- Division of Microbiology, ICAR-Indian Agricultural Research Institute (IARI), New Delhi, India
Interactions among the plant microbiome and its host are dynamic, both spatially and temporally, leading to beneficial or pathogenic relationships in the rhizosphere, phyllosphere, and endosphere. These interactions range from cellular to molecular and genomic levels, exemplified by many complementing and coevolutionary relationships. The host plants acquire many metabolic and developmental traits such as alteration in their exudation pattern, acquisition of systemic tolerance, and coordination of signaling metabolites to interact with the microbial partners including bacteria, fungi, archaea, protists, and viruses. The microbiome responds by gaining or losing its traits to various molecular signals from the host plants and the environment. Such adaptive traits in the host and microbial partners make way for their coexistence, living together on, around, or inside the plants. The beneficial plant microbiome interactions have been exploited using traditional culturable approaches by isolating microbes with target functions, clearly contributing toward the host plants’ growth, fitness, and stress resilience. The new knowledge gained on the unculturable members of the plant microbiome using metagenome research has clearly indicated the predominance of particular phyla/genera with presumptive functions. Practically, the culturable approach gives beneficial microbes in hand for direct use, whereas the unculturable approach gives the perfect theoretical information about the taxonomy and metabolic potential of well-colonized major microbial groups associated with the plants. To capitalize on such beneficial, endemic, and functionally diverse microbiome, the strategic approach of concomitant use of culture-dependent and culture-independent techniques would help in designing novel “biologicals” for various crops. The designed biologicals (or bioinoculants) should ensure the community’s persistence due to their genomic and functional abilities. Here, we discuss the current paradigm on plant-microbiome-induced adaptive functions for the host and the strategies for synthesizing novel bioinoculants based on functions or phylum predominance of microbial communities using culturable and unculturable approaches. The effective crop-specific inclusive microbial community bioinoculants may lead to reduction in the cost of cultivation and improvement in soil and plant health for sustainable agriculture.
Introduction
Cultivated soils are one of the most diverse microbial ecosystems, harboring bacteria, fungi, archaea, viruses, protists, and many others and supporting various biogeochemical cycles and plant growth. Soil microbial communities are critical to plant health and adapt rapidly to different abiotic and biotic stresses (Abdul Rahman et al., 2021). The soils and their microbial members provide humans with 98.8% of the plant foods we eat (FAO, 2018; Kopittke et al., 2019; Soto-Giron et al., 2021). The Food and Agriculture Organization (FAO) predicts that soil erosion could result in between 20 and 80% losses in agricultural yields due to human activities and climate change events. This erosion of topsoil could result in variable agricultural yields, depending on the soil type and the resource use pattern (Kopittke et al., 2019; Christy, 2021). The agrarian management of soils depends on many synthetic chemical inputs for increasing profitability and productivity. Unfortunately, intensive use of these chemical inputs has led to adverse environmental consequences from regional to global scales. To reduce chemical inputs and their associated undesirable effects in the soil and environment, microbial interventions as biological products are becoming an integral part of plant nutrient management programs and pest and disease management practices.
Microbial communities associated with plants, presently referred to as the plant microbiome, extend the host plant genome and their functions (Figure 1). Many studies demonstrate that these microbiomes are the key determinants of plant development, health, and productivity (Conrad et al., 2006; Bulgarelli et al., 2012; Lundberg et al., 2012; Turner et al., 2013; Williams, 2013). The recent investigations have unraveled the complex network of genetic, biochemical, physical, and metabolic interactions among the plant host, the associated microbial communities, and the environment. These interactions shape the microbiome assembly and modulate beneficial traits such as nutrient acquisition and plant health (Trivedi et al., 2021). Nutrient acquisition by plants is mediated by diverse mechanisms that include (i) augmenting the surface area accessed by plant roots for uptake of water and nutrients, (ii) through nitrogen fixation, (iii) P-solubilization, (iv) the production of siderophore and HCN production, and other unknowns. Furthermore, their contributions in protection against biotic (pests and diseases) and abiotic stresses directly or through modulating intrinsic resistance/tolerance have been reported (Pii et al., 2015; Govindasamy et al., 2020; Abiraami et al., 2021). The basis of this review is to highlight strategic approaches for designing novel bioinoculants based on the plant microbiome data generated from both culturable and unculturable approaches. Such plant microbiome-based specific bioinoculants may function in a better way as compared to the conventional bioinoculants with non-specific microbial isolates. The agricultural bioinoculant market is a fast-growing sector with a compound annual growth rate (CAGR) of 6.9% with a predicted value of over 12 billion US dollars by 2025. The growth of the market is driven by increasing health concerns and awareness among consumers, resulting in the inclination toward organic farming practices or low-chemical-input agriculture. Hence, the bioinoculant technology will move forward toward reducing the cost of cultivation while improving soil and plant health for sustainable agriculture.
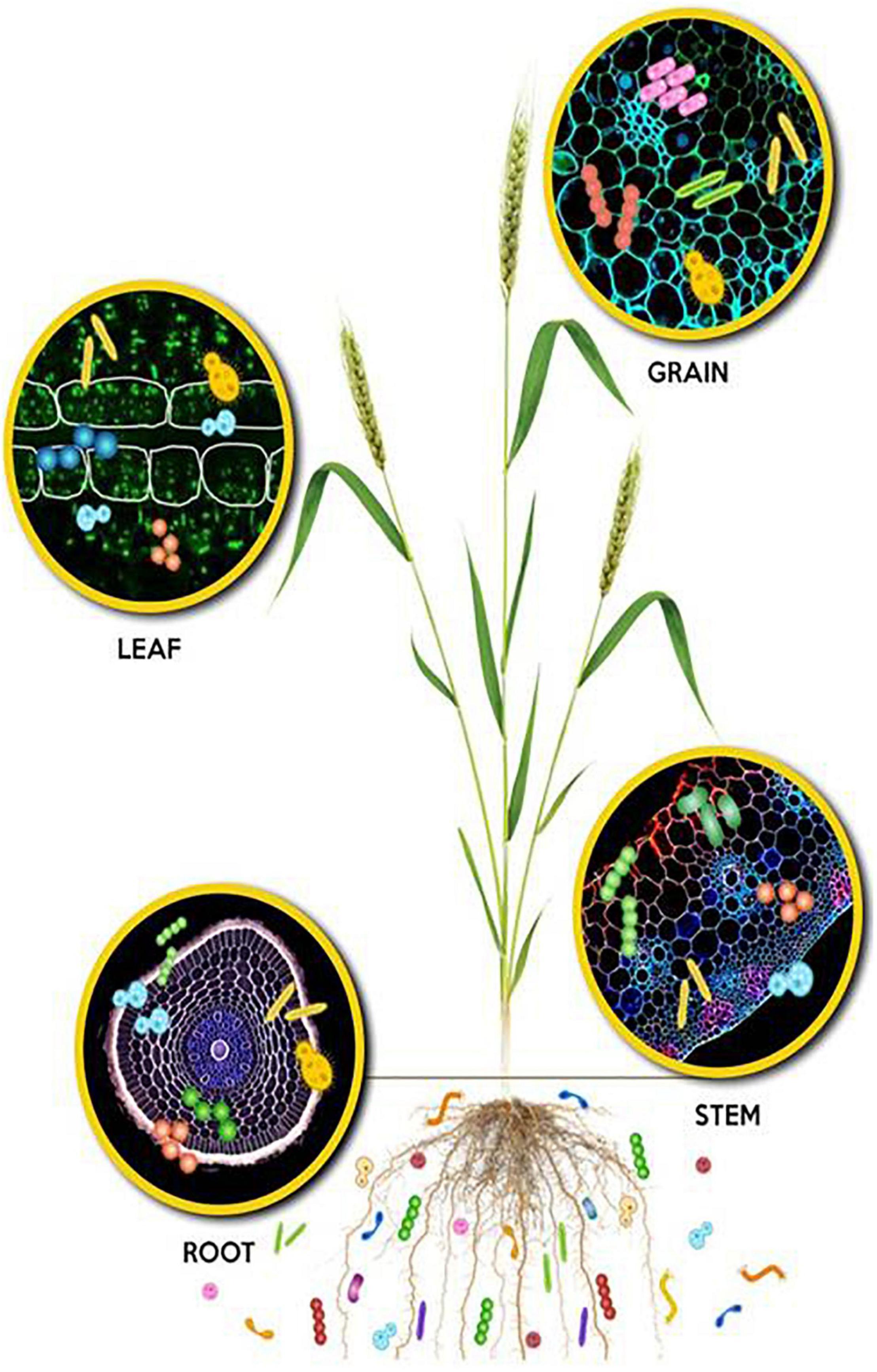
Figure 1. Microbial colonization depicted in different plant niches: Rhizosphere, phyllosphere and endosphere of root, stem, leaf, and grain.
Plant-Microbiome-Mediated Adaptive Functions
The microbiome is playing a significant role, throughout the plant life cycle, in altering the physiologies, and development through phytohormones, metabolites, signals, responses, nutrients, and induction of systemic resistance against pathogens as well as tolerance mechanisms against abiotic stresses such as drought, salinity, or contaminated soils (Mendes et al., 2013; Marag and Suman, 2018; Compant et al., 2019). At the community level, the microbiome functional capability is more than the sum of its individual microbial components as individual microbial species in the microbiome may interact to form a complex network, which interrelates with the host plant(s) in a mutualistic, synergistic, commensalistic, amensalistic, or parasitic mode of relationship. These interactions influence each member of the complex network for their survival, fitness, and propagation. The sum of all these interactions influences plant health vis-a-vis soil fertility (Berg et al., 2020). The advancement in the molecular methods and affordable sequencing has led to a greater understanding of the microbiome composition; however, translating species or gene composition into microbiome functionality still remains a challenge. Using community ecology concepts, Saleem et al. (2019) have indicated that more than individual functions, the overall microbiome biodiversity is critical as the driver of plant growth, soil health, and ecosystem functioning. By meta-analysis of numerous publications on microbial biodiversity and ecosystem functioning (BEF), they indicated that the impacts can be classified into (i) biodiversity effects (negative, no (or unknown), and positive effects of biodiversity on microbial derived services), (ii) assessed functions (nutrient cycling, protection from different stresses, etc.), and (iii) underlying mechanisms (cooperation, mutualism, etc.). Higher diversity can increase the number and resilience of plant-beneficial functions that can be co-expressed and can unlock the expression of plant-beneficial traits that are hard to obtain from any species in isolation. Therefore, the maintenance and modulation of desired microbial activities (functional pools) in the vicinity of the plant system may have more significant potential to provide crops with required nutrition and other protection systems (Figure 2).
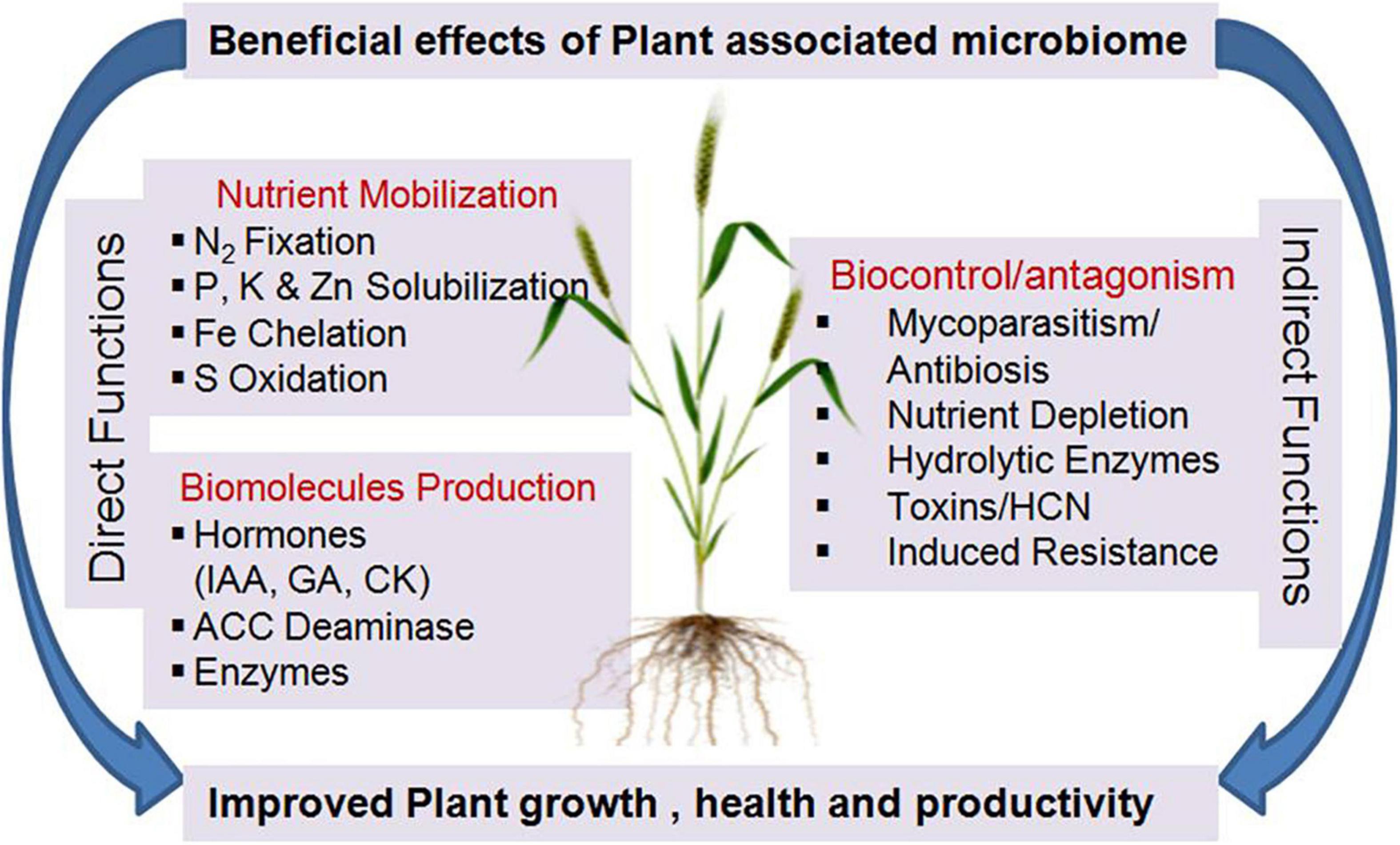
Figure 2. Beneficial functions of Plant associated microbiome. N, Nitrogen; P, Phosphorous; K, Potassium; Zn, Zinc; Fe, Iron; S, Sulfur; IAA, Indole Acetic Acid; GA, Giberrelic Acid; CK, Cytokinin; ACC, 1-AminoCyclopropane Carboxylate; HCN, Hydrocyanic Acid.
With increasing knowledge of plant microbiome vis-à-vis plant performance, approaches are being devised for tapping the potential of plant-growth-promoting (PGP) isolates, by employing both culturable and unculturable approaches. The advent of “omics” technologies understandably provides the tools for a broader understanding of microbial ecosystems and their dynamic interaction with their hosts. These techniques and methods enable the screening of large microbial populations and easily identify the individual or groups of taxa with functional capabilities. Large-scale genomic analyses of plant-associated bacteria have indicated that the bacteria from phyla Actinobacteria, Bacteroidetes, Firmicutes, and Proteobacteria are dominant in different plant niches (Levy et al., 2018a,b). The exhaustive investigations on wheat seeds followed by rhizospheric, epiphytic, and endophytic bacterial diversity, growing in six diverse agro-climatic zones in India, led to more than 200 diverse bacterial isolates with PGP traits (Suman et al., 2016; Verma et al., 2016, 2019; Verma and Suman, 2018; Sai Prasad et al., 2021). The PGP rhizobacteria (PGPR) can adapt easily to adverse conditions and protect the host plants from the deleterious effects of specific environmental stresses (Glick et al., 1997). Several bacteria like Bacillus sp., Azospirillum, Herbaspirillum, and pink-pigmented methylotrophic bacteria have been shown to mitigate stress conditions in maize, wheat, and other crops (Chakraborty et al., 2013; Vurukonda et al., 2016; Curá et al., 2017; Ahlawat et al., 2018). Various factors related to host, microbes, and the environment influence the community composition and diversity of plant microbiome (Dastogeer et al., 2020). Our knowledge on the underlying mechanism(s) of microbiome assemblages and how they influence the host plants is still lacking. How the entire assembly of microbial communities interfere with the host fitness and health remains largely unknown. Connecting the microbiome composition comprising PGP as well as plant-growth-compromising activities and diversity to their function is a great challenge for future research.
These fundamental, microbial-mediated adaptive functions can help address the significant challenges in sustainable food production under the changing climatic conditions. Likewise, the strategic application of microbial communities rather than as individual isolates to improve plant production offers enormous potential, particularly under adverse environmental conditions. Their applications can serve multiple purposes, such as reducing climate change impact and avoiding excessive reliance on chemical fertilizers and pesticides. Earlier studies solely based on culture-dependent techniques have overlooked the benefits of collective microbial functional and genetic diversity and the advantages of the culture-independent methods (Banik and Brady, 2010; Stewart, 2012; Turner et al., 2013; De Souza et al., 2016; Waigi et al., 2017; Armanhi et al., 2018; Mourad et al., 2018).
The cultivable isolates of the microbial community members such as plant probiotics, biofertilizers, or agricultural bioinoculants have shown their distinct influences on plant growth, fitness, and stress resilience but with certain limitations. The developed formulations containing one or more beneficial microorganism strains (or species) can mediate the cycling of several elements from the soil and transform them into the more readily available form of nutrients for plant uptake. Not only do the probiotic action of these formulations increase the growth, yield, and quality of plants, but they are also a tool to produce high-quality functional foods. The use of microbial-based agricultural inputs has a long history, beginning with broad-scale rhizobial inoculation of legumes in the early twentieth century (Desbrosses and Stougaard, 2011). The “Fresh” Green Revolution, perhaps the Bio-Revolution, needs to be based on fewer intensive inputs with reduced environmental impact. It would be based on biological inputs through utilization of the phytomicrobiome (with inoculants, microbially produced compounds, etc.) and improved crops (by manipulation of the phytomicrobiome community structure) (Timmusk et al., 2017; Backer et al., 2018). With increasing data availability on plant microbiome from different ecological niches, strategic approaches based on the concomitant use of culture-dependent and culture-independent techniques, targeting all the plant-beneficial microbial groups, are necessitated to develop novel biological products in all categories like biofertilizers, biopesticides, bioagents, or bioinoculants and biostimulants.
Potential of Bioinoculants for Field Application
The current knowledge on functions, ecological adaptations, host interactions, and putative beneficial traits of microorganisms associated with the host plants mainly revolves around a handful of cultivable rhizospheric and endophytic bacteria or fungi. Many microbial formulations having individual or mixture of strains are developed and used at present. These biological or bioinoculants are nitrogen fixers, phosphate solubilizers, siderophore producers, photohormone producers, and exopolysaccharide producers. Some of them are involved in lytic enzyme production against pests and pathogens, antibiosis, and induced systemic resistance (Gupta et al., 2015; Sruthilaxmi and Babu, 2017).
The bioinoculants are grouped as either biofertilizers or bioagents depending on the intended purpose of plant growth promotion or protection, respectively. The biofertilizers include the individual species of Azotobacter, Azospirillum, and Rhizobium; phosphate-, potassium-, and zinc-solubilizing bacteria; vesicular–arbuscular mycorrhiza (VAM), and Acetobacter. Crop-specific biofertilizers like Gluconacetobacter diazotrophicus for sugarcane or generic biofertilizers like Pantoea isolates showing multi-PGP activities in several crops have demonstrated benefits in improving crop yield and productivity (Suman et al., 2005, 2008). Not only the rhizosphere-colonizing but also several endosphere-colonizing bacteria have been exploited for their beneficial contributions in sustainable agriculture (White et al., 2019). Presently, bioinoculants are available mostly as single entities (Bashan et al., 2014) but are also being formulated as consortia with multiple bacteria and fungi, which have synergistic PGP traits for improving plant production and productivity. Tables 1, 2 summarize the current status of various microbial formulations developed using single, dual, or multiple isolates as bioinoculants to improve nutrient uptake or protect against various biotic and abiotic stresses.
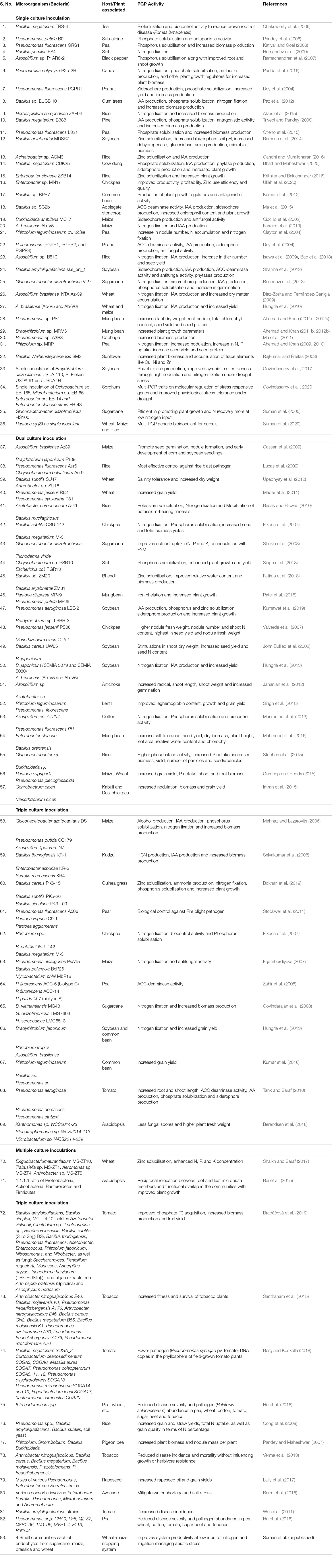
Table 1. Status of various microbial inoculants developed as synthesized microbial communities in use for improving nutrient uptake and protections against plant pathogens.
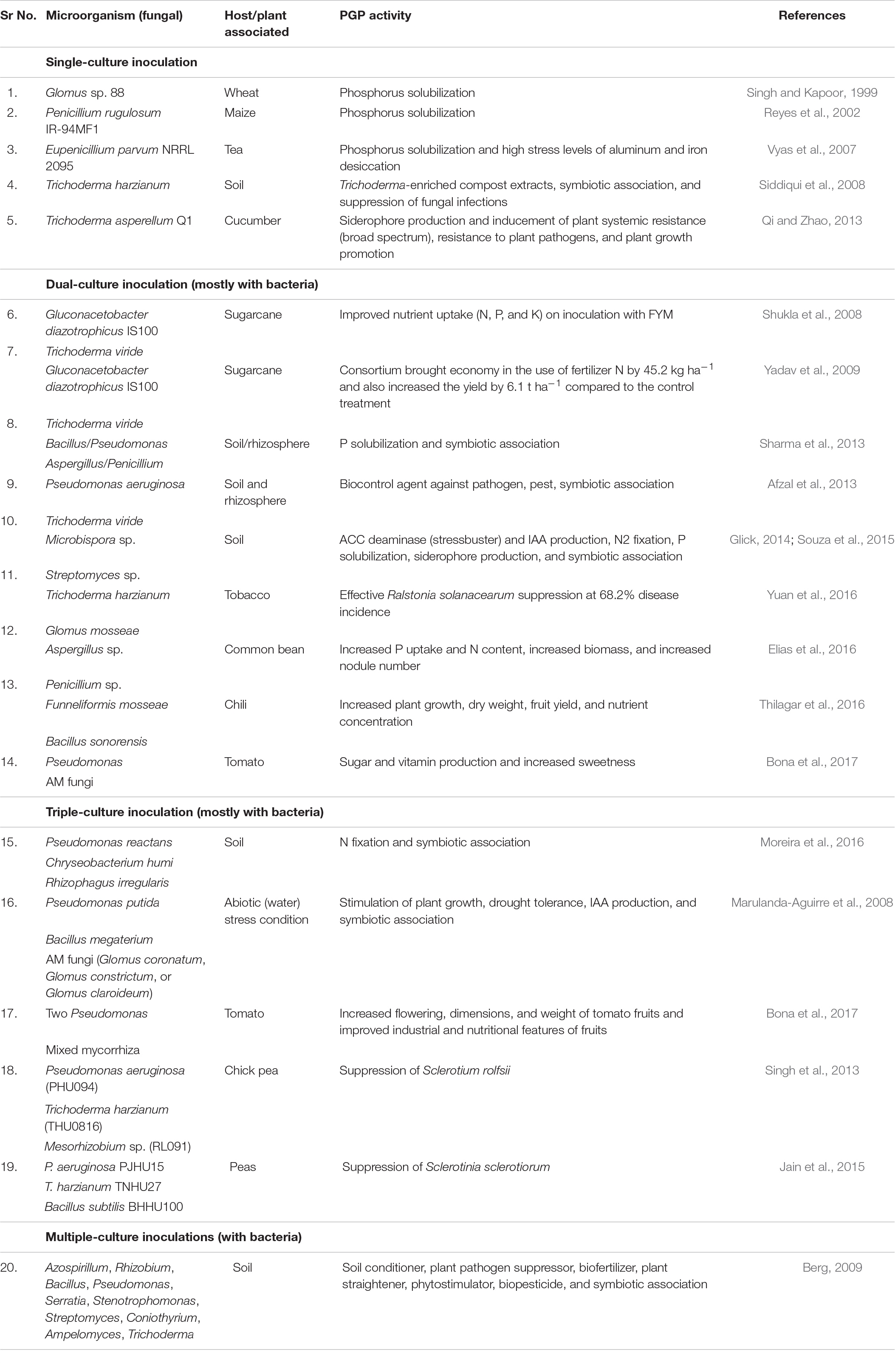
Table 2. Fungal inoculants developed as synthesized microbial communities used for improving nutrient uptake and protections against plant pathogens.
Although the biofertilizer/bioinoculant technology has grown into a proven biological or biotechnological innovation, it is still struggling to get acceptability and popularity with farmers, the end-users. The availability and quality of bioinoculants and their inconsistent performances under field conditions have been identified as significant issues in their adoption by the farming community (Martínez-Hidalgo et al., 2019), which requires the attention of the policymakers in different countries. Along with the development, large-scale production, and assured quality of bioinoculants, one of the most promising ways to increase their efficacy is by introducing effective delivery systems. The farmers may repose the faith, buy these products confidently, and compare their usefulness and cost–benefit ratios with conventional fertilizer inputs. Many studies on bioinoculant development and laboratory-based and field studies proving their worth indicate that these microbial resources must be considered a partial replacement as the application of chemicals may not be wholly replaceable or transferable into biologicals or microbials (Sessitsch et al., 2019).
Designing Targeted Synthetic Bioinoculants
The natural microbial communities are composed of a mix of microbes with often unknown functions. A promising way to overcome the difficulties associated with studying natural communities is to create artificial synthetic communities that retain the key features of their natural counterparts. With reduced complexity, synthetic microbial communities behave like a defined system and can act as a model system to assess the role of key ecological, structural, and functional features of communities in a controlled way (Großkopf and Soyer, 2014).
The existing thought process of top-down and bottom-up approaches for synthesizing microbial communities is based on the functional character of the individual microbial isolate and metabolic interactions among isolates, respectively. Basic motifs of commensalism, competition, predation, cooperation, and amensalism are the key metabolic interactions for the common substrate or metabolites leading to the community formations (Großkopf and Soyer, 2014). Several reviews have summarized the study of ecological interactions among microbes in synthetic as well as in natural microbial communities (Faust and Raes, 2012; Mitri and Richard Foster, 2013). Linking the composition of microbial communities with the functions is a central challenge in microbial ecology. It may be linked in some systems, but not in others, as some functions are restricted to certain taxa (e.g., sulfate reduction), but other functions are widespread across diverse groups (e.g., photosynthesis). A microbiome may contain both phylogenetic and functional redundancy. Many novel insights on the microbial community composition and organization of plant microbiomes of several crops have come from metagenomic studies using high-throughput sequencing (Edwards et al., 2015; Beckers et al., 2016; Wagner et al., 2016). Metagenomics enables the study of all microorganisms, cultured or not, through the analysis of genomic data obtained directly from an environmental sample, providing knowledge of the species present and information regarding the functionality of microbial communities in their natural habitat. Functional metagenomics has been utilized, with much success, to identify many novel genes, proteins, and secondary metabolites such as antibiotics with industrial, biotechnological, pharmaceutical, and medical relevance (Culligan and Sleator, 2016).
A microbiome may contain both phylogenetic and functional redundancy. Phylogenetic redundancy occurs when multiple OTUs from the same lineage are present in a microbiome, while functional redundancy occurs when multiple OTUs perform the same action (e.g., nitrogen fixation) within a microbiome (Shade and Handelsman, 2012). Phylogenetic redundancy is important for defining the core microbiome, which may buffer the ecological disturbances and enable the recovery of community functions. Several reports on human microbiome indicate that gut microbiome disturbances due to heavy antibiotics are restored due to the redundancy of the core group only (Antonopoulos et al., 2009). It carries relevance in agriculture as different agri-management systems lead to the disturbances in soil microbiome vis-a-vis plant microbiome. Recently, Berg et al. (2021) summarized the effects of microbial inoculants on the indigenous plant microbiome and termed this unexplored mode of action as “microbiome modulation.”
Synthetic microbial community analysis in gnotobiotic systems is a valuable approach to create reproducible conditions to experimentally test microbial interactions in situ. Such systems have been developed for animal and plant models including the well-studied plant Arabidopsis thaliana. With established huge volume of data on the metagenome of different crops, there is a need for its translation to certain tailored microbiome-based solutions for promoting plant growth under a range of environmental conditions and increasing resilience to biotic and abiotic stresses. The genomic data with taxonomic status, habitat compatibility, and functional trait knowledge including metabolic potential of plant microbiome communities can be followed as the approach for designing effective microbial inoculants. Here, based on phylogenetic or functional redundancy, two approaches for synthesizing microbial-communities-based bioinoculants are discussed.
Community-Based SB
Microbial colonization in the plant root rhizosphere is the outcome of the interplay between roots exuding chemical compounds that microbes capture as signals and on which their survival and perpetuance depend. The differential abundance of colonizing microbes and the establishment of core-microbiome-based microbial communities forms the basis for plant–microbe interactions. The core members remain present throughout the development of the crop, which may be joined by other taxa during the crop growth. The metagenome data about the relative abundance of colonizing phyla/taxa and core microbiome in the plant rhizosphere and endosphere form the basis for developing Community-Based SB (CSB). Microbial isolates representing the abundant phyla can be sourced either from the crop associated culture bank or with targeted culturomics, for developing the synthetic community. The isolates are expected to be rich in community-forming characteristics like motility, chemotaxis ability, quorum sensing, metabolic diversity, and others. This approach is a direct microbiome manipulation where inoculated CSB may serve to reduce the time required for the rhizosphere microbiome to achieve niche saturation and competitive exclusion of pathogens (Bakker et al., 2012).
Taye et al. (2020) reported that in field-grown Brassica napus, rhizosphere core genera found at each growth stage were generally part of the overall core taxa at the 75% prevalence threshold. Arthrobacter, Bradyrhizobium, and an unclassified Acidobacteria in the class Ellin6075 were present in all growth stages, while other genera joined at the flowering or harvesting stage, as the recruitment of the microbiome is governed majorly by the host plant. Metagenome analysis of more than 600 Arabidopsis thaliana plants from eight diverse, inbred accessions growing at different locations indicated that the core endophytic microbiome is less diverse than their corresponding rhizosphere soil microbiomes. The soil types influenced the microbial communities in the A. thaliana rhizosphere, but the endophytic communities were overlapping and less complex with maximum of actinobacteria and selected proteobacteria. Lundberg et al. (2012) concluded that the host plants influenced the bacterial colonization in the rhizosphere which varied between inbred lines of Arabidopsis, but in the endophytic compartment, it remained consistent across different soil types. An extensive bacterial culture collection that captures a large part of the natural microbial diversity of healthy A. thaliana plants was established (Bai et al., 2015). Carlström et al. (2019) conducted dropout and late introduction experiments by inoculating A. thaliana with synthetic communities from a resource of 62 native bacterial strains to test how arrival order shapes community structure and indicated that individual Proteobacteria (Sphingomonas and Rhizobium) and Actinobacteria (Microbacterium and Rhodococcus) strains have the greatest potential to affect community structure as keystone species.
Similar influences of maize inbred lines growing in different soils and agri-management systems suggested the substantial variation in α- or β-bacterial diversity and relative abundances of taxa with a small proportion of heritable variation across fields. Despite significant differences between the microbial community profiles of maize inbreeds, the estimated α- and β-diversity could not define the kinship of the 27 maize inbreeds to supplement the diversification history of maize (Peiffer et al., 2013). Edwards et al. (2015) resolved the distinct nature in the microbiomes associated with rhizosphere, rhizoplane, and endosphere of rice roots, influenced by the growing conditions and genotypes.
The functional diversity within microbial communities enables metabolic cooperation toward accomplishing more complex functions than those possibly exhibited by a single organism. The consortium members or communities can communicate by exchanging metabolites or molecular signals to coordinate their activity through temporal and spatial expression and further execution of required functions. In contrast with monocultures, microbial members at the community level can self-organize to form spatial patterns, as observed in biofilms or soil aggregates. This self-organization enables them to adapt to the gradient changes, improve resource interception, and exchange metabolites more effectively (Zhang and Wang, 2016; Ben Said and Or, 2017). Hence, the selection and sourcing of microbial members are very important for the construction of CSBs, and they can be from the microbial communities specific to plant niches like rhizosphere (Huang et al., 2018), endosphere, and phyllosphere (Kong and Glick, 2017). Kong et al. (2018) reviewed the strategies for developing synthetic microbial consortium (SMC) and suggested that the crops with good quality can be a good origin of SMC. Based on next-generation sequencing and network analysis, the core microbes can be isolated from the rhizospheric soils or the plant roots using the web-based platform KOMODO (Known Media Database). Herrera Paredes et al. (2018) designed synthetic bacterial communities based on predominant phyla and demonstrated their effect on developing specific and predictable phenotypes in A. thaliana. Using the plant–bacterium binary-association assays, the effect of bacterial community manipulation was observed on the plant response to phosphate (Pi) starvation. This approach might contribute to microbial communities’ rational design and deployment to improve the host response to biotic and nutritional stresses.
In vitro techniques have demonstrated that the host genotypes and abiotic factors influence the composition of plant microbiomes. At the in vivo level, it is a challenge to define the mechanisms controlling the community dynamicity, its assembly, and the beneficial effects on the plant hosts. In an earlier study, the host-mediated natural selection of bacteria by maize roots was employed to select a simplified synthetic bacterial community consisting of seven strains (Enterobacter cloacae, Stenotrophomonas maltophilia, Ochrobactrum pituitosum, Herbaspirillum frisingense, Pseudomonas putida, Curtobacterium pusillum, and Chryseobacterium indologenes) representing the dominant phyla such as Proteobacteria and Actinobacteria (Niu et al., 2017). By assessing the functional role of these bacterial community combinations using axenic maize seedlings, E. cloacae was identified as the keystone member in this model ecosystem. This model community inhibited the phytopathogenic fungus Fusarium verticillioides, both in vitro and in planta, indicating a stronger benefit to the host plant. The reductionist approaches to disentangle the inherent complexity of microbial communities’ interactions have also been suggested for SynComs to be used as inoculants for a given host to decipher their key functions under the gnotobiotic system (Vorholt et al., 2017). Thus, these recent reports support the strategy of combining unculturable and culturable methods, giving the possibility of assembling a representative, yet simplified, bacterial synthetic communities from the pool of dominant genera present in the system. Figure 3 represents an outline for developing CSB based on the metagenome data and bioinformatic applications for predominant taxa and core microbiome. The key functions for developing such communities are collection of available individual isolates representing predominant taxa or isolating them using culturomic tools. Furthermore, such communities can be strengthened by their ecological interactions and probable functional annotations under gnotobiotic conditions.
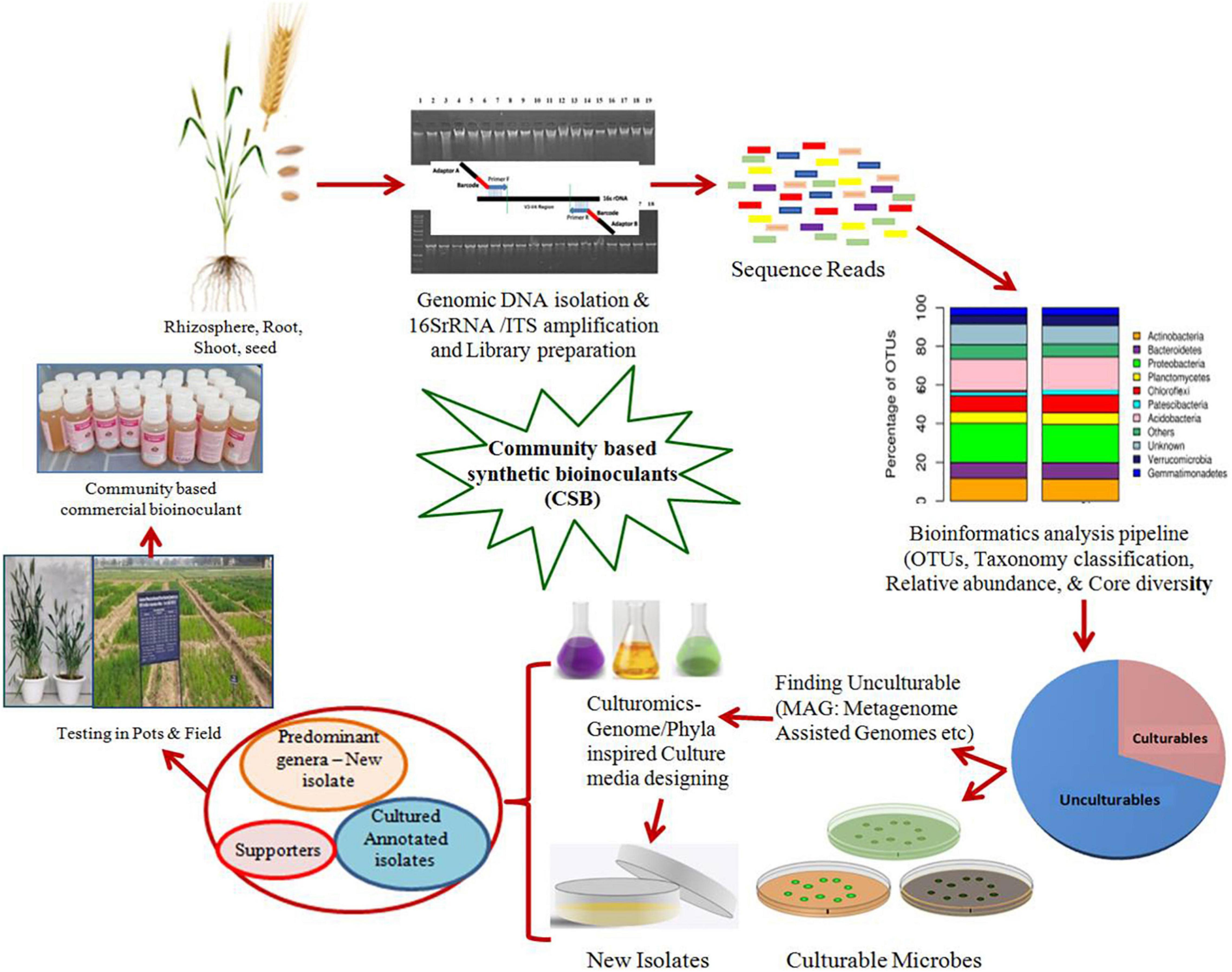
Figure 3. Schematic depiction of different steps for the development of microbial community based synthetic bioinoculants (CSB) by employing metagenomic and bioinformatic techniques.
Function-Based SB
Due to high organic matter, soils with dynamic microbial ecologies typically have lower fertilizer requirements than conventionally managed soils (Bender et al., 2016). Focusing on the functional groups of microorganisms rather than on taxonomic relatedness and manipulating their activities (functional pools) in the vicinity of the plant ecosystem have more significant potential for providing nutrients and stress protection requirements of crops. Further exploration into the mechanisms and specificity of plant growth promotion from these key microorganisms will refine their specific use and maximize the potential inherently possessed by the microbiomes of plants or soils (Parnell et al., 2016). As only a limited proportion of microbial diversity is cultured, there is much scope for culturomics to identify, culture, and include important taxa for their beneficial exploitation (Sarhan et al., 2019). Few commercial products have emerged that take advantage of combining different biofertility products. A bacterial consortium Mammoth P™ consisting of Comamonas testosteroni, P. putida, E. cloacae, and Citrobacter freundii has been reported to enhance phosphate mobility and improve crop productivity twofold (Baas et al., 2016). The combined abilities of Bacillus amyloliquefaciens and the filamentous fungus Trichoderma virens marketed under the trade name QuickRoots® (Monsanto BioAgAlliance, 2015), when applied to field corn, show positive yield improvements ranging from 220 to 500 kg ha–1. Similarly, several microbial consortia have been reported to improve host plants’ nutrition (Shukla et al., 2008; Suman et al., 2008; Dal Cortivo et al., 2018). The synthetic microbial community of P. putida KT2440, Sphingomonas sp. OF178, Azospirillum brasilense Sp7, and Acinetobacter sp. EMM02 has been shown to improve drought stress tolerance in maize (Molina-Romero et al., 2017). Two synthetic microbial communities (SynComs 1 and 2) of known antagonistic Bacillus and other isolates from compost-rich soils inhibited Fusarium wilt symptoms and promoted tomato growth (Tsolakidou et al., 2019). Menéndez and Paço (2020) have explored synergies between rhizobial and non-rhizobial bacteria for beneficial effects on different crops. Woo and Pepe (2018) described Trichoderma and Azotobacter as anchorage microorganisms for developing their respective consortia for promoting plant health and mitigating stress conditions. The established arbuscular mycorrhizal fungi (AMF) system, mainly known for P transport, is also a carrier of endophytes in the plant system, can induce systemic resistance to pathogens, and assists in moisture conservation (Cameron et al., 2013; Rouphael et al., 2015). Through the genomic approach of using multiplex amplicon sequencing of the community-based culture collection, Xu et al. (2016) identified the four most representative genera, Bacillus, Chitinophaga, Rhizobium, and Burkholderia, for the development of bioinoculants. Armanhi et al. (2018) gave a novel methodology for developing a PGP community-based culture collection (CBC) from sugarcane microbiomes, particularly roots and stalks. The CBC recovered 399 unique bacteria, representing 15.9% of the rhizosphere core microbiome and 61.6–65.3% of the endophytic core microbiomes of sugarcane stalks. This synthetic community of highly abundant genera was tested for colonization of maize as the test crop. The inoculated synthetic community efficiently colonized plant organs (53.9%) and improved plant biomass production, indicating their beneficial effects. Hence, the steps for designing Function-Based SB (FSB) essentially involve identifying and culturing the core microbes, selecting the microbes for plant growth functions, optimizing the microbial interactions according to their compatibility and suitable conditions, and assessing the efficacy of these FSBs under in vitro and in vivo conditions for the final release of the formulated product for farmers (Figure 4). Therefore, the FSBs can be foreseen as a small subset of the community from the natural existing microbial communities. Although the FSB may be similar to many other microbial consortia used in different crops, the fundamental difference lies in the functional analysis of the microbiome and the subsequent selection and formulation.
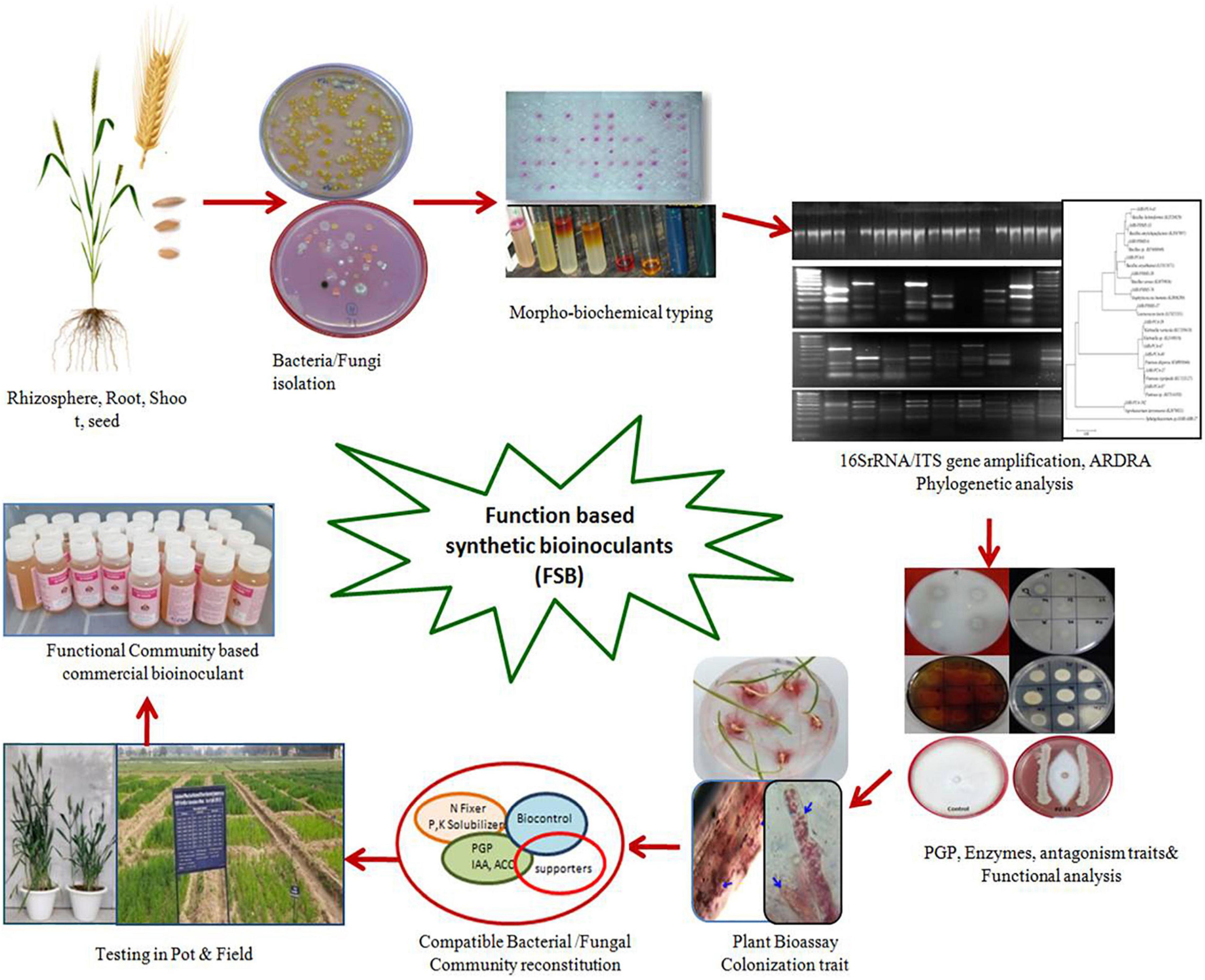
Figure 4. Schematic depiction of different steps for the development of microbial Function based Synthetic Bioinoculants (FSB) using functional characteristics of cultured isolates.
Harmony of Bioinoculants With Sustainable Agriculture Goals
The UN framework of the “2030 Agenda” for 17 Sustainable Development Goals (SDGs) has been adopted by the 193 member states to develop their vision, strategy, and targets for achieving SDGs by effectively making them part of their policies. In its sustainability framework to realize the goal of ending hunger (SDG2), India has several initiatives that include the management of soil health. Successful organic cultivation and integrated agriculture will be highly dependent on the efficient microbiome-based bioinoculants for plant nutrient management and, more importantly, the recycling of crop residues for soil health (Vision 2030, DARE, India). In contrast, many other practices affect the abundance of microbial taxa involved in pest and soil disease suppression and nutrient cycling (Lupatini et al., 2017). The importance of microbiome-based solutions is gaining attention in the interrelated systems of environmental management, sustainable food, and fuel production, and human/animal health (FAO, 2019). There is a strong need for integrated research among soil and microbial scientists, growers, extension clienteles, ecologists, and policymakers to develop strategies to preserve and utilize microbial resources for soil health and crop production (Saleem et al., 2019). The microbiome research also leads to a paradigm shift in preserving axenic samples in culture collections to preserving complex communities such as “microbiome biobanks” with their functional perspectives (Ryan et al., 2021). D’Hondt et al. (2021) have summarized the key role of microbiomes in contributing policies interfacing the SDGs globally and emphasized the investments, collaborations, regulatory changes, and public outreach for innovations in microbiome-based bioeconomies.
Conclusion
The sustainability of the modern agriculture system is critical to feed the continuously growing human and animal populations, wherein the guided use of microbiomes has an inevitable role in promoting plant growth, development, productivity, and nutrient value. The current biofertilizers are based on individual bacterial cultures with specific traits such as N fixation or the solubilization of P or K. But with the detailed diversity and functional analyses of plant-associated microorganisms, a better understanding has emerged that the plant-associated microbiomes have a tremendous and so-far untapped potential to improve the acquisition of nutrients and resilience to abiotic and biotic stresses and, ultimately, the crop yields. The options of generating synthetic communities using taxonomy abundance alone or with functionally annotated predominant taxa are now available for the improved use of microbial resources in crop cultivation. Nevertheless, developing any microbial community requires a collection of promising functionally annotated and compatible isolates in hand, rather than only microbiome data. Hence, it will be appropriate to holistically use the knowledge of unculturable microbiome generated through structural and functional genomics tools and culturable approaches to get the common and rare taxa for synthetic community preparations. The rational workflow for developing community and function-based bioinoculant preparations has been described, which can be used for developing formulations with the targeted functions of nutrient supplementation and stress management in sustainable agriculture.
Author Contributions
AS conceptualized and wrote the manuscript. VG helped in the finalization of tables and figures. KAn and BR gave intellectual input and edited the manuscript. KAs, JS, PS, and DP contributed in data search for the content and table formulation. All authors contributed to the article and approved the submitted version.
Conflict of Interest
The authors declare that the research was conducted in the absence of any commercial or financial relationships that could be construed as a potential conflict of interest.
Publisher’s Note
All claims expressed in this article are solely those of the authors and do not necessarily represent those of their affiliated organizations, or those of the publisher, the editors and the reviewers. Any product that may be evaluated in this article, or claim that may be made by its manufacturer, is not guaranteed or endorsed by the publisher.
Acknowledgments
For this review formulation, authors acknowledge working in ICAR-IARI project “Integrated crop and resource management for enhanced productivity and profitability” under activity “Inquisition, functional annotation and evaluation of endophytic microflora for improved moisture and temperature stress tolerance” (Project 15610190062/1010512) and the DST-SERB project “Exploring seed microbiome of wheat from varied agro-climatic zones to develop synthetic microbials” (Project CRG/2019/002146). The authors acknowledge the NAHEP-CAAST for funding student work. AS acknowledges Joyce S. Khanuja for the figure drawings and the mentor “FloraFauna” foundation for critical suggestions.
References
Abdul Rahman, N. S. N., Abdul Hamid, N. W., and Nadarajah, K. (2021). Effects of abiotic stress on soil microbiome. Int. J. Mol. Sci. 22:9036. doi: 10.3390/ijms22169036
Abiraami, T. V., Suman, A., Singh, B., Aswini, K., and Annapurna, K. (2021). Radiochemical evidence for the contribution of chemotyped siderophore producing bacteria towards plant iron nutrition. Curr. Microbiol. 78, 4072–4083. doi: 10.1007/s00284-021-02658-2658
Afzal, S., Tariq, S., Sultana, V., Ara, J., and Ehteshamul-Haque, S. (2013). Managing the root diseases of okra with endo-root plant growth promoting Pseudomonas and Trichoderma viride associated with healthy okra roots. Pak. J. Bot. 45, 1455–1460.
Ahemad, M., and Khan, M. S. (2009). Toxicity assessment of herbicides quizalafop-p-ethyl and clodinafop towards Rhizobium pea symbiosis. Bull. Environ. Contam. Toxicol. 82, 761–766. doi: 10.1007/s00128-009-9692-x
Ahemad, M., and Khan, M. S. (2010). Comparative toxicity of selected insecticides to pea plants and growth promotion in response to insecticide-tolerant and plant growth promoting Rhizobium leguminosarum. Crop Protection 29, 325–329.
Ahemad, M., and Khan, M. S. (2011a). Effect of tebuconazole-tolerant and plant growth promoting Rhizobium isolate MRP1 on pea-Rhizobium symbiosis. Sci. Horticulturae 129, 266–272. doi: 10.1016/j.scienta.2011.03.036
Ahemad, M., and Khan, M. S. (2011b). Pseudomonas aeruginosa strain PS1 enhances growth parameters of green gram [Vigna radiata (L.) Wilczek] in insecticide-stressed soils. J. Pest Sci. 84, 123–131. doi: 10.1007/s10340-010-0335-330
Ahemad, M., and Khan, M. S. (2012a). Alleviation of fungicide-induced phytotoxicity in greengram [Vigna radiata (L.) Wilczek] using fungicide-tolerant and plant growth promoting Pseudomonas strain. Saudi J. Biol. Sci. 19, 451–459. doi: 10.1016/j.sjbs.2012.06.003
Ahemad, M., and Khan, M. S. (2012b). Productivity of greengram in tebuconazole-stressed soil, by using a tolerant and plant growth-promoting Bradyrhizobium sp. MRM6 strain. Acta Physiol. Plant. 34, 245–254. doi: 10.1007/s11738-011-0823-828
Ahlawat, O. P., Tiwari, R., and Singh, G. P. (2018). Metagenomics of wheat rhizosphere for abiotic stress management. Wheat Barley Res. 10, 64–77. doi: 10.25174/2249-4065/2018/79291
Alves, G. C., Videira, S. S., Urquiaga, S., and Reis, V. M. (2015). Differential plant growth promotion and nitrogen fixation in two genotypes of maize by several Herbaspirillum inoculants. Plant Soil 387, 307–321. doi: 10.1007/s11104-014-2295-2292
Antonopoulos, D. A., Huse, S. M., Morrison, H. G., Schmidt, T. M., Sogin, M. L., and Young, V. B. (2009). Reproducible community dynamics of the gastrointestinal microbiota following antibiotic perturbation. Infect. Immun. 77, 2367–2375. doi: 10.1128/IAI.01520-1528
Armanhi, J. S. L., de Souza, R. S. C., Damasceno, N. D. B., de Araújo, L. M., Imperial, J., and Arruda, P. (2018). A community-based culture collection for targeting novel plant growth-promoting bacteria from the sugarcane microbiome. Front. Plant Sci. 8:2191. doi: 10.3389/fpls.2017.02191
Baas, P., Bell, C., Mancini, L. M., Lee, M. N., Conant, R. T., and Wallenstein, M. D. (2016). Phosphorus mobilizing consortium Mammoth P™ enhances plant growth. PeerJ 4:e2121. doi: 10.7717/peerj.2121
Backer, R., Rokem, J. S., Ilangumaran, G., Lamont, J., Praslickova, D., Ricci, E., et al. (2018). Plant growth-promoting rhizobacteria: context, mechanisms of action, and roadmap to commercialization of biostimulants for sustainable agriculture. Front. Plant Sci. 9:1473. doi: 10.3389/fpls.2018.01473
Bai, Y., Müller, D. B., Srinivas, G., Garrido-Oter, R., Potthoff, E., Rott, M., et al. (2015). Functional overlap of the Arabidopsis leaf and root microbiota. Nature 528, 364–369. doi: 10.1038/nature16192
Bakker, M. G., Manter, D. K., Sheflin, A. M., Weir, T. L., and Vivanco, J. M. (2012). Harnessing the rhizosphere microbiome through plant breeding and agricultural management. Plant Soil 360, 1–13. doi: 10.1007/s11104-012-1361-x
Banik, J. J., and Brady, S. F. (2010). Recent application of metagenomic approaches toward the discovery of antimicrobials and other bioactive small molecules. Curr. Opin. Microbiol. 13, 603–609. doi: 10.1016/j.mib.2010.08.012
Bao, Z., Sasaki, K., Okubo, T., Ikeda, S., Anda, M., Hanzawa, E., et al. (2013). Impact of Azospirillum sp. B510 inoculation on rice-associated bacterial communities in a paddy field. Microbes Environ. 28, 487–490. doi: 10.1264/jsme2.ME13049
Barra, P. J., Inostroza, N. G., Acuña, J. J., Mora, M. L., Crowley, D. E., and Jorquera, M. A. (2016). Formulation of bacterial consortia from avocado (Persea americana Mill.) and their effect on growth, biomass and superoxide dismutase activity of wheat seedlings under salt stress. Appl. Soil Ecol. 102, 80–91. doi: 10.1016/j.apsoil.2016.02.014
Basak, B. B., and Biswas, D. R. (2010). Co-inoculation of potassium solubilizing and nitrogen fixing bacteria on solubilization of waste mica and their effect on growth promotion and nutrient acquisition by a forage crop. Biol. Fertility Soils 46, 641–648. doi: 10.1007/s00374-010-0456-x
Bashan, Y., de-Bashan, L. E., Prabhu, S. R., and Hernandez, J. P. (2014). Advances in plant growth-promoting bacterial inoculant technology: formulations and practical perspectives (1998-2013). Plant Soil 378, 1–33. doi: 10.1007/s11104-013-1956-x
Beckers, B., Op De Beeck, M., Thijs, S., Truyens, S., Weyens, N., et al. (2016). Performance of 16s rDNA primer pairs in the study of rhizosphere and endosphere bacterial microbiomes in metabarcoding studies. Front. Microbiol. 7:650. doi: 10.3389/fmicb.2016.00650
Ben Said, S., and Or, D. (2017). Synthetic microbial ecology: engineering habitats for modular consortia. Front. Microbiol. 8:1125. doi: 10.3389/fmicb.2017.01125
Bender, S. F., Wagg, C., and van der Heijden, M. G. (2016). An underground revolution: biodiversity and soil ecological engineering for agricultural sustainability. Trends Ecol. Evol. 31, 440–452. doi: 10.1016/j.tree.2016.02.016
Beneduzi, A., Moreira, F., Costa, P. B., Vargas, L. K., Lisboa, B. B., Favreto, R., et al. (2013). Diversity and plant growth promoting evaluation abilities of bacteria isolated from sugarcane cultivated in the South of Brazil. Appl. Soil Ecol. 63, 94–104. doi: 10.1016/j.apsoil.2012.08.010
Berendsen, R. L., Vismans, G., Yu, K., Song, Y., de Jonge, R., Burgman, W. P., et al. (2018). Disease-induced assemblage of a plant-beneficial bacterial consortium. ISME J. 12, 1496–1507. doi: 10.1038/s41396-018-0093-91
Berg, G. (2009). Plant-microbe interactions promoting plant growth and health: perspectives for controlled use of microorganisms in agriculture. Appl. Microbiol. Biotechnol. 84, 11–18. doi: 10.1007/s00253-009-2092-7
Berg, G., Kusstatscher, P., Abdelfattah, A., Cernava, T., and Smalla, K. (2021). Microbiome modulation—toward a better understanding of plant microbiome response to microbial inoculants. Front. Microbiol. 12:650610. doi: 10.3389/fmicb.2021.650610
Berg, G., Rybakova, D., Fischer, D., Cernava, T., Vergès, M. C. C., Charles, T., et al. (2020). Microbiome definition re-visited: old concepts and new challenges. Microbiome 8:103. doi: 10.1186/s40168-020-00875-0
Berg, M., and Koskella, B. (2018). Nutrient-and dose-dependent microbiome-mediated protection against a plant pathogen. Curr. Biol. 28, 2487–2492. doi: 10.1016/j.cub.2018.05.085
Bhatt, K., and Maheshwari, D. K. (2020). Zinc solubilizing bacteria (Bacillus megaterium) with multifarious plant growth promoting activities alleviates growth in Capsicum annuum L. 3 Biotech 10:36. doi: 10.1007/s13205-019-2033-2039
Bokhari, A., Essack, M., Lafi, F. F., Andres-Barrao, C., Jalal, R., Alamoudi, S., et al. (2019). Bioprospecting desert plant Bacillus endophytic strains for their potential to enhance plant stress tolerance. Sci. Rep. 9:18154. doi: 10.1038/s41598-019-54685-y
Bona, E., Cantamessa, S., Massa, N., Manassero, P., Marsano, F., Copetta, A., et al. (2017). Arbuscular mycorrhizal fungi and plant growth-promoting pseudomonads improve yield, quality and nutritional value of tomato: a field study. Mycorrhiza 27, 1–11. doi: 10.1007/s00572-016-0727-y
Bradáčová, K., Florea, A. S., Bar-Tal, A., Minz, D., Yermiyahu, U., Shawahna, R., et al. (2019). Microbial consortia versus single-strain inoculants: an advantage in PGPM-assisted tomato production? Agronomy 9:105. doi: 10.3390/agronomy9020105
Bulgarelli, D., Rott, M., Schlaeppi, K., van Themaat, E. V. L., Ahmadinejad, N., Assenza, F., et al. (2012). Revealing structure and assembly cues for Arabidopsis root-inhabiting bacterial microbiota. Nature 488, 91–95. doi: 10.1038/nature11336
Cameron, D. D., Neal, A. L., van Wees, S. C., and Ton, J. (2013). Mycorrhiza-induced resistance: more than the sum of its parts? Trends Plant Sci. 18, 539–545. doi: 10.1016/j.tplants.2013.06.004
Carlström, C. I., Field, C. M., Bortfeld-Miller, M., Müller, B., Sunagawa, S., and Vorholt, J. A. (2019). Synthetic microbiota reveal priority effects and keystone strains in the Arabidopsis phyllosphere. Nat. Ecol. Evol. 3, 1445–1454. doi: 10.1038/s41559-019-0994-z
Cassan, F., Perrig, D., Sgroy, V., Masciarelli, O., Penna, C., and Luna, V. (2009). Azospirillum brasilense Az39 and Bradyrhizobium japonicum E109, inoculated singly or in combination, promote seed germination and early seedling growth in corn (Zea mays L.) and soybean (Glycine max L.). Eur. J. Soil Biol. 45, 28–35. doi: 10.1016/j.ejsobi.2008.08.005
Chakraborty, N., Ghosh, R., Ghosh, S., Narula, K., Tayal, R., Datta, A., et al. (2013). Reduction of oxalate levels in tomato fruit and consequent metabolic remodeling following overexpression of a fungal oxalate decarboxylase. Plant Physiol. 162, 364–378. doi: 10.1104/pp.112.209197
Chakraborty, U., Chakraborty, B., and Basnet, M. (2006). Plant growth promotion and induction of resistance in Camellia sinensis by Bacillus megaterium. J. Basic Microbiol. 46, 186–195. doi: 10.1002/jobm.200510050
Christy, C. (2021). Unearthing the Soil Microbiome, Climate Change, Carbon Storage Nexus. Available online at: https://asm.org/Articles/2021/May (accessed September 25, 2021).
Ciccillo, F., Fiore, A., Bevivino, A., Dalmastri, C., Tabacchioni, S., and Chiarini, L. (2002). Effects of two different application methods of Burkholderia ambifaria MCI 7 on plant growth and rhizospheric bacterial diversity. Environ. Microbiol. 4, 238–245. doi: 10.1046/j.1462-2920.2002.00291.x
Clayton, G. W., Rice, W. A., Lupwayi, N. Z., Johnston, A. M., Lafond, G. P., Grant, C. A., et al. (2004). Inoculant formulation and fertilizer nitrogen effects on field pea: nodulation, N2 fixation and nitrogen partitioning. Canadian J. Plant Sci. 84, 79–88. doi: 10.4141/P02-089
Compant, S., Samad, A., Faist, H., and Sessitsch, A. (2019). A review on the plant microbiome: ecology, functions, and emerging trends in microbial application. J. Adv. Res. 19, 29–37. doi: 10.1016/j.jare.2019.03.004
Cong, P. T., Dung, T. D., Hien, T. M., Hien, N. T., Choudhury, A. T., Kecskés, M. L., et al. (2009). Inoculant plant growth-promoting microorganisms enhance utilisation of urea-N and grain yield of paddy rice in southern Vietnam. Eur. J. Soil Biol. 45, 52–61. doi: 10.1016/j.ejsobi.2008.06.006
Conrad, R., Erkel, C., and Liesack, W. (2006). Rice cluster I methanogens, an important group of Archaea producing greenhouse gas in soil. Curr. Opin. Biotechnol. 17, 262–267. doi: 10.1016/j.copbio.2006.04.002
Culligan, E. P., and Sleator, R. D. (2016). From genes to species: novel insights from metagenomics. Front. Microbiol. 7:1181. 01181 doi: 10.3389/fmicb.2016
Curá, J. A., Franz, D. R., Filosofía, J. E., Balestrasse, K. B., and Burgueño, L. E. (2017). Inoculation with Azospirillum sp. and Herbaspirillum sp. bacteria increases the tolerance of maize to drought stress. Microorganisms 5:41. doi: 10.3390/microorganisms5030041
Dal Cortivo, C., Barion, G., Ferrari, M., Visioli, G., Dramis, L., Panozzo, A., et al. (2018). Effects of field inoculation with VAM and bacteria consortia on root growth and nutrients uptake in common wheat. Sustainability 10:3286. doi: 10.3390/su10093286
Dastogeer, K. M. G., Tumpa, F. H., Sultana, A., Akter, M. A., and Chakraborty, A. (2020). Plant microbiome: an account of the factors that shape community composition and diversity. Curr. Plant Biol. 23:100161. doi: 10.1016/j.cpb.2020.100161
De Souza, R. S. C., Okura, V. K., Armanhi, J. S. L., Jorrín, B., Lozano, N., Da Silva, M. J., et al. (2016). Unlocking the bacterial and fungal communities assemblages of sugarcane microbiome. Sci. Rep. 6:28774. doi: 10.1038/srep28774
Desbrosses, G. J., and Stougaard, J. (2011). Root nodulation: a paradigm for how plant-microbe symbiosis influences host developmental pathways. Cell Host Microbe 10, 348–358. doi: 10.1016/j.chom.2011.09.005
Dey, R. K. K. P., Pal, K. K., Bhatt, D. M., and Chauhan, S. M. (2004). Growth promotion and yield enhancement of peanut (Arachis hypogea L.) by application of plant growth-promoting rhizobacteria. Microbiol. Res. 159, 371–394. doi: 10.1016/j.micres.2004.08.004
D’Hondt, K., Kostic, T., McDowell, R., Eudes, F., Singh, B. K., Sarkar, S., et al. (2021). Microbiome innovations for a sustainable future. Nat. Microbiol. 6, 138–142. doi: 10.1038/s41564-020-00857
Díaz-Zorita, M., and Fernández-Canigia, M. V. (2009). Field performance of a liquid formulation of Azospirillum brasilense on dryland wheat productivity. Eur. J. Soil Biol. 45, 3–11. doi: 10.1016/j.ejsobi.2008.07.001
Edwards, J., Johnson, C., Santos-Medellín, C., Lurie, E., Podishetty, N. K., Bhatnagar, S., et al. (2015). Structure, variation, and assembly of the root-associated microbiomes of rice. Proc. Natl. Acad. Sci. U. S. A. 112, E911–E920. doi: 10.1073/pnas.1414592112
Egamberdiyeva, D. (2007). The effect of plant growth promoting bacteria on growth and nutrient uptake of maize in two different soils. Appl. Soil Ecol. 36, 184–189. doi: 10.1016/j.apsoil.2007.02.005
Elias, F., Woyessa, D., and Muleta, D. (2016). Phosphate solubilization potential of rhizosphere fungi isolated from plants in Jimma Zone, Southwest Ethiopia. Int. J. Microbiol. 2016:5472601. doi: 10.1155/2016/5472601
Elkoca, E., Kantar, F., and Sahin, F. (2007). Influence of nitrogen fixing and phosphorus solubilizing bacteria on the nodulation, plant growth, and yield of chickpea. J. Plant Nutrition 31, 157–171. doi: 10.1080/01904160701742097
FAO (2018). Trade and Nutrition Technical Note. Trade Policy Technical Notes 21. Trade and Food Security. Rome: Markets and Trade Division, Food and Agriculture Organization of the United Nations.
FAO (2019). The Biodiversity That is Crucial for Our Food and Agriculture is Disappearing by the Day. Rome: FAO.
Fatima, I. S., Jamil, M. O., Hussain, A. Z., Mumtaz, M. Z., Luqman, M., Hussain, S., et al. (2018). Zinc solubilizing Bacillus sp. ZM20 and Bacillus aryabhattai ZM31 promoted the productivity in Okra (Abelmoschus esculentus L.). Biologia 64, 179–185. doi: 10.1016/j.micres.2017.06.001
Faust, K., and Raes, J. (2012). Microbial interactions: from networks to models. Nat. Rev. Microbiol. 10, 538–550. doi: 10.1038/nrmicro2832
Ferreira, A. S., Pires, R. R., Rabelo, P. G., Oliveira, R. C., Luz, J. M. Q., and Brito, C. H. (2013). Implications of Azospirillum brasilense inoculation and nutrient addition on maize in soils of the Brazilian Cerrado under greenhouse and field conditions. Appl. Soil Ecol. 72, 103–108. doi: 10.1016/j.apsoil.2013.05.020
Gandhi, A., and Muralidharan, G. (2016). Assessment of zinc solubilizing potentiality of Acinetobacter sp. isolated from rice rhizosphere. Eur. J. Soil Biol. 76, 1–8. doi: 10.1016/j.ejsobi.2016.06.006
Glick, B. R. (2014). Bacteria with ACC deaminase can promote plant growth and help to feed the world. Microbiol. Res. 169, 30–39. doi: 10.1016/j.micres.2013.09.009
Glick, B. R., Liu, C., Ghosh, S., and Dumbroff, E. B. (1997). Early development of canola seedlings in the presence of the plant growth-promoting rhizobacterium Pseudomonas putida GR12-2. Soil Biol. Biochem. 29, 1233–1239. doi: 10.1016/S0038-0717(97)00026-26
Govindarajan, M., Balandreau, J., Kwon, S. W., Weon, H. Y., and Lakshminarasimhan, C. (2008). Effects of the inoculation of Burkholderia vietnamensis and related endophytic diazotrophic bacteria on grain yield of rice. Microb. Ecol. 55, 21–37. doi: 10.1007/s00248-007-9247-9249
Govindasamy, V., George, P., Aher, L., Ramesh, S. V., Thangasamy, A., Anandan, S., et al. (2017). Comparative conventional and phenomics approach to assess symbiotic effectiveness of bradyrhizobia strains in soybean [Glycine max (L.) Merrill] to drought. Sci. Rep. 7:6958. doi: 10.1038/s41598-017-06441-3
Govindasamy, V., George, P., Kumar, M., Aher, L., Raina, S. K., Rane, J., et al. (2020). Multi-trait PGP rhizobacterial endophytes alleviate drought stress in a senescent genotype of sorghum [Sorghum bicolor (L.) Moench]. 3 Biotech 10:13. doi: 10.1007/s13205-019-2001-2004
Großkopf, T., and Soyer, O. S. (2014). Synthetic microbial communities. Curr. Opin. Microbiol. 18, 72–77. doi: 10.1016/j.mib.2014.02.002
Gupta, G., Parihar, S. S., Ahirwar, N. K., Snehi, S. K., and Singh, V. (2015). Plant growth promoting rhizobacteria (PGPR): current and future prospects for development of sustainable agriculture. J. Microbial Biochem. Technol. 7, 096–102. doi: 10.1007/978-981-15-6949-4_9
Gurdeep, K. A. U. R., and Reddy, M. S. (2015). Effects of phosphate-solubilizing bacteria, rock phosphate and chemical fertilizers on maize-wheat cropping cycle and economics. Pedosphere 25, 428–437. doi: 10.1016/S1002-0160(15)30010-30012
Hernandez, J. P., de-Bashan, L. E., Rodriguez, D. J., Rodriguez, Y., and Bashan, Y. (2009). Growth promotion of the freshwater microalga Chlorella vulgaris by the nitrogen-fixing, plant growth-promoting bacterium Bacillus pumilus from arid zone soils. Eur. J. Soil Biol. 45, 88–93. doi: 10.1016/j.ejsobi.2008.08.004
Herrera Paredes, S., Gao, T., Law, T. F., Finkel, O. M., Mucyn, T., Teixeira, P. J. P. L., et al. (2018). Design of synthetic bacterial communities for predictable plant phenotypes. PLoS Biol. 16:e2003962. doi: 10.1371/journal.pbio.2003962
Hu, J., Wei, Z., Friman, V. P., Gu, S. H., Wang, X. F., Eisenhauer, N., et al. (2016). Probiotic diversity enhances rhizosphere microbiome function and plant disease suppression. mBio 7:e001790–16. doi: 10.1128/mBio.01790-1716
Huang, L. H., Yuan, M. Q., Ao, X. J., Ren, A. Y., Zhang, H. B., and Yang, M. Z. (2018). Endophytic fungi specifically introduce novel metabolites into grape flesh cells in vitro. PLoS One 13:e0196996. doi: 10.1371/journal.pone.0196996
Hungria, M., Campo, R. J., Souza, E. M., and Pedrosa, F. O. (2010). Inoculation with selected strains of Azospirillum brasilense and A. lipoferum improves yields of maize and wheat in Brazil. Plant Soil 331, 413–425. doi: 10.1007/s11104-009-0262-260
Hungria, M., Nogueira, M. A., and Araujo, R. S. (2013). Co-inoculation of soybeans and common beans with Rhizobia and Azospirilla: strategies to improve sustainability. Biol. Fertility Soils 49, 791–801. doi: 10.1007/s00374-012-0771-775
Imran, A., Mirza, M. S., Shah, T. M., Malik, K. A., and Hafeez, F. Y. (2015). Differential response of kabuli and desi chickpea genotypes toward inoculation with PGPR in different soils. Front. Microbiol. 6:859. doi: 10.3389/fmicb.2015.00859
Isawa, T., Yasuda, M., Awazaki, H., Minamisawa, K., Shinozaki, S., and Nakashita, H. (2009). Azospirillum sp. strain B510 enhances rice growth and yield. Microbes Environ. 25, 58–61. doi: 10.1007/s00248-002-2022-z
Jahanian, A., Chaichi, M. R., Rezaei, K., Rezayazdi, K., and Khavazi, K. (2012). The effect of plant growth promoting rhizobacteria (PGPR) on germination and primary growth of artichoke (Cynara scolymus). Int. J. Agriculture Crop Sci. 4, 923–929.
Jain, A., Singh, A., Singh, S., and Singh, H. B. (2015). Phenols enhancement effect of microbial consortium in pea plants restrains Sclerotinia sclerotiorum. Biol. Control 89, 23–32. doi: 10.1016/j.biocontrol.2015.04.013
John Bullied, W., Buss, T. J., and Kevin Vessey, J. (2002). Bacillus cereus UW85 inoculation effects on growth, nodulation, and N accumulation in grain legumes: field studies. Canadian J. Plant Sci. 82, 291–298. doi: 10.4141/P01-048
Katiyar, V., and Goel, R. (2003). Solubilization of inorganic phosphate and plant growth promotion by cold tolerant mutants of Pseudomonas fluorescens. Microbiol. Res. 158, 163–168. doi: 10.1078/0944-5013-5188
Kong, Z., and Glick, B. R. (2017). The role of plant growth-promoting bacteria in metal phytoremediation. Adv. Microbial Physiol. 71, 97–132. doi: 10.1016/bs.ampbs.2017.04.001
Kong, Z., Hart, M., and Liu, H. (2018). Paving the way from the lab to the field: using synthetic microbial consortia to produce high-quality crops. Front. Plant Sci. 9:1467. doi: 10.3389/fpls.2018.01467
Kopittke, P. M., Menzies, N. W., Wang, P., McKenna, B. A., and Lombi, E. (2019). Soil and the intensification of agriculture for global food security. Environ. Int. 132:105078. doi: 10.1016/j.envint.2019.105078
Krithika, S., and Balachandar, D. (2016). Expression of zinc transporter genes in rice as influenced by zinc-solubilizing Enterobacter cloacae strain ZSB14. Front. Plant Sci. 7:446. doi: 10.3389/fpls.2016.00446
Kumar, P., Dubey, R. C., and Maheshwari, D. K. (2012). Bacillus strains isolated from rhizosphere showed plant growth promoting and antagonistic activity against phytopathogens. Microbiol. Res. 167, 493–499. doi: 10.1016/j.micres.2012.05.002
Kumar, P., Pandey, P., Dubey, R. C., and Maheshwari, D. K. (2016). Bacteria consortium optimization improves nutrient uptake, nodulation, disease suppression and growth of the common bean (Phaseolus vulgaris) in both pot and field studies. Rhizosphere 2, 13–23. doi: 10.1016/j.rhisph.2016.09.002
Kumawat, K. C., Sharma, P., Sirari, A., Singh, I., Gill, B. S., Singh, U., et al. (2019). Synergism of Pseudomonas aeruginosa (LSE-2) nodule endophyte with Bradyrhizobium sp. (LSBR-3) for improving plant growth, nutrient acquisition and soil health in soybean. World J. Microbiol. Biotechnol. 35:47. doi: 10.1007/s11274-019-2622-2620
Lally, R. D., Galbally, P., Moreira, A. S., Spink, J., Ryan, D., Germaine, K. J., et al. (2017). Application of endophytic Pseudomonas fluorescens and a bacterial consortium to Brassica napus can increase plant height and biomass under greenhouse and field conditions. Front. Plant Sci. 8:2193. doi: 10.3389/fpls.2017.02193
Levy, A., Gonzalez, I. S., Mittelviefhaus, M., Clingenpeel, S., Paredes, S. H., Miao, J., et al. (2018a). Genomic features of bacterial adaptation to plants. Nat. Genet. 50, 138–150. doi: 10.1038/s41588-017-0012-19
Levy, M. M., Evans, L. E., and Rhodes, A. (2018b). The surviving sepsis campaign bundle: 2018 update. Intensive Care Med. 44, 925–928. doi: 10.1007/s00134-018-5085-5080
Lucas, J. A., Solano, B. R., Montes, F., Ojeda, J., Megias, M., and Manero, F. G. (2009). Use of two PGPR strains in the integrated management of blast disease in rice (Oryza sativa) in Southern Spain. Field Crops Res. 114, 404–410. doi: 10.1016/j.fcr.2009.09.013
Lundberg, D. S., Lebeis, S. L., Paredes, S. H., Yourstone, S., Gehring, J., Malfatti, S., et al. (2012). Defining the core Arabidopsis thaliana root microbiome. Nature 488, 86–90. doi: 10.1038/nature11237
Lupatini, M., Korthals, G. W., de Hollander, M., Janssens, T. K. S., and Kuramae, E. E. (2017). Soil microbiome is more heterogeneous in organic than in conventional farming system. Front. Microbiol. 7:2064. doi: 10.3389/fmicb.2016.02064
Ma, Y., Oliveira, R. S., Wu, L., Luo, Y., Rajkumar, M., Rocha, I., et al. (2015). Inoculation with metal-mobilizing plant-growth-promoting rhizobacterium Bacillus sp. SC2b and its role in rhizoremediation. J. Toxicol. Environ. Health Part A 78, 931–944. doi: 10.1080/15287394.2015.1051205
Ma, Y., Rajkumar, M., Luo, Y., and Freitas, H. (2011). Inoculation of endophytic bacteria on host and non-host plants—effects on plant growth and Ni uptake. J. Hazard. Mater. 195, 230–237. doi: 10.1016/j.jhazmat.2011.08.034
Mäder, P., Kaiser, F., Adholeya, A., Singh, R., Uppal, H. S., Sharma, A. K., et al. (2011). Inoculation of root microorganisms for sustainable wheat-rice and wheat-black gram rotations in India. Soil Biol. Biochem. 43, 609–619. doi: 10.1016/j.soilbio.2010.11.031
Mahmood, S., Daur, I., Al-Solaimani, S. G., Ahmad, S., Madkour, M. H., Yasir, M., et al. (2016). Plant growth promoting rhizobacteria and silicon synergistically enhance salinity tolerance of mung bean. Front. Plant Sci. 7:876. doi: 10.3389/fpls.2016.00876
Marag, P. S., and Suman, A. (2018). Growth stage and tissue specific colonization of endophytic bacteria having plant growth promoting traits in hybrid and composite maize (Zea mays L.). Microbiol. Res. 214, 101–113. doi: 10.1016/j.micres.2018.05.016
Marimuthu, S., Ramamoorthy, V., Samiyappan, R., and Subbian, P. (2013). Intercropping system with combined application of Azospirillum and Pseudomonas fluorescens reduces root rot incidence caused by Rhizoctonia bataticola and increases seed cotton yield. J. Phytopathol. 161, 405–411. doi: 10.1111/jph.12084
Martínez-Hidalgo, P., Maymon, M., Pule-Meulenberg, F., and Hirsch, A. M. (2019). Engineering root microbiomes for healthier crops and soils using beneficial, environmentally safe bacteria. Can. J. Microbiol. 65, 91–104. doi: 10.1139/cjm-2018-2315
Marulanda-Aguirre, A., Azcón, R., Ruiz-Lozano, J. M., and Aroca, R. (2008). Differential effects of a Bacillus megaterium strain on Lactuca sativa plant growth depending on the origin of the arbuscular mycorrhizal fungus coinoculated: physiologic and biochemical traits. J. Plant Growth Regulation 27:10. doi: 10.1007/s00344-007-9024-5
Mehnaz, S., and Lazarovits, G. (2006). Inoculation effects of Pseudomonas putida, Gluconacetobacter azotocaptans, and Azospirillum lipoferum on corn plant growth under greenhouse conditions. Microb. Ecol. 51, 326–335. doi: 10.1007/s00248-006-9039-9037
Mendes, R., Garbeva, P., and Raaijmakers, J. M. (2013). The rhizosphere microbiome: significance of plant beneficial, plant pathogenic, and human pathogenic microorganisms. FEMS Microbiol. Rev. 37, 634–663. doi: 10.1111/1574-6976.12028
Menéndez, E., and Paço, A. (2020). Is the application of plant probiotic bacterial consortia always beneficial for plants? exploring synergies between rhizobial and non-rhizobial bacteria and their effects on agro-economically valuable crops. Life 10:24. doi: 10.3390/life10030024
Mitri, S., and Richard Foster, K. (2013). The genotypic view of social interactions in microbial communities. Annu. Rev. Genet. 47, 247–273. doi: 10.1146/annurev-genet-111212-133307
Molina-Romero, D., Baez, A., Quintero-Hernández, V., Castañeda-Lucio, M., Fuentes-Ramírez, L. E., Bustillos-Cristales, M. D. R., et al. (2017). Compatible bacterial mixture, tolerant to desiccation, improves maize plant growth. PLoS One 12:e0187913. doi: 10.1371/journal.pone.0187913
Monsanto BioAgAlliance (2015). QuickRoots: Bacillus Amyloliquefaciens and Trichoderma Virens Based Inoculant for Corn (ProductInformation). Belgium: Monsantobioag.
Moreira, H., Pereira, S. I., Marques, A. P., Rangel, A. O., and Castro, P. M. (2016). Mine land valorization through energy maize production enhanced by the application of plant growth-promoting rhizobacteria and arbuscular mycorrhizal fungi. Environ. Sci. Pollut. Res. 23, 6940–6950. doi: 10.1007/s11356-015-5914-4
Mourad, E. F., Sarhan, M. S., Daanaa, H. S. A., Abdou, M., Morsi, A. T., Abdelfadeel, M. R., et al. (2018). Plant materials are sustainable substrates supporting new technologies of plant-only-based culture media for in vitro culturing of the plant microbiota. Microbes Environ. 33, 40–49. doi: 10.1264/jsme2.ME17135
Niu, B., Paulson, J. N., Zheng, X., and Kolter, R. (2017). Simplified and representative bacterial community of maize roots. Proc. Natl. Acad. Sci. U. S. A. 114, E2450–E2459. doi: 10.1073/pnas.1616148114
Otieno, N., Lally, R. D., Kiwanuka, S., Lloyd, A., Ryan, D., Germaine, K. J., et al. (2015). Plant growth promotion induced by phosphate solubilizing endophytic Pseudomonas isolates. Front. Microbiol. 6:745. doi: 10.3389/fmicb.2015.00745
Padda, K. P., Puri, A., and Chanway, C. P. (2016). Plant growth promotion and nitrogen fixation in canola (Brassica napus) by an endophytic strain of Paenibacillus polymyxa and its GFP-tagged derivative in a long-term study. Botany 94, 1209–1217. doi: 10.1139/cjb-2016-2075
Pandey, A., Trivedi, P., Kumar, B., and Palni, L. M. S. (2006). Characterization of a phosphate solubilizing and antagonistic strain of Pseudomonas putida (B0) isolated from a sub-alpine location in the Indian Central Himalaya. Curr. Microbiol. 53, 102–107. doi: 10.1007/s00284-006-4590-4595
Pandey, P., and Maheshwari, D. K. (2007). Bioformulation of Burkholderia sp. MSSP with a multispecies consortium for growth promotion of Cajanus cajan. Canadian J. Microbiol. 53, 213–222. doi: 10.1139/w06-118
Parnell, J. J., Berka, R., Young, H. A., Sturino, J. M., Kang, Y., Barnhart, D. M., et al. (2016). From the lab to the farm: an industrial perspective of plant beneficial microorganisms. Front. Plant Sci. 7:1110. doi: 10.3389/fpls.2016.01110
Patel, P., Trivedi, G., and Saraf, M. (2018). Iron biofortification in mungbean using siderophore producing plant growth promoting bacteria. Environ. Sustainabil. 1, 357–365. doi: 10.1007/s42398-018-00031-33
Paz, I. C. P., Santin, R. C. M., Guimarães, A. M., Rosa, O. P. P., Dias, A. C. F., Quecine, M. C., et al. (2012). Eucalyptus growth promotion by endophytic Bacillus spp. Genet. Mol. Res. 11, 3711–3720. doi: 10.4238/2012.August.17.9
Peiffer, J. A., Spor, A., Koren, O., Jin, Z., Tringe, S. G., Dangl, J. L., et al. (2013). Diversity and heritability of the maize rhizosphere microbiome under field conditions. Proc. Natl. Acad. Sci. U. S. A. 110, 6548–6553. doi: 10.1073/pnas.1302837110
Pii, Y., Mimmo, T., Tomasi, N., Terzano, R., Cesco, S., and Crecchio, C. (2015). Microbial interactions in the rhizosphere: beneficial influences of plant growth-promoting rhizobacteria on nutrient acquisition process. a review. Biol. Fertility Soils 51, 403–415. doi: 10.1007/s00374-015-0996-991
Qi, W., and Zhao, L. (2013). Study of the siderophore-producing Trichoderma asperellum Q1 on cucumber growth promotion under salt stress. J. Basic Microbiol. 53, 355–364. doi: 10.1002/jobm.201200031
Rajkumar, M., and Freitas, H. (2008). Effects of inoculation of plant-growth promoting bacteria on N uptake by Indian mustard. Bioresour. Technol. 99, 3491–3498. doi: 10.1016/j.biortech.2007.07.046
Ramachandran, K., Srinivasan, V., Hamza, S., and Anandaraj, M. (2007). “Phosphate solubilizing bacteria isolated from the rhizosphere soil and its growth promotion on black pepper (Piper nigrum L.) cuttings,” in Proceedings of the 1st International Meeting on Microbial Phosphate Solubilization (Dordrecht: Springer), 325–331. doi: 10.1007/978-1-4020-5765-6_51
Ramesh, A., Sharma, S. K., Sharma, M. P., Yadav, N., and Joshi, O. P. (2014). Inoculation of zinc solubilizing Bacillus aryabhattai strains for improved growth, mobilization and biofortification of zinc in soybean and wheat cultivated in Vertisols of central India. Appl. Soil Ecol. 73, 87–96. doi: 10.1016/j.apsoil.2013.08.009
Reyes, I., Bernier, L., and Antoun, H. (2002). Rock phosphate solubilization and colonization of maize rhizosphere by wild and genetically modified strains of Penicillium rugulosum. Microb. Ecol. 44, 39–48. doi: 10.1007/s00248-002-1001-8
Rouphael, Y., Franken, P., Schneider, C., Schwarz, D., Giovannetti, M., Agnolucci, M., et al. (2015). Arbuscular mycorrhizal fungi act as biostimulants in horticultural crops. Sci. Horticulturae 196, 91–108. doi: 10.1016/j.scienta.2015.09.002
Ryan, M. J., Schloter, M., Berg, G., Kostic, T., Kinkel, L. L., Eversole, K., et al. (2021). Development of microbiome biobanks-challenges and opportunities. Trends Microbiol. 29, 89–92. doi: 10.1016/j.tim.2020.06.009
Sai Prasad, J., Aswini, K., Sharma, P., Gond, S., and Suman, A. (2021). A Novel Wheat Matrix Medium (WMM) For rapid isolation of hydrolytic enzyme producing bacterial seed endophytes. Int. J. Curr. Microbiol. Appl. Sci. 9, 2181–2197. doi: 10.20546/ijcmas.2020.912.258
Saleem, M., Hu, J., and Jousset, A. (2019). More than the sum of its parts: microbiome biodiversity as a driver of plant growth and soil health. Ann. Rev. Ecol. Evol. Systemat. 50, 145–168. doi: 10.1146/annurev-ecolsys-110617-062605
Santhanam, R., Baldwin, I. T., and Groten, K. (2015). In wild tobacco, Nicotiana attenuata, variation among bacterial communities of isogenic plants is mainly shaped by the local soil microbiota independently of the plants’ capacity to produce jasmonic acid. Commun. Integrative Biol. 8:e1017160. doi: 10.1080/19420889.2015.1017160
Sarhan, M. S., Hamza, M. A., Youssef, H. H., Patz, S., Becker, M., ElSawey, H., et al. (2019). Culturomics of the plant prokaryotic microbiome and the dawn of plant-based culture media-a review. J. Adv. Res. 19, 15–27. doi: 10.1016/j.jare.2019.04.002
Selvakumar, G., Kundu, S., Gupta, A. D., Shouche, Y. S., and Gupta, H. S. (2008). Isolation and characterization of nonrhizobial plant growth promoting bacteria from nodules of Kudzu (Pueraria thunbergiana) and their effect on wheat seedling growth. Curr. Microbiol. 56, 134–139. doi: 10.1007/s00284-007-9062-z
Sessitsch, A., Pfaffenbichler, N., and Mitter, B. (2019). Microbiome applications from lab to field: facing complexity. Trends Plant Sci. 24, 194–198. doi: 10.1016/j.tplants.2018.12.004
Shade, A., and Handelsman, J. (2012). Beyond the Venn diagram: the hunt for a core microbiome. Environ. Microbiol. 14, 4–12. doi: 10.1111/j.1462-2920.2011.02585.x
Shaikh, S., and Saraf, M. (2017). Biofortification of Triticum aestivum through the inoculation of zinc solubilizing plant growth promoting rhizobacteria in field experiment. Biocatalysis Agricultural Biotechnol. 9, 120–126. doi: 10.1016/j.bcab.2016.12.008
Sharma, S. B., Sayyed, R. Z., Trivedi, M. H., and Gobi, T. A. (2013). Phosphate solubilizing microbes: sustainable approach for managing phosphorus deficiency in agricultural soils. SpringerPlus 2:587. doi: 10.1186/2193-1801-2-587
Shukla, S. K., Yadav, R. L., Suman, A., and Singh, P. N. (2008). Improving rhizospheric environment and sugarcane ratoon yield through bioagents amended farm yard manure in udic ustochrept soil. Soil Tillage Res. 99, 158–168. doi: 10.1016/j.still.2008.02.007
Siddiqui, Y., Meon, S., Ismail, M. R., and Ali, A. (2008). Trichoderma-fortified compost extracts for the control of Choanephora wet rot in okra production. Crop Prot. 27, 385–390. doi: 10.1016/j.cropro.2007.07.002
Singh, A. V., Chandra, R., and Goel, R. (2013). Phosphate solubilization by Chryseobacterium sp. and their combined effect with N and P fertilizers on plant growth promotion. Arch. Agronomy Soil Sci. 59, 641–651. doi: 10.1080/03650340.2012.664767
Singh, S., and Kapoor, K. K. (1999). Inoculation with phosphate-solubilizing microorganisms and a vesicular-arbuscular mycorrhizal fungus improves dry matter yield and nutrient uptake by wheat grown in a sandy soil. Biol. Fertil. Soils 28, 139–144. doi: 10.1007/s003740050475
Singh, S. R., Kundu, D. K., Dey, P., Singh, P., and Mahapatra, B. S. (2018). Effect of balanced fertilizers on soil quality and lentil yield in Gangetic alluvial soils of India. J. Agricultural Sci. 156:225. doi: 10.1017/S0021859618000254
Soto-Giron, M. J., Kim, J. N., Schott, E., Tahmin, C., Ishoey, T., Mincer, T. J., et al. (2021). The edible plant microbiome represents a diverse genetic reservoir with functional potential in the human host. Sci. Rep. 11:24017. doi: 10.1016/j.tplants.2021.09.001
Souza, R. D., Ambrosini, A., and Passaglia, L. M. (2015). Plant growth-promoting bacteria as inoculants in agricultural soils. Genet. Mol. Biol. 38, 401–419. doi: 10.1590/S1415-475738420150053
Sruthilaxmi, C. B., and Babu, S. (2017). Microbial bio-inoculants in Indian agriculture: ecological perspectives for a more optimized use. Agriculture Ecosystems Environ. 242, 23–25. doi: 10.1016/j.agee.2017.03.019
Stephen, J., Shabanamol, S., Rishad, K. S., and Jisha, M. S. (2015). Growth enhancement of rice (Oryza sativa) by phosphate solubilizing Gluconacetobacter sp. (MTCC 8368) and Burkholderia sp. (MTCC 8369) under greenhouse conditions. 3 Biotech 5, 831–837. doi: 10.1007/s13205-015-0286-5
Stewart, E. J. (2012). Growing unculturable bacteria. J. Bacteriol. 194, 4151–4160. doi: 10.1128/JB.00345-312
Stockwell, V. O., Johnson, K. B., Sugar, D., and Loper, J. E. (2011). Mechanistically compatible mixtures of bacterial antagonists improve biological control of fire blight of Pear. Phytopathology 101, 299–299. doi: 10.1094/PHYTO-03-10-0098
Suman, A., Gaur, A., Shrivastava, A. K., and Yadav, R. L. (2005). Improving sugarcane growth and nutrient uptake by inoculating Gluconacetobacter diazotrophicus. Plant Growth Regulation 47, 155–162. doi: 10.1007/s10725-005-2847-9
Suman, A., Shrivastava, A. K., Gaur, A., Singh, P., Singh, J., and Yadav, R. L. (2008). Nitrogen use efficiency of sugarcane in relation to its BNF potential and population of endophytic diazotrophs at different N levels. Plant Growth Regulation 54, 1–11. doi: 10.1007/s10725-007-9219-9216
Suman, A., Shukla, L., Marag, P. S., Verma, P., Gond, S., and Sai, et al. (2020). Potential use of plant colonizing Pantoea as generic plant growth promoting bacteria for cereal crops. J. Environ. Biol. 41, 987–994.
Suman, A., Verma, P., Yadav, A. N., Srinivasamurthy, R., Singh, A., and Prasanna, R. (2016). Development of hydrogel based bio-inoculant formulations and their impact on plant biometric parameters of wheat (Triticum aestivum L.). Int. J. Curr. Microbiol. Appl. Sci. 5, 890–901.
Tank, N., and Saraf, M. (2010). Salinity-resistant plant growth promoting rhizobacteria ameliorates sodium chloride stress on tomato plants. J. Plant Interact. 5, 51–58. doi: 10.1080/17429140903125848
Taye, Z. M., Helgason, B. L., Bell, J. K., Norris, C. E., Vail, S., Robinson, S. J., et al. (2020). Core and differentially abundant bacterial taxa in the rhizosphere of field grown Brassica napus genotypes: implications for canola breeding. Front. Microbiol. 10:3007. doi: 10.3389/fmicb.2019.03007
Thilagar, G., Bagyaraj, D. J., and Rao, M. S. (2016). Selected microbial consortia developed for chilly reduces application of chemical fertilizers by 50% under field conditions. Sci. Hortic. 198, 27–35. doi: 10.1016/j.scienta.2015.11.021
Timmusk, S., Behers, L., Muthoni, J., Muraya, A., and Aronsson, A. C. (2017). Perspectives and challenges of microbial application for crop improvement. Front. Plant Sci. 8:49. doi: 10.3389/fpls.2017.00049
Trivedi, P., and Pandey, A. (2008). Plant growth promotion abilities and formulation of Bacillus megaterium strain B 388 (MTCC6521) isolated from a temperate Himalayan location. Indian J. Microbiol. 48, 342–347. doi: 10.1007/s12088-008-0042-41
Trivedi, P., Leach, J. E., Tringe, S. G., Sa, T., and Singh, B. K. (2021). Plant-microbiome interactions: from community assembly to plant health. Nat. Rev. Microbiol. 19:72.
Tsolakidou, M. D., Stringlis, I. A., Fanega-Sleziak, N., Papageorgiou, S., Tsalakou, A., and Pantelides, I. S. (2019). Rhizosphere-enriched microbes as a pool to design synthetic communities for reproducible beneficial outputs. FEMS Microbiol. Ecol. 95:fiz138. doi: 10.1093/femsec/fiz138
Turner, T. R., James, E. K., and Poole, P. S. (2013). The plant microbiome. Genome Biol. 14, 1–10. doi: 10.3390/microorganisms9010188
Ullah, A., Farooq, M., Nadeem, F., Rehman, A., Hussain, M., Nawaz, A., et al. (2020). Zinc application in combination with zinc solubilizing Enterobacter sp. MN17 improved productivity, profitability, zinc efficiency, and quality of Desi chickpea. J. Soil Sci. Plant Nutrition 20, 2133–2144. doi: 10.1007/s42729-020-00281-283
Upadhyay, S. K., Singh, J. S., Saxena, A. K., and Singh, D. P. (2012). Impact of PGPR inoculation on growth and antioxidant status of wheat under saline conditions. Plant Biol. 14, 605–611. doi: 10.1111/j.1438-8677.2011.00533.x
Valverde, A., Burgos, A., Fiscella, T., Rivas, R., Velazquez, E., Rodríguez-Barrueco, C., et al. (2007). “Differential effects of coinoculations with Pseudomonas jessenii PS06 (a phosphate-solubilizing bacterium) and Mesorhizobium ciceri C-2/2 strains on the growth and seed yield of chickpea under greenhouse and field conditions,” in Proceedings of the 1st International Meeting on Microbial Phosphate Solubilization (Dordrecht: Springer), 43–50. doi: 10.1007/978-1-4020-5765-6_5
Verma, J. P., Yadav, J., Tiwari, K. N., and Kumar, A. (2013). Effect of indigenous Mesorhizobium spp. and plant growth promoting rhizobacteria on yields and nutrients uptake of chickpea (Cicer arietinum L.) under sustainable agriculture. Ecol. Eng. 51, 282–286. doi: 10.1016/j.ecoleng.2012.12.022
Verma, P., and Suman, A. (2018). Wheat microbiomes: ecological significances, molecular diversity and potential bioresources for sustainable agriculture. EC Microbiol. 14, 641–665.
Verma, P., Yadav, A. N., Khannam, K. S., Kumar, S., Saxena, A. K., and Suman, A. (2016). Molecular diversity and multifarious plant growth promoting attributes of Bacilli associated with wheat (Triticum aestivum L.) rhizosphere from six diverse agro-ecological zones of India. J. Basic Microbiol. 56, 44–58. doi: 10.1002/jobm.201500459
Verma, P., Yadav, A. N., Khannam, K. S., Mishra, S., Kumar, S., Saxena, A. K., et al. (2019). Appraisal of diversity and functional attributes of thermotolerant wheat associated bacteria from the peninsular zone of India. Saudi J. Biol. Sci. 26, 1882–1895. doi: 10.1016/j.sjbs.2016.01.042
Vorholt, J. A., Vogel, C., Carlström, C. I., and Müller, D. B. (2017). Establishing causality: opportunities of synthetic communities for plant microbiome research. Cell Host Microbe 22, 142–155. doi: 10.1016/j.chom.2017.07.004
Vurukonda, S. S. K. P., Vardharajula, S., Shrivastava, M., and SkZ, A. (2016). Enhancement of drought stress tolerance in crops by plant growth promoting rhizobacteria. Microbiol. Res. 184, 13–24. doi: 10.1016/j.micres.2015.12.003
Vyas, P., Rahi, P., Chauhan, A., and Gulati, A. (2007). Phosphate solubilization potential and stress tolerance of Eupenicillium parvum from tea soil. Mycol. Res. 111, 931–938. doi: 10.1016/j.mycres.2007.06.003
Wagner, M. R., Lundberg, D. S., Tijana, G., Tringe, S. G., Dangl, J. L., and Mitchell-Olds, T. (2016). Host genotype and age shape the leaf and root microbiomes of a wild perennial plant. Nat. Commun. 7:12151. doi: 10.1038/ncomms12151
Waigi, M. G., Sun, K., and Gao, Y. (2017). Sphingomonads in microbe-assisted phytoremediation: tackling soil pollution. Trends Biotechnol. 35, 883–899. doi: 10.1016/j.tibtech.2017.06.014
Wei, Z., Yang, X., Yin, S., Shen, Q., Ran, W., and Xu, Y. (2011). Efficacy of Bacillus-fortified organic fertiliser in controlling bacterial wilt of tomato in the field. Appl. Soil Ecol. 48, 152–159. doi: 10.1016/j.apsoil.2011.03.013
White, J. F., Kingsley, K. L., Zhang, Q., Verma, R., Obi, N., Dvinskikh, S., et al. (2019). Endophytic microbes and their potential applications in crop management. Pest. Manag. Sci. 75, 2558–2565. doi: 10.1002/ps.5527
Williams, S. C. (2013). The other microbiome. Proc. Natl. Acad. Sci. U. S. A. 110, 2682–2684. doi: 10.1073/pnas.1300923110
Woo, S. L., and Pepe, O. (2018). Microbial consortia: promising probiotics as plant biostimulants for sustainable agriculture. Front. Plant Sci. 9:1801. doi: 10.3389/fpls.2018.01801
Xu, W., Liu, L., He, T., Cao, M., Sha, L., Hu, Y., et al. (2016). Soil properties drive a negative correlation between species diversity and genetic diversity in a tropical seasonal rainforest. Sci. Rep. 6:20652. doi: 10.1038/srep20652
Yadav, R. L., Suman, A., Prasad, S. R., and Prakash, O. (2009). Effect of Gluconacetobacter diazotrophicus and Trichoderma viride on soil health, yield and N-economy of sugarcane cultivation under subtropical climatic conditions of India. Eur. J. Agron. 30, 296–303. doi: 10.1016/j.eja.2009.01.002
Yuan, S., Li, M., Fang, Z., Liu, Y., Shi, W., Pan, B., et al. (2016). Biological control of tobacco bacterial wilt using Trichoderma harzianum amended bioorganic fertilizer and the arbuscular mycorrhizal fungi Glomus mosseae. Biol. Control 92, 164–171. doi: 10.1016/j.biocontrol.2015.10.013
Zahir, Z. A., Munir, A., Asghar, H. N., Shaharoona, B., and Arshad, M. (2008). Effectiveness of rhizobacteria containing ACC deaminase for growth promotion of peas (Pisum sativum) under drought conditions. J. Microbiol. Biotechnol. 18, 958–963.
Keywords: bioinoculants, PGPRs, plant microbial communities, novel biologicals, microbiome
Citation: Suman A, Govindasamy V, Ramakrishnan B, Aswini K, SaiPrasad J, Sharma P, Pathak D and Annapurna K (2022) Microbial Community and Function-Based Synthetic Bioinoculants: A Perspective for Sustainable Agriculture. Front. Microbiol. 12:805498. doi: 10.3389/fmicb.2021.805498
Received: 30 October 2021; Accepted: 29 December 2021;
Published: 11 March 2022.
Edited by:
Prem Lal Kashyap, Indian Institute of Wheat and Barley Research (ICAR), IndiaReviewed by:
Ahmed Elhady, Institute for Epidemiology and Pathogen Diagnostics, Julius Kühn-Institute, GermanyPratiksha Singh, Guangxi University for Nationalities, China
Copyright © 2022 Suman, Govindasamy, Ramakrishnan, Aswini, SaiPrasad, Sharma, Pathak and Annapurna. This is an open-access article distributed under the terms of the Creative Commons Attribution License (CC BY). The use, distribution or reproduction in other forums is permitted, provided the original author(s) and the copyright owner(s) are credited and that the original publication in this journal is cited, in accordance with accepted academic practice. No use, distribution or reproduction is permitted which does not comply with these terms.
*Correspondence: Archna Suman, YXJjaHN1bWFuQHlhaG9vLmNvbQ==