- 1Section for Food Safety and Animal Health Research, Department of Animal Health, Welfare and Food Safety, Norwegian Veterinary Institute, Oslo, Norway
- 2Section for Epidemiology, Department of Animal Health, Welfare and Food Safety, Norwegian Veterinary Institute, Oslo, Norway
There are knowledge gaps concerning dynamics of extended-spectrum cephalosporin (ESC)-resistant Escherichia coli and their plasmids in broiler production and the persistence of strains on broiler farms. Thus, we aimed at characterising ESC-resistant Escherichia coli collected from all flocks reared on 10 different farms during a six-months sampling period. All isolates (n = 43) were subjected to whole-genome sequencing, and a subset of isolates (n = 7) were also sequenced using oxford nanopore technology and subsequent hybrid assembly in order to do in-depth characterisation of the ESC resistance plasmids. The 43 isolates belonged to 11 different sequence types, and three different ESC resistance gene/plasmid combinations were present, namely, IncK2/blaCMY-2 (n = 29), IncI1/blaCMY-2 (n = 6) and IncI1/blaCTX-M-1 (n = 8). ESC-resistant E. coli of different STs and with different ESC resistance gene/plasmid combinations could be present on the same farm, while a single ST and ESC resistance gene/plasmid displaying zero or few SNP differences were present on other farms. In-depth characterisation of IncK2/blaCMY-2 plasmids revealed that at least two distinct variants circulate in the broiler production. These plasmids showed close homology to previously published plasmids from other countries. Our longitudinal study show that ESC-resistant E. coli belong to a multitude of different STs and that different ESC resistance genes and plasmids occur. However, there is also indication of persistence of both ESC-resistant E. coli strains and IncK2/blaCMY-2 plasmids on farms. Further studies are warranted to determine the dynamics of strains, plasmids and ESC resistance genes within single broiler flocks.
Introduction
In Norway, the situation regarding occurrence of antimicrobial resistance (AMR) in bacteria of animal origin is favourable (EFSA and ECDC, 2020). Moreover, selection pressure from antimicrobial use in Norwegian broiler production is almost non-existing (Animalia, 2015, 2016, 2017, 2018; Refsum, 2015). Despite this, the broiler production has been associated with occurrence of Escherichia coli displaying resistance to extended-spectrum cephalosporins (ESC; Sunde et al., 2009; Mo et al., 2014, 2019). ESC-resistant E. coli has been introduced with imported breeding material, and subsequently been disseminated through the breeding pyramid (Mo et al., 2014; Myrenås et al., 2018). The odds of a broiler flock being positive have been shown to be significantly higher if the previous flock in the same house was positive (Mo et al., 2016a, 2019). Thus, local re-circulation on farm was suggested as a reason for persistence of ESC-resistant E. coli in Norwegian broiler production. However, we have limited knowledge on the genetic relatedness between ESC-resistant E. coli isolated from consecutive flocks at the same farm, except the gene encoding ESC resistance (Mo et al., 2016a, 2019).
Thus, in this study, we aimed to do in-depth characterisation of ESC-resistant E. coli and their resistance plasmids originating from broiler flocks in houses with multiple positive flocks during a six-months sampling period. This will help us understand the dynamic of ESC-resistant E. coli in broiler houses and on farms. Selected isolates were subjected to long-read sequencing and plasmid characterisation in order to investigate the similarity between ESC resistance plasmids present in different E. coli multilocus sequence types (STs) from flocks reared in the same broiler house. Detailed knowledge of the plasmids can reveal whether they persist and are able to disseminate between different host strains circulating in the broiler production environment.
Materials and Methods
Bacterial Isolates
During May–October 2016, boot-swab and dust samples were collected from all broiler flocks reared in Norway (n = 2,110). The samples were analysed for the presence of ESC-resistant Enterobacteriaceae by plating on MacConkey agar (BD Difco, Beckton, Dickinson and company, Le Pont de Claire, France) supplemented with 1 mg/l cefotaxime (Duchefa, Haarlem, Netherlands) after pre-enrichment in buffered peptone water (BPW-ISO; Mo et al., 2019). This sampling strategy allowed longitudinal sampling on all Norwegian broiler farms (n = 701) for a period of 6 months. In the current study, we have included farms with three or more positive flocks (i.e., E. coli with plasmid-mediated ESC resistance detected in the flock) in one house during the sampling period. If an included producer had more than one house on the farm, any positive flocks from other houses on the farm were also included. If an included flock was sampled at multiple time points, the first positive sample was included. From each positive flock, one ESC-resistant E. coli was included for further analysis. In total, ESC-resistant E. coli from 43 unique broiler flocks were included, originating from 14 houses on 10 different broiler farms, named A to J in the following (Figure 1). The genetic background for ESC resistance and minimum inhibitory concentrations for a panel of antimicrobials were determined in a previous study (Mo et al., 2019; Figure 1 and Supplementary Table S1).
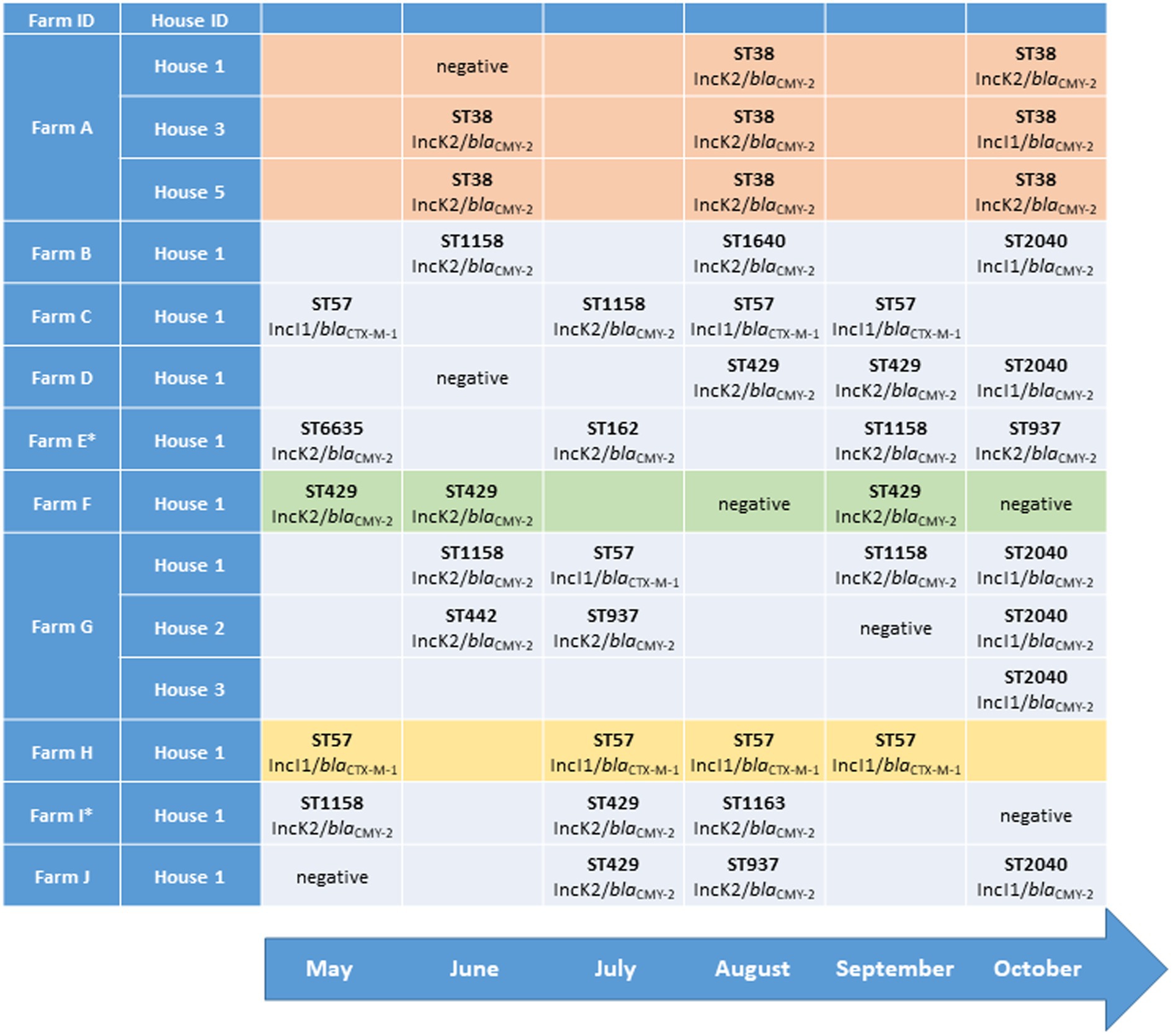
Figure 1. Multilocus sequence type (MLST), plasmid replicon and extended-spectrum cephalosporin (ESC) resistance gene associated with ESC-resistant Escherichia coli isolated from different broiler flocks on 10 broiler farms sampled during May–October 2016.
DNA Isolation and Whole-Genome Sequencing
Short-Read Sequencing
DNA for short-read sequencing of 35 isolates was extracted using the QIAmp DNA mini kit (Qiagen) according to the manufacturers’ protocol. The remaining eight isolates were sequenced in a previous study (Mo et al., 2020a) and the raw reads were available for inclusion in this study (accession number PRJEB45077). The purity of the DNA was determined on a Nanodrop 2000 (Thermo Fischer Scientific) and the DNA concentration was measured on a Qubit™ fluorometer (Thermo Fischer Scientific) using the Qubit dsDNA BroadRange assay kit (Thermo Fischer Scientific). Isolates were prepared with the Nextera Flex library preparation kit (Illumina) and sequenced on an Illumina HiSeq X platform, resulting in 150 bp paired-end reads.
Long-Read Sequencing
Isolates from Farms E (n = 4) and I (n = 3) were subjected to long-read sequencing in order to investigate the similarity between ESC resistance plasmids in E. coli of different STs originating from the same farm (Figure 1). DNA was extracted using the Gentra Puregene Yeast/Bact. kit (Qiagen). DNA purity and concentration were determined as described for short-read sequencing. Isolates were prepared with the SQK-RBK004 rapid barcoding kit (Oxford Nanopore Sequencing [Oxford Nanopore Technology], Oxford, United Kingdom) and sequenced using a FLO-MIN106 flow cell on a MinION device (Oxford Nanopore Sequencing).
In silico Analysis
Analysis of Short-Read Sequences
The Bifrost pipeline (Lagesen, 2020) was used for initial quality control and assembly of raw reads as previously described (Mo et al., 2020b).
All 43 isolates were further characterised using the ELLIPSIS pipeline (Kaspersen, 2021) with draught assemblies as input. The multilocus sequence type (MLST) was determined for each isolate (Larsen et al., 2012) using the Achtman scheme (Wirth et al., 2006). MOB-suite v 3.0.1 (Robertson and Nash, 2018) was used to predict plasmids and chromosomes, and determine the replicon type for each predicted plasmid. The FASTA files outputted by MOB-suite were annotated using Prokka v 1.14.5 (Seemann, 2014) and used as input to ResFinder v 3.2 (Zankari et al., 2012) and PlasmidFinder v 2.1 (Carattoli et al., 2014) to identify AMR genes and plasmid replicons, respectively. When applicable, plasmid MLST (pMLST) was also determined using pMLST finder v.0.1.0 (Carattoli et al., 2014).
Sequence types represented by at least three isolates were included in single-nucleotide polymorphism (SNP) analysis using Snippy1 in order to determine the genetic relatedness between isolates. The fastANI tool (Jain et al., 2018) was used to compare isolates of the same ST to identify a suitable reference to include in the SNP analysis. An isolate with a high similarity to the other isolates belonging to the same ST and good assembly statistics was used as reference.
Analysis of Long-Read Sequences
Raw sequence data from the ONT sequencing were prepared as previously described (Kaspersen et al., 2021). Briefly, base calling was done using Guppy v.3.4.5 (Ueno et al., 2003), demultiplexing using qcat v.1.1.0 (ONT2) and sequence quality of demultiplexed datasets examined using NanoPlot v 1.30.0 (De Coster et al., 2018).
Both short and long reads were used as input in the ELLIPSIS pipeline (Kaspersen, 2021) for the seven isolates subjected to ONT sequencing. Hybrid assemblies were created using Unicycler v.0.4.8 with bold settings (Wick et al., 2017) after removal of reads <1,000 bp using Filtlong v.0.2.0.3 QUAST v.5.0.2 (Gurevich et al., 2013) was used for quality control of assemblies. Thereafter, MOB-suite, Prokka, ResFinder and PlasmidFinder were run as described in the previous section.
To determine if identical or highly similar plasmids were present in E. coli of different STs obtained from the same farm, blaCMY-2 carrying plasmids from the seven ONT sequenced isolates were further compared on farm level. Information regarding number of contigs, total plasmid size (bp), circularity and presence of AMR genes was evaluated (Supplementary Table S2). On Farm E, three plasmids ranging in size from 85,173 to 85,922 bp were compared, while two plasmids of 117,010 bp and 118,095 bp were compared on Farm I. One plasmid from each farm was excluded from the comparisons, as the initial plasmid characterisation showed that the lengths differed from the remaining plasmids on the farms. The plasmid sequences were annotated with Prokka (Seemann, 2014) using the previously published plasmid pDV45 (accession number KR905384) as reference. The annotations were manually curated in CLC Main Workbench 8.0 (CLC bio, Qiagen, Hilden, Germany). Furthermore, we used Snippy4 to identify number and location of SNPs in the core genome of the plasmids. For each farm, the gbk file from the longest plasmid, namely, p16449 and p18539, was used as a reference for the Snippy analysis. The plasmid comparisons were visualised using the BRIG (BLAST Ring Image Generator) programme (Alikhan et al., 2011).
Results
E. coli ST Variability and Genetic Relatedness Between Isolates
In total, 11 different STs were identified among the 43 included isolates (Figure 1). In eight houses (57.1%), all flocks reared during the sampling period were positive for ESC-resistant E. coli (Figure 1; Table 1). In five of the 14 houses included in the study (35.7%), all ESC-resistant E. coli detected belonged to a single ST throughout the sampling period (Figure 1). Three of these houses were on Farm A, with E. coli ST38 present in all flocks (n = 8). Eight out of nine (88.9%) flocks reared on Farm A were positive. In the remaining nine houses included (64.3%), we detected ESC-resistant E. coli belonging to two-four different STs.
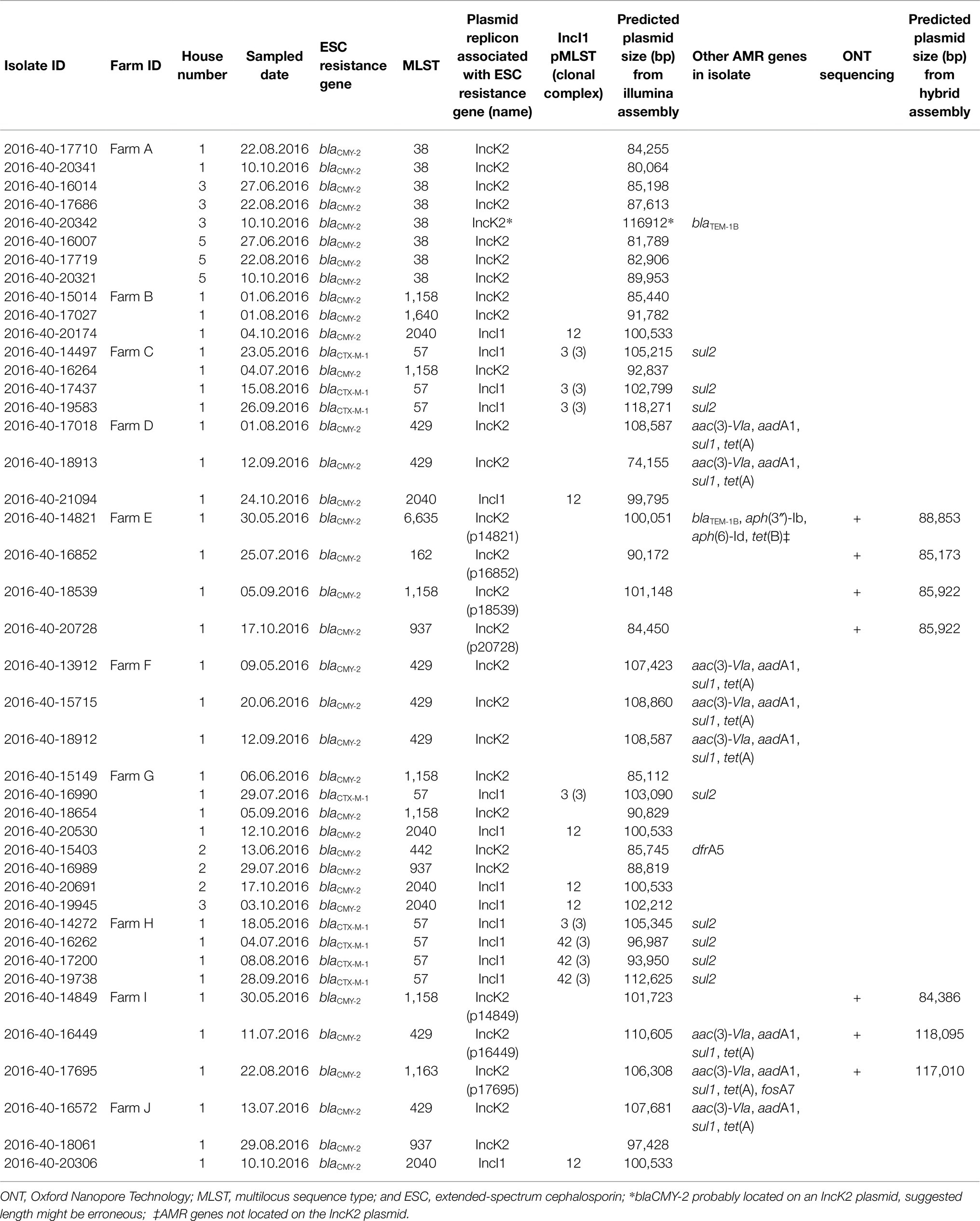
Table 1. Overview of characteristics associated with extended-spectrum cephalosporin-resistant Escherichia coli originating from 42 broiler flocks reared on 10 broiler farms in Norway during May–October 2016.
All ST38 isolates (n = 8) originated from flocks reared in three different houses on Farm A. The SNP analysis showed that the isolates differed by 6–55 SNPs, with 13 or more SNP differences between six of the eight isolates. E. coli ST57 (n = 8) was detected from three different farms and differed by 0–21 SNPs. Three ST57 isolates from Farm C displayed no SNP differences, while four isolates from Farm H differed by 1–6 SNPs. Two ST429 isolates from Farm D differed by 6 SNPs, while three isolates from Farm F differed by 2–6 SNPs. ST1158 isolates were repeatedly recovered only from House 1 on Farm G, and 23 SNP differences were present between the isolates. In addition, E. coli ST1158 were isolated from four other farms, displaying up to 120 SNP differences. Isolates belonging to ST2040 were only detected in the last sampling month, and consecutive isolates from the same farm were therefore not obtained. Three ST2040 isolates were from three different houses on Farm G, and these isolates differed by 4–8 SNPs. Three ST937 isolates from three different farms differed by 23–37 SNPs. An overview of SNP differences present between isolates from the same ST is presented in the supplementary material (Supplementary Tables S3A-F).
Co-resistance to Other Antimicrobials
In 24 (55.8%) of the included isolates, blaCMY-2 was the only AMR gene detected. The most common co-resistance observed among the isolates was sulfamethoxazole resistance (n = 16, 37.2%) encoded by either sul1 in blaCMY-2 carrying isolates (n = 8, 18.6%) or sul2 in blaCTX-M-1 carrying isolates (n = 8, 18.6%). Also, co-resistance towards aminoglycosides (n = 9, 20.9%) or tetracycline (n = 8, 18.6%) was observed. Aminoglycoside resistance was associated with the presence of aac and aad (n = 8, 18.6%) or aph (strA/strB) genes (n = 1, 2.3%), while tetracycline resistance was encoded by tet(A) (n = 8, 18.6%) or tet(B) (n = 1, 2.3%; Table 1).
ESC Resistance Plasmids
The majority of isolates (n = 35, 81.4%) carried the blaCMY-2 gene encoding ESC resistance. blaCMY-2 was frequently associated with IncK2 plasmids (n = 29) but was also found on IncI1 plasmids (n = 6). In eight isolates (18.6%), ESC resistance was mediated by blaCTX-M-1 (Figure 1; Table 1). The blaCTX-M-1 gene was previously found to be associated with IncI1-Iγ plasmids in all isolates (Mo et al., 2020b). IncK2/blaCMY-2 plasmids were detected in nine different STs, with a predicted size of 74–110 kb. IncI1/blaCMY-2 plasmids and IncI1/blaCTX-M-1 plasmids were only found in unique STs, namely, ST2040 and ST57, respectively. The predicted lengths were approximately 100 kb for IncI1/blaCMY-2 plasmids and 94–118 kb for IncI1/blaCTX-M-1 plasmids (Figure 1; Table 1). In a single ST38 isolate (2016-40-20342), MOB-suite predicted blaCMY-2 to be located on an IncI1 plasmid. The contig encoding blaCMY-2 was subjected to a BLAST search and showed 100% identity to previously published IncK2 plasmid sequences. Thus, we assumed that it was erroneously classified as an IncI1 plasmid and that blaCMY-2 was associated to an IncK2 plasmid in this isolate.
In order to investigate possible on farm plasmid persistence and transfer between E. coli host strains, we performed detailed characterisation of selected ESC resistance plasmids. All isolates from Farms E and I (n = 7) were subjected to long-read sequencing and subsequent analysis. Farms E and I were chosen since IncK plasmids with blaCMY-2 were present in E. coli belonging to four and three different STs, respectively (Figure 1; Table 1). Hybrid assemblies with subsequent plasmid characterisation and comparison revealed that all four isolates on Farm E harboured IncK2 plasmids with blaCMY-2 as the only AMR gene, ranging from 85,173 to 88,853 bp in size (Table 1). The plasmids were named p14821, p16852, p18539 and p20728. Plasmids p18539 and p20728 from Farm E differed by eight SNPs. One SNP was in the region encoding the relE gene, five SNPs in non-coding regions and two SNPs in regions encoding uncharacterized proteins (YfeC and YffA). Between p16852 and p18539, 15 SNPs were present in the core genome (98.1%). Four of the SNPs were in regions encoding uncharacterized proteins (YfaB), five SNPs in non-coding sequences, four SNPs were in the impCAB operon, one SNP in the relE gene and one in the traH gene. Plasmids p16852 and p20729 differed by four SNPs in the impCAB operon, one SNP in the traH gene and six SNPs in regions encoding uncharacterized proteins (YfaB, YfeC and YffA), a total of 11 SNPs (Supplementary Tables S4A,B). In two isolates from Farm I, longer IncK2 plasmids were present (117010–118,095 bp), namely, p16449 and p17695. These plasmids harboured aac(3)VIa, aadA1, sul1 and tet(A) in addition to blaCMY-2 (Table 1). No SNP differences were present in the core genome (98.2%) between these two plasmids. The third plasmid from Farm I, named p14849, only harboured blaCMY-2 and was 84,386 bp long. The initial plasmid characterisation revealed that p1821 (Farm E) and p14849 (Farm I) were of different length compared to the remaining plasmids from the farm, and they were therefore excluded from the SNP analyses and visual comparison.
Visual comparisons between the three plasmids from Farm E (p16852, p18539 and p20728) and two plasmids from Farm I (p16449 and p17695), respectively, underlined the close genetic relationship between IncK2/blaCMY-2 plasmids present in different E. coli STs on the same farm (Figures 2, 3). The shufflon region differed among the plasmids, while the remaining parts were highly similar.
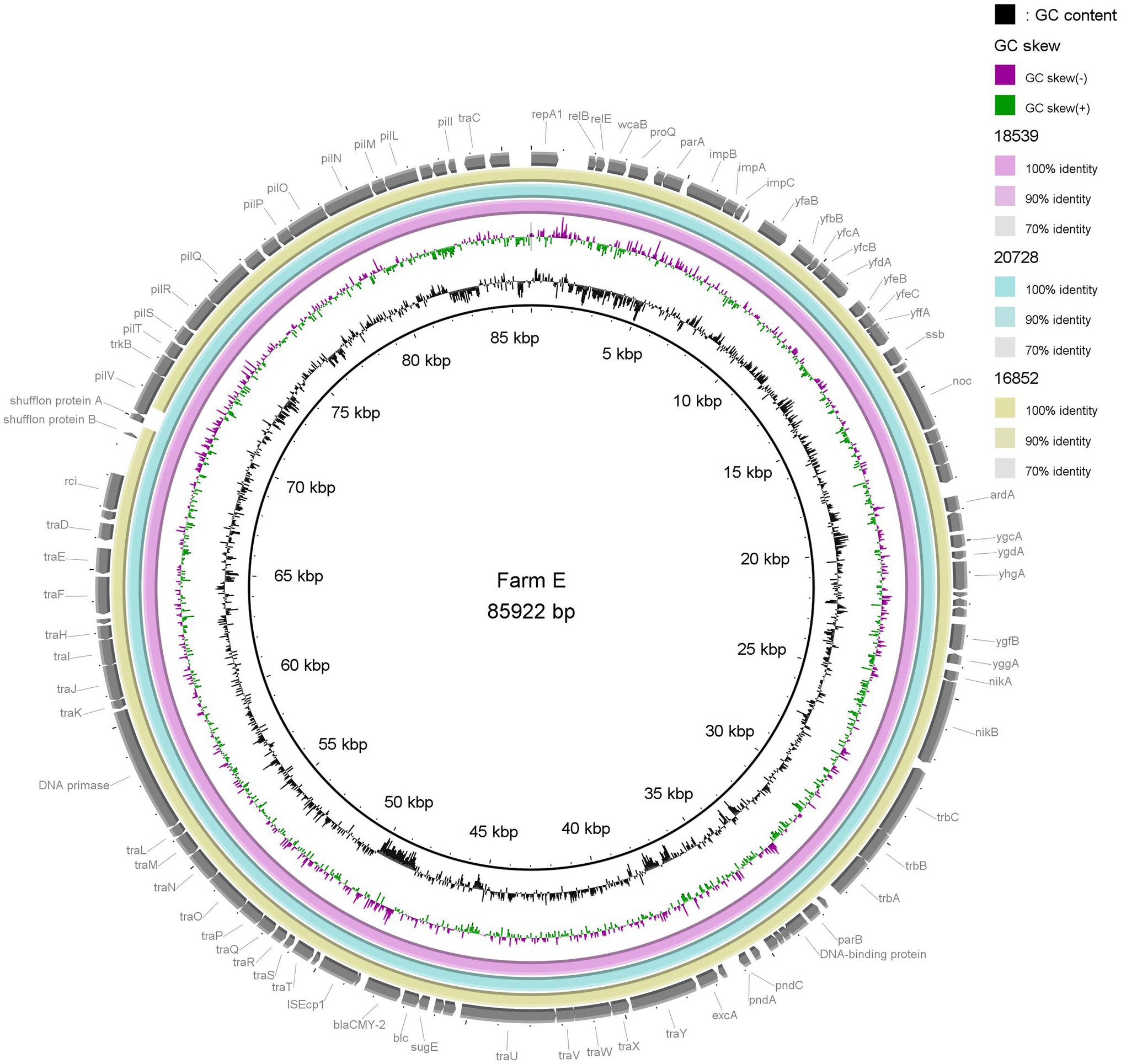
Figure 2. Comparison of three IncK2/blaCMY-2 plasmids (85–86 kb) originating from extended-spectrum cephalosporin-resistant Escherichia coli of three different sequence types isolated from three different flocks on a single farm. Plasmid p18539 was used as reference.
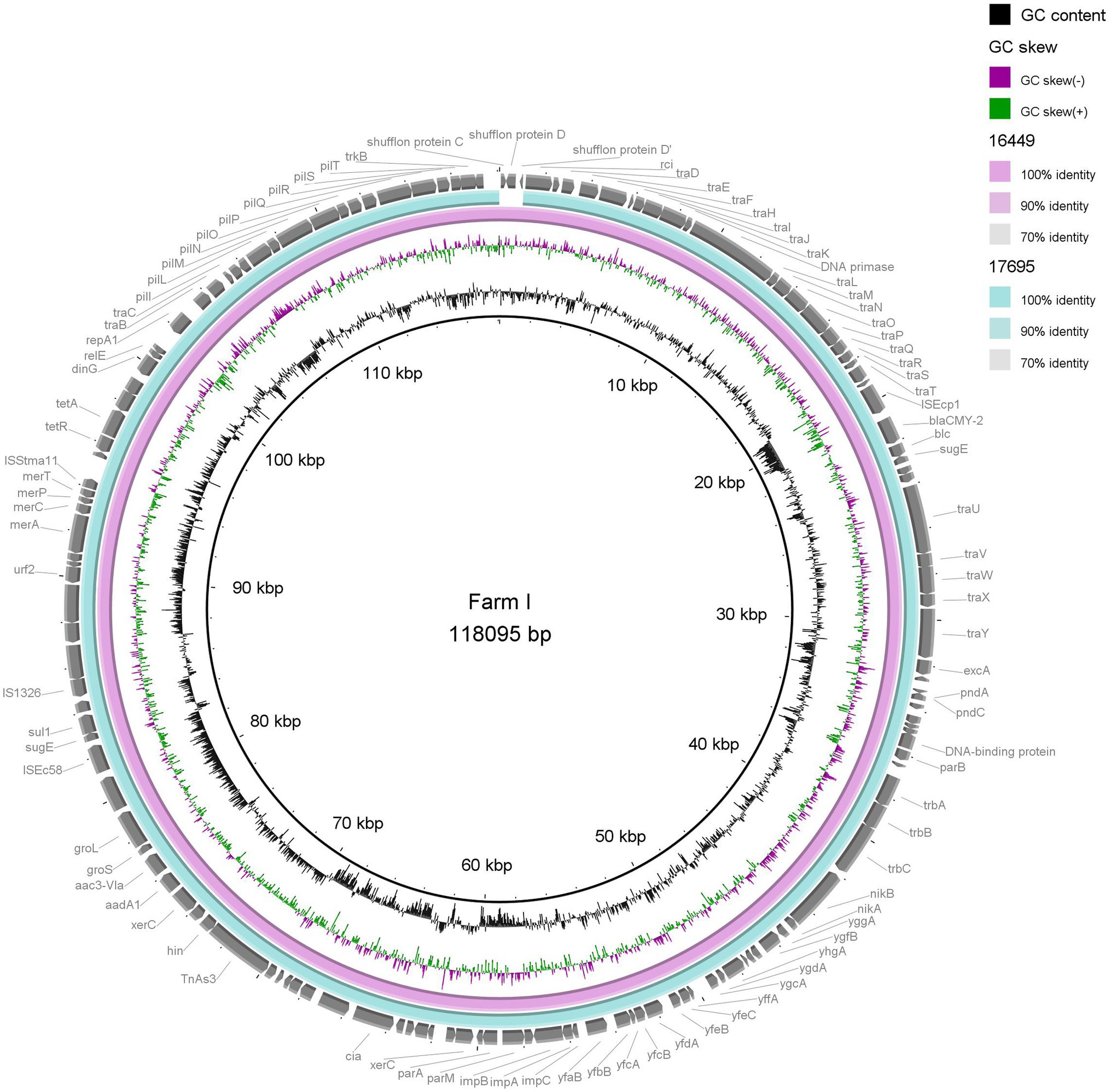
Figure 3. Comparison of two IncK/blaCMY-2 plasmids (117–118 kb) originating from extended-spectrum cephalosporin-resistant Escherichia coli of two different sequence types isolates from two different flocks on the same farm. Plasmid p16449 was used as reference.
BLAST revealed close sequence homology between plasmid pESBL3310 (acc. no. MW390545.1) originating from retail chicken meat in Netherlands and the two plasmids p16449 and p17695 (approx. 117–118 kb) from Farm E. Close homology was also found between pDV45 (broiler, Switzerland, acc. no. KR905384.1), p23C16–2 (broiler chicken, Japan, acc. no. LC501559) and the shorter (approx. 85–86 kb) IncK2 plasmids from Farms E and I (data not shown).
Raw reads are available from the European Nucleotide Archive (ENA; accession number PRJEB47914 for Illumina reads and PRJEB48023 for Oxford Nanopore reads).
Discussion
In this study, we have analysed ESC-resistant E. coli from Norwegian broiler farms sampled longitudinally during a six-month period in 2016. Our overall results indicated genetic diversity at strain level and a multitude of circulating ESC-resistant strains in broiler production, but also potential persistence of ESC-resistant E. coli on farms exemplified by ST57 on Farms C and H, and ST429 on Farms D and F. Although the occurrence of ESC-resistant E. coli is currently very low (0.4%) in Norwegian broiler production (NORM/NORM-VET, 2021), we consider this as valuable knowledge that can be extrapolated to other AMR E. coli.
On Farm A, we found ESC-resistant E. coli ST38 in all sampled flocks. Isolates from flocks reared in the same house were not necessarily more similar than isolates from flocks reared in different houses. Furthermore, up to 55 SNPs between isolates could indicate that E. coli ST38 has been introduced to the farm on several time points, rather than one strain persisting on the farm. For E. coli ST2040, ST429 and ST57, highly similar isolates were present in different flocks on the same farms, but also on different farms. For example, ST57 isolates originating from Farm C differed by zero SNPs and isolates from Farm H differed by one-six SNPs. This indicates potential clonal dissemination of these STs, for example from supplying parent flock(s) and that they may have the capability of persisting on broiler farms and/or in broiler houses between production rounds. ST57 isolates were overall more diverse than ST2040 and ST429 isolates. This could be due to previous introduction to the different farms followed by persistence and local evolution of the strain, or circulation of several ST57 strains in the broiler production. The same was observed for three ST937 isolates. However, we do not have any historical isolates available for comparison, and the reason for the observed diversity therefore remains undetermined. E. coli ST1158 was present on five different farms. At least two different strains were circulating, as up to 120 SNPs were observed. We have previously described the presence of both E. coli ST38 and ST1158 with blaCMY-2 in Norwegian broiler production (Mo et al., 2016b; Buberg et al., 2021). The first ST38 isolate described was from 2011, while ST1158 emerged in 2014 (Mo et al., 2016b). On the contrary, E. coli ST2040 and ST429 were first described from Norwegian broiler production in 2016 and were collected as part of the Norwegian monitoring programme on AMR in the veterinary sector, NORM-VET (Buberg et al., 2021). MLST has not been performed routinely on ESC-resistant E. coli collected in NORM-VET, but we have characterised all ESC-resistant isolates from retail chicken meat (2012–2016) from this programme (Buberg et al., 2021). Thus, it is reasonable to assume that ST38 and ST1158 circulated in the Norwegian broiler production for several years, while ST2040 and ST429 were introduced shortly before or during 2016. This could also be part of the explanation for the higher number of SNPs present among ST38 and ST1158 isolates compared to ST2040 and ST429 isolates.
ESC-resistant E. coli belonging to different STs were present in most included houses, indicating limited clonal persistence in the production. In addition, we detected different ESC resistance genes and plasmids in some of the houses. However, it was not possible to determine whether different genotypes were present simultaneously in a flock, as we only characterised one isolate per sample. Persistence of ESC-resistant strains could therefore be more common than suggested here.
In-depth characterisation revealed that three isolates of different STs from Farm E harboured highly similar IncK2/blaCMY-2 plasmids of approximately 85–86 kb. Furthermore, two isolates of different STs from Farm I carried IncK2/blaCMY-2 plasmids of approximately 117–118 kb with no SNP differences. This suggests possible horizontal transfer of and/or on farm persistence of plasmids. We have previously shown that most IncK/blaCMY-2 plasmids from Norwegian broiler production are self-transferable (Mo et al., 2016b). Thus, it is possible that IncK2/blaCMY-2 plasmids have transferred between different E. coli STs, resulting in dissemination and persistence of ESC resistance in the production pyramid, as suggested by others (Baron et al., 2018; van Hoek et al., 2018). We have previously demonstrated transfer of IncK/blaCMY-2 plasmids into Serratia spp. and back to E. coli. This suggests a potential plasmid reservoir in environmental bacteria, possibly affecting their ability to persist in broiler production (Mo et al., 2017).
On Farm I, two of the characterised isolates harboured a long (approx. 117–118 kb) IncK2/blaCMY-2 plasmid, while the third isolate harboured a shorter version (~84 kb). Thus, we can assume that distinct variants of IncK2/blaCMY-2 plasmids are circulating in the Norwegian broiler production. Based on our strategy of analysing a single isolate per flock, it was not possible to evaluate whether the two IncK2/blaCMY-2 plasmid variants were simultaneously present on Farm I. According to analyses done in MOB-suite using short-read data, the IncK2/blaCMY-2 plasmids included in this study varied from 74 to 110 kb, indicating that more than two variants are circulating. However, long-read sequencing and subsequent hybrid assemblies are necessary to get a more reliable prediction of plasmid length. The IncK2/blaCMY-2 plasmids characterised in the present study showed high sequence homology to previously published plasmids originating from broiler production in Switzerland, Netherlands and Japan. This indicated the presence of endemic plasmids circulating internationally in broiler production, as previously suggested (Mo et al., 2017; Seiffert et al., 2017).
Interestingly, all six E. coli ST2040 were from flocks reared in October 2016 on three farms. These harboured IncI1/blaCMY-2 plasmids, whereas only IncK2/blaCMY-2 and/or IncI1/blaCTX-M-1 plasmids were detected on these farms previously. IncI1/blaCMY-2 plasmids from ST2040 are non-transferable in vitro. Also, ST2040 was detected for the first time in ESC-resistant E. coli from Norwegian retail chicken meat in 2016 (Buberg et al., 2021). This, together with the limited number of SNPs (3–12), could indicate a common source of the ST2040 isolates, and potential vertical dissemination in the production pyramid. However, this could not be confirmed as information regarding hatchery of origin and supplying parent flocks was not available. It was not possible to determine if ST2040 could persist in the production, as the final samples were collected in October 2016.
It is possible that the observed diversity among ESC-resistant E. coli is in fact a reflection of the most abundant E. coli STs present in the broiler gut, but further studies are warranted to confirm this. It has been suggested that several isolates should be investigated in order to reflect the diversity of ESC-resistant E. coli in a sample (van Hoek et al., 2018). If susceptible E. coli were characterised in parallel, it would substantially increase the knowledge regarding within-flock diversity of susceptible and resistant E. coli, but also regarding transmission dynamics of ESC resistance in broiler flocks.
To our best knowledge, this is the first longitudinal study on ESC resistance in E. coli from broilers using both short- and long-read sequencing technology, enabling in-depth characterisation of isolates and resistance plasmids. Our results indicate diversity on strain-, plasmid- and ESC resistance gene level. In conclusion, this study has increased our knowledge regarding the dynamics and diversity of ESC-resistant E. coli in Norwegian broiler production. Seemingly, local persistence and re-circulation of ESC-resistant E. coli strains happen. However, different E. coli STs and even different plasmids carrying ESC resistance were observed in some houses/farms. We further describe the presence of highly similar plasmids in different E. coli STs, which could be due to persistence of ESC resistance plasmids. As we only characterised one isolate per positive flock, we have no knowledge regarding the composition of the ESC-resistant E. coli population. Thus, it is possible that several different E. coli STs carrying ESC resistance plasmids are present simultaneously. In addition, it is possible that some E. coli STs have the ability to persist between production cycles. Further exploration of the diversity of E. coli STs (susceptible and resistant) should be done in order to increase our understanding of the dynamics of ESC-resistant E. coli.
Data Availability Statement
The datasets presented in this study can be found in online repositories. The names of the repository/repositories and accession number(s) can be found in the article/Supplementary Material.
Author Contributions
SM, MN, and MS contributed to the conception and design of the present study, while AU was responsible for the conception and design of the study the isolates originated from. SM, JS, and AT performed the in silico analyses. SM wrote the first draft of the manuscript. All authors contributed to manuscript revision, read, and approved the submitted version.
Funding
This work was supported by funding from the Norwegian Veterinary Institute and the European Union’s Horizon 2020 Research and Innovation programme under grant agreement no 773830: One Health European Joint Programme, OH-EJP-H2020-JRP-AMR-2-ARDIG. Parts of the data were presented during the One Health EJP Annual Scientific Meeting in Dublin, May 2019.
Conflict of Interest
The authors declare that the research was conducted in the absence of any commercial or financial relationships that could be construed as a potential conflict of interest.
Publisher’s Note
All claims expressed in this article are solely those of the authors and do not necessarily represent those of their affiliated organizations, or those of the publisher, the editors and the reviewers. Any product that may be evaluated in this article, or claim that may be made by its manufacturer, is not guaranteed or endorsed by the publisher.
Acknowledgments
The isolates included in this study were collected in the project “NoResist-combating AMR in the Norwegian food production chain” funded by the Research Council of Norway (grant number 250212/E50) and the Norwegian Veterinary Institute. The sequencing service was provided by the Norwegian Sequencing Centre (www.sequencing.uio.no), a national technology platform hosted by the University of Oslo and supported by the ‘Functional Genomics’ and ‘Infrastructure’ programmes of the Research Council of Norway and the Southeastern Regional Health Authorities. The bioinformatics work was performed on resources provided by UNINETT Sigma2 – the National Infrastructure for High Performance Computing and Data Storage in Norway. Thongpan Leangapichart, Fiona Franklin-Alming and Cathrine Arnason Bøe are acknowledged for technical assistance, and Håkon Kaspersen is acknowledged for contributing with expertise in bioinformatics.
Supplementary Material
The Supplementary Material for this article can be found online at: https://www.frontiersin.org/articles/10.3389/fmicb.2021.795127/full#supplementary-material
Footnotes
1. ^https://github.com/tseemann/snippy
2. ^https://github.com/nanoporetech/qcat
References
Alikhan, N. F., Petty, N. K., Ben Zakour, N. L., and Beatson, S. A. (2011). BLAST ring image generator (BRIG): simple prokaryote genome comparisons. BMC Genomics 12:402. doi: 10.1186/1471-2164-12-402
Animalia (2015). Actions to combat antimicrobial resistance works (Tiltak mot antibiotikaresistens virker; in Norwegian) [Online]. Available: https://www.animalia.no/no/Dyr/antibiotikaresistens/aktuelt--antibiotikaresistens/tiltak-mot-antibiotikaresistens-virker/ (Accessed August 3, 2019).
Animalia (2016). Continued decrease in the occurrence of resistant bacteria in poultry (Fortsatt nedgang i forekomst av resistente bakterier hos fjørfe; in Norwegian) [Online]. Available: https://www.animalia.no/no/animalia/aktuelt/fortsatt-nedgang-i-forekomst-av-resistente-bakterier-hos-fjorfe/ (Accessed August 3, 2019).
Animalia (2017). Status for the poultry industry action plan against resistant bacteria in 2016 (Status for fjørfenæringas handlingsplan mot resistante bakterier i 2016; in Norwegian) [Online]. Available: https://www.animalia.no/no/animalia/aktuelt/status-for-fjorfenaringas-handlingsplan-mot-resistente-bakterier-i-2016/ (Accessed August 3, 2019).
Animalia (2018). Action plan: No detection of ESBL in samples from imported poultry in 2017 (Handlingsplan: Ingen funn av ESBL i prøver fra fjørfeimporter i 2017; in Norwegian) [Online]. Available: https://www.animalia.no/no/Dyr/antibiotikaresistens/aktuelt--antibiotikaresistens/fjorfenaringas-handlingsplan-ingen-funn-av-esbl-i-prover-fra-fjorfeimporter-i-2017/ (Accessed August 3, 2019).
Baron, S., Le Devendec, L., Touzain, F., Jouy, E., Lucas, P., de Boisseson, C., et al. (2018). Longitudinal study of Escherichia coli plasmid resistance to extended-spectrum cephalosporins in free-range broilers. Vet. Microbiol. 216, 20–24. doi: 10.1016/j.vetmic.2018.01.012
Buberg, M. L., Mo, S. S., Sekse, C., Sunde, M., Wasteson, Y., and Witsø, I. L. (2021). Population structure and uropathogenic potential of extended-spectrum cephalosporin-resistant Escherichia coli from retail chicken meat. BMC Microbiol. 21:94. doi: 10.1186/s12866-021-02160-y
Carattoli, A., Zankari, E., Garcia-Fernandez, A., Voldby Larsen, M., Lund, O., Villa, L., et al. (2014). In silico detection and typing of plasmids using PlasmidFinder and plasmid multilocus sequence typing. Antimicrob. Agents Chemother. 58, 3895–3903. doi: 10.1128/AAC.02412-14
De Coster, W., D'Hert, S., Schultz, D. T., Cruts, M., and Van Broeckhoven, C. (2018). NanoPack: visualizing and processing long-read sequencing data. Bioinformatics 34, 2666–2669. doi: 10.1093/bioinformatics/bty149
EFSA, and ECDC (2020). The European Union summary report on antimicrobial resistance in zoonotic and indicator bacteria from humans, animals and food in 2017/2018. EFSA J. 18:e06007. doi: 10.2903/j.efsa.2020.6007
Gurevich, A., Saveliev, V., Vyahhi, N., and Tesler, G. (2013). QUAST: quality assessment tool for genome assemblies. Bioinformatics 29, 1072–1075. doi: 10.1093/bioinformatics/btt086
Jain, C., Rodriguez, R. L., Phillippy, A. M., Konstantinidis, K. T., and Aluru, S. (2018). High throughput ANI analysis of 90K prokaryotic genomes reveals clear species boundaries. Nat. Commun. 9:5114. doi: 10.1038/s41467-018-07641-9
Kaspersen, H. (2021). Plasmid assembly, gene identification and annotation pipeline Ellipsis. https://github.com/NorwegianVeterinaryInstitute/Ellipsis (accessed 7 June 2021).
Kaspersen, H., Haverkamp, T. H. A., Ilag, H. K., Oines, O., Sekse, C., and Slettemeas, J. S. (2021). Complete genome sequences of 12 quinolone-resistant Escherichia coli strains containing qnrS1 based on hybrid assemblies. Microbiol. Resour. Announc. 10:e01190-20. doi: 10.1128/MRA.01190-20
Lagesen, K. (2020). Bifrost pipeline, https://github.com/NorwegianVeterinaryInstitute/Bifrost/tree/v1.2 (accessed 25 April 2020).
Larsen, M. V., Cosentino, S., Rasmussen, S., Friis, C., Hasman, H., Marvig, R. L., et al. (2012). Multilocus sequence typing of total-genome-sequenced bacteria. J. Clin. Microbiol. 50, 1355–1361. doi: 10.1128/JCM.06094-11
Mo, S. S., Kristoffersen, A. B., Sunde, M., Nødtvedt, A., and Norström, M. (2016a). Risk factors for occurrence of cephalosporin-resistant Escherichia coli in Norwegian broiler flocks. Prev. Vet. Med. 130, 112–118. doi: 10.1016/j.prevetmed.2016.06.011
Mo, S. S., Norström, M., Slettemeås, J. S., Løvland, A., Urdahl, A. M., and Sunde, M. (2014). Emergence of AmpC-producing Escherichia coli in the broiler production chain in a country with a low antimicrobial usage profile. Vet. Microbiol. 171, 315–320. doi: 10.1016/j.vetmic.2014.02.002
Mo, S. S., Slettemeås, J. S., Berg, E. S., Norström, M., and Sunde, M. (2016b). Plasmid and host strain characteristics of Escherichia coli resistant to extended-spectrum cephalosporins in the Norwegian broiler production. PLoS One 11:e0154019. doi: 10.1371/journal.pone.0154019
Mo, S. S., Sunde, M., Ilag, H. K., Langsrud, S., and Heir, E. (2017). Transfer potential of plasmids conferring extended-spectrum-cephalosporin resistance in Escherichia coli from poultry. Appl. Environ. Microbiol. 83:e00654-17. doi: 10.1128/AEM.00654-17
Mo, S. S., Telke, A. A., Osei, K. O., Sekse, C., Slettemeas, J. S., Urdahl, A. M., et al. (2020a). bla CTX-M- 1/IncI1-Igamma plasmids circulating in Escherichia coli from Norwegian broiler production are related, but distinguishable. Front. Microbiol. 11:333. doi: 10.3389/fmicb.2020.00333
Mo, S. S., Telke, A. A., Osei, K. O., Sekse, C., Slettemeas, J. S., Urdahl, A. M., et al. (2020b). blaCTX-M-1/IncI1-Igamma plasmids circulating in Escherichia coli from Norwegian broiler production are related, but distinguishable. Front. Microbiol. 11:333. doi: 10.3389/fmicb.2020.00333
Mo, S. S., Urdahl, A. M., Nesse, L. L., Slettemeas, J. S., Ramstad, S. N., Torp, M., et al. (2019). Occurrence of and risk factors for extended-spectrum cephalosporin-resistant Enterobacteriaceae determined by sampling of all Norwegian broiler flocks during a six month period. PLoS One 14:e0223074. doi: 10.1371/journal.pone.0223074
Myrenås, M., Slettemeås, J. S., Thorsteinsdottir, T. R., Bengtsson, B., Börjesson, S., Nilsson, O., et al. (2018). Clonal spread of Escherichia coli resistant to cephalosporins and quinolones in the Nordic broiler production. Vet. Microbiol. 213, 123–128. doi: 10.1016/j.vetmic.2017.11.015
NORM/NORM-VET (2021). “NORM/NORM-VET 2020. Usage of Antimicrobials and Occurrence of Antimicrobial Resistance in Norway”. (Tromsø/Oslo. ISSN:1502–2307 (print)/1890–9965 (electronic)).
Refsum, T. (2015). Antimicrobial use in the Norwegian poultry production (Antibiotikabehandling i norsk fjørfeproduksjon; in Norwegian). Go’ mørning p.17. Available at: https://www.animalia.no/globalassets/publikasjoner/gm-4-15-web.pdf (accessed 8 march 2019).
Robertson, J., and Nash, J. H. E. (2018). MOB-suite: software tools for clustering, reconstruction and typing of plasmids from draft assemblies. Microb. Genom 4:e000206. doi: 10.1099/mgen.0.000206
Seemann, T. (2014). Prokka: rapid prokaryotic genome annotation. Bioinformatics 30, 2068–2069. doi: 10.1093/bioinformatics/btu153
Seiffert, S. N., Carattoli, A., Schwendener, S., Collaud, A., Endimiani, A., and Perreten, V. (2017). Plasmids carrying blaCMY -2/4 in Escherichia coli from poultry, poultry meat, and humans belong to a novel IncK subgroup designated IncK2. Front. Microbiol. 8:407. doi: 10.3389/fmicb.2017.00407
Sunde, M., Tharaldsen, H., Slettemeås, J. S., Norström, M., Carattoli, A., and Bjorland, J. (2009). Escherichia coli of animal origin in Norway contains a blaTEM-20-carrying plasmid closely related to blaTEM-20 and blaTEM-52 plasmids from other European countries. J. Antimicrob. Chemother. 63, 215–216. doi: 10.1093/jac/dkn445
Ueno, Y., Arita, M., Kumagai, T., and Asai, K. (2003). Processing sequence annotation data using the Lua programming language. Genome Inform. 14, 154–163.
van Hoek, A., Veenman, C., Florijn, A., Huijbers, P. M. C., Graat, E. A. M., de Greeff, S., et al. (2018). Longitudinal study of ESBL Escherichia coli carriage on an organic broiler farm. J. Antimicrob. Chemother. 73, 3298–3304. doi: 10.1093/jac/dky362
Wick, R. R., Judd, L. M., Gorrie, C. L., and Holt, K. E. (2017). Unicycler: resolving bacterial genome assemblies from short and long sequencing reads. PLoS Comput. Biol. 13:e1005595. doi: 10.1371/journal.pcbi.1005595
Wirth, T., Falush, D., Lan, R., Colles, F., Mensa, P., Wieler, L. H., et al. (2006). Sex and virulence in Escherichia coli: an evolutionary perspective. Mol. Microbiol. 60, 1136–1151. doi: 10.1111/j.1365-2958.2006.05172.x
Keywords:Escherichia coli, longitudinal, ESC, plasmid, broiler
Citation: Mo SS, Norström M, Slettemeås JS, Urdahl AM, Telke AA and Sunde M (2021) Longitudinal Sampling Reveals Persistence of and Genetic Diversity in Extended-Spectrum Cephalosporin-Resistant Escherichia coli From Norwegian Broiler Production. Front. Microbiol. 12:795127. doi: 10.3389/fmicb.2021.795127
Edited by:
Maria Jorge Campos, Polytechnic Institute of Leiria, PortugalReviewed by:
Tiago Casella, Faculdade de Medicina de São José do Rio Preto, BrazilAlessandra Piccirillo, University of Padua, Italy
Copyright © 2021 Mo, Norström, Slettemeås, Urdahl, Telke and Sunde. This is an open-access article distributed under the terms of the Creative Commons Attribution License (CC BY). The use, distribution or reproduction in other forums is permitted, provided the original author(s) and the copyright owner(s) are credited and that the original publication in this journal is cited, in accordance with accepted academic practice. No use, distribution or reproduction is permitted which does not comply with these terms.
*Correspondence: Solveig Sølverød Mo, U29sdmVpZy5tb0B2ZXRpbnN0Lm5v