- 1Department of Microbial and Biochemical Pharmacy, School of Pharmacy, China Medical University, Shenyang, China
- 2Division of Food and Drug Evaluation Science, Kobe University Graduate School of Medicine, Kobe, Japan
Aberration in the control of cell cycle contributes to the development and progression of many diseases including cancers. Ksg1 is a Schizosaccharomyces pombe fission yeast homolog of mammalian phosphoinositide-dependent protein kinase 1 (PDK1) which is regarded as a signaling hub for human tumorigenesis. A previous study reported that Ksg1 plays an important role in cell cycle progression, however, the underlying mechanism remains elusive. Our genomic library screen for novel elements involved in Ksg1 function identified two serine/threonine kinases, namely SAD family kinase Cdr2 and another PDK1 homolog Ppk21, as multicopy suppressors of the thermosensitive phenotype of ksg1-208 mutant. We found that overexpression of Ppk21 or Cdr2 recovered the defective cell cycle transition of ksg1-208 mutant. In addition, ksg1-208 Δppk21 cells showed more marked defects in cell cycle transition than each single mutant. Moreover, overexpression of Ppk21 failed to recover the thermosensitive phenotype of the ksg1-208 mutant when Cdr2 was lacking. Notably, the ksg1-208 mutation resulted in abnormal subcellular localization and decreased abundance of Cdr2, and Ppk21 deletion exacerbated the decreased abundance of Cdr2 in the ksg1-208 mutant. Intriguingly, expression of a mitotic inducer Cdc25 was significantly decreased in ksg1-208, Δppk21, or Δcdr2 cells, and overexpression of Ppk21 or Cdr2 partially recovered the decreased protein level of Cdc25 in the ksg1-208 mutant. Altogether, our findings indicated that Cdr2 is a novel downstream effector of PDK1 homologs Ksg1 and Ppk21, both of which cooperatively participate in regulating cell cycle progression, and Cdc25 is involved in this process in fission yeast.
Introduction
The eukaryotic cell cycle, as one of the most important and evolutionary conserved processes of cells, plays a crucial role in the growth, development, repair, and reproduction of living organisms. Dysregulation of cell cycle procession caused by mutation or aberrant gene expression can cause a myriad of cellular pathologies including defects in cell shape, abnormal cell growth and aneuploidy, potentially leading to the occurrence and development of cancer (Abbastabar et al., 2018; Zheng et al., 2019). Therefore, a better understanding of regulatory mechanisms on cell cycle procession will provide a theoretical basis for further research on cell cycle-related diseases and promote the development of therapeutic strategies.
The 3-phosphoinositide-dependent protein kinase 1 (PDK1) is a widely conserved serine/threonine-protein kinase in eukaryotes. Alterations of PDK1 functions have a relevant role in pathologies such as Alzheimer’s disease, diabetes, and cancer (Hashimoto et al., 2006; Pietri et al., 2013; Jiang Q. et al., 2021). Increasing pieces of evidence have shown that the expression of PDK1 is dysregulated in multiple cancer types, and PDK1 is implicated in signaling pathways frequently altered in cancer (Gagliardi et al., 2012; Zabkiewicz et al., 2014; Bai et al., 2016; Wang et al., 2016). PDK1 has been known to play a central role in cellular signaling by phosphorylating members of the AGC family of kinases, including protein kinase C (PKC), protein kinase B (PKB), p70/p90 ribosomal S6 kinases (RSK and S6K), and the catalytic subunit of cAMP-dependent protein kinase (PKA) (Alessi et al., 1997; Stokoe et al., 1997; Mora et al., 2004). Several other proteins not belonging to the AGC kinase family were also reported to participate in the PDK1 signaling pathway (Gagliardi et al., 2015), however, the detailed mechanisms are not well understood. Therefore, further studies are still required to gain an insight into the PDK1 signaling pathway, which may pave a way for further understanding the molecular mechanisms of the PDK1 regulatory network and providing clues for rational PDK1 targeting therapies in the future.
The fission yeast Schizosaccharomyces pombe (S. pombe) has been known for years to be an excellent model system for the investigation of the fundamental regulations and control mechanisms of various cellular processes, due to its high homology with mammals and advantages in gene manipulation (Forsburg, 2005; Liu et al., 2018, 2020; Jiang G. et al., 2021; Vyas et al., 2021). Ksg1 (kinase responsible for sporulation and growth 1), which is essential for the cell viability in fission yeast, is a homologous protein of mammalian PDK1 (Niederberger and Schweingruber, 1999). It has been reported that decrease of Ksg1 kinase activity reduces cell wall integrity and mating efficiency of fission yeast significantly, which is mediated by the Ksg1 substrates Pck1/Pck2 (pombe C kinase-like 1/2) and Pka1 (protein kinase A1) (Matsuo et al., 2003; Tang and Mcleod, 2004; Madrid et al., 2014). Remarkably, the Ksg1 substrates in fission yeast are homologous to that of PDK1 in mammalian cells (Kobayashi and Cohen, 1999; Gagliardi et al., 2018), suggesting the significant conservation between Ksg1 and PDK1 signaling pathways. Thus, further studies of the Ksg1-regulated pathway could provide novel references for the PDK1 signaling network in higher eukaryotes. A previous study showed that a mutation of Ksg1 leads to the cell cycle arrest in G2/M transition under high temperature (Niederberger and Schweingruber, 1999), indicating the crucial role of Ksg1 in cell cycle regulation. However, the underlying mechanism by which Ksg1 regulates cell cycle progression has not been reported so far.
In this study, in an attempt to excavate new elements and reveal novel signaling pathways for Ksg1 in regulating cell cycle, we screened for multicopy suppressors of ksg1-208 mutant cells. Two genes encoding serine/threonine-protein kinases were identified, namely the SAD (synapses of the amphid defective) kinase Cdr2 (changed division response 2) (Young and Fantes, 1987; Crump et al., 2001) and another PDK1 homolog Ppk21 (putative protein kinases 21, also known as Pdk1) (Bimbó et al., 2005a). We investigated the role of Ppk21 and Cdr2 in the Ksg1-mediated cell cycle progression, and found that Ksg1 and Ppk21 cooperatively regulate cell cycle progression through Cdr2. Moreover, overexpression of ppk21+ or cdr2+ restored the decreased protein level of a mitotic inducer Cdc25 in the ksg1-208 mutant. Our results revealed a novel function of Ppk21 and Cdr2 in Ksg1-regulated cell cycle progression, which provides a theoretical basis for enriching the PDK1-associated regulatory network in humans. To our knowledge, this is the first demonstration of a cross-talk between PDK1 signaling and SAD kinase signaling.
Materials and Methods
Strains, Media, and Genetic and Molecular Biology Methods
The S. pombe strains used in this study are listed in Table 1. The complete medium yeast extract-peptone-dextrose (YPD) and the normal minimal medium Edinburgh minimal medium (EMM) have been described previously (Toda et al., 1997). Standard S. pombe genetic and recombinant-DNA methods were performed as described previously unless mentioned (Moreno et al., 1991). Gene disruptions are denoted by lower-case letters representing the disrupted gene followed by two colons and the wild-type gene marker used for disruption (for example, cdr2::ura4+). Gene disruptions are abbreviated by the gene preceded by Δ (for example, Δcdr2). Proteins are denoted by Roman letters and only the first letter is capitalized (for example, Cdr2).
Multicopy Suppressor Screen
To identify multicopy suppressor of the thermosensitivity of ksg1-208 mutant, a genomic library cloned into the vector pDB248 (Beach et al., 1982) was transformed into the ksg1-208 mutant cells. The Leu+ transformants were replica-plated onto YPD plates and incubated at 35°C for 4 days. The plasmid DNA was recovered from transformants that showed a plasmid-dependent rescue. Using DNA sequencing and sequence alignment, the suppressing genes were confirmed. Here we focus on the genes cdr2+ (SPAC57A10.02) and ppk21+ (SPBC1778.10c).
Gene Deletion
To knockout the cdr2+ gene, a one-step gene disruption by homologous recombination was performed (Rothstein, 1989). The cdr2::ura4+ disruption was constructed as follows. The 2.3-kb cdr2+ fragment with BamHI site at both ends, which was amplified by PCR using primers cdr2+ sense/ cdr2+ antisense (Table 2) and the genomic DNA of wild-type cells as a template, was subcloned into the BamHI site of BlueScriptSK(+). Then, an ura4+ fragment was inserted into the SphI site of the previous construct. The fragment containing the disrupted cdr2+ gene was transformed into haploid cells. Stable integrants were selected on medium without uracil, and the disruption was confirmed by PCR and Southern blot (our unpublished data). We generated ksg1-208 Δcdr2 double mutant by a genetic cross between ksg1-208 and cdr2::ura4+.
To knockout the ppk21+ gene, we used the similar method to that of Cdr2 disruption. The 1.9-kb XhoI/NotI fragment containing ppk21+ gene was amplified by PCR using primers ppk21+ sense/ ppk21+ antisense (Table 2) and the genomic DNA of wild-type cells as a template, and then was inserted into the XhoI/NotI sites of BlueScriptSK(+). Then, BamHI digested ura4+ fragment was inserted into the BglII site of ppk21+ in the previous construct. The ura4+ disrupted ppk21+ fragment was transformed into haploid cells, and the stable integrants were selected on medium without uracil, and the disruption was confirmed by PCR and Southern blot (our unpublished data). A genetic cross was employed between ksg1-208 and ppk21::ura4+ to generate ksg1-208 Δppk21 double mutant.
Gene Expression
To construct overexpression plasmids, the cdr2+ and ppk21+ genes were amplified by PCR using the genomic DNA of wild-type cells as a template. The primers were used as mentioned above (Table 2). Then, the cdr2+ fragment was inserted into the BamHI restriction site of the pREP1 expression vector, which harbors a thiamine-repressible nmt1+ promoter, while the ppk21+ fragment was inserted into the XhoI and NotI restriction sites of the pREP1 expression vector. These constructs were validated by sequencing, and were further confirmed to be fully functional as demonstrated by their complementation of the phenotypes associated with Δcdr2 or Δppk21, respectively (our unpublished data).
To examine the effect of Ksg1 or Ppk21 on the subcellular localization or protein abundance of Cdr2, we constructed the chromosome integrated strains by genetic cross using strain expressing Cdr2-mEGFP with ksg1-208, ppk21::ura4+ or their double mutant, respectively, to generate ksg1-208 cdr2-mEGFP::KanMX6, ppk21::ura4+ cdr2-mEGFP::KanMX6, or ksg1-208 ppk21::ura4+ cdr2-mEGFP::KanMX6.
To investigate the effect of Ksg1, Ppk21, or Cdr2 on the abundance of Cdc25 protein, we constructed CM244, CM568, and CM574 by genetic cross between cdc25-6HA::ura4+ and ksg1-208, ppk21::KanMX4 or cdr2::natR, respectively.
Microscopy and Miscellaneous Methods
Methods in light microscopy, such as fluorescence microscopy and differential interference contrast (DIC) microscopy, were performed as described previously (Kita et al., 2004; Fang et al., 2008) by using a Nikon Eclipse Ni-U microscope equipped with a DS-Qi2 camera (Nikon Instruments Inc., Japan). Cell length and septation index were measured using the ImageJ software.1 Database searches were performed using the National Center for Biotechnology Information BLAST network service and the fission yeast S. pombe database search service.2
Immunoblot Assays
Cells expressing Cdr2-mEGFP or Cdc25-HA under wild-type or mutant background were cultured at 27°C in normal EMM media with 0.226 g/L leucine overnight to mid-log phase. The cdr2+ or ppk21+ overexpressed Cdc25-HA cells were incubated in normal EMM media to mid-log phase. Total proteins were extracted in buffer (92.5% 2 M NaOH, 7.5% β-mercaptoethanol), and precipitated by adding an equal volume of 50% trichloroacetic acid. After washing with Tris-HCl buffer, the resulting protein extracts were dissolved in sample buffer (62.5 mM Tris-HCl, pH 6.8, 2% SDS, 10% glycerine, 1 mM β-mercaptoethanol). Following resolution by SDS-polyacrylamide gel electrophoresis, proteins were transferred to a polyvinylidene difluoride membrane (Millipore, United States) under 150 mA constant current for 1 h in transfer buffer (12.5 mM Tris-HCl, 192 mM glycine, and 10% methanol; pH 8.3). Purified polyclonal anti-GFP an monoclonal anti-HA (Cell Signaling Technology, 1:10,000) antibodies were used to visualize protein bands.
Statistical Analysis
Quantitative data are expressed as the mean ± standard deviation of the mean (SD). Statistical analyses were performed using GraphPad Prism 8.3.0 (GraphPad Software, La Jolla, CA). One-way ANOVA and Tukey’s multiple range test were conducted to determine the significance of differences. For all statistical analyses, a single asterisk (*) denotes p-values < 0.05, two asterisks (**) indicate p-values < 0.01, three asterisks (***) indicate p-values < 0.001, and four asterisks (****) indicate p-value < 0.0001.
Results
Isolation of the ppk21+ and cdr2+ Genes as Multicopy Suppressors of ksg1-208 Mutant
Ksg1, a homolog of PDK1 which was recognized as a phosphoinositide signaling regulator, was reported to be responsible for the control of cell cycle previously, however, the mechanism remains elusive (Niederberger and Schweingruber, 1999). To identify novel genes that are involved in Ksg1 function, we performed a multicopy suppressor screen of the thermosensitive ksg1-208 strain. The strain has a single mutation (G159E) between the ATP binding domain and the active site of Ksg1, which results in decreased kinase activity and growth defects at restrictive temperature (Niederberger and Schweingruber, 1999). A high-copy genomic library bearing the Leu1+ marker (Beach et al., 1982) was used for selecting genes that when overexpressed could rescue the ksg1-208 growth defects at a restrictive temperature of 35°C. DNA sequencing identified the genomic insert of each suppressing plasmid, and two of them were investigated in this study, namely ppk21+ and cdr2+. The ppk21+ gene encodes a non-essential Pkh (PKB-activating kinase homologs) kinase Ppk21 which is another homologous protein of PDK1 in fission yeast, and the cdr2+ gene encodes an 85.97 kDa SAD family kinase Cdr2 (Breeding et al., 1998; Kanoh and Russell, 1998; Casamayor et al., 1999; Bimbó et al., 2005b). As shown in Figure 1, the ksg1-208 cells grew well in the YPD medium at a permissive temperature of 27°C, but failed to grow at 35°C, whereas wild-type cells grew well. Notably, overexpression of either ppk21+ or cdr2+ partially rescued the temperature sensitivity of the ksg1-208 cells at 35°C (Figures 1A,B). We also examined the effects of overexpression of another SAD kinase Cdr1 (Wu and Russell, 1993; Kanoh and Russell, 1998) on the growth defect of ksg1-208 cells at 35°C, and the results showed that overexpression of cdr1+ could not rescue the temperature sensitivity of ksg1-208 cells (Figure 1A). These results indicated that Ppk21 and Cdr2 might be involved in Ksg1-related cell growth regulation.
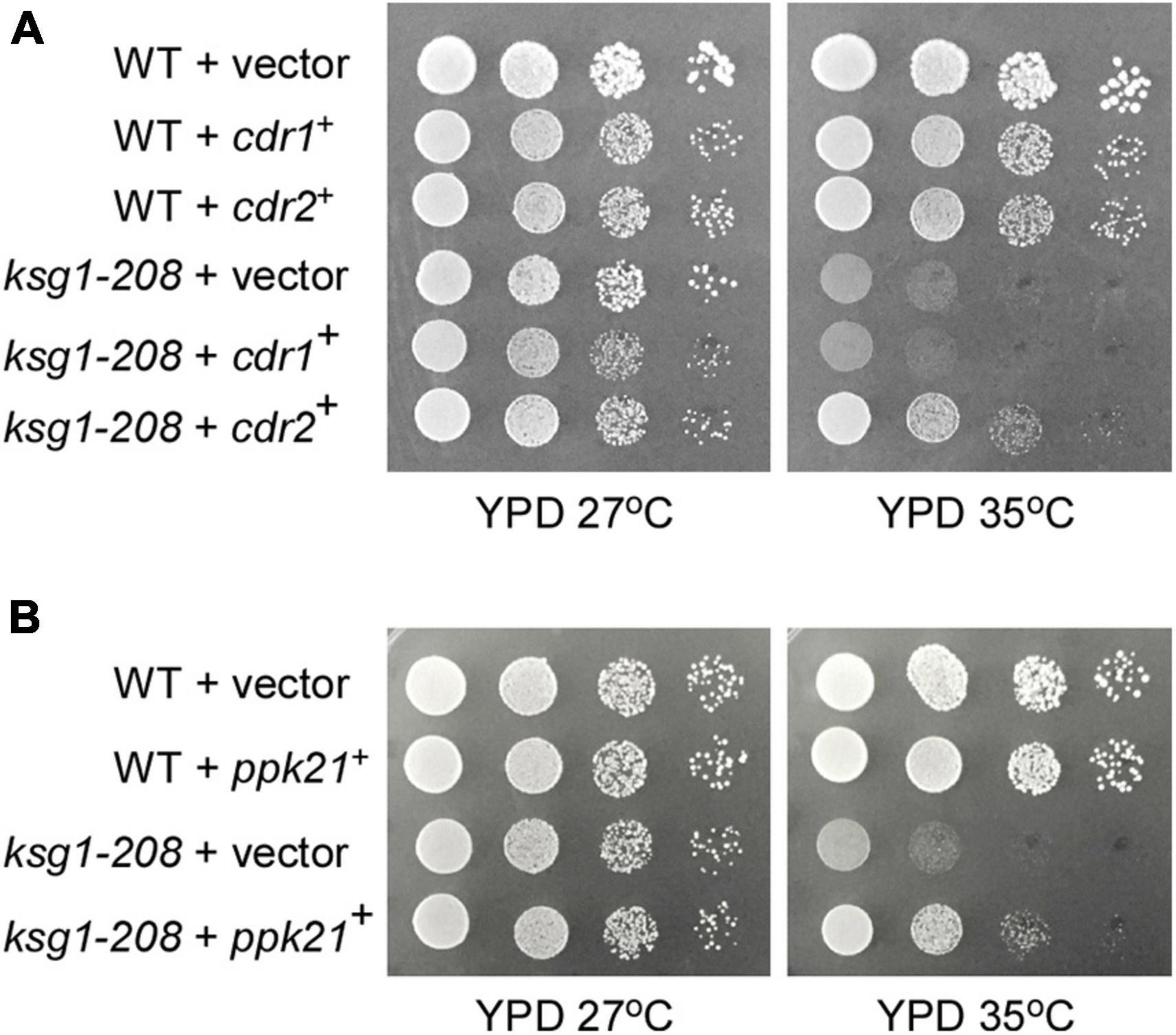
Figure 1. Isolation of Cdr2 and Ppk21 as multicopy suppressors of the ksg1-208 mutant cells. The wild type cells (WT) or ksg1-208 mutant cells were transformed with either the pDB248 empty vector or the vector containing cdr1+, cdr2+ (A) or ppk21+ (B). Cells were spotted onto YPD plated as indicated in serial 10-fold dilutions starting with OD660 = 0.3 of log-phase cells and then incubated for 4 days at 27°C or 35°C.
Ppk21 Shares a Redundant Function With Ksg1 in Cell Cycle Regulation
A previous study reported that cells deleted for ppk21+ displayed multiple defects in mitosis (Bimbó et al., 2005b), indicating an important role of Ppk21 in cell cycle regulation. This prompted us to investigate whether overexpression of ppk21+ played a role in repairing the defective G2/M transition of the ksg1-208 cells. Fission yeast cells enter into mitosis at a reproducible mean size of 13∼15 μm, and a longer cell length at division indicates the delay of G2/M transition, and vice versa (Mitchison and Nurse, 1985; Scotchman et al., 2021). Then we measured the cell length of the ksg1-208 cells at division, and determined the effects of Ppk21 overexpression on the cell length of ksg1-208 cells. Consistent with the notion that Ksg1 is important for cell cycle G2/M transition (Niederberger and Schweingruber, 1999; Matsuo et al., 2003), our results showed that the mean length of ksg1-208 cells was significantly longer than that of wild-type cells at 27°C (Figure 2A). Remarkably, overexpression of ppk21+ recovered the defects in the cell length of the ksg1-208 cells (Figure 2A). In view of the fact that septation initiates after mitosis, and to some extent, the ratio of septation reflects the proportion of cells that have gone through G2/M transition and mitosis, we further determined the effects of Ppk21 overexpression on the septation index of ksg1-208 cells. As shown in Figure 2B, the septation index of ksg1-208 cells decreased sharply at 35°C which was recovered by the overexpression of ppk21+ (Figure 2B). These results indicated that overexpressed ppk21+ helped the ksg1-208 cells to pass through the G2/M transition of the cell cycle.
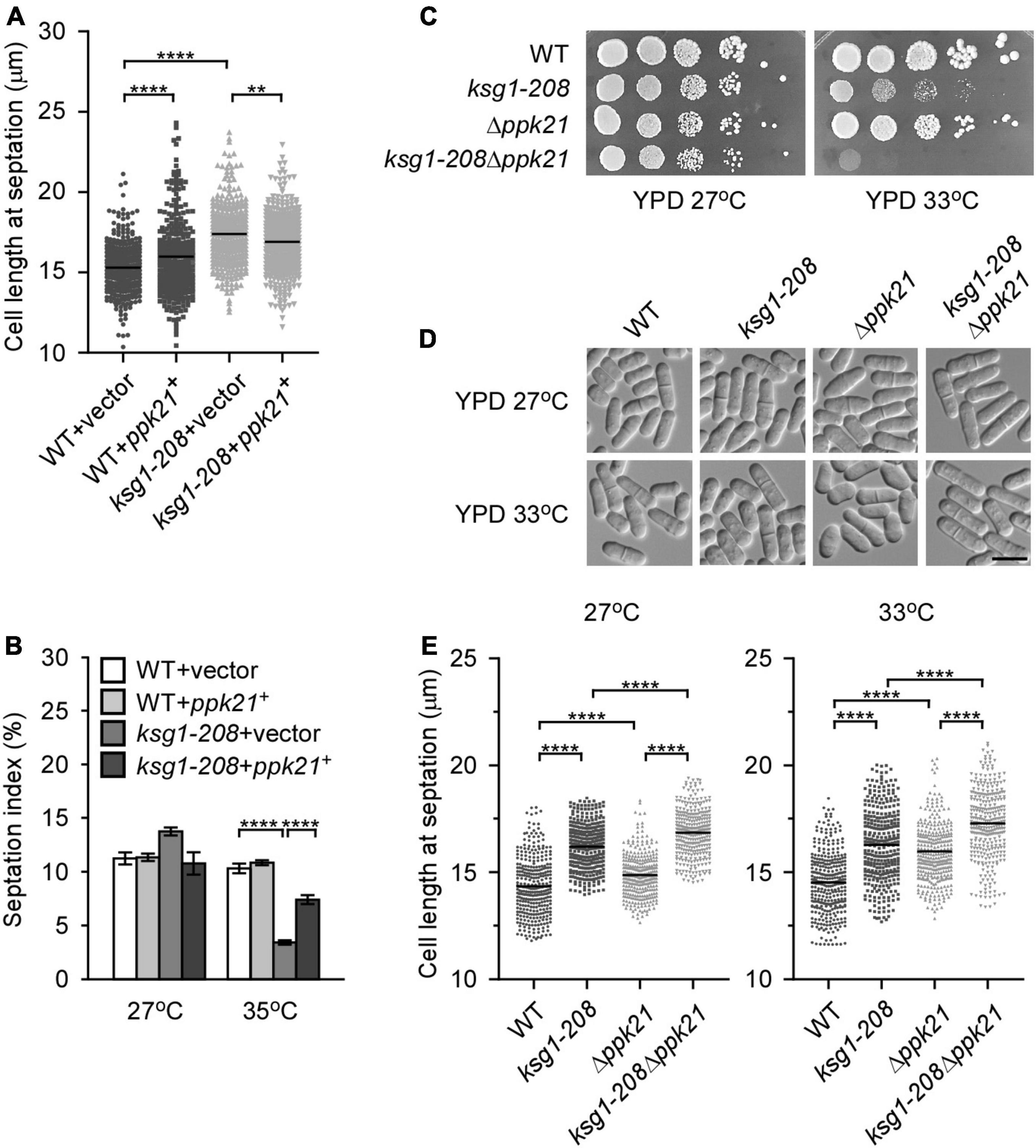
Figure 2. Ppk21 shares a redundant function with Ksg1 in cell cycle regulation. (A) Quantification of the cell length at septation for each ppk21+ overexpressed strains cultured with EMM plated without thiamine. Black bars represent mean (n ≥ 300). ** indicates p-value < 0.01 and **** indicates p-value < 0.0001. (B) Septation index of ppk21+ overexpressed strains cultured with EMM plated without thiamine (n ≥ 1000). Data represents mean (± SD) of three biological replicates. **** indicates p-value < 0.0001. (C) Wild-type, ksg1-208, Δppk21 or ksg1-208 Δppk21 cells were spotted onto YPD plates and then incubated for 4 days at 27°C or 33°C. (D) Live-cell images of wild-type, ksg1-208, Δppk21, or ksg1-208 Δppk21cells. Cells were grown to early log-phase in YPD plates at 27°C and then transferred to 33°C for 3 h and images were acquired using differential interference contrast (DIC) microscope immediately. Scale bar, 10 μm. (E) Quantification of the cell length at septation for each strain as indicated. Black bars represent mean (n ≥ 300). **** indicates p-value < 0.0001.
Since Ppk21 is another homologous protein of PDK1 in fission yeast (Bimbó et al., 2005b), we then asked whether Ppk21 functions redundantly with Ksg1 in cell cycle regulation. To determine the role of ppk21+ in Ksg1-regulated cell cycle progression, we constructed a double mutant of ksg1+ and ppk21+, namely ksg1-208 Δppk21. We then compared the phenotypes of ksg1-208 Δppk21 cells with Δppk21 or ksg1-208 cells. As shown in Figure 2C, the ksg1-208 Δppk21 cells showed more marked temperature sensitivity than either ksg1-208 cells or Δppk21 cells (Figure 2C). In addition, we performed the cell length assay, and the results showed that ksg1-208 Δppk21 cells exhibited a longer cell length than either ksg1-208 or Δppk21 cells at both 27 and 33°C (Figures 2D,E). These results indicated that deleting the ppk21+ gene exacerbated the cell cycle delay and temperature sensitivity of the ksg1-208 mutant, and Ppk21 shares a redundant function with Ksg1 in the cell cycle regulation. Meanwhile, we noticed that the septation ring at 33°C seems off-centered for the ksg1-208 Δppk21 mutant. To determine whether ksg1-208 mutation or Ppk21 deletion cause division site mis-positioning, we measured septum position in ksg1-208, Δppk21, and ksg1-208 Δppk21 cells by quantification of the ratio of the short to long daughter cell as described previously (Willet et al., 2019). The results showed that the septation ring of the ksg1-208 Δppk21 double mutant was off-centered at 33°C, which was more severe than that of ksg1-208 cells (Figure 2D and Supplementary Figure 1). Thus, not only G2 arrest but also mis-septation may be responsible for the growth defect of ksg1-208 Δppk21 double mutant.
Overexpression of cdr2+ Recovers the Defect of Cell Cycle Transition in ksg1-208 Mutant
The SAD kinase Cdr2 is a key component of the signaling network that prevents mitotic entry until cells reach a critical and reproducible size (Martin and Berthelot-Grosjean, 2009; Moseley et al., 2009; Allard et al., 2018). The pivotal role of Cdr2 in cell cycle regulation prompted us to investigate whether overexpression of cdr2+ rescued the defect of G2/M transition in ksg1-208 cells. We found that, similar to the ppk21+, overexpression of cdr2+ also reversed the defects in the cell length and the septation index of ksg1-208 cells (Figures 3A,B), suggesting that the mitotic entry returned to normal in cdr2+ overexpressed ksg1-208 cells. It should be noted that cdr2+ overexpressed wild-type cells showed a shorter cell length at 27°C, but a longer cell length at 35°C than cdr2+ non-overexpressed wild-type cells (Figure 3B). In addition, the cdr2+ overexpressed wild-type cells showed a higher septation index than cdr2+ non-overexpressed wild-type cells at 35°C (Figure 3C). A previous study reported that overexpression of cdr2+ in the wild-type strain was lethal and generated elongated, highly branched cells that contained two or more septa (Breeding et al., 1998). The differences between the previous report and our present data may be due to the use of wild-type cells with different auxotrophic properties and genetic backgrounds. To this end, we overexpressed cdr2+ in another wild type strain, KP93006, which possesses the same auxotrophic properties with the strain used in previous report (Kim et al., 2010), and then cultured the cells in the same condition together with the cdr2+ overexpressed wild type cells HM123 described in Figure 3C. Consistent with the previous report, our results showed that overexpression of cdr2+ in KP93006 generated elongated and branched cells, whereas overexpression of cdr2+ in HM123 showed a higher septation index (Supplementary Figure 2). Collectively, our results suggested that overexpression of cdr2+ rescued the defective cell cycle transition of ksg1-208 mutant cells.
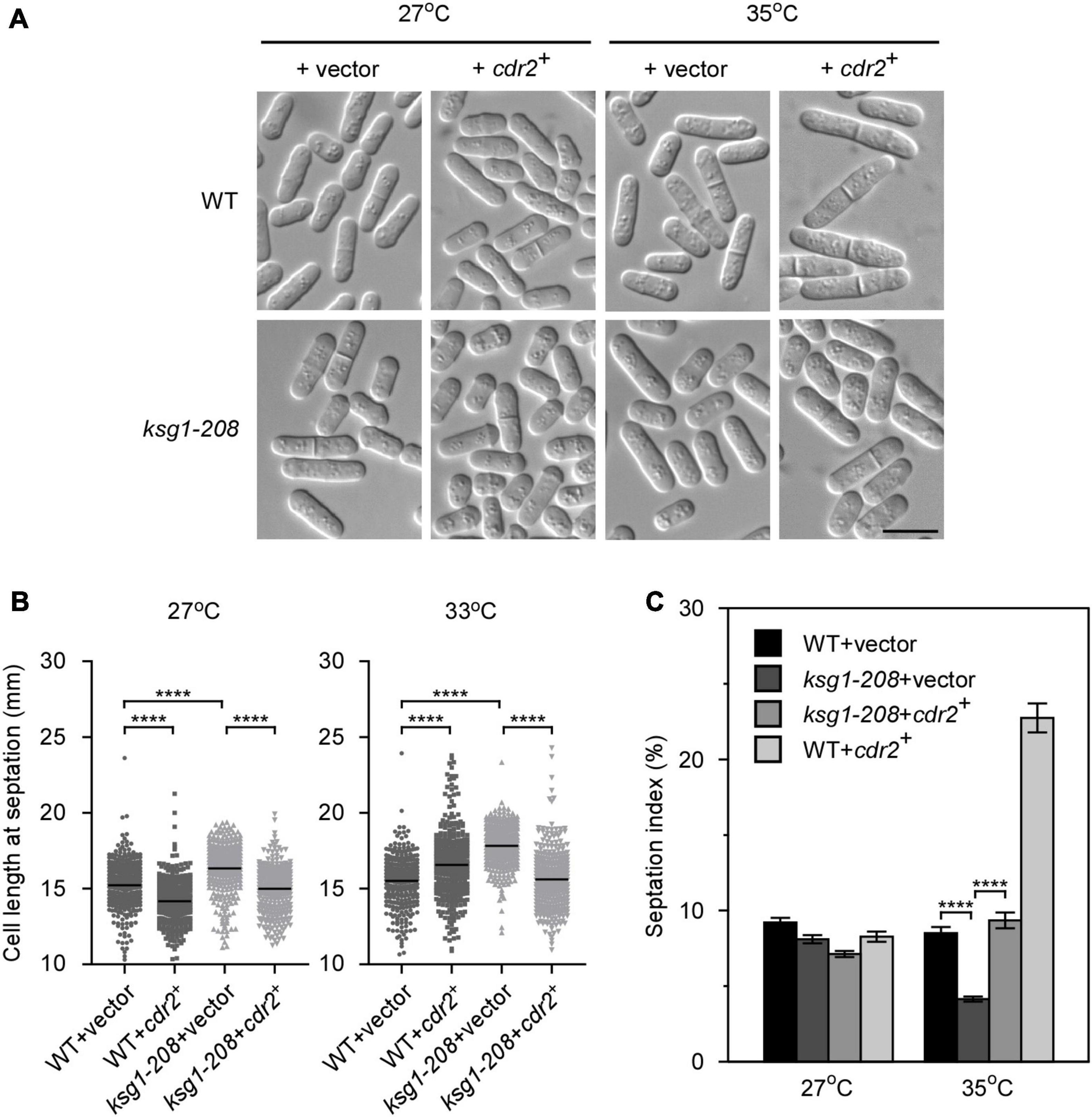
Figure 3. The overexpression of cdr2+ partially rescues the phenotypes of ksg1+ mutant cells. (A) Live-cell images of wild-type or ksg1-208 cells overexpressing cdr2+ gene. Wild-type or ksg1-208 cells were transformed with pREP1 empty vector or pREP1 vector containing cdr2+ and grown to early log-phase in EMM plates without thiamine at 27°C. Then cells were transferred to 35°C for 3 h and images were acquired using differential interference contrast (DIC) microscope immediately. Scale bar, 10 μm. (B) Quantification of the cell length at septation for each indicated strain grown at 27°C or transferred to 33°C for 3 h. Black bars represent mean (n ≥ 300). **** indicates p-value < 0.0001. (C) Septation index of each indicated strain grown at 27°C or transferred to 35°C for 3 h (n ≥ 1000). Data represent mean (± SD) of three biological replicates. **** indicates p-value < 0.0001.
Cdr2 Works Downstream of Ksg1 and Ppk21 in the Regulation of Cell Cycle
The fact that overexpressed cdr2+ facilitated the mitotic entry of ksg1-208 mutant cells prompted us to test the effects of ksg1+ mutation on the protein level of Cdr2. Using integrated GFP tags, we found that the protein level of Cdr2 in ksg1-208 cells was significantly lower than that in wild-type cells (Figures 4A,B), indicating that ksg1-208 mutation reduced the protein abundance of Cdr2. Notably, the protein level of Cdr2 in ksg1-208 Δppk21 cells was significantly lower than that in ksg1-208 cells, despite the Cdr2 protein level in Δppk21 cells showed no obvious difference compared with that in wild-type cells (Figures 4A,B). These results suggested that Ksg1 and Ppk21 regulated the abundance of Cdr2 synergistically.
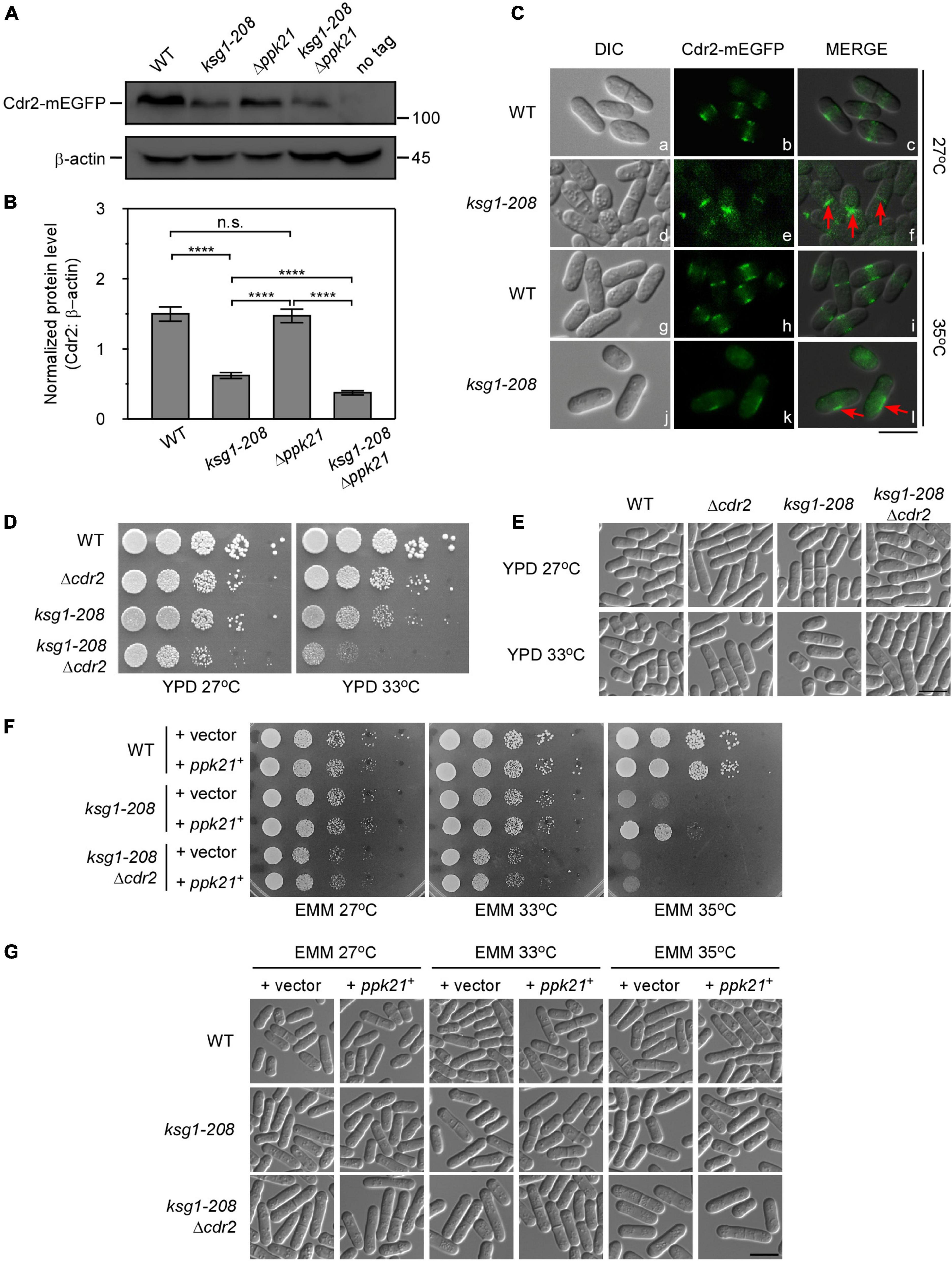
Figure 4. Cdr2 works downstream of Ksg1 and Ppk21. (A) Western blots of Cdr2 protein level in wild-type, ksg1-208, Δppk21, and ksg1-208 Δppk21 cells. Indicated strains were grown to early log-phase in EMM solution with 0.226 g/L leucine but without thiamine at 27°C. A wild-type sample (no tag) was loaded as a control. Total Cdr2 level was blotted by anti-GFP antibody, and β-actin was used as a loading control. (B) Quantification of total Cdr2 level in (A). Values were normalized by β-actin, and data represents mean (±SD) of three biological replicates. **** indicates p-value < 0.0001 while n.s. indicates no significant differences according to Tukey’s test. (C) Subcellular localization of Cdr2 in wild-type or ksg1-208 mutant cells grown at 27°C or 35°C. Indicated cells were grown to early log-phase in EMM containing 4 μM thiamine at 27°C and then transferred to 35°C for 3 h. Red arrows imply mis-localized Cdr2-mEGFP. Scale bar, 10 μm. (D) Wild-type, ksg1-208, Δcdr2 or ksg1-208 Δcdr2 cells were spotted onto YPD plates and then incubated for 4 days at 27°C or 33°C. (E) Live-cell images of strains in (D). Cells were grown to early log-phase in YPD plates and then were transferred to 33°C for 3 h and images were acquired using differential interference contrast (DIC) microscope immediately. Scale bar, 10 μm. (F) Overexpressed ppk21+ failed to rescue the temperature sensitivity of ksg1-208 mutant when the cdr2+ gene was deleted. Wild-type, ksg1-208 or ksg1-208 Δcdr2 cells overexpressing ppk21+ were spotted onto EMM plates without thiamine and then incubated for 4 days at 27°C, 33°C, or 35°C. (G) Live-cell images of strains in (F). Each strain were cultured in EMM plates without thiamine to early log-phase at 27°C and then were transferred to 33°C or 35°C for 3 h, and DIC images were acquired by microscope immediately. Scale bar, 10 μm.
The proper localization is crucial for Cdr2 to control cell cycle progression. Reportedly, Cdr2 forms a central broad band with other cortical node proteins through much of the G2 phase and during very early mitosis (Akamatsu et al., 2014). Then in G1 and S phase, Cdr2 proteins dissociate from the cortex and diffuse in the cytoplasm, and have no specific cell surface localization between septum formation and cell separation (Akamatsu et al., 2014; Rincon et al., 2017). As expected, in wild-type cells, Cdr2-mEGFP localized to the central cortex in the cell middle, whereas diffused to the cytoplasm in the dividing cells (Figure 4C). In contrast, a fraction of ksg1-208 cells showed septum or division site localized Cdr2-mEGFP in the dividing cells at 27°C, indicating the cortex dissociation of Cdr2 was hindered. Notably, asymmetrical off-centered localization of Cdr2 appeared in the majority of the ksg1-208 cells at 35°C, and the fluorescence intensity became much weaker than that of the wild-type cells (Figure 4C). These results suggest that Ksg1 acts upstream of Cdr2.
To further explore the relationship between Cdr2 and Ksg1, we constructed the ksg1-208 Δcdr2 cells. As shown in Figure 4D, the ksg1-208 Δcdr2 cells showed more marked temperature sensitivity than that of ksg1-208 cells or Δcdr2 cells. Consistent with this, the cell length of ksg1-208 Δcdr2 cells is longer than that of ksg1-208 cells (Figure 4E). Importantly, we found that overexpressed ppk21+ failed to rescue the temperature sensitivity of the ksg1-208 cells in the absence of the cdr2+ gene (Figures 4F,G). These results further demonstrate that Ppk21 also acts upstream of Cdr2.
Overexpression of ppk21+ or cdr2+ Partially Reversed the Decreased Cdc25 Level Caused by ksg1+ Mutation
Cdc25, a well-known G2/M inducer, was reported to regulate the cell size by size-dependent expression, which provided a mechanism for cells to trigger cell division when they reach a threshold concentration of Cdc25 (Keifenheim et al., 2017). Since ksg1 mutation led to a longer cell length and a defective G2/M transition, we then sought to investigate whether Cdc25 was involved in Ksg1-regulated cell cycle progression. Western blot analysis showed that the level of Cdc25 protein was dramatically lower in ksg1-208 cells than that in wild-type cells, indicating that Ksg1 played a crucial role in the accumulation of Cdc25 protein (Figure 5A). In addition, the Cdc25 protein level decreased in Δppk21 and Δcdr2 cells as well, indicating the role of Ppk21 and Cdr2 on regulating Cdc25 protein level (Figure 5A). Intriguingly, overexpression of ppk21+ or cdr2+ regained the Cdc25 protein level partially in ksg1-208 cells (Figures 5B,C). Given the regulatory role of Ksg1 and Ppk21 on Cdr2, we concluded that Cdr2 works downstream of Ksg1 and Ppk21 to adjust the Cdc25 protein level.
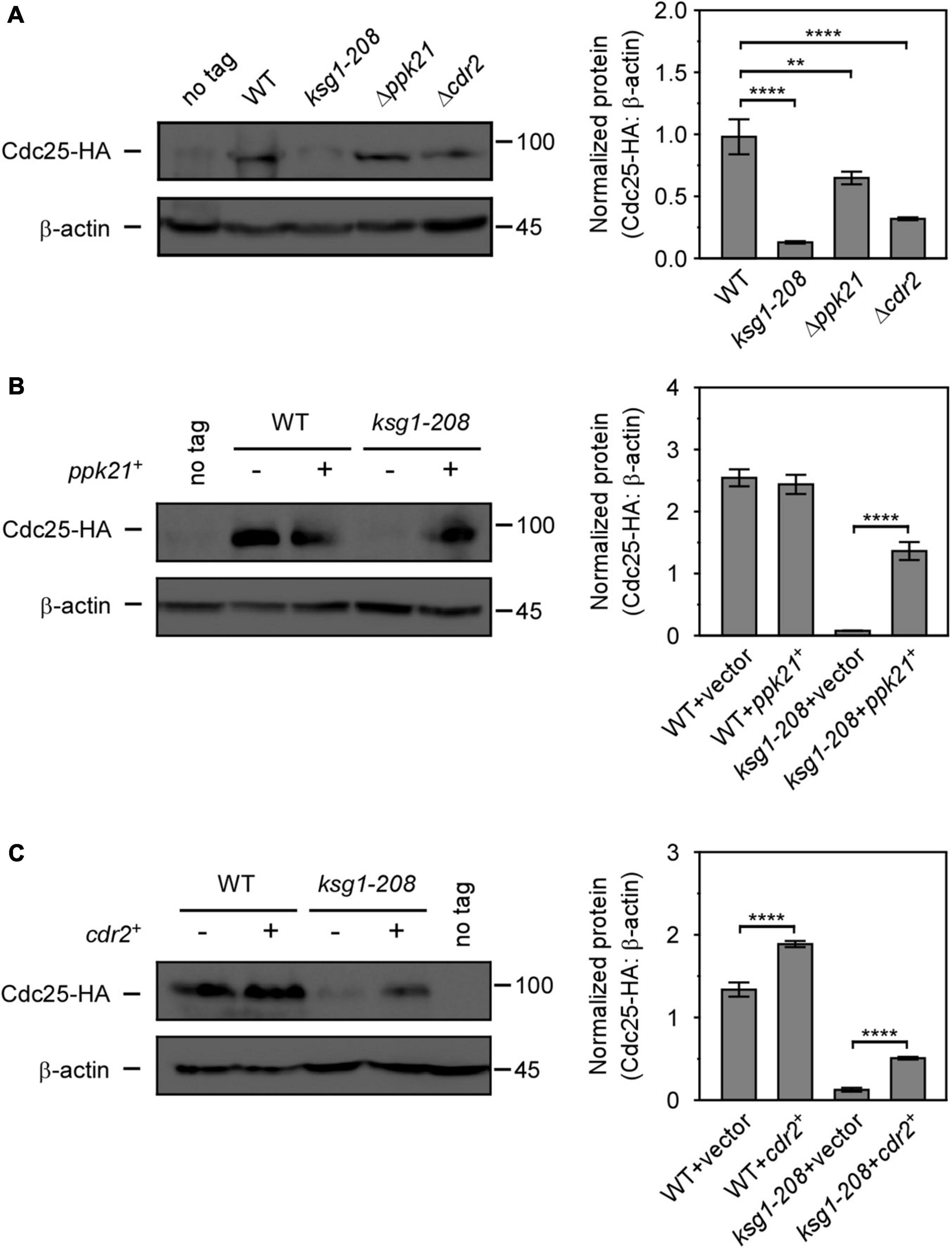
Figure 5. Overexpressed ppk21+ or cdr2+ gene partially reversed the decreased Cdc25 level caused by ksg1+ mutation. (A) Western blots (left) and quantification (right) of Cdc25-HA in wild-type, ksg1-208,Δppk21 and Δcdr2 cells. Cells were grown to early log-phase in EMM solution containing 0.226 g/L leucine but without thiamine at 27°C and then harvested for total protein extraction. (B,C) Western blots of Cdc25-HA protein level in ksg1-208 cells overexpressing ppk21+ or cdr2+. A wild-type sample (no tag) was loaded as a control. Total Cdc25 level was blotted by anti-HA antibody, and β-actin was used as a loading control. Quantification of Cdc25 level was normalized by β-actin. Data represents mean (±SD) of three biological replicates. ** indicates p-value < 0.01 and **** indicates p-value < 0.0001.
Discussion
The PDK1 homolog Ksg1 was identified as an essential kinase in fission yeast responsible for growth, mating, and sporulation by phosphorylating the activation loop of AGC kinases (Niederberger and Schweingruber, 1999; Matsuo et al., 2003; Tang and Mcleod, 2004). It was reported that mutation of ksg1 leads to growth arrest in the G2/M phase of the cell cycle (Niederberger and Schweingruber, 1999), however, the mechanisms remain unclear. In this study, we identified SAD kinase Cdr2 and another PDK1 homolog Ppk21 as novel elements involved in Ksg1 function, and revealed the important role of these two elements in the process of cell cycle regulated by Ksg1. To our knowledge, this is the first report of the involvement of Ppk21 and Cdr2 in Ksg1-regulated cell cycle progression.
Ppk21 was originally recognized as a key regulator involved in mitosis and cytokinesis (Bimbó et al., 2005b). In this study, several lines of evidence support the idea that Ksg1 and Ppk21 function redundantly in cell cycle regulation. First, similar to Ksg1, Ppk21 is another PDK1 homolog. Second, ksg1-208 cells exhibited temperature sensitivity, longer cell length, and lower septation index, which were rescued by overexpression of ppk21+. Third, the ksg1-208 Δppk21 cells exhibited more marked temperature sensitivity than the ksg1-208 mutant, although the Δppk21 cells did not show temperature sensitivity. In addition, the ksg1-208 Δppk21 cells displayed a longer cell length than each single mutant, suggesting that deletion of Ppk21 exacerbated the defective cell cycle transition of ksg1-208 mutant. All these suggest that Ksg1 and Ppk21 were functionally redundant, and Ksg1 may be the major one in cell cycle regulation. Moreover, ksg1-208 Δppk21 cells showed enhanced division plane-positioning defects compared to ksg1-208 cells at 33°C, further indicating the synergistic effect of Ksg1 and Ppk21. It is possible that Ksg1 and Ppk21 share some substrates, thereby leading to the redundant function.
The integration of cell growth and cell cycle progression ensures the reproducible cell division size. The key component of this integration in fission yeast is Cdr2, which organizes cortical nodes in the cell middle to sense cell size and promote mitotic entry (Breeding et al., 1998; Kanoh and Russell, 1998; Martin and Berthelot-Grosjean, 2009; Moseley et al., 2009; Rincon et al., 2017; Allard et al., 2018; Facchetti et al., 2019). The activity of Cdr2 increases as cells grow in the G2 phase while the total level keeps constant throughout the cell cycle (Deng et al., 2014). In addition, the periodic change of Cdr2 localization regulated by phosphorylation status is also a crucial premise for its function (Rincon et al., 2017; Willet et al., 2019). In our study, we found that the protein level of Cdr2 significantly decreased in ksg1-208 mutant cells (Figures 4A,B), suggesting the important role of Ksg1 in stabilizing the protein level of Cdr2. Besides, anomalies in Cdr2 localization appeared in ksg1-208 mutant cells at both permissive and restrictive temperatures (Figure 4C). The obvious septum or division site localization of Cdr2 in ksg1-208 cells at 27°C implied the defects in dissociation from the cortex, similar to the localization of Cdr2 mutated at two SIN-dependent phosphorylation sites (Rincon et al., 2017). The asymmetrical off-centered localization of Cdr2 at 35°C further revealed the defects in the spatial distribution of Cdr2 in ksg1-208 mutant cells. In particular, the absence of Ppk21 exacerbated the decrease of Cdr2 protein level in ksg1-208 cells. More importantly, overexpression of ppk21+ could rescue the temperature sensitivity of ksg1-208 cells, but failed to rescue the temperature sensitivity when the cdr2+ gene was deleted. Taken into the effect of overexpressed cdr2+ on cell length and septation of ksg1-208 cells, these data together demonstrated that Cdr2 is a novel downstream effector of Ksg1 and Ppk21 in cell cycle control. A previous study reported that overexpression of cdr2+ was toxic and generated cells that contained two or more septa (Breeding et al., 1998). Consistently, we found that overexpression of cdr2+ caused the wild-type cells a longer cell length and a higher septation index (Figures 3B,C). Interestingly, overexpression of cdr2+ did not cause the ksg1-208 cells a longer length and a higher septation index (Figures 3B,C), suggesting the necessity of Ksg1 activity for the function of Cdr2. This further demonstrated that Cdr2 functions downstream of Ksg1.
Then, how does Ksg1-Ppk21-Cdr2 signaling promote the cell cycle progression? In eukaryotes, the cell cycle G2/M phase transition is controlled by mitotic cyclin-dependent kinase complex (Cdc2-cyclin B), which is inactivated by Wee1 family protein kinases and activated by the opposing phosphatase Cdc25 (Russell and Nurse, 1986, 1987; Karlsson-Rosenthal and Millar, 2006; Glover, 2012). Previous studies have shown that Cdr2 recruits Wee1 to cortical nodes through interacting with the N-terminal of Wee1 and negatively regulates the latter together with another SAD family kinase Cdr1 to promote mitotic entry (Allard et al., 2018). However, a recent study showed that both Cdr1 and Cdr2 promote the phosphorylation of Wee1 in vivo, but only Cdr1 inhibits the kinase activity of Wee1 (Opalko et al., 2019). Taken together with our results that overexpression of cdr2+, but not cdr1+, rescued the growth defect of ksg1-208 cells, we speculate that Ksg1 regulates cell cycle progression via Cdr2, which may be independent of modifying the kinase activity of Wee1. Thus, Cdc25 came into our consideration. Cdc25 is a mitotic inducer whose expression is dependent on cell size. The concentration of Cdc25 increases as cells grow and triggers cell division when it reaches a threshold (Keifenheim et al., 2017). In this study, we found that the protein level of either Cdr2 or Cdc25 was significantly lower in ksg1-208 cells than that in the wild-type cells, indicating that the abundance of both Cdr2 and Cdc25 was repressed when Ksg1 kinase activity was damaged. In addition, the absence of Ppk21 or Cdr2 also reduced the protein level of Cdc25. In particular, overexpression of the ppk21+ or cdr2+ gene partially recovered the reduced Cdc25 protein level in ksg1-208 cells. These results indicated a regulatory role of Ksg1, Ppk21, and Cdr2 on Cdc25, and Ksg1-Ppk21-Cdr2 signaling may promote the cell cycle progression through Cdc25. A previous study reported that loss of Cdr2 activity delays mitosis in strains that have no active Cdc25 protein, and concluded that Cdc25 may not be the primary target of Cdr2 regulation (Kanoh and Russell, 1998). Probably, Cdc25 is regulated slightly but significantly by Cdr2, whereas unidentified factors exist in Ksg1-regulated signaling network to mainly control the Cdc25 abundance. Meanwhile, we could not exclude the possibility that Cdc25 is regulated by Cdr2 indirectly, and unidentified molecular(s) may be involved in this process. The detailed mechanisms need to be further illustrated.
In conclusion, we propose a mechanism by which Ksg1 controls cell cycle progression according to our results: PDK1 homologs Ksg1 and Ppk21 function redundantly and they act upstream of Cdr2, thereby controlling cell cycle progression and Cdc25 is involved in this process in fission yeast. Our findings provide a novel theoretical foundation for enriching the PDK1 regulatory network in humans. Whether Ksg1 and Ppk21 involve in Cdr2 phosphorylation as well as how they regulate Cdc25 are important questions to be addressed in the future.
Data Availability Statement
The original contributions presented in the study are included in the article/Supplementary Material, further inquiries can be directed to the corresponding author/s.
Author Contributions
YF designed the study. KL, QL, YS, JF, and YZ performed the experiments. NS and TK analyzed the data. KL and YF wrote the manuscript with suggestions from all authors.
Funding
This work was supported by grants from the Natural Science Foundation of Liaoning Province, China (No. 2019-MS-384) and the Innovative Talents Support Program in University of Liaoning Province in 2020 to YF. This work was also supported by research fund from Doctoral Start-up Foundation of Liaoning Province, China (No. 2020-BS-096) to QL. The funders had no role in study design, data collection, and interpretation, or the decision to submit the work for publication.
Conflict of Interest
The authors declare that the research was conducted in the absence of any commercial or financial relationships that could be construed as a potential conflict of interest.
Publisher’s Note
All claims expressed in this article are solely those of the authors and do not necessarily represent those of their affiliated organizations, or those of the publisher, the editors and the reviewers. Any product that may be evaluated in this article, or claim that may be made by its manufacturer, is not guaranteed or endorsed by the publisher.
Supplementary Material
The Supplementary Material for this article can be found online at: https://www.frontiersin.org/articles/10.3389/fmicb.2021.807148/full#supplementary-material
Footnotes
References
Abbastabar, M., Kheyrollah, M., Azizian, K., Bagherlou, N., Tehrani, S. S., Maniati, M., et al. (2018). Multiple functions of p27 in cell cycle, apoptosis, epigenetic modification and transcriptional regulation for the control of cell growth: a double-edged sword protein. DNA Repair 69, 63–72. doi: 10.1016/j.dnarep.2018.07.008
Akamatsu, M., Berro, J., Pu, K. M. I, Tebbs, R., and Pollard, T. D. (2014). Cytokinetic nodes in fission yeast arise from two distinct types of nodes that merge during interphase. J. Cell Biol. 204, 977–988. doi: 10.1083/jcb.201307174
Alessi, D. R., James, S. R., Downes, C. P., Holmes, A. B., Gaffney, P. R. J., Reese, C. B., et al. (1997). Characterization of a 3-phosphoinositide-dependent protein kinase which phosphorylates and activates protein kinase Bα. Curr. Biol. 7, 261–269. doi: 10.1016/s0960-9822(06)00122-9
Allard, C. A. H., Opalko, H. E., Liu, K. E., Medoh, U., and Moseley, J. B. (2018). Cell size-dependent regulation of Wee1 localization by Cdr2 cortical nodes. J. Cell Biol. 217, 1589–1599. doi: 10.1083/jcb.201709171
Bai, X., Li, P., Xie, Y., Guo, C., Sun, Y., Xu, Q., et al. (2016). Overexpression of 3-phosphoinositide-dependent protein kinase-1 is associated with prognosis of gastric carcinoma. Tumor Biol. 37, 2333–2339. doi: 10.1007/s13277-015-4024-8
Beach, D., Piper, M., and Nurse, P. (1982). Construction of a Schizosaccharomyces pombe gene bank in a yeast bacterial shuttle vector and its use to isolate genes by complementation. Mol. Gen. Genet. 187, 326–329. doi: 10.1007/BF00331138
Bimbó, A., Jia, Y., Poh, S. L., Karuturi, R. K. M., den Elzen, N., Peng, X., et al. (2005a). Systematic deletion analysis of fission yeast protein kinases. Eukaryotic Cell 4, 799–813. doi: 10.1128/EC.4.4.799-813.2005
Bimbó, A., Liu, J., and Balasubramanian, M. K. (2005b). Roles of Pdk1p, a fission yeast protein related to phosphoinositide-dependent protein kinase, in the regulation of mitosis and cytokinesis. Mol. Biol. Cell 16, 3162–3175. doi: 10.1091/mbc.e04-09-0769
Breeding, C. S., Hudson, J., Balasubramanian, M. K., Hemmingsen, S. M., Young, P. G., and Gould, K. L. (1998). The cdr2+ gene encodes a regulator of G2/M progression and cytokinesis in Schizosaccharomyces pombe. Mol. Biol. Cell 9, 3399–3415. doi: 10.1091/mbc.9.12.3399
Casamayor, A., Torrance, P. D., Kobayashi, T., Thorner, J., and Alessi, D. R. (1999). Functional counterparts of mammalian protein kinases PDK1 and SGK in budding yeast. Curr. Biol. 9, 186–197. doi: 10.1016/s0960-9822(99)80088-8
Crump, J. G., Zhen, M., Jin, Y., and Bargmann, C. I. (2001). The SAD-1 kinase regulates presynaptic vesicle clustering and axon termination. Neuron 29, 115–129. doi: 10.1016/s0896-6273(01)00184-2
Deng, L., Baldissard, S., Kettenbach, A., Gerber, S., and Moseley, J. B. (2014). Dueling kinases regulate cell size at division through the SAD kinase cdr2. Curr. Biol. 24, 428–433. doi: 10.1016/j.cub.2014.01.009
Facchetti, G., Knapp, B., Flor-Parra, I., Chang, F., and Howard, M. (2019). Reprogramming Cdr2-dependent geometry-based cell size control in fission yeast. Curr. Biol. 29, 350–358. doi: 10.1016/j.cub.2018.12.017
Fang, Y., Sugiura, R., Yan, M., Yada-Matsushima, T., Umeno, H., and Kuno, T. (2008). Cation diffusion facilitator Cis4 is implicated in Golgi membrane trafficking via regulating zinc homeostasis in fission yeast. Mol. Biol. Cell 19, 1295–1303. doi: 10.1091/mbc.e07-08-0805
Forsburg, S. L. (2005). The yeasts Saccharomyces cerevisiae and Schizosaccharomyces pombe, models for cell biology research. Gravit. Space Biol. 18, 3–9.
Furnari, B., Rhind, N., and Russell, P. (1997). Cdc25 mitotic inducer targeted by Ck1 DNA damage checkpoint kinase. Science 277, 1495–1497. doi: 10.1126/science.277.5331.1495
Gagliardi, P. A., Blasio, L. D., and Primo, L. (2015). PDK1: a signaling hub for cell migration and tumor invasion. Biochim. Biophys. Acta 1856, 178–188. doi: 10.1016/j.bbcan.2015.07.003
Gagliardi, P. A., di Blasio, L., Orso, F., Seano, G., Sessa, R., Taverna, D., et al. (2012). 3-phosphoinositide-dependent kinase 1 controls breast tumor growth in a kinase-dependent but Akt-independent manner. Neoplasia 14, 719–731. doi: 10.1593/neo.12856
Gagliardi, P. A., Puliafito, A., and Primo, L. (2018). PDK1: at the crossroad of cancer signaling pathways. Semin. Cancer Biol. 48, 27–35. doi: 10.1016/j.semcancer.2017.04.014
Glover, D. M. (2012). The overlooked greatwall: a new perspective on mitotic control. Open Biol. 2:120023. doi: 10.1098/rsob.120023
Hashimoto, N., Kido, Y., Uchida, T., Asahara, S. I., Shigeyama, Y., Matsuda, T., et al. (2006). Ablation of PDK1 in pancreatic β cells induces diabetes as a result of loss of β cell mass. Nat. Genet. 38, 589–593.
Jiang, G., Liu, Q., Kato, T., Miao, H., Gao, X., Liu, K., et al. (2021). Role of mitochondrial complex III/IV in the activation of transcription factor Rst2 in Schizosaccharomyces pombe. Mol. Microbiol. 115, 1323–1338. doi: 10.1111/mmi.14678
Jiang, Q., Zheng, N., Bu, L., Zhang, X., Zhang, X., Wu, Y., et al. (2021). SPOP-mediated ubiquitination and degradation of PDK1 suppresses AKT kinase activity and oncogenic functions. Mol. Cancer 20:100. doi: 10.1186/s12943-021-01397-5
Kanoh, J., and Russell, P. (1998). The protein kinase Cdr2, related to Nim1/Cdr1 mitotic inducer, regulates the onset of mitosis in fission yeast. Mol. Biol. Cell 9, 3321–3334. doi: 10.1091/mbc.9.12.3321
Karlsson-Rosenthal, C., and Millar, J. B. A. (2006). Cdc25: mechanisms of checkpoint inhibition and recovery. Trends Cell Biol. 16, 285–292. doi: 10.1016/j.tcb.2006.04.002
Keifenheim, D., Sun, X. M., D’Souza, E., Ohira, M. J., Magner, M., Mayhew, M. B., et al. (2017). Size-dependent expression of the mitotic activator Cdc25 suggests a mechanism of size control in fission yeast. Curr. Biol. 27, 1491–1497. doi: 10.1016/j.cub.2017.04.016
Kim, D. U., Hayles, J., Kim, D., Wood, V., Park, H. O., Won, M., et al. (2010). Analysis of a genome-wide set of gene deletions in the fission yeast Schizosaccharomyces pombe. Nat. Biotechnol. 28, 617–623. doi: 10.1038/nbt.1628
Kita, A., Sugiura, R., Shoji, H., He, Y., Deng, L., Lu, Y., et al. (2004). Loss of Apm1, the μ1 subunit of the clathrin-associated adaptor-protein-1 complex, causes distinct phenotypes and synthetic lethality with calcineurin deletion in fission yeast. Mol. Biol. Cell 15, 2920–2931. doi: 10.1091/mbc.e03-09-0659
Kobayashi, T., and Cohen, P. (1999). Activation of serum- and glucocorticoid-regulated protein kinase by agonists that activate phosphatidylinositide 3-kinase is mediated by 3-phosphoinositide-dependent protein kinase-1 (PDK1) and PDK2. Biochem. J. 339, 319–328. doi: 10.1042/0264-6021:3390319
Liu, Q., Guo, X., Jiang, G., Wu, G., Miao, H., Liu, K., et al. (2020). NADPH-cytochrome P450 reductase Ccr1 is a target of Tamoxifen and participates in its antifungal activity via regulating cell wall integrity in fission yeast. Antimicrob. Agents Chemother. 64:e00079-e20. doi: 10.1128/AAC.00079-20
Liu, Q., Yao, F., Jiang, G., Xu, M., Chen, S., Zhou, L., et al. (2018). Dysfunction of Prohibitin 2 results in reduced susceptibility to multiple antifungal drugs via activation of the oxidative stress-responsive transcription factor Pap1 in fission yeast. Antimicrob. Agents Chemother. 62, e00860-e18. doi: 10.1128/AAC.00860-18
Madrid, M., Jiménez, R., ánchezmir-Mir, L. S., Soto, T., Franco, A., Vicente-Soler, J., et al. (2014). Multiple layers of regulation influence cell integrity control by the PKC ortholog Pck2 in fission yeast. J. Cell Sci. 128, 266–280. doi: 10.1242/jcs.158295
Martin, S. G., and Berthelot-Grosjean, M. (2009). Polar gradients of the DYRK-family kinase Pom1 couple cell length with the cell cycle. Nature 459, 852–856. doi: 10.1038/nature08054
Matsuo, T., Kubo, Y., Watanabe, Y., and Yamamoto, M. (2003). Schizosaccharomyces pombe AGC family kinase Gad8p forms a conserved signaling module with TOR and PDK1-like kinases. EMBO J. 22, 3073–3083. doi: 10.1093/emboj/cdg298
Mitchison, J. M., and Nurse, P. (1985). Growth in cell length in the fission yeast Schizosaccharomyces pombe. J. Cell Sci. 75, 357–376. doi: 10.1242/jcs.75.1.357
Mora, A., Komander, D., van Aalten, D. M. F., and Alessi, D. R. (2004). PDK1, the master regulator of AGC kinase signal transduction. Semin. Cell Dev. Biol. 15, 161–170. doi: 10.1016/j.semcdb.2003.12.022
Moreno, S., Klar, A., and Nurse, P. (1991). Molecular genetic analysis of fission yeast Schizosaccharomyces pombe. Methods Enzymol. 194, 795–823.
Moseley, J. B., Mayeux, A., Paoletti, A., and Nurse, P. (2009). A spatial gradient coordinates cell size and mitotic entry in fission yeast. Nature 459, 857–860. doi: 10.1038/nature08074
Niederberger, C., and Schweingruber, M. E. (1999). A Schizosaccharomyces pombe gene, ksg1, that shows structural homology to the human phosphoinositide-dependent protein kinase PDK1, is essential for growth, mating and sporulation. Mol. General Genet. 261, 177–183. doi: 10.1007/s004380050955
Opalko, H. E., Nasa, I., Kettenbach, A. N., and Moseley, J. B. (2019). A mechanism for how Cdr1/Nim1 kinase promotes mitotic entry by inhibiting Wee1. Mole. Biol. Cell 30, 3015–3023. doi: 10.1091/mbc.E19-08-0430
Pietri, M., Dakowski, C., Hannaoui, S., Alleaume-Butaux, A., Hernandez-Rapp, J., Ragagnin, A., et al. (2013). PDK1 decreases TACE-mediated α-secretase activity and promotes disease progression in prion and Alzheimer’s diseases. Nat. Med. 19, 1124–1131. doi: 10.1038/nm.3302
Rincon, S. A., Estravis, M., Dingli, F., Loew, D., Tran, P. T., and Paoletti, A. (2017). SIN-dependent dissociation of the SAD kinase Cdr2 from the cell cortex resets the division plane. Curr. Biol. 27, 534–542. doi: 10.1016/j.cub.2016.12.050
Rothstein, R. J. (1989). One-step gene disruption in yeast. Recomb. DNA Methodol. 101, 202–211. doi: 10.1016/0076-6879(83)01015-0
Russell, P., and Nurse, P. (1986). cdc25+ functions as an inducer in the mitotic control of fission yeast. Cell 45, 145–153. doi: 10.1016/0092-8674(86)90546-5
Russell, P., and Nurse, P. (1987). Negative regulation of mitosis by wee1+, a gene encoding a protein kinase homolog. Cell 49, 559–567. doi: 10.1016/0092-8674(87)90458-2
Scotchman, E., Kume, K., Navarro, F. J., and Nurse, P. (2021). Identification of mutants with increased variation in cell size at onset of mitosis in fission yeast. J. Cell Sci. 134:jcs251769. doi: 10.1242/jcs.251769
Stokoe, D., Stephens, L. R., Copeland, T., Gaffney, P. R., Reese, C. B., Painter, G. F., et al. (1997). Dual role of phosphatidylinositol-3,4,5-trisphosphate in the activation of protein kinase B. Science 277, 567–570. doi: 10.1126/science.277.5325.567
Tang, Y., and Mcleod, M. (2004). In vivo activation of protein kinase A in Schizosaccharomyces pombe requires threonine phosphorylation at its activation loop and is dependent on PDK1. Genetics 168, 1843–1853. doi: 10.1534/genetics.104.032466
Toda, T., Dhut, S., Superti-Furga, G., Gotoh, Y., Nishida, E., Sugiura, R., et al. (1997). The fission yeast pmk1+ gene encodes a novel mitogen-activated protein kinase homolog which regulates cell integrity and functions coordinately with the protein kinase C pathway. Mol. Cell. Biol. 16, 6752–6764. doi: 10.1128/MCB.16.12.6752
Vyas, A., Freitas, A. V., Ralston, Z. A., and Tang, Z. (2021). Fission yeast Schizosaccharomyces pombe, a unicellular “micromammal” model organism. Curr. Protoc. 1, 1–24.
Wang, J., Liu, F., Ao, P., Li, X., Zheng, H., Wu, D., et al. (2016). Correlation of PDK1 expression with clinicopathologic features and prognosis of hepatocellular carcinoma. OncoTargets Ther. 9, 5597–5602. doi: 10.2147/OTT.S110646
Willet, A. H., DeWitt, A. K., Beckley, J. R., Clifford, D. M., and Gould, K. L. (2019). NDR kinase Sid2 drives anillin-like Mid1 from the membrane to promote cytokinesis and medial division site placement. Curr. Biol. 29, 1055–1063. doi: 10.1016/j.cub.2019.01.075
Wu, L., and Russell, P. (1993). Nim1 kinase promotes mitosis by inactivating Wee1 tyrosine kinase. Nature 363, 738–741. doi: 10.1038/363738a0
Young, P. G., and Fantes, P. A. (1987). Schizosaccharomyces pombe mutants affected in their division response to starvation. J. Cell Sci. 88, 295–304. doi: 10.1242/jcs.88.3.295
Zabkiewicz, J., Pearn, L., Hills, R. K., Morgan, R. G., Tonks, A., Burnett, A. K., et al. (2014). The PDK1 master kinase is over-expressed in acute myeloid leukemia and promotes PKC-mediated survival of leukemic blasts. Haematologica 99, 858–864. doi: 10.3324/haematol.2013.096487
Keywords: Schizosaccharomyces pombe, Ksg1, Ppk21, Cdr2, Cdc25, cell cycle regulation
Citation: Liu K, Liu Q, Sun Y, Fan J, Zhang Y, Sakamoto N, Kuno T and Fang Y (2022) Phosphoinositide-Dependent Protein Kinases Regulate Cell Cycle Progression Through the SAD Kinase Cdr2 in Fission Yeast. Front. Microbiol. 12:807148. doi: 10.3389/fmicb.2021.807148
Received: 01 November 2021; Accepted: 15 December 2021;
Published: 10 January 2022.
Edited by:
Zhaomin Yang, Virginia Tech, United StatesReviewed by:
Lin Deng, Shenzhen Bay Laboratory, ChinaThomas T. Eng, Lawrence Berkeley National Laboratory, United States
Copyright © 2022 Liu, Liu, Sun, Fan, Zhang, Sakamoto, Kuno and Fang. This is an open-access article distributed under the terms of the Creative Commons Attribution License (CC BY). The use, distribution or reproduction in other forums is permitted, provided the original author(s) and the copyright owner(s) are credited and that the original publication in this journal is cited, in accordance with accepted academic practice. No use, distribution or reproduction is permitted which does not comply with these terms.
*Correspondence: Yue Fang, eWZhbmdAY211LmVkdS5jbg==