstrap replications. Tamura-Nei distance was used to implement NJ.
Bioactivity of Beauveria bassiana against Tetranychus truncatus
Tetranychus truncatus culture
Isofemale lines of T. truncatus culture was raised on clean mulberry (Morus alba) leaves on sterilized foam sheet saturated in water in an insect proof climate control chamber with a temperature of 27 ± 1°C, 12:12 l:D photoperiod and a relative humidity of 65 ± 5%. One to 2 days old females were used for the bioassay.
Preparation of conidial suspension of Beauveria bassiana
Thirty B. bassiana isolates and one commercial formulation (Beveroz: B. bassiana, Utkarsh®) were grown on PDA medium plates at 25 ± 2°C for 7–10 days until extensive conidiation formed. By flooding the plates with sterile aqueous (0.02%) Tween-80, the conidia were extracted from 2-week-old cultures. To remove any mycelial pieces, the suspension was filtered through sterile muslin fabric (Sasidharan and Varma, 2005). A Neubauer hemocytometer was used to measure conidia concentration under a phase contrast microscope, and the concentration was adjusted to 1 × 108 conidia/mL by diluting with Tween-80 solution. Spreaded the 100 μl of a 1 × 108 conidia/mL suspension onto the surface of PDA Petri plates and assessed conidial viability under compound microscope. The numbers of live and non-viable conidia were counted under a microscope after incubation at 28°C for 24 h. If the germ tube was longer than the diameter of the conidium, it was regarded as germinated (Sevim et al., 2010b).
In vitro bioassay of Beauveria bassiana
By using completely randomized design (CRD) experiment, the pathogenicity of 30 B. bassiana isolates from various hosts along with one commercial formulation (Beveroz) was assessed against T. truncatus under in vitro conditions. Mulberry leaves were cut into 4 cm2, both sides of leaf discs were sprayed with 1 ml of 1 × 108 conidia per mL suspensions of 30 collected and one commercial B. bassiana isolates using potters tower and allowed to dry in room temperature, after drying leaf discs were placed upside down on half inch damp white foam sheets in a 11 cm disposable Petri dishes. On each plate 30 mites were transferred on to the leaf disc. Each isolate was replicated five times. Control was maintained by spraying water containing 0.02% Tween 80 on both sides of leaf discs. Under stereomicroscope, even after being touched with a brush or needle, T. truncatus did not move; we can conclude that the mites have dead mites. Like this, the number of T. truncatus mortality data obtained from 31 treatments (30 B. bassiana isolates + one commercial formulation- Beveroz treated T. truncatus) was recorded under stereomicroscope at 3 DAI, 5 DAI and 7 DAI. Similarly, after 5 DAI and 8 DAI, on the body surface of T. truncatus was completely covered by B. bassiana mycelium (mycosis); this mycosis data was also recorded under a stereomicroscope.
Statistical analysis
The T. truncatus mortality data obtained from 31 treatments (30 B. bassiana isolates + one commercial formulation-Beveroz treated T. truncatus) in the study was converted to percentage values. Percentage mortality was corrected by Abbott (1925). The corrected mortality was transformed using the arc-sin transformation, and then examined using one way—analysis of variance (ANOVA). Duncan’s Multiple Comparison Test was used to compare the percentage mortality rates. SPSS 23.0 was used to conduct all statistical analyses (IBM Corp, 2013).
Results
Morphological observations
The colony colour, shape, and growth rate of B. bassiana isolates were observed macroscopically. Bb5 developed orangish white, Bb17 and Bb18 isolates developed yellowish white colonies, while all other isolates showed white colonies (Supplementary Figures 1A,B). In terms of colony shape, all colonies were circular; no significant among isolates were found. The conidia produced on elongated sympodial rachis of flask shaped phialides were single celled, hyaline and round with a size of 1.7–2.5 × 1.6–1.8 μm (Supplementary Figure 2; Figure 1; Table 1). In terms of mean colony growth rate, the Bb16 isolate outperformed the other 29 isolates (Supplementary Table 2).
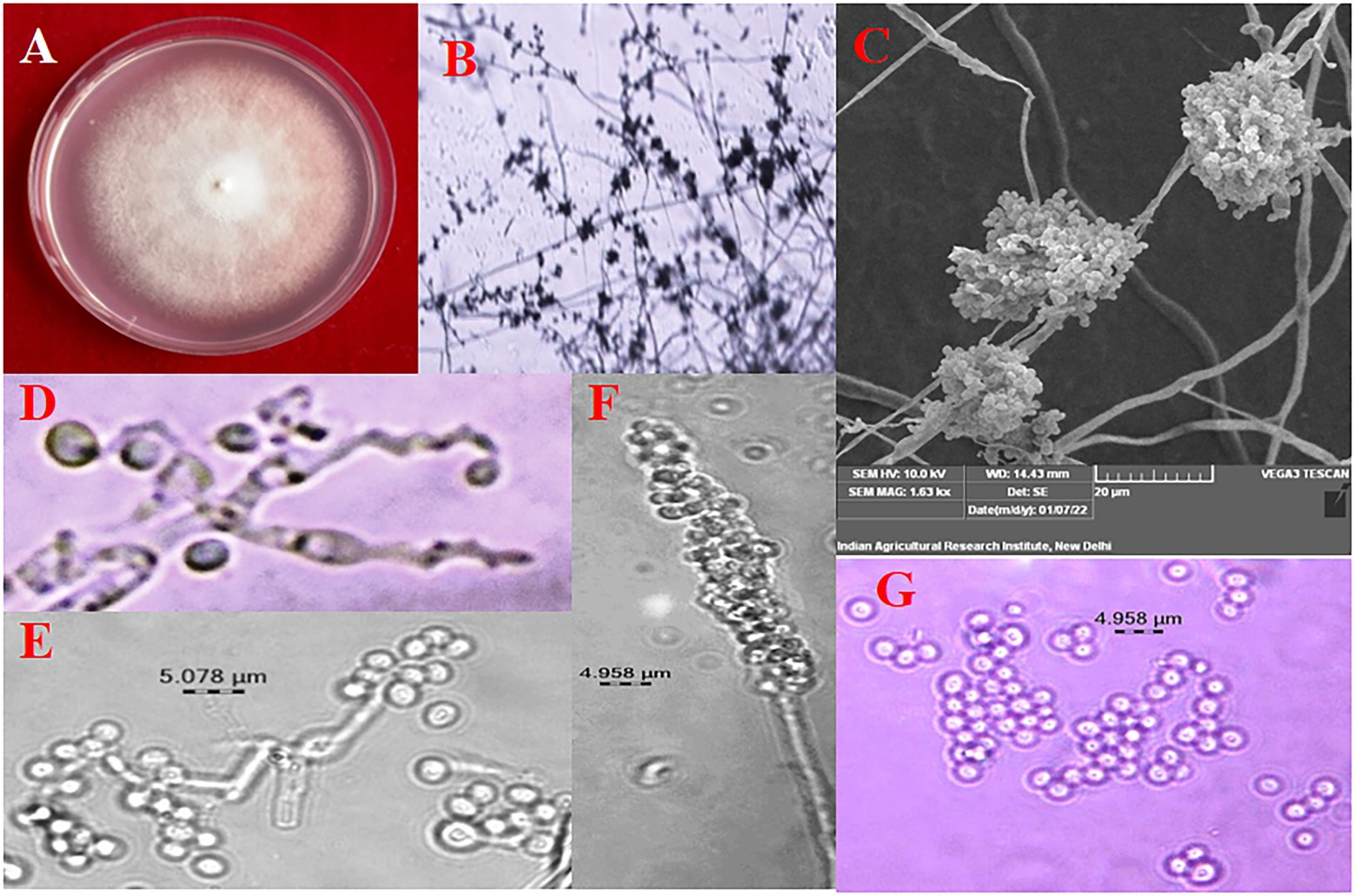
Figure 1. Morphological Characterization of B. bassiana (A) colony on PDA, (B) conidiophore branching under 10X, (C) conidial cluster under SEM, (D) elongated sympodial rachis, (E) and (F) conidia production on rachis, (G) conidia.
Molecular identification and phylogenetic analysis
Three loci (ITS, EF1-α and Bbchit1) gene amplified products of all the B. bassiana isolates were confirmed under gel electrophoresis (Supplementary Figures 3–5). The generated sequences of all the isolates were identified as B. bassiana based on NCBI BLAST analysis. All three loci gene sequences of 30 isolates were deposited in Gen Bank and the accession numbers were obtained (Supplementary Table 3). Phylogenetic analyses based on combined partial ITS+ EF1- α + Bbchit1 sequences of B. bassiana isolates were performed using the ML (Figure 2), NJ (Supplementary Figure 6) and ME (Supplementary Figure 7) methods of the MEGA version 10 programme and compared with representative sequences from the work of Sevim et al. (2010a). The nucleotides of 2,544, ITS+EF1-α + Bbchit1 gene sites were examined for the 32 samples with base compositions of A = 25%, T = 25%, C = 25%, and G = 25%.
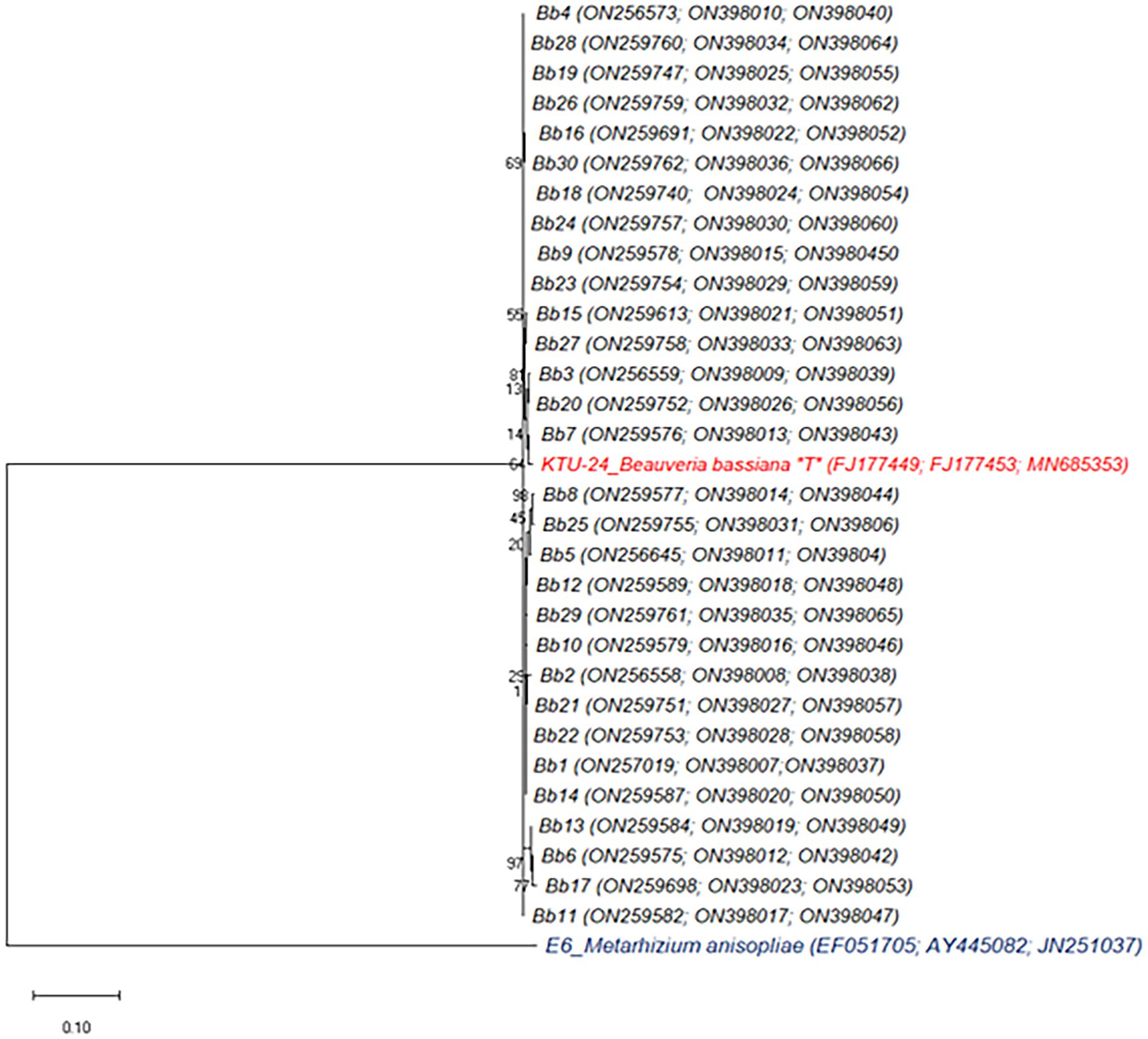
Figure 2. Phylogenetic analysis of B. bassiana isolates based on combined partial ITS+TEF + Bbchit1 sequences. The dendrogram was constructed by using Maximum Likelihood method with the kimura 2-parameter model with MEGA 10 version. Isolate representing ex-type material is marked with “T.” Bootstrap values shown next to nodes are based on 1,000 replicates. The tree was rooted using isolate Metarhizium anisopliae E6 as the outgroup.
The score of the ML tree was -lnL = 7628.42 log likelihood. ML analytical method executed dissimilar tree topology with NJ and ME (Figure 2). In NJ and ME tree, except six isolates viz., Bb10, Bb11, Bb13, Bb14, Bb19 and Bb20 remaining all other isolates were clustered with B. bassiana reference strain (KTU-24) but in case of ML tree, three isolates viz., Bb3, Bb7 and Bb20 clustered very closely with KTU-24 and other showed the higher population diversity. TN93 + G was the best-fit model to explore the dataset (substitution rate matrix R, a = 0.00, b = 0.00, c = 0.07, d = 0.55, e = 4.37). The proportion of invariable sites (I) was 0.00 and gamma distribution shape parameter = 0.22. The pairwise genetic distances among the B. bassiana isolates are shown in Supplementary Table 4. The isolates (Bb1 to Bb30) were genetically not closely related (D ranged between 0.003 and 0.036).
Bioassay of Beauveria bassiana
Under laboratory conditions, all 30 B. bassiana isolates exhibited different levels of mortality (Table 2) and mycosis (Table 3) of T. truncatus. Bb6 isolate collected from tomato fruit borer showed extremely high virulence (97.78%) against T. truncatus by killing of mites, followed by Bb15 and Bb12 (96.73 and 94.50%, respectively), which were collected from pupa of diamondback moth and silkworm, respectively, at 7 days after inoculation (DAI) at a concentration of 1 × 108 conidia/mL. After 5–8 DAI, the T. truncatus body was covered with mycelium and conidia, which was confirmed by observing under compound microscope (Figure 3) and SEM (Figure 4). The other isolates of B. bassiana were significantly less virulent against T truncatus including commercial isolate. As a result, Bb6, a native novel isolate of B. bassiana was considered as a highly virulent isolate of India against T truncates. This isolate was very effective in the mortality of T. truncatus even at different stages of growth (Figure 5A). In addition, when compared to other isolates including commercial one, Bb6 and Bb15 isolates produced the greatest mycosis rate of 88% at 8 DAI (highest sporulation), followed by Bb12 (85%; Figure 5B). Mortality rates and mycosis levels in all other isolates ranged from 11 to 89.12% and 0 to 81%, respectively (Supplementary Figures 8, 9). The highly virulent B. bassiana isolates grown on mites were re-isolated and maintained for subsequent research work.
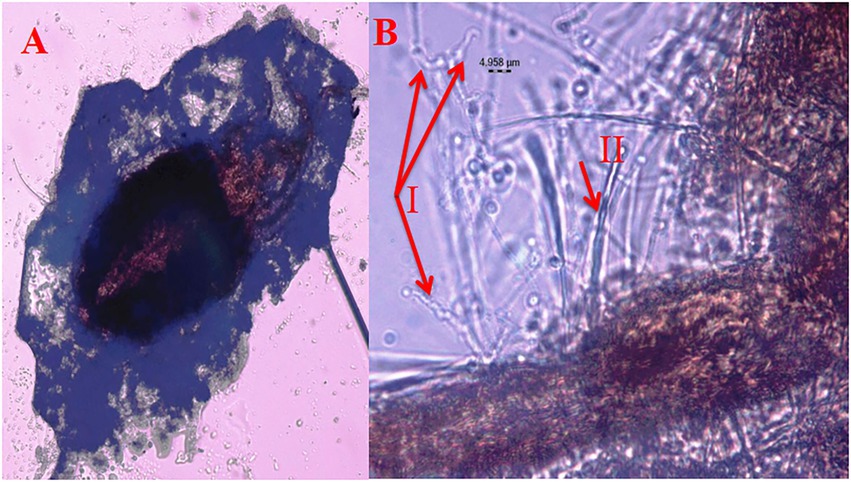
Figure 3. Mortality of T. truncatus due to B. bassiana (A) T. truncatus infected with B. bassiana under compound microscope, (B) details of B. bassiana (I.phialide and rachis) and T. truncatus (II trichomes).
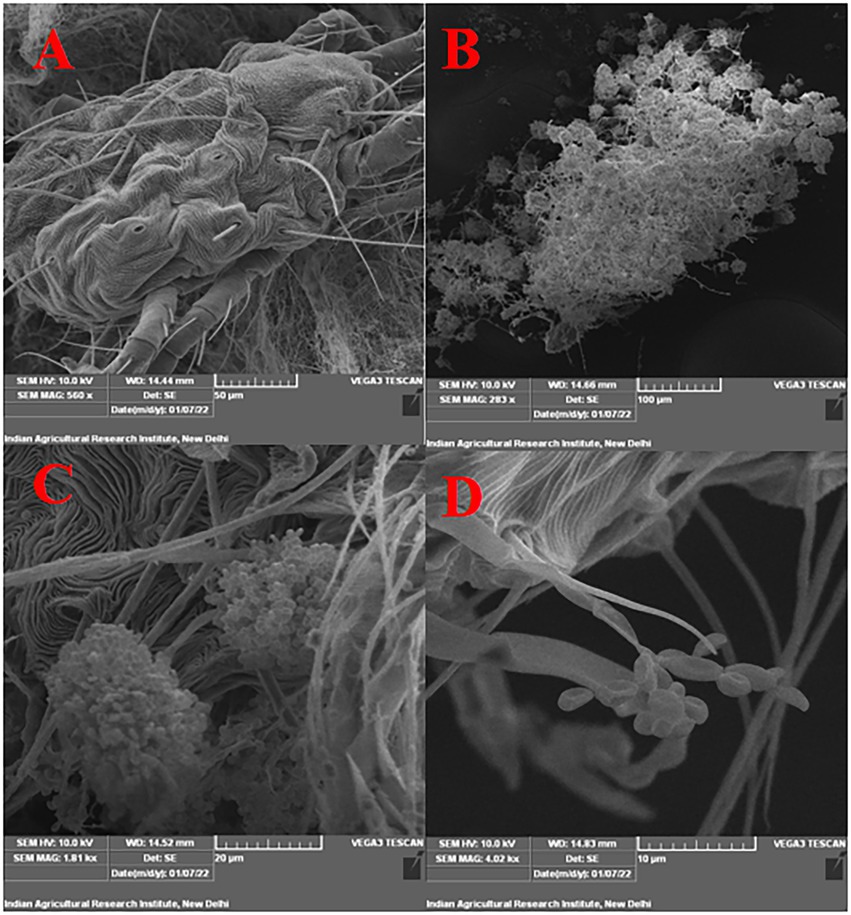
Figure 4. Infection confirmation under SEM (A) untreated control (no mortality), (B) growth and sporulation of B. bassiana on dead T. truncatus under 100 μm scale, (C) under 20 μm scale, (D) under 10 μm scale (phialides and conidia are seen).
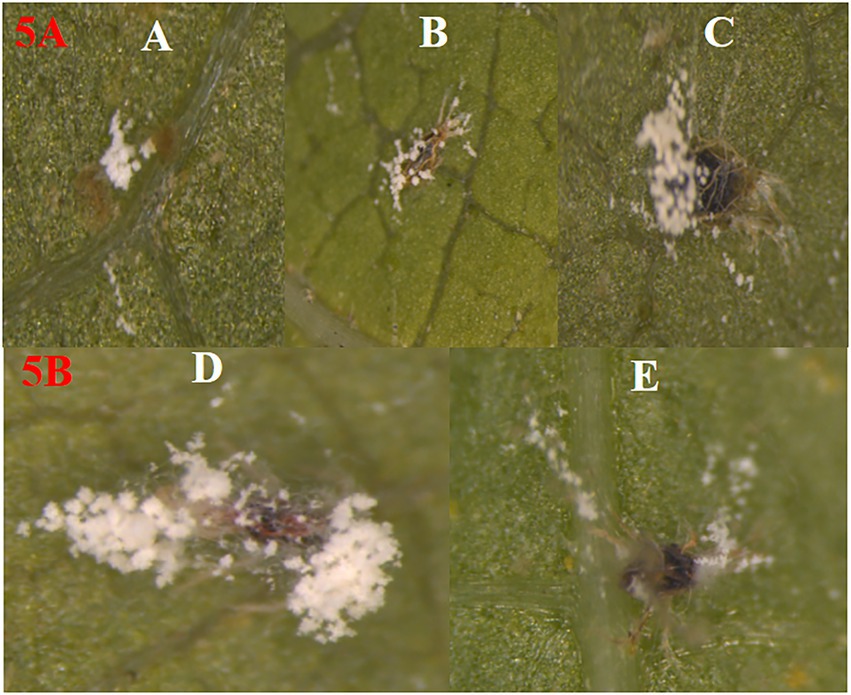
Figure 5. (5A) Effect of B. bassiana at all growth stages of T. truncatus. Mortality of T. truncatus at different growth stages due to B. bassiana infection (A) egg, (B) nymph, (C) adult. (5B) Growth and sporulation differences of (D) potential (Bb6) and (E) non potential (Bb23) isolates of B. bassiana. on T. truncatus. Cadaver.
Discussion
The results showed that the agricultural soils and crop pests are important reservoirs of entomopathogenic fungus B. bassiana, based on morphological and genetic characterization of this fungus collected from various hosts at different geographical locations. In this regard, it is critical to investigate local conditions in order to identify potential, virulent fungal species. According to Humber’s identification key, all collected 30 isolates were identified as B. bassiana based on colony characters and conidial shape and size. All isolates had comparable conidial morphology with hyaline, smooth, and round to oval conidia, which were similar to those previously described (Brady, 1979). Conidia are produced holoblastically from basally inflated conidiogenous cells and grouped amid aerial hyphae as white, spherical clusters. Swollen at the base, the conidiogenous cells stretched into a slender, geniculate or irregularly curved, and denticulate rachis. The isolates morphology matches Glare and Inwood (1998). Phylogenetic analysis of combined three partial sequences of ITS+EF1-α + Bbchit1genes revealed that out of 30 B. bassiana isolates, 24 isolates were clustered with B. bassiana reference strain (KTU-24) in NJ and ME tree but in case of ML tree, only three isolates viz., Bb3, Bb7 and Bb20 closely clustered with KTU-24. That means ML tree is most appropriate to analyze the population diversity among the B. bassiana isolates. The more diverse isolate will give the more chance to prove as potential bio-control agent against insect pests.
The analysis of macroscopic and microscopic features is the basis for traditional morphological identification of fungi (Piontelli, 2015). The most commonly utilized characteristics for determining the species of Beauveria are its conidial shape and size (Humber, 2012). Although extremely useful, this identification frequently has limitations in terms of the genetic variability and phenotypic flexibility of the features employed to distinguish species (Paz et al., 2011). Now a days, rDNA genes and ITS allow the investigation of the evolutionary relationships among several groups of entomopathogenic fungi, in addition to morphological identification (Rehner and Buckley, 2005). Therefore, it is crucial to compare the outcomes of morphological characterization and molecular approaches in order to generate more precision in the identification of fungal isolates and study their genetic variability (Hibbett et al., 2011). However, molecular techniques should be utilized to supplement the research to produce a comprehensive, accurate, and appropriately descriptive identification rather than to replace or eliminate the requirement for the usage of classical morphological taxonomy tools (Hyde et al., 2010). Similarly, in the present work in morphological identification, out of 30 Beauveria isolates, only three isolates (Bb5, Bb17 and Bb18) had distinct colony shape, color, and shape appearance. But in the case of phylogenetic analysis, out of 30 B. bassiana isolates, only three isolates, viz., Bb3, Bb7 and Bb20 clustered closely with KTU-24 (reference strain). So, we can observe that in both cases, not the same three isolates show the same characteristics. Therefore, a technique of morphological and molecular identification for biocontrol agents should be perfect when used as a biological control system against pests (Coates et al., 2002). Pathogenicity related gene identification, in addition to ITS and Ef-1α (housekeeping) genes, aids in determining the virulence nature of pathogenic fungi. Chitin is a key component of inset cuticle, which serves as the first line of defense against pathogens. Over expression of Bbchit1 improved B. bassiana ability to digest insect cuticle, resulting in higher insect pathogenicity (Murad et al., 2007). In this investigation, all B. bassiana isolates showed amplification at 1047 bp, indicating that all isolates have the chitinase producing gene, which can be correlated with their bio-control ability and expressional analysis. Pallavi (2004) found that entomopathogenic fungal pathogens produced extracellular chitinase against Helicoverpa armigera, which supports this finding.
Phylogenetic analysis of combined three partial sequences of ITS+EF1-α + Bbchit1genes revealed that out of 30 B. bassiana isolates, 24 isolates were clustered with B. bassiana reference strain (KTU-24) in NJ and ME tree but in case of ML tree, only three isolates viz., Bb3, Bb7 and Bb20 closely clustered with KTU-24. That means ML tree is most appropriate to analyze the population diversity among the B. bassiana isolates. The more diverse isolate will give the more chance to prove as potential bio-control agent against insect pests. Apart from that, Bb5, Bb17, and Bb18 isolates had distinct colony appearances when compared to the remaining isolates, but.
We also tested all B. bassiana isolates for pathogenicity against T. truncatus. Most entomopathogenic fungi having acaricidal activities against T. truncatus can be attributed to disruption of mite development via penetration and subsequent nutrient uptake, according to the earlier studies (Shi and Feng, 2004; Zhang et al., 2014). In the present study, Bb6 isolate seems to have a good potential as a biological control agent against T. truncatus as this isolate caused the highest mortality rate and greater mycosis (sporulation) than the other isolates. The findings of this study are similar to Gatarayiha et al. (2012), who were all used entomopathogenic fungi to control mites. The blastospore and aerial conidia efficiency of two B. bassiana isolates against T. urticae at various stages of development were tested by Al Khoury et al. (2020). 109 blastospores/ml of B. bassiana strain exhibited the highest mortality against eggs, motile juveniles, and adults (52, 67.09 and 95.3%, respectively). Similarly, Yun et al. (2017), recorded 77 to 100% mortality rates for T. urticae at 7 DAI of aerial conidia, culture filtrate and blastospores of B. bassiana 2R-3-3-1 strain against T. urticae. Similarly, Yanar et al. (2018), observed mortality rates of T. urticae adults from the range of 32.5–72.5% at the end of 72 h incubation period by applying a single dose (5 × 106 conidia ml−1) of B. bassiana isolates. He also reported mycosis of T. urticae, which was ranging between 2.5 and 40.0%. Gatarayiha et al. (2012) reported that mortality caused by 62 B. bassiana isolates of T. urticae adults ranged between 0.5 and 92.8%; and 23 isolates caused more than 50% mortality. According to De la Rosa et al. (1997), differences in virulence of entomopathogenic fungal isolates are likely due to the presence of enzymes that influence the fungus penetration process. Secondary metabolites, such as toxins like beauvericin found in B. bassiana, could also play a role in the observed virulence variation (Roberts and St Leger, 2004). Al Khoury et al. (2019), observed the acaricidal activity of beauvericin against motile stages of T. urticae with a concentration of 10, 100 and 1,000 μg/g. In this investigation, Bb6, Bb15, and Bb12 were originally isolated from pest, which caused higher mortality of T. truncatus but few isolates isolated from the soil also exhibited more than 50% mortality (Bb17, Bb19, Bb20, Bb24, Bb26, Bb27, Bb28, Bb29 and Bb30 showed 70.64, 69.59, 76.02, 89.12, 88.1, 83.63, 83.68 and 77.13% mortality respectively). The present investigation revealed that there was no correlation exists among mite mortality, geographical location and source of isolation (host). Furthermore, Bb6 isolate caused the highest percentage of mycosis (88%) in mites cadavers. Because sporulation on the host has a key role in fungal spread in the field, isolate Bb6 which showed high percent mortality of T. truncatus and also produced high sporulation on dead insect can be used as a microbial pest control agent after field evaluation.
Conclusion
The local B. bassiana isolates were identified using morph-molecular approach and the population diversity was established through phylogenetic analysis of combined ITS+EF1-α + Bbchit1sequences and Bb6 isolate was found as virulent isolate which showed a significant pathogenicity to T. truncatus. Further studies are needed to determine the potentiality of Bb6 isolate under field conditions and their molecular bipartite interaction of B. bassiana and T. truncatus.
Data availability statement
The original contributions presented in the study are included in the article/Supplementary material; further inquiries can be directed to the corresponding author.
Author contributions
MC, TP, and DK conceived the review idea and plan. MC did the lab work and statistics and wrote the first draft. MC, TP, DK, LP, SS, and SNB finalize the outline and prepare schematics. LP helped with statistical data analysis and editing of the manuscript. SS and SNB helped in testing the pathogenicity of Beauveria bassiana against Tetranychus truncatus. All authors contributed to the article and approved the submitted version.
Acknowledgments
The authors are thankful to Gautam Chawla, Principal Scientist, Division of Nematology, ICAR-IARI, New Delhi, for capturing SEM photos.
Conflict of interest
The authors declare that the research was conducted in the absence of any commercial or financial relationships that could be construed as a potential conflict of interest.
Publisher’s note
All claims expressed in this article are solely those of the authors and do not necessarily represent those of their affiliated organizations, or those of the publisher, the editors and the reviewers. Any product that may be evaluated in this article, or claim that may be made by its manufacturer, is not guaranteed or endorsed by the publisher.
Supplementary material
The Supplementary material for this article can be found online at: https://www.frontiersin.org/articles/10.3389/fmicb.2022.1007017/full#supplementary-material
References
Abbott, W. S. (1925). Formula for correction of observed mortality due to natural mortality. Econ. Entomol. 18, 265–267. doi: 10.1093/jee/18.2.265a
Al Khoury, C., Guillot, J., and Nemer, N. (2019). Lethal activity of beauvericin, a Beauveria bassiana mycotoxin, against the two-spotted spider mites, Tetranychus urticae Koch. J. Appl. Entomol. 143, 974–983. doi: 10.1111/jen.12684
Al Khoury, C., Guillot, J., and Nemer, N. (2020). Susceptibility and development of resistance of the mite Tetranychus urticae to aerial conidia and blastospores of the entomopathogenic fungus Beauveria bassiana. Syst. Appl. Acarol. 25, 429–443. doi: 10.11158/saa.25.3.5
Boucias, D. G., and Pendland, J. C. (1998). “Insect pathogenic bacteria,” in Principles of Insect Pathology (Boston, MA: Springer), 177–216.
Castrillo, L. A., Vandenberg, J. D., and Wraight, S. P. (2003). Strain-specific detection of introduced Beauveria bassiana in agricultural fields by use of sequence-characterized amplified region markers. J. Invertebr. Pathol. 82, 75–83. doi: 10.1016/S0022-2011(02)00190-8
Chen, W. H., Liu, M., Huang, Z. X., Yang, G. M., Han, Y. F., Liang, J. D., et al. (2018). Beauveria majiangensis, a new entomopathogenic fungus from Guizhou, China. Phytotaxa 333, 243–250. doi: 10.11646/phytotaxa.333.2.8
Coates, B., Hellmich, R., and Lewis, L. (2002). Allelic variation of a Beauveria bassiana (Ascomycota: Hypocreales) minisatellite is independent of host range and geographic origin. Genome 45, 125–132. doi: 10.1139/g01-132
De la Rosa, W., Alatorre, R., Trujillo, J., and Barrera, J. F. (1997). Virulence of Beauveria bassiana (Deuteromycetes) strains against the coffee berry borer (Coleoptera: Scolytidae). J. Econ. Entomol. 90, 1534–1538. doi: 10.1093/jee/90.6.1534
Fang, W., Leng, B., Xiao, Y., Jin, K., Ma, J., Fan, Y., et al. (2005). Cloning of Beauveria bassiana chitinase gene Bbchit1 and its application to improve fungal strain virulence. Appl. Environ. Microbiol. 71, 363–370. doi: 10.1128/AEM.71.1.363-370.2005
Gatarayiha, M. C., Laing, M. D., and Miller, R. M. (2012). Selection of Beauveria bassiana strains against the two spotted spider mite, Tetranychus urticae Koch in laboratory and greenhouse trials. Afr. J. Microbiol. Res. 6, 2694–2703. doi: 10.5897/AJMR11.1184
Gillespie, J. P., Bailey, A. M., Cobb, B., and Vilcinskas, A. (2000). Fungi as elicitors of insect immune responses. Arch. Insect Biochem. Physiol. 44, 49–68. doi: 10.1002/1520-6327(200006)44:2<49::AID-ARCH1>3.0.CO;2-F
Glare, T. R., and Inwood, A. J. (1998). Morphological and genetic characterization of Beauveria spp. from New Zealand. Mycol. Res. 102, 250–256. doi: 10.1017/S0953756297005005
Hegedus, D. D., and Khachatourians, G. G. (1996). Identification and differentiation of the entomopathogenic fungus Beauveria bassiana using polymerase chain reaction and single-strand conformation polymorphism analysis. J. Invertebr. Pathol. 67, 289–299. doi: 10.1006/jipa.1996.0044
Hibbett, D. S., Ohman, A., Glotzer, D., Nuhn, M., Kirk, P., and Nilsson, R. H. (2011). Progress in molecular and morphological taxon discovery in fungi and options for formal classification of environmental sequences. Fungal Biol. Rev. 25, 38–47. doi: 10.1016/j.fbr.2011.01.001
Humber, R. (1997). “Fungi: identification,” in Manual of techniques in insect pathology. ed. L. A. Lacey (San Diego: Academic Press), 153–185.
Humber, R. (2012). “Identification of entomopathogenic fungi,” in Manual of techniques in invertebrate pathology. ed. L. Lacey. 2nd Edn. (San Diego: Academic Press).
Hyde, K., Abd-Elsalam, K., and Cai, L. (2010). Morphology: still essential in a molecular world. Mycotaxon 14, 439–451. doi: 10.5248/114.439
Imoulan, A., Ibnsouda, S. K., and Meziane, A. E. (2016). Molecular characterization and the effectiveness of native entomopathogenic Beauveria bassiana strains against adults of Mediterranean fruit fly (Ceratitis capitata). J. Biosci. Biotech. Disc. 1, 6–16. doi: 10.31248/JBBD2016.008
Inglis, G. D., Ivie, T. J., Duke, G. M., and Goettel, M. S. (2001). Influence of rain and conidial formulation on persistence of Beauveria bassiana on potato leaves and Colorado potato beetle larvae. Biol. Control 18, 55–64. doi: 10.1006/bcon.1999.0806
Khemika, S., Tawee, D., Paranya, C., and Meyer, C. R. (2006). Cloning and Expression of Chitinase Gene isolated from insect pathogenic fungi, Beauveria bassiana in Escherichia coli. Chiang Mai. J. Sci. 33, 347–355.
Jaber, L. R., and Ownley, B. H. (2018). Can we use entomopathogenic fungi as endophytes for dual biological control of insect pests and plant pathogens? Biol. Control 116, 36–45. doi: 10.1016/j.biocontrol.2017.01.018
Kramer, K. J., Corpuz, L., Choi, H. K., and Muthukrishnan, S. (1993). Sequence of a cDNA and expression of the gene encoding epidermal and gut chitinases of Manduca sexta. Insect Biochem. Mol. Biol. 23, 691–701. doi: 10.1016/0965-1748(93)90043-R
Moret, Y., and Moreau, J. (2012). The immune role of the arthropod exoskeleton. Invert. Surviv. J. 9, 200–206.
Murad, A. M., Laumann, R. A., Mehta, A., Noronha, E. F., and Franco, O. L. (2007). Screening and secretomic analysis of entomopathogenic Beauveria bassiana isolates in response to cowpea weevil (Callosobruchus maculatus) exoskeleton. Comp. Biochem. Physiol. Part - C: Toxicol. 145, 333–338. doi: 10.1016/j.cbpc.2007.01.010
Pallavi, B. N. (2004). Development of biocontrol agents for the control of pests in agriculture using chitin metabolism as target. [Ph.D Thesis]. [Pune]: Division of Biochemical Sciences. National Chemical Laboratory. Pune.
Pang, B. P., Zhou, X. R., Shi, L., and Mu, H. B. (2004). Performance of Tetranychus truncatus Ehara (Acarina: Tetranychidae) reared on different host plants. Acta Entomol. Sin. 47, 55–58.
Paz, A., Gonzalez, M., and Crawford, A. J. (2011). Codigos de barras de la vida: introduccion y perspectiva. Acta biologica colombiana 16, 161–176.
Posada, D., and Crandall, K. A. (1998). Model test: testing the model of DNA substitution. Bioinformatics (Oxford, England) 14, 817–818. doi: 10.1093/bioinformatics/14.9.817
Rehner, S. A., and Buckley, E. (2005). A Beauveria phylogeny inferred from nuclear ITS and EF1-α sequences: evidence for cryptic diversification and links to Cordyceps teleomorphs. Mycologia 97, 84–98. doi: 10.3852/mycologia.97.1.84
Robene-Soustrade, I., Jouen, E., Pastou, D., Payet-Hoarau, M., Goble, T., Linderme, D., et al. (2015). Description and phylogenetic placement of Beauveria hoplocheli sp. nov. used in the biological control of the sugarcane white grub, Hoplochelus marginalis, on Reunion Island. Mycologia 107, 1221–1232. doi: 10.3852/14-344
Roberts, D. W., and St Leger, R. J. (2004). Metarhizium spp., cosmopolitan insect-pathogenic fungi: mycological aspects. Adv. Appl. Microbiol. 54, 1–70. doi: 10.1016/S0065-2164(04)54001-7
Sakunwarin, S., Chandrapatya, A., and Baker, G. T. (2003). Biology and life table of the cassava mite, Tetranychus truncatus Ehara (Acari: Tetranychidae). Syst Appl Acarol. 8, 13–24. doi: 10.11158/saa.8.1.2
Sasidharan, K. R., and Varma, R. V. (2005). Laboratory evaluation of Beauveria bassiana (Balsamo) Vuillemin against Indarbela quadrinotata Walker (Lepidoptera: Metarbelidae)-a key pest of Casuarina equisetifolia L. in Tamil Nadu. J. Biol. Control. 19, 197–200.
Sevim, A., Demir, I., and Demirbag, Z. (2010a). a. Molecular characterization and virulence of Beauveria spp. from the pine processionary moth, Thaumetopoea pityocampa (Lepidoptera: Thaumetopoeidae). Mycopathologia 170, 269–277. doi: 10.1007/s11046-010-9321-6
Sevim, A., Demir, I., Hofte, M., Humber, R. A., and Demirbag, Z. (2010b). Isolation and characterization of entomopathogenic fungi from hazelnut-growing region of Turkey. Biol. 55, 279–297. doi: 10.1007/s10526-009-9235-8
Shi, W. B., and Feng, M. G. (2004). Lethal effect of Beauveria bassiana, Metarhizium anisopliae, and Paecilomyces fumosoroseus on the eggs of Tetranychus cinnabarinus (Acari: Tetranychidae) with a description of a mite egg bioassay system. Biol. Control 30, 165–173. doi: 10.1016/j.biocontrol.2004.01.017
Stadler, M., Lambert, C., Wibberg, D., Kalinowski, J., Cox, R. J., Kolarik, M., et al. (2020). Intragenomic polymorphisms in the ITS region of high-quality genomes of the Hypoxylaceae (Xylariales, Ascomycota). Mycol. Prog. 19, 235–245. doi: 10.1007/s11557-019-01552-9
Swofford, D. L. (2002). PAUP: Phylogenetic analysis using parsimony and other methods, version 4.0 Beta 10. Sinauer Associates, Sunderland.
Ullah, M. S., and Gotoh, T. (2013). Laboratory-based toxicity of some acaricides to Tetranychus macfarlanei and Tetranychus truncatus (Acari: Tetranychidae). Int. J. Acarol. 39, 244–251. doi: 10.1080/01647954.2012.758655
Yanar, D., Yanar, Y., Belgüzar, S., Eser, I., and Unalan, H. K. (2018). Efficacy of entomopathogenic fungus Beauveria bassiana isolates against the two-spotted spider mite, Tetranychus urticae Koch (Acari: Tetranychidae). Appl. Ecol. Environ. Res. 16, 7903–7911. doi: 10.15666/aeer/1606_79037911
Yun, H. G., Kim, D. J., Lee, J. H., Ma, J. I., Gwak, W. S., and Woo, S. D. (2017). Comparative evaluation of conidia, blastospores and culture filtrates from entomopathogenic fungi against Tetranychus urticae. Int. J. Indust. Entomol. 35, 58–62.
Keywords: Beauveria bassiana, morphological characteristics, molecular identification, multigene phylogenetics diversity, Tetranychus truncatus
Citation: Chaithra M, Prameeladevi T, Bhagyasree SN, Prasad L, Subramanian S and Kamil D (2022) “Multilocus sequence analysis for population diversity of indigenous entomopathogenic fungus Beauveria bassiana and its bio-efficacy against the cassava mite, Tetranychus truncatus Ehara (Acari: Tetranychidae)”. Front. Microbiol. 13:1007017. doi: 10.3389/fmicb.2022.1007017
Edited by:
Spyridon Ntougias, Democritus University of Thrace, GreeceReviewed by:
Abhishek Katoch, Chandigarh University, IndiaKgabo Martha Pofu, University of Limpopo, South Africa
Nabil Michel Nemer, Holy Spirit University of Kaslik, Lebanon
Copyright © 2022 Chaithra, Prameeladevi, Bhagyasree, Prasad, Subramanian and Kamil. This is an open-access article distributed under the terms of the Creative Commons Attribution License (CC BY). The use, distribution or reproduction in other forums is permitted, provided the original author(s) and the copyright owner(s) are credited and that the original publication in this journal is cited, in accordance with accepted academic practice. No use, distribution or reproduction is permitted which does not comply with these terms.
*Correspondence: Deeba Kamil, ZGVlYmFrYW1pbEBnbWFpbC5jb20=