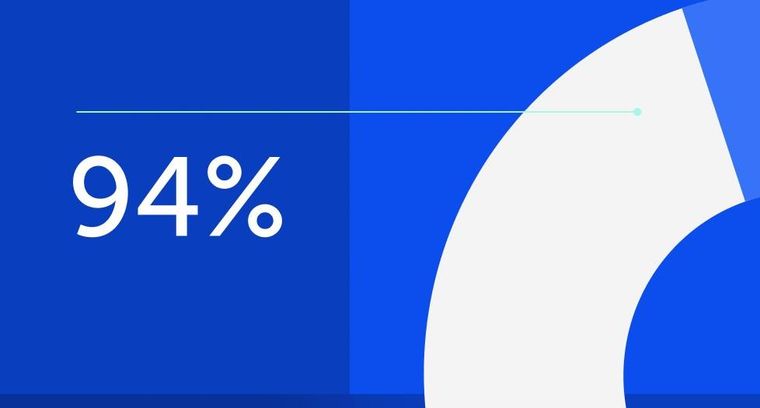
94% of researchers rate our articles as excellent or good
Learn more about the work of our research integrity team to safeguard the quality of each article we publish.
Find out more
ORIGINAL RESEARCH article
Front. Microbiol., 26 September 2022
Sec. Microbe and Virus Interactions with Plants
Volume 13 - 2022 | https://doi.org/10.3389/fmicb.2022.1002459
This article is part of the Research TopicSoil Microorganisms Under Ecological PlantingView all 14 articles
Multiple agricultural practices are being applied to increase crop yield in order to overcome the food shortage. Green manure has emerged as an appropriate practice to improve soil fertility and crop yield. However, the potential functions of arbuscular mycorrhizal fungi (AMF) in the below-ground ecosystems following the application of green manure in Ultisols remain largely unexplored. In this study, qPCR and high-throughput sequencing were used to investigate the response of AMF abundance and communities in different treatment groups, i.e., control (without fertilization), mineral fertilization (NPK), mineral fertilization with returning peanut straw (NPKS), and with green manure (hairy vetch; NPKG). The NPKG treatment significantly increased soil fertility compared to other treatment groups. Compared with control, the NPK, NPKS, and NPKG treatments increased peanut yield by 12.3, 13.1, and 25.4%, respectively. NPKS and NPKG treatments significantly altered the AMF community composition decreased the AMF diversity and increased AMF abundance compared to the control. The AMF network of the NPKG treatment group showed the highest complexity and stability compared to other treatment groups. The structural equation modeling revealed that the application of hairy vetch improved soil nutrients and peanut yield by increasing the soil AMF abundance and network stability. Overall, the results suggested that the application of hairy vetch might trigger positive feedback between the peanut and AMF community, contributing to fertility and yield improvement in the dryland of Ultisol.
Ultisol, one of the most important soil types, occupies approximately 21% land area of China. Over time, high-strength and long-term farming in Ultisol have adversely impacted the ecosystem in the form of soil acidification, fertility depletion, and a decline in crop yield (Xiang et al., 2020). However, the use of organic materials (e.g., animal excreta) in agriculture practices effectively alleviates these adverse effects. The application of animal excreta to agricultural soil increased soil fertility and suppressed plant pathogens (Shahbaz et al., 2017). However, deleterious effects of animal excreta application, such as the enrichment of heavy metals and residual antibiotics have gained increasing attention (Sager, 2007; Yan et al., 2022). Thus, suitable and sustainable agricultural practice in Ultisol demands urgent investigation.
Crop straw and green manure are important renewable organic resources (Zhou et al., 2019). Maize and rice straw returning improved soil nitrogen content (Akhtar et al., 2018) and contributed to the accumulation of soil organic carbon (Lee et al., 2020; Dutta et al., 2022). However, the direct return of peanut straw to the field might result in soil-borne diseases and obstacles in continuous cropping (Li et al., 2014). Thus, the effects of returning composted peanut straw to the agroecosystem should be investigated further. Peanuts are planted in mid-late April and harvested at the end of August in South China. Monoculture is commonly practiced in Ultisols due to severe seasonal drought. The 8-month peanut planting interval provides enough time and space for planting green manure (Xiang et al., 2021). Planting green manure triggered long-term effects such as improving soil quality, and immediate effects such as the increasing supply of available nutrients for subsequent crops (Mohanty et al., 2020; Ansari et al., 2022).
Soil microbes are involved in several functions in agroecosystems, such as nutrient availability, pathogen control, and resilience to abiotic stresses (de Vries et al., 2020; Chen et al., 2021). The application of crop straw and green manure to the field triggered changes in soil microbial diversity and biomass (Xiang et al., 2021). Besides, the application of green manure improved soil fertility and crop production by activating the functions of below-ground soil microbes (Khan et al., 2020). Recently, microbial network analysis was particularly useful to statistically identify the key microbial taxa that regulate the microbial functions in the below-ground ecosystem (Banerjee et al., 2018; Yuan et al., 2021). However, few studies have explored key taxa involved in the regulation of agroecosystem using network analysis following returning crop straw and planting green manure.
Arbuscular mycorrhizal fungi (AMF) are present in the roots of plants and live in mutualistic symbiosis with most plants. AMF also improved the absorption of nutrients for plants in exchange for carbohydrates (Smith and Read, 2008). In addition, AMF improved the soil structure and increased ecosystem functions by decreasing nutrient loss from leaching (van der Heijden, 2010; Martin et al., 2012). Mineral fertilization directly increased soil fertility to decrease the dependence of plants on their AMF partners. This phenomenon might shift the relationship between the plants and AMF from mutualism to parasitism (Kiers et al., 2011). However, the previous study has shown that the addition of pig manure increased the AMF biomass (Jiang et al., 2020) and richness (Liu et al., 2020). However, the response of the AMF communities to the application of green manure and crop straw remains largely unknown in the Ultisol.
In this study, we evaluate the effects of green manure and peanut straw on soil fertility, crop yield, and AMF communities in the dryland of Ultisol using qPCR and sequencing (Illumina MiSeq). Hairy vetch (Vicia villosa Roth L.) was selected as the experimental green manure. Peanut straw was composted for 8 months and then returned to the field. This study aimed to investigate the effects of (a) composted straw and green manure on soil fertility and peanut yield, (b) composted straw and green manure on the AMF communities and key taxa within the AMF network, and (c) feedback among AMF, soil fertility, and peanut yield following different agricultural practices in Ultisol.
The experimental site is located at the Comprehensive Experimental Station of red soil (28°10′59″N, 106°35′11″E, 50 m), Dongxiang County, Jiangxi Province, China. The soil type was classified as Ultisol. This region experiences a typical subtropical warm and humid monsoon climate. The annual average temperature and precipitation are 18°C and 2,180 mm, respectively. This experiment was established in 2014. The soil properties before the experiments were as follows: 5.34 pH, 7.69 g kg–1 organic C, 1.02 g kg–1 total N, 85.9 mg kg–1 alkaline N, 0.68 g kg–1 total P, and 12.7 mg kg–1 available P.
The experiments included four treatment groups with six replicates, i.e., (a) without fertilizer (control), (b) mineral fertilization (NPK), (c) mineral fertilization with returning composted peanut straw (NPKS), and (d) mineral fertilization with planting green manure (NPKG). Peanut straw was removed in the control, NPK, and NPKG treatment groups. Composted peanut straw was plowed into the soil 2 weeks before the next peanut planting. Dry straw is about 1,300 kg ha–1yr–1 in NPKS treatment. Green manure was applied at a seeding rate of 20 kg ha–1 in mid-October every year with a yield of around 2 × 104 kg ha–1yr–1 in NPKG treatment. The green manure was cut into 5 cm small pieces and plowed into the soil 2 weeks before the next peanut planting. The field site was used to cultivate peanuts (cv. Yueyou 256). Peanuts were sown in a row at a spacing of 40 cm and a whole spacing of 20 cm. Two seeds were planted in each cave, and the planting density was about 1.25 × 105 points hm–2. The peanut seedlings were planted in mid-April, and they ripened in mid to late August.
The application rates of mineral fertilizers to peanuts were: 135 kg ha–1 urea (containing 46.4% N), 81 kg ha–1 calcium superphosphate (containing 12.0% P2O5), and 135 kg ha–1 potassium chloride (containing 60.0% K2O). The 50% of N and K fertilizers were broadcasted as basal application, while the other 50% was applied at the peanut needle stage. All P fertilizers were applied as basal fertilizers. Basal fertilizer was thoroughly incorporated into the soil by plowing one day before the peanut plantation.
Rhizospheric soils were sampled at the pod stage on 15 July 2017. One composite rhizospheric soil sample was taken from each plot consisting of five randomly selected peanut plants. After gently pulling out the peanuts, we tap the entire root system to dislodge all the loose soil and the remaining soil was collected as a rhizospheric soil sample (Smalla et al., 2001).
The soil water suspension (1:2.5 wt/volume) was shaken for 30 min, and then the pH of the soil water solution was determined. The potassium dichromate oxidation method was used to determine soil organic carbon (SOC). Total nitrogen (TN) was determined using an elemental analyzer (multi-EA 5,000, Jean, Germany). The molybdenum blue colorimetry method was used for measuring total phosphorus (TP) (Bowman, 1988). Alkaline nitrogen (AN) (Ai et al., 2017) was determined using the alkaline hydrolysis diffusion method. Available phosphorus (AP) (Stahlberg, 1980) was extracted using sodium bicarbonate and AP content was determined using the molybdenum blue colorimetric method.
The soil DNA was extracted from 0.5 g of soil using the Fast DNA® SPIN Kit (MP Biomedicals, Santa Ana, CA, USA) according to the manufacturer’s instructions.
Primer pair AMV4.5NF/AMDGR were used to determine AMF abundance by CFX96 Optical Real-Time Detection System (Bio-Rad, Laboratories Inc., Hercules, CA, USA). Each PCR reaction was carried out in a 25 μl qPCR reaction mixture containing 12.5 μl SYBR®Premix Ex Taq (TliRNaseH Plus, 2×, Takara Bio, Japan), 0.5 μl PCR forward and reverse primers (both 10 μM), 2 μl DNA template, and 9.5 μl double distilled water (ddH2O). Quantitative real-time PCR reactions were set to 95°C for 5 min, followed by 35 cycles of 95°C for 30 s, 58°C for 30 s, and 72°C for 1 min. The melting curve analysis showed excellent specificity of the qPCR with an efficiency of 98.5% (R2 = 0.9997).
The PCR was performed in a 50-μL reaction mixture and the mixture contained a concentration of 1.25 mM deoxynucleoside triphosphate, 15 μM of forward and reverse primers (i.e., AMV4.5NF/AMDGR) in each, 2 U of Taq DNA polymerase (TaKaRa, Japan). Each PCR reaction mixture contained 1 μl of DNA as a template. The cycling parameters were as follows: 35 cycles of 95°C for 45 s, 58°C for 45 s, and 72°C for 1 min, with a final extension at 72°C for 10 min.
Raw data were processed using qiime2-2020.2 (Bolyen et al., 2019). The deblur algorithm was used to filter poor-quality sequences (Amir et al., 2017). Then, the chimeras of amplicon sequence variants (ASVs) were filtered using vsearch (Rognes et al., 2016). All singleton ASVs were removed. Annotations for ASV were conducted by classify-sklearn using the MaarjAM database (Öpik et al., 2010). A subset of 21,000 sequences was randomly selected per sample for further analysis.
The differences in the AMF index (i.e., AMF abundance, AMF richness, and the dominant taxa) and soil properties among different treatments were tested using one-way ANOVA. Canonical analysis of principal coordinates (CAP), ANOSIM, and RDA analyses were conducted using the R v.4.0.4 software. The primary factors affecting AMF abundance, diversity, and relative abundance of dominant taxa were determined using the Random Forest model (Trivedi et al., 2016). The differences in AMF species were detected using STAMP 2.1.3 software among treatment groups. The co-occurrence network was performed using high-frequency ASVs (>3 samples) with robust (Spearman’s r > 0.6 or <−0.6), and significant (P < 0.05) Benjamini–Hochberg multiple testing correction. Topological features (i.e., average degree, natural connectivity, and the average clustering coefficient) were determined using the igraph package in R v.4.0.4 software. The structural equation modeling (SEM) was performed using the lavaan package in R v.4.0.4 software to assess the direct and indirect contributions of abiotic (soil nutrient) and biotic (AMF index) variables to peanut yield.
Different agricultural practices triggered significant changes in the soil properties (Table 1). The soil pH in NPKS and NPKG treatment groups was decreased to 4.97 and 4.71, respectively, compared to control (pH 5.21). Besides, the NPKG treatment significantly increased soil fertility levels. Compared to control, peanut yield was significantly increased by 12.3% in NPK, 13.1% in NPKS, and 25.4% in NPKG (Table 1).
The NPKS and NPKG treatments increased AMF abundance by 10.9 times and 12.2 times, respectively, while NPK treatment had little effect on the AMF abundance compared to the control (Figure 1A). The AMF abundance was significantly correlated with the soil pH, SOC, and AN (Supplementary Figure 1). Compared with the control, the NPK, NPKS, and NPKG treatments significantly decreased the AMF richness, and the lowest AMF richness was observed in the NPKG treatment group (Figure 1B). The ASV richness was significantly correlated with the soil pH and soil nutrient contents (Supplementary Figure 1).
Figure 1. The variation of arbuscular mycorrhizal fungi (AMF) abundance (A) and ASV richness (B) in the soil among different treatments. The bottom and top of the box denote the first and third quartiles; the band inside the box denotes the median. Letters represent significant differences from the one-way ANOVA with Tukey’s HSD comparisons (P < 0.05).
The organic application significantly altered the soil AMF community composition. However, mineral fertilization had little impact on the AMF community composition (Figure 2 and Supplementary Figure 2). The shifts in the AMF community composition were driven by practice-mediated changes in the soil pH and nutrients (Figure 2). At the order level, compared to control, the NPK and NPKG treatments decreased and increased the relative abundance of Glomerales, respectively (Supplementary Figure 3). The NPKG treatment decreased the relative abundances of Diversisporales and Paraglomerales compared to the control (Supplementary Figure 3). At the family level, compared to the control, the NPKS and NPKG treatments significantly increased the relative abundance of Glomeraceae and decreased the relative abundance of Claroideoglomeraceae. The relative abundances of Acaulosporaceae and Gigasporaceae were significantly increased in the NPK treatment group and decreased in the NPKG treatment group compared to the control (Supplementary Figure 4). The STAMP analysis showed that NPKS treatment significantly decreased the relative abundance of Claroideoglomus, and NPKG treatment significantly increased the relative abundance of Glomus and decreased the relative abundance of Claroideoglomus and Acaulospora (Supplementary Figure 5).
Figure 2. Redundancy analysis (RDA) plot showing AMF community composition across different treatments. Differences in the soil AMF community composition among different treatments were determined using Analyses of Similarities (ANOSIM). The explanation rate for each factor is placed in the upper left corner. SOC, soil organic carbon; TN, total nitrogen; AN, alkaline nitrogen; AP, available phosphorus; and TP, total phosphorus. **P < 0.01.
The soil AMF network was investigated for each agricultural practice separately in this study (Figure 3). The lower number of nodes (e.g., taxa) and edges (interactions among taxa) were found in the networks of control, NPK, and NPKS treatment groups. The network of NPKG consisted of a higher number of nodes and edges. The average degree and the average clustering coefficient in the NPKG network were also considerably higher than in the other three networks. The NPKG network exhibited higher robustness and stability than the other three networks (Supplementary Figure 6). Furthermore, the topological characteristics of the sub-network within Glomeraceae were also significantly higher in the NPKG treatment group than in other treatment groups (Supplementary Figure 7).
Figure 3. Co-occurrence AMF network for each treatment. Each node in the figure represents an amplicon sequence variant (ASV), and the size of the point represents the degree of the node.
The SEM analysis demonstrated that the application of green manure was associated with a higher AMF abundance, network complexity, and relative abundance of Glomus (Figure 4). Specifically, planting green manure improved the soil nutrient level indirectly by increasing the AMF abundance and the relative abundance of Glomus. Soil nutrients, AMF network complexity, and relative abundance of Glomus showed a significant and positive correlation with the peanut yield (Figure 4).
Figure 4. The structural equation model shows the relationships among AMF index, soil nutrient, and crop yield. The solid line represents a significant relationship, and the dotted line represents an insignificant relationship. *, **, and *** in the figure represents significance at the probability of 0.05, 0.01, and 0.001 level, respectively. All variances attributable to the constrained factor and the significance of the factor are portrayed at the bottom of the plot.
Previous studies have shown that the application of green manure improved fertility and yield (Mohanty et al., 2020; Ansari et al., 2022; Table 1). In this study, shifts in certain AMF taxa might be crucial to improving the yield in addition to increasing soil fertility following the application of hairy vetch (Bonanomi et al., 2020). Soil AMFs are beneficial for the absorption of soil nutrients (de Gruyter et al., 2022). The Glomeraceae showed the highest P uptake efficiency and antagonistic activities against plant pathogens (Kaur et al., 2022). However, Gigasporaceae showed a negative impact on the plant growth potential (Li et al., 2008). In this study, the application of hairy vetch increased the relative abundance of Glomeraceae and decreased the relative abundance of Gigasporaceae (Supplementary Figure 4). These results indicated that the application of hairy vetch might benefit the agricultural ecosystem by optimizing soil AMF taxa and regulating below-ground AMF functions.
The AMF could recruit soil saprotrophs to decompose organic matter following organic amendment (Xiang et al., 2015; Frey, 2019; Wang et al., 2021). Especially, the AMF genus Glomus might act as a conduit to transport plant-derived monosaccharides from the roots to the soil aggregates (Johnson et al., 2002; Jeewani et al., 2021), which might regulate soil saprotrophs for the transformation of organic matters. In this study, the application of hairy vetch significantly increased the relative abundance of Glomus (Supplementary Figure 5). This demonstrates that certain beneficial taxa (i.e., Glomus) might accelerate organic degradation to increase mineral fertilizers levels following the application of hairy vetch (Table 1).
Previous studies have shown the negative response of AMF diversity to nutrient enrichment (Alguacil et al., 2010; Camenzind et al., 2014; Figure 1B and Supplementary Figure 1). However, the decreased microbial diversity did not completely reflect the loss of ecological functions due to the functional redundancy of the microbes (Louca et al., 2018; Frew et al., 2022). Moreover, previous studies have shown that the primary driver of soil multifunctionality was microbial biomass/abundance rather than microbial diversity (Garland et al., 2021; Wang et al., 2021; Frew et al., 2022). In line with the previous studies, planting hairy vetch significantly increased the soil AMF abundance (Jiang et al., 2021; Figure 1A). A higher AMF abundance could result in the degradation of organic matter to release mineral nutrients for plants (Treseder and Cross, 2006; Figure 4).
The soil microbial networks reveal the microbial structure and their response to environmental fluctuations (Wagg et al., 2019; Yuan et al., 2021). This study showed that the application of hairy vetch had a significantly more intricate network than other treatments (Figure 3). Previous studies have shown that the networks with more sophisticated architecture were more stable to environmental fluctuations and might support higher ecosystem multifunctionality (Wagg et al., 2019). Thus, the application of hairy vetch might increase the complexity of the soil AMF network to strengthen below-ground multifunctionality, contributing to nutrient level and crop productivity.
In this study, the effects of returning composted straw on the soil nutrients and peanut yields were not remarkable compared to the application of hairy vetch (Table 1). The peanut straw showed a higher carbon content and lower nitrogen and phosphorus contents (Zhou et al., 2019). More nutrient loss occurred after nearly 8 months of composting. Thus, returning composted straw might not be an appropriate practice in the dryland of Ultisol. Besides, the application of the hairy vetch increased AMF abundance and optimized AMF community structure to increase soil nutrients and peanut yields (Figures 1–4 and Table 1). However, the results of this study also demonstrated that the soil pH decreased sharply following planting green manure. Thus, attention should be paid to the negative effect of soil acidification following the long-term application of green manure.
This study demonstrated the differential feedback between peanut and their AMF partners in different agricultural practices. Planting hairy vetch significantly increased the AMF abundance, and network complexity, and optimized soil AMF taxa, contributing to the nutrient and yield improvement in the dryland of Ultisol. The application of hairy vetch might trigger positive feedback to strengthen the mutualism between crops and their AMF partners in the agroecosystem. In addition, returning peanut straw, without increasing nutrient levels but triggering soil acidification, might be an inappropriate practice in Ultisol. Overall, this study added to a growing body of knowledge on underlying feedback between plants and their AMF partners following different practices in Ultisol. However, there are certain limitations to this study. The AMF infection rate which could evaluate the feedback between plant and AMF was not measured. As the application of leguminous green manure increased the soil nitrogen content, we did not clarify the role of microbes within nitrogen cycling following the application of hairy vetch. These limitations should be addressed in future studies.
The datasets presented in this study can be found in online repositories. The names of the repository/repositories and accession number(s) can be found in the article/Supplementary material.
JZ, JL, and XX designed the experiments. JZ, GL, KL, LS, WQ, and CP completed the field sampling. JZ, GL, and XX performed the data analysis and prepared the figures. JZ and GL wrote the manuscript. YJ, CX, JL, and XX contributed to the revision of manuscript. All authors contributed to the article and approved the submitted version.
This work was supported by the National Key Research and Development Program of China (No. 2021YFD1901201-05), China Agriculture Research System of MOF and MARA (No. CARS-22), the National Natural Science Foundation of China (Nos. 31801989, 41661052, and 32060333), the Natural Science Foundation of Anhui Province (No. 2008085MC62), and Anhui University Scientific Research Foundation (No. S020118002/038).
We thank Miss Lele Jin from Anhui University for her help in data analysis.
The authors declare that the research was conducted in the absence of any commercial or financial relationships that could be construed as a potential conflict of interest.
The reviewer PL was currently organizing a research topic with one of the author JL.
All claims expressed in this article are solely those of the authors and do not necessarily represent those of their affiliated organizations, or those of the publisher, the editors and the reviewers. Any product that may be evaluated in this article, or claim that may be made by its manufacturer, is not guaranteed or endorsed by the publisher.
The Supplementary Material for this article can be found online at: https://www.frontiersin.org/articles/10.3389/fmicb.2022.1002459/full#supplementary-material
Ai, Z. M., He, L. R., Xin, Q., Yang, T., Liu, G. B., and Xue, S. (2017). Slope aspect affects the nonstructural carbohydrates and C: N: P stoichiometry of Artemisia sacrorum on the Loess Plateau in China. Catena 152, 9–17. doi: 10.1016/j.catena.2016.12.024
Akhtar, K., Wang, W. Y., Ren, G. X., Khan, A., Feng, Y. Z., and Yang, G. H. (2018). Changes in soil enzymes, soil properties, and maize crop productivity under wheat straw mulching in Guanzhong, China. Soil Till. Res. 182, 94–102. doi: 10.1016/j.still.2018.05.007
Alguacil, M. M., Lozano, Z., Campoy, M. J., and Roldán, A. (2010). Phosphorus fertilization management modifies the biodiversity of AM fungi in a tropical savanna forage system. Soil Biol. Biochem. 42, 1114–1122. doi: 10.1016/j.soilbio.2010.03.012
Amir, A., McDonald, D., Navas-Molina, J. A., Kopylova, E., Morton, J. T., Zech, et al. (2017). Deblur rapidly resolves single-nucleotide community sequence patterns. mSystems 2, e191–e116. doi: 10.1128/mSystems.00191-16
Ansari, M. A., Choudhury, B. U., Layek, J., Das, A., Lal, R., and Mishra, V. K. (2022). Green manuring and crop residue management: Effect on soil organic carbon stock, aggregation, and system productivity in the foothills of Eastern Himalaya (India). Soil Till. Res. 218:105318. doi: 10.1016/j.still.2022.105318
Banerjee, S., Schlaeppi, K., and van der Heijden, M. G. A. (2018). Keystone taxa as drivers of microbiome structure and functioning. Nat. Rev. Microbiol. 16, 567–576. doi: 10.1038/s41579-018-0024-1
Bolyen, E., Rideout, J. R., Dillon, M. R., Bokulich, N. A., Abnet, C. C., Caporaso, J. G., et al. (2019). Reproducible, interactive, scalable and extensible microbiome data science using QIIME 2. Nat. Biotechnol. 37, 852–857. doi: 10.1038/s41587-019-0209-9
Bonanomi, G., De Filippis, F., Zotti, M., Idbella, M., Cesarano, G., Abd-ElGawad, A., et al. (2020). Repeated applications of organic amendments promote beneficial microbiota, improve soil fertility and increase crop yield. Appl. Soil Ecol. 156:103714. doi: 10.1016/j.apsoil.2020.103714
Bowman, R. A. (1988). A rapid method to determine total phosphorus in soils. Soil Sci. Soc. Am. J. 52, 1301–1304. doi: 10.2136/sssaj1988.03615995005200050016x
Camenzind, T., Hempel, S., Homeier, J., Horn, S., Velescu, A., Rillig, M. C., et al. (2014). Nitrogen and phosphorus additions impact arbuscular mycorrhizal abundance and molecular diversity in a tropical montane forest. Glob. Change Biol. 20, 3646–3659. doi: 10.1111/gcb.12618
Chen, Q. L., Hu, H. W., He, Z. Y., Cui, L., Zhu, Y. G., and He, J. Z. (2021). Potential of indigenous crop microbiomes for sustainable agriculture. Nat. Food 2, 233–240. doi: 10.1038/s43016-021-00253-5
de Gruyter, J., Weedon, J. T., Elst, E. M., Geisen, S., van der Heijden, M. G., and Verbruggen, E. (2022). Arbuscular mycorrhizal inoculation and plant response strongly shape bacterial and eukaryotic soil community trajectories. Soil Biol. Biochem. 165:108524. doi: 10.1016/j.soilbio.2021.108524
de Vries, F. T., Griffiths, R. I., Knight, C. G., Nicolitch, O., and Williams, A. (2020). Harnessing rhizosphere microbiomes for drought-resilient crop production. Science 368, 270–274. doi: 10.1126/science.aaz5192
Dutta, A., Bhattacharyya, R., Chaudhary, V. P., Sharma, C., Nath, C. P., Parmar, B., et al. (2022). Impact of long-term residue burning versus retention on soil organic carbon sequestration under a rice-wheat cropping system. Soil Till. Res. 221:105421. doi: 10.1016/j.still.2022.105421
Frew, A., Antunes, P. M., Cameron, D. D., Hartley, S. E., Johnson, S. N., Bennett, A. E., et al. (2022). Plant herbivore protection by arbuscular mycorrhizas: A role for fungal diversity? New Phytol. 233, 1022–1031. doi: 10.32942/osf.io/g6c3j
Frey, S. D. (2019). Mycorrhizal fungi as mediators of soil organic matter dynamics. Annu. Rev. Ecol. Evol. S. 50, 237–259. doi: 10.1146/annurev-ecolsys-110617-062331
Garland, G., Edlinger, A., Banerjee, S., Degrune, F., Rillig, M. C., van der Heijden, M. G. A., et al. (2021). Crop cover is more important than rotational diversity for soil multifunctionality and cereal yields in European cropping systems. Nat. Food 2, 28–37. doi: 10.1038/s43016-020-00210-8
Jeewani, P. H., Luo, Y., Yu, G. H., Fu, Y. Y., He, X. H., Xu, J. M., et al. (2021). Arbuscular mycorrhizal fungi and goethite promote carbon sequestration via hyphal-aggregate mineral interactions. Soil Biol. Biochem. 162:108417. doi: 10.1016/j.soilbio.2021.108417
Jiang, S. T., An, X. R., Shao, Y. D., Kang, Y. L., Chen, T. S., Shen, Q. R., et al. (2021). Responses of Arbuscular Mycorrhizal Fungi Occurrence to Organic Fertilizer: A meta-analysis of field studies. Plant Soil 469, 89–105. doi: 10.1007/s11104-021-05153-y
Jiang, Y. J., Luan, L., Hu, K. J., Liu, M. Q., Chen, Z. Y., Sun, B., et al. (2020). Trophic interactions as determinants of the arbuscular mycorrhizal fungal community with cascading plant-promoting consequences. Microbiome 8, 1–14. doi: 10.1186/s40168-020-00918-6
Johnson, D., Leake, J. R., Ostle, N., Ineson, P., and Read, D. J. (2002). In situ13CO2 pulse-labelling of upland grassland demonstrates a rapid pathway of carbon flux from arbuscular mycorrhizal mycelia to the soil. New Phytol. 153, 327–334. doi: 10.1046/j.0028-646X.2001.00316.x
Kaur, S., Campbell, B. J., and Suseela, V. (2022). Root metabolome of plant-arbuscular mycorrhizal symbiosis mirrors the mutualistic or parasitic mycorrhizal phenotype. New Phytol. 234, 672–687. doi: 10.1111/nph.17994
Khan, M. I., Gwon, H. S., Alam, M. A., Song, H. J., Das, S., and Kim, P. J. (2020). Short term effects of different green manure amendments on the composition of main microbial groups and microbial activity of a submerged rice cropping system. Appl. Soil Ecol. 147:103400. doi: 10.1016/j.apsoil.2019.103400
Kiers, E. T., Duhamel, M., Beesetty, Y., Mensah, J. A., Franken, O., Bucking, H., et al. (2011). Reciprocal rewards stabilize cooperation in the mycorrhizal symbiosis. Science 333, 880–882. doi: 10.1126/science.1208473
Lee, J. H., Lee, J. G., Jeong, S. T., Gwon, H. S., Kim, P. J., and Kim, G. W. (2020). Straw recycling in rice paddy: trade-off between greenhouse gas emission and soil carbon stock increase. Soil Till. Res. 199:104598. doi: 10.1016/j.still.2020.104598
Li, H. Y., Smith, F. A., Dickson, S., Holloway, R. E., and Smith, S. E. (2008). Plant growth depressions in arbuscular mycorrhizal symbioses: Not just caused by carbon drain? New Phytol. 178, 852–862. doi: 10.1111/j.1469-8137.2008.02410.x
Li, X. G., Ding, C. F., Zhang, T. L., and Wang, X. X. (2014). Fungal pathogen accumulation at the expense of plant-beneficial fungi as a consequence of consecutive peanut monoculturing. Soil Biol. Biochem. 72, 11–18. doi: 10.1016/j.soilbio.2014.01.019
Liu, J., Zhang, J., Li, D. M., Xu, C. X., and Xiang, X. J. (2020). Differential responses of arbuscular mycorrhizal fungal communities to mineral and organic fertilization. MicrobiologyOpen 9:e00920. doi: 10.1002/mbo3.920
Louca, S., Polz, M. F., Mazel, F., Albright, M. B., Huber, J. A., Parfrey, L. W., et al. (2018). Function and functional redundancy in microbial systems. Nat. Ecol. Evol. 2, 936–943. doi: 10.1038/s41559-018-0519-1
Martin, S. L., Mooney, S. J., Dickinson, M. J., and West, H. M. (2012). The effects of simultaneous root colonisation by three Glomus species on soil pore characteristics. Soil Biol. Biochem. 49, 167–173. doi: 10.1016/j.soilbio.2012.02.036
Mohanty, S., Nayak, A. K., Swain, C. K., Dhal, B. R., Kumar, A., and Behera, K. K. (2020). Impact of integrated nutrient management options on GHG emission, N loss and N use efficiency of low land rice. Soil Till. Res. 200:104616. doi: 10.1016/j.still.2020.104616
Öpik, M., Vanatoa, A., Vanatoa, E., Moora, M., Davison, J., Zobel, M., et al. (2010). The online database MaarjAM reveals global and ecosystemic distribution patterns in arbuscular mycorrhizal fungi (Glomeromycota). New Phytol. 188, 223–241. doi: 10.1111/j.1469-8137.2010.03334.x
Rognes, T., Flouri, T., Nichols, B., Quince, C., and Mahé, F. (2016). VSEARCH: a versatile open source tool for metagenomics. PeerJ 4:e2584. doi: 10.7717/peerj.2584
Sager, M. (2007). Trace and nutrient elements in manure, dung and compost samples in Austria. Soil Biol. Biochem. 39, 1383–1390. doi: 10.1016/j.soilbio.2006.12.015
Shahbaz, M., Kuzyakov, Y., Maqsood, S., Wendland, M., and Heitkamp, F. (2017). Decadal nitrogen fertilization decreases mineral-associated and subsoil carbon: A 32-year study. Land Degrad. Dev. 28, 1463–1472. doi: 10.1002/ldr.2667
Smalla, K., Wieland, G., Buchner, A., Zock, A., Parzy, J., Berg, G., et al. (2001). Bulk and rhizosphere soil bacterial communities studied by denaturing gradient gel electrophoresis: plant-dependent enrichment and seasonal shifts revealed. Appl. Environ. Microb. 67, 4742–4751. doi: 10.1128/AEM.67.10.4742-4751.2001
Stahlberg, S. (1980). A new extraction method for estimation of plant-available P, K and Mg: a trial application in Swedish cultivated soils. Acta Agr. Scand. 30, 93–107. doi: 10.1080/00015128009435700
Treseder, K. K., and Cross, A. (2006). Global distributions of arbuscular mycorrhizal fungi. Ecosystems 9, 305–316. doi: 10.1007/s10021-005-0110-x
Trivedi, P., Delgado-Baquerizo, M., Trivedi, C., Hu, H., Anderson, I. C., Singh, B. K., et al. (2016). Microbial regulation of the soil carbon cycle: Evidence from gene- enzyme relationships. ISME J. 10, 2593–2604. doi: 10.1038/ismej.2016.65
van der Heijden, M. G. A. (2010). Mycorrhizal fungi reduce nutrient loss from model grassland ecosystems. Ecology 91, 1163–1171. doi: 10.1890/09-0336.1
Wagg, C., Schlaeppi, K., Banerjee, S., Kuramae, E. E., and van der Heijden, M. G. (2019). Fungal-bacterial diversity and microbiome complexity predict ecosystem functioning. Nat. Commun. 10, 1–10. doi: 10.1038/s41467-019-12798-y
Wang, T., Duan, Y., Liu, G. D., Shang, X. W., Liu, L. F., Fang, W. P., et al. (2021). Tea plantation intercropping green manure enhances soil functional microbial abundance and multifunctionality resistance to drying-rewetting cycles. Sci. Total Environ. 15:151282. doi: 10.1016/j.scitotenv.2021.151282
Xiang, X. J., Adams, J. M., Qiu, C. F., Qin, W. J., Chen, J. R., Liu, J., et al. (2021). Nutrient improvement and soil acidification inducing contrary effects on bacterial community structure following application of hairy vetch (Vicia villosa Roth L.) in Ultisol. Agr. Ecosyst. Environ. 312:107348. doi: 10.1016/j.agee.2021.107348
Xiang, X. J., Gibbons, S. M., Yang, J., Kong, J. J., Sun, R. B., and Chu, H. Y. (2015). Arbuscular mycorrhizal fungal communities show low resistance and high resilience to wildfire disturbance. Plant Soil 397, 347–356. doi: 10.1007/s11104-015-2633-z
Xiang, X. J., Liu, J., Zhang, J., Li, D. M., Xu, C. X., and Kuzyakov, Y. (2020). Divergence in fungal abundance and community structure between soils under long-term mineral and organic fertilization. Soil Till. Res. 196:104491. doi: 10.1016/j.still.2019.104491
Yan, X. T., Zhai, Y. Q., Cai, Y. Y., Guo, Z., Zhang, Q. Q., and Ying, G. G. (2022). Hypothetical scenarios estimating and simulating the fate of antibiotics: Implications for antibiotic environmental pollution caused by manure application. Sci. Total Environ. 822:153177. doi: 10.1016/j.scitotenv.2022.153177
Yuan, M. M., Guo, X., Wu, L. W., Zhang, Y., Xiao, N. J., Zhou, J. Z., et al. (2021). Climate warming enhances microbial network complexity and stability. Nat. Clim. Change 11, 343–348. doi: 10.1038/s41558-021-00989-9
Keywords: AMF community, hairy vetch, soil fertility, peanut yield, sequencing
Citation: Xiang X, Zhang J, Li G, Leng K, Sun L, Qin W, Peng C, Xu C, Liu J and Jiang Y (2022) Positive feedback between peanut and arbuscular mycorrhizal fungi with the application of hairy vetch in Ultisol. Front. Microbiol. 13:1002459. doi: 10.3389/fmicb.2022.1002459
Received: 25 July 2022; Accepted: 29 August 2022;
Published: 26 September 2022.
Edited by:
Fayuan Wang, Qingdao University of Science and Technology, ChinaReviewed by:
Janusz Blaszkowski, West Pomeranian University of Technology, PolandCopyright © 2022 Xiang, Zhang, Li, Leng, Sun, Qin, Peng, Xu, Liu and Jiang. This is an open-access article distributed under the terms of the Creative Commons Attribution License (CC BY). The use, distribution or reproduction in other forums is permitted, provided the original author(s) and the copyright owner(s) are credited and that the original publication in this journal is cited, in accordance with accepted academic practice. No use, distribution or reproduction is permitted which does not comply with these terms.
*Correspondence: Jia Liu, bGl1amlhNDIyQDEyNi5jb20=; Yuji Jiang, eWpqaWFuZ0Bpc3Nhcy5hYy5jbg==
†These authors have contributed equally to this work
Disclaimer: All claims expressed in this article are solely those of the authors and do not necessarily represent those of their affiliated organizations, or those of the publisher, the editors and the reviewers. Any product that may be evaluated in this article or claim that may be made by its manufacturer is not guaranteed or endorsed by the publisher.
Research integrity at Frontiers
Learn more about the work of our research integrity team to safeguard the quality of each article we publish.