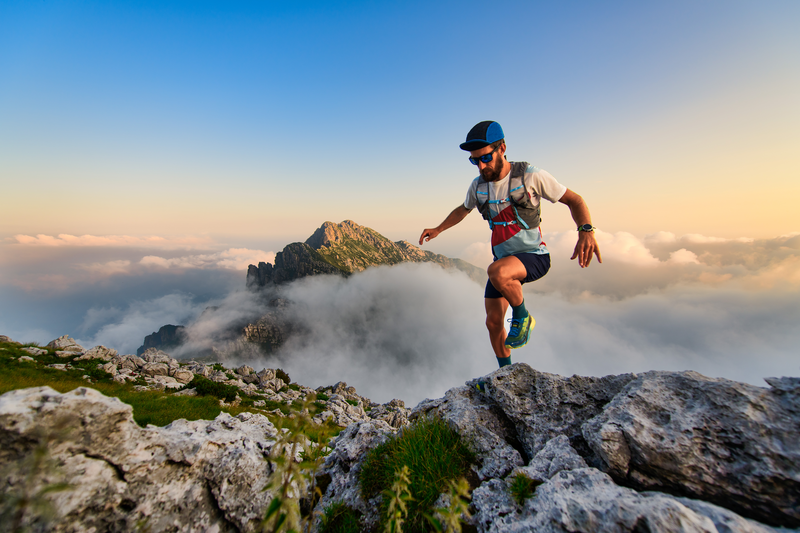
94% of researchers rate our articles as excellent or good
Learn more about the work of our research integrity team to safeguard the quality of each article we publish.
Find out more
ORIGINAL RESEARCH article
Front. Microbiol. , 18 February 2022
Sec. Microbial Symbioses
Volume 12 - 2021 | https://doi.org/10.3389/fmicb.2021.802035
This article is part of the Research Topic Advanced Microbial Biotechnologies For Sustainable Agriculture, Volume II View all 18 articles
The secretory insecticidal protein Sip1Ab and crystal protein Cry8Ca from Bacillus thuringiensis (Bt) are widely recognized for their coleopteran insecticidal activities. It is worthwhile to investigate the insecticidal mechanisms of these two proteins against Colaphellus bowringi Baly, which is a serious pest of cruciferous vegetables in China and other Asian countries. To that end, the genes encoding the Sip1Ab and Cry8Ca proteins were amplified from the strain QZL38 genome, then expressed in Escherichia coli, after which bioassays were conducted in C. bowringi larvae. After feeding these two proteins, the histopathological changes in the midguts of C. bowringi larvae were observed using transmission electron microscopy (TEM), and the Brush Border Membrane Vesicle (BBMV) was extracted for competition binding assays. TEM showed that ingestion of Sip1Ab caused a significant reduction in growth of the larvae, disruption of midgut microvilli, and expansion of intercellular spaces. Competition binding assays demonstrated that Sip1Ab bound to C. bowringi BBMV with a high binding affinity. However, a mixture of the two proteins in equal proportions showed no significant difference in insecticidal activity from that of Sip1Ab. These results could provide a molecular basis for the application of Sip1Ab in coleopteran insect control and contribute to the study of the Sip1Ab insecticidal mechanism as well.
The cabbage beetle Colaphellus bowringi Baly (Coleoptera: Chrysomelidae) is a serious insect pest of crucifers in mountainous areas of China (Xue et al., 2002). Moreover, the application of chemical pesticides does not always effectively manage it (Gao et al., 2011; Shu et al., 2013).
Bacillus thuringiensis (Bt) is a Gram-positive bacterium belonging to the Bacillus cereus family. It can produce insecticidal proteins harmless to humans and the environment during growth and metabolism (Gómez et al., 2020). According to the protein location and secretion mode, these proteins are characterized as δ-endotoxins or exotoxins (Singh et al., 2021).
The δ-endotoxin is a protein with crystal structure encoded by the crystal (cry) and cytolitic (cyt) genes (Swiecicka et al., 2008). Current research shows that many cry genes have insecticidal activity against coleopteran pests, including cry3, cry7, cry8, cry18, cry23, cry37, and cry43. The cry8 genes are currently the most studied, since they have specific insecticidal activity against many coleopteran insects, for example, the beetle family, the leaf beetle family, the weevil family, and so on (Jiang et al., 2017). In 1994, Beard et al. cloned a new insecticidal gene named cry8ca1 from a B. thuringiensis strain with high toxicity to coleopteran beetles for the first time (Beard et al., 2001). On the basis of the established PCR-RFLP identification system of cry8 genes, Liu and co-workers have cloned a novel cry8C gene from strain HBF-1, designated cry8ca2 (AY518201) (Liu et al., 2004). Wu’s Laboratory has established a purification method for the Cry8Ca2 active toxin, and bioassay results have suggested that the insecticidal protein had good activity against the larvae of Anomala corpulenta and Anomala exoleta Fald (Wu et al., 2007). To date, a series of Cry8 proteins which contain about 1,200 amino acids, with the molecular weight of approximately 130 kDa, have been identified to have the high insecticidal activity to coleopteran pests (Noguera and Ibarra, 2010).
Exotoxin is a metabolite which is secreted outside the cell during metabolism of Bt, such as Vegetative insecticidal protein (Vip) and Secreted insecticidal protein (Sip) (Palma et al., 2014). It is now well established from a number of studies that Sip has the insecticidal activity against coleopteran larvae. Donovan et al. (2006) reported that Bt strain EG2158 secreted the coleopteran active protein Sip1Aa, which had insecticidal activity against larvae of the potato beetle (Leptinotarsa decemlineata), the southern corn rootworm (Diabrotica undecimpunctata howardi), and the western corn rootworm (Diabrotica virgifera virgifera). The gene encoding Sip1Aa, which has been reported as the first member of Bt secreted proteins having activity against coleopteran larvae, was 1,104 bp in length and encoded a polypeptide of 367 amino acids (Donovan et al., 2006). In 2012, Liu and co-workers successfully cloned and identified another sip gene from strain QZL26 encoding a 39 kDa protein, which shared a 91.83% identity with Sip1Aa (Liu et al., 2012). In 2015, our laboratory identified a sip gene from strain DQ89, which was 1,095 bp and encoded a protein of 364 amino acids with a calculated mass of 42 kDa (Zhang et al., 2015). The protein expressed by this gene showed high toxicity to C. bowringi with a lethal concentration 50 (LC50) of 1.542 μg/ml (Zhang et al., 2015). Sha et al. (2018) showed that Bt strain QZL38 contained a new sip gene designated as sip1Ab from 540 soil samples, which contained an open reading frame of 1,095 bp encoding a 364 amino acid polypeptide. The results of bioassays of four truncated mutant proteins indicated that the truncated protein SipA, from which the signal peptide (the first 30 amino acids) was removed, showed insecticidal activity against C. bowringi, with an LC50 of 1.078 μg/ml and showed no significant differences compared with Sip (Sha et al., 2018).
In previous studies, many Bt insecticidal proteins demonstrated synergistic or antagonistic effects (Lemes et al., 2017). Some studies have found that when Cry1Aa and Cry1Ac were mixed in various proportions, their insecticidal activities against Lymantria dispar varied. The insecticidal activity was increased by 3.8 times when the proteins were mixed in a proportion of 1:1, and it increased by 7.3 times when mixed in proportion of 1:2. However, a mixture of Cry1Aa and Cry1Ab showed antagonism (Xue et al., 2005). Moreover, a combination of Cry6Aa and Cry55Aa had significant synergistic toxicity to Meloidogyne incognita, and the insecticidal activity of these two proteins was increased five times when they were combined in a ratio of 1:1 (Peng et al., 2011). Additionally, in a study of the toxicity of Cry1 and Vip3A to Diatraea saccharalis, the proteins did not interact, but Cry1Ab and Cry1Fa had synergistic effects with Cry1Ca (Pavani, 2013).
Unlike Sip, the insecticidal mechanisms of the Cry and Vip toxins have been studied extensively. A study of the insecticidal mechanism of Cry toxins found that Cry was hydrolyzed and activated by a protease in the midgut of the target insect. The intestinal epithelium cell membrane was destroyed after the Cry toxins bound to a specific receptor, which led to the death of the insect pests (Deist et al., 2014). For the Vips, it has been found that Vip3Aa could bind to the intestinal cells of insects, then become activated by the alkaline midgut fluid, leading to cell fragmentation via binding to specific proteins in the midgut cells (Zhang et al., 2018). However, Wang et al. (2020) have indicated that the receptor for Sip might be located in the midgut of the C. bowringi larvae.
In previous research in our laboratory, it was found that strain QZL38 contained both the cry8Ca and sip1Ab genes (unpublished data), both of which may have synergistic or antagonistic effects. Given that, the sip1Ab and cry8Ca genes were cloned using the genome of QZL38 as a template, and the corresponding proteins were induced and expressed. By adding a mixture of the proteins Sip and Cry, changes in the midgut of C. bowringi larvae were observed under the electron microscope and compared with the group in which Sip alone was added. Competitive binding assays were carried out for further verification. One of the purposes of this investigation was the exploration of the relationship between Sip1Ab and the midgut of C. bowringi larvae; another was to verify whether Cry8Ca had a synergistic effect with Sip. This study indicates new directions for further research of protein interactions, and new resources for the prevention and control of coleopteran pests. It is hoped that this research will contribute to a deeper understanding of the insecticidal mechanism of the Sip toxin.
The genomic DNA of strain QZL38 was extracted by using the EasyPure® Bacteria Genomic DNA Kit (TransGen Biotech, Beijing, China) according to the manufacturer’s protocol and quantified using a Nano Photometer P300 (Implen, Inc., Munich, Germany).
The sip1Ab (KP231523.1) and cry8Ca (GU270856.1) genes were amplified by polymerase chain reaction (PCR) from the QZL38 genomic DNA sample. KOD -Plus- DNA Polymerase (Toyobo Co., Ltd., Osaka, Japan) was used for all PCR reactions. The reaction system consisted of 1× -KOD- Plus buffer, 0.2 mM of dNTPs, 1 μM of MgSO4, 0.3 μM of primer (for each), 200 ng of genomic DNA, and 1 U -KOD- Plus polymerase with a supplement of ddH2O up to a total volume of 50 μl. Amplification was performed for 35 cycles after pre-denaturation at 95°C for 2 min. Cycling conditions were set to denaturation at 95°C for 15 s, annealing at 59°C for 30 s, and extension at 67°C for 90 s. The amplification products were analyzed by electrophoresis on a 1% (w/v) agarose gel and purified using an Axygen® DNA Gel Extraction Kit (Axygen Biosciences, Union City, CA, United States) following the manufacturer’s instructions. The DNA sequences of the oligonucleotides used in this study are listed in Table 1.
Restriction digestion was performed on pET21b at Xho I and Sal I sites to obtain a linearized plasmid. The insert fragment and the linearized pET21b were blunted by using a ClonExpress™ II One Step Cloning Kit (Vazyme, Nanjing, China). The recombinant plasmids were then transformed into Escherichia coli DH5α using the heat-shock method, and the recombinant strains were incubated on solid LB medium (containing ampicillin) at 37°C for 12–16 h. Verification of successfully constructed plasmids was performed via colony PCR and sequencing.
The recombinant plasmids were extracted using an Axygen® Plasmid Miniprep Kit. A second transformation was carried out in E. coli BL21 (DE3) using the same method as above. Plasmid pET21b without an inserted fragment was used as a control in downstream experiments. The obtained transformants were inoculated into 5 ml of LB medium supplemented with ampicillin and pre-cultured overnight at 37°C, shaking at 220 rpm. These liquid cultures were then inoculated with 1% (v/v) into a 500 ml flask containing 100 ml of LB medium supplemented with ampicillin and incubated at 37°C, 220 rpm to an optical density at 600 nm (OD600) of 0.6. For inducing expression, isopropyl-β-D-thiogalactopyranoside (IPTG) was added into the cultures to a final concentration of 0.5 mM. After induction for 16 h at 16°C, 160 rpm, cells were harvested by centrifugation at 8,000 × g for 5 min at 4°C and washed at least twice with 10 mM phosphate buffered saline (PBS, pH 7.4). The cells were disrupted by ultrasonic treatment in an ice-water mixture for 3 s pulses for 10 min with 5 s intervals. Debris and unbroken cells were removed by centrifuging twice at 12,000 × g for 15 min at 4°C. The supernatant was filtered through a 0.22 μm filter and purified using a Ni-NTA Fast Start Kit (Qiagen, Hilden, Germany) according to the instructions in the manual.
Samples of the purified products were mixed with 2× SDS loading buffer [100 mM Tris–HCl pH 6.8, 200 mM β-mercaptoethanol, 4% (w/v) SDS, 2% (w/v) bromophenol blue, and 20% (v/v) Glycerol], immersed in a boiling water bath for 10 min, and centrifuged at 10,000 × g for 10 min. The supernatant was collected for protein separation using SDS-PAGE and visualized with a Coomassie blue stain. The protein concentration was determined using bovine serum albumin (BSA) as a standard following the user manual of the BSA Protein Assay Kit (TransGen Biotech, Beijing, China).
The C. bowringi standard used in this study was donated by the Institute of Plant Protection (IPP), Chinese Academy of Agricultural Sciences (CAAS). C. bowringi insect eggs were incubated in a biochemical incubator at 25°C for 4–5 days. Fresh rape leaves were picked, washed, and placed on filter paper. The initially hatched C. bowringi larvae were gently inserted with a brush, and 5–6 larvae were placed in each Petri dish, which was placed in a biochemical incubator at 25°C (Liu et al., 2014). An appropriate amount of sterilized water was sprayed in the biochemical incubator in the morning and evening to maintain the air humidity. The leaves and filter paper in the Petri dish were changed every 24 h in the initial stage (6 days before incubation); the new filter paper was wetted, and it was ensured that the filter paper and rape leaves had no water droplets. After the initial stage, the Petri dish and the dead larvae were cleaned every day and the leaves and filter paper in the Petri dish were replaced every 12 h. The insect bodies were immersed in a pre-cooled saline solution. Sip1Ab, Cry8Ca, and a mixture of Sip1Ab and Cry8Ca in equal proportions were separately solubilized in 20 mM phosphate buffer before use in the bioassays. An analysis of the toxicity to C. bowringi was conducted on second instar larvae with fresh rape using the leaf-dip bioassay (Omer et al., 1993). The trays were incubated at 25 ± 2°C, 70 ± 5% RH, and a 10/8 h light/dark cycle, and mortality was scored at the second day. The quantitative bioassays were replicated at least three times using different concentrations of Sip1Ab, Cry8Ca, and a mixture of Sip1Ab and Cry8Ca. An insecticidal protein solution of PBS buffer was used as the control. The pET21b plasmid was used as the negative control. The LC50 value was measured with SPSS software. It is generally believed that the toxicity ratio of LC50 measured is expected to be 0.5–2.6. A value higher than 1.5 was considered synergistic, and less than 0.5 as antagonistic.
After starvation for 48 h, the C. bowringi larvae were fed the corresponding sensitive Bt proteins. Fresh and identical rapeseed leaves were selected. Protein samples were prepared and added with 0.1% detergent evenly smeared on both sides of the leaves with sterilized brushes. The leaves coated with protein samples were dried on the fresh-keeping film, and PBS buffer was applied as control. The concentration of Sip1Ab was 0.5 μg/ml. The sublethal worms and controls were dissected at 1, 2, and 3 days under microscopy. The midgut was immersed in PBS and diluted into 2.5% glutaraldehyde and fixed overnight at room temperature. The obtained midguts were prepared according to the method of transmission electron microscopy (TEM) sample preparation of Sinha et al. (2021). Uranium acetate peroxide dyeing was conducted for 10 min, lead acetate dyeing for 10 min, and a JEM-1230 (Joel, Tokyo, Japan) was used for electron microscopic observation.
To obtain the C. bowringi Brush Border Membrane Vesicles (BBMVs), the insect midguts were poured into a pre-cooled conical flask, then 30 ml Buffer A (300 mmol/L mannitol, 5 mmol/L EGTA, 17 mmol/L Tris, and pH 7.5) was added. The mixture was subsequently ground on ice and placed on ice for 15 min after 30 ml MgCl2 was added to the conical flask. Centrifugation (4,000 rpm) was conducted for 15 min. The supernatant was poured into a conical flask, and 15 ml MgCl2 was added. The supernatant was placed on ice for 15 min and centrifuged at 4,000 rpm for 15 min. The supernatant was decanted into 50 ml centrifuge tube and centrifuged at 10,000 rpm for 30 min. The precipitate was dissolved in 1 ml Buffer B (150 mmol/L NaCl, 5 mmol/L EGTA, 20 mmol/L Tris, 1% CHAPS, and pH7.5). The total BBMV protein was quantified with a Bradford Protein Assay Kit (Merck, NJ, United States).
Proteins were biotinylated using the Pierce™ Cell Surface Protein Biotinylation and Isolation Kit (Thermo Fisher, MA, United States). Various concentrations of biotinylated Sip (NHS-Biotin-Sip) were added to BBMV in a range 25–100 nM at intervals of 25 nM. Additionally, 100 nM biotinylated Sip was separately added with a 10-, 15-, and 20-fold excess of unmarked Cry8Ca into the BBMV binding system. For the competitive binding assays, 0.1% BSA in PBS was added to all binding systems to make them up to 100 μl. All the systems were incubated for 1 h at room temperature. Unbound toxin was removed by centrifugation (10 min at 18,000 rpm), and the pellets were washed twice with PBS (pH 7.6, 0.1% BSA). The precipitate was suspended in 10 μl 1× SDS loading buffer, loaded on an SDS-PAGE gel, and electrotransferred to a PVDF membrane (GE Healthcare, MA, United States). The PVDF membrane was then incubated with Streptavidin-HRP (1:3000 dilutions) for 1 h at room temperature, followed by three wash cycles with PBST, 15 min per cycle. Binding was visualized using an ECL chemiluminescent kit (Thermo Scientific, MA, Untied States).
The designed full-length primers sipF/sipR and cry8CaF/cry8CaR were used to amplify the sip1Ab and cry8Ca by PCR. The amplified fragments of a size of 1,044 and 3,522 bp were consistent with the expected fragment size. The agarose electrophoresis results are shown in the figure below (Figure 1).
Figure 1. Verification of the sip1Ab and cry8Ca by PCR. (A) M: DL2000 DNA Marker, 1 and 2: the amplified sip1Ab from QZL38 genome with sipF and sipR primers. (B) M: DL5000 DNA Marker, 1 and 2: the amplified cry8Ca from QZL38 genome with cry8CaF and cry8CaR primers.
Expression from recombinant plasmids sip1Ab-pET21b and cry8Ca-pET21b was induced by IPTG. With SDS-PAGE, the expressed products were shown to include a recombinant protein at approximately 37 kDa (Figure 2A) and 130 kDa (Figure 2C), indicating that both proteins were successfully expressed in E. coli BL21 (DE3). Subsequently, the His-tagged recombinant proteins were purified from the crude extract using Ni-NTA agarose affinity chromatography (GE Healthcare, MA, United States) (Figures 2B,D).
Figure 2. Expression and purification of Sip1Ab and Cry8Ca. LM: Protein Molecular Weight Marker (Low) (Takara Biomedical Technology Co., Ltd., Beijing, China), HM: Protein Molecular Weight Marker (High) (Takara Biomedical Technology Co., Ltd., Beijing, China), CK: whole protein of pET21b-BL21 (DE3). Samples were subjected to SDS-PAGE followed by Coomassie Blue staining. (A) Expression of soluble Sip1Ab in E. coli. (B) The purified Sip1Ab. (C) Expression of soluble Cry8Ca in E. coli. (D) The purified Cry8Ca.
The expression from recombinant strains was induced by IPTG. In the qualitative bioassays for the insecticidal activity of the proteins, second-instar C. bowringi were used as the test insects, and in that of the protein expressed in E. coli, pET21b was used as the negative control. The protein concentrations for bioassay were 0.5, 5, and 20 mg/ml. Forty-eight insects were tested for each protein, and the death of the insects was assessed 48 h later. The experiment was repeated three times. The test results are shown in Figure 3.
The insecticidal activity of Cry8Ca and Sip1Ab is shown in Table 2. The results showed that the insecticidal activity of a mixture of Cry8Ca and Sip1Ab (1:1) showed no significant difference from that of Sip1Ab.
Figure 4A shows that the midgut microvilli of healthy C. bowringi were dense, slender, and well-distributed and the membrane structure was normal. One day after treatment with Sip1Ab, the microvilli swelled and shed slightly and the structure was sparse (Figure 4C). After treatment with Sip1Ab for 2 days, a number of microvilli were exfoliated, and many vesicle structures were formed (Figure 4D). After 3 days of treatment with Sip, the microvilli were almost shed and disordered (Figure 4E). No significant change was seen in the group treated with Cry8Ca (Figure 4B).
Figure 4. Pathological effects of Sip1Ab and Cry8Ca on midgut microvilli of larvae of Colaphellus bowringi (A) Control group fed PBS for 3 days, (B) Cry8Ca treatment for 3 days, and (C–E): 1, 2, and 3 days after treatment with Sip1Ab, respectively.
As can be seen in Figure 5, the gap between the two cells in the midgut of the healthy C. bowringi was very small, with sporadic vesicles (Figure 5A). After 3 days of treatment, the gap between the two cells in the midgut appeared to be unaffected by Cry8Ca (Figure 5B). After 1 day of treatment with Sip1Ab, the gap between the two cells in the midgut increased significantly, and the slight packing structure increased (Figure 5C). During 2–3 days of treatment with Sip1Ab, not only did the gap between the two cells in the midgut increase significantly, but also the break-up and separation of cell membranes appeared (Figures 5D,E).
Figure 5. Pathological effects of the Sip1Ab and Cry8Ca on midgut space of larvae of Colaphellus bowringi (A) Control group fed PBS for 3 days, (B) Cry8Ca treatment for 3 days, and (C–E): 1, 2, and 3 days after treatment with Sip1Ab, respectively.
About 5 g of midgut tissue was extracted from around 1,500 C. bowringi fifth instar larvae. BBMV was extracted from the C. bowringi midgut (Figure 6). The protein concentration was determined by the Bradford method.
Figure 6. Proteins in C. bowringi BBMV were separated by SDS-PAGE. HM: Protein Molecular Weight Marker (High).
In the binding test, the binding of biotinylated Sip1Ab to C. bowringi BBMV was tested in vitro. The ligand-blotting analysis demonstrated the binding of biotin labeled toxin (Sip1Ab) to protein in the C. bowringi BBMV (Figure 7). The binding activity of Sip1Ab could be clearly observed at a concentration of 100 nM.
Figure 7. Determination of saturated binding between biotinylated Sip toxin and C. bowringi BBMV. 1–4: 25, 50, 75, and 100 nM biotinylated Sip, respectively.
To determine whether Cry8Ca exhibits competition during interactions between Sip1Ab and C. bowringi BBMV, a mixture of these two proteins was added to the BBMV binding system. As shown in Figure 8, excess Cry8Ca up to 20× was unable to compete with the binding of Sip1Ab to C. bowringi BBMV, suggesting that this could be a unique binding protein for Sip1Ab toxin.
Figure 8. Competitive determination of Cry8Ca between biotinylated Sip binding in C. bowringi BBMV. 1: 100 nM biotinylated Sip, 2–4: Biotinylated Sip + Cry8Ca in 10-, 15-, and 20-fold excess, respectively.
Colaphellus bowringi is a common agricultural pest which occurs in most parts of China, and mainly damages cruciferous vegetables such as the Brassica rapa Chinensis Group, Raphanus sativus, Brassica oleracea var. botrytis, B. rapa var. botrytis, and so on (Zhu et al., 2019). A series of studies has shown that Sip has high insecticidal activity against coleopteran pests such as L. decemlineata and C. bowringi (Donovan et al., 2006; Sha et al., 2018; Wang et al., 2020). This observation provides a foundation for the discovery of novel genes of Bt and for expanding its insecticidal spectrum. However, to date, sip has only been studied with mutants and truncated genes. Histopathological changes in the midgut due to poisoning by Sip toxin, and studies on the heterologous competition between Sip1Ab and another Bt toxin, have not yet been reported. So, TEM was used to observe the midgut tissue. Additionally, corresponding competition assays were carried out to gain more insight into the insecticidal mechanism of Sip and the biocontrol of C. bowringi. Extensive research has shown that Cry8Ca was reported to have high insecticidal activity against coleopteran beetles (Jiang et al., 2017). Since Bt strain QZL38 contained sip1Ab and cry8Ca, it was speculated that there was a linkage between these two toxins vis a vis toxicity to C. bowringi. According to the results of bioactivity assay, Sip1Ab is almost certainly has high insecticidal activity, while Cry8Ca may have no insecticidal activity against C. bowringi. Furthermore, compared with a mixture of Sip1Ab and Cry8Ca, no significant difference in the LC50 of Sip1Ab was noted. A possible explanation is that no synergistic or antagonistic effect between these two toxins exists. Histopathological changes of the midgut were observed, further verifying the bioassay results. This inconsistency may be due to the different receptors for Sip1Ab and Cry8Ca in the midgut. In future investigations, on the one hand, it is possible that Sip1Ab and Cry8Ca have synergistic or antagonistic effects on the control of other coleopteran pests. For example, a study might be carried out on the basis of the known insecticidal activity of Sip against L. decemlineata. On the other hand, to develop a full picture of the correlation between Sip1Ab and Cry8Ca, additional studies could establish the basis of the insecticidal activity of Cry8Ca against A. corpulenta and A. exoleta Fald.
The midgut receptor was initially found to be a protein that could bind to membrane surface glycoproteins (Mayerson and Hall, 1986). Some studies have shown that a high affinity binding site exists between Bt toxins and BBMV in the larval midgut, and the corresponding effect was positively associated with the concentration of the toxins (Höfte and Whiteley, 1989). The findings of this study suggested that Sip interacted with BBMV. Analogous to the insecticidal mechanism of the Cry toxins, it is possible that the Sip toxins must be hydrolyzed by midgut proteases into active fragments to function. Further work should focus on determining the receptors for Sip1Ab in the BBMV via pull-down or yeast two hybrids. Nevertheless, Sip1Ab and Cry8Ca did not compete with each other in the combination assays. A possible explanation for this might be that Sip1Ab and Cry8Ca may have synergistic or antagonistic effects in different pests. Therefore, Sip1Ab may be beneficial for the avoidance of the risk of cross-resistance of insects to Cry8Ca toxins. Moreover, as has been observed in many laboratory and field pests, pyramiding with another toxin with a different mode of action and binding site would be desirable to circumvent the development of resistance to an insecticidal protein, and Sip toxins may be candidates for this.
In summary, while this study did not confirm that a specific receptor of Sip1Ab exists in the midgut, it did substantiate Sip1Ab has interactions with the BBMV. To the best of our knowledge, it is the first time pathological changes in the C. bowringi larvae midgut due to poisoning by Sip1Ab were observed using TEM. Additionally, the interaction between Sip1Ab and the BBMV in the midgut was confirmed, which has advanced our understanding of the specific insecticidal mechanism of Sip1Ab. It can also provide genetic resources for the control of coleopteran pests and shine new and important light in the field of crossing resistance improvement strategy design.
The datasets presented in this study can be found in online repositories. The names of the repository/repositories and accession number(s) can be found in the article/supplementary material.
DC, CX, and QF: conceptualization. DC, XL, RL, and HL: methodology. CX: data analysis. DC and QF: writing—original draft preparation. CX and HL: writing—review and editing. JG: supervision, project administration, and funding acquisition. All authors have read and agreed to the published version of the manuscript.
This research was funded by Heilongjiang Provincial National Science Foundation (LH2020C007).
The authors declare that the research was conducted in the absence of any commercial or financial relationships that could be construed as a potential conflict of interest.
All claims expressed in this article are solely those of the authors and do not necessarily represent those of their affiliated organizations, or those of the publisher, the editors and the reviewers. Any product that may be evaluated in this article, or claim that may be made by its manufacturer, is not guaranteed or endorsed by the publisher.
Special acknowledgment is given to all the participants in this research and their relatives for their cordial guidance and material support during the laboratory work. We thank the Heilongjiang Provincial National Science Foundation (LH2020C007) and the open fund of State Key Laboratory of Biology for Plant Diseases and Insect Pests for support.
Beard, C. E., Ranasinghe, C., and Akhurst, R. J. (2001). Screening for novel cry genes by hybridization. Lett. Appl. Microbiol. 33, 241–245. doi: 10.1046/j.1472-765x.2001.00982.x
Deist, B. R., Rausch, M. A., Fernandez-Luna, M. T., Adang, M. J., and Bonning, B. C. (2014). Bt toxin modification for enhanced efficacy. Toxins (Basel) 6, 3005–3027. doi: 10.3390/toxins6103005
Donovan, W. P., Engleman, J. T., Donovan, J. C., Baum, J. A., Bunkers, G. J., Chi, D. J., et al. (2006). Discovery and characterization of Sip1A: a novel secreted protein from Bacillus thuringiensis with activity against coleopteran larvae. Appl. Microbiol. Biotechnol. 72, 713–719. doi: 10.1007/s00253-006-0332-7
Gao, Y., Jurat-Fuentes, J. L., Oppert, B., Fabrick, J. A., Liu, C., Gao, J., et al. (2011). Increased toxicity of Bacillus thuringiensis Cry3Aa against crioceris quatuordecimpunctata, phaedon brassicae and colaphellus bowringi by a tenebrio molitor cadherin fragment. Pest Manage. Sci. 67, 1076–1081. doi: 10.1002/ps.2149
Gómez, I., Ocelotl, J., Sánchez, J., Aguilar-Medel, S., Peña-Chora, G., Lina-Garcia, L., et al. (2020). Bacillus thuringiensis Cry1Ab domain III β-22 mutants with enhanced toxicity to spodoptera frugiperda (J. E. Smith). Appl. Environ. Microbiol. 86:e01580-20. doi: 10.1128/aem.01580-20
Höfte, H., and Whiteley, H. R. (1989). Insecticidal crystal proteins of Bacillus thuringiensis. Microbiol. Rev. 53, 242–255. doi: 10.1128/mr.53.2.242-255.1989
Jiang, J., Huang, Y., Shu, C., Soberón, M., Bravo, A., Liu, C., et al. (2017). Holotrichia oblita midgut proteins that bind to Bacillus thuringiensis Cry8-like toxin and assembly of the H. oblita midgut tissue transcriptome. Appl. Environ. Microbiol. 83:e00541-17. doi: 10.1128/aem.00541-17
Lemes, A. R. N., Figueiredo, C. S., Sebastião, I., Marques da Silva, L., da Costa Alves, R., de Siqueira, H. A., et al. (2017). Cry1Ac and vip3aa proteins from Bacillus thuringiensis targeting cry toxin resistance in Diatraea flavipennella and Elasmopalpus lignosellus from sugarcane. PeerJ 5:e2866. doi: 10.7717/peerj.2866
Liu, X., Song, F., Wen, S., Wang, S., Huang, D., and Zhang, J. (2004). Study of microarray detection method of cry genes from Bacillus thuringiensis. Sci. Agric. Sin. 37, 987–992.
Liu, X. P., He, H. M., and Xue, F. S. (2014). The influence of female age on male mating preference and reproductive success in cabbage beetle, Colaphellus bowringi. Insect Sci. 21, 515–522. doi: 10.1111/1744-7917.12051
Liu, Y., Li, H., Liu, R., and Gao, J. (2012). Bt new gene sip cloning, expression, and bioinformatics analysis. Biotechol. Bull. 12, 101–105.
Mayerson, P. L., and Hall, M. O. (1986). Rat retinal pigment epithelial cells show specificity of phagocytosis in vitro. J. Cell Biol. 103, 299–308. doi: 10.1083/jcb.103.1.299
Noguera, P. A., and Ibarra, J. E. (2010). Detection of new cry genes of Bacillus thuringiensis by use of a novel PCR primer system. Appl. Environ. Microbiol. 76, 6150–6155. doi: 10.1128/aem.00797-10
Omer, A. D., Johnson, M. W., Tabashnik, B. E., Costa, H. S., and Ullman, D. E. (1993). Sweetpotato whitefly resistance to insecticides in hawaii: intra-island variation is related to insecticide use. Entomol. Exp. Et Appl. 67, 173–182. doi: 10.1111/j.1570-7458.1993.tb01666.x
Palma, L., Muñoz, D., Berry, C., Murillo, J., and Caballero, P. (2014). Bacillus thuringiensis toxins: an overview of their biocidal activity. Toxins (Basel) 6, 3296–3325. doi: 10.3390/toxins6123296
Pavani, C. D. (2013). Efeitos Da Interação e Toxicidade Das Proteínas Cry1 e Vip3Aa De Bacillus thuringiensis, Berliner em Spodoptera frugiperda (J. E. Smith) (Lepidoptera: Noctuidae). Ph. D, Thesis. Brazil: Universidade Estadual Paulista.
Peng, D., Chai, L., Wang, F., Zhang, F., Ruan, L., and Sun, M. (2011). Synergistic activity between Bacillus thuringiensis Cry6Aa and Cry55Aa toxins against meloidogyne incognita. Microb. Biotechnol. 4, 794–798. doi: 10.1111/j.1751-7915.2011.00295.x
Sha, J., Zhang, J., Chi, B., Liu, R., Li, H., and Gao, J. (2018). Sip1Ab gene from a native Bacillus thuringiensis strain QZL38 and its insecticidal activity against Colaphellus bowringi baly. Biocontrol. Sci. Technol. 28, 459–467. doi: 10.1080/09583157.2018.1460313
Shu, C., Su, H., Zhang, J., He, K., Huang, D., and Song, F. (2013). Characterization of cry9Da4, cry9Eb2, and cry9Ee1 genes from Bacillus thuringiensis strain T03B001. Appl. Microbiol. Biotechnol. 97, 9705–9713. doi: 10.1007/s00253-013-4781-5
Singh, D., Samiksha, Thayil, S. M., Sohal, S. K., and Kesavan, A. K. (2021). Exploration of insecticidal potential of cry protein purified from Bacillus thuringiensis VIID1. Int. J. Biol. Macromol. 174, 362–369. doi: 10.1016/j.ijbiomac.2021.01.143
Sinha, A., Ischia, G., Straffelini, G., and Gialanella, S. (2021). A new sample preparation protocol for SEM and TEM particulate matter analysis. Ultramicroscopy 230:113365. doi: 10.1016/j.ultramic.2021.113365
Swiecicka, I., Bideshi, D. K., and Federici, B. A. (2008). Novel isolate of Bacillus thuringiensis subsp. thuringiensis that produces a quasicuboidal crystal of cry1ab21 toxic to larvae of Trichoplusia ni. Appl. Environ. Microbiol. 74, 923–930. doi: 10.1128/aem.01955-07
Wang, J., Ding, M. Y., Wang, J., Liu, R. M., Li, H. T., and Gao, J. G. (2020). In silico structure-based investigation of key residues of insecticidal activity of sip1aa protein. Front. Microbiol. 11:984. doi: 10.3389/fmicb.2020.00984
Wu, H., Zhang, Y., Guo, S., Zhang, J., Wang, R., and Gao, J. (2007). A study on purification and activity of Cry8Ca2 protein from Bacillus thuringiensis. Plant Protection 33, 29–32.
Xue, F., Li, A., Zhu, X., Gui, A., Jiang, P., and Liu, X. (2002). Diversity in life history of the leaf beetle, Colaphellus bowringi baly. Acta Entomol. Sin. 45, 494–498.
Xue, J. L., Cai, Q. X., Zheng, D. S., and Yuan, Z. M. (2005). The synergistic activity between cry1aa and cry1c from Bacillus thuringiensis against spodoptera exigua and helicoverpa armigera. Lett. Appl. Microbiol. 40, 460–465. doi: 10.1111/j.1472-765X.2005.01712.x
Zhang, J., Li, H., Liu, R., Shu, C., and Gao, J. (2015). Explore sip gene from Bt strain DQ89 and its insecticidal activity against Colaphellus bowringi baly. Chin. J. Biol. Control 31, 598–602.
Zhang, J., Pan, Z. Z., Xu, L., Liu, B., Chen, Z., Li, J., et al. (2018). Proteolytic activation of Bacillus thuringiensis Vip3Aa protein by Spodoptera exigua midgut protease. Int. J. Biol. Macromol. 107, 1220–1226. doi: 10.1016/j.ijbiomac.2017.09.101
Zhu, L., Tian, Z., Guo, S., Liu, W., Zhu, F., and Wang, X. P. (2019). Circadian clock genes link photoperiodic signals to lipid accumulation during diapause preparation in the diapause-destined female cabbage beetles Colaphellus bowringi. Insect. Biochem. Mol. Biol. 104, 1–10. doi: 10.1016/j.ibmb.2018.11.001
Keywords: Colaphellus bowringi, Sip, Bacillus thuringiensis, BBMV, insecticidal mechanism, competition binding assay
Citation: Cao D, Xiao C, Fu Q, Liu X, Liu R, Li H and Gao J (2022) The Combination Analysis Between Bacillus thuringiensis Sip1Ab Protein and Brush Border Membrane Vesicles in Midgut of Colaphellus bowringi Baly. Front. Microbiol. 12:802035. doi: 10.3389/fmicb.2021.802035
Received: 26 October 2021; Accepted: 29 December 2021;
Published: 18 February 2022.
Edited by:
Ying Ma, University of Coimbra, PortugalReviewed by:
Fengliang Jin, South China Agricultural University, ChinaCopyright © 2022 Cao, Xiao, Fu, Liu, Liu, Li and Gao. This is an open-access article distributed under the terms of the Creative Commons Attribution License (CC BY). The use, distribution or reproduction in other forums is permitted, provided the original author(s) and the copyright owner(s) are credited and that the original publication in this journal is cited, in accordance with accepted academic practice. No use, distribution or reproduction is permitted which does not comply with these terms.
*Correspondence: Haitao Li, bGloYWl0YW9AbmVhdS5lZHUuY24=; Jiguo Gao, Z2FvamlndW8xOTYxQGhvdG1haWwuY29t
†These authors have contributed equally to this work
Disclaimer: All claims expressed in this article are solely those of the authors and do not necessarily represent those of their affiliated organizations, or those of the publisher, the editors and the reviewers. Any product that may be evaluated in this article or claim that may be made by its manufacturer is not guaranteed or endorsed by the publisher.
Research integrity at Frontiers
Learn more about the work of our research integrity team to safeguard the quality of each article we publish.