- 1Trauma Department, Hannover Medical School (MHH), Hannover, Germany
- 2Institute for Medical Microbiology and Hospital Epidemiology, Hannover Medical School (MHH), Hannover, Germany
- 3Department of Rehabilitation Medicine, Hannover Medical School (MHH), Hannover, Germany
Background: Transcutaneous osseointegrated prosthesis systems (TOPS) are alternative rehabilitation methods to socket prosthetics, after limb amputation. TOPS compromise a two-step surgery: starting with the implantation of the stem which is then followed by the creation of the transcutaneous stoma through which the exoprosthesis can be connected. Immediately after surgery, this opening is permanently exposed to pathogens. This study aimed to investigate the dynamics of bacterial colonization of the stoma to analyze whether obligate bacterial colonization leads to a risk of periprosthetic infections after TOPS treatment.
Methods: This prospective study analyzed data from 66 patients (aged 26–75 years) after TOPS treatment between 2017 and 2019. Microbiological swabs from the stoma were analyzed on the first postoperative day and 3, 6, 12, and 24 months after stoma creation. Infection rates, laboratory values (CRP, leukocyte count, hemoglobin), and body temperature were recorded at these points in time. Statistical analysis was performed using SPSS 28.
Results: The results show the formation of a stable environment dominated by Gram-positive bacteria in the stoma of TOPS patients over 24 months. Staphylococcus aureus, Staphylococcus spp., and Streptococcus spp. were the most common species found. With regard to the cohort up to the 3 months follow-up, 7.9% (five patients) developed infections surrounding the TOPS procedure. In relation to the whole cohort with loss to follow-up of 80.3% at the 24 months follow-up the infection rates increased up to 38.3%.
Conclusion: The soft tissue inside and around the transcutaneous stoma is colonialized by multiple taxa and changes over time. A stable Gram-positive dominated bacterial taxa could be a protective factor for ascending periprosthetic infections and could possibly explain the relatively low infection rate in this study as well as in literature.
Introduction
The prevalence of amputation of extremities has increased considerably in the last years and is expected to rise even further in the upcoming years (Ziegler-Graham et al., 2008). Especially amputations of the lower limb, such as transfemoral amputations, result in a high deprivation of quality of life. The utilization of a prosthesis can restore participation in daily life and play the most crucial role in rehabilitation of patients with lower limb amputation (Frengopoulos et al., 2021; Knight et al., 2021). However, the standard socket suspension for attachment of the prosthesis has limitations (Cheifetz et al., 2007; Chislett et al., 2020). The socket prosthesis often leads to local irritation, and skin damage. Furthermore, weight-dependent changes of the soft tissue of the stump can lead to an insufficient attachment of the prothesis (Hoffmeister et al., 2017; Beck et al., 2019); but there are optional methods to attach the prosthetic leg to the tight stump. The treatment of amputations with transcutaneous osseointegrated prosthetic systems (TOPS) has been established throughout the last two decades in Germany, Sweden, the Netherlands, and Australia (Aschoff et al., 2009, 2010; Juhnke and Aschoff, 2015; Juhnke et al., 2015; Aschoff, 2017a,b; Pospiech et al., 2020). Compared to the most frequently used “socket suspension” method for prosthetic limb attachment, this method offers a variety of new options (Hagberg et al., 2005; Aschoff et al., 2009; Frossard et al., 2009; Van de Meent et al., 2013; Hoffmeister et al., 2017; Al Muderis et al., 2018). Furthermore, TOPS shows unique advantages, such as osseoperception, which leads to an improved sense of grounding with the prosthetic foot, and prosthetic limb control (Hagberg et al., 2008; Hagberg and Branemark, 2009; Örgel et al., 2021a). Another advantage is a higher physical functional performance with a better range of motion, a higher sitting comfort, and an unlimited “wearing time” of the prothesis (Hagberg et al., 2005).
Regarding the implantation, the surgical procedure is quite simple. The endo-fix stem is press-fitted into the bone in the first surgery, and after 4–6 weeks, the transcutaneous stoma is created in a second step (Örgel et al., 2021b). Currently, steps one and two are often performed in a single step together (Al Muderis et al., 2017; Reif et al., 2021). Despite all the advantages of this procedure, one major danger lies in the transcutaneous stoma which leads to the absence of a physiological skin seal which remains lifelong. This fact constantly exposes the implanted transcutaneous device to pathogens from the external environment.
Due to this circumstance, one may primarily assume a high-risk factor for infections. Interestingly the literature shows only low to moderate infection rates, which in most cases can be treated with antibiotic therapy (Tsikandylakis et al., 2014; Al Muderis et al., 2016; Hebert et al., 2017; Kunutsor et al., 2018; Atallah et al., 2020; Reetz et al., 2020; Wang et al., 2021). Infection rates differ between surgical centers, surgical techniques, and types of implants (Hagberg and Branemark, 2009; Aschoff et al., 2010; Tillander et al., 2010; Tsikandylakis et al., 2014; Al Muderis et al., 2016; Ranker et al., 2020). Besides tissue infections, rare cases of osteomyelitis, and bone necrosis were also observed (Tillander et al., 2010, 2017; Tsikandylakis et al., 2014).
Along with the low infection rates with external fixators, it remains unclear how the normal skin flora develops with TOPS. In a longitudinal cohort study, Beck et al. observed 10 patients with TOPS with skin and stomal swabs over a 1-year period (Beck et al., 2019). They showed that the microbiota on the stomal site develops over time and forms a stable skin flora with colonialization dominated by Streptococcus spp., Corynebacterium spp., and/or Staphylococcus spp.
As mentioned in the study from Örgel et al. (2022), this prospective study investigates the dynamics of bacterial colonization on the stomal site and elucidates whether treatment with TOPS leads to a large number (>10%) of periprosthetic and stomal infections. Laboratory values during the course were also monitored.
This leads to the following hypotheses:
- Null hypothesis: Treatment with a transcutaneous osseointegrated prosthetic system does not lead to a large number (>10%) of periprosthetic and stomal infections.
- Alternative hypothesis: Treatment with a transcutaneous osseointegrated prosthetic system leads to a large number (>10%) of periprosthetic and stomal infections.
Methods
Study design and participants
This prospective study followed the “Strengthening the Reporting of Observational Studies in Epidemiology (STROBE)” reporting guideline. Informed consent was obtained from each patient at all times. This paper reports the results of a data analysis within one cohort of patients treated with the Endo-Exo-Prosthesis (ESKA Orthopaedic Handels GmbH®, Osterweide 2c, 23562 Lübeck, Germany), which belongs to TOPS after transfemoral amputation. Overall, n = 66 patients were enrolled. All patients were treated surgically with TOPS between 2017 and 2019 in one center. The two-step procedure was performed in each case (Aschoff et al., 2010, 2011). Each patient was screened for oxacillin/methicillin-resistant Staphylococcus aureus colonization prior to surgical procedure. Idem to Beck et al., all patients received a single intravenous dose of cephazolin (2 g) for each surgery (step one and step two). Each dose was given within 30 min before incision (Beck et al., 2019). In cases of known penicillin allergy, clindamycin (600 mg) was used instead. Before each surgery, the skin was disinfected three times using Braunoderm (Braun Medical AG, Seesatz 17, 6204 Switzerland). The microbiological swabs, and the blood samples for monitoring the laboratory values were taken during the inpatient stay in the context of the operations, and the follow-up assessment in our outpatient clinic. The blood samples were analyzed for standardized laboratory values (c-reactive protein, leucocytes, hemoglobin, body temperature) on the first, third, and fifth, postoperative day, and 3, 6, 12, and 24 months after surgery. The microbiological swabs were collected during the second surgery (intraoperative), on the first day after the second surgery, and 3, 6, 12, and 24 months after surgery.
Swab material and technique
Every patient was screened via stomal microbial sampling with a swab (Amies medium, No. 108, transystem®, HAIN. Lifescience/Copan) taken in a circular motion around the double cone, which is directly anchored to the Endo-Fix-Stem. The swab was inserted deep into the inner lining until resistance was felt. The inner lining consists of soft tissue and is the space around the endoprosthetic parts which are not fixed in the bone (Juhnke et al., 2015; Ranker et al., 2020). A medical doctor took the swabs. Swabs were sent to the microbiology department immediately after sampling. Culture conditions were set according to national guidelines for microbiological diagnostic with an overall incubation time of 14 days. Matrix Assisted Laser Desorption/Ionization Time-of-Flight (MALDI TOF) Mass Spectrometry Analysis or other specific tests were mainly used for identifying bacterial isolates. For this analysis we classified the taxa into two groups, Gram-positive and Gram-negative bacteria. Gram-positive bacteria were distinguished into Staphylococcus aureus, Staphylococcus spp., Streptococcus spp., Corynebacteria, and other Gram-positive bacteria. Gram-negative bacteria were differentiated into Pseudomonas spp., Acinetobacter spp., Enterobacteriaceae, and other Gram-negative bacteria. Finally, we documented the incidence of yeasts.
Laboratory values
A qualified nurse took blood samples during the inpatient stay and during the visit at our outpatient clinic. After disinfection of the skin, and venous blood stasis, the venous puncture was performed with a 20G needle. The samples were sent to our clinical chemistry department for standard processing. The infection laboratory includes c-reactive protein (CRP; reference is ≤5 mg according to the specifications of our laboratory) in milligram per liter (mg/l), and leucocytes in thousand per microliter (tsd/μl; reference between 3.9 and 10.2 tsd/μl) as well as hemoglobin (Hb) in gram per deciliter [g/dl; reference between 13.5 and 7.2 (g/dl)], and the body temperature in Celsius degree (°C). Body temperatures between 37.5 and ≤38°C are considered elevated body temperature. A body temperature of >38°C is defined as fever. A nurse measured the body temperature with a digital ear thermometer. A daily reporting system protocoled the laboratory values via our hospital's internal software. All patients were instructed to clean their stoma from the fifth postoperative day on, one to two times a day, with clear water and optional non-alcoholic soap. Swimming in stationary waters like lakes and swimming pools was not allowed. Swimming in the sea and rivers was allowed. We did not consider these parameters during data collection.
The classification of infection
We used the international accepted classification of stomal infections by Al Muderis et al. (2016). In this two-center cohort study, the authors divided the level of severity of infection into low and high-grade soft-tissue infection, as well as into bone infection and implant failure. Except implant failure, the groups were separated into subunits A-C; A includes oral antibiotics, B parenteral antibiotics, and C surgical intervention. Implant failure contains parenteral antibiotics and explanation without dividing into subgroups.
Data collection and loss to follow-up
Within a standardized protocol clinical data from each patient were systematically collected. Included are every result of the standardized stomal swabs and infection laboratory values. Loss to follow-up is shown in Figure 1.
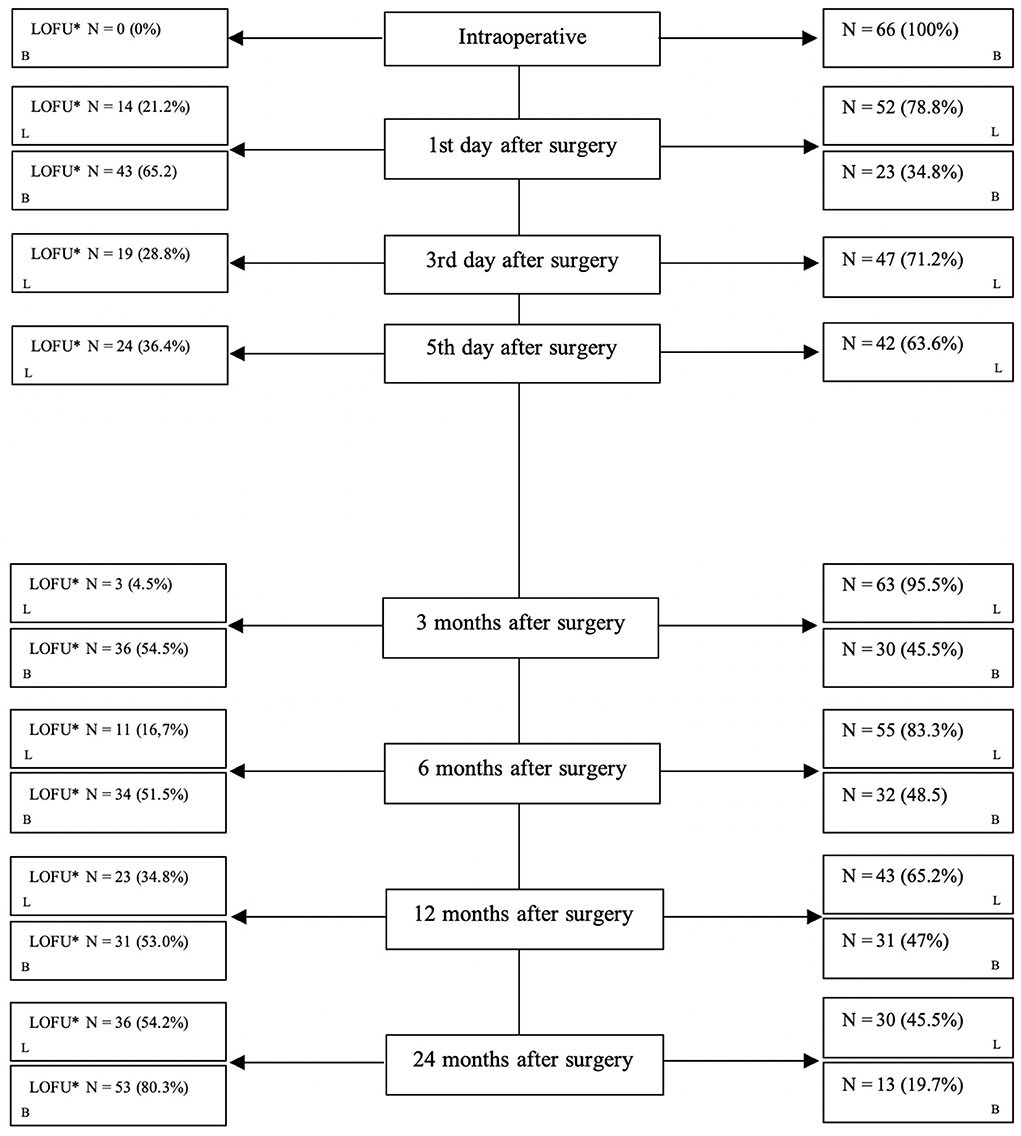
Figure 1. Loss to follow-up during each step. *LOFU, loss to follow-up; N, number; L, laboratory value; B, bacterial swabs.
Statistical analysis
In August 2021, all relevant data of the study hypothesis were systematically evaluated. Descriptive analysis was performed for the stomal swabs, and the infection laboratory values. Besides, the descriptive evaluation of bacterial colonization on the stoma, all infections were numerically captured and underlying the causing bacterial taxa. The whole dataset was checked for normal distribution. Statistical analysis was performed using SPSS 28 (IBM, SPSS Inc., Chicago, IL). Subgroup analysis was carried out according to Gram-staining of bacteria and follow-up of patients.
Results
Main results
From 2017 to 2019, n = 66 patients underwent the TOPS procedure after transfemoral amputation in our tertiary care hospital in northern Germany (Örgel et al., 2022). Over time, the loss to follow-up increased as expected. Figure 1 shows in detail the loss to follow-up over 24 months.
The mean age of the patients was 50.8 ± 12.3 years (Örgel et al., 2022). One Patient died due to secondary disease. The most common reason for amputation was trauma. With regard to the cohort up to the 13 months follow-up, 7.9% (five patients) developed infections surrounding the TOPS procedure. In relation to the whole cohort with loss to follow-up, of 80.3% at the 24 months follow-up the infection rates increased up to 38.3%. Detailed information about the cohort is shown in Table 1.
Figure 2 shows the results of the microbiological examination of the swabs. During every sampling of the 24 months follow-up the most common taxa found at the skin implant interface were Staphylococcus aureus, Staphylococcus spp., and Streptococcus spp. (Örgel et al., 2022). None of the Staphylococcus aureus were Oxacillin or Methicillin-resistant. After the first day, we noted a rare count of Gram-negative bacteria with a fairly stable proportion over the entire study period. At the 3 months follow-up, we had two swabs identifying Candida albicans.
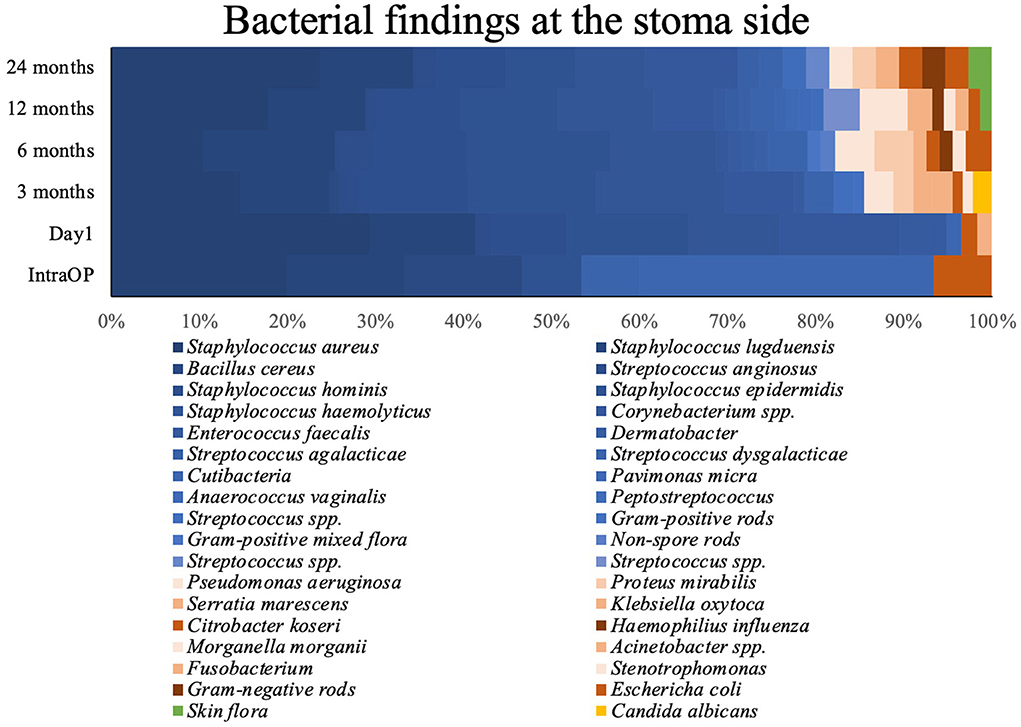
Figure 2. Bacterial findings at the stomal side during the observed period. Blue, GPB; red, GNB; yellow, Candida albicans; green, Skin flora.
Detailed information is shown in Figure 2 and Table 2. Figure 3 shows the long-term course of the infection values [CRP (mg/l), leucocyte (tsd/μl)], hemoglobin (g/dl), and body temperature (°C).
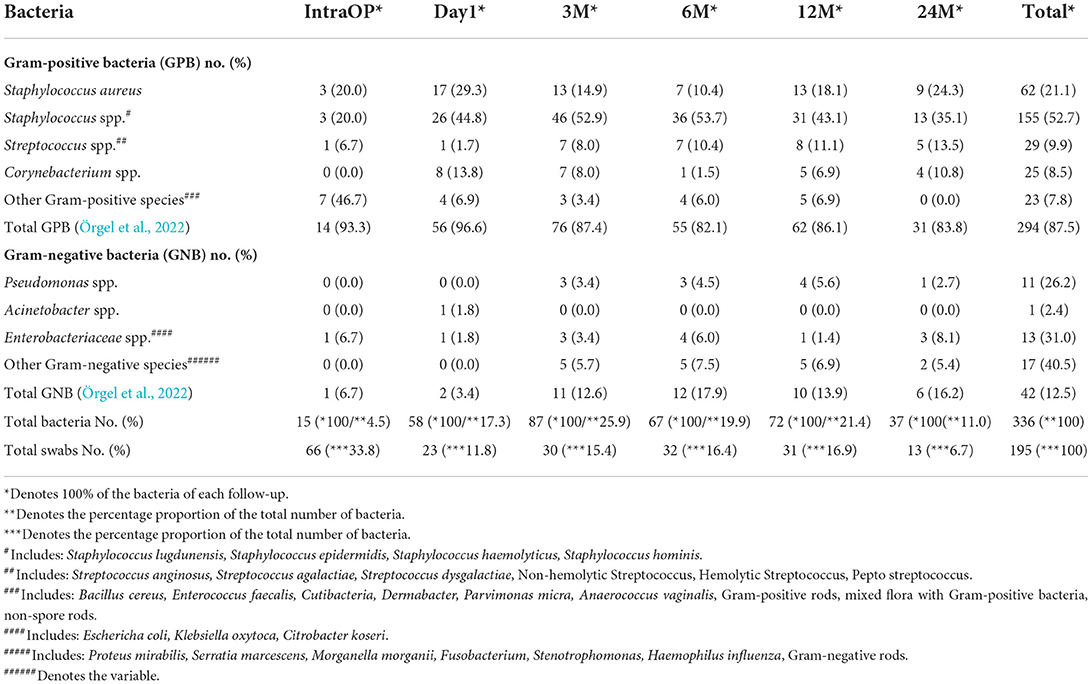
Table 2. Presentation of the most common bacterial species at the intervals “during the surgery (IntraOP)”, “first day after surgery (Day1)”, “3, 6, 12, and 24 months (m) after surgery”.
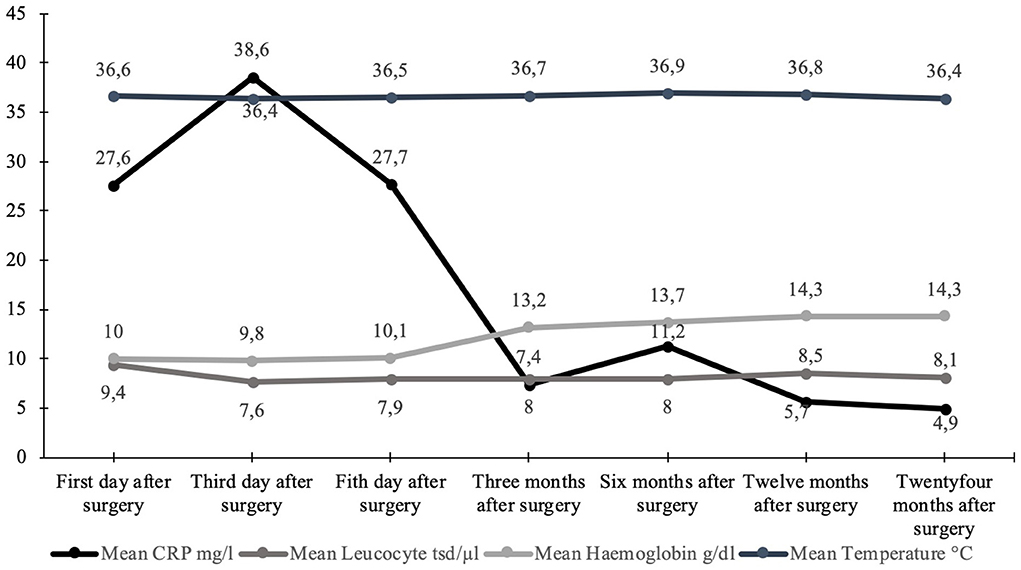
Figure 3. Graphical visualization of the mean values of the long-term course of body temperature (°C), hemoglobin (g/dl), and infection values [leucocytes (tsd/μl) + CRP (mg/l)].
Infection
Five documented infections occurred. The infections were classified according to Al Muderis et al. (2016). We had one high-grade (2C), two low-grade (1A), one bone infection before the first surgery, and one infection of a hematoma after the first surgery. The infections were cured with species-specific resistogram antibiotic treatment, and in some cases, with additional surgery (abscess splitting). No implant loosening or sepsis was observed. Detailed information is shown in Tables 3, 4.
Discussion
One of the most frequent questions in the context of TOPS concerns the risk of infections due to the lack of a physiological skin barrier of the stoma. Skin flora has several functions. This circumstance includes the protective function against pathogen invasion, the development and establishment of the immune system, and the catabolism of natural products (Kong and Segre, 2012; Scharschmidt and Fischbach, 2013; Belkaid and Segre, 2014). In addition to fungi, viruses, archaea, and small arthropods, the normal skin flora consists of millions of bacteria (Byrd et al., 2018; Swaney and Kalan, 2021). This prospective data analysis showed infection rates of 7.9% at the 3 months follow-up. With regard to our 24 months follow-up, the infection rate increases up to 38.5% according to the loss to follow-up of 80.3%. This circumstance is concordant to the literature, which reports infection rates ranging from 5.1 to 68.2% and the null hypothesis could be accepted.
The stoma of TOPS is mainly colonized by microbiological organisms of the normal skin flora (Cundell, 2018). Staphylococcus aureus, Staphylococcus spp., and Streptococcus spp. are the most common identified bacterial species. Subsequently, the findings were split into Gram-positive and Gram-negative bacteria. Gram-positive was the most common taxa at the stoma. This fact confirms existing results. Beck et al. showed comparable findings in their prospective longitudinal scientific work without infection prevalence. They made a slightly different classification than we did. In their study, staphylococci, streptococci, and corynebacteria were the dominant taxa (Beck et al., 2019).
Regarding the taxa of the normal skin flora, we did not identify Propioniebacteria in the stomal area, which also belong to the main taxa of normal skin flora. Cutibacterium is more likely to be found in dry and sebaceous regions than in the region of the thigh skin (Byrd et al., 2018; Timm et al., 2020). Contrary to our protocol, Beck et al. acquired a microbiological swab from the skin surface of the ipsilateral and the opposite side and a stomal swab.
The distribution of bacterial species between the contralateral and ipsilateral thighs was comparable (Beck et al., 2019). Oh et al. published results in which they analyzed the structural and functional constitution of the human skin microbiome prospectively and longitudinally. They took swabs from various body sites, such as the glabella, external auditory canal, manubrium, antecubital fossa, or inguinal crease, popliteal fossa, the toe box, and plantar fossa (Schommer and Gallo, 2013; Oh et al., 2016; Cundell, 2018; Timm et al., 2020). In addition to virus and eukaryota, they equally identify bacterial taxa as shown by Beck et al. and the present results (Oh et al., 2016; Beck et al., 2019). With increasing time after creating the stoma, an equilibrium of the bacterial flora with the leading taxa Staphylococci developed, which could have a protective effect. In the course of 24 months we could not detect any specific bacterial pathogen. Our patients with TOPS had no attributable risk during the observed period with a probable biofilm formation of Staphylococcae, or yeasts. Enterobacterales were only rarely detected and in equal counts over the 3–24 months screening. Pseudomonadaceae, as a potential pathogenic species for chronic wound infections and often linked with a humid environment, were scarcely found in our microbiological cultures of the swabs without any attributable infection. Concerning dental implants, which are the origin of the development of TOPS, Lafaurie et al. showed in their systematic review that the taxa differ in peri-implantitis, periodontitis, and healthy implants.
Therefore, it is hypothesized that stable bacterial flora at the stoma side, according to the normal skin flora, could inhibit infections, so regular stoma hygiene (without a specific disinfection protocol) is required to obtain a stable bacterial flora. This circumstance is consistent with the results of our study, as we had only three minor infections after creating the stoma, which could be cured with antibiotics. The other two infections (Table 3) had a presumptive predictive increased risk of infections because the patients had multiple pre-surgeries in the Middle East after sustaining war injuries (Murray et al., 2009, 2011a,b; Al Muderis et al., 2016), although the laboratory values showed a normal dynamic for each (Figure 3). Our study concludes that a stable bacterial flora might have a protective function regarding pathogens with high infection risk. Although patients treated with TOPS will have a permanent stoma, it seems that during maturation and regaining a stable balance of different physiological taxa, the risk for deep infections is much lower than expected.
Limitations
This study shows the results of a 24-month follow-up period, but it is limited in showing long-term infectious complications and does not allow a differentiation between short- and long-term complications, and possible varying pathogens. No control group was included.
Moreover, the infection rates are calculated for the whole cohort. Loss to follow-up was 65.2% on the first day after surgery and 80.3% after 24 months after surgery. This aspect was considered when interpreting the results. In addition, this circumstance is caused by the fact that some patients visited our follow-up appointments irregularly due to minor compliance. However, this study is the first prospective study including TOPS patients, screening their bacterial taxa at the stoma side over 24 months.
Conclusion
Despite the permanently open environment of the stoma with its bacterial taxa and the consecutive connection to the prosthesis, the stoma of our cohort shows a dominated stable Gram-positive taxa with an infection rate of 7.9% at the 3 months follow-up and 38.5% at the 24 months follow up. Thus, this study shows that the soft tissue inside and around the transcutaneous stoma is immediately after surgery colonialized by multiple taxa which are changing over time. The stable Gram-positive dominated bacterial taxa could be seen as a protective factor against ascending periprosthetic infections and could possibly explain the relatively low infection rate in this study as well as in literature.
Data availability statement
The raw data supporting the conclusions of this article will be made available by the authors, without undue reservation.
Ethics statement
All procedures performed in studies involving human participants were in accordance with the ethical standards of the institutional and/or national research committee and with the 1964 Helsinki Declaration and its later amendments or comparable ethical standards. Due to the retrospective data collection ethical approval was given as a waiver (No. 10513_BO_K_2022) and consent was granted by the Ethics Committee of Hannover Medical School.
Author contributions
Conceptualization: MÖ and AR. Data curation: MÖ, SR, H-HA, AR, and TG. Formal analysis: MÖ, LS, and H-HA. Funding acquisition. Investigation: MÖ, SR, AR, and TG. Methodology: MÖ, LS, CK, SR, and AR. Project administration: MÖ, SR, and LS. Resources and writing—original draft: MÖ and SR. Software: MÖ, LS, CK, and AR. Supervision: MÖ, LS, and AR. Validation: MÖ, SR, LS, AR, and TG. Visualization: MÖ, AR, and TG. Writing—review and editing: MÖ, LS, CK, H-HA, and AR. All authors contributed to the article and approved the submitted version.
Conflict of interest
The authors declare that the research was conducted in the absence of any commercial or financial relationships that could be construed as a potential conflict of interest.
Publisher's note
All claims expressed in this article are solely those of the authors and do not necessarily represent those of their affiliated organizations, or those of the publisher, the editors and the reviewers. Any product that may be evaluated in this article, or claim that may be made by its manufacturer, is not guaranteed or endorsed by the publisher.
References
Al Muderis, M., Khemka, A., Lord, S. J., Van de Meent, H., and Frolke, J. P. (2016). Safety of osseointegrated implants for transfemoral amputees: a two-center prospective cohort study. J. Bone Joint Surg. Am. 98, 900–909. doi: 10.2106/JBJS.15.00808
Al Muderis, M., Lu, W., Tetsworth, K., Bosley, B., and Li, J. J. (2017). Single-stage osseointegrated reconstruction and rehabilitation of lower limb amputees: the Osseointegration Group of Australia Accelerated Protocol-2 (OGAAP-2) for a prospective cohort study. BMJ Open 7, e013508. doi: 10.1136/bmjopen-2016-013508
Al Muderis, M. M., Lu, W. Y., Li, J. J., Kaufman, K., Orendurff, M., Highsmith, M. J., et al. (2018). Clinically relevant outcome measures following limb osseointegration; systematic review of the literature. J. Orthop. Trauma. 32, e64–e75. doi: 10.1097/BOT.0000000000001031
Aschoff, H. H. (2017a). Transcutaneous osseointegration after limb amputation: a review over 27 years. Unfallchirurg 120, 278–284. doi: 10.1007/s00113-017-0329-y
Aschoff, H. H. (2017b). TOPS - transkutane osseointegrierte Prothesensysteme. Orthopädie Unfallchirurgie 12, 7–105. doi: 10.1055/s-0042-111336
Aschoff, H. H., Clausen, A., and Hoffmeister, T. (2009). Die Endo-Exo-Femurprothese – ein neues Konzept zur knochengeführten, prothetischen Versorgung von oberschenkelamputierten Patienten. Z. Orthop. Unfall. 147, 610–615. doi: 10.1055/s-0029-1185893
Aschoff, H. H., Clausen, A., Tsoumpris, K., and Hoffmeister, T. (2011). Implantation of the endo-exo femur prosthesis to improve the mobility of amputees. Oper. Orthop. Traumatol. 23, 462–472. doi: 10.1007/s00064-011-0054-6
Aschoff, H. H., Kennon, R. E., Keggi, J. M., and Rubin, L. E. (2010). Transcutaneous, distal femoral, intramedullary attachment for above-the-knee prostheses: an endo-exo device. J. Bone Joint Surg. Am. 92(Suppl. 2), 180–186. doi: 10.2106/JBJS.J.00806
Atallah, R., van de Meent, H., Verhamme, L., Frolke, J. P., and Leijendekkers, R. A. (2020). Safety, prosthesis wearing time and health-related quality of life of lower extremity bone-anchored prostheses using a press-fit titanium osseointegration implant: A Prospective one-year follow-up cohort study. PLoS ONE 15, e0230027. doi: 10.1371/journal.pone.0230027
Beck, J. P., Grogan, M., Bennett, B. T., Jeyapalina, S., Agarwal, J., Bartow-McKenney, C., et al. (2019). Analysis of the stomal microbiota of a percutaneous osseointegrated prosthesis: a longitudinal prospective cohort study. J. Orthop. Res. 37, 2645–2654. doi: 10.1002/jor.24421
Belkaid, Y., and Segre, J. A. (2014). Dialogue between skin microbiota and immunity. Science 346, 954–959. doi: 10.1126/science.1260144
Byrd, A. L., Belkaid, Y., and Segre, J. A. (2018). The human skin microbiome. Nat. Rev. Microbiol. 16, 143–155. doi: 10.1038/nrmicro.2017.157
Cheifetz, O., Bayley, M., Grad, S., Lambert, D., Watson, C., Minor, K., et al. (2007). The lower limb amputee measurement scale: reliability and predictive validity. Prosthet. Orthot. Int. 31, 300–312. doi: 10.1080/03093640601044311
Chislett, M., Ploughman, M., and McCarthy, J. (2020). Factors associated with prolonged length of stay and failed lower limb prosthetic fitting during inpatient rehabilitation. Arch. Rehabil. Res. Clin. Transl. 2, 100084. doi: 10.1016/j.arrct.2020.100084
Cundell, A. M. (2018). Microbial ecology of the human skin. Microb. Ecol. 76, 113–120. doi: 10.1007/s00248-016-0789-6
Frengopoulos, C., Fuller, K., Payne, M. W. C., Viana, R., and Hunter, S. W. (2021). Rehabilitation outcomes after major lower limb amputation in the oldest old: a systematic review. Prosthet. Orthot. Int. doi: 10.1097/PXR.0000000000000038
Frossard, L., Hagberg, K., Haggstrom, E., and Branemark, R. (2009). Load-relief of walking AIDS on osseointegrated fixation: instrument for evidence-based practice. IEEE Trans. Neural Syst. Rehabil. Eng. 17, 9–14. doi: 10.1109/TNSRE.2008.2010478
Hagberg, K., and Branemark, R. (2009). One hundred patients treated with osseointegrated transfemoral amputation prostheses–rehabilitation perspective. J. Rehabil. Res. Dev. 46, 331–344. doi: 10.1682/JRRD.2008.06.0080
Hagberg, K., Häggström, E., Jönsson, S., Rydevik, B., and Brånemark, R. (2008). “Osseoperception and osseointegrated prosthetic limbs,” in Psychoprosthetics, eds P. Gallagher, D. Desmond, and M. MacLachlan (London: Springer London), 131–140.
Hagberg, K., Haggstrom, E., Uden, M., and Branemark, R. (2005). Socket versus bone-anchored trans-femoral prostheses: hip range of motion and sitting comfort. Prosthet. Orthot. Int. 29, 153–163. doi: 10.1080/03093640500238014
Hebert, J. S., Rehani, M., and Stiegelmar, R. (2017). osseointegration for lower-limb amputation: a systematic review of clinical outcomes. JBJS Rev. 5, e10. doi: 10.2106/JBJS.RVW.17.00037
Hoffmeister, T., Schwarze, F., and Aschoff, H. H. (2017). The endo-exo prosthesis treatment concept : Improvement in quality of life after limb amputation. Unfallchirurg 120, 371–377. doi: 10.1007/s00113-017-0350-1
Juhnke, D. L., and Aschoff, H. H. (2015). Endo-exo prostheses following limb-amputation. Orthopade 44, 419–425. doi: 10.1007/s00132-015-3117-9
Juhnke, D. L., Beck, J. P., Jeyapalina, S., and Aschoff, H. H. (2015). Fifteen years of experience with Integral-Leg-Prosthesis: cohort study of artificial limb attachment system. J. Rehabil. Res. Dev. 52, 407–420. doi: 10.1682/JRRD.2014.11.0280
Knight, A. D., Dearth, C. L., and Hendershot, B. D. (2021). Deleterious musculoskeletal conditions secondary to lower limb loss: considerations for prosthesis-related factors. Adv Wound Care 10, 671–684. doi: 10.1089/wound.2019.1079
Kong, H. H., and Segre, J. A. (2012). Skin microbiome: looking back to move forward. J. Invest. Dermatol. 132, 933–939. doi: 10.1038/jid.2011.417
Kunutsor, S. K., Gillatt, D., and Blom, A. W. (2018). Systematic review of the safety and efficacy of osseointegration prosthesis after limb amputation. Br. J. Surg. 105, 1731–1741. doi: 10.1002/bjs.11005
Murray, C. K., Hospenthal, D. R., Kotwal, R. S., and Butler, F. K. (2011a). Efficacy of point-of-injury combat antimicrobials. J Trauma 71(2 Suppl. 2), S307–S313. doi: 10.1097/TA.0b013e318227af79
Murray, C. K., Wilkins, K., Molter, N. C., Li, F., Yu, L., Spott, M. A., et al. (2011b). Infections complicating the care of combat casualties during operations Iraqi Freedom and Enduring Freedom. J. Trauma 71, S62–73. doi: 10.1097/TA.0b013e3182218c99
Murray, C. K., Wilkins, K., Molter, N. C., Yun, H. C., Dubick, M. A., Spott, M. A., et al. (2009). Infections in combat casualties during operations Iraqi and Enduring Freedom. J. Trauma 66, S138–S144. doi: 10.1097/TA.0b013e31819d894c
Oh, J., Byrd, A. L., Park, M., Program, N. C. S., Kong, H. H., Segre, J. A., et al. (2016). Temporal stability of the human skin microbiome. Cell 165, 854–866. doi: 10.1016/j.cell.2016.04.008
Örgel, M., Aschoff, H. H., Sedlacek, L., Graulich, T., Krettek, C., Roth, S., et al. (2022). Analysis of stomal bacterial colonialization after transcutaneous osseointegrated prosthetic systems surgery. JAMA Netw Open 5, e2223383. doi: 10.1001/jamanetworkopen.2022.23383
Örgel, M., Elareibi, M., Graulich, T., Krettek, C., Neunaber, C., Aschoff, H. H., et al. (2021a). Osseoperception in transcutaneous osseointegrated prosthetic systems (TOPS) after transfemoral amputation: a prospective study. Arch. Orthop. Trauma. Surg. doi: 10.1007/s00402-021-04099-1
Örgel, M., Ranker, A., Harb, A., Krettek, C., and Aschoff, H. H. (2021b). Transcutaneous osseointegrated prosthetic systems after major amputation of the lower extremity: a retrospective 3-year analysis. Orthopade.50, 4–13.
Pospiech, P. T., Wendlandt, R., Aschoff, H. H., Ziegert, S., and Schulz, A. P. (2020). Quality of life of persons with transfemoral amputation: comparison of socket prostheses and osseointegrated prostheses. Prosthet. Orthot. Int. 309364620948649. doi: 10.1177/0309364620948649
Ranker, A., Örgel, M., Beck, J. P., Krettek, C., and Aschoff, H. H. (2020). Transcutaneous Osseointegrated Prosthetic Systems (TOPS) for transfemoral amputees - a six-year retrospective analysis of the latest prosthetic design in Germany. Rehabilitation 59, 357–365.
Reetz, D., Atallah, R., Mohamed, J., van de Meent, H., Frolke, J. P. M., Leijendekkers, R., et al. (2020). Safety and performance of bone-anchored prostheses in persons with a transfemoral amputation: a 5-year follow-up study. J. Bone Joint Surg. Am. 102, 1329–1335. doi: 10.2106/JBJS.19.01169
Reif, T. J., Khabyeh-Hasbani, N., Jaime, K. M., Sheridan, G. A., Otterburn, D. M., Rozbruch, S. R., et al. (2021). Early experience with femoral and tibial bone-anchored osseointegration prostheses. JB JS Open Access 6. doi: 10.2106/JBJS.OA.21.00072
Scharschmidt, T. C., and Fischbach, M. A. (2013). What lives on our skin: ecology, genomics and therapeutic opportunities of the skin microbiome. Drug Discov Today Dis Mech. (2013) 10. doi: 10.1016/j.ddmec.2012.12.003
Schommer, N. N., and Gallo, R. L. (2013). Structure and function of the human skin microbiome. Trends Microbiol. 21, 660–668. doi: 10.1016/j.tim.2013.10.001
Swaney, M. H., and Kalan, L. R. (2021). living in your skin: microbes, molecules, and mechanisms. Infect. Immun. 89. doi: 10.1128/IAI.00695-20
Tillander, J., Hagberg, K., Berlin, Ö., Hagberg, L., and Brånemark, R. (2017). Osteomyelitis risk in patients with transfemoral amputations treated with osseointegration prostheses. Clin. Orthop. Relat. Res. 475, 3100–3108. doi: 10.1007/s11999-017-5507-2
Tillander, J., Hagberg, K., Hagberg, L., and Brånemark, R. (2010). Osseointegrated titanium implants for limb prostheses attachments: infectious complications. Clin. Orthopaed. Relat. Res. 468, 2781–2788. doi: 10.1007/s11999-010-1370-0
Timm, C. M., Loomis, K., Stone, W., Mehoke, T., Brensinger, B., Pellicore, M., et al. (2020). Isolation and characterization of diverse microbial representatives from the human skin microbiome. Microbiome 8, 58. doi: 10.1186/s40168-020-00831-y
Tsikandylakis, G., Berlin, O., and Branemark, R. (2014). Implant survival, adverse events, and bone remodeling of osseointegrated percutaneous implants for transhumeral amputees. Clin. Orthop. Relat. Res. 472, 2947–2956. doi: 10.1007/s11999-014-3695-6
Van de Meent, H., Hopman, M. T., and Frolke, J. P. (2013). Walking ability and quality of life in subjects with transfemoral amputation: a comparison of osseointegration with socket prostheses. Arch. Phys. Med. Rehabil. 94, 2174–2178. doi: 10.1016/j.apmr.2013.05.020
Wang, H., Agrawal, A., Wang, Y., Crawford, D. W., Siler, Z. D., Peterson, M. L., et al. (2021). An ex vivo model of medical device-mediated bacterial skin translocation. Sci. Rep. 11, 5746. doi: 10.1038/s41598-021-84826-1
Keywords: infection, bacterial colonization, transcutaneous osseointegrated prosthetic system (TOPS), amputation, osseointegration
Citation: Örgel M, Aschoff H-H, Sedlacek L, Graulich T, Krettek C, Roth S and Ranker A (2022) Twenty-four months of bacterial colonialization and infection rates in patients with transcutaneous osseointegrated prosthetic systems after lower limb amputation—A prospective analysis. Front. Microbiol. 13:1002211. doi: 10.3389/fmicb.2022.1002211
Received: 24 July 2022; Accepted: 07 September 2022;
Published: 31 October 2022.
Edited by:
Axel Cloeckaert, Institut National de recherche pour l'agriculture, l'alimentation et l'environnement (INRAE), FranceReviewed by:
James Beck, United States Department of Veterans Affairs, United StatesCarlo Luca Romano', University of Milan, Italy
Copyright © 2022 Örgel, Aschoff, Sedlacek, Graulich, Krettek, Roth and Ranker. This is an open-access article distributed under the terms of the Creative Commons Attribution License (CC BY). The use, distribution or reproduction in other forums is permitted, provided the original author(s) and the copyright owner(s) are credited and that the original publication in this journal is cited, in accordance with accepted academic practice. No use, distribution or reproduction is permitted which does not comply with these terms.
*Correspondence: Marcus Örgel, M.Oergel@gmx.de
†These authors have contributed equally to this work and share last authorship
‡ORCID: Marcus Örgel orcid.org/0000-0001-9996-2864