- 1Agricultural Bio-Resources Research Institute, Fujian Academy of Agricultural Sciences, Fuzhou, China
- 2Fujian Provincial Key Laboratory of Soil Environmental Health and Regulation, College of Resources and Environment, Fujian Agriculture and Forestry University, Fuzhou, China
Five strictly anaerobic strains, designated RG2T, RG3, RG10T, RF4T, and RG29, were isolated from paddy soils in China. Strains RG2T, RF4T, RG10T, RG3, and RG29 grew at temperatures ranging 5–42°C and pH ranging 5.5–8.5. Strains RG2T, RF4T, RG3, and RG29 could tolerate NaCl up to 0–0.7% (w/v) while strain RG10T could tolerate NaCl up to 0–0.8% (w/v). The isolated strains showed the highest 16S rRNA gene sequence similarities to the type strains of Geomonas terrae Red111T and Geomonas paludis Red736T. In phylogenetic (based on 16S rRNA gene sequence) and phylogenomic trees, strains clustered with the members of the genus Geomonas. Menaquinone-8 was the predominant quinone present in all strains. The major fatty acid profiles of all strains were C15:1 ω6c, C16:0, iso-C15:0, and Summed Feature 3. The digital DNA–DNA hybridization (dDDH) and average nucleotide identity (ANI) values between the isolated strains and the closely related Geomonas species were lower than the cutoff value (ANI 95–96% and dDDH 70%) for prokaryotic species delineation. Based on physiological, biochemical, and chemotaxonomic properties, strains RG2T, RG10T, and RF4T could easily be differentiated with the members of the genus Geomonas. Additionally, all the isolated strains possessed nifHDK clusters and catalytic compartments of nitrogenase. Based on the above results, the isolated five strains represent three novel species of the genus Geomonas, for which the names Geomonas oryzisoli sp. nov., Geomonas subterranea sp. nov., and Geomonas nitrogeniifigens sp. nov. are proposed. The type strains are RG10T (= GDMCC1.2537T = KCTC 26318T), RG2T (= GDMCC1.2536T = KCTC 25317T), and RF4T (= GDMCC 1.2547T = KCTC 25316T).
Introduction
The genus Geomonas was established by Xu et al. (2019). Members of the genus Geomonas are strictly anaerobic, rod-shaped, and capable of reducing ferric iron to ferrous iron and form reddish colonies due to the presence of c-type cytochromes. At the time of writing, there were nine validly published Geomonas species1 that were isolated from anoxic habitats, such as paddy soils and forest soils (Xu et al., 2019; Itoh et al., 2021).
Biological nitrogen fixation (BNF) is an essential chemical process that involves nitrogen-fixing bacteria, and these bacteria have the potential to minimize the use of N fertilizers in agriculture and reduce the negative impact of nitrogen fertilizers on the environment (Soumare et al., 2020). It has been confirmed that a large amount of nitrogen fertilizer utilization could inhibit and decrease the diversity and ability of nitrogen-fixing bacteria (Feng et al., 2018; Lin et al., 2018; Fan et al., 2019). The dominant diazotrophs in paddy soils were members of the Geobacteraceae family with iron reduction abilities (Masuda et al., 2017). In the present study, we isolated five (designated, RG2T, RG3, RG10T, RF4T, and RG29) nitrogen-fixing and iron-reducing strains from paddy soil samples obtained from a greenhouse in Fujian Province, China, where no fertilizer was administered for an extended period. The isolated five strains showed low 16S rRNA gene sequence similarity to members of the genus Geomonas; hence, the current investigation was carried out to determine their taxonomic position.
Materials and Methods
Isolation and Maintenance
Paddy soil samples were collected from a rice greenhouse belonging to Rice Research Institute, Fujian Academy of Agricultural Sciences, Fuzhou City, Fujian Province, China (N26.1076°, E119.3014°) in January 2021. All strains were isolated based on the soil slurry incubation method according to the description by Xu et al. (2019). Briefly, 10 g fresh paddy soil samples were added aseptically into 120 ml bottles containing 90 ml autoclaved oxygen-free water and shaken in the incubator for half an hour with a speed of 100 rpm at 30°C. The anaerobic and sterile water was obtained by inflating with N2:CO2 (80:20, v/v) gas mixture for at least 1 h and then autoclaved. Under sterile and oxygen-free conditions, soil suspensions were serially diluted in oxygen-free water at a concentration ranging 10–1 to 10–3. Then, 200 μl soil suspension from each dilution was spread on R2A agar plates (Hopebio, Qingdao, China) containing 40 mM disodium fumarate (Macklin, Shanghai, China) (modified R2A medium). The plates were then incubated at 30°C for 10 days in Whitley DG250 Anaerobic Workstation (Don Whitley Scientific, Bingley, United Kingdom). The red colonies were picked and purified, repeatedly re-streaked on modified R2A medium, and incubated anaerobically at 30°C for 3 days until pure single colonies were obtained. The purified strains were preserved at −80°C in modified R2A broth supplemented with 10% dimethyl sulfoxide (DMSO) and stored at −80°C. In addition, the reference strains Geomonas terrae MCCC 1K04029T, Geomonas paludis MCCC 1K03950T, and Geomonas oryzae MCCC 1K03691T (obtained from Marine Culture Collection of China through strains exchange) were cultured on the same medium mentioned as above.
Microscopic, Physiological, and Biochemical Characteristics
Gram staining was conducted using the KOH method (Gregersen, 1978). Catalase activity was determined by investigating bubble production with 3% (v/v) H2O2. Oxidase activity was determined using 1% (v/v) tetramethyl-p-phenylenediamine (Kovacs, 1956). Cell morphology was observed under a transmission electron microscope (Hitachi, Tokyo, Japan) with negative staining by 1% phosphotungstic acid. The temperature range for growth on the modified R2A agar plate was determined at different temperatures (5, 10, 12, 15, 20, 25, 30, 33, 35, 37, 40, and 42°C) for 5–14 days. The pH range (5.0–9.0 at an interval of 0.5) for growth on modified R2A medium was determined at 30°C for 7 days. The pH of the basal medium was adjusted using the buffer system, as described by Narsing Rao et al. (2020). The effect of NaCl concentration on growth was determined on modified R2A medium supplemented with 0–1.0% (w/v) NaCl. Other enzymatic activities were examined using the API-ZYM microbiological identification system (bioMerieux, Marcy-l’Étoile, France) by following the manufacturer’s instructions. The sensitivity of antibiotics such as clindamycin (2 μg), streptomycin (10 μg), vibramycin (30 μg), erythromycin (15 μg), gentamicin (120 μg), rifampicin (5 μg), vancomycin (30 μg), azithromycin (15 μg), penicillin G (10 μg), cefazolin (30 μg), ampicillin (10 μg), chloromycetin (30 μg), tetracycline (30 μg), oxacillin (1 μg), amikacin (30 μg), polymyxin B (300 IU), gentamicin (10 μg), kanamycin (30 μg), and neomycin (30 μg) was tested.
16S rRNA Gene Sequencing and Phylogenetic Analysis
Genomic DNA was extracted using the TIANamp Bacterial DNA kit (TIANGEN, Beijing, China) according to the manufacturer’s instruction. PCR amplification was performed as described by Dong et al. (2019). Pairwise 16S rRNA gene sequence similarities among the isolated five strains and against other type strains were calculated using the EzBioCloud platform (Yoon et al., 2017a). For phylogenetic analysis, 16S rRNA gene sequences of the closely related type strains were downloaded from the EzBioCloud platform. Phylogenetic trees were reconstructed based on the neighbor-joining (NJ) (Saitou and Nei, 1987), maximum likelihood (ML) (Felsenstein, 1981), and maximum-parsimony (Fitch, 1971) methods with the Kimura two-parameter model (Kimura, 1980) and 1,000 bootstrap replications (Felsenstein, 1985) using MEGA version X (Kumar et al., 2018).
Chemotaxonomic Characterization
For fatty acid detection, the biomass of the isolated five strains and their related type strains was collected from cultures grown for 3 days at 30°C. The cellular fatty acids in the cell walls were extracted and analyzed according to the standard protocol of the Microbial Identification System (MIDI) tested by gas chromatography (model 7890, Agilent) (Sasser, 2001). The biomass of the isolated five strains for menaquinone analysis was collected from cultures grown for 7 days at 30°C in modified R2A broth and then analyzed as described by Collins et al. (1977) using reverse-phase HPLC (Kroppenstedt, 1982).
Genome Sequencing and Analysis
The complete genomes of strains RG2T, RG3, RG10T, RF4T, and RG29 were sequenced using the Nanopore PromethION platform and Illumina NovaSeq PE150 at the Novogene Bioinformatics Technology Company, Ltd. (Beijing, China). A library was reconstructed with the Nanopore PromethION platform (10 kb) and Illumina NovaSeq PE150 (350 bp). Unicycler software (Wick et al., 2017) was used to combine PE150 and Nanopore data, screen the chromosome and plasmid sequence, and assemble the chromosome sequence into a circular genome. Genome quality was estimated by CheckM (Parks et al., 2015). Transfer RNA (tRNA) genes were predicted with tRNAscan-SE version 1.3.1 (Lowe and Eddy, 1997). Ribosomal RNA (rRNA) genes were analyzed with rRNAmmer (Lagesen et al., 2007). Genomic relatedness was estimated based on the average nucleotide identity (ANI) and digital DNA–DNA hybridization (dDDH), which were calculated using the EzBioCloud platform (Yoon et al., 2017b) and Genome-to-Genome Distance Calculator version 2.1 (Meier-Kolthoff et al., 2013), respectively. The phylogenomic tree was reconstructed using the Anvi’o tool (Eren et al., 2015). 38 marker genes of ribosomal proteins (L1, L2, L13, L14, L16, L17, L18p, L19, L20, L21p, L22, L23, L24, L27, L27A, L28, L29, L3, L32p, L35p, L4, L5, L6, L9 C, S10, S11, S13, S15, S16, S17, S19, S2, S3 C, S6, S7, S8, S9, and S20p) were extracted and aligned using MUSCLE (Edgar, 2004) followed by generation of a phylogenomic tree using FastTree (Price et al., 2009). The resulting tree was visualized by MEGA version X (Kumar et al., 2018). Functional annotation was performed by KofamKOALA (Aramaki et al., 2020) using the anvi-run-kegg-kofams program (Eren et al., 2015). The genes related to iron oxidation, iron reduction, iron gene regulation, iron storage, iron transport, magnetosome, and siderophore were checked using FeGenie software (Garber et al., 2020).
Nitrogen Fixation and Electron Donors and Acceptors
Five isolated strains were grown in 100 ml of modified R2A liquid medium at 30°C for 3 days under anaerobic conditions. Late-log-phase cells were harvested by centrifugation (∼3 × 108 cells/ml) and then washed three times using sterilized ammonium-free MFM (L–1: including 2.0 g KHCO3, 0.02 g MgSO4 7H2O, 0.3 g KH2PO4, 0.1 g MgCl2⋅6H2O, 0.08 g CaCl2⋅2H2O, 0.6 g NaCl, 9.52 g HEPES, 20 mM acetate, 20 mM disodium fumarate), 10.0 ml vitamin stock solution (L–1, 2 mg biotin, 2 mg folic acid, 10 mg pyridoxine-HCl, 5 mg thiamine-HCl, 5 mg nicotinic acid, 5 mg aminobenzoic acid, 5 mg Ca-pantothenate, 0.01 mg vitamin B12, and 5 mg lipoic acid), and 10.0 ml mineral stock solution (L–1, 12.8 g nitrilotriacetic acid, 1.35 g FeCl3⋅6H2O, 0.1 g MnCl2⋅4H2O, 0.024 g CoCl2⋅6H2O, 0.1 g CaCl2⋅2H2O, 0.1 g ZnCl2, 0.025 g CuCl2⋅2H2O, 0.01 g H3BO3, 0.024 g Na2MoO4⋅2H2O, 1 g NaCl, 0.12 g NiCl2⋅6H2O, 4 mg Na2SeO3⋅5H2O, 4 mg Na2WO4, and pH 6.5) liquid medium under sterile conditions. The washed cells were resuspended with 30 ml volumes of sterilized ammonium-free medium in a 100-ml bottle and incubated at 30°C without shaking after being sealed under mixed gas He:C2H2 (90:10, v/v). The negative control was set up using pure He gas in replacement of He/C2H2 gas. Nitrogen fixation activity was measured by the traditional acetylene reduction activity (ARA) method based on C2H2 reduction into C2H4 by nitrogenase (Postgate, 1972). C2H4 production in the gaseous phase was measured by gas chromatography equipped with a fused-silica column (Porapak; Hychrom) as described by Nakajima et al. (2012). Cell densities were determined by direct cell counting using a hemocytometer according to the manufacturer’s instructions. The PolF/PolR primer set (Poly et al., 2001) was used to amplify the nifH gene, and PCR amplification was carried out as described by Lee et al. (2019).
Electron donor and acceptor utilization tests were performed using 10 ml of degassed modified freshwater medium (MFM) in a 20 ml serum tube under a N2/CO2 (4:1, v/v) atmosphere at 30°C without shaking. Acetate (10 mM) was used as an electron donor for all electron acceptor tests, while Fe (III)-NTA (5 mM) was used as an electron acceptor for all electron donor tests. The utilized electron donors were determined according to cell growth. The utilization of electron acceptors of Fe (III) compounds was determined by the color change from reddish-brown/green to colorless, whereas those of nitrate, fumarate, and sulfur were determined by cell growth. Fermentation growth in the absence of electron acceptors was evaluated with fumarate, lactate, glycerol, pyruvate, malate, and ethanol by checking the optical density at 600 nm.
Cytochromes and Ferric Reduction
Cytochrome analysis was prepared from the cells cultured in modified R2A broth. 5 ml cultures were collected and resuspended in 9 ml of 20 mM PIPES buffer (pH 7.0). A dithionite-reduced minus air-oxidized difference spectrum of the whole cells was checked using a UV-2600 (Shimadzu, Japan) spectrophotometer (Lovley et al., 1993). The ferric reduction ability was measured using 10 ml MM (L–1: including 2.5 g NaHCO3, 0.25 g NH4Cl, 0.68 g NaH2PO4⋅2H2O, 0.1 g KCl, 10.0 ml vitamin stock solution, and 10.0 ml mineral stock solution) medium in a 20 ml serum tube supplemented with 10 mM acetate and 20 mM ferrihydrite, under a 80:20 mixture gas of N2/CO2. The vessel was incubated at 30°C without shaking, and every set of experiments was performed in triplicate. The soluble ferrous concentration was detected immediately using ferrozine buffer as described by Onley et al. (2018). Absorbance was measured using a spectrophotometer (Shimadzu, Japan) at a wavelength of 562 nm. A standard curve was prepared with ferrous ammonium sulfate hexahydrate spanning a dilution concentration range of 0.1–0.8 mM ferrous ions.
Results and Discussion
Microscopic, Physiological, and Biochemical Characters
Cells of strains RG2T, RG3, RG10T, RF4T, and RG29 were Gram-stain-negative, non-spore-forming, strictly anaerobic, and motile and produced clear red colonies on a modified R2A agar plate. These characters were similar to Geomonas species (Xu et al., 2019; Itoh et al., 2021). Cells of strains were catalase and oxidase negative. TEM images showed rod-shaped cells with peritrichous flagella (Supplementary Figure 1). Strains RG2T, RF4T, RG10T, RG3, and RG29 grew at temperatures 12–42°C (optimum 30°C), 10–40°C (optimum 30–33°C), 16–42°C (optimum 30–33°C), 5–42°C (optimum 30–33°C), and 10–40°C (optimum 30–35°C), respectively. All strains grew at pH ranging 5.5–8.5. Strains RG2T, RF4T, RG3, and RG29 could tolerate NaCl up to 0–0.7% (w/v) while strain RG10T could tolerate NaCl up to 0–0.8% (w/v). Strains RG2T, RG3, RG10T, RF4T, and RG29 were positive for alkaline phosphatase, but G. terrae MCCC 1K04029T and G. oryzae S43T (Xu et al., 2019) were negative. Strains RG3, RG29, and G. paludis MCCC 1K03950T and G. oryzae S43T (Xu et al., 2019) were positive for esterase lipase (C8) but not strains RG2T, RG10T, RF4T, and G. terrae MCCC 1K04029T. For the antibiotic sensitivity test, five isolated strains RG2T, RG3, RG10T, RF4T, and RG29 were sensitive to clindamycin, chloromycetin, amikacin, kanamycin, rifampin, polymyxin B, vibramycin, tetracycline, and erythromycin, but not to gentamicin, streptomycin, vancomycin, and azithromycin. Strain RG10T was sensitive to oxacillin, but the other four stains were not. Strain RF4T was not sensitive to cefazolin, penicillin G, ampicillin, oxacillin, and neomycin whereas the other four strains were sensitive. Detailed differential features of the isolated five strains and closely related members are listed in Table 1.
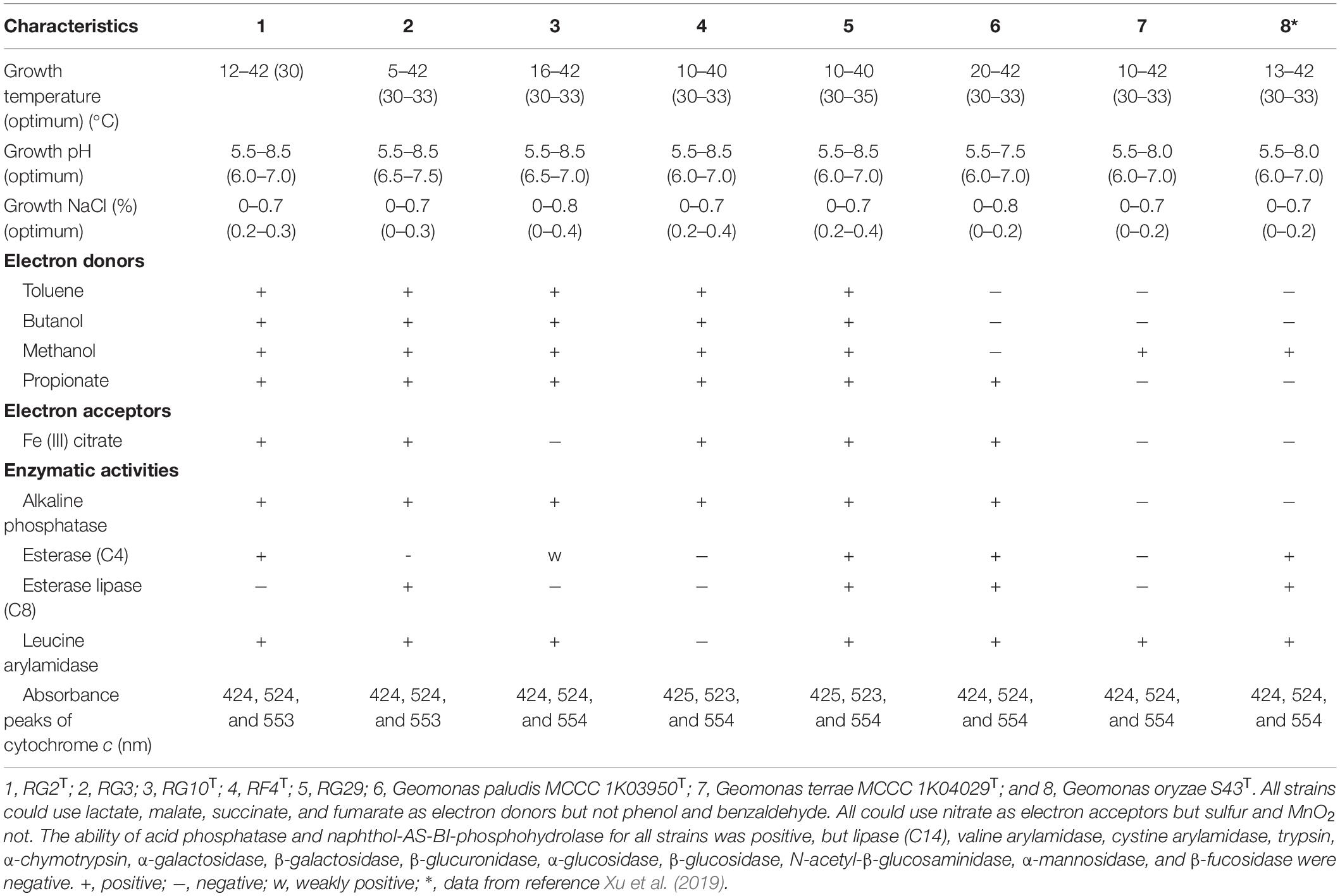
Table 1. Differential characteristics of five isolated strains and closely related members of the genus Geomonas.
16S rRNA Gene Sequencing and Phylogenetic Analysis
The isolated strains, RG2T, RG3, RG10T, RF4T, and RG29 had 98.5–99.9% 16S rRNA gene sequence similarity to each other. Strains RG2T, RG3, and RG29 showed the highest 16S rRNA gene sequence similarities to the type strain of G. terrae Red 111T (98.2–98.5%) (Supplementary Table 1). These values were below the threshold (98.65%) for species delineation (Kim et al., 2014) but above the threshold (94.55–95.05%) for genus delineation (Yarza et al., 2014), suggesting that these strains were different at the species level within the genus Geomonas. Strains RF4T and RG10T had the highest similarity to the type strain of G. paludis Red 736T (99.1–99.3%). Despite the fact that the sequence similarity of RF4T and RG10T was quite high, numerous earlier studies have revealed that two strains with 16S rRNA gene similarity greater than 99.0% were verified as separate species (Tohno et al., 2017; Hahn et al., 2018; Qin et al., 2020). A range of thresholds (98.2–99.0%) was also recommended by Meier-Kolthoff et al. (2013). Therefore, strains RG10T and RF4T might be novel species of the genus Geomonas.
The maximum likelihood phylogenetic tree based on the 16S rRNA gene sequences showed that strains RG2T, RG3, RG10T, RF4T, and RG29 clade with Geomonas members (Figure 1). The phylogenetic position was also confirmed by the trees generated using neighbor-joining (Supplementary Figure 2) and maximum parsimony (Supplementary Figure 3) methods. In the phylogenomic tree, all strains clustered with the members of the genus Geomonas (Figure 2).
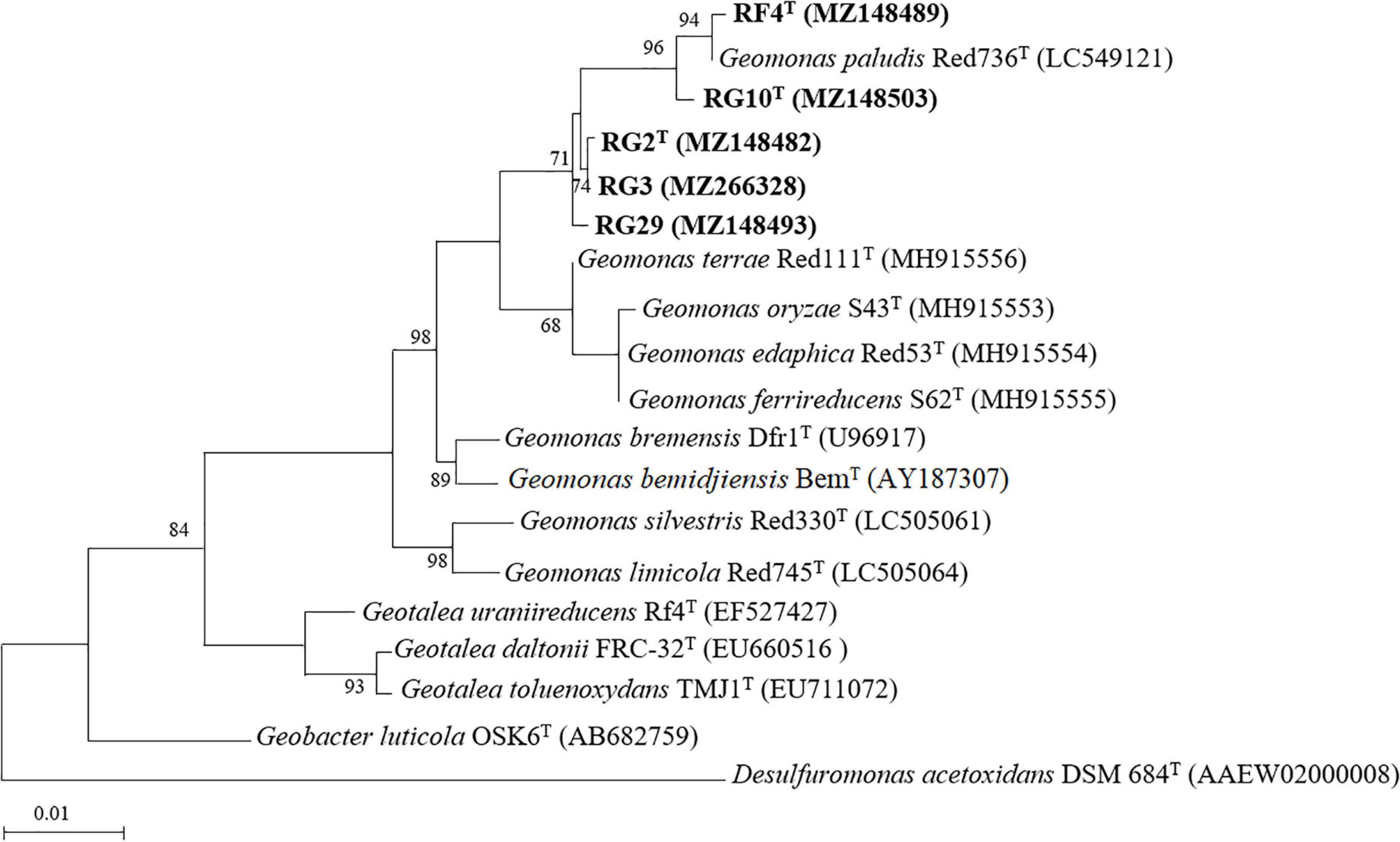
Figure 1. Maximum-likelihood phylogenetic tree based on 16S rRNA gene sequence of five isolated strains and their closely related type strains. Bootstrap values (expressed as percentages of 1,000 replications) greater than 50% are shown at branch points. Bar, 0.01 substitutions per nucleotide position. Desulfuromonas acetoxidans DSM 684T is used as an outgroup. Number in the parenthesis is the GenBank accession number.
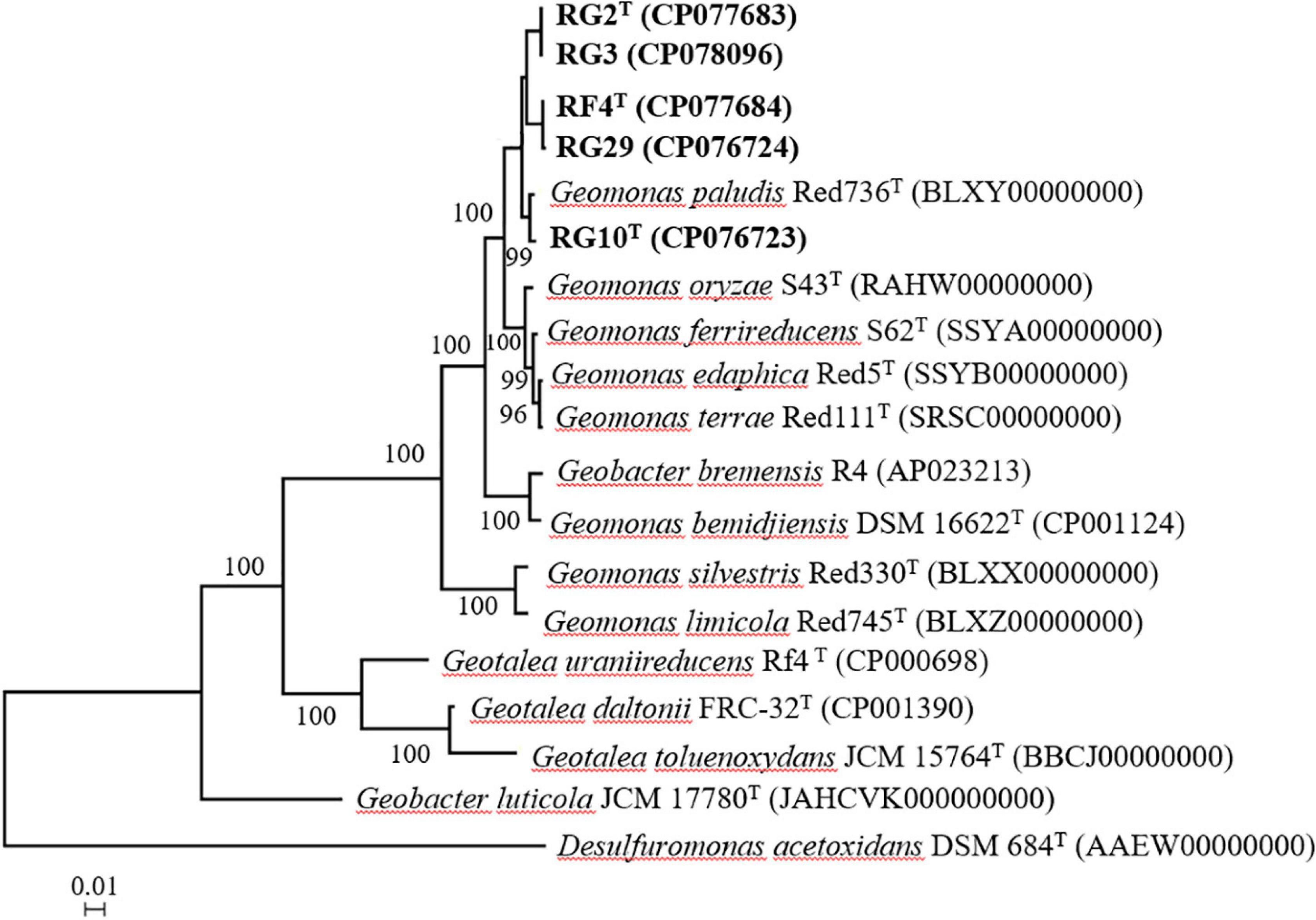
Figure 2. Phylogenomic tree based on the amino acid sequences of 38 ribosomal proteins of five strains and their closely related strains. Bootstrap values (expressed as percentages of 1,000 replications) greater than 50% are shown at branch points. Bar, 0.01 substitutions per nucleotide position. Desulfuromonas acetoxidans DSM 684T is used as an outgroup. Number in the parenthesis is the genome accession number.
Chemotaxonomic Characters
The fatty acid profiles clearly distinguished the isolated five strains RG2T, RG3, RG10T, RF4T, RG29, and their related type strains. All five strains contained C15:1 ω6c, iso-C15:0, C16:0, and Summed Feature 3 as major fatty acid profiles, but their proportion was distinguished. Strains RG2T, RG3, RG10T, RF4T, and RG29 had iso-C15:0 and Summed Feature 3 levels more than 10% (Table 2), but it was < 10% in G. paludis MCCC 1K03950T and G. oryzae MCCC 1K03691T. Fatty acid C17:1 ω6c presented in strains RG2T, RG3, and type strain G. paludis MCCC 1K03950T was above 5% whereas it was much lower than 5% in other three strains and related type strains G. terrae MCCC 1K04029T and G. oryzae MCCC 1K03691T. C15:1 ω6c was high in strain RG2T and C16:0 in RG10T; this value was less when compared with G. paludis MCCC 1K03950T. The iso-C15:0 value in G. terrae MCCC 1K04029T and G. oryzae MCCC 1K03691T was higher compared with other strains. Detailed comparative fatty acid profiles of strains RG2T, RG3, RG10T, RF4T, RG29, G. paludis MCCC 1K03950T, G. oryzae MCCC 1K03691T, and G. terrae MCCC 1K04029T are mentioned in Table 2.
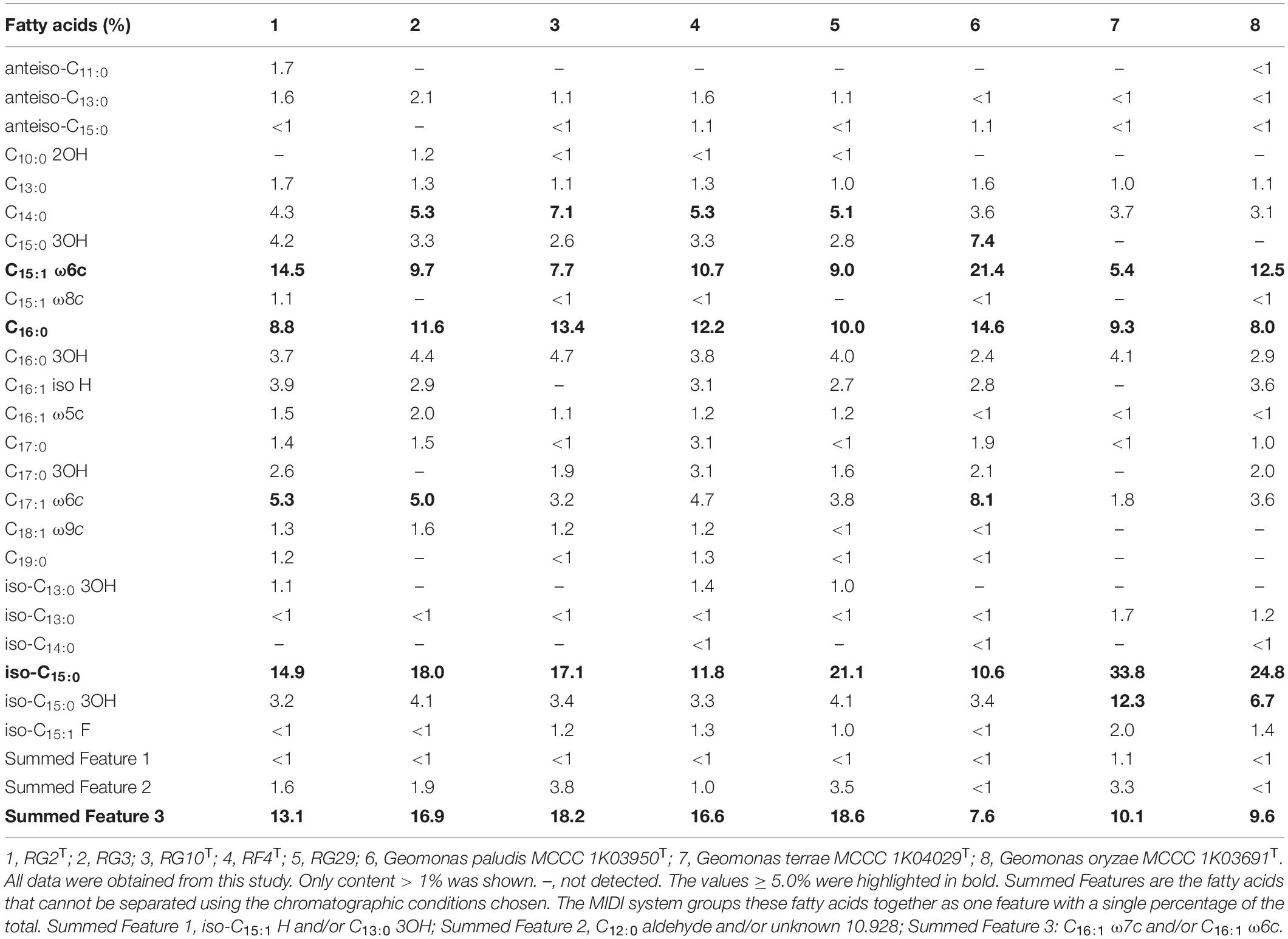
Table 2. Fatty acid profile of isolated five strains and closely related species of the genus Geomonas.
The menaquinone composition was detected for all five isolated strains in the present study, menaquinone-8 (MK-8) was characterized as the predominant respiratory quinone, and small amounts of MK-7 and MK-9 were also identified for all strains, which was consistent with other type strains of the genus Geomonas (Xu et al., 2019; Itoh et al., 2021).
Genome Analysis
The genome size of strains RG2T, RG3, RG10T, RF4T, and RG29 was ∼4.76–4.99 Mb, containing 4,394, 4,341, 4,181, 4,224, and 4,138 protein-coding genes, respectively (Supplementary Table 2). All five isolated strains had 12 rRNAs including 4 of 5S, 4 of 16S, and 4 of 23S rRNAs (Supplementary Table 2). The 16S rRNA gene of strains RG2T, RG3, RG10T, RF4T, and RG29 obtained genome was similar to the 16S rRNA gene obtained by PCR (Supplementary Table 2). The genomic DNA G + C content of strains RG2T, RG3, RG10T, RF4T, and RG29 was 61.9, 61.9, 61.8, 61.7, and 62.0%, respectively (Supplementary Table 2). The genomic similarity values between the five isolated strains and the type strains of Geomonas species were below 96% for ANI and 70% for dDDH. Moreover, the ANI and dDDH values among the five isolated strains were 87.2–99.4% and 32.4–100%, respectively (Supplementary Table 3). Considering the recommended thresholds for species delineation, 96% for ANI, and 70% for dDDH (Goris et al., 2007; Richter and Rosselló-Móra, 2009; Meier-Kolthoff et al., 2013), strains RG2T, RG10T, and RF4T were suggested to be three different species of genus Geomonas.
Functional annotation (Supplementary Table 4) analysis suggests that all strains encode key genes for glycolysis and tricarboxylic acid but only strains RG2T and RG3 encode genes for the pentose phosphate pathway.
Autotrophic prokaryotic representatives play an important role in ecosystems by supplying organic carbon to heterotrophs (Hügler et al., 2005). The Calvin–Benson–Bassham cycle is the most significant autotrophic carbon fixation mechanism that exists today (Bassham et al., 1954; Hügler et al., 2005). Despite its worldwide importance, it is limited to species having a high-energy production from a chemotrophic or phototrophic lifestyle. Microorganisms found in extreme environments, such as high temperatures and anaerobic or acidic conditions, generally use different CO2 fixation pathways, and three alternative pathways are currently known: the reductive tricarboxylic acid cycle, the reductive acetyl coenzyme A (CoA) pathway, and the 3-hydroxypropionate cycle (Hügler et al., 2005). The key genes for the Calvin–Benson–Bassham and 3-hydroxypropionate cycle were not noticed in any strains, but the key genes related to the reductive tricarboxylic acid cycle were present in all strains.
BNF is the most common method of converting atmospheric nitrogen gas (N2) to ammonia, and all known diazotrophs include at least one of three closely related nitrogenase subtypes: Nif, Vnf, and Anf. The nifHDK core set and catalytic compartment of nitrogenase (Dos Santos et al., 2012) were noticed in all strains (Supplementary Tables 2, 4). Furthermore, genes related to dissimilatory nitrate reduction (narGHI and nrfA) were also noticed in all strains.
Members of the genus Geomonas were known for metal reduction (Xu et al., 2019). It has been demonstrated that certain c-type cytochromes may form a conductive channel capable of crossing the inner membrane, periplasm, and outer membrane, allowing Geobacteraceae species to directly transport electrons from the quinone/quinol pool to metal oxides (Guo et al., 2021). The bacteria develop electrically conductive protein nanowires, known as e pili, which are built from the PilA pilin monomer to perform long-range extracellular electron transfer to metal oxide particles (Lovley and Walker, 2019; Guo et al., 2021). Furthermore, protein components such as CymA, MtrA, MtrB, MtrC, and OmcA have also been reported for Fe(III) oxide reduction (Shi et al., 2012). In the present study, all strains encode genes for iron reduction, iron gene regulation, iron acquisition-iron transport, and siderophore transport (Supplementary Figure 4). The details of the key genes for iron reduction, iron gene regulation, iron acquisition-iron transport, and siderophore transport are mentioned in Supplementary Table 5. All strains harbored merA but not merB, indicating that they might be susceptible to mercury. Although previous studies indicated that certain Geomonas species contained kaiBC genes (encoding essential proteins in circadian rhythms) (Wang et al., 2019; Itoh et al., 2021), we did not detect this in our study. Details of all the metabolic potential of the present study strains are mentioned in Supplementary Table 4.
Nitrogen Fixation and Electron Donors and Acceptors
In all strains, the nifH gene was amplified (Supplementary Figure 5A). Nitrogen fixation activity was also estimated based on acetylene reduction activity (ARA). The ARAs of strains RG2T, RG3, RG10T, RF4T, and RG29 were (1.7 ± 0.2) × 10–6, (2.1 ± 0.4) × 10–6, (1.2 ± 0.7) × 10–6, (3.2 ± 0.2) × 10–6, and (2.8 ± 0.2) × 10–6 nmol C2H4/h/cell, respectively (Supplementary Figure 5B). Therefore, it suggests that all the isolated five strains could fix nitrogen. Supplementary Figure 5B clearly shows that strain RF4T had the highest nitrogen-fixing ability among all the strains.
The availability of 10 electron donors for the isolated five strains was similar, while electron acceptors were different (Table 1); for example, strains RG2T, RG3, RF4T, and RG29 could utilize Fe (III) citrate as an electron acceptor but strain RG10T could not. For the fermentation growth, strains RF4T, RG2T, RG3, RG10T, and RG29 showed weak growth using fumarate as a substrate in the absence of electron acceptors, but RF4T and RG29 did not grow with malate and pyruvate. Strain RG10T did not grow with lactate and glycerol. Strain RG3 grew with ethanol but not strain RG2T.
Cytochromes and Ferric Reduction
Five isolated strains possessed ferric reducing ability, which reduced ferrihydrite to ferrous iron in 25 days. Although they had ferric reducing ability, their reduction rates were different (Supplementary Figure 6). Strain RF4T showed the highest iron reduction ability among the five strains.
The spectra of whole cells of all the isolated five strains suggest they could produce c-type cytochromes (Supplementary Figure 7). The absorbance peaks of reduced cytochrome c of strains RG2T, RG3, RG10T, RF4T, and RG29 were 424, 524, and 553 nm; 424, 524, and 553 nm; 424, 524, and 554 nm; 425, 523, and 554 nm; 425, 523, and 554 nm, respectively, which were quite similar to those of the type strains of Geomonas species (Table 1).
Taxonomic Conclusion
Based on the above results, three strains, RG10T, RG2T, and RF4T represent three novel species of the genus Geomonas, for which the names Geomonas oryzisoli sp. nov., Geomonas subterranea sp. nov., and Geomonas nitrogeniifigens sp. nov. are proposed.
Description of Geomonas oryzisoli sp. nov.
Geomonas oryzisoli (o.ry.zi.so’li L. fem. n. oryza, rice; L. neut. n. solum soli, of the soil; N.L. gen. n. oryzisoli of rice root soil).
Cells are Gram-stain-negative, non-spore-forming, motile (with peritrichous flagella), strictly anaerobic, and rod-shaped. Colonies are small, red, and circular. Growth occurs at 16–42°C (optimum 30–33°C), pH 5.5–8.5 (optimum 6.5–7.0), and 0–0.8% (w/v) NaCl (optimum 0–0.4%). Catalase and oxidase tests are negative. With Fe (III)-NTA as an electron acceptor, acetate, toluene, butanol, methanol, lactate, malate, propionate, succinate, and fumarate can be used as electron donors but not phenol and benzaldehyde. With acetate as electron donor, Fe (III)-NTA, ferrihydrite, fumarate, nitrate, and malate can be utilized as electron acceptors, but not Fe (III)-citrate, oxygen, sulfur, and MnO2. Weak growth is observed using fumarate, ethanol, malate, and pyruvate as a substrate in the absence of electron acceptors but not with lactate and glycerol. It is positive for alkaline phosphatase, esterase (C4), leucine arylamidase, acid phosphatase, and naphthol-AS-BI-phosphohydrolase but negative for esterase (C4), esterase lipase (C8), lipase (C14), valine arylamidase, cystine arylamidase, trypsin, α-chymotrypsin, α-galactosidase, β-galactosidase, β-glucuronidase, α-glucosidase, β-glucosidase, N-acetyl-β-glucosaminidase, α-mannosidase, and β-fucosidase. The genome consists of a dinitrogenase core gene set of nifHDK. The major fatty acids (>5%) are C14:0, C15:1 ω6c, C16:0, iso-C15:0, and Summed Feature 3. MK-8 is the major respiratory quinone. The genomic DNA G + C content is 61.8%.
The type strain, RG10T (= GDMCC1.2537T = KCTC 26318T), was isolated from paddy soil collected from the greenhouse in Fujian Province, China. The 16S rRNA gene and whole-genome sequence of strain RG10T have been deposited in the GenBank/ENA/DDBJ under the accession numbers MZ148503 and CP076723, respectively.
Description of Geomonas subterranea sp. nov.
Geomonas subterranea (sub.ter.ra’ne.a L. fem. adj. subterranea, underground).
Cells are Gram-stain-negative, non-spore-forming, motile (with peritrichous flagella), strictly anaerobic, and rod-shaped. Colonies are small, red, and circular. Growth occurs at 12–42°C (optimum 30°C), pH 5.5–8.5 (optimum 6.0–7.0), and 0–0.7% (w/v) NaCl (optimum 0.2–0.3%). Catalase and oxidase tests are negative. With Fe (III)-NTA as an electron acceptor, acetate, toluene, butanol, methanol, lactate, malate, propionate, succinate, and fumarate can be used as electron donors but not phenol and benzaldehyde. With acetate as electron donor, Fe (III)-NTA, Fe (III)-citrate, ferrihydrite, fumarate, nitrate, and malate can be utilized as electron acceptors, but not oxygen, sulfur, and MnO2. Weak growth is observed using fumarate, glycerol, malate, lactate, pyruvate, and fumarate as a substrate in the absence of electron acceptors, but not with ethanol. It is positive for alkaline phosphatase, esterase (C4), leucine arylamidase, acid phosphatase, and naphthol-AS-BI-phosphohydrolase, but negative for esterase lipase (C8), lipase (C14), valine arylamidase, cystine arylamidase, trypsin, α-chymotrypsin, α-galactosidase, β-galactosidase, β-glucuronidase, α-glucosidase, β-glucosidase, N-acetyl-β-glucosaminidase, α-mannosidase, and β-fucosidase. The major fatty acids (>5%) are C15:1 ω6c, C16:0, C17:1 ω6c, iso-C15:0, and Summed Feature 3. The genome consists of a dinitrogenase core gene set of nifHDK. MK-8 is the major respiratory quinone. The genomic DNA G + C content is 61.9%.
The type strain, RG2T (= GDMCC1.2536T = KCTC 25317T), was isolated from paddy soil collected from the greenhouse in Fujian Province, China. The 16S rRNA gene and whole-genome sequence of strain RG2T have been deposited in the GenBank/ENA/DDBJ under the accession numbers MZ148482 and CP077683, respectively.
Description of Geomonas nitrogeniifigens sp. nov.
Geomonas nitrogeniifigens (ni.tro.ge.ni.i’fi.gens N.L. neut. n. nitrogenium nitrogen; L. pres. part. figens fixing; N.L. part. adj. nitrogeniifigens, nitrogen-fixing).
Cells are Gram-stain-negative, non-spore-forming, motile (with peritrichous flagella), strictly anaerobic, and rod-shaped. Colonies are small, red, and circular. Growth occurs at 10–40°C (optimum 30–33°C), pH 5.5–8.5 (optimum pH 6.0–7.0), and 0–0.7% (w/v) NaCl (optimum 0.2–0.4%). Catalase and oxidase are negative. With Fe (III) –NTA as an electron acceptor, acetate, toluene, butanol, methanol, lactate, malate, propionate, succinate, and fumarate can be used as electron donors but not phenol and benzaldehyde. With acetate as electron donor, Fe (III)-citrate, Fe (III)-NTA, ferrihydrite, fumarate, and nitrate can be utilized as electron acceptors, but not malate, oxygen, and sulfur and MnO2. Weak growth is observed using fumarate, ethanol, glycerol, and lactate as a substrate in the absence of electron acceptors, but not with malate and pyruvate. It is positive for alkaline phosphatase, acid phosphatase, and naphthol-AS-BI-phosphohydrolase, but negative for esterase (C4), esterase lipase (C8), lipase (C14), leucine arylamidase, valine arylamidase, cystine arylamidase, trypsin, α-chymotrypsin, α-galactosidase, β-galactosidase, β-glucuronidase, α-glucosidase, β-glucosidase, N-acetyl-β-glucosaminidase, α-mannosidase, and β-fucosidase. The genome consists of a dinitrogenase core gene set of nifHDK. The major fatty acids are C14:0, C15:1 ω6c, C16:0, iso-C15:0, and Summed Feature 3. MK-8 is the major respiratory quinone. The genomic DNA G + C content is 61.7%.
The type strain, RF4T (= GDMCC 1.2547T = KCTC 25316T), was isolated from paddy soil collected from the greenhouse in Fujian Province, China. The 16S rRNA gene and whole-genome sequence of strain RF4T have been deposited in GenBank/ENA/DDBJ under the accession numbers MZ148489 and CP077684, respectively.
Accession Numbers
The GenBank accession numbers for 16S rRNA gene and genome sequence of strains RG2T, RG3, RG10T, RF4T, and RG29 were MZ148482 and CP077683, MZ266328 and CP078096, MZ148503 and CP076723, MZ148489 and CP077684, and MZ148493 and CP076724, respectively.
Data Availability Statement
The datasets presented in this study can be found in online repositories. The names of the repository/repositories and accession number(s) can be found below: https://www.ncbi.nlm.nih.gov/nuccore/CP077683.1, https://www.ncbi.nlm.nih.gov/nuccore/CP078096.1, https://www.ncbi.nlm.nih.gov/nuccore/CP076723.1, https://www.ncbi.nlm.nih.gov/nuccore/CP077684.1, and https://www.ncbi.nlm.nih.gov/nuccore/CP076724.1.
Author Contributions
G-HL and S-GZ designed the research and project outline. SY and RT performed the DNA extraction and chemotaxonomic analysis. C-JX performed the genome analysis. G-HL drafted the manuscript. All authors read and approved the final manuscript.
Funding
This work was financially supported by a grant from the Fujian Special Fund for Scientific Research Institutes in the Public Interest (2020R1034001).
Conflict of Interest
The authors declare that the research was conducted in the absence of any commercial or financial relationships that could be construed as a potential conflict of interest.
Publisher’s Note
All claims expressed in this article are solely those of the authors and do not necessarily represent those of their affiliated organizations, or those of the publisher, the editors and the reviewers. Any product that may be evaluated in this article, or claim that may be made by its manufacturer, is not guaranteed or endorsed by the publisher.
Acknowledgments
We thank Jianfu Zhang of Rice Research Institute, Fujian Academy of Agricultural Sciences for providing soil samples, and A. Oren for his advice on nomenclature.
Supplementary Material
The Supplementary Material for this article can be found online at: https://www.frontiersin.org/articles/10.3389/fmicb.2021.801462/full#supplementary-material
Abbreviations
dDDH, digital DNA-DNA hybridization; ANI, average nucleotide identity.
Footnotes
References
Aramaki, T., Blanc-Mathieu, R., Endo, H., Ohkubo, K., Kanehisa, M., Goto, S., et al. (2020). KofamKOALA: KEGG Ortholog assignment based on profile HMM and adaptive score threshold. Bioinformatics 36, 2251–2252. doi: 10.1093/bioinformatics/btz859
Bassham, J. A., Benson, A. A., Kay, L. D., Harris, A. Z., Wilson, A. T., and Calvin, M. (1954). The path of carbon in photosynthesis. XXI. The cyclic regeneration of carbon dioxide acceptor. J. Am. Chem. Soc. 76, 1760–1770. doi: 10.1021/ja01636a012
Collins, M. D., Pirouz, T., Goodfellow, M., and Minnikin, D. E. (1977). Distribution of menaquinones in actinomycetes and corynebacteria. J. Gen. Microbiol. 100, 221–230. doi: 10.1099/00221287-100-2-221
Dong, Z. Y., Narsing Rao, M. P., Wang, H. F., Fang, B. Z., Liu, Y. H., Li, L., et al. (2019). Transcriptomic analysis of two endophytes involved in enhancing salt stress ability of Arabidopsis thaliana. Sci. Total Environ. 686, 107–117. doi: 10.1016/j.scitotenv.2019.05.483
Dos Santos, P. C., Fang, Z., Mason, S. W., Setubal, J. C., and Dixon, R. (2012). Distribution of nitrogen fixation and nitrogenase-like sequences amongst microbial genomes. BMC Genomics 13:162. doi: 10.1186/1471-2164-13-162
Edgar, R. C. (2004). MUSCLE: multiple sequence alignment with high accuracy and high throughput. Nucleic Acids Res. 32, 1792–1797. doi: 10.1093/nar/gkh340
Eren, A. M., Esen, ÖC., Quince, C., Vineis, J. H., Morrison, H. G., Sogin, M. L., et al. (2015). Anvi’o: an advanced analysis and visualization platform for ’omics data. PeerJ. 3:e1319. doi: 10.7717/peerj.1319
Fan, K., Delgado-Baquerizo, M., Guo, X., Wang, D., Wu, Y., Zhu, M., et al. (2019). Suppressed N fixation and diazotrophs after four decades of fertilization. Microbiome 7:143. doi: 10.1186/s40168-019-0757-8
Felsenstein, J. (1981). Evolutionary trees from DNA sequences: a maximum likelihood approach. J. Mol. Evol. 17, 368–376. doi: 10.1007/bf01734359
Felsenstein, J. (1985). Confidence limits on phylogenies: an approach using the bootstrap. Evolution 39, 783–791. doi: 10.1111/j.1558-5646.1985.tb00420.x
Feng, M., Adams, J. M., Fan, K., Shi, Y., Sun, R., Wang, D., et al. (2018). Long-term fertilization influences community assembly processes of soil diazotrophs. Soil Biol. Biochem. 126, 151–158. doi: 10.1016/j.soilbio.2018.08.021
Fitch, W. M. (1971). Toward defining the course of evolution: minimum change for a specific tree topology. Syst. Zool. 20, 406–416. doi: 10.2307/2412116
Garber, A. I., Nealson, K. H., Okamoto, A., McAllister, S. M., Chan, C. S., Barco, R. A., et al. (2020). FeGenie: a comprehensive tool for the identification of iron genes and iron gene neighborhoods in genome and metagenome assemblies. Front. Microbiol. 11:37. doi: 10.3389/fmicb.2020.00037
Goris, J., Konstantinidis, K. T., Klappenbach, J. A., Coenye, T., Vandamme, P., and Tiedje, J. M. (2007). DNA-DNA hybridization values and their relationship to whole-genome sequence similarities. Int. J. Syst. Evol. Microbiol. 57, 81–91. doi: 10.1099/ijs.0.64483-0
Gregersen, T. (1978). Rapid method for distinction of gram-negative from gram-positive bacteria. Eur. J. Appl. Microbiol. Biotechnol. 5, 123–127. doi: 10.1007/BF00498806
Guo, Y., Aoyagi, T., and Hori, T. (2021). Comparative insights into genome signatures of ferric iron oxide- and anode-stimulated Desulfuromonas spp. strains. BMC Genomics 22:475. doi: 10.1186/s12864-021-07809-6
Hahn, M. W., Koll, U., Schmidt, J., Huymann, L. R., Karbon, G., and Lang, E. (2018). Polynucleobacter hirudinilacicola sp. nov. and Polynucleobacter campilacus sp. nov., both isolated from freshwater systems. Int. J. Syst. Evol. Microbiol. 68, 2593–2601. doi: 10.1099/ijsem.0.002880
Hügler, M., Wirsen, C. O., Fuchs, G., Taylor, C. D., and Sievert, S. M. (2005). Evidence for autotrophic CO2 fixation via the reductive tricarboxylic acid cycle by members of the epsilon subdivision of proteobacteria. J. Bacteriol. 187, 3020–3027. doi: 10.1128/JB.187.9.3020-3027.2005
Itoh, H., Xu, Z., Masuda, Y., Ushijima, N., Hayakawa, C., Shiratori, Y., et al. (2021). Geomonas silvestris sp. nov., Geomonas paludis sp. nov. and Geomonas limicola sp. nov., isolated from terrestrial environments, and emended description of the genus Geomonas. Int. J. Syst. Evol. Microbiol. 71:004607. doi: 10.1099/ijsem.0.004607
Kim, M., Oh, H. S., Park, S. C., and Chun, J. (2014). Towards a taxonomic coherence between average nucleotide identity and 16S rRNA gene sequence similarity for species demarcation of prokaryotes. Int. J. Syst. Evol. Microbiol. 64, 346–351. doi: 10.1099/ijs.0.059774-0
Kimura, M. (1980). A simple method for estimating evolutionary rates of base substitutions through comparative studies of nucleotide sequences. J. Mol. Evol. 16, 111–120. doi: 10.1007/bf01731581
Kovacs, N. (1956). Identification of Pseudomonas pyocyanea by the oxidase reaction. Nature 178:703. doi: 10.1038/178703a0
Kroppenstedt, R. M. (1982). Separation of Bacterial Menaquinones by HPLC Using Reverse Phase (RP18) and a Silver Loaded Ion Exchanger as Stationary Phases. J. Liq. Chromatogr. 5, 2359–2367. doi: 10.1080/01483918208067640
Kumar, S., Stecher, G., Li, M., Knyaz, C., and Tamura, K. (2018). MEGA X: molecular evolutionary genetics analysis across computing platforms. Mol. Biol. Evol. 35, 1547–1549. doi: 10.1093/molbev/msy096
Lagesen, K., Hallin, P., Rødland, E. A., Staerfeldt, H. H., Rognes, T., and Ussery, D. W. (2007). RNAmmer: consistent and rapid annotation of ribosomal RNA genes. Nucleic Acids Res. 35, 3100–3108. doi: 10.1093/nar/gkm160
Lee, T. K., Han, I., Kim, M. S., Seong, H. J., Kim, J. S., and Sul, W. J. (2019). Characterization of a nifH-harboring bacterial community in the soil-limited Gotjawal forest. Front. Microbiol. 10:1858. doi: 10.3389/fmicb.2019.01858
Lin, Y., Ye, G., Liu, D., Ledgard, S., Luo, J., Fan, J., et al. (2018). Long-term application of lime or pig manure rather than plant residues suppressed diazotroph abundance and diversity and altered community structure in an acidic Ultisol. Soil Biol. Biochem. 123, 218–228. doi: 10.1016/j.soilbio.2018.05.018
Lovley, D. R., Giovannoni, S. J., White, D. C., Champine, J. E., Phillips, E. J., Gorby, Y. A., et al. (1993). Geobacter metallireducens gen. nov. sp. nov., a microorganism capable of coupling the complete oxidation of organic compounds to the reduction of iron and other metals. Arch. Microbiol. 159, 336–344. doi: 10.1007/bf00290916
Lovley, D. R., and Walker, D. J. F. (2019). Geobacter protein nanowires. Front. Microbiol. 10:2078. doi: 10.3389/fmicb.2019.02078
Lowe, T. M., and Eddy, S. R. (1997). tRNAscan-SE: a program for improved detection of transfer RNA genes in genomic sequence. Nucleic Acids Res. 25, 955–964. doi: 10.1093/nar/25.5.955
Masuda, Y., Itoh, H., Shiratori, Y., Isobe, K., Otsuka, S., and Senoo, K. (2017). Predominant but previously-overlooked prokaryotic drivers of reductive nitrogen transformation in paddy soils, revealed by metatranscriptomics. Microbes Environ. 32, 180–183. doi: 10.1264/jsme2.ME16179
Meier-Kolthoff, J. P., Auch, A. F., Klenk, H. P., and Göker, M. (2013). Genome sequence-based species delimitation with confidence intervals and improved distance functions. BMC Bioinform. 14:60. doi: 10.1186/1471-2105-14-60
Nakajima, A., Aono, T., Tsukada, S., Siarot, L., Ogawa, T., and Oyaizu, H. (2012). Lon protease of Azorhizobium caulinodans ORS571 is required for suppression of reb gene expression. Appl. Environ. Microbiol. 78, 6251–6261. doi: 10.1128/aem.01039-12
Narsing Rao, M. P., Dong, Z. Y., Kan, Y., Dong, L., Li, S., Xiao, M., et al. (2020). Description of Paenibacillus tepidiphilus sp. nov., isolated from a tepid spring. Int. J. Syst. Evol. Microbiol. 70, 1977–1981. doi: 10.1099/ijsem.0.004004
Onley, J. R., Ahsan, S., Sanford, R. A., and Löffler, F. E. (2018). Denitrification by Anaeromyxobacter dehalogenans, a common soil bacterium lacking the nitrite reductase genes nirS and nirK. Appl. Environ. Microbiol. 84, e01985–17. doi: 10.1128/aem.01985-17
Parks, D. H., Imelfort, M., Skennerton, C. T., Hugenholtz, P., and Tyson, G. W. (2015). CheckM: assessing the quality of microbial genomes recovered from isolates, single cells, and metagenomes. Genome Res. 25, 1043–1055. doi: 10.1101/gr.186072.114
Poly, F., Ranjard, L., Nazaret, S., Gourbière, F., and Monrozier, L. J. (2001). Comparison of nifH gene pools in soils and soil microenvironments with contrasting properties. Appl. Environ. Microbiol. 67, 2255–2262. doi: 10.1128/AEM.67.5.2255-2262.2001
Postgate, J. R. (1972). “Chapter XIII The acetylene reduction test for nitrogen fixation,” in Methods in Microbiology, eds J. R. Norris and D. W. Ribbons (Cambridge: Academic Press), 343–356. doi: 10.1016/s0580-9517(08)70604-4
Price, M. N., Dehal, P. S., and Arkin, A. P. (2009). FastTree: computing large minimum evolution trees with profiles instead of a distance matrix. Mol. Biol. Evol. 26, 1641–1650. doi: 10.1093/molbev/msp077
Qin, J., Hu, Y., Wu, W., Feng, Y., and Zong, Z. (2020). Pseudomonas defluvii sp. nov., isolated from hospital sewage. Int. J. Syst. Evol. Microbiol. 70, 4199–4203. doi: 10.1099/ijsem.0.004272
Richter, M., and Rosselló-Móra, R. (2009). Shifting the genomic gold standard for the prokaryotic species definition. Proc. Natl. Acad. Sci. U. S. A. 106, 19126–19131. doi: 10.1073/pnas.0906412106
Saitou, N., and Nei, M. (1987). The neighbor-joining method: a new method for reconstructing phylogenetic trees. Mol. Biol. Evol. 4, 406–425. doi: 10.1093/oxfordjournals.molbev.a040454
Sasser, M. (2001). Identification of Bacteria by Gas Chromatography of Cellular Fatty Acids Technical Note # 101. Newark: Microbial ID, Inc.
Shi, L., Rosso, K. M., Clarke, T. A., Richardson, D. J., Zachara, J. M., and Fredrickson, J. K. (2012). Molecular underpinnings of Fe(III) oxide reduction by Shewanella Oneidensis MR-1. Front. Microbiol. 3:50. doi: 10.3389/fmicb.2012.00050
Soumare, A., Diedhiou, A. G., Thuita, M., Hafidi, M., Ouhdouch, Y., Gopalakrishnan, S., et al. (2020). Exploiting biological nitrogen fixation: a route towards a sustainable agriculture. Plants (Basel) 9:1011. doi: 10.3390/plants9081011
Tohno, M., Tanizawa, Y., Irisawa, T., Masuda, T., Sakamoto, M., Arita, M., et al. (2017). Lactobacillus silagincola sp. nov. and Lactobacillus pentosiphilus sp. nov., isolated from silage. Int. J. Syst. Evol. Microbiol. 67, 3639–3644. doi: 10.1099/ijsem.0.002196
Wang, C. Y., Zhou, X., Guo, D., Zhao, J. H., Yan, L., Feng, G. Z., et al. (2019). Soil pH is the primary factor driving the distribution and function of microorganisms in farmland soils in northeastern China. Ann. Microbiol. 69, 1461–1473. doi: 10.1007/s13213-019-01529-9
Wick, R. R., Judd, L. M., Gorrie, C. L., and Holt, K. E. (2017). Unicycler: resolving bacterial genome assemblies from short and long sequencing reads. PLoS Comput. Biol. 13:e1005595. doi: 10.1371/journal.pcbi.1005595
Xu, Z., Masuda, Y., Itoh, H., Ushijima, N., Shiratori, Y., and Senoo, K. (2019). Geomonas oryzae gen. nov., sp. nov., Geomonas edaphica sp. nov., Geomonas ferrireducens sp. nov., Geomonas terrae sp. nov., four ferric-reducing bacteria isolated from paddy soil, and reclassification of three species of the genus Geobacter as members of the genus Geomonas gen. nov. Front. Microbiol. 10:2201. doi: 10.3389/fmicb.2019.02201
Yarza, P., Yilmaz, P., Pruesse, E., Glöckner, F. O., Ludwig, W., Schleifer, K. H., et al. (2014). Uniting the classification of cultured and uncultured bacteria and archaea using 16S rRNA gene sequences. Nat. Rev. Microbiol. 12, 635–645. doi: 10.1038/nrmicro3330
Yoon, S. H., Ha, S. M., Kwon, S., Lim, J., Kim, Y., Seo, H., et al. (2017a). Introducing EzBioCloud: a taxonomically united database of 16S rRNA gene sequences and whole-genome assemblies. Int. J. Syst. Evol. Microbiol. 67, 1613–1617. doi: 10.1099/ijsem.0.001755
Keywords: Geomonas oryzisoli, Geomonas subterranea, Geomonas nitrogeniifigens, nitrogen fixation activity, iron reduction, paddy soil, genome analysis
Citation: Liu G-H, Yang S, Tang R, Xie C-J and Zhou S-G (2022) Genome Analysis and Description of Three Novel Diazotrophs Geomonas Species Isolated From Paddy Soils. Front. Microbiol. 12:801462. doi: 10.3389/fmicb.2021.801462
Received: 25 October 2021; Accepted: 14 December 2021;
Published: 07 February 2022.
Edited by:
Yurong Liu, Huazhong Agricultural University, ChinaReviewed by:
Hilal Ay, Ondokuz Mayıs University, TurkeyDao-Feng Zhang, Hohai University, China
Yong Yuan, Guangdong University of Technology, China
Copyright © 2022 Liu, Yang, Tang, Xie and Zhou. This is an open-access article distributed under the terms of the Creative Commons Attribution License (CC BY). The use, distribution or reproduction in other forums is permitted, provided the original author(s) and the copyright owner(s) are credited and that the original publication in this journal is cited, in accordance with accepted academic practice. No use, distribution or reproduction is permitted which does not comply with these terms.
*Correspondence: Shun-Gui Zhou, sgzhou@fafu.edu.cn