- Department of Nutrition, Dietetics and Food Sciences, Utah State University, Logan, UT, United States
Organic acids are widely employed in the food industry to control growth of microbial pathogens such as Listeria monocytogenes and Escherichia coli. There is substantial evidence that intracellular accumulation of acid anions is a major inhibitor to cell viability, and that some bacteria are able to combat the toxic effects of anion accumulation via their ability to continue active metabolism at a lower intracellular pH (pHi). This study followed the accumulation of acid anion into the cell pellet and parallel changes in pHi in two human pathogenic strains of L. monocytogenes (N1-227 and R2-499) and in E. coli O157:H7 after exposure to sub-bacteriostatic levels of lactic and acetic acids at mildly acidic pH 6. The methodology employed in these studies included independent measures of pHi and intracellular anion accumulation. For the latter work, cells were pelleted through bromododecane to strip off extracellular water and solutes. Listeria strains accumulated 1.5-fold acetate or 2.5-fold lactate as compared to the external environment while mounting a defense against anion accumulation that included up to a 1-unit pHi drop from 7.5 to 6.5 for strain R2-499. E. coli accumulated 2.5-fold acetate but not lactate and apparently made use of combat mechanisms other than lowering pHi not explored in this study. Inulin was employed to estimate the fractional volume of cell pellet present as intracellular space. That intracellular fraction was 0.24 for E. coli, which infers that acid accumulation into the intercellular space was minimally 4 × that measured for the entire pellet. An intercellular fraction of pellet was not measurable for strains of L. monocytogenes. The data also bring into question the efficacy across bacterial species of the common, but confounding, practice of using intracellular anion accumulation as a measure of pHi, and vice versa.
Introduction
Listeria monocytogenes and Escherichia coli are pathogens of concern for foodborne illness, and each is among the top five causes of food-related death (Mead et al., 1999; Scallan et al., 2011). Both pathogens are hardy organisms that can survive in many different types of environments, including food production facilities (LeJeune et al., 2001; Popovic et al., 2014). The food industry often uses organic acids in the environment and as food additives to limit growth of these and other pathogens. For example, acetic and lactic acids are used to decontaminate meat surfaces of E. coli and L. monocytogenes (Berry and Cutter, 2000) and as antilisterial additive in meat products (Dziezak, 2016).
It is hypothesized that intracellular accumulation of acid anions is a primary mechanism by which organic acids exert antimicrobial activity (Mani-López et al., 2012), and that such accumulation is driven by the difference in external and internal pH (Carpenter and Broadbent, 2009). It is also understood that some bacteria can lower their internal pH (pHi) as a primary mechanism to combat accumulation of organic acid anions (Russell, 1992; Diez-Gonzalez and Russell, 1997). Other researchers have focused on the external protonated acid driving internalization of acid anion (Buchanan et al., 1994; Romick and Fleming, 1998; Pieterse et al., 2005). In contrast, Carpenter and Broadbent (2009) assert in their hypothesis paper that external protonated acid is merely a shuttle, not a driving force for intracellular anion accumulation. Their hypothesis paper points out that a failure to control external anion concentration has confounded results and likely led to misleading conclusions regarding the antimicrobial action of organic acids.
Hence, this study explored the extent to which mid-log phase cells of two human pathogenic strains of L. monocytogenes and one E. coli O157:H7 strain accumulate acid anions and respond with altered pHi when exposed to inhibitory, but less-than-bacteriostatic, levels of organic acids at mildly acidic pH 6 typical of mildly acidic foods such as processed meats. External concentration of organic acids was set at 4.75 mM for both lactic and acetic acid consistent with our previous studies using the two Listeria strains (Zhang et al., 2014; Zhang et al., 2015; Li et al., 2021). E. coli O157:H7, a Gram-negative and broadly studied human pathogen, was included to provide a basis for comparison.
Materials and Methods
Experimental Overview and Statistical Analysis
Experiments were conducted to follow the extent to which added organic acids induced relative accumulation of organic acid anions into the cell pellet and to identify the fractional volume of cell pellet not available to inulin (i.e., intracellular space). Parallel experiments were conducted to follow the impact of treatments on internal pH of the bacterial cells. Independent experiments were performed using two human pathogenic strains of L. monocytogenes and E. coli O157:H7. Each experiment included four trials, with three replications per trial.
For the acid accumulation experiments, treatment levels included TSB media containing 4.75 mM added lactic or acetic acid and adjusted to pH 6 with HCl. The dependent measure was a relative accumulation factor calculated as:
The accumulation factor was considered significant when its 95% CI > 1. Treatment means were compared using one-way analysis of variance (ANOVA), and differences were identified by Tukey’s test at p < 0.05.
For the inulin experiments, one treatment level (standard TSB adjusted to pH 6 with HCl) was applied to identify the fraction of the pellet volume unavailable to inulin (i.e., a fractional internal cell volume). This fractional volume was calculated as the C14:H3 ratio of pellet as compared to the C14:H3 ratio of supernatant. For example, a C14:H3 ratio in pellet that was only 80% of the C14:H3 ratio in the supernatant corresponds to a fractional internal cell volume of 0.2. Calculation of the fractional internal cell volume was only warranted if the C14:H3 ratio in the pellet was lower than the C14:H3 ratio in the supernatant (p < 0.05, t-test).
Four treatments were included in the pHi experiments: the baseline control (TSB pH 7.4), acid control (TSB adjusted to pH 6 with HCl), and TSB with 4.75 mM added lactic acid or acetic acid and adjusted to pH 6 with HCl. The dependent variable was the pHi measured using a pH-sensitive fluorescent dye as described later. Treatment means were compared using one-way analysis of variance (ANOVA), and differences were identified by Tukey’s test at p < 0.05.
Bacterial Strains and Growth Conditions
Two strains of L. monocytogenes were selected for this study, FSL R2-499 and FLS N1-227. The R2-499 strain was sourced from a human isolate associated with a United States outbreak linked to sliced turkey and the N1-227 strain was sourced from a food isolate associated with a United States outbreak (Li et al., 2021). One strain of E. coli O157:H7 was selected, H1730. This strain was sourced from a human isolate associated with a lettuce outbreak (Jensen et al., 2015).
Parent cultures were stored as frozen stocks at −80°C in tryptic soy broth (TSB, pH 7.4; Becton, Dickinson and Company, Sparks, MD, United States) supplemented with 20% v/v glycerol. Prior to use, cultures were first propagated on tryptic soy agar (TSA; Becton, Dickinson and Company, Sparks, MD, United States) plate and incubated at 37°C for 24 h. A single colony from the TSA plate was transferred into TSB and incubated overnight at 37°C with shaking (220 rpm).
Accumulation of Organic Acid and Inulin Into Cell Pellets
Bacterial cells habituated to treatment media containing added organic acids are expected to proportionately accumulate acid anions into their cytoplasm relative to the supernatant owing to the protonated acid freely entering the cell but then dissociating into a proton and acid anion. On the other hand, bacterial cells habituated to treatment media containing inulin are expected to have proportionally less inulin in the pellet (which holds the cytoplasmic fraction) relative to the supernatant owing to inulin not being able to enter the cell. The proportionate amounts of organic acids and inulin was followed using their C14 radio-labeled tracers, and a tracer of tritium was simultaneously employed because it will freely equilibrate across all cell compartments and the media. Hence, the ratio of C14:H3 indicated relative concentration of the organic acids or inulin in pellet and in supernatant.
Overnight cultures of each strain were harvested by centrifugation (2,500 × g for 10 min; Sorvall RT1, Thermo Fisher Scientific, Germany) at 4°C, and then diluted to an optical density at 600 nm (OD600) of 0.03 in TSB. Cells were acid habituated as described by Zhang et al. (2014). A 1% inoculum (v/v) of diluted overnight cultures was transferred into 10 ml of standard TSB (pH 7.4) and incubated at 37°C for 3 h for E. coli and 4 h for L. monocytogenes with shaking (220 rpm) to reach mid-log phase. Cell density was approximately 107 CFU/ml (Zhang et al., 2014). The cultures were collected by centrifugation (2,500 × g for 10 min at 4°C) and then resuspended in 10 ml of a treatment solution made from standard TSB (pH 6.0 adjusted with HCl, Becton, Dickinson and Company, Sparks, MD, United States). The two acid treatments contained 10 μCi tritiated water (Perkin Elmer, Waltham, MA, United States) and either 4.75 mM acetic acid (Johnson Matthey Company, Ward Hill, MA, United States) containing 1 μCi C14-labeled acetic acid (Perkin Elmer, Waltham, MA, United States), or 4.75 mM L-lactic acid (Sigma Chemicals, St. Louis, MO, United States) containing 1 μCi C14-labeled lactic acid (Perkin Elmer, Waltham, MA, United States). The inulin treatment contained 10 μCi tritiated water and 1 μCi C14-labeled inulin (Perkin Elmer, Waltham, MA, United States).
Cells were incubated for 1 h at 37°C with shaking (220 rpm). After incubation, 0.5 ml of bromododecane (Sigma Aldrich, St. Louis, MO, United States) was added to the centrifuge tube to create a layer between the supernatant and pellet after centrifugation. Cells were collected by centrifugation (2,500 × g for 10 min at 4°C) and then the supernatant was decanted and diluted so that disintegrations per minute (DPM) would be similar to that of the pellet. One milliliter of the diluted supernatant was added to 18 ml of liquid scintillation cocktail (Perkin Elmer, Waltham, MA, United States). The entire cell pellet was cut out of the centrifuge tube using guillotine clippers and then added to 18 ml of liquid scintillation cocktail along with 1 ml of TSB. Liquid volume in both the supernatant and pellet samples was identical (1 ml) to ensure similar counting efficiency. Counts were measured using a scintillation counter (Beckman Coulter, Brea, CA, United States).
Measurement of pHi
The pHi was determined using 5(6)-carboxyfluorescein diacetate N-succinimidyl ester (CFSE; Sigma Aldrich, St. Louis, MO, United States), a membrane-permeant fluorescein-based dye that at 492 nm is sensitive to pH (Siegumfeldt et al., 1999). This procedure generally followed that described by Siegumfeldt et al. (1999) and Cheng et al. (2015). The first step was to determine a calibration curve that would relate fluorescent values to pHi values. Ten milliliters of mid log cells grown in TSB were collected by centrifugation and resuspended in 10 ml of CFSE staining solution (10 μM; prepared from a concentrated stock solution in DMSO by dilution in a 10 mM KH2PO4 buffer) and incubated at 37°C for 30 min. The cells were collected by centrifugation and then suspended in 10 ml of KH2PO4 buffer (pH 6.0) supplemented with 10 mM glucose (to energize the cells) and incubated at 37°C for another 30 min to remove unbound dye. To permeabilize cells and equilibrate intracellular and external pH for the calibration curve, the stained cells were then placed in ethanol (63%, v/v) for 30 min at 37°C. The bacterial cells were harvested by centrifugation (2,500 × g for 10 min at 4°C) and suspended in 10 ml of TSB broth medium whose pH was adjusted with HCl or NaOH to range from 5.0 to 8.0 in 0.5 increments. Fluorescence was measured using a microplate fluorometric reader (BioTek, Winooski, VT, United States). The 492/435 fluorescent ratio was obtained by dividing fluorescence at 492 nm by that at 435 nm. These wavelengths are pH sensitive and pH insensitive, respectively. Undyed cells in TSB were used as a blank to correct for any naturally occurring fluorescence. The calibration curve was plotted by polynomial fitting between ratio 492/435 and the pHi of the equilibrated cells corresponding to the broth pH (5.0 to 8.0), respectively. It was necessary to establish a different calibration curve for each strain because of the nature of the intracellular dye binding to molecules within the cell that could vary by strain. After the curve was established, polynomial fitting was used to establish an equation for each strain relating fluorescence values (492/435 nm) to pHi.
Mid log cells (cell density approximately 107 CFU/ml) were exposed to one of four treatments described in the experimental overview and as described by Li et al. (2021). Cells were incubated at 37°C with shaking in the microplate fluorometric reader for 60 min. Fluorescence values were measured at 492 and 435 nm, and the pHi was calculated from the mean value plotted against the pH calibration curve.
Results and Discussion
Accumulation of Acid Anions
Results from acid accumulation studies are presented in Figure 1. Lactate accumulated 1.5- and 1.7-fold in the pellets of Listeria N1-227 and R2-499, respectively, as compared to levels found in the supernatants. No significant accumulation of lactate was detected in the E. coli pellet. In comparison, the accumulation of acetate into the cell pellet was 2.3-, 2.4-, and 2.6-fold greater than in the supernatant for all L. monocytogenes N1-227, R2-499, and E. coli O157:H7, respectively. Overall, all three strains accumulated acetate anion into the cell pellet as compared to the supernatant to a greater extent than they accumulated lactic acid.
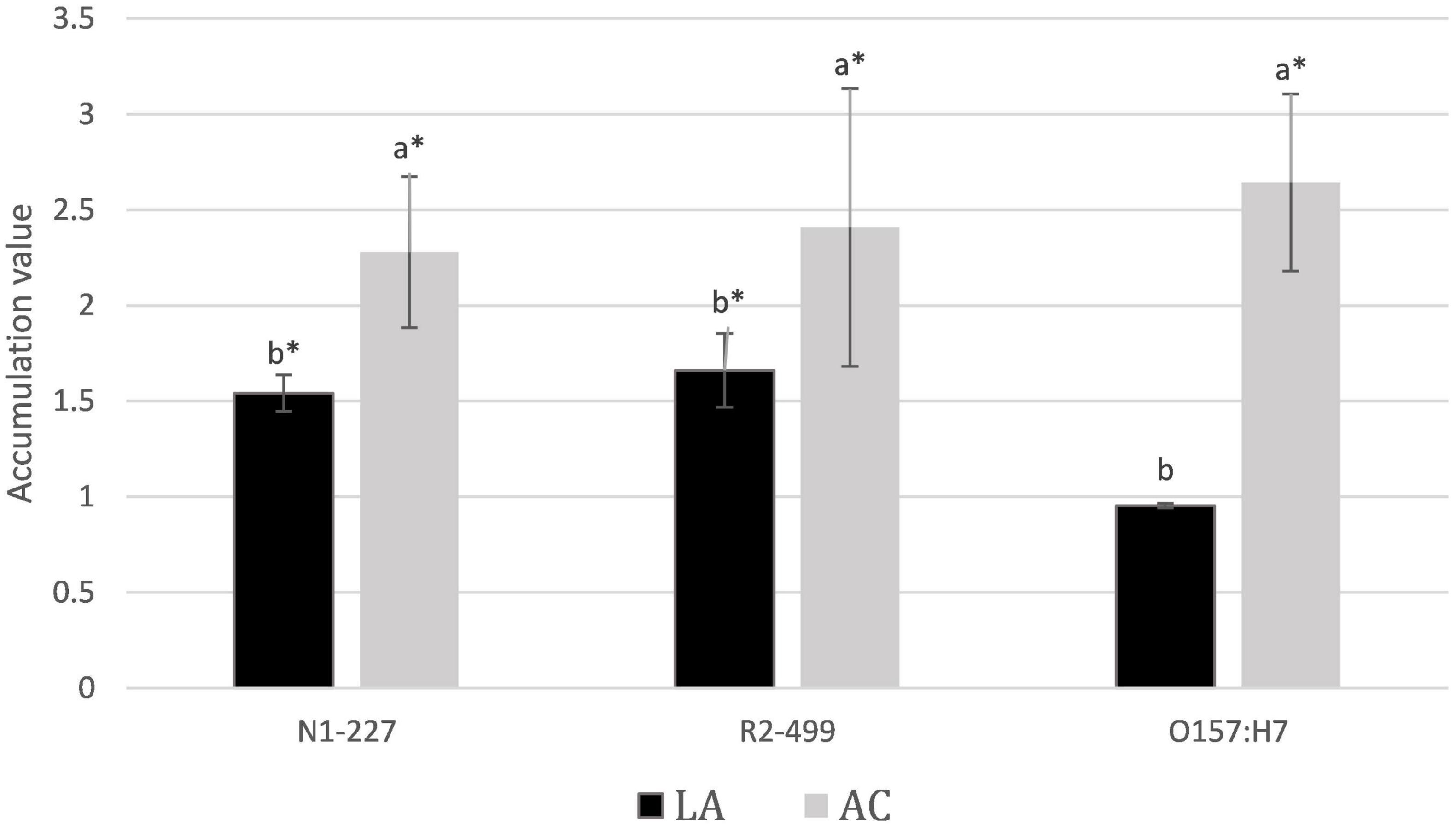
Figure 1. Average relative acid anion accumulation after 1 h. Relative acid anion accumulation into pellets of Listeria monocytogenes N1-227, L. monocytogenes R2-499, and Escherichia coli O157:H7 following 1 h in habituation media. Values represent treatment means and include bars of the standard error. Treatment details: LA, lactic acid treatment (TSB adjusted to pH 6.0 with HCl containing 4.75 mM lactic acid); AC, acetic acid treatment (TSB adjusted to pH 6.0 with HCl containing 4.75 mM acetic acid). Superscripts identify significant differences within strains (p < 0.05). Asterisk signifies that accumulation was significantly greater than 1 (CI < 95%).
Prior studies report that acetic acid inhibits growth of L. monocytogenes more than lactic acid in terms of total acid added as weight by volume (Farber et al., 1989; Sorrells et al., 1989; George et al., 1996), and the results presented here suggest that this may be due to the greater relative accumulation of acetate into the cell. Roe et al. (1998) reported that at pH 6, growth in 8 mM acetate resulted in an internal pool of 240 mM acetate anion in E. coli representing a 30-fold relative accumulation of acetate. Results here were much lower in comparison. Thus, C14 inulin was employed as a control to try and assess how much of the pellet volume was truly intracellular. The fractional volume of the pellet not available to inulin as compared to water was calculated as representative of the relative intracellular space. A fractional volume of 0.24 was identified for E. coli, suggesting that intracellular accumulation of anion is possibly fourfold greater than measured in the pellet. Both L. monocytogenes strains did not present a fractional volume significantly different from zero, suggesting that it was below the detection limit of our procedure. While the methodology employed here was able to identify significant accumulation of organic acids into the pellet, our results with inulin suggest that the pellet values underestimate intracellular concentrations of anion, and the true extent of intracellular anion accumulation is likely much higher than measured for the pellet.
One possible explanation for not finding a significant fractional internal volume in Listeria may be related to past observations that the OD600 for Listeria strains does not correlate well with actual viable cell numbers (Zhang et al., 2014). The reason for this discrepancy is unknown, but we hypothesize that it could be due to cell clumping. If this hypothesis is correct, clumping could result in co-precipitation of media components with the pellet (despite centrifugation through bromododecane) and thereby obscure measures specific to the cell itself. Another consideration is that Gram-negative bacteria like E. coli may experience greater carry-along due to their periplasm that serves as a multipurpose compartment separate from the cytoplasm (Miller and Salama, 2018) and possibly having a different pH than the cytoplasm (Lund et al., 2014).
Intracellular pH
Results from pHi studies are presented in Figure 2. Habituation to the acid control induced lowered pHi in R2-499 as compared to baseline, and the trend was in that direction for N1-277. Habituation to acetic acid resulted in a significantly lower (p < 0.05) pHi compared to exposure to the baseline control or to the acid control in both Listeria species. Additionally, the acid control and lactic acid were intermediate (though not necessarily significantly different) from the baseline control or acetic acid in both Listeria species. While the extent of the response was strain- and acid-dependent, it appears that both Listeria strains tolerate a lower pHi to combat the toxic impacts of either external acidity or the presence of organic acids. The ability to continue active metabolism at lower pHi is an important characteristic contributing to the capacity of some bacteria to resist the toxicity of organic acids (Russell, 1992; Diez-Gonzalez and Russell, 1997). It has not been previously reported that Listeria develops a lower pHi in response to just external acidity (Budde and Jakobsen, 2000; Shabala et al., 2002), although this finding has been reported in lactic acid bacteria (Siegumfeldt et al., 2000). Regarding acid type, both lactate and acetate are part of normal metabolism in L. monocytogenes (Kelly and Patchett, 1996; Wallace et al., 2017), and strains may have alternative methods and varying capacity to export lactate and acetate. It is likely that the cell only develops a lowered cell pHi once the rate of anion export becomes overwhelmed by passive uptake of acid, and acetic acid is significantly more lipophilic than lactic acid.
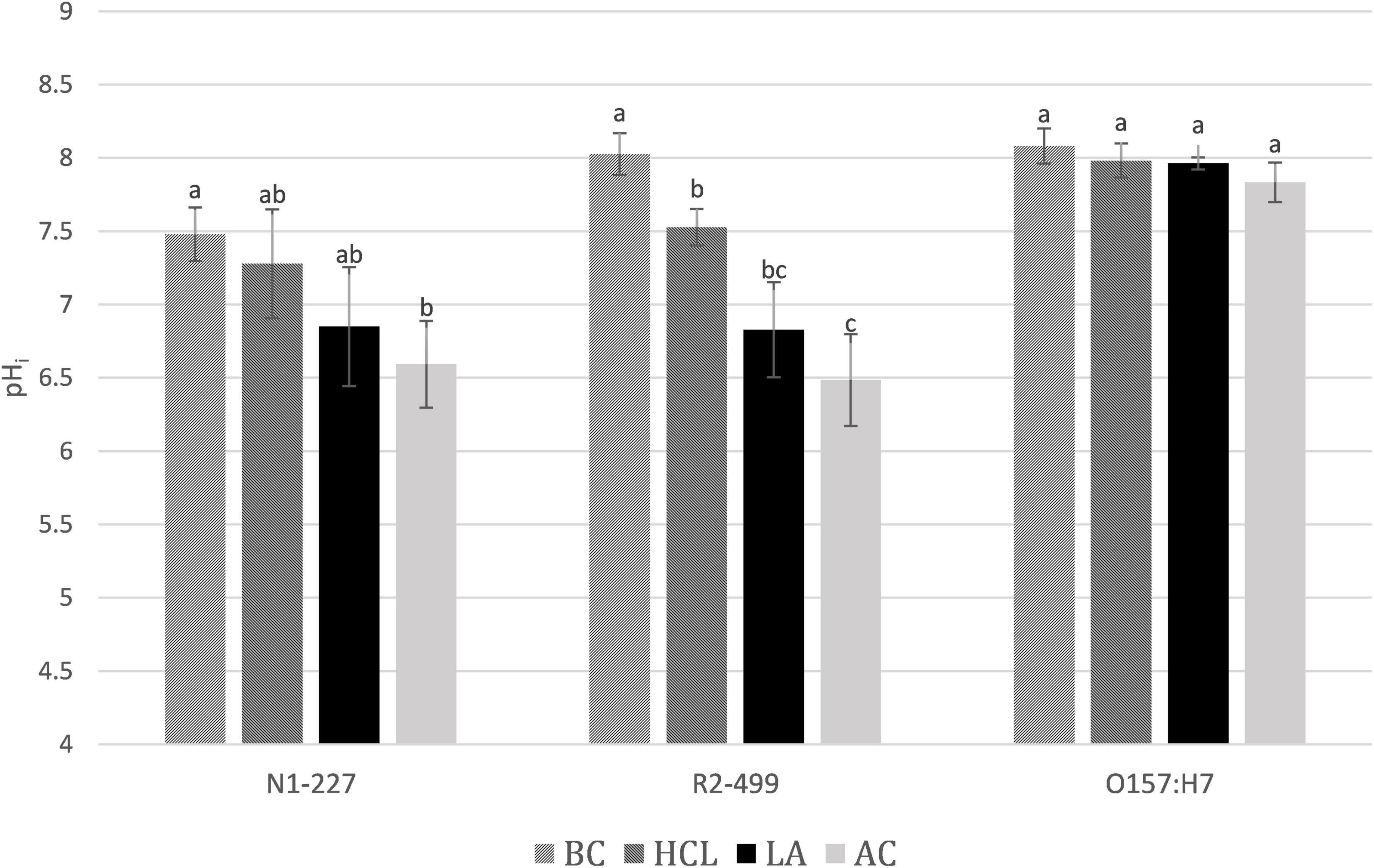
Figure 2. Average pHi after 1 h. pHi values of Listeria monocytogenes N1-227, L. monocytogenes R2-499, and Escherichia coli O157:H7 after 1 h in habituation treatment media. Error bars represent standard error of the mean. Treatment details: BC, baseline control (TSB pH 7.4); HCl, pH control (TSB adjusted to pH 6.0 with HCl); LA, lactic acid treatment (TSB adjusted to pH 6.0 with HCl containing 4.75 mM lactic acid); AC, acetic acid treatment (TSB adjusted to pH 6.0 with HCl containing 4.75 mM acetic acid). Superscripts identify significant differences within strains.
In contrast to what was observed for Listeria, pHi of E. coli did not significantly change in response to any of the four treatments. However, the pHi was measured after 60 min of habituation, which would miss the transient change in pHi reported by Martinez et al. (2012). The near-neutral pHi of E. coli predicts that acid anions will accumulate to a much greater extent than for the Listeria strains. Yet this research found that E. coli accumulated acetate at a level that was comparable to both Listeria strains and accumulated even less lactate than either Listeria strain. We speculate that E. coli adapts over 60 min to combat the accumulation of acid anions by mechanisms that are not available to the Listeria strains used in this work. For example, E. coli may change their membrane composition or upregulate export mechanisms (Kelly and Patchett, 1996; De Jonge et al., 2003).
Conclusion and Future Research
This study used two parallel experiments to investigate how L. monocytogenes and E. coli O157:H7 respond to organic acids in mildly acidic pH environments. The two Listeria strains have been studied previously in our laboratory under similar conditions, thereby building on a long history with these two strains, while E. coli was used as an outside “control” and has been studied by other researchers. It is recognized that the use of only two strains of L. monocytogenes does not establish any characteristic that should be viewed as universally shared among all strains. The use of two strains has, however, allowed for more in-depth study of various phenomena and cellular mechanisms of great potential impact for food safety.
It is also worthwhile to note that these results bring into question the risks of using intracellular anion accumulation as a measure of pHi as done in previous studies (Kroll and Booth, 1981; Roe et al., 1998). The methodology employed in these studies was based on independent measures of pHi and intracellular anion accumulation, and the results here bring into question the common, but confounding, practice of using intracellular anion accumulation as a measure of pHi, and vice versa. Prior studies employed the common approach of utilizing C14-labeled organic acid to follow intracellular anion accumulation and subsequently enter the measured intracellular anion concentration along with extracellular pH into the Henderson-Hasselbach equation to predict pHi (Kroll and Booth, 1981; Roe et al., 1998). Calculation of intracellular concentration of acid anion required drying of the cell pellet and calculation based on a previously established relationship between dry cell weight and internal cell volume. The risks of doing so include differences in how various species may handle acid exposure and accumulation of anions plus differences in physiology especially of Gram-positive vs. Gram negative bacteria that may interact with specific experimental protocols. This research instead used ratios of C14:H3 in the pellet and supernatant to determine the relative intracellular accumulation of acid anion in comparison to the external environment. Furthermore, a pH-sensitive fluorescent dye was employed as an independent means of measuring pHi.
Future research should track changes in intracellular anion accumulation and pHi over time and in response to various external pH to better understand their interaction. Future studies should additionally examine the role of other mechanisms in combating the intracellular accumulation of anions including anion-specific export systems or non-specific systems such as membrane changes to combat anion accumulation (De Jonge et al., 2003; Guan and Liu, 2020). Finally, our lab has previously studied transcriptomic data of the two Listeria strains exposed to these conditions, and it is being considered to reexamine that transcriptomic data in connection with these results. Results from this study bring to the forefront the potential of bacteria to survive and adapt to organic acid exposure through various mechanisms including lowering pHi to combat intracellular anion accumulation. While Listeria may lower pHi as a primary mechanism to combat anion accumulation, E. coli may, in the long term, largely rely on means other than lower pHi to combat the accumulation of anion. Additional research should be done at different external pH and external acid concentrations to truly understand the cellular response to the organic acids, and more strains will need to be studied to make broader conclusions.
Data Availability Statement
The original contributions presented in the study are included in the article/supplementary material, further inquiries can be directed to the corresponding author.
Author Contributions
CC and JB: conceptualization, data curation, funding, acquisition, project administration, supervision, validation, and writing—review and editing. SB: formal analysis, investigation, methodology, data collection, software, visualization, and writing—original draft. All authors contributed to the article and approved the submitted version.
Funding
This research was supported by the Utah Agricultural Experiment Station, Utah State University, and approved as journal manuscript #9533. Additional support was from the Western Dairy Center through the BUILD Dairy program at Utah State University.
Conflict of Interest
The authors declare that the research was conducted in the absence of any commercial or financial relationships that could be construed as a potential conflict of interest.
Publisher’s Note
All claims expressed in this article are solely those of the authors and do not necessarily represent those of their affiliated organizations, or those of the publisher, the editors and the reviewers. Any product that may be evaluated in this article, or claim that may be made by its manufacturer, is not guaranteed or endorsed by the publisher.
Acknowledgments
We thank Martin Wiedmann and the International Life Sciences Institute for supplying the L. monocytogenes strains used in this study as well as Don Schaffner at Rutgers University for supplying the E. coli strain used.
References
Berry, E. D., and Cutter, C. N. (2000). Effects of acid adaptation of Escherichia coli O157:H7 on efficacy of acetic acid spray washes to decontaminate beef carcass tissue. Appl. Environ. Microbiol. 66, 1493–1498. doi: 10.1128/AEM.66.4.1493-1498.2000
Buchanan, R. L., Golden, M. H., Whiting, R. C., Phillips, J. G., and Smith, J. L. (1994). Non-thermal inactivation models for Listeria monocytogenes. J. Food Sci. 59, 179–188. doi: 10.1111/j.1365-2621.1994.tb06928.x
Budde, B. B., and Jakobsen, M. (2000). Real-time measurements of the interaction between single cells of Listeria monocytogenes and nisin on a solid surface. Appl. Environ. Microbiology 66, 3586–3591. doi: 10.1128/AEM.66.8.3586-3591.2000
Carpenter, C. E., and Broadbent, J. R. (2009). External concentration of organic acid anions and pH: key independent variables for studying how organic acids inhibit growth of bacteria in mildly acidic foods. J. Food Sci. 74, R12–R15. doi: 10.1111/j.1750-3841.2008.00994.x
Cheng, C., Yang, Y., Dong, Z., Wang, X., Fang, C., Yang, M., et al. (2015). Listeria monocytogenes varies among strains to maintain intracellular pH homeostasis under stresses by different acids as analyzed by a high-throughput microplate-based fluorometry. Front. Microbiol. 6:15. doi: 10.3389/fmicb.2015.00015
De Jonge, R., Takumi, K., Ritmeester, W. S., and van Leusden, F. M. (2003). The adaptive response of Escherichia coli O157 in an environment with changing pH. J. Appl. Microbiol. 94, 555–560. doi: 10.1046/j.1365-2672.2003.01865.x
Diez-Gonzalez, F., and Russell, J. B. (1997). The ability of Escherichia coli O157:H7 to decrease its intracellular pH and resist the toxicity of acetic acid. Microbiol. (Read. Engl.) 143(Pt 4), 1175–1180. doi: 10.1099/00221287-143-4-1175
Dziezak, J. D. (2016). Acids: natural acids and acidulants. Encycl. Food Health 16, 15–18. doi: 10.1016/B978-0-12-384947-2.00004-0
Farber, J., Sanders, G., Dunfield, S., and Prescott, R. (1989). The effect of various acidulants on the growth of Listeria monocytogenes. Lett. Appl. Microbiol. 9, 181–183. doi: 10.1111/j.1472-765X.1989.tb00319.x
George, S. M., Richardson, L. C., and Peck, M. W. (1996). Predictive models of the effect of temperature, pH and acetic and lactic acids on the growth of Listeria monocytogenes. Int. J. Food Microbiol. 32, 73–90. doi: 10.1016/0168-1605(96)01108-7
Guan, N., and Liu, L. (2020). Microbial response to acid stress: mechanisms and applications. Appl. Microbiol. Biotechnol. 104, 51–65. doi: 10.1007/s00253-019-10226-1
Jensen, D. A., Friedrich, L. M., Harris, L. J., Danyluk, M. D., and Schaffner, D. W. (2015). Cross contamination of Escherichia coli O157:H7 between lettuce and wash water during home-scale washing. Food Microbiol. 46, 428–433. doi: 10.1016/j.fm.2014.08.025
Kelly, A. F., and Patchett, R. A. (1996). Lactate and acetate production in Listeria innocua. Lett. Appl. Microbiol. 23, 125–128. doi: 10.1111/j.1472-765x.1996.tb00046.x
Kroll, R. G., and Booth, I. R. (1981). The role of potassium transport in the generation of a pH gradient in Escherichia coli. Biochem. J. 198, 691–698. doi: 10.1042/bj1980691
LeJeune, J. T., Besser, T. E., and Hancock, D. D. (2001). Cattle water troughs as reservoirs of Escherichia coli O157. Appl. Environ. Microbiol. 67, 3053–3057. doi: 10.1128/AEM.67.7.3053-3057.2001
Li, M., Carpenter, C. E., and Broadbent, J. R. (2021). Organic acid exposure enhances virulence in some Listeria monocytogenes strains using the Galleria mellonella infection model. Front. Microbiol. 12:675241. doi: 10.3389/fmicb.2021.675241
Lund, P., Tramonti, A., and De Biase, D. (2014). Coping with low pH: molecular strategies in neutralophilic bacteria. FEMS Microbiol. Rev. 38, 1091–1125. doi: 10.1111/1574-6976.12076
Mani-López, E., Hugo, G., and Aurelio, L.-M. (2012). Organic acids as antimicrobial to control Salmonella in meat and poultry products. Food Res. Int. 45, 713–721. doi: 10.1016/j.foodres.2011.04.043
Martinez, K. A., Kitko, R. D., Mershon, J., Adcox, H. E., Malek, K., Berkmen, M. B., et al. (2012). Cytoplasmic pH response to acid stress in individual cells of Escherichia coli and Bacillus subtilis observed by fluorescence ratio imaging microscopy. Appl. Environ. Microbiol. 78, 3706–3714. doi: 10.1128/AEM.00354-12
Mead, P. S., Slutsker, L., Dietz, V., McCaig, L. F., Bresee, J. S., Shapiro, C., et al. (1999). Food-related illness and death in the United States. Emerg. Infect. Dis. 5, 607–625. doi: 10.3201/eid0505.990502
Miller, S. I., and Salama, N. R. (2018). The gram-negative bacterial periplasm: Size matters. PLoS Biol. 16:e2004935. doi: 10.1371/journal.pbio.2004935
Pieterse, B., Leer, R. J., Schuren, F., and van der Werf, M. J. (2005). Unravelling the multiple effects of lactic acid stress on Lactobacillus plantarum by transcription profiling. Microbiol. (Read. Engl.) 151(Pt 12), 3881–3894. doi: 10.1099/mic.0.28304-0
Popovic, I., Heron, B., and Covacin, C. (2014). Listeria: an Australian perspective (2001-2010). Foodb. Path. Dis. 11, 425–432. doi: 10.1089/fpd.2013.1697
Roe, A. J., McLaggan, D., Davidson, I., O’Byrne, C., and Booth, I. R. (1998). Perturbation of anion balance during inhibition of growth of Escherichia coli by weak acids. J. Bacteriol. 180, 767–772. doi: 10.1128/JB.180.4.767-772.1998
Romick, T. L., and Fleming, H. P. (1998). Acetoin production as an indicator of growth and metabolic inhibition of Listeria monocytogenes. J. Appl. Microbiol. 84, 18–24. doi: 10.1046/j.1365-2672.1997.00302.x
Russell, J. (1992). Another explanation for the toxicity of fermentation acids at low pH: anion accumulation versus uncoupling. J. Appl. Microbiol. 73, 363–370. doi: 10.1111/j.1365-2672.1992.tb04990.x
Scallan, E., Hoekstra, R. M., Angulo, F. J., Tauxe, R. V., Widdowson, M. A., Roy, S. L., et al. (2011). Foodborne illness acquired in the United States–major pathogens. Emerg. Infect. Dis. 17, 7–15. doi: 10.3201/eid1701.p11101
Shabala, L., Budde, B., Ross, T., Siegumfeldt, H., Jakobsen, M., and McMeekin, T. (2002). Responses of Listeria monocytogenes to acid stress and glucose availability revealed by a novel combination of fluorescence microscopy and microelectrode ion-selective techniques. Appl. Environ. Microbiol. 68, 1794–1802. doi: 10.1128/AEM.68.4.1794-1802.2002
Siegumfeldt, H., Rechinger, K. B., and Jakobsen, M. (1999). Use of fluorescence ratio imaging for intracellular pH determination of individual bacterial cells in mixed cultures. Microbiology. 145, 1703–1709. doi: 10.1099/13500872-145-7-1703
Siegumfeldt, H., Björn Rechinger, K., and Jakobsen, M. (2000). Dynamic changes of intracellular pH in individual lactic acid bacterium cells in response to a rapid drop in extracellular pH. Appl. Environ. Microbiol. 66, 2330–2335. doi: 10.1128/AEM.66.6.2330-2335.2000
Sorrells, K. M., Enigl, D. C., and Hatfield, J. R. (1989). Effect of pH, acidulant, time, and temperature on the growth and survival of Listeria monocytogenes. J. Food Protect. 52, 571–573. doi: 10.4315/0362-028X-52.8.571
Wallace, N., Newton, E., Abrams, E., Zani, A., and Sun, Y. (2017). Metabolic determinants in Listeria monocytogenes anaerobic listeriolysin O production. Arch. Microbiol. 199, 827–837. doi: 10.1007/s00203-017-1355-4
Zhang, Y., Carpenter, C. E., Broadbent, J. R., and Luo, X. (2014). Habituation to organic acid anions induces resistance to acid and bile in Listeria monocytogenes. Meat Sci. 96, 1152–1157. doi: 10.1016/j.meatsci.2013.10.034
Keywords: organic acid, Listeria monocytogenes, Escherichia coli, anion accumulation, intracellular pH
Citation: Branson SR, Broadbent JR and Carpenter CE (2022) Internal pH and Acid Anion Accumulation in Listeria monocytogenes and Escherichia coli Exposed to Lactic or Acetic Acids at Mildly Acidic pH. Front. Microbiol. 12:803271. doi: 10.3389/fmicb.2021.803271
Received: 27 October 2021; Accepted: 20 December 2021;
Published: 24 February 2022.
Edited by:
Michael Gänzle, University of Alberta, CanadaReviewed by:
Francisco Diez-Gonzalez, University of Georgia, United StatesArunachalam Muthaiyan, University of New Mexico Gallup, United States
Copyright © 2022 Branson, Broadbent and Carpenter. This is an open-access article distributed under the terms of the Creative Commons Attribution License (CC BY). The use, distribution or reproduction in other forums is permitted, provided the original author(s) and the copyright owner(s) are credited and that the original publication in this journal is cited, in accordance with accepted academic practice. No use, distribution or reproduction is permitted which does not comply with these terms.
*Correspondence: Charles E. Carpenter, chuck.carpenter@usu.edu