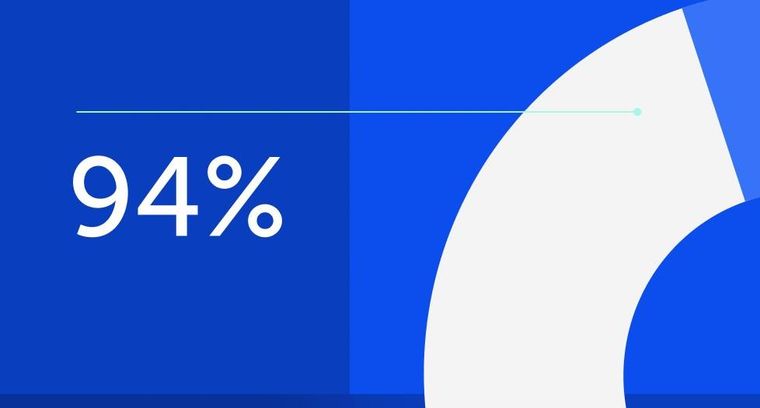
94% of researchers rate our articles as excellent or good
Learn more about the work of our research integrity team to safeguard the quality of each article we publish.
Find out more
ORIGINAL RESEARCH article
Front. Microbiol., 13 January 2022
Sec. Antimicrobials, Resistance and Chemotherapy
Volume 12 - 2021 | https://doi.org/10.3389/fmicb.2021.798750
This article is part of the Research TopicStreptococcus spp. and Corynebacterium spp.: Clinical and Zoonotic Epidemiology, Virulence Potential, Antimicrobial Resistance and Genomic Trends and ApproachesView all 12 articles
Background: Streptococcus pneumoniae is an important human pathogen that can cause severe invasive pneumococcal diseases (IPDs). The aim of this multicenter study was to investigate the serotype and sequence type (ST) distribution, antimicrobial susceptibility, and virulence of S. pneumoniae strains causing IPD in China.
Methods: A total of 300 invasive S. pneumoniae isolates were included in this study. The serotype, ST, and antimicrobial susceptibility of the strains, were determined by the Quellung reaction, multi-locus sequence typing (MLST) and broth microdilution method, respectively. The virulence level of the strains in the most prevalent serotypes was evaluated by a mouse sepsis model, and the expression level of well-known virulence genes was measured by RT-PCR.
Results: The most common serotypes in this study were 23F, 19A, 19F, 3, and 14. The serotype coverages of PCV7, PCV10, PCV13, and PPV23 vaccines on the strain collection were 42.3, 45.3, 73.3 and 79.3%, respectively. The most common STs were ST320, ST81, ST271, ST876, and ST3173. All strains were susceptible to ertapenem, levofloxacin, moxifloxacin, linezolid, and vancomycin, but a very high proportion (>95%) was resistant to macrolides and clindamycin. Based on the oral, meningitis and non-meningitis breakpoints, penicillin non-susceptible Streptococcus pneumoniae (PNSP) accounted for 67.7, 67.7 and 4.3% of the isolates, respectively. Serotype 3 strains were characterized by high virulence levels and low antimicrobial-resistance rates, while strains of serotypes 23F, 19F, 19A, and 14, exhibited low virulence and high resistance rates to antibiotics. Capsular polysaccharide and non-capsular virulence factors were collectively responsible for the virulence diversity of S. pneumoniae strains.
Conclusion: Our study provides a comprehensive insight into the epidemiology and virulence diversity of S. pneumoniae strains causing IPD in China.
Streptococcus pneumoniae is a common Gram-positive coccus that can cause serious invasive pneumococcal diseases (IPDs) such as pneumonia, meningitis, and sepsis, especially in children and the elderly. It is estimated that IPD is responsible for approximately 826,000 deaths in children aged 1–59 months annually worldwide (O’Brien et al., 2009). Furthermore, the incidence of IPD increases with age, and with higher mortality reported in people over 65 years of age (Marrie et al., 2018).
The capsular polysaccharides of S. pneumoniae play an important role in pneumococcal disease pathogenesis, and at least 100 serotypes have been identified based on the capsule synthesis locus (cps) gene differences (Ganaie et al., 2020). The use of vaccines targeting specific serotypes has significantly reduced the morbidity and mortality of pneumococcal disease. S. pneumoniae vaccines PPV23 (covering serotypes 1–5, 6B, 7F, 8, 9 N, 9V, 10A, 11A, 12F, 14, 15B, 17F, 18C, 19A, 19F, 20, 22F, 23F, and 33F), PCV7 (covering serotypes 4, 6B, 9 V, 14, 18C, 19F, and 23F), PCV10 (covering PCV7 serotypes plus serotypes 1, 5, and 7F) and PCV13 (covering PCV10 serotypes plus serotypes 3, 6A, and 19A) were commercially introduced in the United States of America (USA) as early as 1983, 2000, 2008, and 2009 (Kim et al., 2020). At present, developed countries in Europe and the United States have already included PCV and PPV vaccines in their national vaccination programs, greatly reducing IPD caused by serotypes covered by these vaccines (Richter et al., 2013; Esposito et al., 2014). In China, several vaccines such as PCV7, PCV13, and PPV23 are currently available, but vaccination rates are still low due to the high cost of the vaccines (Boulton et al., 2016; Liang et al., 2016; Chen et al., 2018). However, the use of vaccines results in a shift in S. pneumoniae serotype distribution, a phenomenon known as “serotype replacement”, in which the prevalence of serotypes covered in the vaccines decrease whilst that of serotypes not included in the vaccines increase (Geno et al., 2015). Besides, S. pneumoniae can undergo efficient intra- and interspecies DNA recombination, leading to changes in the capsule composition, molecular typing, antibiotic resistance, and virulence factors (Varghese et al., 2019). These phenomena present great challenges to the prevention and treatment of pneumococcal diseases.
There is still a scarcity of comprehensive studies on the epidemiological characteristics as well as the virulence levels of S. pneumoniae causing invasive infections in China. The aim of this multicenter study was to investigate the serotype and sequence type (ST) distribution, antimicrobial susceptibility, and virulence of S. pneumoniae isolates causing IPD in China.
A total of 300 invasive S. pneumoniae isolates from 27 teaching hospitals in 13 provinces in China, collected during the period January 2010–October 2015, were included in this study (Supplementary Figure 1). All isolates were non-replicate and obtained from sterile sites, with only the first isolate selected from the same patient. The majority (141/300, 47%) of the isolates were isolated from younger children (0 to 5 years), whilst 29 (9.7%) were from older children (5 to 18 years), 92 (30.7%) from adults aged 18 to 65 years, and 38 (12.7%) from patients >65 years old. The major specimen type was blood (72.7%, 218/300), followed by cerebrospinal fluid (CSF) (19.0%, 57/300), and then pleural effusion (5.7%, 17/300). Other specimen types included ascetic fluid (n = 4), joint tissue (n = 2), thoracic drainage fluid (n = 1) and lung tissue (n = 1). All isolates were identified as S. pneumoniae using Vitek MS system (BioMerieux, Rhône, France) and optochin susceptibility test.
All the isolates were serotyped by the Quellung reaction, which was considered the gold standard for serotyping. Firstly, the S. pneumoniae serogroup/type was preliminarily determined by latex agglutination test using the checkerboard typing system (Lalitha et al., 1999). Then specific antiserum was mixed with the bacterial suspension and capsular swelling was observed under the microscope. If the Quellung reaction was negative with all antisera, it was classified as non-typeable (NT) type.
The DNA of the S. pneumoniae isolates was extracted using the AxyGenamp DNA Mini Extraction Kit (Axygen, United States) according to the manufacturer’s instructions. Using the extracted DNA as the template, the seven housekeeping genes (aroE, gdh, gki, recP, spi, xpt, ddl) were amplified using polymerase chain reaction (PCR). The primer sequences used are detailed in Supplementary Table 1. The amplified products were sequenced and aligned with the sequences on the MLST website to determine the sequence type (ST) of the isolate. If a new allele combination was obtained, the corresponding sequence and strain-related information were submitted to the MLST databases1 for assigning of a new ST accession number after approval.
The minimum inhibitory concentrations (MICs) of S. pneumoniae isolates against penicillin (P), amoxicillin/clavulanic (AMC), cefuroxime (CXM), ceftriaxone (CRO), and several other antibiotics (cefepime, ertapenem, imipenem, meropenem, levofloxacin, trimethoprim/sulfamethoxazole, clindamycin, clarithromycin, erythromycin, linezolid and vancomycin), were determined by broth microdilution method. S. pneumoniae ATCC 49619 and Escherichia coli ATCC25922 were used as quality control strains. Each batch of isolates was tested simultaneously with the quality control strains, and the results were considered valid when the MIC values of both strains were within the expected quality control range. The antimicrobial susceptibility testing results were interpreted according to CLSI, 2019 document (CLSI, 2019).
This study was approved by the Medical Ethics Committee of Peking Union Medical College Hospital (No. S-263). The strains from the most prevalent serotypes in this study, and all serogroup six strains, were tested for virulence using a mouse sepsis model. Overall, 65 S. pneumoniae isolates were tested in this model, including serotypes 23F (n = 8), 19F (n = 8), 19A (n = 8), 3 (n = 8), 14 (n = 8), 6A (n = 12), 6B (n = 11), and 6C (n = 2).
We established an intraperitoneal infection model for S. pneumoniae using 6-week-old outbred CD-1 female mice (obtained from Beijing Vital River Laboratory Animal Technology Co. Ltd). For each isolate tested, a group of six mice were inoculated intraperitoneally with 200 μl of bacterial suspension containing approximately 1 × 104 CFU of S. pneumoniae per mouse. The mortality of the mice was assessed daily until the 7th day. Isoflurane (Sigma-Aldrich, United States) was used at a dose of 6 mg/kg of the mouse weight for anesthetization at the time of blood collection. Orbital vein blood samples were obtained every 12 h for the first 2 days after inoculation and then daily for the next 5 days. Quantification of bacteria in the blood was determined by serial dilution and plating on trypticase soy agar with 3% sheep blood.
RNA from 25 strains of serogroup 6 was extracted using Magen RNA Extraction Kit (Magen Bio, China) according to manufacturer’s instructions. Reverse transcription of the RNA was performed using the FastKing RT Kit (Tiangen Bio, China). Quantitative PCR reactions were performed using the SYBR Premix ExTaq™ PCR kit (Takara Bio, Japan) on a LightCycler 480 instrument (Roche Molecular Diagnostics, Rotkreuz, Switzerland). Using 16S rRNA as an internal reference gene, the expression of six non-capsule-associated virulence genes (ply, lytA, nanA, psaA, pspA, and HylA) (Ogunniyi et al., 2002; LeMessurier et al., 2006) was calculated using the 2–ΔΔCt method. The primer sequences used are detailed in Supplementary Table 1.
Data on the distribution of different serotypes and vaccine coverage was analysed using Excel 2019 software (Microsoft Inc., United States). Differences in antimicrobial susceptibility were analyzed by MIC range, MIC50 and MIC90, and statistical analysis was performed by chi-square test or Fisher’s exact probability test using SPSS software (version 22.0, SPSS Inc., Chicago, IL, United States). Differences in survival time and gene expression between and within groups were analyzed by independent sample t-test and one-way ANOVA. P-values < 0.05 were considered statistically significant.
The serotypes of 299 S. pneumoniae strains were accurately identified by Quellung reaction, and the remaining one isolate was considered as non-typeable (NT). Overall, 40 serotypes were identified, with the 5 commonest being 23F (14.3%, 43/300), 19F (13.7%, 41/300), 19A (13.7%, 41/300), 3 (10.3%, 31/300), and 14 (9.0%, 27/300) (Supplementary Table 2). Based on the specific serotypes included in the vaccines, the coverage rates of PCV7, PCV10, PCV13, and PPV23 vaccines on this bacterial collection were 42.3% (127/300), 45.3% (136/300), 73.3% (220/300), and 79.3% (238/300), respectively (Figure 1A). The most prevalent serotypes isolated in the adult and children’s groups were similar. In the adult group, serotypes 23F (16.9%) and 19A (16.9%) were dominant, followed by 19F (14.6%), 14 (7.7%), and 3 (6.15%), whereas in the children group, serotype 3 was dominant (13.5%), followed by 19F (12.9%), 23F (12.4%), 19A (11.2%), and 14 (10.0%). There was no significant difference in the distribution of vaccine coverage between the adult and children’s groups: PCV7 (43.8% vs 41.2%) (P = 0.7386), PCV10 (47.7% vs 43.5%) (P = 0.5438), PCV13 (74.6% vs 72.4%) (P = 0.4224) and PPV23 (79.2% vs79.4%) (P = 0.9192) (Figure 1B).
Figure 1. Serotype distribution of 300 invasive S. pneumoniae isolates. (A) Vaccine coverage S. pneumoniae isolates involved in this study. (B) Serotype distribution of isolates in different age groups in adult and children. (C) Changes in isolation rates of PCV13 and non-PCV13 covered serotypes strains during the study period 2010–2015. (D) Changes in isolation rates of PCV23 and non-PCV23 covered serotypes strains during the study period 2010–2015.
Serotypes 23F, 19F, 19A, 3, and 14 were dominant from 2010 to 2015, ranging from 52.0 to 76.4%, albeit slight yearly variation in the distribution. In contrast, from the year 2010–2015, there was a decrease in the isolation rates of serotypes 23F, 19A, and 14, while that of 19F, 3, 6A, and 6B increased, although the differences were not statistically significant (Supplementary Figure 2). Noticeably, for both PCV13 and PPV23 vaccines, the vaccine serotype coverage among the isolates showed a decreasing trend from 2010 to 2015 (PCV13: 85.3 to 68%; PPV23: 91.2 to 72%), while the proportion of non-vaccine covered serotypes increased (non-PCV13: 14.7 to 32%; non-PPV23: 8.8 to 28%), though the difference was not statistically significant (P = 0.2053 for PCV13 and P = 0.1114 for PPV23) (Figures 1C,D).
Among the 300 isolates studied, 123 STs were identified by MLST analysis (Supplementary Table 3). Of these, ST320 (11.3%, 34/300) predominated, followed closely by ST81 (9.3%, 28/300), ST271 (8.7%, 26/300), ST876 (8.7%, 26/300) and ST3173 (2.7%, 8/300). There were 33 STs identified for the first time here, including ST12901-ST12920, ST13199-ST13200, ST14346-ST14351, ST14705-ST14707, and ST14726-ST14727.
A phylogenetic tree was constructed based on single-locus variants (SLV) in seven housekeeping genes, and a total of 18 clonal complexes (CC) and 65 singletons were identified (Figure 2). The most common clonal complex was CC320 (13.3%), followed by CC271 (12.3%), CC81 (11.0%), CC876 (6.7%), and CC1263 (3.3%) (Table 1). Based on the Pneumococcal Molecular Epidemiology Network database (PMEN), seven international resistance clones containing 46 strains were identified in this study, including Spain23F-1 (ST81, n = 28), Netherlands3-31 (ST180, n = 6), Taiwan19F-14 (ST236, n = 4), Spain6B-2 (ST90, n = 4), Taiwan23F-15 (ST242, n = 2), Denmark14-32 (ST230, n = 1), and Colombia23F-26 (ST338, n = 1) (Supplementary Table 4).
Figure 2. The minimun spanning tree (MST) of the 300 invasive S. pneumoniae isolates based on MLST. Note: Each circle corresponds to a sequence type (ST). The number besides the circle represents ST number. The circle size represents the number of strains. The circle color represents different ST categories with isolate number ≥3. The lines between circles indicate the similarity between profiles: bold solid line, six of seven MLST alleles in common; normal solid line, five alleles in common; dashed line, four alleles in common; dotted line, ≤3 alleles. The gray halo surrounding the STs in Figure denotes STs belonging to different MLST clusters/clonal complexes.
In terms of annual distribution, ST320 dominated in 2010 (26.5%; 9/34), 2011 (12%, 3/25) and 2013 (12.8%, 12/94). Likewise, ST271 dominated in 2012, accounting for 12.5% (6/48) of the isolates, whilst ST81 (10.8%, 8/74), ST320 (12.0%, 3/25) and ST271 (12.0%, 3/25) dominated in 2014 and 2015, respectively. A declining trend in the prevalence of ST320 (from 26.5% in 2010 to 12% in 2015) and ST81 (from 14.7% in 2010 to 4% in 2015) was observed as can be seen in Supplementary Figure 3, whilst an increasing trend is obvious for ST271 (from 8.8% in 2010 to 12% in 2015) and ST876 (from 2.9% in 2010 to 10.8% in 2014) (Supplementary Figure 3).
The distribution of STs among the different serotypes is shown in Figure 3. Only one ST was described in each of the following serotypes; 12F (n = 4), 28F (n = 3), 28A (n = 2), 4, 25F, 22F, 17, 2, 17A, and 18C (all n = 1, each). However, other serotypes showed diverse ST distribution. Serotype 23F was dominated by ST81 (63.0%, 27/43), whilst serotype 19F was dominated by ST271 (63.4%, 26/41) and serotypes 19A by ST320 (80.5%, 33/41). In addition, serotype 3 was dominated by ST505 (19.4%, 6/31) and ST180 (19.4%, 6/31), and serotype 14 by ST876 (66.7%, 18/27).
Figure 3. Sequence type distribution of 300 invasive S. pneumoniae isolates among different serotypes.
Antimicrobial susceptibility results of the 300 invasive S. pneumoniae isolates are shown in Table 2. All the isolates were susceptible to ertapenem, levofloxacin, moxifloxacin, linezolid, and vancomycin, with MIC90 values of 0.25 μg/ml, 1 μg/ml, 0.125 μg/ml, 1 μg/ml and 0.5 μg/ml, respectively. This was closely followed by amoxicillin/clavulanic acid with a susceptible rate of 97.7% among the isolates. Over 90% of the strains were resistant to azithromycin, clarithromycin, erythromycin, clindamycin, tetracycline, and chloramphenicol. Notably, MIC50 and MIC90 values for azithromycin, clarithromycin and erythromycin were higher than 1024 μg/ml each. Based on the non-meningitis (R ≥ 8 μg/ml) breakpoint for penicillin, no resistant strains were observed among the isolates for this antibiotic, but only an intermediate rate of 4.3%. However, based on the meningitis (R ≥ 0.12 μg/ml) and oral (R ≥ 2 μg/ml) breakpoints for penicillin, the resistance rates for this antibiotic among the isolates were 67.7 and 44.7%, respectively. The resistance rate of the isolates to trimethoprim/sulfamethoxazole, cefuroxime and cefaclor was ≥60% each, with similar MIC90 values for ceftriaxone and cefepime at 2 μg/ml each. As per the meningitis breakpoint for cefepime, the resistance rate for cefepime was slightly higher than that of ceftriaxone (29.7% vs 25.7%), but slightly lower than that of ceftriaxone (4.0% vs 7.3%) when based on the non-meningitis breakpoint. A very small proportion of the isolates was resistant to imipenem (3.7%) and meropenem (2.7%), but the intermediate rate was higher at 37.7% (imipenem) and 46.0% (meropenem).
The susceptibility profiles of several predominant serotypes to different antimicrobial agents are shown in Table 3. Overall, serotypes 23F, 19F, 19A, and 14 exhibited a higher resistance rate to all the antimicrobials tested, while serotype 3 had a relatively lower resistance rate. All serotypes showed high resistance rates to macrolides (87.1–100%), chloramphenicol (87.8–100%) and tetracycline (83.9–100%). Furthermore, all serotypes except serotype 3, exhibited high resistance rates to penicillin (meningitis and oral breakpoint), cefuroxime (63.6–100%) and cefaclor (72.7–100%). Serotype 19F had higher resistance rates to ceftriaxone (39.0% based on the non-meningitis breakpoint and 75.6% based on the meningitis breakpoint) and cefepime (14.6% based on the non-meningitis breakpoint and 78.0% based on the meningitis breakpoint) compared to other serotypes.
Table 3. Antimicrobial susceptibility results of the 300 S. pneumoniae isolates with different serotypes in China.
Serotype 14 showed a high rate of resistance to imipenem (7.4%), serotype 6B showed a high rate of resistance to meropenem (9.1%), whereas serotype 6A exhibited a low level of resistance to trimethoprim/sulfamethoxazole (25.0%). We also observed that both PCV and PPV23 vaccine-covered serotype strains showed higher resistance rates to most antimicrobial drugs than the corresponding non-vaccine-covered serotype strains, including for penicillin, cefuroxime, ceftriaxone, cefepime (meningitis breakpoint), and cefaclor (Supplementary Table 5). In addition, a higher proportion of PCV-covered serotype strains were resistant to clindamycin than non-covered strains (97.3% vs 91.2%, P = 0.0482). Likewise, a higher percentage of PPV23-covered serotype strains were resistant to cotrimoxazole than non-covered serotype strains (68.9% vs 51.6%, P = 0.0164).
An analysis of overall resistance rates of the isolates to different antimicrobial drugs by year is shown in Table 4. Collectively, over the six-year study period, a decreasing trend in antibiotic resistance rates to penicillin, cefuroxime, ceftriaxone, cefepime, cefaclor and trimethoprim/sulfamethoxazole, was observed. However, the resistance rates for ertapenem, levofloxacin, moxifloxacin, linezolid, and vancomycin remained at zero. On the other hand, clindamycin, azithromycin, clarithromycin, erythromycin, chloramphenicol, and tetracycline, maintained high levels of resistance rates (81.2–100%) across the six-year period. The resistance rate of imipenem also underwent a fluctuation (2.9% in 2010, 0% in 2011 and 2012, and then 8.1 and 8.0% in 2014 and 2015) during the 6 years. For other antibiotics, such as amoxicillin/clavulanic acid and meropenem, resistant strains have emerged but the overall resistance rate was still no more than 5%.
Table 4. Antimicrobial susceptibility results of the 300 S. pneumoniae isolates during the study period in China.
The results of the virulence level assessment among the predominant serotype strains are shown in Figure 4A. We observed extremely low virulence levels for S. pneumoniae strains in serotypes 23F, 19A, 19F, and 14, with an average mice survival time uniformly exceeding 7 days. Conversely, 1 × 104 CFU of serotype 3 strains killed all the mice within 2 days, with a mean survival time of 24 h. In addition, the number of bacteria in the blood of mice infected with serotype 3 strains (n = 8) increased rapidly to 105 CFU/ml in the first 12 h of inoculation, and then increased up to 109 CFU/ml at 24 h. For serotypes 23F, 19F, 19A, and 14 strains, the bacterial load remained undetectable over the course of the experiment. These results suggest that the virulence levels of S. pneumoniae isolates is somehow dependent on capsular serotypes. For instance, serotype 3 strains were highly virulent in the mouse model whilst serotypes 23F, 19A, 19F, and 14 were lowly virulent. However, for serogroup 6 strains, virulence level variation existed among the strains.
Figure 4. Virulence assessment of pneumococcal isolates in mouse sepsis model. (A) Relationship of the virulence levels and the serotypes of S. pneumoniae. Virulence level was determined by the mean survival time of mice i.p. infected with pneumococcal isolates. One-way ANOVA was used to compare differences among groups. (B–D) Blood burden of bacteria in mice infected with different pneumococcal strains in serotype 6A, 6B, and 6C. *P < 0.05.
We defined strains with maximal bacterial load in the blood of ≥105 CFU/ml and a 7-day survival rate ≤20% as high virulent strains (HVS), while those with bacterial blood load of ≤104CFU/ml and a 7-day survival rate ≥80%, were defined as low virulence strains (LVS). The remainder was classified as intermediate virulent strains (IVS). Based on this criterion, six (S165, S219, S221, S249, S260, and S292) of the 12 strains of serotype 6A were HVS, two (S142 and S179) were LVS and the rest (S33, S197, S273, S276) IVS (Figure 4B). For serotype 6B, only one strain (S78) was classified as HVS, two (S4 and S14) as IVS and the remaining eight (S70, S159, S178, S183, S209, S290, S299, and S300) as LVS (Figure 4C). There were only two strains of serotype 6C, and one was classified as HVS (S119) and the other (S227) as LVS (Figure 4D).
The virulence level variation among strains within the same serotype suggests that some capsule-independent factors may have contributed to virulence. Thus, the expression levels of six non-capsule-associated virulence genes (ply, lytA, nanA, psaA, pspA, and hylA) were measured by RT-PCR (Figure 5). In serotype 6A, strain S292 (HVS) had the highest-level expression of ply and nanA genes, whilst isolate S260 (HVS) had the highest level of expression of psaA and pspA genes. Furthermore, isolate S219 (HVS) had the highest level of expression of hylA, whilst isolate 179 (IVS) had the highest level of expression of lytA. In contrast to serotype 6A, all genes were expressed at the highest level in the LVS serotype 6B isolate, save for the hylA gene which was expressed at the highest level in the HVS isolate (S78). For serotype 6C, the expression levels of ply, lytA, psaA, and hylA genes were higher in the HVS strain (S119) than in the LVS strain (S227), whereas the expression levels of nanA and pspA were higher in the LVS strain (S227) than in the HVS strain (S119).
Figure 5. Comparison of virulence gene expression levels among serogroup 6 strains. Expression levels of ply (A), lytA (B), nanA (C), psaA (D), pspA (E) and hylA (F) were measured and compared with the S300 strain (marked in black) as the control strain. Gray, green and red represent the low virulence strains (LVS), intermediate virulent strains (IVS) and high virulent strains (HVS), respectively. *P < 0.05.
Overall, we found great variation in the expression of these virulence genes in different serotypes and even among different strains within the same serotypes. In general, capsular, and non-capsular properties together determined the virulence of S. pneumoniae strains. The regulation of virulence levels is complex and elaborate, determined by a variety of virulence genes.
The 300 S. pneumoniae isolates studied were isolated from 27 teaching hospitals in China, all from sterile body sites, including blood, cerebrospinal fluid, and pleural effusion, which can result in severe IPD. IPD is an aggressive disease with an annual incidence of 6.6–14 per 100,000 people, and a mortality rate of 10–30% (Sousa et al., 2018). Given that S. pneumoniae has become a major challenge to public health systems worldwide, a comprehensive understanding of its clinical characteristics is of great importance.
It has been reported that the distribution of S. pneumoniae serotypes exhibits association of specific serotypes with geographical locale, attack rate, age, tendency to cause outbreaks, and propensity to acquire antimicrobial resistance genes (Jourdain et al., 2011). Furthermore, the introduction of vaccines covering specific serotypes has been an effective way to prevent IPD caused by S. pneumoniae. The five most common serotypes in this study were: 23F (43, 14.3%), 19A (41, 13.7%), 19F (41, 13.7%), 3 (31, 10.3%), and 14 (27, 9.0%). The serotype coverage rates of PCV7, PCV10, PCV13, and PPV23 vaccines on our bacterial collection was 42.3, 45.3, 73.3 and 79.3%, respectively. The distribution of serotypes and vaccine coverage in our study was similar between the children and adult groups.
Based on reports from the Canadian Public Health Laboratory Network (Demczuk et al., 2013), the predominant S. pneumoniae serotypes causing IPD in Canada during the years 2010–2012 were 19A, 7F, 3, and 22F, with PCV13 vaccine coverage ranging from 41 to 66%. Among Turkish children, the most common serotypes causing IPD in 2015–2018 were 19F, 1, and 3, with PCV13 serotypes accounting for 56.2%, as reported by Mehmet Ceyhan et al. (Ceyhan et al., 2020). In Japan, an Asian neighbor of China, the most common serotypes in a study that included 177 patients with IPD from September 2016 to April 2018, were 12F, 3, 23, 19F, 10A, 6C, and 22F, while PCV7, PCV13, and PCV23 had 2.8, 28.2 and 61.0% coverage, respectively (Yanagihara et al., 2021). These findings demonstrate that the distribution of S. pneumoniae serotypes and vaccine coverage varies by country, time of isolation, age, etc., which may be related to differences in antibiotic use, health conditions, economic situation, and time of vaccine introduction in various regions.
A study from two tertiary children’s hospitals in Beijing (2012–2017) showed that the dominant S. pneumoniae serotypes in IPD cases in children were 19F, 19A, 14, 23F, and 6B, with PCV13 having a coverage of 90.1% of the strains (Shi et al., 2019). Likewise, in another study carried out at a hospital in Shenyang, a city in northeast China, from 2010 to 2014, the most common S. pneumoniae serotypes among isolates from pediatric IPD cases were 19A, 14, 19F, 23F, and 6B, and the coverage of PCV7, PCV10 and PCV13 vaccines was 53.9, 56.3 and 93.8%, respectively (Zhou et al., 2016). The prevalent serotypes in the two studies mentioned above are similar to ours, but with slightly higher vaccine coverage, which may be due to differences in geographical distribution of the serotypes (Zhou et al., 2016).
PCV7 was introduced in China in 2008, while PCV13 was approved in 2016 and PPV23 was only available for children older than 2 years (Shi et al., 2019). However, vaccination rates of the above-mentioned vaccines are relatively low in most regions of China due to the prohibitive high cost. Hence, many children and elderly people are still vulnerable to the lethal threats of IPD caused by S. pneumoniae. Moreover, based on our research, it is worth noting that the serotype coverage of PCV13 has increased significantly relative to PCV7 and PCV10, but not significantly different from that of PPV23. This may be attributed to the continuous rise in the prevalence of PCV13-associated serotypes 19A, 6A, and 3. On this basis, we recommend vaccinating the population in China with PCV13 or PPV23 to reduce the incidence of IPD.
Although the introduction of vaccines has greatly reduced the incidence of IPD for vaccine-covered serotypes, on the other hand, the incidence of IPD caused by non-vaccine-covered serotypes has increased, a phenomenon commonly referred to as “serological replacement”. Data from the Danish National Serological Institute from 1999–2014 showed that the incidence of IPD caused by non-vaccine covered serotypes 8, 9N, 11A, 12F, 15A, 20, 22F, 23B, 24F, and 33F, increased for all ages after the introduction of PCV13 vaccine, compared to the pre-introduction period (Slotved et al., 2016). A review showed that the most common serotypes in European countries before the introduction of the PCV7 vaccine were 14, 6B, 19F, and 23, but after the introduction of this vaccine, the dominant serotypes switched to 1, 19A, 3, 6A, and 7F, which were not covered by PCV7 (Isaacman et al., 2010). In our study, serological replacement was observed for both PCV13 and PPV23 vaccines, although the distribution of prevalent serotypes varied from 2010 to 2015. Therefore, continuous monitoring of the distribution of non-vaccine covered serotypes is essential to assess the effectiveness of vaccine preventative efforts and to effectively control IPD.
Multi-locus sequence typing (MLST) analysis revealed a considerable diversity among the strains, with the most common STs being ST320, ST81, ST271, ST876, and ST3173. Phylogenetic tree analysis revealed that CC320, CC271, CC81, CC876, and CC1263 were the most common clonal complexes. This was consistent with previous studies in which CC320 and CC271 were prevalent complex groups in China (Li et al., 2019). A previous study in Catalonia, Spain, during the year 2009, which consisted of 614 IPD cases, showed that the most common clonal lineages were ST306, CC191, CC230, CC156, ST304, CC1223, and CC180 (Muñoz-Almagro et al., 2011). However, in Singapore, an Asian country, the most common STs causing IPD in children were ST9, ST156, ST236, and ST90 (Jefferies et al., 2011). Therefore, the clonal lineage of S. pneumoniae may vary by geographical location.
We observed that the decline in the prevalence of ST320 and ST81 (from 2010–2015) in our study was accompanied by an increase in the prevalence of ST271 and ST876. Given the relationship between serotypes and STs, serotype 19A is dominated by ST320 (80.5%). S. pneumoniae strain 19A ST320, derived from an international Taiwan (19F)-14 (ST236) clone, has been reported to be more competitive than its ancestral clone (Hsieh et al., 2013; Chen et al., 2019). Meanwhile, in the current study, serotype 19A ST320 had a higher rate of drug resistance than other STs, and is often associated with multidrug resistance in Asian countries (Shin et al., 2011). Despite a gradual decline (2011–2015) in the isolation rate of serotype 19A ST320 in our study, possibly owing to vaccine pressure, it remained the predominant ST in 2015. In view of its notoriety, the dissemination and sequence type switching of serotype 19A still requires continuous monitoring in China and elsewhere.
In the present study, ST81 was mainly associated with serotype 23F, accounting for 63% of this serotype. The S. pneumoniae 23F ST81 lineage was one of the first pandemic clones identified, and was implicated in nearly 40% of penicillin-resistant pneumococcal infections in the United States in the late 1990s (Muñoz et al., 1991). Since then, a study from Beijing raised an alarm that serotype 23F ST81 was non-susceptible to β-lactam antibiotics and was on the rise (Ma et al., 2013). In the present study, although the isolation rate of 23F ST81 went from 14.7% in 2010 to 4% in 2015, the isolation rate in 2014 was still relatively high (10.8%). The discrepancy may be explained by variation in the number of isolates between the years or by a shift in the ST under vaccine pressure. Hence, the distribution of serotype 23F ST81 deserves continuous attention.
ST271 and ST876 were correlated with serotypes 19F and 14, respectively, and were in a rising trend (2010–2015). A previous study demonstrated a worrying rising trend in the 19F CC271 lineage among S. pneumoniae strains from acute respiratory infections in Chinese children, from 14.3% in 1997–1998 to 92% in 2010, and was responsible for the increased non-susceptibility rate to β-lactam antibiotics of serotype 19F (Li et al., 2013). Although serotype 19F has been included in the PCV7 vaccine, it presented huge challenges in its manipulation to elicit a satisfactory protective immune response in the human host as it is more resistant to C3 deposition and less sensitive to opsono-phagocytosis (Melin et al., 2009; Poolman et al., 2011). In agreement with this, the isolation rate of serotype 19F in the present study increased from 11.8% in 2010 to 16% in 2015. Thus, trends in serotype 19F and ST271 distribution need to be monitored continuously in the future, especially after the vaccine becomes more widely available.
The isolation rate for ST876 in the present study was relatively low, although it increased alarmingly from 2.9% in 2010 to 10.8% in 2014. Previous studies reported very dramatic increases in the detection rate of S. pneumoniae serotype 14 CC876 lineage, increasing notably from 0% in 1997–2000 to 96.4% in 2010 in children with acute respiratory infections in China, completely replacing the previously dominant clonal group CC875 (from 84.2 to 0%) and exhibiting a high level of non-susceptibility rates to β-lactam antibiotics (He et al., 2015). While serotype 14-ST876 did not currently dominate in this study, the increasing isolation rate, and the potent clonal expansiveness of ST876 still sounded alarm bells.
Interestingly, 10 serotypes (12F, 28F, 28A, 4, 25F, 22F, 17, 2, 17A, and 18C) matched only one ST each, implying that the non-vaccine covered serotypes may have a lower clonal diversity. As discussed above, the numbers of non-vaccine covered serotypes are growing and therefore further molecular typing studies of the non-vaccine covered serotypes are needed in the future to assess the effects of vaccines on the epidemiology of S. pneumoniae. In addition to this, seven groups of internationally resistance clones such as Spain23F-1, were detected in the present study by sequence alignment with those on the PMEN website. It can be assumed that the increasing international interactions have led to the widespread dissemination of these resistant clones worldwide.
The use of antibiotics is the mainstay of IPD treatment, so it is particularly important to choose effective antimicrobial drugs. All strains in the present study were susceptible to ertapenem, levofloxacin, moxifloxacin, linezolid, and vancomycin, which is in line with most studies in China and elsewhere (Xue et al., 2010; Cai et al., 2018; Golden et al., 2019). These antimicrobial agents may be a valuable reference for empirical medication in IPD treatment.
Penicillin has been recognized as an important choice for the treatment of invasive S. pneumoniae infections, but the rising rate of resistance among strains in recent years has sparked concern. In our study, we found that the proportion of PNSP based on the oral breakpoint was 67.7%, compared to 67.7 and 4.3% based on the meningitis and non-meningitis breakpoints, respectively. An analysis involving 1517 invasive S. pneumoniae strains from children in Germany between 1997 and 2004, showed that only 5.1 and 1.1% of the isolates were penicillin-intermediate and resistant, respectively (Reinert et al., 2007).
In China’s bordering country, Cambodia, 46% of S. pneumoniae strains from children with invasive infections (2007–2012) were PNSP based on the meningitis breakpoint (Moore et al., 2016). The proportion of PNSP in our study was significantly higher than those in the two studies mentioned above, which may be explained by variation in the population included and the misuse of these over-the-counter antibiotics in China due to their availability and convenience from numerous licensed and non-licensed sources (Troy, 1992). Furthermore, the present study included isolates from both adults and children whereas the previous studies only focused on the pediatric population. In general, the adult population tends to be exposed to antibiotics more than children, resulting in an increased overall non-susceptibility rate.
Notably, most of the studied isolates (over 95%) were resistant to macrolides (azithromycin, clarithromycin, erythromycin) and clindamycin. This may be related to the frequent clinical use of these antibiotics during the past years, and imply that these antibiotics should no longer be recommended for treating S. pneumoniae infections. The widespread clinical use of macrolide antibiotics was strongly associated with the rise in resistance, and the major resistance mechanism is the acquisition of the erm(B) gene encoding a 23S methylase or the mef gene encoding an active (proton dependent) efflux pump (Cornick and Bentley, 2012). Besides, the high resistance rate of S. pneumoniae to clindamycin is noteworthy and further studies are needed to elucidate the exact resistance mechanism involved.
In terms of the distribution of antibiotic drug resistance across serotypes, serotypes 23F, 19F, 19A, and 14, exhibited higher resistance rates, while serotype 3 exhibited a relatively lower resistance rate. As previously mentioned, high levels of resistance in serotypes 23F, 19F, 19A, and 14 may be associated with the widespread international spread of ST81, ST271, ST320, and ST876, respectively. As for serotype 3, a similar resistance pattern was observed in Mexico, where 196 S. pneumoniae strains were susceptible to almost all the antibiotics tested, the predominant clonal complex group being CC180 (71.4%) (Echániz-Aviles et al., 2019). In our study, serotype 3 had a highly clonal diversity, dominated by ST505 (19.4%) and ST180 (19.4%). By whole genome sequencing of 616 strains of serotype 3 from England and Wales over a 15-year period, Groves et al. found that the composition of their clade changed along with shifts in antibiotic resistance (Groves et al., 2019). Thus, the clonal lineage and resistance pattern of serotype 3 should be closely monitored.
Another noticeable finding in this study was that the resistance rates of vaccine-covered serotypes were generally higher than those of non-vaccine-covered serotypes, which is in agreement with a previous report (Zhao et al., 2020). With the increasing number of non-vaccines covered serotypes causing IPD, the resistance levels of these serotypes should be carefully monitored under the dual pressure of antibiotics and vaccines.
An analysis of the annual antibiotic resistance rates showed a decrease from 2010 to 2015 for penicillin, cefuroxime, ceftriaxone, cefepime, cefaclor and trimethoprim/sulfamethoxazole. Regarding penicillin, we speculated that this phenomenon may be related to the penicillin breakpoint update in 2008 and the introduction of the PCV7 vaccine. Karlowsky et al. (2018) reported that 6001 S. pneumoniae isolates from Canada showed a small increase (P < 0.05) in susceptibility to penicillin and ceftriaxone during the period 2011–2015. In our study, the increased susceptibility of S. pneumoniae to cephalosporin antibiotics may be due to a decrease in serotypes 19A and 23F, which were resistant to a variety of antibiotics, including cephalosporins. Furthermore, a 20-year continuous global monitoring by the SENTRY Antimicrobial Surveillance Program (1997–2016) found that the susceptibility rates of ceftriaxone, erythromycin, clindamycin, tetracycline, and trimethoprim-sulfamethoxazole decreased in the first 12–14 years, and increased thereafter in the last 6–8 years (Sader et al., 2019). From a long-term point of view, a global collaboration with large samples and a prolonged time scale will help elucidate the current and changing trends of drug resistance to combat invasive infections caused by S. pneumoniae effectively.
Virulence is a key indicator for evaluating pathogenicity. We performed in vivo animal experiments to assess the virulence levels of the prevalent serotypes using the intraperitoneal infection model. Our study showed that serotypes 23F, 19A, 19F, and 14 strains were the prevalent avirulent serotypes while serotype 3 exhibited high virulence levels. However, the virulence levels of strains in serogroup 6 varied among serotypes and strains. A previous meta-analysis consisting of nine studies showed that the outcome of IPD was strongly associated with the serotype (Weinberger et al., 2010). In that study, serotypes 1, 7F, and 8 were associated with decreased risk ratio (RR) while serotypes 3, 6A, 6B, 9N, and 19F, were associated with increased relative risk (RR). Another study (Briles et al., 1992) demonstrated the strong association between capsular serotypes and virulence of the S. pneumoniae strains through in vivo virulence experiments in mice. Based on their observation, all serotype 4 strains, 40% of serotype 3 and 60% of serogroup 6, were virulent for mice, whilst strains of serogroups/types 14, 19, and 23, were avirulent. This was partly in line with our findings. Furthermore, according to our investigations, all serotype 3 strains from various clonal origins were virulent while all strains of serotypes 23F, 19F, 19A, and 14 from diverse clonal origins were avirulent, suggesting that the different capsular polysaccharides conferred pneumococci with intrinsically different virulence properties. Intriguingly, we found that strains of serotype 3 were characterized by high virulence and low resistance, while strains of serotypes 23F, 19F, 19A, and 14 were of low virulence and high resistance. Our findings are similar to those of Azoulay-Dupuis et al. (2000). In addition, a case-control study also showed that the clinical presentation of adult pneumonia caused by PNSP was milder than that of PSSP, suggesting that it is possible that resistance “carries a cost” (Einarsson et al., 1998). We speculated that strains of low virulent serotypes may be more prone to long-term colonization to acquire resistance through exposure to more antibiotics.
The virulence level of strains in serogroup 6 as per our mouse sepsis model was considerably complicated and complex, with great variation observed in the virulence levels of different strains within the same serotype (6A, 6B or 6C). Previous research (Briles et al., 1992) has shown that only 60% of strains in serogroup 6 were virulent for mice, which reinforced the heterogeneity of the virulence of serogroup 6 pneumococci. The fact that strains with the same serotype exhibit different virulence levels suggest presence of virulence determinants other than capsular polysaccharides. It is also worth noting that several studies (Polissi et al., 1998; Lau et al., 2001) using signature-tagged mutagenesis have unveiled a large set of genes that can influence the virulence of S. pneumoniae. Numerous non-capsular virulence genes, including ply, lytA, nanA, psaA, pspA and hylA, have been shown to be involved in the regulation of pneumococcal virulence (Ogunniyi et al., 2002; LeMessurier et al., 2006). Ply encoding pneumolysin, which can contribute to the early development of IPD by facilitating the invasion of S. pneumoniae from the lung into the bloodstream. Significantly, pneumolysin is a multifunctional toxin exhibiting both hemolytic and complement activities, and its deficiency would lead to a reduction in the virulence of the S. pneumoniae strain (Rubins et al., 1996). LytA, encoding an amidase with autolytic activity, exerts its virulence by releasing pneumolysin and inflammatory peptidoglycan from lysing bacteria (Kadioglu et al., 2008). It was reported that LytA-negative S. pneumoniae exhibited a lower virulence level through murine models of pneumonia and bacteremia (Canvin et al., 1995). All S. pneumoniae strains express nanA which encoding neuraminidase, it is essential for promoting S. pneumoniae adhesion to and invasion of uman brain microvascular endothelial cells (Uchiyama et al., 2009). PsaA is thought to encode a pneumococcal adhesin. In murine models of pneumonia, bacteremia and colonization, knockout of psaA abolished the virulence of S. pneumoniae (Marra et al., 2002). PspA impairs the fixation of complement component C3 on the surface of pneumococcal cells, thereby inhibiting complement-mediated opsonization. In addition, pspA encodes a lactoferrin-binding protein that protects bacteria from being killed by apolactoferrin (Kadioglu et al., 2008). HylA encodes the hyaluronate lyase enzyme which degrades hyaluronan, then destroying the structure of the connective tissue and exposing it to endo- and exogenous factors, including various bacterial toxins (Jedrzejas et al., 2002).
Therefore, the expression level of above genes amongst serogroup 6 strains was measured and compared. Our results revealed that the expression of virulence genes varied among these strains, but did not correspond significantly to the virulence phenotype of the in vivo experiments. This prompted us to think that pneumococcal virulence may be collaboratively regulated by multiple virulence genes, and that this regulation is highly complex and multifactorial. Robinson et al. (2002) identified the major clones of 212 S. pneumoniae strains from 39 countries by MLST, and the results indicated that the virulent serogroup 6 strains originated from the avirulent ancestral clones and went through multiple evolution to become virulent. It is possible that horizontal recombination or evolution of virulence genes between strains contributed to a diversity in virulence. Overall, our study suggests that capsular polysaccharide and non-capsular virulence genes are collectively responsible for the virulence diversity of S. pneumoniae strains.
This study has several limitations. Firstly, the strains collected in this study were from different time periods, which could lead to bias in the results. Secondly, the strains were mainly from tertiary teaching hospitals in China; samples from remote and rural areas were not included. Thirdly, the virulence of some of the rarer serotypes and non-vaccine covered serotypes were not evaluated.
Overall, our study provides a comprehensive insight into the epidemiological and virulence diversity of S. pneumoniae strains causing IPD in China. The most common serotypes in this study were 23F, 19A, 19F, 3, and 14. The serotype coverages of PCV7, PCV10, PCV13, and PPV23 vaccines on the bacterial collection were 42.3, 45.3, 73.3 and 79.3%, respectively. The most common STs were ST320, ST81, ST271, ST876, and ST3173. All strains were susceptible to ertapenem, levofloxacin, moxifloxacin, linezolid, and vancomycin, but highly resistant to macrolides and clindamycin (>95%). Serotype 3 strains were characterized by high virulence levels and low antimicrobial-resistance rates, while strains of serotypes 23F, 19F, 19A, and 14, exhibited low virulence and high resistance rates to antibiotics.
The original contributions presented in the study are included in the article/Supplementary Material, further inquiries can be directed to the corresponding author/s.
The animal study was reviewed and approved by the Medical Ethics Committee of Peking Union Medical College Hospital (No. S-263).
ZL, HZ, YX, and JZ conceived and designed the work. MZ, HA, CQ, BJ, and YW performed the experiments. MZ, ZW, LZ, and TK performed the data analysis and wrote the manuscript. All authors read and approved the final manuscript.
This work was supported by Special Foundation for National Science and Technology Basic Research Program of China (grant No. 2019FY101200), Beijing Key Clinical Specialty for Laboratory Medicine–Excellent Project (grant No. ZK201000), Graduate Innovation Fund of Peking Union Medical College (grant No. 2017-1002-1-21).
The authors declare that the research was conducted in the absence of any commercial or financial relationships that could be construed as a potential conflict of interest.
All claims expressed in this article are solely those of the authors and do not necessarily represent those of their affiliated organizations, or those of the publisher, the editors and the reviewers. Any product that may be evaluated in this article, or claim that may be made by its manufacturer, is not guaranteed or endorsed by the publisher.
The Supplementary Material for this article can be found online at: https://www.frontiersin.org/articles/10.3389/fmicb.2021.798750/full#supplementary-material
Azoulay-Dupuis, E., Rieux, V., Muffat-Joly, M., Bédos, J. P., Vallée, E., Rivier, C., et al. (2000). Relationship between capsular type, penicillin susceptibility, and virulence of human Streptococcus pneumoniae isolates in mice. Antimicrob. Agents Chemother. 44, 1575–1577. doi: 10.1128/aac.44.6.1575-1577.2000
Boulton, M. L., Ravi, N. S., Sun, X., Huang, Z., and Wagner, A. L. (2016). Trends in childhood pneumococcal vaccine coverage in Shanghai, China, 2005-2011: a retrospective cohort study. BMC Public Health 16:109. doi: 10.1186/s12889-016-2785-7
Briles, D. E., Crain, M. J., Gray, B. M., Forman, C., and Yother, J. (1992). Strong association between capsular type and virulence for mice among human isolates of Streptococcus pneumoniae. Infect. Immun. 60, 111–116. doi: 10.1128/iai.60.1.111-116.1992
Cai, K., Wang, Y., Guo, Z., Xu, X., Li, H., and Zhang, Q. (2018). Clinical characteristics and antimicrobial resistance of pneumococcal isolates of pediatric invasive pneumococcal disease in China. Infect. Drug Resist. 11, 2461–2469. doi: 10.2147/idr.S183916
Canvin, J. R., Marvin, A. P., Sivakumaran, M., Paton, J. C., Boulnois, G. J., Andrew, P. W., et al. (1995). The role of pneumolysin and autolysin in the pathology of pneumonia and septicemia in mice infected with a type 2 pneumococcus. J. Infect. Dis. 172, 119–123. doi: 10.1093/infdis/172.1.119
Ceyhan, M., Aykac, K., Gurler, N., Ozsurekci, Y., Öksüz, L., and Altay Akısoglu, Ö, et al. (2020). Serotype distribution of Streptococcus pneumonia in children with invasive disease in Turkey: 2015-2018. Hum. Vaccin. Immunother. 16, 2773–2778. doi: 10.1080/21645515.2020.1747931
Chen, K., Zhang, X., Shan, W., Zhao, G., and Zhang, T. (2018). Serotype distribution of Streptococcus pneumoniae and potential impact of pneumococcal conjugate vaccines in China: a systematic review and meta-analysis. Hum. Vaccin. Immunother. 14, 1453–1463. doi: 10.1080/21645515.2018.1435224
Chen, Y. Y., Wang, J. T., Lin, T. L., Gong, Y. N., Li, T. H., Huang, Y. Y., et al. (2019). Prophage excision in Streptococcus pneumoniae serotype 19A ST320 promote colonization: insight into its evolution from the Ancestral Clone Taiwan 19F-14 (ST236). Front. Microbiol. 10:205. doi: 10.3389/fmicb.2019.00205
CLSI (2019). Performance Standards for Antimicrobial Susceptibility Testing, 29th Edn, CLSI Supplement M100. Wayne, PA: Clinical and Laboratory Standards Institute.
Cornick, J. E., and Bentley, S. D. (2012). Streptococcus pneumoniae: the evolution of antimicrobial resistance to beta-lactams, fluoroquinolones and macrolides. Microbes Infect. 14, 573–583. doi: 10.1016/j.micinf.2012.01.012
Demczuk, W. H., Martin, I., Griffith, A., Lefebvre, B., McGeer, A., Lovgren, M., et al. (2013). Serotype distribution of invasive Streptococcus pneumoniae in Canada after the introduction of the 13-valent pneumococcal conjugate vaccine, 2010-2012. Can. J. Microbiol. 59, 778–788. doi: 10.1139/cjm-2013-0614
Echániz-Aviles, G., Guerreiro, S. I., Silva-Costa, C., Mendes, C. I., Carriço, J. A., Carnalla-Barajas, M. N., et al. (2019). Streptococcus pneumoniae serotype 3 in Mexico (1994 to 2017): decrease of the unusual clonal complex 4909 lineage following PCV13 introduction. J. Clin. Microbiol. 57:e01354-18., doi: 10.1128/jcm.01354-18
Einarsson, S., Kristjansson, M., Kristinsson, K. G., Kjartansson, G., and Jonsson, S. (1998). Pneumonia caused by penicillin-non-susceptible and penicillin-susceptible pneumococci in adults: a case-control study. Scand. J. Infect. Dis. 30, 253–256. doi: 10.1080/00365549850160882
Esposito, S., Principi, N., and Cornaglia, G. (2014). Barriers to the vaccination of children and adolescents and possible solutions. Clin. Microbiol. Infect. 20 Suppl 5, 25–31. doi: 10.1111/1469-0691.12447
Ganaie, F., Saad, J. S., McGee, L., van Tonder, A. J., Bentley, S. D., Lo, S. W., et al. (2020). A new pneumococcal capsule type, 10D, is the 100th serotype and has a large cps fragment from an oral streptococcus. mBio 11:e00937-20. doi: 10.1128/mBio.00937-20
Geno, K. A., Gilbert, G. L., Song, J. Y., Skovsted, I. C., Klugman, K. P., Jones, C., et al. (2015). Pneumococcal capsules and their types: past, present, and future. Clin. Microbiol. Rev. 28, 871–899. doi: 10.1128/cmr.00024-15
Golden, A. R., Baxter, M. R., Davidson, R. J., Martin, I., Demczuk, W., Mulvey, M. R., et al. (2019). Comparison of antimicrobial resistance patterns in Streptococcus pneumoniae from respiratory and blood cultures in Canadian hospitals from 2007-16. J. Antimicrob. Chemother. 74(Suppl 4), iv39–iv47. doi: 10.1093/jac/dkz286
Groves, N., Sheppard, C. L., Litt, D., Rose, S., Silva, A., Njoku, N., et al. (2019). Evolution of Streptococcus pneumoniae serotype 3 in England and Wales: a major vaccine evader. Genes (Basel) 10:845. doi: 10.3390/genes10110845
He, M., Yao, K., Shi, W., Gao, W., Yuan, L., Yu, S., et al. (2015). Dynamics of serotype 14 Streptococcus pneumoniae population causing acute respiratory infections among children in China (1997-2012). BMC Infect. Dis. 15:266. doi: 10.1186/s12879-015-1008-7
Hsieh, Y. C., Lin, T. L., Chang, K. Y., Huang, Y. C., Chen, C. J., Lin, T. Y., et al. (2013). Expansion and evolution of Streptococcus pneumoniae serotype 19A ST320 clone as compared to its ancestral clone, Taiwan19F-14 (ST236). J. Infect. Dis. 208, 203–210. doi: 10.1093/infdis/jit145
Isaacman, D. J., McIntosh, E. D., and Reinert, R. R. (2010). Burden of invasive pneumococcal disease and serotype distribution among Streptococcus pneumoniae isolates in young children in Europe: impact of the 7-valent pneumococcal conjugate vaccine and considerations for future conjugate vaccines. Int. J. Infect. Dis. 14, e197–e209. doi: 10.1016/j.ijid.2009.05.010
Jedrzejas, M. J., Mello, L. V., de Groot, B. L., and Li, S. (2002). Mechanism of hyaluronan degradation by Streptococcus pneumoniae hyaluronate lyase. Structures of complexes with the substrate. J. Biol. Chem. 277, 28287–28297. doi: 10.1074/jbc.M112009200
Jefferies, J. M. C., Tee, W. S. N., and Clarke, S. C. (2011). Molecular analysis of Streptococcus pneumoniae clones causing invasive disease in children in Singapore. J. Med. Microbiol. 60(Pt 6), 750–755. doi: 10.1099/jmm.0.030007-0
Jourdain, S., Drèze, P. A., Vandeven, J., Verhaegen, J., Van Melderen, L., and Smeesters, P. R. (2011). Sequential multiplex PCR assay for determining capsular serotypes of colonizing S. pneumoniae. BMC Infect. Dis. 11:100. doi: 10.1186/1471-2334-11-100
Kadioglu, A., Weiser, J. N., Paton, J. C., and Andrew, P. W. (2008). The role of Streptococcus pneumoniae virulence factors in host respiratory colonization and disease. Nat. Rev. Microbiol. 6, 288–301. doi: 10.1038/nrmicro1871
Karlowsky, J. A., Adam, H. J., Golden, A. R., Baxter, M. R., Nichol, K. A., Martin, I., et al. (2018). Antimicrobial susceptibility testing of invasive isolates of Streptococcus pneumoniae from Canadian patients: the SAVE study, 2011-15. J. Antimicrob. Chemother. 73(suppl_7), vii5–vii11. doi: 10.1093/jac/dky156
Kim, S. H., Chung, D. R., Song, J. H., Baek, J. Y., Thamlikitkul, V., Wang, H., et al. (2020). Changes in serotype distribution and antimicrobial resistance of Streptococcus pneumoniae isolates from adult patients in Asia: emergence of drug-resistant non-vaccine serotypes. Vaccine 38, 6065–6073. doi: 10.1016/j.vaccine.2019.09.065
Lalitha, M. K., Thomas, K., Kumar, R. S., and Steinhoff, M. C. (1999). Serotyping of Streptococcus pneumoniae by coagglutination with 12 pooled antisera. J. Clin. Microbiol. 37, 263–265. doi: 10.1128/jcm.37.1.263-265.1999
Lau, G. W., Haataja, S., Lonetto, M., Kensit, S. E., Marra, A., Bryant, A. P., et al. (2001). A functional genomic analysis of type 3 Streptococcus pneumoniae virulence. Mol. Microbiol. 40, 555–571. doi: 10.1046/j.1365-2958.2001.02335.x
LeMessurier, K. S., Ogunniyi, A. D., and Paton, J. C. (2006). Differential expression of key pneumococcal virulence genes in vivo. Microbiology (Reading) 152(Pt 2), 305–311. doi: 10.1099/mic.0.28438-0
Li, Q. H., Yao, K. H., Yu, S. J., Ma, X., He, M. M., Shi, W., et al. (2013). Spread of multidrug-resistant clonal complex 271 of serotype 19F Streptococcus pneumoniae in Beijing, China: characterization of serotype 19F. Epidemiol. Infect. 141, 2492–2496. doi: 10.1017/s0950268813000514
Li, X. X., Xiao, S. Z., Gu, F. F., Zhao, S. Y., Xie, Q., Sheng, Z. K., et al. (2019). Serotype distribution, antimicrobial susceptibility, and Multilocus Sequencing Type (MLST) of Streptococcus pneumoniae from adults of three hospitals in Shanghai, China. Front. Cell. Infect Microbiol. 9:407. doi: 10.3389/fcimb.2019.00407
Liang, Q., Li, G. F., and Zhu, F. C. (2016). Vaccine profile of PPV23: Beijing Minhai Biotech 23-valent pneumococcal vaccine. Expert. Rev. Vaccines 15, 1351–1359. doi: 10.1080/14760584.2016.1239536
Ma, X., Yao, K. H., Yu, S. J., Zhou, L., Li, Q. H., Shi, W., et al. (2013). Genotype replacement within serotype 23F Streptococcus pneumoniae in Beijing, China: characterization of serotype 23F. Epidemiol. Infect. 141, 1690–1696. doi: 10.1017/s0950268812002269
Marra, A., Lawson, S., Asundi, J. S., Brigham, D., and Hromockyj, A. E. (2002). In vivo characterization of the psa genes from Streptococcus pneumoniae in multiple models of infection. Microbiology (Reading) 148(Pt 5), 1483–1491. doi: 10.1099/00221287-148-5-1483
Marrie, T. J., Tyrrell, G. J., Majumdar, S. R., and Eurich, D. T. (2018). Effect of age on the manifestations and outcomes of invasive pneumococcal disease in adults. Am. J. Med. 131, 100.e1–100.e7. doi: 10.1016/j.amjmed.2017.06.039
Melin, M., Jarva, H., Siira, L., Meri, S., Käyhty, H., and Väkeväinen, M. (2009). Streptococcus pneumoniae capsular serotype 19F is more resistant to C3 deposition and less sensitive to opsonophagocytosis than serotype 6B. Infect. Immun. 77, 676–684. doi: 10.1128/iai.01186-08
Moore, C. E., Giess, A., Soeng, S., Sar, P., Kumar, V., Nhoung, P., et al. (2016). Characterisation of invasive Streptococcus pneumoniae isolated from Cambodian children between 2007 – 2012. PLoS One 11:e0159358. doi: 10.1371/journal.pone.0159358
Muñoz, R., Coffey, T. J., Daniels, M., Dowson, C. G., Laible, G., Casal, J., et al. (1991). Intercontinental spread of a multiresistant clone of serotype 23F Streptococcus pneumoniae. J. Infect. Dis. 164, 302–306. doi: 10.1093/infdis/164.2.302
Muñoz-Almagro, C., Ciruela, P., Esteva, C., Marco, F., Navarro, M., Bartolome, R., et al. (2011). Serotypes and clones causing invasive pneumococcal disease before the use of new conjugate vaccines in Catalonia. Spain. J. Infect. 63, 151–162. doi: 10.1016/j.jinf.2011.06.002
O’Brien, K. L., Wolfson, L. J., Watt, J. P., Henkle, E., Deloria-Knoll, M., McCall, N., et al. (2009). Burden of disease caused by Streptococcus pneumoniae in children younger than 5 years: global estimates. Lancet 374, 893–902. doi: 10.1016/S0140-6736(09)61204-6
Ogunniyi, A. D., Giammarinaro, P., and Paton, J. C. (2002). The genes encoding virulence-associated proteins and the capsule of Streptococcus pneumoniae are upregulated and differentially expressed in vivo. Microbiology (Reading) 148(Pt 7), 2045–2053. doi: 10.1099/00221287-148-7-2045
Polissi, A., Pontiggia, A., Feger, G., Altieri, M., Mottl, H., Ferrari, L., et al. (1998). Large-scale identification of virulence genes from Streptococcus pneumoniae. Infect. Immun. 66, 5620–5629. doi: 10.1128/iai.66.12.5620-5629.1998
Poolman, J., Frasch, C., Nurkka, A., Käyhty, H., Biemans, R., and Schuerman, L. (2011). Impact of the conjugation method on the immunogenicity of Streptococcus pneumoniae serotype 19F polysaccharide in conjugate vaccines. Clin. Vaccine Immunol. 18, 327–336. doi: 10.1128/cvi.00402-10
Reinert, R. R., van der Linden, M., Seegmüller, I., Al-Lahham, A., Siedler, A., Weissmann, B., et al. (2007). Molecular epidemiology of penicillin-non-susceptible Streptococcus pneumoniae isolates from children with invasive pneumococcal disease in Germany. Clin. Microbiol. Infect. 13, 363–368. doi: 10.1111/j.1469-0691.2006.01676.x
Richter, S. S., Heilmann, K. P., Dohrn, C. L., Riahi, F., Diekema, D. J., and Doern, G. V. (2013). Pneumococcal serotypes before and after introduction of conjugate vaccines, United States, 1999-2011(1.). Emerg. Infect. Dis. 19, 1074–1083. doi: 10.3201/eid1907.121830
Robinson, D. A., Briles, D. E., Crain, M. J., and Hollingshead, S. K. (2002). Evolution and virulence of serogroup 6 pneumococci on a global scale. J. Bacteriol. 184, 6367–6375. doi: 10.1128/jb.184.22.6367-6375.2002
Rubins, J. B., Charboneau, D., Fasching, C., Berry, A. M., Paton, J. C., Alexander, J. E., et al. (1996). Distinct roles for pneumolysin’s cytotoxic and complement activities in the pathogenesis of pneumococcal pneumonia. Am. J. Respir. Crit. Care Med. 153(4 Pt 1), 1339–1346. doi: 10.1164/ajrccm.153.4.8616564
Sader, H. S., Mendes, R. E., Le, J., Denys, G., Flamm, R. K., and Jones, R. N. (2019). Antimicrobial susceptibility of Streptococcus pneumoniae from North America, Europe, Latin America, and the Asia-Pacific Region: results from 20 years of the SENTRY antimicrobial surveillance program (1997–2016). Open Forum Infect. Dis. 6(Suppl 1), S14–S23. doi: 10.1093/ofid/ofy263
Shi, W., Li, J., Dong, F., Qian, S., Liu, G., Xu, B., et al. (2019). Serotype distribution, antibiotic resistance pattern, and multilocus sequence types of invasive Streptococcus pneumoniae isolates in two tertiary pediatric hospitals in Beijing prior to PCV13 availability. Expert. Rev. Vaccines 18, 89–94. doi: 10.1080/14760584.2019.1557523
Shin, J., Baek, J. Y., Kim, S. H., Song, J. H., and Ko, K. S. (2011). Predominance of ST320 among Streptococcus pneumoniae serotype 19A isolates from 10 Asian countries. J. Antimicrob. Chemother. 66, 1001–1004. doi: 10.1093/jac/dkr048
Slotved, H. C., Dalby, T., and Hoffmann, S. (2016). The effect of pneumococcal conjugate vaccines on the incidence of invasive pneumococcal disease caused by ten non-vaccine serotypes in Denmark. Vaccine 34, 769–774. doi: 10.1016/j.vaccine.2015.12.056
Sousa, A., Pérez-Rodríguez, M. T., Nodar, A., Martínez-Lamas, L., Vasallo, F. J., Álvarez-Fernández, M., et al. (2018). Clinical and microbiological characteristics of unusual manifestations of invasive pneumococcal disease. Enferm. Infecc. Microbiol. Clin. 36, 284–289. doi: 10.1016/j.eimc.2017.05.003
Uchiyama, S., Carlin, A. F., Khosravi, A., Weiman, S., Banerjee, A., Quach, D., et al. (2009). The surface-anchored NanA protein promotes pneumococcal brain endothelial cell invasion. J. Exp. Med. 206, 1845–1852. doi: 10.1084/jem.20090386
Varghese, R., Neeravi, A., Subramanian, N., Pavithra, B., Kavipriya, A., Kumar, J. L., et al. (2019). Clonal similarities and sequence-type diversity of invasive and carriage Streptococcus pneumoniae in India among children under 5 years. Indian J. Med. Microbiol. 37, 358–362. doi: 10.4103/ijmm.IJMM_19_348
Weinberger, D. M., Harboe, Z. B., Sanders, E. A., Ndiritu, M., Klugman, K. P., Rückinger, S., et al. (2010). Association of serotype with risk of death due to pneumococcal pneumonia: a meta-analysis. Clin. Infect. Dis. 51, 692–699. doi: 10.1086/655828
Xue, L., Yao, K., Xie, G., Zheng, Y., Wang, C., Shang, Y., et al. (2010). Serotype distribution and antimicrobial resistance of Streptococcus pneumoniae isolates that cause invasive disease among Chinese children. Clin. Infect. Dis. 50, 741–744. doi: 10.1086/650534
Yanagihara, K., Kosai, K., Mikamo, H., Mukae, H., Takesue, Y., Abe, M., et al. (2021). Serotype distribution and antimicrobial susceptibility of Streptococcus pneumoniae associated with invasive pneumococcal disease among adults in Japan. Int. J. Infect. Dis. 102, 260–268. doi: 10.1016/j.ijid.2020.10.017
Zhao, C., Xie, Y., Zhang, F., Wang, Z., Yang, S., Wang, Q., et al. (2020). Investigation of antibiotic resistance, serotype distribution, and genetic characteristics of 164 invasive Streptococcus pneumoniae from North China between April 2016 and October 2017. Infect. Drug Resist. 13, 2117–2128. doi: 10.2147/idr.S256663
Zhou, X., Liu, J., Zhang, Z., Liu, Y., Wang, Y., and Liu, Y. (2016). Molecular characteristics of penicillin-binding protein 2b, 2x and 1a sequences in Streptococcus pneumoniae isolates causing invasive diseases among children in Northeast China. Eur. J. Clin. Microbiol. Infect. Dis. 35, 633–645. doi: 10.1007/s10096-016-2582-3
Keywords: Streptococcus pneumoniae, serotype distribution, molecular epidemiology, antimicrobial susceptibility, virulence
Citation: Zhou M, Wang Z, Zhang L, Kudinha T, An H, Qian C, Jiang B, Wang Y, Xu Y, Liu Z, Zhang H and Zhang J (2022) Serotype Distribution, Antimicrobial Susceptibility, Multilocus Sequencing Type and Virulence of Invasive Streptococcus pneumoniae in China: A Six-Year Multicenter Study. Front. Microbiol. 12:798750. doi: 10.3389/fmicb.2021.798750
Received: 20 October 2021; Accepted: 08 December 2021;
Published: 13 January 2022.
Edited by:
Mattias Collin, Lund University, SwedenReviewed by:
Luchang Zhu, Houston Methodist Research Institute, United StatesCopyright © 2022 Zhou, Wang, Zhang, Kudinha, An, Qian, Jiang, Wang, Xu, Liu, Zhang and Zhang. This is an open-access article distributed under the terms of the Creative Commons Attribution License (CC BY). The use, distribution or reproduction in other forums is permitted, provided the original author(s) and the copyright owner(s) are credited and that the original publication in this journal is cited, in accordance with accepted academic practice. No use, distribution or reproduction is permitted which does not comply with these terms.
*Correspondence: Zhengyin Liu, emhlbmd5aW5sQGhvdG1haWwuY29t; Hong Zhang, emhhbmdob25nMzAxMEB2aXAuMTI2LmNvbQ==; Jingren Zhang, emhhbmdsYWJAbWFpbC50c2luZ2h1YS5lZHUuY24=
†These authors have contributed equally to this work
Disclaimer: All claims expressed in this article are solely those of the authors and do not necessarily represent those of their affiliated organizations, or those of the publisher, the editors and the reviewers. Any product that may be evaluated in this article or claim that may be made by its manufacturer is not guaranteed or endorsed by the publisher.
Research integrity at Frontiers
Learn more about the work of our research integrity team to safeguard the quality of each article we publish.