- 1Department of Natural Sciences, School of Arts and Sciences, Lebanese American University, Byblos, Lebanon
- 2Department of Biochemistry and Molecular Genetics, Faculty of Medicine, American University of Beirut, Beirut, Lebanon
- 3Division of Genomics and Translational Biomedicine, College of Health and Life Sciences, Hamad Bin Khalifa University, Doha, Qatar
The COVID-19 pandemic involved millions of people and diabetes was identified as an associated comorbidity. Initiation of systemic corticosteroids in patients suffering from severe COVID-19 was associated with lower mortality. A surge of invasive fungal infections of the maxillofacial region, namely mucormycosis, was linked to a deadly infection known as black fungus. Black fungus, diabetes, corticosteroids, and coronavirus disease 2019 (COVID-19) all have a dysregulated immune response in common, which partly could also be attributed to interleukin 37 (IL-37). IL-37, a new cytokine of the IL-1 family, known for broadly reducing innate inflammation as well as acquired immune responses. The use of corticosteroids in diabetic COVID-19 patients, crowded hospitals, and lack of medical oxygen should be carefully considered to reduce COVID-associated secondary infections.
Introduction
In early December 2019, the first pneumonia cases of unknown origin were identified in Wuhan caused by severe acute respiratory syndrome coronavirus 2 (SARS-CoV-2). The disease is known as coronavirus disease 2019 (COVID-19) with severity ranging from mild to lethal. Symptoms of COVID-19, include, but are not limited to, fever, cough, shortness of breath, muscle aches, anosmia, fatigue, and diarrhea (Molteni et al., 2021). The pandemic involved millions of people with some chronic conditions being associated with severe outcomes. People with preexisting conditions such as diabetes, hyperglycemia, cardiovascular diseases, hypertension, and obesity were linked to higher hospitalization and mortality rates (Rawshani et al., 2018; Corrao et al., 2021). The global diabetes prevalence in 2019 was estimated to be 9.3% (463 million people), with half a billion people suffering (Colagiuri et al., 2019) from diabetes worldwide, and the number was estimated to increase by 25% in 2030. Untreated chronic hyperglycemia imposes damage on numerous cell types and is strongly correlated with limb amputations, kidney failure, and cardiovascular diseases (World Health Organization, 2019). To add a new level of complexity to an already challenging situation, diabetic patients present a high-risk factor for COVID-19 infection. It was pointed out that abnormally high concentrations of glucose in the blood, a feature of diabetes mellitus, may increase the virulence of some pathogens (Joshi et al., 1999; Casqueiro et al., 2012). People with type 2 diabetes (T2D) do not exhibit increased susceptibility to SARS-CoV-2 infection. However, they had more than twice the likelihood for COVID-19-related hospitalization and intensive care admission with a dramatic increase in mortality (Roberts et al., 2020). Guan et al. (2020) showed that 16.2% of the SARS-CoV-2-infected patients that manifested severe symptoms had diabetes. Moreover, results obtained by Zhang et al. (2020) provided further evidence that diabetes was among the most common comorbidities seen (Kayina et al., 2020) among COVID-19 patients. Higher blood glucose levels were also detected in COVID-19 patients. In diabetic this can be attributable to (i) interference of macrophages and T-cell cytokines release diminishing neutrophil recruitment (Delamaire et al., 1997) (ii) reduction of interferon-α (IFN-α) production by blood dendritic cells (Summers et al., 2006) and (iii) disruption of innate immune responses (Zykova et al., 2000). Additionally, the virus surface spike protein facilitates its entry through (Hoffmann et al., 2020) attaching to the human angiotensin-converting enzyme 2 (ACE2) via its receptor-binding domain, and targeting ACE2 in the endocrine tissues of the pancreas leading as a result to islets damage (Yang et al., 2010). Liu et al. (2020) revealed the significant pancreatic damage that could occur in patients infected with SARS-CoV-2. The authors expressed mounting concern about the long-term effects of SARS-CoV-2 infection on patients that may be due to its increased infectivity and affinity relative to the other coronaviruses. Here, we review and highlight the interrelationship between T2D and severe clinical course and outcomes of COVID-19 patients.
Type 2 Diabetes Pathogenesis and the Role Interleukin 37
Obesity leads to adipose tissue dysfunction that was directly linked to the development of health hazards such as diabetes mellitus and cardiovascular diseases. The adipose tissue expansion occurs through hypertrophy (increase in existing adipocyte size) and hyperplasia (increase in adipocyte number) (Greenberg and Obin, 2006). The fat tissue was recognized as a highly active endocrine organ mainly producing inflammatory mediators known as adipokines (Kershaw and Flier, 2004). Adipokines are a family of hormones having anti- or pro-inflammatory effects (Tilg and Moschen, 2006). In the event of obesity, more pro-inflammatory cytokines were produced when compared to the anti-inflammatory ones (Shibata et al., 2017). For that, T2D is associated with low-grade chronic inflammation linked to excessive visceral adipose tissue, affecting homeostatic glucose regulation and peripheral insulin sensitivity (Iacobellis, 2020). Tumor necrosis factor α (TNF-α), resistin, and interleukin-6 (IL-6) were linked previously to insulin resistance, while adiponectin was found to enhance insulin sensitivity, increase glucose transepithelial transport, lower glucose synthesis in the liver, and suppress IL-6 and TNF-α (Yamauchi and Kadowaki, 2008).
Inflammation has an active role in the pathogenesis of hyperglycemia (Gianchandani et al., 2020), and acute viral respiratory infection by SARS-CoV was linked to transient insulin resistance and hyperglycemia induced by a temporary inflammation of the pancreas’ islet cells (Yang et al., 2010). Stress hyperglycemia, which is a temporary increase in blood sugar, may have a negative impact in acute illness (Corrao et al., 2021). Chronic hyperglycemia and inflammation can cause an abnormal and ineffective immune response with the combined elevated levels of interleukin-1β (IL-1β), IL-6, and C-reactive protein (CRP) being predictive of T2D (Manson et al., 2001; Figure 1). Hypoxia and cell death of expanding adipose tissue, activation of the nuclear factor-κB (NF-κB) and JUN N-terminal kinase (JNK) pathway, activation of interleukin-1β (IL-1β), and recruitment and activation of immune cells were all linked to the inflammatory state in T2D (Donath and Shoelson, 2011; Gianchandani et al., 2020). IL-1 family cytokines play a crucial role in immune homeostasis. Strikingly, most proinflammatory factors present at high levels in the blood of patients with T2D were IL-1 dependent, with IL-37 adjusting obesity-induced inflammation and insulin resistance (Ballak et al., 2014; Table 1). IL-37 plays an important role in metabolic and autoimmune diseases as well as infections. The development in the in silico research of human databases has led to the discovery of IL-37 by several independent groups (Boraschi et al., 2011). Fragments of mRNA sequence encoding an IL-1-like molecule were expressed in human databases (accession number AI014548) and characterized by having five different isoforms (IL-37a-e) (Busfield et al., 2000; Kumar et al., 2000; Pan et al., 2001). IL-37, which can be produced in intra- and extracellular domains, was linked to anti-inflammatory actions through inhibiting innate responses (Dinarello and Bufler, 2013) and detected in the nucleus affecting gene transcription. The expression levels of TNF, IL-1, IL-6, and chemokine MIP-2 were significantly decreased in lipopolysaccharide (LPS)-stimulated macrophages transfected with human IL-37 when compared to those transfected with an empty plasmid (Nold et al., 2010). Sharma et al. (2008) showed that efficient caspase-1 inhibitors can reduce IL-37 entry to the nucleus, and hence caspase-1 processing could be fundamental for IL-37 translocation. Translocation to the nucleus, after caspase-1 processing, involves SMAD3, which is part of TGF-β anti-inflammatory signaling pathway (Figure 1). Investigations were conducted to confirm the functional interaction of SMAD3 with IL-37. Nold et al. (2010) observed the formation of IL-37-SMAD3 complex in perinuclear and cytosolic regions in IL-37-transfacted A549 cells. This correlated favorably with the results obtained previously (Bar et al., 2004) and which demonstrated that the inhibition of SMAD3 could be accountable in reversing the inhibition of IL-6 and IL-1 expression in cells transfected with IL-37. Furthermore, it was exhibited that the potential for IL-37 to reduce the production of IL-1, IL-9, IL-6 and TNF decreased after introducing short hairpin RNA (shRNA) into human macrophages and which inhibited SMAD3 expression (Nold et al., 2010). IL-37 exerts inhibitory properties on the innate inflammatory and acquired immune responses and has a dualfunction with both intra- and extracellular mechanisms of action (Bulau et al., 2014). IL-18 induces activation of IL-1 receptor-associated kinase and tumor necrosis factor (TNF) receptor-associated factor 6 (TRAF-6), with the net outcome of activating the kinases being the translocation of NF-κB (Matsumoto et al., 1997). IL-37 may also inhibit IL-18 dependent pro-inflammatory cytokine production (Ballak et al., 2014). IL-37b and IL-18 are phylogenetically related, and both have two symmetric key structural amino acid residues (Glu-35 and Lys-124) (Dinarello and Bufler, 2013). IL-37 could also interact and bind, but with low affinity, to IL-18 receptor α subunit (IL-18Rα) (Dinarello and Bufler, 2013). IL-37, however, binds to the IL-18 binding protein (IL-18BP), the natural inhibitor of IL-18, and forms a complex reducing IL-18’s activity. IL-37b is the receptor antagonist of IL-18, it binds to the IL-18 binding protein (IL-18BP) followed by binding to the IL-18Rα, recruiting IL-1R8, and forming the IL-37/IL-1R8/IL-18Rα complex (Donath and Shoelson, 2011).
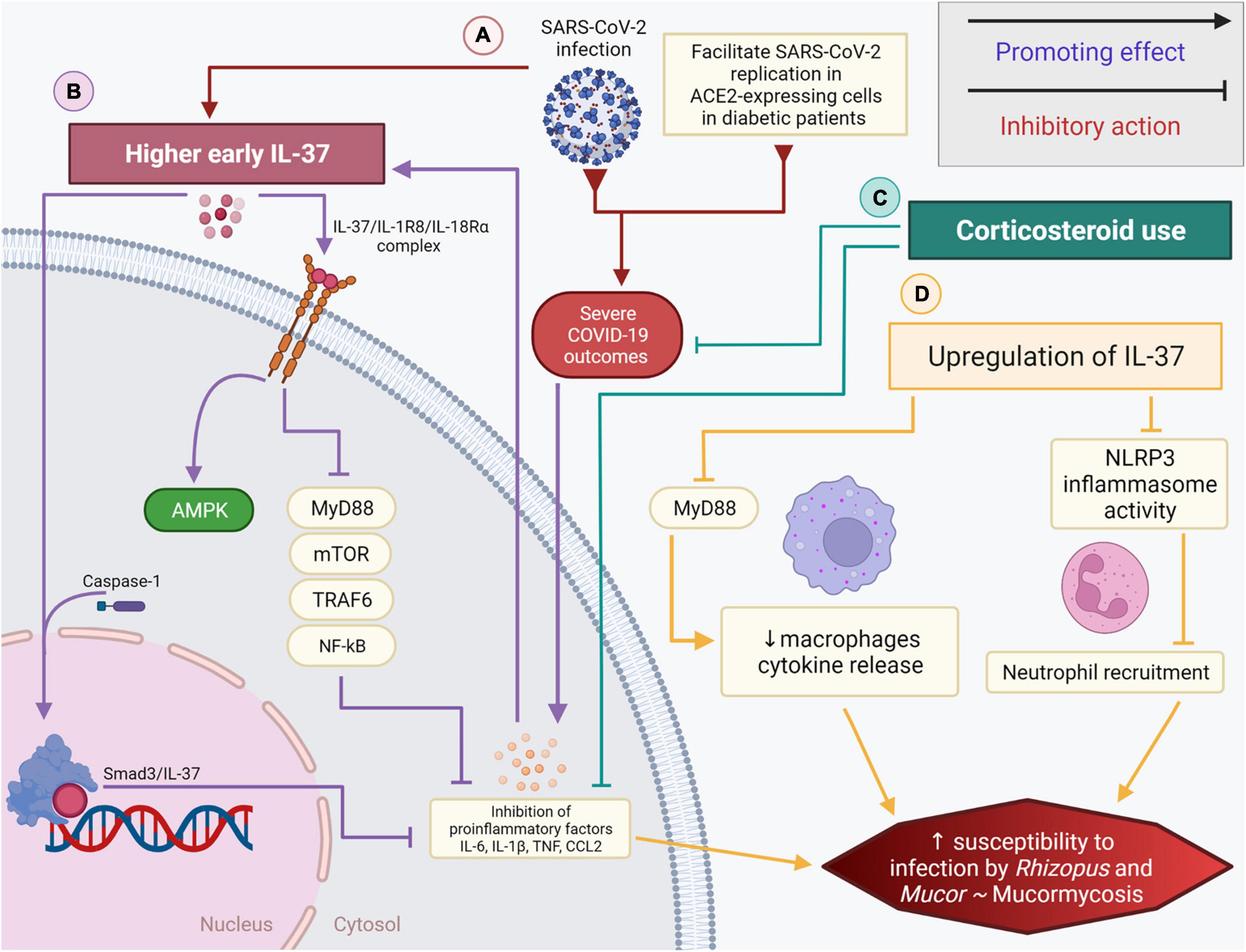
Figure 1. The role of IL-37 and corticosteroids in diabetic COVID-19 patients and their association with the black fungus infection. (A) SARS-CoV-2 infection in hyperglycemic patients promotes a dysregulated pro-inflammatory cytokine storm while also promoting higher early expression of IL-37 (B) IL-37/IL-1R8/IL-18Rα complex inhibits the mobilization of MyD88, mTOR, TRAF6, NF-κB, subsequently impeding the secretion of pro-inflammatory cytokines. IL-37 could be directly processed by caspase-1, intracellularly, and associates with SMAD3 in the nucleus. (C) Corticosteroids and the loss of immune homeostasis linked partly to IL-37, coupled with diabetes, can increase the risk of black fungus infection/mucormycosis. (D) Higher early IL-37 and corticosteroid use in diabetic COVID-19 patients inhibit proinflammatory signals. The upregulation of IL-37 interferes with the innate immune system driven inflammation by promoting the decrease of macrophage cytokine release, reducing the NLRP3 inflammasome activity and inhibiting neutrophil recruitment. These factors, when combined, significantly elevate the risk and susceptibility to mucormycosis.
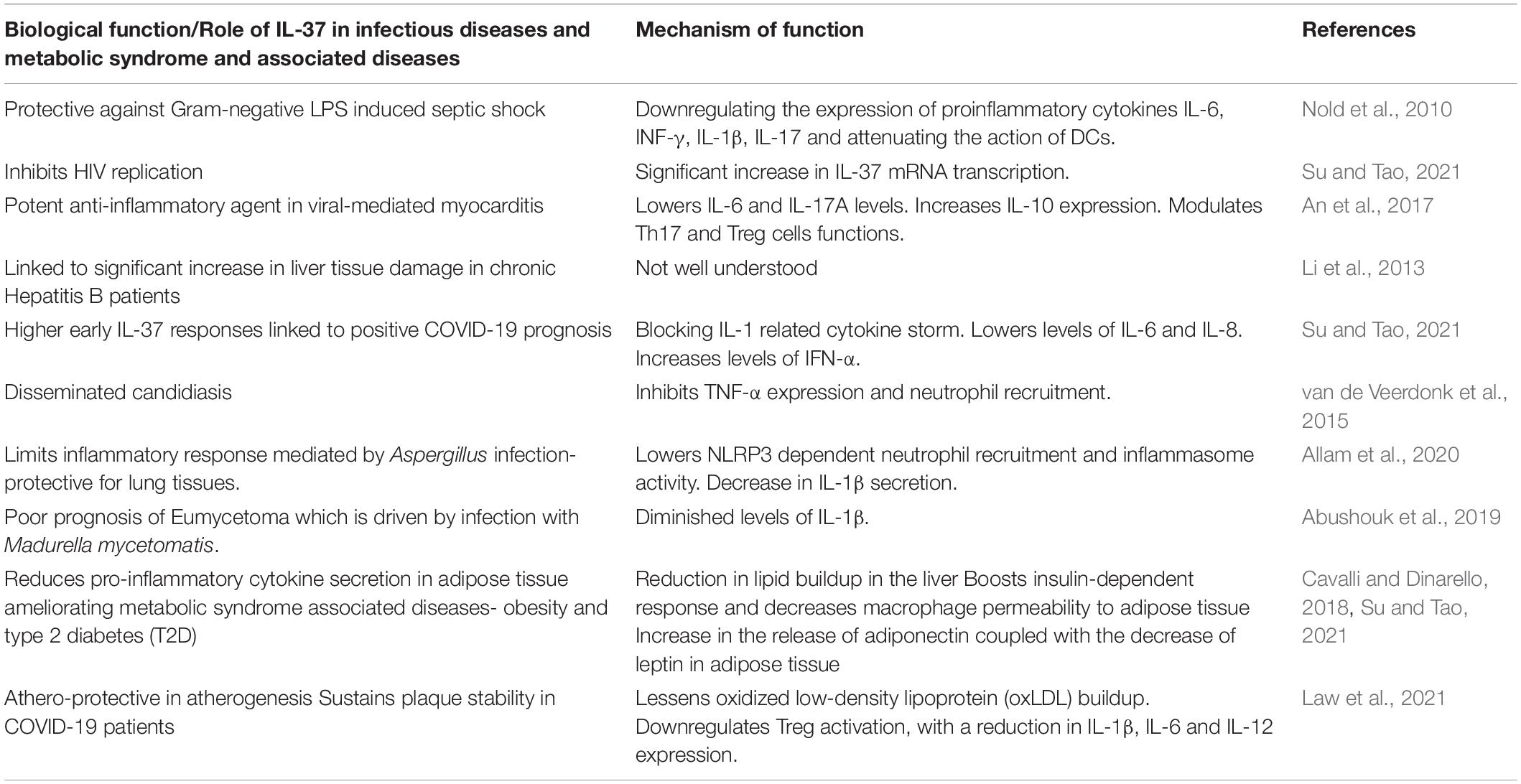
Table 1. The role of IL-37 and its biological functions in infectious and metabolic syndrome associated diseases.
Correlation Between Interleukin 37 and COVID-19
The potential involvement of IL-37 in suppressing the cytokine storm that contributes to poor prognosis and mortality in COVID-19 patients was studied (Ragab et al., 2020). The mass release of pro-inflammatory cytokines causes acute respiratory distress syndrome and multiple organ failure (Ragab et al., 2020). Controlling the cytokine storm is critical in preventing the progression of the disease and saving patients’ lives. A noteworthy strategy that comes into play are anti-inflammatory factors, such as IL-37, that can maintain biochemical homeostasis. Since IL-37 is considered as the natural inhibitor of IL-1, the main modulator of pro-inflammatory cytokines and inflammatory responses, it is safe to assume that IL-37 could provide relief in systemic inflammation that occurs after COVID-19 infection (Table 1). Recently, the correlation between IL-37 production, pro-inflammatory cytokines level, and clinical outcomes in SARS-CoV-2 infection was revealed (Li et al., 2021). An enzyme linked immunosorbent assay demonstrated significantly higher concentrations of IL-37 in the plasma of SARS-CoV-2-infected subjects when compared to healthy ones. Li et al. (2021), showed that mild and severe clinical outcomes are directly associated with reduced and elevated plasma levels of IL-37, respectively. Given the fact that high early IL-37 responses were accompanied with speedy recovery among hospitalized patients (earlier viral clearance, chest tomographic improvement, and cough relief) (Table 1), the authors suggested that IL-37 administration may be used to maintain a balanced inflammatory response (Li et al., 2021). Moreover, Ahmed and Ad’hiah (2021a) revealed the role of IL-37 in the virus-mediated pathogenesis. In a case-control study involving 200 patients, the sequencing of the IL37 gene (Gene ID: 27178) identified a couple of single nucleotide polymorphisms (SNPs) that were directly linked with the susceptibility to COVID-19. The two variants of IL37 gene with rs3811046 and rs3811047 SNPs, may increase the risk of developing COVID-19 by 2.55 and 2.02-fold. The authors showed a significant correlation between the presence of these polymorphisms and increased incidence of severe COVID-19. The data revealed that SNP rs3811046 detection was significantly higher in patients (61%) when compared to controls (38.0%), which was also the case with SNP rs3811047 (patients 39% vs. controls 24%). In contrast to the previous findings, a more recent study showed that IL-37 expression was suppressed in COVID-19 patients but was prominent in obese patients (Ahmed and Ad’hiah, 2021b). Therefore, it is evident that low IL-37 plasma levels could be used as COVID-19 severity marker. IL-37 was also found to ameliorate influenza pneumonia in vivo (Qi et al., 2019). Interestingly, treatment of H1N1-infected mice using IL-37 reduced (by 2 days) the body weight recovery time. The body weights of mice treated with oseltamivir phosphate in combination with IL-37 and oseltamivir phosphate alone began to increase at 7- and 9-days post-inoculation, respectively. The intravenous IL-37 administration improved the survival rates in H1N1- infected mice with a total of 71% of the mice surviving, compared to 57% survival when using a single dose of oseltamivir phosphate. IL-37 additionally relieved H1N1-induced lung tissue damage. The correlation between IL-37 and severity of viral infection was noteworthy. IL-37 treatment modulates (suppresses) the immune response by shifting the cytokine equilibrium away from excess inflammation. Qi et al. (2019) also demonstrated that IL-37 can reduce the levels of pro-inflammatory modulators in mice infected with respiratory viruses. Using RT-qPCR as well as Cytometric Beads Array, they showed that IL-37 treatment significantly reduced the excessive production of IL-6, TNF-α, MCP-1, IL-1α, IL-1β, MIP-1α, MIP-1β, IP-10, MIG, RANTES, IFN-γ and IL-10 in the lung tissues of infected mice.
Diabetes and COVID-19
Diabetes has been identified as comorbidity associated with COVID-19 severity. Higher risks of COVID-19-related mortality was linked to cardiovascular and renal complications associated with diabetes, glycemic control, and body mass index (BMI) (Holman et al., 2020). Blood glucose level was revealed to be a key mediator of severe symptoms in COVID-19. Persistent inflammation is associated with a compensatory anti-inflammatory response. T2D-related immunosuppression may facilitate SARS-CoV-2 replication in ACE2-expressing cells, promoting cytokine storms and inducing immune dysregulation, partly explaining the more severe COVID-19 outcomes in patients with diabetes (Roberts et al., 2020). IL-37 performs its immunosuppressive activity by acting on mTOR and increasing the adenosine monophosphate (AMP) kinase. It inhibits class II histocompatibility complex (MHC) molecules and inflammation in inflammatory diseases by suppressing MyD88 and subsequently IL-1β, IL-6, TNF, and CCL2 (Conti et al., 2020; Figure 1). Li et al. (2019) revealed the association between IL-37 and T2D. The authors showed an overexpression of IL-37 in the peripheral blood monocyte cells of T2D patients when compared to healthy controls. A significant difference was detected using ELISA between the serum level of IL-37 in T2D vs. control subjects. Additionally, the study showed that gut microbiota dysbiosis significantly contributed to the development of T2D and that a better response to insulin therapy was seen in patients with higher IL-37 levels (Ballak et al., 2014; Li et al., 2019). Furthermore, a mouse model receiving high-fat diet treatment provided further evidence on the link between IL-37 and T2D. IL-37 increases insulin sensitivity and reduces gut microbiota dysbiosis.
Corticosteroids and the Black Fungus
Numerous therapeutic options to modulate the inflammatory response against SARS-CoV-2-infection were considered. It is widely known that corticosteroids administration successfully prohibits extensive immune responses and accordingly used in controlling hyperinflammation and acute respiratory distress syndrome (Barnes, 2006). During the COVID-19 pandemic, an alarmingly high prevalence of self-medication was reported (Quispe-Cañari et al., 2021), with the corticosteroids being one of the most drugs used due to their high affordability and accessibility (Chaudhry et al., 2021). The RECOVERY Collaborative Group demonstrated that the incidence of death was lower in patients treated with dexamethasone (29.3%) when compared to those who received usual care (41.4%) (Recovery Collaborative Group, 2021). The World Health Organization (WHO) has recommended against using corticosteroids for COVID-19 treatment, but this recommendation was later reversed (World Health Organization, 2020). Corticosteroids regulate inflammation and can block the function of transcription factors mediating inflammatory responses, such as NF-κB and activator protein 1 (AP-1) and inhibit the production of proinflammatory cytokines (IL-1, TNFα, IL-6) (Cain and Cidlowski, 2017). However, the utilization of corticosteroids appears to be a double-edged sword against COVID-19. Their use in respiratory viral infection was linked to an increased risk of secondary opportunistic bacterial or fungal infections (Conti et al., 2020). A surge of invasive fungal infections (IFI) of the maxillofacial region, namely mucormycosis, was reported in India and linked to a rare and possibly deadly infection known as black fungus. Mucormycosis, previously known as zygomycosis, is rare, though increasingly prevalent, and a dangerous angio-invasive infection caused by mucormycetes (Skiada et al., 2020). The most common causative agents of the disease are Rhizopus and Mucor (Roden et al., 2005). These ubiquitous fungi can be found particularly in the soil in association with decaying leaves, compost piles, and rotten wood (Sridhara et al., 2005). Mucormycetes infections occur more frequently in summer and fall compared to the winter and spring and affect the sinuses and the lungs after inhaling fungal conidia (Sugar, 1990). The fungus can also be introduced through a break in the skin such as a cut, burn, or other types of skin injury (Roden et al., 2005). The mucormycetes invade blood vessels which may reduce the blood and nutrient flow to downstream organs consequently leading to necrosis (Skiada et al., 2020). Hosts susceptible to mucormycosis include diabetics, those on systemic corticosteroid use, and immunocompromised individuals. Healthcare-associated mucormycosis is a matter of concern and was previously linked to the use of contaminated devices or environmental reservoirs (Rammaert et al., 2012b).
Opportunistic fungal infections, such as the black fungus commonly seen in diabetics, hyperglycemia, COVID-19 patients, and linked to corticosteroids have all in common a dysregulated immune response that partly could also be attributed to IL-37 (Rammaert et al., 2012a; van Paassen et al., 2020; Kumar et al., 2021). A significant correlation exists between well-controlled blood glucose and lower serum levels of inflammatory markers such as IL-6, high sensitivity c-reactive protein (CRP), and lactate dehydrogenase in COVID-19 patients (Gianchandani et al., 2020). Corticosteroids, on the other hand, can downregulate the expression of IL-37, which plays an essential regulatory role in innate and adaptive immunity. Its expression in human adipose tissue was associated with enhanced insulin sensitivity and reduced adipose tissue inflammation (Table 1; Ballak et al., 2014). IL-37 exerts significant anti-inflammatory and metaboregulatory effects, dramatically reducing cytokine secretion in macrophages and dendritic cells, with higher early IL-37 responses in COVID-19 patients leading to earlier viral RNA negative conversion (Wang et al., 2018; Yi et al., 2020). It protects the body by inhibiting excessive immune response. Still, it could also lead to increased infection and mortality caused by some pathogens, possibly due to its inhibitory effects on the immune response. The regulatory role it plays could explain the link between the severity of COVID-19 in some diabetic patients being treated with corticosteroids and their potential to additionally acquire black fungus disease in poorly maintained critical care units.
Interleukin 37 and Its Central Role in COVID-19, Diabetes, and Mucormycosis
Most serious fungal disease arises from poorly cleared infection or disrupted barrier integrity (Rautemaa-Richardson and Richardson, 2021). Mucormycosis is an invasive and potentially life-threatening opportunistic fungal infection. The predisposing conditions vary geographically with diabetes mellitus being the most common risk factor in Asia (Hoang et al., 2020). Different subtypes of monocytes and macrophages play a pivotal role in the production of pro-inflammatory mediators and initiation of an inflammatory response. Previously Waldorf et al. (1984) showed that bronchoalveolar macrophages formed part of an efficient defense against Rhizopus oryzae by inhibiting germination and interfering with the conversion of R. oryzae to its tissue invasive phase. Neutrophils and monocytes are critical components of the host response to mucormycosis which efficiently eliminate fungal spores and hyphae by oxidative and non-oxidative killing mechanisms (Diamond et al., 1982; Waldorf et al., 1984). Enhanced susceptibility to mucormycosis was observed in human diabetics. Hyperglycemia and acidosis are known to impair chemotaxis and the killing activity of phagocytic cells against Mucorales by weakening oxidative and non-oxidative mechanisms (Chinn and Diamond, 1982). Corticosteroids, on the other hand, act on monocytes and macrophages, induce anti-inflammatory mediators and differentiation of anti-inflammatory phenotypes, and limit T-cell activation (Ehrchen et al., 2019).
IL-37, as mentioned above, is also an inhibitor of innate immunity through the caspase-1-dependent nuclear translocation or by the extracellular mechanism engaging the IL-18 receptor (Figure 1 and Table 1; Bulau et al., 2014). The timing of IL-37 expression at the site of inflammation is critical. IL-37 based anti-inflammatory strategies could have a negative impact on susceptibility to infections by inhibiting host defenses or be beneficial for the outcome of the host. Higher early IL-37 responses were correlated with earlier viral RNA negative conversion, chest computed tomographic improvement, and cough relief in COVID-19 patients (Li et al., 2021). In contrast, IL-37 interfered with the innate protective anti-Candida host response by reducing the production of proinflammatory cytokines and suppressing neutrophil recruitment in response to Candida (Table 1), resulting in an increased susceptibility to disseminated candidiasis (van de Veerdonk et al., 2015).
Conclusion
There are several measures that can be employed to minimize COVID-19 mortalities and reduce the risk of mucormycosis in people with diabetes. Maintaining and monitoring the glucose level is essential to improve outcomes and reduce secondary infections, renal dysfunction, ICU stay, and ventilator dependence. The pro-inflammatory metabolic state in diabetic patients could promote a virus-linked inflammatory surge leading to insulin resistance and severe hyperglycemia. People with diabetes and COVID-19 should be monitored for inflammatory and prothrombotic markers (Gianchandani et al., 2020). CRP is a good inflammatory marker revealing the association between diabetes and adverse COVID-19 outcomes, including the need for ICU admission, dyspnea, and length of stay (Koh et al., 2021), whereas fibrinogen for coagulation dysfunction (Varikasuvu et al., 2021). The use of corticosteroids can modulate the risk when used prudently. Limitations to their use associated with corticosteroid-mediated inhibition of glucose uptake and reductions in insulin sensitivity in COVID-19 patients and their role in increasing the susceptibility to acquire other infections are among the major concerns. IL-37 is a cytokine that exerts anti-inflammatory effects both intracellularly and extracellularly and is a therapeutic approach that should be considered and further studied. Finally, epidemiological observations combined with molecular epidemiology and experimental data are needed to understand and uncover risk factors related to the host, the pathogen, and variants of concern.
Author Contributions
ST: conceptualization. ST, GM, CA, and GN: investigation, writing—original draft, and writing—review and editing. ST and GN: validation. All authors contributed to the article and approved the submitted version.
Conflict of Interest
The authors declare that the research was conducted in the absence of any commercial or financial relationships that could be construed as a potential conflict of interest.
Publisher’s Note
All claims expressed in this article are solely those of the authors and do not necessarily represent those of their affiliated organizations, or those of the publisher, the editors and the reviewers. Any product that may be evaluated in this article, or claim that may be made by its manufacturer, is not guaranteed or endorsed by the publisher.
Acknowledgments
We would like to thank the insightful comments of the reviewers of this article.
References
Abushouk, A., Nasr, A., Masuadi, E., Allam, G., Siddig, E. E., and Fahal, A. H. (2019). The Role of Interleukin-1 cytokine family (IL-1β, IL-37) and interleukin-12 cytokine family (IL-12, IL-35) in eumycetoma infection pathogenesis. PLoS Negl. Trop. Dis. 13:e0007098. doi: 10.1371/journal.pntd.0007098
Ahmed, A. A., and Ad’hiah, A. H. (2021a). Interleukin-37 gene polymorphism and susceptibility to coronavirus disease 19 among Iraqi patients. Meta Gene 31:100989. doi: 10.1016/j.mgene.2021.100989
Ahmed, A. A., and Ad’hiah, A. H. (2021b). Interleukin-37 is down-regulated in serum of patients with severe coronavirus disease 2019 (COVID-19). Cytokine 148:155702. doi: 10.1016/j.cyto.2021.155702
Allam, G., Gaber, A. M., Othman, S. I., and Abdel-Moneim, A. (2020). The potential role of interleukin-37 in infectious diseases: role of IL-37 in HIV-1, viral myocarditis, HCV, HBV, tuberculosis, leprosy, pneumonia, listeria, aspergillosis, candidiasis, and eumycetoma infection. Int. Rev. Immunol. 39, 3–10. doi: 10.1080/08830185.2019.1677644
An, B., Liu, X., Li, G., and Yuan, H. (2017). IL-37 ameliorates coxsackievirus B3-induced viral myocarditis by modulating the Th17/Treg immune response. J. Cardiovasc. Pharmacol. 69, 305–313.
Ballak, D. B., Van Diepen, J. A., Moschen, A. R., Jansen, H. J., Hijmans, A., Groenhof, G., et al. (2014). IL-37 protects against obesity-induced inflammation and insulin resistance. Nat. Commun. 5, 1–13.
Bar, D., Apte, R. N., Voronov, E., Dinarello, C. A., and Cohen, S. (2004). A continuous delivery system of IL-1 receptor antagonist reduces angiogenesis and inhibits tumor development. FASEB J. 18, 161–163. doi: 10.1096/fj.03-0483fje
Barnes, P. J. (2006). How corticosteroids control inflammation: quintiles prize lecture 2005. Br. J. Pharmacol. 148, 245–254. doi: 10.1038/sj.bjp.0706736
Boraschi, D., Lucchesi, D., Hainzl, S., Leitner, M., Maier, E., Mangelberger, D., et al. (2011). IL-37: a new anti-inflammatory cytokine of the IL-1 family. Eur. Cytokine Netw. 22, 127–147. doi: 10.1684/ecn.2011.0288
Bulau, A., Nold, M. F., Li, S., Nold-Petry, C. A., Fink, M., Mansell, A., et al. (2014). Role of caspase-1 in nuclear translocation of IL-37, release of the cytokine, and IL-37 inhibition of innate immune responses. Proc. Natl. Acad. Sci. U.S.A. 111, 2650–2655. doi: 10.1073/pnas.1324140111
Busfield, S. J., Comrack, C. A., Yu, G., Chickering, T. W., Smutko, J. S., Zhou, H., et al. (2000). Identification and gene organization of three novel members of the IL-1 family on human chromosome 2. Genomics 66, 213–216. doi: 10.1006/geno.2000.6184
Cain, D. W., and Cidlowski, J. A. (2017). Immune regulation by corticosteroids. Nat. Rev. Immunol. 17, 233–247.
Casqueiro, J., Casqueiro, J., and Alves, C. (2012). Infections in patients with diabetes mellitus: a review of pathogenesis. Indian J. Endocrinol. Metab. 16:S27. doi: 10.4103/2230-8210.94253
Cavalli, G., and Dinarello, C. A. (2018). Suppression of inflammation and acquired immunity by IL-37. Immunol. Rev. 281, 179–190. doi: 10.1111/imr.12605
Chaudhry, Z., Shawe-Taylor, M., Rampling, T., Cutfield, T., Bidwell, G., Chan, X. H. S., et al. (2021). Short durations of corticosteroids for hospitalised COVID-19 patients are associated with a high readmission rate. J. Infect. 82, 276–316. doi: 10.1016/j.jinf.2021.03.002
Chinn, R. Y., and Diamond, R. D. (1982). Generation of chemotactic factors by Rhizopus oryzae in the presence and absence of serum: relationship to hyphal damage mediated by human neutrophils and effects of hyperglycemia and ketoacidosis. Infect. Immun. 38, 1123–1129. doi: 10.1128/iai.38.3.1123-1129.1982
Colagiuri, S., Guariguata, L., Motala, A. A., and Ogurtsova, K. (2019). Global and regional diabetes prevalence estimates for 2019 and projections for 2030 and 2045: results from the international diabetes federation diabetes atlas, 9 (th) edition. Diabetes Res. Clin. Pract. 157:107843. doi: 10.1016/j.diabres.2019.107843
Conti, P., Ronconi, G., and Caraffa, A. (2020). Induction of pro-inflammatory cytokines (Interleukin-1 and Interleukin-6) and lung inflammation by Coronavirus-19 (COVI-19 or SARS-CoV-2): anti-inflammatory strategies. J. Biol. Regul. Homeost. Agents 34:327–331. doi: 10.23812/CONTI-E
Corrao, S., Pinelli, K., Vacca, M., Raspanti, M., and Argano, C. (2021). Type 2 diabetes mellitus and COVID-19: a narrative review. Front. Endocrinol. 12:609470. doi: 10.3389/fendo.2021.609470
Delamaire, M., Maugendre, D., Moreno, M., Le Goff, M., Allannic, H., and Genetet, B. (1997). Impaired leucocyte functions in diabetic patients. Diabetic Med. 14, 29–34. doi: 10.1002/(SICI)1096-9136(199701)14:1<29::AID-DIA300>3.0.CO;2-V
Diamond, R. D., Haudenschild, C. C., and Erickson, N. F. III (1982). Monocyte-mediated damage to Rhizopus oryzae hyphae in vitro. Infect. Immun. 38, 292–297.
Donath, M. Y., and Shoelson, S. E. (2011). Type 2 diabetes as an inflammatory disease. Nat. Rev. Immunol. 11, 98–107.
Ehrchen, J. M., Roth, J., and Barczyk-Kahlert, K. (2019). More than suppression: glucocorticoid action on monocytes and macrophages. Front. Immunol. 10:2028. doi: 10.3389/fimmu.2019.02028
Gianchandani, R., Esfandiari, N. H., Ang, L., Iyengar, J., Knotts, S., Choksi, P., et al. (2020). Managing hyperglycemia in the COVID-19 inflammatory storm. Diabetes 69, 2048–2053. doi: 10.2337/dbi20-0022
Greenberg, A. S., and Obin, M. S. (2006). Obesity and the role of adipose tissue in inflammation and metabolism. Am. J. Clin. Nutr. 83, 461S–465S. doi: 10.1093/ajcn/83.2.461S
Guan, W., Ni, Z., Hu, Y., Liang, W., Ou, C., He, J., et al. (2020). Clinical characteristics of coronavirus disease 2019 in China. N. Engl. J. Med. 382, 1708–1720.
Hoang, K., Abdo, T., Reinersman, J. M., Lu, R., and Higuita, N. I. A. (2020). A case of invasive pulmonary mucormycosis resulting from short courses of corticosteroids in a well-controlled diabetic patient. Med. Mycol. Case Rep. 29, 22–24. doi: 10.1016/j.mmcr.2020.05.008
Hoffmann, M., Kleine-Weber, H., Schroeder, S., Krüger, N., Herrler, T., Erichsen, S., et al. (2020). SARS-CoV-2 cell entry depends on ACE2 and TMPRSS2 and is blocked by a clinically proven protease inhibitor. Cell 181, 271–280. e8.
Holman, N., Knighton, P., Kar, P., O’Keefe, J., Curley, M., Weaver, A., et al. (2020). Risk factors for COVID-19-related mortality in people with type 1 and type 2 diabetes in England: a population-based cohort study. Lancet Diabetes Endocrinol. 8, 823–833. doi: 10.1016/S2213-8587(20)30271-0
Iacobellis, G. (2020). COVID-19 and diabetes: can DPP4 inhibition play a role? Diabetes Res. Clin. Pract. 162:108125.
Joshi, N., Caputo, G. M., Weitekamp, M. R., and Karchmer, A. W. (1999). Infections in patients with diabetes mellitus. N. Engl. J. Med. 341, 1906–1912.
Kayina, C. A., Maitra, S., Anand, R. K., Ray, B. R., Baidya, D. K., and Subramaniam, R. (2020). Sars-cov-2 infection presenting with hyperglycemia and ketosis: a case series of three diabetic patients. Indian J. Crit. Care Med. 24:1141. doi: 10.5005/jp-journals-10071-23595
Kershaw, E. E., and Flier, J. S. (2004). Adipose tissue as an endocrine organ. J. Clin. Endocrinol. Metab. 89, 2548–2556.
Koh, H., Moh, A. M. C., Yeoh, E., Lin, Y., Low, S. K. M., Ooi, S. T., et al. (2021). Diabetes predicts severity of COVID-19 infection in a retrospective cohort: a mediatory role of the inflammatory biomarker C-reactive protein. J. Med. Virol. 93, 3023–3032. doi: 10.1002/jmv.26837
Kumar, M., Sarma, D. K., Shubham, S., Kumawat, M., Verma, V., Singh, B., et al. (2021). Mucormycosis in COVID-19 pandemic: risk factors and linkages. Curr. Res. Microb. Sci. 2:100057. doi: 10.1016/j.crmicr.2021.100057
Kumar, S., McDonnell, P. C., Lehr, R., Tierney, L., Tzimas, M. N., Griswold, D. E., et al. (2000). Identification and initial characterization of four novel members of the interleukin-1 family. J. Biol. Chem. 275, 10308–10314. doi: 10.1074/jbc.275.14.10308
Law, C. C., Puranik, R., Fan, J., Fei, J., Hambly, B. D., and Bao, S. (2021). Clinical implications of IL-32, IL-34 and IL-37 in atherosclerosis: speculative role in cardiovascular manifestations of COVID-19. Front. Cardiovasc. Med. 8:882. doi: 10.3389/fcvm.2021.630767
Li, A., Ling, Y., Song, Z., Cheng, X., Ding, L., Jiang, R., et al. (2021). Correlation between early plasma interleukin 37 responses with low inflammatory cytokine levels and benign clinical outcomes in severe acute respiratory syndrome coronavirus 2 infection. J. Infect. Dis. 223, 568–580. doi: 10.1093/infdis/jiaa713
Li, C., Ji, H., Cai, Y., Ayana, D. A., Lv, P., Liu, M., et al. (2013). Serum interleukin-37 concentrations and HBeAg seroconversion in chronic HBV patients during telbivudine treatment. J. Interferon Cytokine Res. 33, 612–618. doi: 10.1089/jir.2013.0001
Li, T., Li, H., Li, W., Chen, S., Feng, T., Jiao, W., et al. (2019). Interleukin-37 sensitize the elderly type 2 diabetic patients to insulin therapy through suppressing the gut microbiota dysbiosis. Mol. Immunol. 112, 322–329. doi: 10.1016/j.molimm.2019.06.008
Liu, F., Long, X., Zhang, B., Zhang, W., Chen, X., and Zhang, Z. (2020). ACE2 expression in pancreas may cause pancreatic damage after SARS-CoV-2 infection. Clin. Gastroenterol. Hepatol. 18:2128. doi: 10.1016/j.cgh.2020.04.040
Manson, J. E., Rifai, N., Buring, J. E., and Ridker, P. M. (2001). C-reactive protein, interleukin 6, and risk of developing type 2 diabetes mellitus. JAMA 286, 327–334. doi: 10.1001/jama.286.3.327
Matsumoto, S., Tsuji-Takayama, K., Aizawa, Y., Koide, K., Takeuchi, M., Ohta, T., et al. (1997). Interleukin-18 activates NF-κB in murine T helper type 1 cells. Biochem. Biophys. Res. Commun. 234, 454–457. doi: 10.1006/bbrc.1997.6665
Molteni, E., Astley, C. M., Ma, W., Sudre, C. H., Magee, L. A., Murray, B., et al. (2021). Symptoms and syndromes associated with SARS-CoV-2 infection and severity in pregnant women from two community cohorts. Sci. Rep. 11, 1–11. doi: 10.1038/s41598-021-86452-3
Nold, M. F., Nold-Petry, C. A., Zepp, J. A., Palmer, B. E., Bufler, P., and Dinarello, C. A. (2010). IL-37 is a fundamental inhibitor of innate immunity. Nat. Immunol. 11, 1014–1022.
Pan, G., Risser, P., Mao, W., Baldwin, D. T., Zhong, A. W., Filvaroff, E., et al. (2001). IL-1H, an interleukin 1-related protein that binds IL-18 receptor/IL-1Rrp. Cytokine. 13, 1–7. doi: 10.1006/cyto.2000.0799
Qi, F., Liu, M., Li, F., Lv, Q., Wang, G., Gong, S., et al. (2019). Interleukin-37 ameliorates influenza pneumonia by attenuating macrophage cytokine production in a MAPK-dependent manner. Front. Microbiol. 10:2482. doi: 10.3389/fmicb.2019.02482
Quispe-Cañari, J. F., Fidel-Rosales, E., Manrique, D., Mascaró-Zan, J., Huamán-Castillón, K. M., Chamorro–Espinoza, S. E., et al. (2021). Self-medication practices during the COVID-19 pandemic among the adult population in Peru: a cross-sectional survey. Saudi Pharm. J. 29, 1–11. doi: 10.1016/j.jsps.2020.12.001
Ragab, D., Salah Eldin, H., Taeimah, M., Khattab, R., and Salem, R. (2020). The COVID-19 cytokine storm; what we know so far. Front. Immunol. 11:1446. doi: 10.3389/fimmu.2020.01446
Rammaert, B., Lanternier, F., Zahar, J., Dannaoui, E., Bougnoux, M., Lecuit, M., et al. (2012b). Healthcare-associated mucormycosis. Clin. Infect. Dis. 54, S44–S54.
Rammaert, B., Lanternier, F., Poirée, S., Kania, R., and Lortholary, O. (2012a). Diabetes and mucormycosis: a complex interplay. Diabetes Metab. 38, 193–204. doi: 10.1016/j.diabet.2012.01.002
Rautemaa-Richardson, R., and Richardson, M. D. (2021). Systemic fungal infections. Medicine 45, 757–762.
Rawshani, A., Rawshani, A., Franzén, S., Sattar, N., Eliasson, B., Svensson, A., et al. (2018). Risk factors, mortality, and cardiovascular outcomes in patients with type 2 diabetes. N. Engl. J. Med. 379, 633–644. doi: 10.1056/NEJMoa1800256
Recovery Collaborative Group (2021). Dexamethasone in hospitalized patients with Covid-19. N. Engl. J. Med. 384, 693–704.
Roberts, J., Pritchard, A. L., Treweeke, A. T., Rossi, A. G., Brace, N., Cahill, P., et al. (2020). Why is COVID-19 more severe in patients with diabetes? The role of angiotensin-converting enzyme 2, endothelial dysfunction and the immunoinflammatory system. Front. Cardiovasc. Med. 7:629933. doi: 10.3389/fcvm.2020.629933
Roden, M. M., Zaoutis, T. E., Buchanan, W. L., Knudsen, T. A., Sarkisova, T. A., Schaufele, R. L., et al. (2005). Epidemiology and outcome of zygomycosis: a review of 929 reported cases. Clin. Infect. Dis. 41, 634–653. doi: 10.1086/432579
Sharma, S., Kulk, N., Nold, M. F., Gräf, R., Kim, S., Reinhardt, D., et al. (2008). The IL-1 family member 7b translocates to the nucleus and down-regulates proinflammatory cytokines. J. Immunol. 180, 5477–5482. doi: 10.4049/jimmunol.180.8.5477
Shibata, R., Ouchi, N., Ohashi, K., and Murohara, T. (2017). The role of adipokines in cardiovascular disease. J. Cardiol. 70, 329–334.
Skiada, A., Pavleas, I., and Drogari-Apiranthitou, M. (2020). Epidemiology and diagnosis of mucormycosis: an update. J. Fungi 6:265. doi: 10.3390/jof6040265
Sridhara, S. R., Paragache, G., Panda, N. K., and Chakrabarti, A. (2005). Mucormycosis in immunocompetent individuals: an increasing trend. J. Otolaryngol. 34, 402–406.
Su, Z., and Tao, X. (2021). Current understanding of IL-37 in human health and disease. Front. Immunol. 12:2562. doi: 10.3389/fimmu.2021.696605
Sugar, A. M. (1990). “Agents of mucormycosis and related species,” in Principles And Practice Of Infectious Diseases, eds G. L. Mandell, R. G. Douglas Jr., and J. E. Bennett (Boston, MA: Department of Medicine, Boston University School of Medicine), 1962–1972.
Summers, K. L., Marleau, A. M., Mahon, J. L., McManus, R., Hramiak, I., and Singh, B. (2006). Reduced IFN-α secretion by blood dendritic cells in human diabetes. Clin. Immunol. 121, 81–89. doi: 10.1016/j.clim.2006.05.015
Tilg, H., and Moschen, A. R. (2006). Adipocytokines: mediators linking adipose tissue, inflammation and immunity. Nat. Rev. Immunol. 6, 772–783. doi: 10.1038/nri1937
van de Veerdonk, F. L., Gresnigt, M. S., Oosting, M., van der Meer, J. W., Joosten, L. A., Netea, M. G., et al. (2015). Protective host defense against disseminated candidiasis is impaired in mice expressing human interleukin-37. Front. Microbiol. 7:762. doi: 10.3389/fmicb.2014.00762
van Paassen, J., Vos, J. S., Hoekstra, E. M., Neumann, K. M., Boot, P. C., and Arbous, S. M. (2020). Corticosteroid use in COVID-19 patients: a systematic review and meta-analysis on clinical outcomes. Crit. Care 24, 1–22. doi: 10.1186/s13054-020-03400-9
Varikasuvu, S. R., Varshney, S., and Dutt, N. (2021). Markers of coagulation dysfunction and inflammation in diabetic and non-diabetic COVID-19. J. Thromb. Thrombolysis 51, 941–946. doi: 10.1007/s11239-020-02270-w
Waldorf, A. R., Ruderman, N., and Diamond, R. D. (1984). Specific susceptibility to mucormycosis in murine diabetes and bronchoalveolar macrophage defense against Rhizopus. J. Clin. Invest. 74, 150–160. doi: 10.1172/JCI111395
Wang, L., Quan, Y., Yue, Y., Heng, X., and Che, F. (2018). Interleukin-37: a crucial cytokine with multiple roles in disease and potentially clinical therapy. Oncol. Lett. 15, 4711–4719. doi: 10.3892/ol.2018.7982
World Health Organization (2019). Classification Of Diabetes Mellitus. Geneva: World Health Organization.
World Health Organization (2020). No Title: Corticosteroids for COVID-19: Living Guidance, 2 September 2020. Geneva: World Health Organization.
Yamauchi, T., and Kadowaki, T. (2008). Physiological and pathophysiological roles of adiponectin and adiponectin receptors in the integrated regulation of metabolic and cardiovascular diseases. Int. J. Obes. 32, S13–S18. doi: 10.1038/ijo.2008.233
Yang, J., Lin, S., Ji, X., and Guo, L. (2010). Binding of SARS coronavirus to its receptor damages islets and causes acute diabetes. Acta Diabetol. 47, 193–199. doi: 10.1007/s00592-009-0109-4
Yi, Y., Lagniton, P. N., Ye, S., Li, E., and Xu, R. (2020). COVID-19: what has been learned and to be learned about the novel coronavirus disease. Int. J. Biol. Sci. 16:1753. doi: 10.7150/ijbs.45134
Zhang, J., Dong, X., Cao, Y., Yuan, Y., Yang, Y., Yan, Y., et al. (2020). Clinical characteristics of 140 patients infected with SARS-CoV-2 in Wuhan, China. Allergy 75, 1730–1741. doi: 10.1111/all.14238
Keywords: COVID-19, T2D, corticosteroids, mucormycosis, IL-37
Citation: Tokajian S, Merhi G, Al Khoury C and Nemer G (2022) Interleukin-37: A Link Between COVID-19, Diabetes, and the Black Fungus. Front. Microbiol. 12:788741. doi: 10.3389/fmicb.2021.788741
Received: 03 October 2021; Accepted: 23 December 2021;
Published: 13 January 2022.
Edited by:
Leland Shapiro, University of Colorado Anschutz Medical Campus, United StatesReviewed by:
Yongming Sang, Tennessee State University, United StatesBruno Andrade, Universidade Estadual do Sudoeste da Bahia, Brazil
Copyright © 2022 Tokajian, Merhi, Al Khoury and Nemer. This is an open-access article distributed under the terms of the Creative Commons Attribution License (CC BY). The use, distribution or reproduction in other forums is permitted, provided the original author(s) and the copyright owner(s) are credited and that the original publication in this journal is cited, in accordance with accepted academic practice. No use, distribution or reproduction is permitted which does not comply with these terms.
*Correspondence: Sima Tokajian, c3Rva2FqaWFuQGxhdS5lZHUubGI=