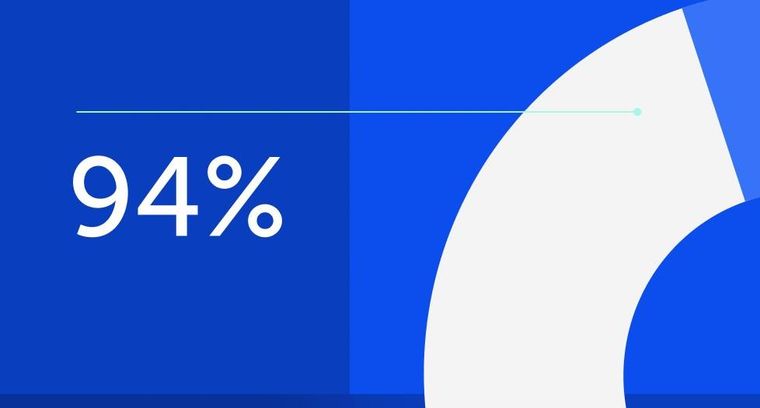
94% of researchers rate our articles as excellent or good
Learn more about the work of our research integrity team to safeguard the quality of each article we publish.
Find out more
ORIGINAL RESEARCH article
Front. Microbiol., 06 January 2022
Sec. Microbial Immunology
Volume 12 - 2021 | https://doi.org/10.3389/fmicb.2021.800807
This article is part of the Research TopicEmerging roles of extracellular vesicles in immunomodulation during host-pathogen interactionsView all 5 articles
Although mycobacterial proteins in exosomes from peripheral serum of patients with tuberculosis (TB) have been identified, other exact compositions of exosomes remain unknown. In the present study, a comprehensive proteomics analysis of serum exosomes derived from patients with active TB (ATB) was performed. Exosomes from patients with ATB were characterized using nanoparticle tracking analysis (NTA), transmission electron microscopy (TEM), and western blotting analysis. Then identified protein components were quantified by label-free proteomics and were determined via bioinformatics analysis. A total of 123 differential proteins were identified in ATB serum exosomes and analyzed with Gene Ontology (GO) analysis. Among these proteins heat shock protein70 (HSP70), CD81, major histocompatibility complex-I (MHC-I ) and tumor susceptibility gene101 (TSG101) were present in exosomes of ATB and normal individuals confirmed via western blotting. In addition, among identified exosomal proteins lipopolysaccharide binding protein (LBP) increased significantly, but CD36 and MHC-I decreased significantly in ATB exosomes. Meanwhile, MHC-I was down-expressed in serum and peripheral blood mononuclear cells (PBMCs) of ATB, but interestingly CD36 was down-regulated in serum and up-expressed in PBMCs of ATB patients validated with ELISA and flow cytometry. CD36 was up-regulated by M. tuberculosis H37Ra infection in macrophages and suppressed in exosomes from H37Ra infected macrophages detected by western blotting. This study provided a comprehensive description of the exosome proteome in the serum of patients with ATB and revealed certain potential biomarkers associated with TB infection.
Mycobacterium tuberculosis (M. tuberculosis), the pathogen of tuberculosis (TB), remains an important public health problem around world (Biadglegne et al., 2021). According to a World Health Organization (WHO) report of 2021, there are about 5.8 million new TB cases globally, and nearly 1.5 million patients died from related diseases caused by TB (World Health Organization, 2021). In the past 10 years, a new generation of tools, including genome sequencing and proteomics, have been developed to help us to further understand the disease progression of pathogens in host cells after being processed by the immune system (Sukumar et al., 2014; Cadena et al., 2016). The main techniques for diagnosing active TB are direct microscopic demonstration, culture and polymerase chain reaction (PCR) (Suárez et al., 2019). These traditional diagnostic methods are complicated and time-consuming, which hinders the rapid diagnosis of tuberculosis. Therefore, it is particularly urgent to find diagnostic markers for rapid diagnosis of tuberculosis (Goletti et al., 2016).
Exosomes are 30–150-nm extracellular membrane vesicles and may be a promising research target for the diagnosis and therapy in the infection of tuberculosis, since they are derived from hematopoietic and non-hematopoietic cells and involved in intercellular communication (Wang et al., 2018). Exosomes have important functions in signal transduction, immunomodulation (Alipoor et al., 2016) and the material transportation including lipids, nucleic acids, proteins, and other biochemical substances (Théry, 2011), and they also act as cellular “trash bags” to remove excess intracellular substances (Subramanian et al., 2016), and promote intercellular communication by transporting various molecules from donor cells to recipient cells (Zhang et al., 2018).
Exosomes derived from M. tuberculosis infected host cells such as macrophages, nature killer cells trigger different immune responses, such as inflammatory response, antigen presentation, and activate subsequent pathways, highlighting the critical function of exosomes in anti- M. tuberculosis immune response (Sun et al., 2021). Exosomes from M. avium-infected macrophages induced naïve macrophages to generate proinflammatory factors, such as tumor necrosis factor α (TNFα), inducible nitric oxide synthase (iNOS) and regulated the activation of T cells (Bhatnagar and Schorey, 2007; Bhatnagar et al., 2007). Giri et al. (2010) demonstrated that exosomes derived from M. avium infected macrophages contain bacterial pathogenic glycopeptidolipids. Subsequently, various exosome proteomics studies have revealed that exosomes could carry numerous antigens of M. tuberculosis. For example, Kruh-Garcia et al. (2014) revealed 41 M. tuberculosis proteins within exosomes from macrophages infected by M. tuberculosis or M. tuberculosis culture filtrate via analysis proteomics. In addition, 20 M. tuberculosis proteins in exosomes from the serum of TB patients, such as antigens 85b, Mpt64, GlcB, and BfrB, have been identified (Singh et al., 2011). This evidence suggests that exosomes regulate the immune functions of recipient cells by carrying mycobacterial constituents. These literatures provide valuable information on exosomes in the M. tuberculosis infectious process and provide insights into the development of potential exosomal biomarkers for TB diagnosis.
In this study, systematic comparative proteomics profiling analysis of serum exosomes of normal individuals and patients with active TB was performed. Our results revealed different exosomal protein expression panels and indicated that proteins are selectively packaged into exosomes under different physiological conditions. Pathway and functional analyses further indicated the functions of differential proteins in samples from healthy individuals and patients with ATB. These results provide significant evidences on the functions of exosomal proteins during the M. tuberculosis infectious process and indicate potential exosomal biomarkers for the diagnosis of TB.
In the present study, human blood samples of normal individuals and patients with ATB were collected in the Clinical Laboratory of Affiliated Kunshan Hospital of Jiangsu University from January 2020 to July 2021. Blood (5 mL/donors) was collected into tubes with heparin anticoagulant and centrifugated at 2,000 × g for 10 min to obtain serum the serum was stored at –80°C for further analysis. Blood (5 mL/donors) in tubes with EDTA anticoagulant was centrifugated at 2,000 × g, for 10 min through Ficoll Hypaque (5 mL, Thermo Fisher Scientific, United States) density gradient centrifugation, then mononuclear cells that retaining the interface between plasma and stratification fluid were acquired. Total 55 ATB patients were diagnosed according to combined clinical criteria from the WHO (World Health Organization et al., 2001). A total of 45 non-M. tuberculosis infected individuals with the results of negative tuberculin skin tests were included in the normal group as negative control (NC). There was no significant differences in age, sex ratio, body mass index (BMI), and smoking history among ATB patients and NC groups (P > 0.05) (Table 1).
This study was ethically approved by the Ethics Committee of Affiliated Kunshan Hospital of Jiangsu University (Suzhou, China, approval no. IEC-C-007-A07-V3.0). The experiments were performed in accordance with the Declaration of Helsinki Principles, and all ATB patients and normal individuals provided their written informed consent to participate in the present study.
Inactivated M. tuberculosis H37Ra was provided by the Center for Chinese Disease Prevention and Control (Beijing, China). THP-1 cells were purchased from The Cell Bank of Type Culture Collection of Chinese Academy of Sciencess (Shanghai, China). THP-1 cells were cultured in wells or flasks with RPMI-1640 containing 10% exosome-depleted FBS, 100 U/mL penicillin and 0.1 mg/mL streptomycin at 37°C with 5% CO2. THP-1 cells were induced into macrophages using Phorbol 12-myristate 13-acetate (0.1 mM) for 24 h.
Macrophages were infected with H37Ra at a multiplicity of infection of H37Ra: cell = 100:1 for 24 h. Subsequently, the infected macrophages were washed with PBS three times for next experiments.
Small interfering RNA (siRNA) of CD36 (50 nM; Shanghai GenePharma Co., Ltd., Shanghai, China) was transfected into macrophages grown in 6-well plates at 75% confluence using Lipofectamine 3000® reagent (Invitrogen; Thermo Fisher Scientific, Inc., Waltham, MA, United States) at 37°C for 24 h according to the manufacturer’s protocols. CD36-siRNA (50 pM) was added into serum-free diluent and mixed to siRNA diluent (25 μL). Lipofectamine 3000® reagent (1 μL) was added into serum-free diluent (24 μL) and mixed to Lipofectamine 3,000 diluent at room temperature for 5 min. Then, Lipofectamine 3,000 diluent and RNA diluent were mixed together at room temperature for 15 min to form the transfection complex. The transfection complex (50 μL) was added into 2 × 105 macrophages with whole medium (0.45 mL) and mixed gently, and transfection cells were cultured for 24 h after transfection. The CD36 siRNA sequence was 5′-GGAAUCCCUGUGUAUAGAUTTAUCUAUACACAGGGAU UCCTT-3′ and the scrambled siRNA sequence was 5′-UUCU CCGAACGUGUCACGUTTACGUGACACGUUCGGAGAA TT-3′.
Exosomes from serum and cell culture supernatants were isolated with 0.9% sucrose solution via differential centrifugation. Briefly, all serum and cell supernatants were centrifuged at 300 × g for 10 min to remove cells, 3,000 × g for 15 min to remove cell debris, 10,000 × g for 60 min to remove microvesicles and 100,000 × g for 2 h to acquired exosomes, every steps followed by two washes with PBS buffer and all performed at 4°C. The purified exosomes were identified by with NTA, TEM, and western blotting. The total protein concentration of exosomes was measured with BCA Assay (Thermo Fisher Scientific, Inc., Waltham, MA, United States). Before the experiment, all isolated exosomes were stored at −80°C.
The isolated exosomes were suspended in PBS solution (100 μL). After fully shaking and mixing, exosomes (10 μL) were diluted with ultrapure water to 1 mL, and injected into the Nano-sight S300 nano-particle size analyzer. The particle size distribution and concentration were measured according to the standard process at 4°C. Each exosomes sample was analyzed three times using Nano-sight NTA (V3.2) software.
Exosomes were re-suspended in PBS (100 μL). A total of exosome diluted solution (10 μL) was dropped on the copper wire at 25°C for 5 min, and washed with ultrapure water three times. After air drying, 10 μL phosphotungstic acid solution was added on copper mesh coloring for 2 min for air drying at room temperature. Test samples were analyzed with Hitachi H-600 transmission electron microscope (Hitachi, Ltd., Tokyo, Japan) to observe the morphology of exosomes.
Exosomes (30 μg) were added to beads (10 μL) and attached to aldehyde/sulfate latex beads (Invitrogen; Thermo Fisher Scientific, Inc., United States) at 25°C for 15 min. Subsequently, the aforementioned treated exosomes were diluted with PBS and incubated at 4°C overnight. Exosome-bound beads were washed with PBS containing 0.5% bovine serum albumin (BSA) three times. After centrifugation, the bead pellet was resuspended in PBS (0.5 mL) containing 0.5% BSA. Coated beads were incubated with CD9, CD63, and CD81 antibodies (Biolegend, Inc., San Diego, CA, United States) at 4°C for 30 min.
Fresh peripheral blood (100 μL) from health individuals and ATB patients was incubated with MHC-I, CD14, CD36, and CD69 antibodies (respectively, 5 μL) (Biolegend, Inc., San Diego, CA, United States) for 15 min. After that, red blood cell lysate was added and cell precipitation was obtained by 300 × g centrifugation, and resuspend with PBS (500 μL) for next step. Analysis were performed on a BD FACSCanto™ II flow cytometer (Becton, Dickinson and Company, United States). Fluorescence intensity values were obtained, and mean fluorescence intensities were used for statistical analysis.
In the present study, the exosome samples were divided into two groups: One group comprising exosomes purified from the serum of normal individuals and another group comprising exosomes purified from the serum of patients with ATB, with three replicates per group.
Exosomes were extracted with SDT buffer (4% SDS, 100 mM Tris-HCl, 1 mM DTT, pH 7.6), and the protein content was monitored with a BCA assay kit (Bio-Rad, United States). Then the exosomal proteins were digested by trypsin as per the filter-assisted sample preparation from Matthias Mann. The resulting peptides were desalted on Empore SPE Cartridges C18 (standard density, bed I.D. 7 mm, volume 3 mL, Sigma), vacuum-centrifuged and reconstructed in 40 μL of 0.1% (v/v) formic acid. Proteins (200 μg) were loaded into 30 μL of another SDT buffer (4% SDS, 100 mM DTT, 150 mM Tris-HCl pH 8.0). The detergent DTT and other low-molecular-weight substances were repeatedly ultrafiltered with a UA buffer (8 M Urea, 150 mM Tris-HCl, pH 8.0) (Microcon units, 10 kD). Then the reduced cysteine residues were blocked by adding 100 μL of iodoacetamide (100 mM IAA in the UA buffer) and cultured for 30 min in the dark. The filters were cleaned first with 100 μL of the UA buffer three times and then with 100 μL of 25 mM NH4HCO3 twice. Finally, the protein suspensions were digested with 4 μg of trypsin (Promega Corporation, United States) in 40 μL of the NH4HCO3 buffer at 37°C overnight, and the final peptides were harvested as a filtrate.
The UV spectral density at 280 nm of the peptides were measured using an extinction coefficient of 1.1 of a 0.1% (g/l) solution that was computed as per the frequency of tryptophan and tyrosine in vertebrate proteins.
LC-MS/MS was run on a Q-Exactive MS meter (Thermo Fisher Scientific, United States) equipped with Easy nLC (Thermo Fisher Scientific, United States) for 120 min. The peptides were placed onto a PepMap100 reversed trap column (Thermo Fisher Scientific Acclaim, 100 μm*2 cm, nanoViper C18) linked to a C18-reversed analytical column (Thermo Fisher Scientific Easy Column, 10 cm long, 75 μm I.D., 3 μm resin) in buffer A (0.1% Formic acid) and isolated with a linear gradient of buffer B (84% acetonitrile, 0.1% Formic acid) at a flow speed of 300 nl/min managed by IntelliFlow. MS data in the positive ion mode were collected using a data-based top10 approach to dynamically select the richest precursor ions from the scan range of 300–1,800 m/z for HCD decomposition. Maximum injection time, automatic gain control target and dynamic exclusion duration were set at 10 ms, 3e6 and 40.0 s, respectively. Survey scanning resolution, HCD spectral resolution, and isolation width were 70,000 at m/z 200, 17,500 at m/z 200, and 2 m/z, respectively. Normalized collision energy was 30 eV, and the under fill ratio, referring to the lowest percent of the target value to be met at longest fill time, was 0.1%. The peptide recognition mode was chosen here.
The raw MS data for each sample were integrated and searched on MaxQuant 1.5.3.17 for identification and quantification.
Hierarchical clustering analysis was performed by Cluster 3.0.1 In addition to tree diagrams, heat maps and volcano plot are usually presented as visual aids. The subcellular localization of exosomal proteins was predicted with CELLO subcellular localization predictive system.2
Based on the online Kyoto Encyclopedia of Genes and Genomes (KEGG) database3 (Huang et al., 2009), we conducted a blast of differential exosomal proteins to retrieve their KEGG orthology identification, and then mapped to the path in KEGG.
The enrichment analysis is based on Fisher’s exact test, using the entire quantitative protein as the background data set. A P-value < 0.05 was set to distinguish statistically significant enrichment results. The functional interactions between proteins can be plotted to illustrate the molecular mechanisms and signaling pathways of cellular processing. The protein–protein interaction (PPI) of exosomal proteins was analyzed with Retrieval of Interacting Genes (STRING)4 database (Szklarczyk et al., 2015), and subsequently was visualized using Cytoscape5 (Shannon et al., 2003).
MHC-I, CD36, and lipopolysaccharide binding protein (LBP) levels in the serum of 40 normal individuals and 40 patients with ATB were measured by using ELISA. After the serum was treated by ultrasound to broke serum exosomes with intermittent ultrasonic treatment, the concentrations of MHC-I, CD36, and LBP in serum were examined according to the reagent operating instructions (Invitrogen; Thermo Fisher Scientific, Inc., Waltham, MA, United States).
Briefly, Standards or serum samples (100 μL) were added in appropriate wells incubated for 0.5 h at 37°C, and then wells were washed 3 times with wash solution. Biotinylated antibodies (100 μL) of MHC-I, CD36, and LBP were added to each well incubated for 1 h at 37°C, were washed 3 times with wash solution. Streptavidin solution (100 μL) was added in each well incubated for 45 min at 37°C and then wells were washed 3 times with wash solution. MB substrate reagent (100 μL) was added to each well incubated for 30 min at 37°C in the dark and finally, stop solution (50 μL) was added to each well. Absorbance was detected at 450 nm using a Spectra Max 190 ELISA reader (Molecular Devices, LLC, Sunnyvale, CA, United States) and the concentrations were quantified against the standard curve.
Total proteins were isolated from fresh macrophages cells (5.5 × 106) or frozen exosomes (100 μg) were lysed using RIPA peptide lysis buffer (Beyotime Institute of Biotechnology, Haimen, China). Proteins (30 μg) were loaded on 10% SDS-PAGE gels, electrophoresed, and then transferred to PVDF membranes (MilliporeSigma, Burlington, MA, United States). Subsequently, the membranes were incubated with primary antibodies against CD81 (at 1:2,000 dilution, ProteinTech Group, Inc., Chicago, United States), HSP70 (at 1:3,400 dilution, Biolegend, Inc., San Diego, CA, United States), LBP (at 1:2,400 dilution, ProteinTech Group, Inc.), CD36 (at 1:2,000 dilution, Biolegend, Inc.), MHC-I (at 1:3,000 dilution, Biolegend, Inc.) and TSG101 (at 1:2,000 dilution, ProteinTech Group, Inc.) at 4°C overnight. Then, PVDF membranes were washed three times with TBS-T under shaking. Finally, the membranes were incubated with secondary antibody solution (mouse anti-rabbit IgG-HRP at a 1:10,000) for 60 min at room temperature with gentle shaking, and washed with TBS-T under shaking three times again. Protein bands were imaged and analyzed using the Chemiluminescent Substrate System (Thermo Fisher Scientific, Inc., Waltham, MA, United States).
All data in this study are presented as the mean ± standard error of the mean. Statistical analysis was performed with GraphPad Prism 5 (GraphPad Software Inc., San Diego, CA, United States). Differences among groups were analyzed using one-way ANOVA or a paired Student’s t-test. The statistical results are considered to be statistically significant differences (P < 0.05).
The exosomes were typical cup-shaped vesicles as observed by TEM, and no differences in shape between the exosomes from the serum of normal individuals and patients with ATB were observed (Figure 1A). NTA analysis revealed that the particle size distribution was within 30–150 nm. Furthermore, the diameter of most particles was 150 nm, indicating that these particles were exosomes (Figure 1B). Western blot analysis further confirmed the presence of surface markers of exosomes, such as HSP-70, CD81, and TSG101 (Figures 1C,D). The flow cytometry results suggested that the exosomes were positive for CD9, CD63, and CD81, and negative for immunoglobulin G (Figure 1E). In conclusion, pure exosomes were obtained by differential ultracentrifugation, which laid the foundation for subsequent proteomics (Figure 1F).
Figure 1. Biological characterization of exosomes isolated from serum. (A) Transmission electron micrographs of isolated exosomes from the serum of normal individuals and patients with ATB. Representative image of exosomes at a magnification of × 200. (B) Size distribution of exosomes was determined by using NTA. Particle size (150 nm) and concentrations (2.01E10 particles/mL). (C,D) HSP70 and CD81 in exosomes from normal individuals (N#1, N#12, and N #33) and patients with ATB (T#3, T#22, and T #36) were quantified via western blotting. TSG1-101 was used as a control. (E) Exosomal marker proteins CD9, CD63, and CD81 on exosomes were detected by using flow cytometry. (F) Schematic diagram of separation and proteomic analysis of serum exosomes.
To determine the exosomes protein profile in the serum of patients with ATB, total exosomal proteins were identified through label-free quantitative proteomics analysis by Applied Protein Technology Co., Ltd. Protein database searching of tandem mass spectrometry (MS/MS) data resulted in the identification of 886 exosomal proteins in the serum of patients with ATB and 869 exosomal proteins in the serum of normal volunteers. Furthermore, 480 exosomal proteins were identified in both experimental groups, as shown in the Venn diagram (Figure 2A). Bioinformatics analysis of proteomics shows that there were 123 differentially expressed proteins including 40 up-regulated proteins and 83 down-regulated proteins were indicated by histogram, cluster analysis (Figure 2B), heat map (Figure 2C), and volcano plot (Figure 2D). Furthermore, 13 up-expressed and 30 down-expressed proteins are listed in Tables 2, 3, respectively. Interestingly, LBP, CD36, and MHC-I molecules were confirmed by western blotting (Figures 2E,F).
Figure 2. Bioinformatics analysis of exosome differential proteins. (A) Venn diagram illustrating overlapping proteins in the two independent MS experiments (T vs. N). (B) Histogram of quantitative difference results of two groups of MS experimental proteins, including 40 up-regulated proteins and 83 down-regulated proteins. (C) Heat map of the protein levels of shared proteins among exosomes released from the serum of normal individuals and patients with ATB. (D) Volcano diagram of differential expression of exosome proteins between the two groups (T vs. N). (E,F) Differential proteins (LBP, CD36, and MHC-I ) in exosomes of the two groups (T vs. N) were quantified via western blotting. *p < 0.05, ***p < 0.001.
Table 2. List of 13 up-regulated proteins with significant interesting in exosomes from ATB patients.
Table 3. List of 30 down-expressed proteins with significant interesting in exosomes from ATB patients.
Subcellular localization analysis of exosomes differential proteins revealed that the identified proteins were enriched in the extracellular region (103 proteins), cytoplasm region (105 proteins), nuclear region (63 proteins), membrane region (22 proteins), mitochondrial region (22 proteins), and 11 other proteins (Figure 3A).
Figure 3. Functional analysis of exosomes differential proteins. (A) Subcellular localization pie chart of differentially expressed proteins in two groups of exosomes (T vs. N). (B) GO enrichment map of exosomal differential proteins, including BP, MF, and CC. (C) Bubble diagram of KEGG pathway enrichment of exosomal differential proteins. (D) Interaction network of exosomes differentially expressed proteins.
The Gene Ontology (GO) analysis grouped the results according to biological processes (BP), cellular components (CC), and molecular functions (MF). In terms of biological processes, exosome proteins were mainly involved in cellular process, biological regulation or process, response stimulus, and other functions (Figure 3B). As for molecular functions, exosomal proteins were enriched in molecule with molecular binding and catalytic activities (Figure 3B). In terms of the cellular components, exosomal proteins were enriched in cell, intracellular and cytoplasm region (Figure 3B). The KEGG pathway analysis revealed that exosomal proteins were enriched in focal adhesion, platelet activation, viral carcinogenesis etc. (Figure 3C). Based on intact database, the network diagram of differential protein interaction in exosomes was obtained using Cytoscape software, and the interaction relationship of differential proteins were clearly displayed (Figure 3D).
The analyses indicated that these identified exosomal proteins act as a tool for exosomes research and emphasize the need for further experimental evaluation of the functions of the identified exosomal proteins in physiology and pathophysiology of patients with ATB.
The differential exosomal proteins MHC-I, CD36, and LBP in the serum were confirmed in normal individuals and patients with ATB by using ELISA. The expression levels of MHC-I and CD36 were decreased, but LBP expression was markedly increased in patients with ATB compared with normal controls (Figure 4A). In addition, the diagnostic performances of these three protein were analyzed using receiver operating characteristic (ROC) curves (Figure 4B). The area under the curve values of MHC-I, CD36, and LBP were 62.44, 60.47, and 67.69%, respectively, revealing that MHC-I, CD36, and LBP may be potential biomarkers for the diagnosis of ATB infection.
Figure 4. MHC-I, CD36, and LBP expression in the serum of normal individuals and patients with ATB detected using ELISA kits. (A) Concentrations of MHC-I, CD36, and LBP in the serum were analyzed. (B) MHC-I, CD36, and LBP in the serum to distinguish patients with ATB infection from healthy controls. The AUC values of MHC-I, CD36, and LBP were 62.44, 60.47, and 67.69%, indicating that they can be used to distinguish patients with ATB infection from healthy controls (ROC, receiver operating characteristic). *p < 0.05, **p < 0.01.
MHC-I, CD14, CD36, and CD69 expression on PBMCs of 40 healthy individuals and 55 patients with TB was examined via flow cytometry (Figures 5A,B). The experimental results demonstrated that MHC-I and CD14 were markedly down-regulated, however, CD36 and CD69 were markedly up-regulated on PBMCs of patients with ATB compared with healthy individuals (Figure 5C). Interestingly, these results of the expression levels of MHC-I and CD36 were consistent with the results of exosome proteomics and ELISA for serum analyses.
Figure 5. MHC-I, CD36, CD69, and CD14 expression in the PBMCs of normal individuals and patients with ATB. (A,B) Scatter diagram and three dimensional results of MHC-I, CD36, CD69, and CD14 expressions in PBMCs of normal individual-32# and ATB patient-40# were detected by flow cytometry and analyzed using flowjo10 software. (C) Scatter statistical results for MHC-I, CD36, CD69, and CD14 in 40 normal individuals and 55 ATB patients were quantified using GraphPad Prism 5 software. *p < 0.05, ***p < 0.001.
In view of the expression levels of CD36 on exosomes proteomics, serum ELISA and peripheral blood mononuclear cells, CD36 was selected as the target of our in-depth study. To explore the effect of H37Ra infection on CD36 in macrophages, CD36 was knocked down in macrophages. CD36 protein expression was markedly decreased on treated macrophages and exosomes derived from these compared with the expression levels in the NC or mock groups (Figures 6A,B). Additionally, H37Ra infection promoted CD36 expression on macrophages (Figures 6C,D) but CD36 expression was down-regulated on exosomes from normal macrophages and siRNA-transfected macrophages (Figures 6E,F). These findings revealed that H37Ra infection regulated CD36 expression and transmission via exosomes.
Figure 6. CD36 expression in H37Ra-infected macrophages or exosomes determined by western blotting. (A) CD36 in macrophages was knocked down using siRNA. (B) CD36 expression was analyzed in exosomes derived from CD36-knockdown macrophages. (C,D) CD36 expression was promoted in H37Ra-infected macrophages and CD36-knockdown macrophages. (E,F) H37Ra infection suppressed the expression levels of CD36 in macrophages and CD36-knockdown macrophages. *p < 0.05, **p < 0.01, ***p < 0.001.
Accumulating studies have reported that a number of mycobacterial proteins in exosomes have been identified using MS (Dobos et al., 2011; Wang et al., 2019). Furthermore, these exosomal proteins are immunogenic and function in intracellular communication, for example, by stimulating the T cell immune response (Giri and Schorey, 2008; Singh et al., 2011). Interestingly, several markers, including HSP70 and tetraspanins (CD9, CD63, and CD81), are conserved on exosomes derived from various cells (Anand et al., 2010; Wang et al., 2014; Kowal et al., 2017). These specific differentially expressed exosome molecules are potential molecular markers for the diagnosis of M. tuberculosis infection. In the present study, exosomes isolated from the serum of patients with ATB and healthy individuals were round and 30–150 nm in diameter. The characteristic proteins CD9, CD63, CD81, HSP70, and TSG-101 were all expressed on exosomes from ATB patients. According to the size and marker molecule expression of these vesicles, our experiments have obtained high-purity exosomes, which are essential for downstream proteomics analysis.
An inspection of the proteome dataset for exosomes derived from the serum of patients with ATB and healthy individuals by liquid chromatography with MS/MS revealed the presence of various proteins common to exosomes from various origins, including HSPs, integrins, cytoskeletal proteins, and enzymes, which may be associated with functions of structure, biogenesis, and trafficking of exosomes. 480 overlapping exosomal proteins from the serum of normal volunteers and patients with ATB were revealed in the Venn diagram by comparing the gene symbols of the identified exosomal proteins from the two exosome groups using ExoCarta. However, there were 123 proteins with significant differences, including 40 up-regulated proteins (such as: complement component C9, carbonic anhydrase, aminopeptidase, lipopolysaccharide-binding protein, transferrin receptor protein 1, alpha-1-acid glycoprotein 2, etc.) and 83 down-regulated proteins (for example: glycoprotein IIIb, MHC class I antigen, profilin-1, junctional adhesion molecule 1, cofilin, actin-related protein 2, etc.). These differential exosomal proteins were revealed by the quantitative difference histogram, the cluster analysis heat map and the volcanic map. At the same time, we also found that there are 115 no differentially expressed exosomal proteins (for instance: afamin, alpha-2-HS-glycoprotein, vitamin D-binding protein, catalase, etc.) in the serum of ATB patients.
To obtain systematic insights into the proteome profiles of exosomes, the present study examined the differentially expressed proteins by using bioinformatics analysis, including GO, KEGG, and protein-protein interaction analysis. The most enriched pathways were cellular process and biological regulation. In addition, among these differential proteins, LBP, CD36, and MHC-I attracted our interest and these three molecules are closely related to the infection of tuberculosis, especially CD36. Recent report show that CD36 elevated in exosomes derived from bladder cancer cells by proteomics analysis (Welton et al., 2010). So they were selected to be as a research target and confirmed further by western blotting. The results demonstrated that LBP expression was increased and the expression levels of CD36 and MHC-I were decreased in exosomes from the serum of patients with ATB. In addition, LBP was increased, but CD36 and MHC-I were decreased in the serum of ATB patients determined by ELISA analysis. At the same time, the ROC analysis of LBP, CD36, and MHC-I indicated that they possessed the value of diagnostic biomarker for ATB.
LBP is important for the recognition of lipopolysaccharides by the host and thereby for the induction of an adequate innate immune response to Gram-negative bacteria (Liu et al., 2021). However, Branger et al. (2005) reported that endogenous LBP is not important for host defense against virulent M. tuberculosis or avirulent M. smegmatis. Furthermore, it was revealed that LBP is markedly up-regulated in the serum of patients with TB. Although there is almost no literature regarding LBP in M. tuberculosis infection, its abnormal change in the serum of patients with ATB suggests that it may be associated with the infection of M. tuberculosis, but its roles in the infection process has not yet been discovered.
MHC-I-restricted cytotoxic T lymphocytes are known to serve an important role in the control of M. tuberculosis infection (Wang et al., 2011). At the same time, MHC-I could present antigens of M. bovis BCG, which are associated with the biosynthesis and transport of lipids and are located at the cell membrane (Bettencourt et al., 2020). In the present study, it was revealed that MHC-I expression was markedly down-regulated in the serum and PBMCs of patients with TB. Low expression levels of MHC-I in vivo lead to weakening of the presentation ability of TB antigens and ineffective resistance to infection with M. tuberculosis.
Three cell surface molecules (CD14, CD69, and CD36) closely associated with ATB infection were also investigated. Our study indicated that the expression levels of CD14 were down-regulated, but the expression levels of CD69 and CD36 were enhanced in PBMCs of patients with ATB. CD36 is a pattern recognition receptor expressed on macrophages and could recognize M. tuberculosis (Silverstein and Febbraio, 2009; Lao et al., 2017). It has been demonstrated that macrophages deficient in CD36 restrict the growth of multiple mycobacterial species in vitro, and CD36 deficiency confers resistance to mycobacterial infection in mice (Hawkes et al., 2010). Surprisingly, the present results revealed that CD36 was down-regulated in the serum of patients with ATB but was up-regulated in PBMCs. It is not clear why these opposite results for CD36 expression in the serum and PBMCs were observed. CD36 mediates surfactant lipid uptake of macrophages in vitro, and this increases the growth of M. tuberculosis (Castaño et al., 2011). Based on previous studies investigating the roles of CD36 in M. tuberculosis infection and our experimental results, it was hypothesized that the up-regulation of CD36 in PBMCs is closely related to M. tuberculosis infection in patients with ATB. Nevertheless, low CD36 expression in the serum may promote the infection of M. tuberculosis further in vivo. This expression pattern of CD36 may be the common result of M. tuberculosis infection and resistance to M. tuberculosis infection.
M. tuberculosis infected macrophages from immunologically naïve guinea pigs increased the expression of CD36 in vivo (Palanisamy et al., 2012), and CD36 is increased in macrophages and mice infected by M. tuberculosis (Il’in and Shkurupy, 2019). In order to explore the roles of CD36 in the process of M. tuberculosis infection and the functions of exosomal CD36 in this infection process further, CD36 expression was knocked down using siRNA technology to analyze the interaction between M. tuberculosis and CD36. The results demonstrated that M. tuberculosis promoted CD36 expression on macrophages and in CD36-knockdown macrophages. Meanwhile, infection of M. tuberculosis down-regulated CD36 expression in the aforementioned cells. The opposite CD36 expression in macrophages and exosomes suggests that exosomal CD36 may serve critical roles in the process of M. tuberculosis infection.
The present study finally elucidated the profiles of exosome proteins in the peripheral blood of patients with ATB and analyzed the functions of significantly different proteins. The identified differentially expressed proteins associated with ATB infection, such as MHC-I and CD36, and LBP may be potential markers for the diagnosis of M. tuberculosis infection confirmed by ROC analysis in serum. However, their specific molecular mechanism in the process of M. tuberculosis infection needs to be studied further.
The original contributions presented in the study are included in the article/supplementary material, further inquiries can be directed to the corresponding author/s.
The studies involving human participants were reviewed and approved by the Ethics Committee of Affiliated Kunshan Hospital of Jiangsu University. The patients/participants provided their written informed consent to participate in this study.
LT and JW conceived, designed, discussed the work, and revised the manuscript. MZ, YX, SL, and XY did the experiments. MZ, YX, and SL wrote and edited the manuscript. All authors read and approved the final version.
This work was supported by grants from the National Natural Science Foundation of China (No. 82002111), the Medical Scientific Research Project of Jiangsu Provincial Health Commission (No. Z2019001), the Science and Technology Project of Suzhou (No. KJXW2018068), the Key Project of New Technology of Kunshan First People’s Hospital (No. 2020I 03), the Changsha Municipal Natural Science Foundation (No. kq2007065), the Natural Science Foundation of Hunan Province (No. 2021JJ30908), and the Health Youth Backbone Talent of National Mentor System (No. Qngg2021043).
The authors declare that the research was conducted in the absence of any commercial or financial relationships that could be construed as a potential conflict of interest.
All claims expressed in this article are solely those of the authors and do not necessarily represent those of their affiliated organizations, or those of the publisher, the editors and the reviewers. Any product that may be evaluated in this article, or claim that may be made by its manufacturer, is not guaranteed or endorsed by the publisher.
Alipoor, S. D., Mortaz, E., Garssen, J., Movassaghi, M., Mirsaeidi, M., and Adcock, I. M. (2016). Exosomes and exosomal miRNA in respiratory diseases. Mediators Inflamm. 2016:5628404. doi: 10.1155/2016/5628404
Anand, P. K., Anand, E., Bleck, C. K., Anes, E., and Griffiths, G. (2010). Exosomal Hsp70 induces a pro-inflammatory response to foreign particles including mycobacteria. PLoS One 5:e10136. doi: 10.1371/journal.pone.0010136
Bettencourt, P., Müller, J., Nicastri, A., Cantillon, D., Madhavan, M., Charles, P. D., et al. (2020). Identification of antigens presented by MHC for vaccines against tuberculosis. NPJ Vaccines 5:2. doi: 10.1038/s41541-019-0148-y
Bhatnagar, S., and Schorey, J. S. (2007). Exosomes released from infected macrophages contain Mycobacterium avium glycopeptidolipids and are proinflammatory. J. Biol. Chem. 282, 25779–25789. doi: 10.1074/jbc.M702277200
Bhatnagar, S., Shinagawa, K., Castellino, F. J., and Schorey, J. S. (2007). Exosomes released from macrophages infected with intracellular pathogens stimulate a proinflammatory response in vitro and in vivo. Blood 110, 3234–3244. doi: 10.1182/blood-2007-03-079152
Biadglegne, F., König, B., Rodloff, A. C., Dorhoi, A., and Sack, U. (2021). Composition and clinical significance of exosomes in tuberculosis: a systematic literature review. J. Clin. Med. 10:145. doi: 10.3390/jcm10010145
Branger, J., Leemans, J. C., Florquin, S., Speelman, P., Golenbock, D. T., and van der Poll, T. (2005). Lipopolysaccharide binding protein-deficient mice have a normal defense against pulmonary mycobacterial infection. Clin. Immunol. 116, 174–181. doi: 10.1016/j.clim.2005.03.014
Cadena, A. M., Flynn, J. L., and Fortune, S. M. (2016). The Importance of first impressions: early events in mycobacterium tuberculosis infection influence outcome. mBio 7, e342–e316. doi: 10.1128/mBio.00342-16
Castaño, D., Barrera, L. F., and Rojas, M. (2011). Mycobacterium tuberculosis alters the differentiation of monocytes into macrophages in vitro. Cell Immunol. 268, 60–67. doi: 10.1016/j.cellimm.2011.02.006
Dobos, K., Schorey, J. S., and Kruh-Garcia, N. (2011). Tuberculosis Biomarkers: Prospects from the Bench to the Clinic, Vol. 1. Hague: Open Access Publisher.
Giri, P. K., Kruh, N. A., Dobos, K. M., and Schorey, J. S. (2010). Proteomic analysis identifies highly antigenic proteins in exosomes from M. tuberculosis-infected and culture filtrate protein-treated macrophages. Proteomics 10, 3190–3202. doi: 10.1002/pmic.200900840
Giri, P. K., and Schorey, J. S. (2008). Exosomes derived from M. Bovis BCG infected macrophages activate antigen-specific CD4+ and CD8+ T cells in vitro and in vivo. PLoS One 3:e2461. doi: 10.1371/journal.pone.0002461
Goletti, D., Petruccioli, E., Joosten, S. A., and Ottenhoff, T. H. (2016). Tuberculosis biomarkers: from diagnosis to protection. Infect. Dis. Rep. 8:6568. doi: 10.4081/idr.2016.6568
Hawkes, M., Li, X., Crockett, M., Diassiti, A., Finney, C., Min-Oo, G., et al. (2010). CD36 deficiency attenuates experimental mycobacterial infection. BMC Infect. Dis. 10:299. doi: 10.1186/1471-2334-10-299
Huang, W., Sherman, B. T., and Lempicki, R. A. (2009). Systematic and inte–grative analysis of large gene lists using DAVID bioinformatics resources. Nat. Protoc. 4, 44–57.
Il’in, D. A., and Shkurupy, V. A. (2019). In vitro analysis of the expression of CD11, CD29, CD36, and DC-STAMP molecules during the formation of multinuclear macrophages in BCG-infected mice. Bull. Exp. Biol. Med. 167, 653–655. doi: 10.1007/s10517-019-04591-0
Kowal, E. J. K., Ter-Ovanesyan, D., Regev, A., and Church, G. M. (2017). Extracellular vesicle isolation and analysis by western blotting. Methods Mol. Biol. 1660, 143–152. doi: 10.1007/978-1-4939-7253-1_12
Kruh-Garcia, N. A., Wolfe, L. M., Chaisson, L. H., Worodria, W. O., Nahid, P., Schorey, J. S., et al. (2014). Detection of Mycobacterium tuberculosis peptides in the exosomes of patients with active and latent M. tuberculosis infection using MRM-MS. PLoS One 9:e103811. doi: 10.1371/journal.pone.0103811
Lao, W., Kang, H., Jin, G., Chen, L., Chu, Y., Sun, J., et al. (2017). Evaluation of the relationship between MARCO and CD36 single-nucleotide polymorphisms and susceptibility to pulmonary tuberculosis in a Chinese Han population. BMC Infect. Dis. 17:488. doi: 10.1186/s12879-017-2595-2
Liu, Y., Zha, H., Han, X., Yu, S., Chai, Y., Zhong, J., et al. (2021). Molecular characterization and functional analysis of the bactericidal permeability-increasing protein/LPS-binding protein (BPI/LBP) from roughskin sculpin (Trachidermus fasciatus). Dev. Comp. Immunol. 123:104133. doi: 10.1016/j.dci.2021.104133
Palanisamy, G. S., Kirk, N. M., Ackart, D. F., Obregón-Henao, A., Shanley, C. A., Orme, I. M., et al. (2012). Uptake and accumulation of oxidized low-density lipoprotein during Mycobacterium tuberculosis infection in guinea pigs. PLoS One 7:e34148. doi: 10.1371/journal.pone.0034148
Shannon, P., Markiel, A., Ozier, O., Baliga, N. S., Wang, J. T., Ramage, D., et al. (2003). Cytoscape: a software environment for integrated models of biomolecular interaction networks. Genome Res. 13, 2498–2504.
Silverstein, R. L., and Febbraio, M. (2009). CD36, a scavenger receptor involved in immunity, metabolism, angiogenesis, and behavior. Sci. Signal. 2:re3. doi: 10.1126/scisignal.272re3
Singh, P. P., LeMaire, C., Tan, J. C., Zeng, E., and Schorey, J. S. (2011). Exosomes released from M. tuberculosis infected cells can suppress IFN-γ mediated activation of naïve macrophages. PLoS One 6:e18564. doi: 10.1371/journal.pone.0018564
Suárez, I., Fünger, S. M., Kröger, S., Rademacher, J., Fätkenheuer, G., and Rybniker, J. (2019). The diagnosis and treatment of tuberculosis. Dtsch. Arztebl. Int. 116, 729–735.
Subramanian, A., Gupta, V., Sarkar, S., Maity, G., Banerjee, S., Ghosh, A., et al. (2016). Exosomes in carcinogenesis: molecular palkis carry signals for the regulation of cancer progression and metastasis. J. Cell Commun. Signal. 10, 241–249. doi: 10.1007/s12079-016-0338-6
Sukumar, N., Tan, S., Aldridge, B. B., and Russell, D. G. (2014). Exploitation of Mycobacterium tuberculosis reporter strains to probe the impact of vaccination at sites of infection. PLoS Pathog. 10:e1004394. doi: 10.1371/journal.ppat.1004394
Sun, Y. F., Pi, J., and Xu, J. F. (2021). Emerging role of exosomes in tuberculosis: from immunity regulations to vaccine and immunotherapy. Front. Immunol. 12:628973. doi: 10.3389/fimmu.2021.628973
Szklarczyk, D., Franceschini, A., Wyder, S., Forslund, K., Heller, D., Huerta Cepas, J., et al. (2015). STRING v10: protein protein interaction networks, integrated over the tree of life. Nucleic Acids Res. 43D, D447D452.
Théry, C. (2011). Exosomes: secreted vesicles and intercellular communications. F1000 Biol. Rep. 3:15. doi: 10.3410/B3-15
Wang, J., Wang, Y., Tang, L., and Garcia, R. C. (2019). Extracellular vesicles in mycobacterial infections: their potential as molecule transfer vectors. Front. Immunol. 10:1929. doi: 10.3389/fimmu.2019.01929
Wang, J., Yao, Y., Chen, X., Wu, J., Gu, T., and Tang, X. (2018). Host derived exosomes-pathogens interactions: potential functions of exosomes in pathogen infection. Biomed. Pharmacother. 108, 1451–1459. doi: 10.1016/j.biopha.2018.09.174
Wang, J. J., Chen, C., Xie, P. F., Pan, Y., Tan, Y. H., and Tang, L. J. (2014). Proteomic analysis and immune properties of exosomes released by macrophages infected with Mycobacterium avium. Microbes Infect. 16, 283–291. doi: 10.1016/j.micinf.2013.12.001
Wang, M., Tang, S. T., Stryhn, A., Justesen, S., Larsen, M. V., Dziegiel, M. H., et al. (2011). Identification of MHC class II restricted T-cell-mediated reactivity against MHC class I binding Mycobacterium tuberculosis peptides. Immunology 132, 482–491. doi: 10.1111/j.1365-2567.2010.03383.x
Welton, J. L., Khanna, S., Giles, P. J., Brennan, P., Brewis, I. A., Staffurth, J., et al. (2010). Proteomics analysis of bladder cancer exosomes. Mol. Cell Proteomics 9, 1324–1338. doi: 10.1074/mcp.M000063-MCP201
World Health Organization (2021). Global Tuberculosis Report 2021. Geneva: World Health Organization.
World Health Organization, International Union Against Tuberculosis and Lung Disease, and Royal Netherlands Tuberculosis Association (2001). Revised international definitions in tuberculosis control. Int. J. Tuberc. Lung Dis. 5, 213215.
Keywords: active tuberculosis, exosomes, proteomics, CD36, PBMCs
Citation: Zhang M, Xie Y, Li S, Ye X, Jiang Y, Tang L and Wang J (2022) Proteomics Analysis of Exosomes From Patients With Active Tuberculosis Reveals Infection Profiles and Potential Biomarkers. Front. Microbiol. 12:800807. doi: 10.3389/fmicb.2021.800807
Received: 24 October 2021; Accepted: 07 December 2021;
Published: 06 January 2022.
Edited by:
Budhaditya Mukherjee, Indian Institute of Technology Kharagpur, IndiaReviewed by:
Kuntal Pal, Adamas University, IndiaCopyright © 2022 Zhang, Xie, Li, Ye, Jiang, Tang and Wang. This is an open-access article distributed under the terms of the Creative Commons Attribution License (CC BY). The use, distribution or reproduction in other forums is permitted, provided the original author(s) and the copyright owner(s) are credited and that the original publication in this journal is cited, in accordance with accepted academic practice. No use, distribution or reproduction is permitted which does not comply with these terms.
*Correspondence: Lijun Tang, dGxqeGllQGNzdS5lZHUuY24=; Jianjun Wang, d2FuZ2ppYW5qdW4wNTIwQDE2My5jb20=
†These authors have contributed equally to this work
Disclaimer: All claims expressed in this article are solely those of the authors and do not necessarily represent those of their affiliated organizations, or those of the publisher, the editors and the reviewers. Any product that may be evaluated in this article or claim that may be made by its manufacturer is not guaranteed or endorsed by the publisher.
Research integrity at Frontiers
Learn more about the work of our research integrity team to safeguard the quality of each article we publish.