- 1Heilongjiang Province Key Laboratory of Forest Protection, School of Forest, Northeast Forestry University, Harbin, China
- 2School of Biological Sciences, Guizhou Education University, Guiyang, China
- 3College of Forestry, Guizhou University, Guiyang, China
Juniper essential oil (JEO), which is mostly known as an immune system booster and effective detoxifier, has substantial antimicrobial activity. A comparison of the inhibitory effects of three plant essential oils from juniper (Juniperus rigida), cedarwood (Juniperus virginiana), and cypress (Crupressus sempervirens) on four plant pathogenic fungi indicated that JEO was the most effective at inhibiting the growth of gray mold (Botrytis cinerea). Additional studies were subsequently conducted to explore the in vivo and in vitro antifungal activity and possible mechanism of JEO against B. cinerea. The results show that JEO inhibited the germination of spores and mycelial growth of B. cinerea in a concentration-dependent manner and exhibited strong inhibition when its concentration exceeded 10 μL/mL. JEO also significantly inhibited the incidence of disease and diameters of gray mold lesions on cherry tomato fruit (Solanum lycopersicum). After 12 h of treatment with JEO, the extracellular conductivity, and the contents of soluble protein, malondialdehyde, and hydrogen peroxide were 3.1, 1.2, 7.2, and 4.7 folds higher than those of the control group, respectively (P < 0.05), which indicated that JEO can damage membranes. Scanning electron microscopy observations revealed that JEO affected the morphology of mycelia, causing them to shrivel, twist and distort. Furthermore, JEO significantly improved the activities of the antioxidant-related enzymes superoxide dismutase and catalase but reduced the pathogenicity-related enzymes polygalacturonase (PG), pectin lyase and endoglucanase of B. cinerea (P < 0.05). In particular, PG was reduced by 93% after treatment with JEO for 12 h. Moreover, the 18 constituents of JEO were identified by gas chromatography/mass spectrometry (GC-MS) analysis, mainly limonene (15.17%), γ-terpinene (8.3%), β-myrcene (4.56%), terpinen-4-ol (24.26%), linalool (8.73%), α-terpineol (1.03%), o-cymene (8.35%) and other substances with antimicrobial activity. Therefore, JEO can be an effective alternative to prevent and control gray mold on cherry tomato fruit.
Introduction
Cherry tomato fruit (Solanum lycopersicum) are popular worldwide owing to their color, taste, and high levels of nutrients (Guo et al., 2021). However, as a climacteric fruit, they have a short postharvest life, and they are also vulnerable to environmental factors and phytopathogenic fungi that can cause the fruit to decay and deteriorate (Raynaldo et al., 2021). B. cinerea (gray mold) can infect all the organs of tomato except the roots, causing lesions in cherry tomatoes before and after picking, thus, resulting in reduced tomato yields (Guerra et al., 2015; Álvarez et al., 2021). In addition, B. cinerea can overwinter in the soil as spores or hyphae and reinfect the following year (Rguez et al., 2020). Gray mold frequently occurs in tomatoes during postharvest transport and storage, affecting the postharvest and shelf life of the fruit (Wang et al., 2010). Although synthetic fungicides do not deleteriously affect the appearance of fruit and can effectively control the occurrence of cherry tomato diseases, the excessive use of synthetic fungicides can lead to pesticide residues in the fruit and increased resistance to pathogenic fungi, endangering human health and environmental safety (Romanazzi et al., 2016). Therefore, it is necessary to find an environmentally friendly fungicide that can be used to sustainably control the occurrence of gray mold on cherry tomatoes (Touba et al., 2012; Ji et al., 2018).
Plant essential oils (PEOs) are volatile compounds that are extracted from various organs of plants through distillation and extraction. Many studies have shown that they can have a wide range of insecticidal, antibacterial, antifungal, antioxidant (Raut and Karuppayil, 2014), and anticancer properties (Hong et al., 2022). For example, the essential oil from Pulicaria crispa has an antibacterial effect on Gram-positive bacteria (AlMotwaa and Al-Otaibi, 2022). Ziziphora clinopodioides essential oil (ZCEO) inhibits mycelial growth and spore germination of Sclerotinia sclerotiorum (Ma et al., 2016). The essential oil from Origanum vulgare has an inhibitory effect on B. cinerea (Zhao et al., 2021). In addition, cinnamon oil, origanum oil, fennel oil and thyme oil have been found to inhibit Fusarium oxysporum occurred in strawberries (Park et al., 2017). The US Food and Drug Administration (FDA) has classified some PEOs as safe for human consumption because they cause less harm to the environment and human health (Hu et al., 2019; Bajac et al., 2022). Morales-Rabanales et al. (2022) found that a complex membrane of Schinus mole essential oil and chitosan inhibited the infestation of tomato fruit by Fusarium oxysporum f. sp. lycopersici.
Juniperus rigida Sieb. et Zucc., the temple juniper, is widely distributed in temperate regions of the northern hemisphere (Ghorbanzadeh et al., 2021). It is native to northern China, Korea and Japan, and is used in traditional Tibetan and Mongolian medicine to treat rheumatoid arthritis, edema, coughs, and skin diseases (Kim et al., 2010). Juniper extracts are known to contain phenolic substances, including flavonoids, chlorogenic acid, and catechin, terpenoids, podophyllotoxin, and other active substances that possess antibacterial, anticancer, and antioxidant activities (Gordien et al., 2009; Feng et al., 2020). Juniper essential oil (JEO) also contains a large number of terpenes, including α-pinene, limonene, β-pinene, and β-laurene (Zheljazkov et al., 2017). Although there is slight variation in the composition of the essential oil extracted from juniper in different regions, it is highly effective at inhibiting the human bacterial pathogens Clostridium perfringens and Staphylococcus aureus and the pathogenic yeast Candida clabrata (Zheljazkov et al., 2018).
Recently, there has been a substantial amount of study on the methods of extraction, determination of the chemical components and antioxidant effects of JEO, but there are few studies on whether JEO inhibits the growth of phytopathogenic fungi. In this study, the inhibitory effects of three plant essential oils, including juniper (Juniperus rigida) essential oil (JEO), cedarwood (Juniperus virginiana) essential oil (CWEO), and cypress (Cupressus sempervirens) essential oil (CPEO), on B. cinerea, F. oxysporum, Pestalotiopsis neglecta, and Alternaria alternata were compared. JEO was the most effective at inhibiting gray mold in vitro. Thus, the ability of JEO to control gray mold in cherry tomato fruit in vivo was explored. Moreover, the possible mechanism of action involved was investigated. This study provides a theoretical basis for the wider application of JEO to prevent and control gray mold in cherry tomato and possibly even other plant diseases.
Materials and methods
Materials
All the unilateral essential oils, including JEO, CWEO, and CPEO, were purchased from Jiangxi Yisenyuan Plant Fragrance Co., Ltd. (Nanchang, China). Test kits of malondialdehyde (MDA) content, hydrogen peroxide (H2O2) content, superoxide dismutase (SOD), catalase (CAT), polygalacturonase (PG), pectin lyase (PL), and endo-β-1-4 glucanase (EG) enzyme activity were obtained from Suzhou Grace Biotechnology Co., Ltd., (Suzhou, China). Other chemicals were analytical grade or better, purchased from Fuyu Fine Chemical Co., Ltd. (Tianjin, China).
Healthy and mature fresh cherry tomatoes were picked at the Kaiyang Lake Picking Orchard in Harbin, Heilongjiang Province, China.
Pathogen and cultures
The phytopathogenic fungi B. cinerea, F. oxysporum, P. neglecta, and A. alternata were provided by the Heilongjiang Province Key Laboratory of Forest Protection (Northeast Forestry University, Harbin, China) and cultured on potato dextrose agar (PDA) plates at 25°C.
The spore suspensions of B. cinerea were harvested and filtered through sterilized cotton as described by Ji et al. (2018). The spore concentrations were adjusted to 1 × 106 spores/mL using a hemocytometer under 400 × magnification via inversed optical microscopy.
Mycelial growth inhibition
The inhibitory effects of the three essential oils against the mycelial growth of B. cinerea, F. oxysporum, P. neglecta, and A. alternata were determined as described by Xu et al. (2021) with slight modifications. Ten percent Tween 80 (v/v) was used as a cosolvent to add JEO, CWEO and CPEO to the PDA media at final concentrations of 0, 2.5, 5, 10, 20, and 40 μL/mL for each treatment. Mycelial plugs (5 mm in diameter) taken from 7-day-old fungal cultures were placed at the center of 60 mm Petri dishes, and the plates were then sealed and placed in a 25°C incubator in the dark. The percentage of mycelial growth was calculated after 5 d by measuring the radial growth diameter. Each treatment was performed in triplicate.
Effect of juniper essential oil on the spore germination of Botrytis cinerea
The hanging drop method (Qiu et al., 2014) was used to assess the effect of JEO on the germination of B. cinerea spores. A suspension of 20 μL of spores was mixed with 20 μL liquid medium potato dextrose broth (PDB) that contained JEO to final concentrations of 0, 1.25, 2.5, 5, 10, 20, and 40 μL/mL. A volume of 40 μL was added to the hemocytometer. The percentage of spore germination was recorded after incubation at 25°C for 12 h. Each treatment was conducted in triplicate, and five fields of view were randomly selected for microscopic examination in each replicate.
Effect of juniper essential oil at controlling gray mold in cherry tomato fruit
Fresh and healthy cherry tomato fruit were selected. Their surfaces were disinfected with 0.05% sodium hypochlorite for 2 min, and then rinsed with sterile distilled water 3 times for 30 s each time. The fruit were punctured in the middle (2 mm in diameter and 3 mm in depth) with a sterile stainless steel needle. A volume of 10 μL of JEO at concentrations of 40, 80, and 160 μL/mL were added to the wounds, while the control group was treated with Tween 80. Each concentration was considered as a different treatment, contained 10 tomatoes, and was repeated three times. The treated fruits were air-dried for 4 h, and 10 μL of spore suspension was added to the wound. They were then placed in 160 mm Petri dishes covered with plastic wrap and incubated at 25°C. After 4 d, the rate of incidence rate of the infection of cherry tomatoes was recorded, and the diameter of the lesions was determined using a Vernier caliper (Jiao et al., 2020).
Effects of juniper essential oil on extracellular conductivity and soluble protein leakage
Five mycelial plugs of B. cinerea (diameter 5 mm) were placed in 180 mL of PDB media and cultured shaking at 150 rpm at 25°C for 48 h. Add 20 mL of a mixture of JEO and Tween 80, and the cultivation was continued. The JEO content was 1.1216 and 0.865 mL respectively, and the final medication-containing medium concentrations were 5.608 μL/mL (EC50) and 4.325 μL/mL (EC30). The control group contained an equal amount of Tween 80. Mycelial samples and supernatant were collected 3, 6, 9, and 12 h after dosing. The mycelia were filtered through a sterile silk cloth, rinsed three times with 0.9% NaCl, and dried with sterile filter paper for later use. A DDS-11 digital conductivity meter (Shanghai Precision Scientific Instrument Co., Ltd., Shanghai, China) was used to measure the extracellular conductivity (μS/cm). Soluble protein leakage was measured as described by Bradford (1976). Each treatment was conducted in triplicate.
Effects of juniper essential oil on the contents of malondialdehyde and hydrogen peroxide
The membrane damage of B. cinerea owing to exposure to JEO was assessed in more detail by measuring the contents of MDA and hydrogen peroxide (H2O2). The mycelia obtained were processed following the kit instructions and measured using an N600 double-beam UV/Vis spectrophotometer (Shanghai Ruckus Instrument Co., Ltd., Shanghai, China). Each treatment was conducted in triplicate.
Effect of juniper essential oil on enzyme activities
The mycelia and supernatant were collected as described above and processed following the kit instructions. The enzyme activities including superoxide dismutase (SOD), catalase (CAT), polygalacturonase (PG), pectin lyase (PL), and endo-β-1-4-glucanase (EG) were determined according to the manufacturer’s instructions, using an N600 dual-beam UV/Vis spectrophotometer.
Mycelial morphology observation
A scanning electron microscope (SEM) was used to study the effect of JEO on mycelial morphology during the growth of B. cinerea. One disc (7.5 mm in diameter) of the mycelial plug was inoculated into PDA media that contained 0 and 5.608 μL/mL JEO. A sterilized coverslip was then inserted at a 45° angle at 2–3 cm from the mycelial plug and cultured upside down for 5 d. After the mycelia grew on the coverslip, the samples were processed as described by Li et al. (2017b) with slight modifications and observed at 10,000 × magnification under an SEM.
Gas chromatography/mass spectrometry analysis
The composition of JEO was analyzed by GC-MS, JEO diluted 1:100 with hexane, Model 7890A–7000B (Agilent Technologies Inc., USA). Helium was used as the carrier gas at a flow rate of 1 mL/min, with a split ratio of 1:40 using an HP-5MS (30 × 0.25 mm id, 0.25μm film thickness) capillary column, the temperature at the inlet was 250°C. The GC column temperature was programmed as follows: Initial temperature 60°C, hold for 1 min, increase to 250°C at 3°C/min for 5 min. Ionization voltage: 70 eV, full scan.
Statistical analysis
All the experiments were performed in triplicate. Microsoft Excel 2019 (Redmond, WA, USA) was used to calculate the mean values and standard deviations (n = 3). The effective concentration that inhibited 50% of the mycelial growth (EC50) and its corresponding 95% confidence limits were estimated by Probit analysis using SPSS 26.0 (IBM, Inc., Armonk, NY, USA). In addition, a one-way analysis of variance (ANOVA) was performed, and statistical significance was set as P < 0.05. Origin 9.8 (OriginLab, Inc., Northampton, MA, USA) and Microsoft Publisher 2010 (Redmond, USA) were used to prepare the graphics.
Results
Juniper essential oil, cedarwood essential oil and cypress essential oil inhibit the mycelial growth of phytopathogenic fungi
Compared with the control groups, the growth of fungi was inhibited in the three essential oils. The inhibitory effects of JEO, CWEO, and CPEO on the mycelial growth of phytopathogenic fungi are shown in Figure 1. The three essential oils inhibited the mycelial growth in a concentration-dependent manner, and there was a significant difference between the effects of essential oils at high concentrations (40 μL/mL) and low concentrations (2.5 μL/mL) (P < 0.05). In addition, different agents at the same concentration have varying inhibitory effects on pathogens. For example, 40 μL/mL of the three essential oils inhibited F. oxysporum by 74.2, 20.2, and 19.8%, respectively. The EC50 values and the virulence regression equation of the three essential oils against the four phytopathogenic fungi are shown in Table 1.
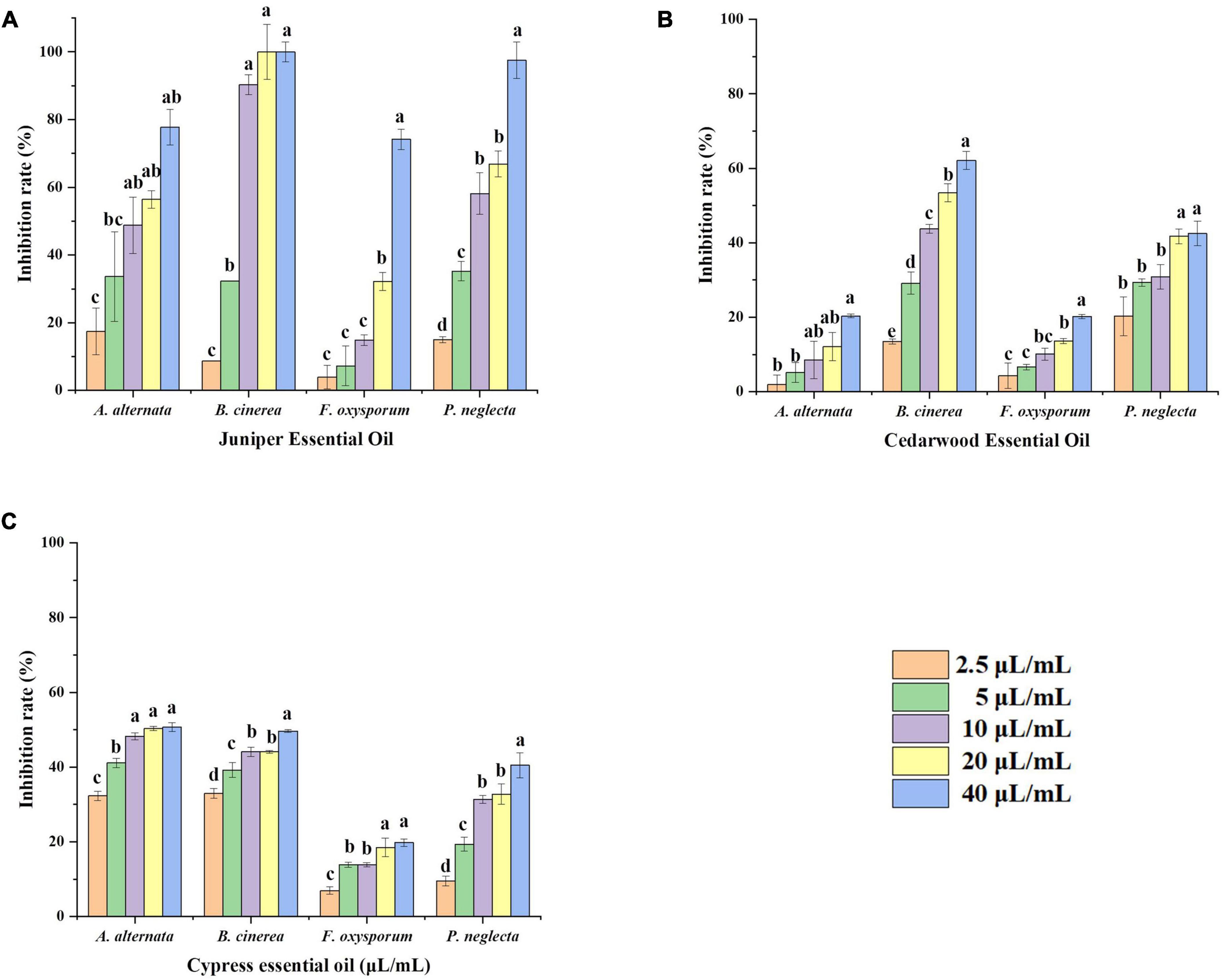
Figure 1. Effect of different concentrations of plant essential oils on the rate of inhibition of mycelial growth of four pathogenic fungi. Mycelial growth inhibition rates were calculated for JEO (A) CWEO (B), and CPEO (C) after treatment with different concentrations (0, 2.5, 5, 10, 20, and 40 μL/mL) of plant essential oils and incubated for 5 d at 25°C. Bars represent the standard error of the mean (n = 3). Letters (a, b, c, d) indicate statistically significant differences between concentrations according to Duncan’s multiple range test (P < 0.05). CPEO, cypress essential oil; CWEO, cedar wood essential oil; JEO, juniper essential oil.
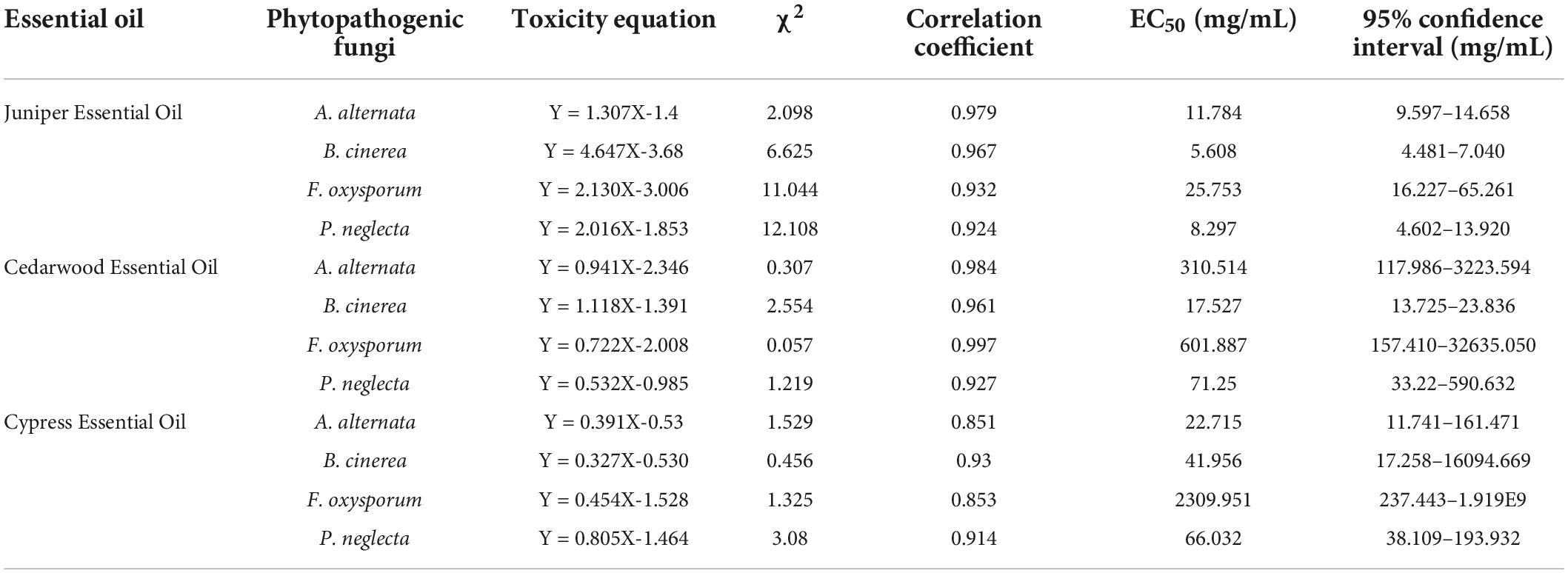
Table 1. Toxicity equations for juniper essential oil, cedarwood essential oil, and cypress essential oil against Alternaria alternata, Botrytis cinerea, Fusarium oxysporum, and Pestalotiopsis neglecta.
Effect of juniper essential oil on the spore germination of Botrytis cinerea
The germination of B. cinerea spores was significantly inhibited by JEO at different concentrations (P < 0.05) as shown in Figure 2. After incubation for 12 h, 90% of the spores in the control group had germinated. The germinating spores in the 1.25 μL/mL JEO treatment were inhibited by 2.64%, while the spore germination was completely inhibited at concentrations of 10–40 μL/mL.
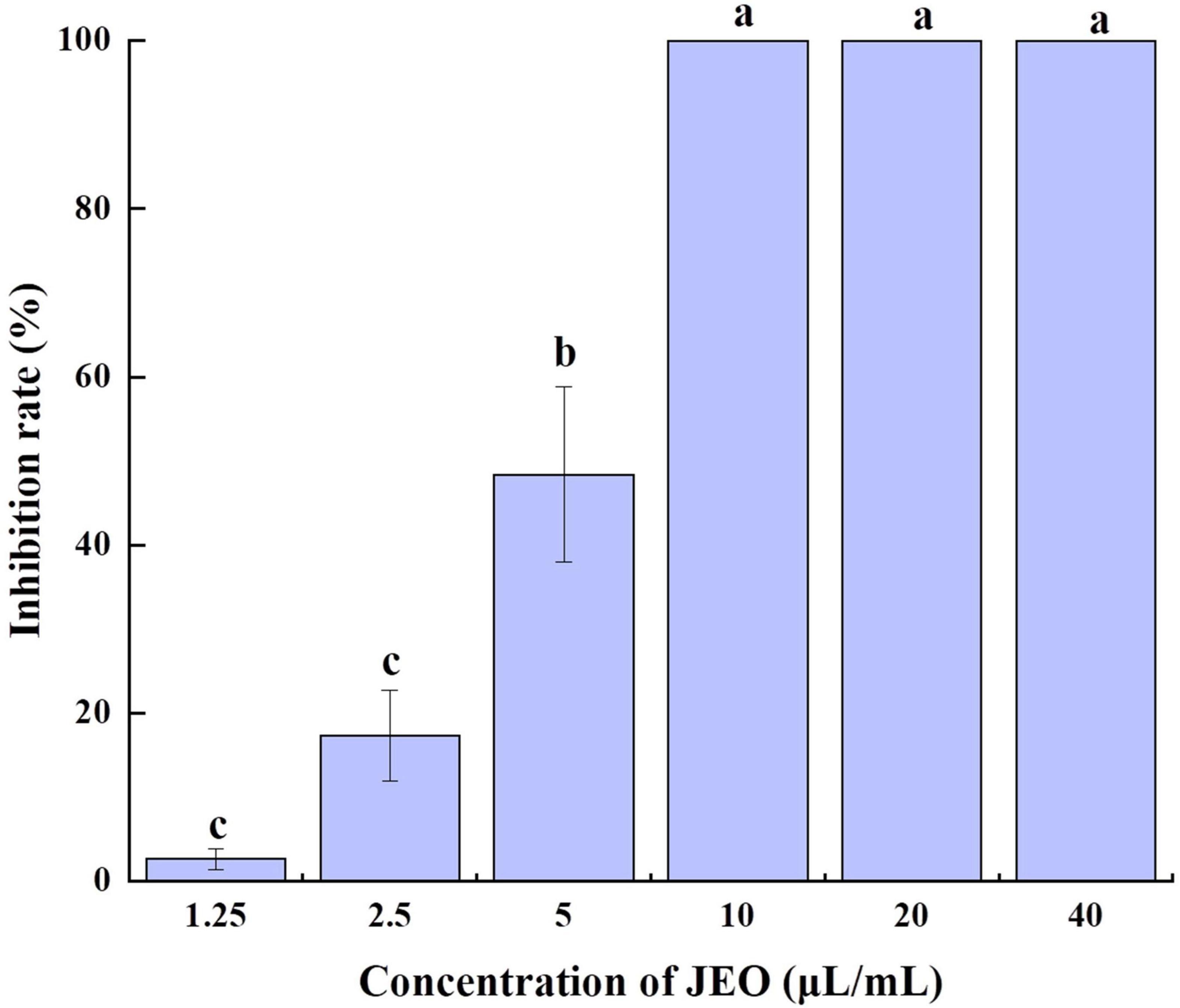
Figure 2. Effects of different concentrations of JEO on the spore germination of B. cinerea. The spores were treated with JEO at different concentrations (0, 1.25, 2.5, 5, 10, 20, and 40 μL/mL) and then incubated at 25 °C for 12 h. The bars represent the standard error of the mean (n = 3). Letters (a, b, c, d) represent statistically significant differences between different concentrations according to Duncan’s multiple range test (P < 0.05). JEO, juniper essential oil.
Ability of juniper essential oil to control gray mold in cherry tomato fruit
As shown in Figure 3, JEO inhibits the lesion diameters or disease incidence of gray mold caused by B. cinerea on cherry tomato fruit. The incidence of gray mold on cherry tomato fruit was significantly reduced (P < 0.05) when the amount of JEO was increased from 0 (control) to 160 μL/mL. However, there was no significant difference in lesion diameter after treatment with 40 and 80 μL/mL of JEO, respectively. The disease incidence and lesion diameter were reduced by 72.2 and 66.4% in the 160 μL/mL JEO treatment group, respectively.
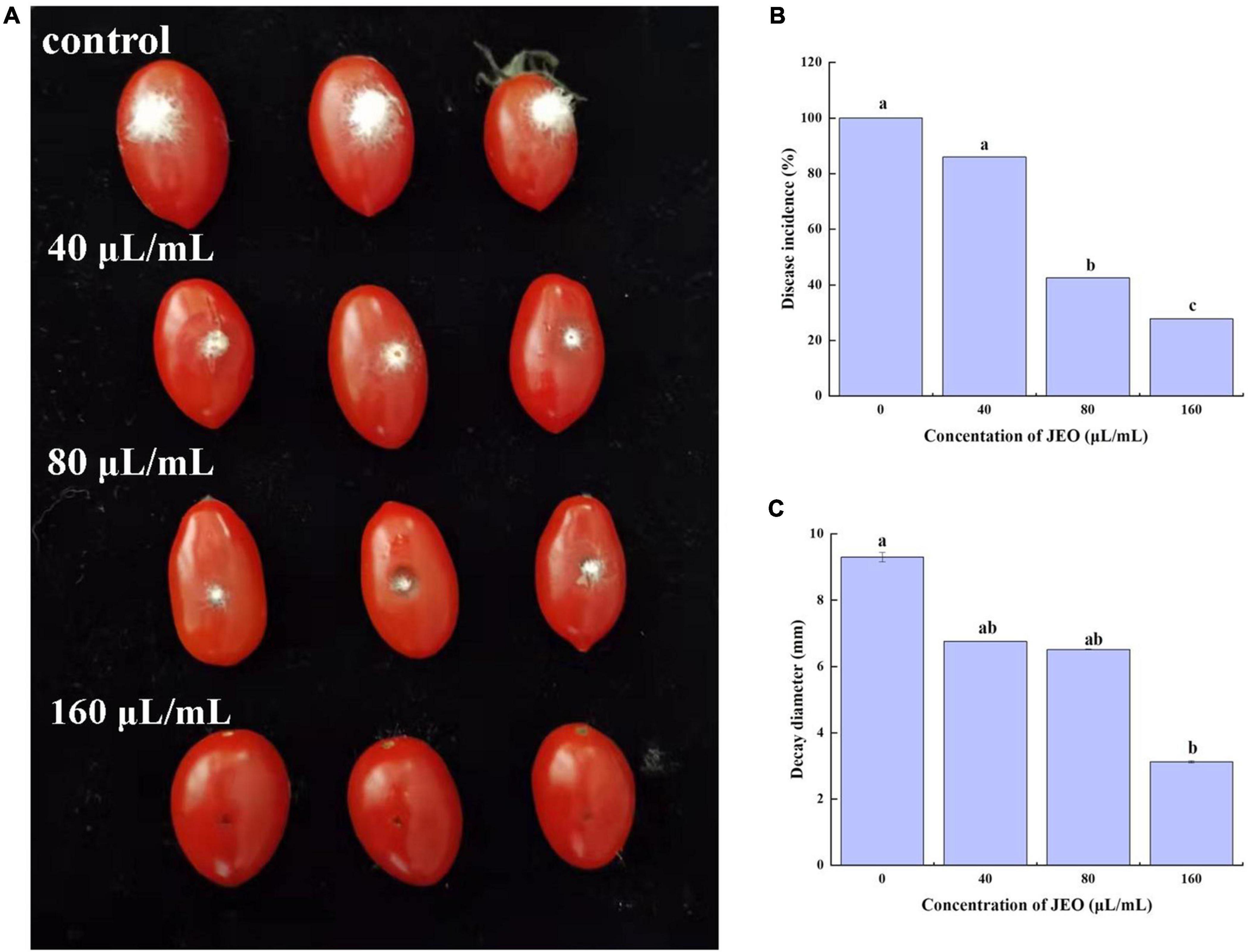
Figure 3. Effect of JEO on infection with B. cinerea in cherry tomato fruits after harvest. Each cherry tomato was inoculated with a 10 μL spore suspension of B. cinerea at 1 × 106 spores/mL and then treated with JEO at different concentrations (0 as control, 40, 80, and 160 μL/mL) for 4 d at 25°C. The representative photographs (A), disease incidence (B) and decay diameter (C) of the cherry tomatoes were recorded on day 4 after inoculation. The bars represent the standard error of the mean (n = 3). Letters (a, b, c, d) represent statistically significant differences between different concentrations according to Duncan’s multiple range test (P < 0.05). JEO, juniper essential oil.
Effects of juniper essential oil on extracellular conductivity and soluble protein leakage
As shown in Figure 4A, the value of extracellular conductivity in the control group was maintained at a low level for 12 h despite a slight increase. However, the values for the treatment group with JEO increased significantly with time (P < 0.05). The conductivity value of the EC50 treatment was 1.45- and 3.1-fold higher than that of the EC30 and control group, respectively, after 12 h of incubation. The data indicated that JEO can damage the cell membranes of B. cinerea.
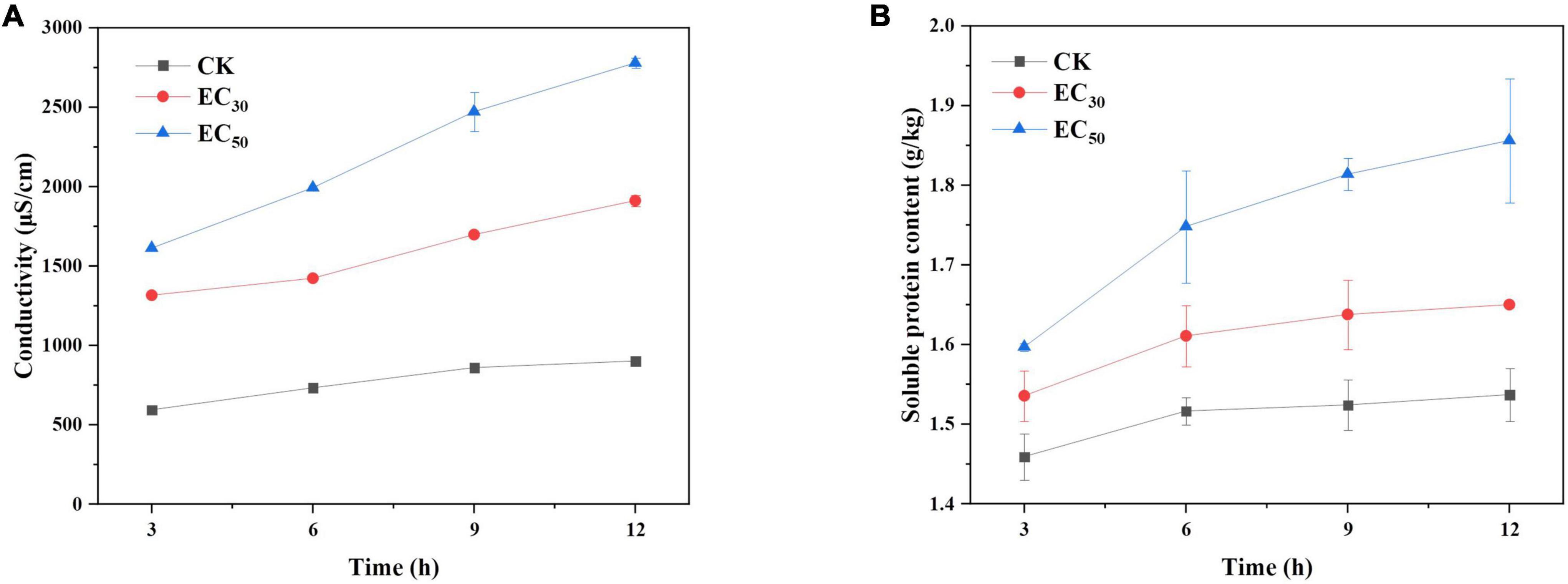
Figure 4. Effect of JEO on extracellular conductivity (A) and soluble protein content (B) of B. cinerea mycelia during 12 h of incubation. B. cinerea was treated with different concentrations of JEO (0 as control, EC30, and EC50). The bars represent the standard error of the mean (n = 3).
The effect of JEO on soluble protein leakage of B. cinerea is shown in Figure 4B. The trend was similar to the extracellular conductivity in a time- and concentration-dependent manner. The content of soluble protein was significantly higher than that of the control group after the EC50 treatment for 12 h (P < 0.05), indicating that a high concentration of JEO aggravated the leakage of soluble proteins of B. cinerea.
Effects of juniper essential oil on the contents of malondialdehyde and hydrogen peroxide
MDA and H2O2 are major markers for the peroxidation of membrane lipids. The contents of MDA and H2O2 in B. cinerea treated with JEO are shown in Figure 5. They were relatively low and had changed little in the control group. However, the treatment groups were significantly higher than those of the control group (P < 0.05) in a time-dependent manner. The contents of MDA and H2O2 of B. cinerea in the EC50 treatment were 7.2- and 4.7-fold higher than those of the control group, respectively, after 12 h incubation.
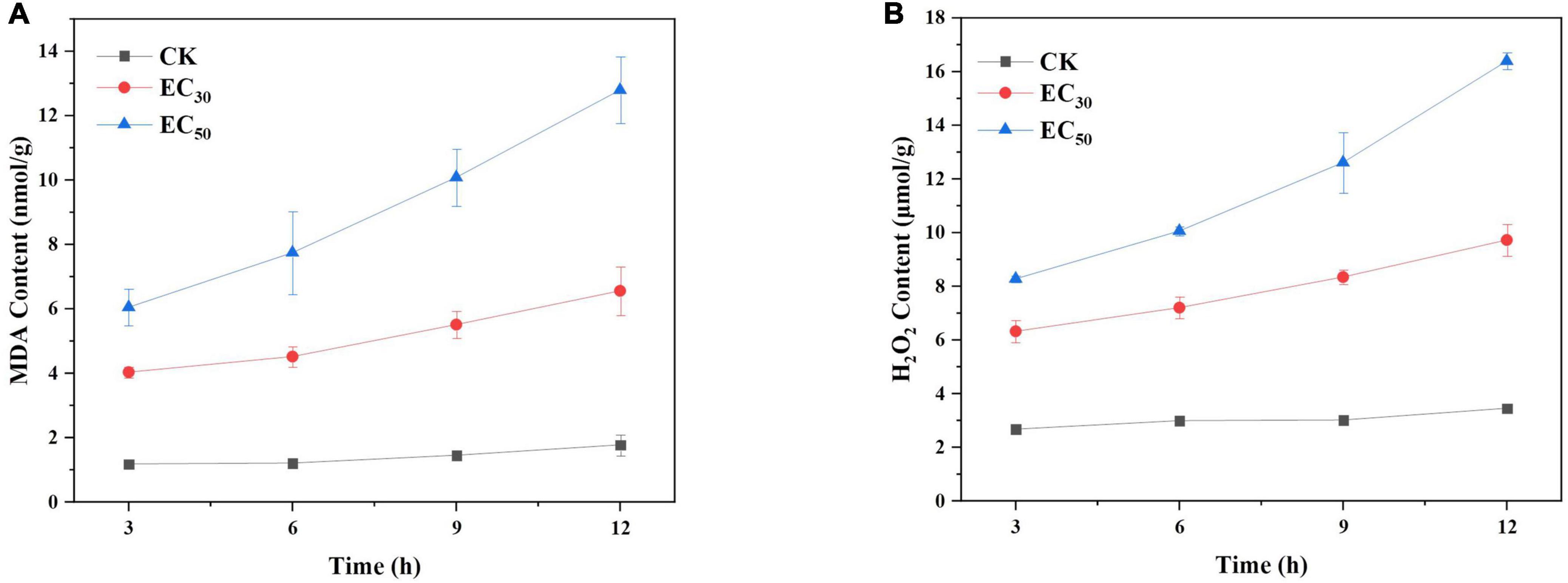
Figure 5. Effects of JEO on MDA content (A) and H2O2 content (B) from B. cinerea mycelia during 12 h of incubation. The mycelia were treated with different concentrations of JEO (0 as control, EC30, and EC50). The bars represent the standard error of the mean (n = 3). JEO, juniper essential oil; MDA, malondialdehyde.
Effect of juniper essential oil on the enzyme activity of Botrytis cinerea
Effects of juniper essential oil on the activity of antioxidative-related enzymes of Botrytis cinerea
As shown in Figure 6, the SOD and CAT activities in B. cinerea treated with JEO were significantly higher than those in the control groups and tended to increase followed by a tendency to decrease within 12 h. The maximum values occurred at 9 h and were significantly higher than those at other times (P < 0.05). The SOD and CAT of B. cinerea treated with EC50 were 9.6- and 9.5-fold higher than those of the control at 9 h, respectively.
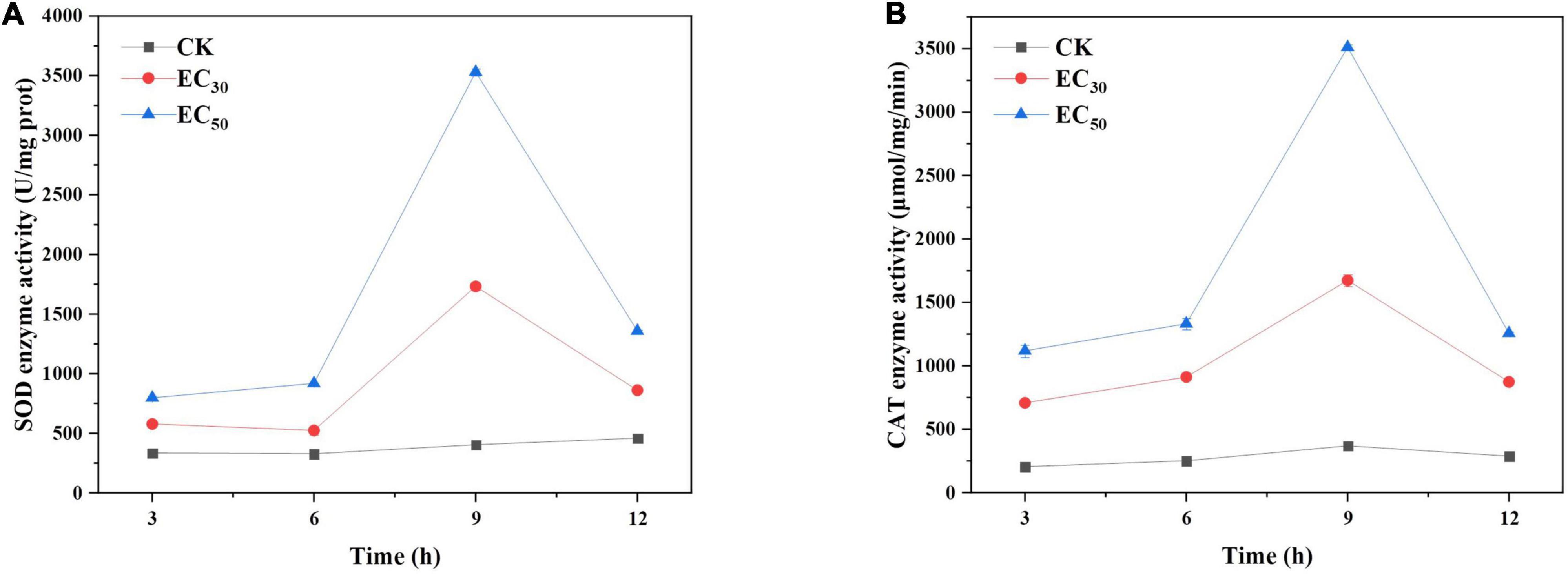
Figure 6. Effects of JEO on the activities of CAT (A) and SOD (B) secreted from B. cinerea mycelia during 12 h of incubation. B. cinerea was treated with different concentrations of JEO (0 as control, EC30, and EC50). The bars represent the standard error of the mean (n = 3). CAT, catalase; JEO, juniper essential oil; SOD, superoxide dismutase.
Effect of juniper essential oil on the pathogenic enzyme activities of Botrytis cinerea
The activities of enzymes related to pathogenicity (EG, PG, and PL) were significantly lower than those of the control groups following treatment with JEO (P < 0.05), respectively (Figure 7). In addition, they decreased gradually as the time of treatment was prolonged. Compared with the control group, the activities of EG, PG and PL decreased by 51.5 and 85.6%; 52.1 and 93%; and 54.2 and 87% following treatment with the EC30 and EC50 of JEO at 12 h, respectively.
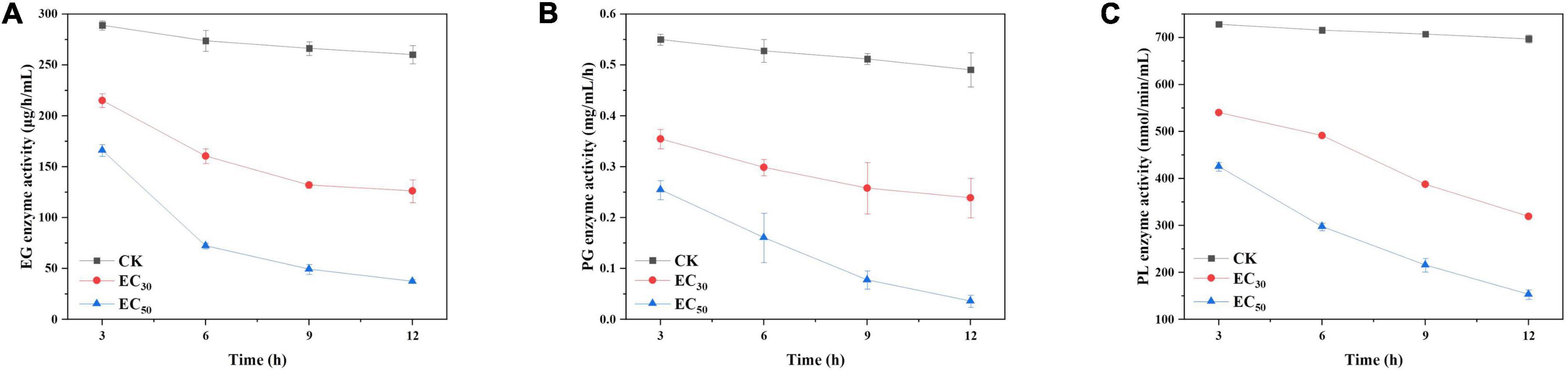
Figure 7. Effects of JEO on the enzyme activities of EG (A), PG (B), and PL (C) secreted from B. cinerea following treatment with different concentrations of JEO (0 as control, EC30, and EC50). The bars represent the standard error of the mean (n = 3). EG, endoglucanase; JEO, juniper essential oil; PG, polygalacturonase; PL, pectin lyase.
Effects of juniper essential oil on mycelial morphology
To explore whether the mycelial morphology of B. cinerea was affected by JEO (0 and EC50), SEM was utilized to evaluate the morphological alteration. Observation at 10,000 × magnification showed that the untreated hyphae were shaped normally, uniformly thick, smooth on their surface, intact, and undamaged (Figure 8A). However, the mycelia were severely shrunken, distorted, uneven in thickness, and broken after treatment with the EC50 of JEO (Figure 8B).
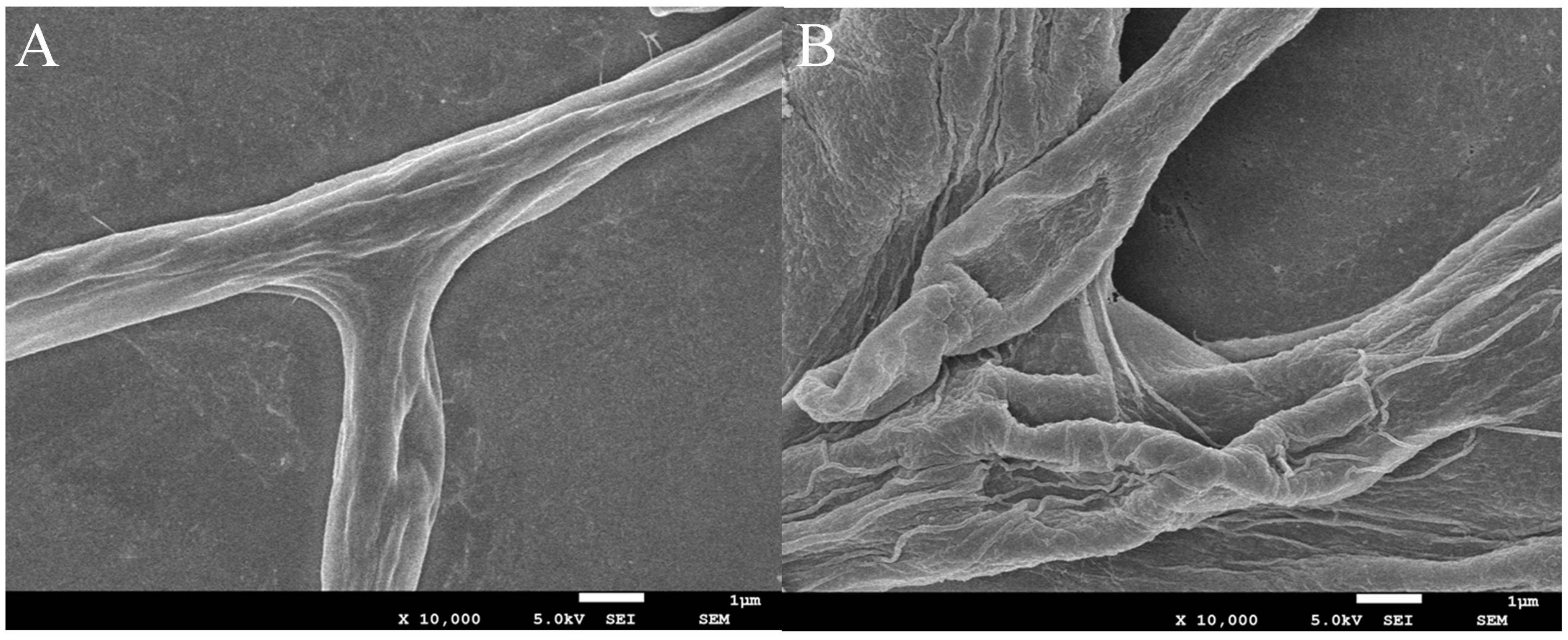
Figure 8. Effect of JEO on the morphology of B. cinerea. Mycelia treated with sterilized distilled water as the control (A) and EC50 of JEO (B) were observed by scanning electron microscopy (SEM) at 10,000× magnification. JEO, juniper essential oil.
Gas chromatography/mass spectrometry analysis of the active components in juniper essential oil
The composition of JEO was identified by GC-MS analysis. Eighteen compounds were identified by comparing the mass spectra, relative molecular masses, retention times, and molecular formulae with the NIST library (Table 2). Eighteen compounds accounted for 93.99% of the total content, with terpenes as the main component (51.02%), including limonene (15.71%), 2-carene (10.94%),γ-Terpinene (8.3%), β-Myrcene (4.56%), α-Phellandrene (4.13%), Terpinolene (0.12%), 3-carene (0.07%), α-pinene (0.06%), Humulene (0.06%), Longifolene (0.13%), Camphene (3.89%) and (+)-2-Bornanone (3.05%). This was followed by higher levels of alcohols (34.62%) and alkanes (8.35%) including terpinen-4-ol (24.26%), linalool (8.73%), α-terpineol (1.03%), dl-menthol (0.49%), isoborneo (0.11%), o-cymene (8.35%).
Discussion
Juniper has a wide range of medicinal and commercial values as a renewable resource (Kuang et al., 2019). JEO is a collection of natural compounds extracted from the needles of juniper and is primarily composed of terpenes, similar in chemical composition to juniper berry essential oil (JEBO), which has antibacterial and antioxidant properties (Pandey et al., 2018; Bajac et al., 2022). JEO can inhibit both Gram-positive (Bacillus cereus and B. subtilis) and Gram-negative bacteria (Pseudomonas aeruginosa and Salmonella typhimurium) (Falcão et al., 2018). In addition, studies have found that JEO can inhibit Klebsiella pneumoniae, leading to protein leakage and cellular deformation (Meng et al., 2016). JEO is widely available, more accessible and has a greater value for exploitation. Therefore, investigating the inhibitory effect of JEO on pathogenic fungi and its possible mechanism provides theoretical support for the additional development of JEO as an environmentally friendly antifungal agent.
In this study, all three PEOs showed different degrees of fungal inhibitory activity, but JEO was the most effective at inhibiting B. cinerea. JEO effectively inhibited mycelial growth, spore germination, and the incidence of the gray mold of cherry tomatoes in a concentration-dependent manner. These results are similar to the in vitro and in vivo inhibition of B. cinerea caused by sodium pheophorbide a (SPA) and tea tree oil (TTO) (Li et al., 2017a; Ji et al., 2020).
The cell membrane acts as a barrier to protect the cell by regulating the exchange of compounds inside and outside of the cell and maintaining the stability of the intracellular environment (Tao et al., 2014b). It is the main target for the action of PEOs. For example, the essential oil of mandarin orange (Citrus reticulata) disrupted the permeability of the cell membranes of Penicillium italicum and P. digitatum, leading to the leakage of cell contents and an increase in extracellular conductivity and total lipid content (Tao et al., 2014a). Mint and thyme essential oils reduce the ability of Rhizopus stolonifer to infect strawberries by disrupting cell membrane integrity and causing membrane lipid peroxidation (Yan et al., 2021). JEO treatment of Klebsiella pneumoniae causes the cells to implode and leak proteins and nucleic acids (Meng et al., 2016). Consistent with the results of this study, JEO similarly increased the extracellular conductivity and soluble protein content of B. cinerea, indicating that JEO disrupts the cell membrane integrity of B. cinerea.
MDA and H2O2 are key regulators of the oxidative stress response, and their levels are important parameters that reflect the potential antioxidant capacity of an organism, as well as the degree of cell membrane damage. The terpenoids cembratrien-diols and hinokitiol have been shown to cause an increase in MDA content and induce membrane lipid peroxidation in B. cinerea (Wang et al., 2020; Yang et al., 2020). SEM observations revealed that JEO caused alterations in the ultrastructure of the mycelia of B. cinerea, with crumpled and broken mycelial surfaces. Relevant studies suggest that JEO may inhibit B. cinerea by disrupting its cell membranes (Xu et al., 2021).
In addition, the activities of antioxidant-related enzymes SOD and CAT of B. cinerea increased significantly after 9 h of treatment with JEO but decreased at 12 h. This indicates that JEO causes some degree of oxidative stress in B. cinerea and has antioxidant capacity. This corresponds to the findings of Zheljazkov et al. (2017). However, the cell wall degrading enzymes (PL, PG, and EG) of B. cinerea gradually decreased with increasing time after treatment with JEO. The cell wall degrading enzymes (CWDEs)including cellulase (CL), PG, PL help the pathogenic fungus invade their host by degrading pectin and cellulose, which causes the plant cell walls to rupture (Chinisaz et al., 2014; Zhang et al., 2021). A decrease in the enzyme activities of CWDEs indicates a reduction in the ability of pathogenic fungi to infect their hosts. Yang et al. (2021) found that the pathogenicity-related enzyme activity of both B. cinerea and A. alternata decreased after treatment with vanillin. Similarly, PG activity was lower in P. neglecta treated with SPA than that in the control (Yang et al., 2019), which was consistent with the results of our study.
The 18 compounds in JEO were determined by GC-MS. Previous studies have found that limonene, β-myrcene, and linalool are present in the volatile oils of citrus fruit peels and inhibit Gram-negative bacteria (Enterobacter aerogenes, Escherichia coli), Gram-positive bacteria (Bacillus cereus), fungi (Saccharomyces cerevisiae, Candida Albicans) and viral (Gupta et al., 2021; Sevindik et al., 2021). The citrus essential oil contains γ-terpinene which inhibits the fungus Alternaria, Hypocrea, Trichoderma, and Geosmithia on pine wood, protecting it from discoloration (Martínez-Pacheco et al., 2022). Limonene, α-pinene, and β-pinene are the main components of the essential oil from Campomanesia adamantium (Cambess.) O. Berg leaves during flowering. It has high antibacterial activity against Staphylococcus aureus, Pseudomonas aeruginosa, and Candida albicans (Coutinho et al., 2009). Foeniculum vulgare Miller essential oil containing o-cymene, α-phellandrene, and α-pinene has anti-candida activity (Garzoli et al., 2018) α-terpineol present in Thymus vulgaris essential oil is a typical terpenoid that inhibits Penicillium fingertip resulting in damage to its cell membrane and cell wall (Jing et al., 2015). (-)-4-Terpineol is present in the essential oil of Melaleuca alternifolia and has inhibitory effects on Aspergillus ochraceus (Kong et al., 2019), yeast, and filamentous fungi (Pinto et al., 2014). These substances are also the main components of JEO, laying the foundation for its antimicrobial effect of JEO.
Conclusion
In conclusion, this study revealed the in vivo and in vitro antifungal activity of JEO against gray mold on cherry tomatoes caused by B. cinerea. Moreover, JEO inhibits B. cinerea by disrupting the permeability of cell membranes, causing oxidative damage, altering mycelial morphology, and inhibiting pathogenesis-related enzyme activity. Therefore, JEO has the potential to prevent and control gray mold in cherry tomatoes and even other plant diseases.
Data availability statement
The original contributions presented in this study are included in the article/supplementary material, further inquiries can be directed to the corresponding authors.
Author contributions
Y-XW: methodology, investigation, and writing—original draft. Y-DZ: investigation, data curation, and writing—review and editing. NL: validation. D-DW: methodology. Q-ML: validation. Y-ZC: data curation. G-CZ and JY: resources, conceptualization, methodology, and supervision. All authors contributed to the article and approved the submitted version.
Funding
This work was supported by the Central Financial Fund for Forestry Science and Technology Promotion (grant nos. H[2021]TG12 and H[2021]TG08) and the Natural Science Special (Special Post) Research Fund of Guizhou University (grant no. [2022]02). The funding sources had no role in the study design (collection, analysis, and interpretation of data), report writing, and decision to submit the article for publication.
Conflict of interest
The authors declare that the research was conducted in the absence of any commercial or financial relationships that could be construed as a potential conflict of interest.
Publisher’s note
All claims expressed in this article are solely those of the authors and do not necessarily represent those of their affiliated organizations, or those of the publisher, the editors and the reviewers. Any product that may be evaluated in this article, or claim that may be made by its manufacturer, is not guaranteed or endorsed by the publisher.
References
AlMotwaa, S. M., and Al-Otaibi, W. A. (2022). Determination of the chemical composition and antioxidant, anticancer, and antibacterial properties of essential oil of Pulicaria crispa from Saudi Arabia. J. Indian Chem. Soc. 99:100341. doi: 10.1016/j.jics.2022.100341
Álvarez, A., Manjarres, J. J., Ramírez, C., and Bolívar, G. (2021). Use of an exopolysaccharide-based edible coating and lactic acid bacteria with antifungal activity to preserve the postharvest quality of cherry tomato. Lwt 151:112225. doi: 10.1016/j.lwt.2021.112225
Bajac, J., Nikolovski, B., Lončarević, I., Petrović, J., Bajac, B., Ðurović, S., et al. (2022). Microencapsulation of juniper berry essential oil (Juniperus communis L.) by spray drying: microcapsule characterization and release kinetics of the oil. Food Hydrocolloids 125:107430. doi: 10.1016/j.foodhyd.2021.107430
Bradford, M. M. (1976). A rapid and sensitive method for the quantitation of microgram quantities of protein utilizing the principle of protein-dye binding. Anal. Biochem. 72, 248–254. doi: 10.1016/0003-2697(76)90527-3
Chinisaz, M., Ghasemi, A., Larijani, B., and Ebrahim-Habibi, A. (2014). Amyloid formation and inhibition of an all-beta protein: A study on fungal polygalacturonase. J. Mol. Struct. 1059, 94–100. doi: 10.1016/j.molstruc.2013.11.011
Coutinho, I. D., Lima Cardoso, C. A., Re-Poppi, N., Melo, A. M., Vieira, M. d. C, Honda, N. K., et al. (2009). Gas Chromatography-Mass Spectrometry (GC-MS) and evaluation of antioxidant and antimicrobial activities of essential oil of Campomanesia adamantium (Cambess.) O. Berg (Guavira). Braz. J. Pharm Sci. 45, 767–776. doi: 10.1590/s1984-82502009000400022
Falcão, S., Bacém, I., Igrejas, G., Rodrigues, P. J., Vilas-Boas, M., and Amaral, J. S. (2018). Chemical composition and antimicrobial activity of hydrodistilled oil from juniper berries. Indust. Crops Products 124, 878–884. doi: 10.1016/j.indcrop.2018.08.069
Feng, X., Liu, J., Zhang, Y., Wu, W., Pan, Y., Wang, D., et al. (2020). Podophyllotoxin profiles combined with SRAP molecular markers in Juniperus rigida: A promising alternative source of podophyllotoxin. Indust. Crops Products 153:112547. doi: 10.1016/j.indcrop.2020.112547
Garzoli, S., Bozovic, M., Baldisserotto, A., Sabatino, M., Cesa, S., Pepi, F., et al. (2018). Essential oil extraction, chemical analysis and anti-Candida activity of Foeniculum vulgare Miller - new approaches. Nat. Prod. Res. 32, 1254–1259. doi: 10.1080/14786419.2017.1340291
Ghorbanzadeh, A., Ghasemnezhad, A., Sarmast, M. K., and Ebrahimi, S. N. (2021). An analysis of variations in morphological characteristics, essential oil content, and genetic sequencing among and within major Iranian Juniper (Juniperus spp.) populations. Phytochemistry 186:112737. doi: 10.1016/j.phytochem.2021.112737
Gordien, A. Y., Gray, A. I., Franzblau, S. G., and Seidel, V. (2009). Antimycobacterial terpenoids from Juniperus communis L. (Cuppressaceae). J. Ethnopharmacol. 126, 500–505. doi: 10.1016/j.jep.2009.09.007
Guerra, I. C. D., de Oliveira, P. D. L., de Souza Pontes, A. L., Lucio, A., Tavares, J. F., Barbosa-Filho, J. M., et al. (2015). Coatings comprising chitosan and Mentha piperita L. or Mentha x villosa Huds essential oils to prevent common postharvest mold infections and maintain the quality of cherry tomato fruit. Int. J. Food Microbiol. 214, 168–178. doi: 10.1016/j.ijfoodmicro.2015.08.009
Guo, X. X., Zhao, D., Zhuang, M. H., Wang, C., and Zhang, F. S. (2021). Fertilizer and pesticide reduction in cherry tomato production to achieve multiple environmental benefits in Guangxi, China. Sci. Total Environ. 793:148527. doi: 10.1016/j.scitotenv.2021.148527
Gupta, A., Jeyakumar, E., and Lawrence, R. (2021). Journey of limonene as an antimicrobial agent. J. Pure Appl. Microbiol. 15, 1094–1110. doi: 10.22207/jpam.15.3.01
Hong, Y., Liu, X., Wang, H., Zhang, M., and Tian, M. (2022). Chemical composition, anticancer activities and related mechanisms of the essential oil from Alpinia coriandriodora rhizome. Indust. Crops Products 176:114328. doi: 10.1016/j.indcrop.2021.114328
Hu, F., Tu, X. F., Thakur, K., Hu, F., Li, X. L., Zhang, Y. S., et al. (2019). Comparison of antifungal activity of essential oils from different plants against three fungi. Food Chem. Toxicol. 134:110821. doi: 10.1016/j.fct.2019.110821
Ji, D., Chen, T., Ma, D., Liu, J., Xu, Y., and Tian, S. (2018). Inhibitory effects of methyl thujate on mycelial growth of Botrytis cinerea and possible mechanisms. Postharvest Biol. Technol. 142, 46–54. doi: 10.1016/j.postharvbio.2018.04.003
Ji, J. Y., Yang, J., Zhang, B. W., Wang, S. R., Zhang, G. C., and Lin, L. N. (2020). Sodium pheophorbide a controls cherry tomato gray mold (Botrytis cinerea) by destroying fungal cell structure and enhancing disease resistance-related enzyme activities in fruit. Pestic. Biochem. Physiol. 166:104581. doi: 10.1016/j.pestbp.2020.104581
Jiao, W., Liu, X., Chen, Q., Du, Y., Li, Y., Yue, F., et al. (2020). Epsilon-poly-l-lysine (ε-PL) exhibits antifungal activity in vivo and in vitro against Botrytis cinerea and mechanism involved. Posthar. Biol. Technol. 168:111270. doi: 10.1016/j.postharvbio.2020.111270
Jing, G. X., Tao, N. G., Jia, L., and Zhou, H. E. (2015). Influence of alpha-terpineol on the growth and morphogenesis of Penicillium digitatum. Bot. Stud. 56:35. doi: 10.1186/s40529-015-0116-4
Kim, C.-S., Jo, K., and Kim, J. S. (2010). Anti-lipase and Lipolytic Activities of EtOH Extract from Juniperus rigida. Kor. J. Pharmacog. 41, 216–220.
Kong, Q., Zhang, L., An, P., Qi, J., Yu, X., Lu, J., et al. (2019). Antifungal mechanisms of alpha-terpineol and terpene-4-alcohol as the critical components of Melaleuca alternifolia oil in the inhibition of rot disease caused by Aspergillus ochraceus in postharvest grapes. J. Appl. Microbiol. 126, 1161–1174. doi: 10.1111/jam.14193
Kuang, S., Liu, L., Qing, M., Zhang, Y., Feng, X., Wang, D., et al. (2019). Anatomical, phytochemical, and histochemical study of Juniperus rigida needles at different altitudes. Microsc. Microanal. 25, 1213–1223. doi: 10.1017/s1431927619014818
Li, Y., Shao, X., Xu, J., Wei, Y., Xu, F., and Wang, H. (2017a). Effects and possible mechanism of tea tree oil against Botrytis cinerea and Penicillium expansum in vitro and in vivo test. Can. J. Microbiol. 63, 219–227. doi: 10.1139/cjm-2016-0553
Li, Y., Shao, X., Xu, J., Wei, Y., Xu, F., and Wang, H. (2017b). Tea tree oil exhibits antifungal activity against Botrytis cinerea by affecting mitochondria. Food Chem. 234, 62–67. doi: 10.1016/j.foodchem.2017.04.172
Ma, B.-X., Ban, X.-Q., He, J.-S., Huang, B., Zeng, H., Tian, J., et al. (2016). Antifungal activity of Ziziphora clinopodioides Lam. essential oil against Sclerotinia sclerotiorum on rapeseed plants (Brassica campestris L.). Crop Protect. 89, 289–295. doi: 10.1016/j.cropro.2016.07.003
Martínez-Pacheco, M. M., Reynoso, W. E. G., León, J. C. d, González, D. R., García, A. F., Rojas, A. M., et al. (2022). Pinewood protection against sapstain using citrus essential oils. Rev. Árvore 46: e4616. doi: 10.1590/1806-908820220000016
Meng, X., Li, D., Zhou, D., Wang, D., Liu, Q., and Fan, S. (2016). Chemical composition, antibacterial activity and related mechanism of the essential oil from the leaves of Juniperus rigida Sieb. et Zucc against Klebsiella pneumoniae. J. Ethnopharmacol. 194, 698–705. doi: 10.1016/j.jep.2016.10.050
Morales-Rabanales, Q. N., Coyotl-Pérez, W. A., Rubio-Rosas, E., Cortes-Ramírez, G. S., Sánchez Ramírez, J. F., and Villa-Ruano, N. (2022). Antifungal properties of hybrid films containing the essential oil of Schinus molle: Protective effect against postharvest rot of tomato. Food Control 134:108766. doi: 10.1016/j.foodcont.2021.108766
Pandey, S., Tiwari, S., Kumar, A., Niranjan, A., Chand, J., Lehri, A., et al. (2018). Antioxidant and anti-aging potential of Juniper berry (Juniperus communis L.) essential oil in Caenorhabditis elegans model system. Indust. Crops Products 120, 113–122. doi: 10.1016/j.indcrop.2018.04.066
Park, J. Y., Kim, S. H., Kim, N. H., Lee, S. W., Jeun, Y. C., and Hong, J. K. (2017). Differential inhibitory activities of four plant essential oils on in vitro growth of Fusarium oxysporum f. sp. fragariae Causing Fusarium Wilt in Strawberry Plants. Plant Pathol. J. 33, 582–588. doi: 10.5423/PPJ.OA.06.2017.0133
Pinto, E., Gonçalves, M. J., Oliveira, P., Coelho, J., Cavaleiro, C., and Salgueiro, L. (2014). Activity of Thymus caespititius essential oil and α-terpineol against yeasts and filamentous fungi. Indust. Crops Products 62, 107–112. doi: 10.1016/j.indcrop.2014.08.004
Qiu, M., Wu, C., Ren, G., Liang, X., Wang, X., and Huang, J. (2014). Effect of chitosan and its derivatives as antifungal and preservative agents on postharvest green asparagus. Food Chem. 155, 105–111. doi: 10.1016/j.foodchem.2014.01.026
Raut, J. S., and Karuppayil, S. M. (2014). A status review on the medicinal properties of essential oils. Indust. Crops Products 62, 250–264. doi: 10.1016/j.indcrop.2014.05.055
Raynaldo, F. A., Dhanasekaran, S., Ngea, G. L. N., Yang, Q., Zhang, X., and Zhang, H. (2021). Investigating the biocontrol potentiality of Wickerhamomyces anomalus against postharvest gray mold decay in cherry tomatoes. Sci. Horticult. 285:110137. doi: 10.1016/j.scienta.2021.110137
Rguez, S., Ben Slimene, I., Abid, G., Hammemi, M., Kefi, A., Elkahoui, S., et al. (2020). Tetraclinis articulata essential oil reduces Botrytis cinerea infections on tomato. Sci. Horticult. 266:109291. doi: 10.1016/j.scienta.2020.109291
Romanazzi, G., Smilanick, J. L., Feliziani, E., and Droby, S. (2016). Integrated management of postharvest gray mold on fruit crops. Postharv. Biol. Technol. 113, 69–76. doi: 10.1016/j.postharvbio.2015.11.003
Sevindik, E., Aydın, S., Sujka, M., Apaydın, E., Yıldırım, K., and Palas, G. (2021). GC-MS analysis and evaluation of antibacterial and antifungal activity of essential oils extracted from fruit Peel of Citrus aurantium L. (Rutaceae) Grown in the West Anatolian Area. Erwerbs-Obstbau 63, 135–142. doi: 10.1007/s10341-021-00549-7
Tao, N., Jia, L., and Zhou, H. (2014a). Anti-fungal activity of Citrus reticulata Blanco essential oil against Penicillium italicum and Penicillium digitatum. Food Chem. 153, 265–271. doi: 10.1016/j.foodchem.2013.12.070
Tao, N., OuYang, Q., and Jia, L. (2014b). Citral inhibits mycelial growth of Penicillium italicum by a membrane damage mechanism. Food Control 41, 116–121. doi: 10.1016/j.foodcont.2014.01.010
Touba, E. P., Zakaria, M., and Tahereh, E. (2012). Anti-fungal activity of cold and hot water extracts of spices against fungal pathogens of Roselle (Hibiscus sabdariffa) in vitro. Microb. Pathog. 52, 125–129. doi: 10.1016/j.micpath.2011.11.001
Wang, Y., Liu, X., Chen, T., Xu, Y., and Tian, S. (2020). Antifungal effects of hinokitiol on development of Botrytis cinerea in vitro and in vivo. Postharv. Biol. Technol. 159:111038. doi: 10.1016/j.postharvbio.2019.111038
Wang, Y., Yu, T., Xia, J., Yu, D., Wang, J., and Zheng, X. (2010). Biocontrol of postharvest gray mold of cherry tomatoes with the marine yeast Rhodosporidium paludigenum. Biol. Control 53, 178–182. doi: 10.1016/j.biocontrol.2010.01.002
Xu, Y., Wei, J., Wei, Y., Han, P., Dai, K., Zou, X., et al. (2021). Tea tree oil controls brown rot in peaches by damaging the cell membrane of Monilinia fructicola. Postharv. Biol. Technol. 175:111474. doi: 10.1016/j.postharvbio.2021.111474
Yan, J., Wu, H., Shi, F., Wang, H., Chen, K., Feng, J., et al. (2021). Antifungal activity screening for mint and thyme essential oils against Rhizopus stolonifer and their application in postharvest preservation of strawberry and peach fruits. J. Appl. Microbiol. 130, 1993–2007. doi: 10.1111/jam.14932
Yang, J., Chen, Y. Z., Yu-Xuan, W., Tao, L., Zhang, Y. D., Wang, S. R., et al. (2021). Inhibitory effects and mechanisms of vanillin on gray mold and black rot of cherry tomatoes. Pestic. Biochem. Physiol. 175:104859. doi: 10.1016/j.pestbp.2021.104859
Yang, J., Lin, L. N., Zhang, X. B., Wu, Y., Bi, B., Zhang, G. C., et al. (2019). Sodium pheophorbide a has photoactivated fungicidal activity against Pestalotiopsis neglecta. Pestic. Biochem. Physiol. 158, 25–31. doi: 10.1016/j.pestbp.2019.04.003
Yang, Q., Wang, J., Zhang, P., Xie, S., Yuan, X., Hou, X., et al. (2020). In vitro and in vivo antifungal activity and preliminary mechanism of cembratrien-diols against Botrytis cinerea. Indust. Crops Products 154:112745. doi: 10.1016/j.indcrop.2020.112745
Zhang, J., Yu, X., Zhang, C., Zhang, Q., Sun, Y., Zhu, H., et al. (2021). Pectin lyase enhances cotton resistance to Verticillium wilt by inducing cell apoptosis of Verticillium dahliae. J. Hazard Mater. 404:124029. doi: 10.1016/j.jhazmat.2020.124029
Zhao, Y., Yang, Y. H., Ye, M., Wang, K. B., Fan, L. M., and Su, F. W. (2021). Chemical composition and antifungal activity of essential oil from Origanum vulgare against Botrytis cinerea. Food Chem. 365:130506. doi: 10.1016/j.foodchem.2021.130506
Zheljazkov, V. D., Kacaniova, M., Dincheva, I., Radoukova, T., Semerdjieva, I. B., Astatkie, T., et al. (2018). Essential oil composition, antioxidant and antimicrobial activity of the galbuli of six juniper species. Indust. Crops Products 124, 449–458. doi: 10.1016/j.indcrop.2018.08.013
Keywords: juniper essential oil, antifungal activity, Botrytis cinerea, cherry tomato, gray mold, enzyme activity, GC-MS
Citation: Wu Y-X, Zhang Y-D, Li N, Wu D-D, Li Q-M, Chen Y-Z, Zhang G-C and Yang J (2022) Inhibitory effect and mechanism of action of juniper essential oil on gray mold in cherry tomatoes. Front. Microbiol. 13:1000526. doi: 10.3389/fmicb.2022.1000526
Received: 22 July 2022; Accepted: 31 August 2022;
Published: 23 September 2022.
Edited by:
Hui Ni, Jimei University, ChinaReviewed by:
Md Aktaruzzaman, University of Georgia, United StatesYingying Wei, Ningbo University, China
Zhigang Ouyang, Gannan Normal University, China
Copyright © 2022 Wu, Zhang, Li, Wu, Li, Chen, Zhang and Yang. This is an open-access article distributed under the terms of the Creative Commons Attribution License (CC BY). The use, distribution or reproduction in other forums is permitted, provided the original author(s) and the copyright owner(s) are credited and that the original publication in this journal is cited, in accordance with accepted academic practice. No use, distribution or reproduction is permitted which does not comply with these terms.
*Correspondence: Guo-Cai Zhang, emhhbmc2NDAzMDhAMTI2LmNvbQ==; Jing Yang, amluZ3lhbmdAZ3p1LmVkdS5jbg==