- 1Institute of Bast Fiber Crops, Chinese Academy of Agricultural Sciences, Changsha, China
- 2Hunan Institute of Animal and Veterinary Science, Changsha, China
- 3College of Veterinary Medicine, Hunan Agricultural University, Changsha, China
Given the desirable results of using probiotics and enzyme preparations as feed supplements in poultry health, here, the effects of Bacillus and Non-starch Polysaccharase (NSPase) on the growth performance, serum antioxidant profiles, and gut microbial communities of early stage ducks is investigated. A total of 400 Zhijiang ducks (of similar body weight and 1 day age) was selected and randomly divided into four groups. The feeding period was 28 days. Each group contained 10 replicates of 10 birds. Control group (I) was fed with basal diet, while treatment groups II to IV were fed, respectively, with 150 mg/kg NSPases, 25 mg/kg Bacillus probiotics, and 150 mg/kg NSPases + 25 mg/kg Bacillus probiotics in their basal diet. The results demonstrated that dietary Bacillus (25 mg/kg) increased average final weight, average daily gain (ADG), and decreased the malonaldehyde (MDA) in birds (P < 0.05). Dietary Bacillus (25 mg/kg) and NSPases + Bacillus (150 mg/kg + 25 mg/kg) presented much higher glutathione (GSH) and activities of superoxide dismutase (SOD) in birds (P < 0.05). Additionally, as revealed by β-diversity indices and analysis of similarities, dietary NSPases + Bacillus could affect the ileum microbial abundances and diversities at the genera level (P < 0.05), but it had no effect on the caecal microbiota. Also, 16S rRNA sequencing revealed that dietary Bacillus and NSPases + Bacillus increased the populations of Ruminococcaceae genera in the cecum (P < 0.05), and S24-7_group and Lactobacillus genera in the ileum (P < 0.05). However, dietary NSPases and Bacillus alone and in combination could significantly decrease the content of Bacteroides in the ileum (P < 0.05). According to Spearman correlation analysis, 7 ilea bacterial microbiomes (S24-7 group, Lactobacillus, Subgroup 2, Subgroup 1, Kitasatospora, Candidatus Solibacter, and Akkermansia) were positively correlated with SOD (P < 0.05). In conclusion, Bacillus (25 mg/kg) and NSPases (150 mg/kg) included in the diet could efficiently enhance the growth performance by altered gut microbiota composition at the genera level and antioxidant indices of ducks.
Introduction
Due to the antibiotic resistance of bacteria being on the rise (Guo et al., 2016) and the increasing consumer concern regarding poultry products, antibiotic-free flocks (Gadde et al., 2017), and environmental sustainability (Xing et al., 2015), a prohibition on antibiotics had been imposed in Europe (Stolker et al., 2007), South Korea (Kumar et al., 2012), and China (Hu and Cowling, 2020) since 2006, 2012, and 2020, respectively. Hence, replacing antibiotics with alternative products (Han et al., 2017) has become a hot research topic in recent years. Probiotics and enzymes are attracting much attention as important alternatives to antibiotics.
Bacillus is widely used in poultry diets as a type of probiotic, which improves the productive performance of poultry (Naumova et al., 2021) by producing naturally synthesized antimicrobial peptides, maintaining microbial flora balance in the intestie, accelerating the increase of beneficial microbiota along the gastrointestinal tract, and adjusting the immunological function and gut morphology (Sen et al., 2012; Grant et al., 2018). Many findings confirmed the significant improvement of growth performance, immune response, cecal microbial population, and intestinal morphology of weanling pig (Lee et al., 2014), Cherry Valley ducks (Guo et al., 2016), and Ross broiler chicks (Sen et al., 2012) with Bacillus-based diets. In addition, lacking enough enzymes to fully digest fiber, birds rely on exogenous enzymes in their diets to improve the fiber digestion process (Alagawany et al., 2018). As one of the most important exogenous enzymes, non-starch polysaccharidase (NSPase) is commonly used in poultry diets and plays many essential roles such as breaking down the non-starch polysaccharides, releasing nutrients encapsulated by the cell wall, reducing intestinal viscosity, improving animals’ utilization of nutrients, and affecting the composition and metabolic potential of bacterial populations (Kiarie et al., 2013; Ravindran, 2013; Amerah, 2015). Ao et al. (2010) and Coppedge et al. (2012) reported the supplementation of NSPase could improve the growth performance of market broilers and pigs and be used as a strategy to degrade antinutritional compounds so as to reduce dietary energy levels and costs.
Both Bacillus and NSPase can improve the productive performance of poultry, and are able to directly or indirectly affect the intestinal flora. However, it is not clear whether there is a synergistic effect between them. A few studies demonstrated that there was a certain synergistic effect on improving intestinal health, promoting nutrient digestion and growth in broiler chickens, when both Bacillus and NSPase were used as a supplement in the feed (Lin Q. et al., 2012; Wealleans et al., 2017). However, no such study has been conducted in ducks. In the present study, we aimed to study the effects of Bacillus and NSPase on the growth performance, serum antioxidant profiles, intestine morphology, and intestinal microbiota composition in ducks.
Materials and Methods
All the experimental procedures of this study were approved by the Animal Care and Use Committee of the Institute of Bast Fiber Crops, Chinese Academy of Agricultural Sciences.
Experimental Design, Diets, and Birds
Zhijiang duck, an important indigenous bird breed in Southern China, is characterized by rapid growth, strong disease resistance, unique flavor, and delicious meat. Usually, they are sold as products in the marketplace at the age of 56 days, often with an average body weight of around 2,650 g for a single duck. From the age of 1–28 days, Zhijiang duck are at the critical stage of intestinal development and maximum growth. Therefore, a total of 400 female Zhijiang ducks (aged 1 day and free of infectious disease) were obtained from Hunan Hexiang Duck Industrial Co., Ltd. They were then transferred into the laboratory of the Bast Fiber Crops Institute, Chinese Academy of Agricultural Sciences for a feeding period of 28 days. Feed and water were provided ad libitum during the whole trial period. Each Zhijiang duck was weighed at the beginning to obtain the average initial weight, in order to divide them into four groups without significant difference among groups. Each group (100 Zhijiang ducks) was further subdivided into 10 cages (10 ducks/cage), and the dimension of each cage was 150 cm × 150 cm. Group I received a basal diet (BD), Group II received BD supplemented with 150 mg/kg NSPases (Manufactured by Shanghai CJYOUTELL Biotechnology Co., Ltd., China); the main components of this NSPases are cellulase (≥ 2,000 IU/g) and xylanase (≥ 30,000 IU/g). Group II received BD supplemented with 25 mg/kg Bacillus probiotics; the main components being Bacillus subtilis and Bacillus lichenif or mi s, 5 × 1012CFU/g, manufactured by Wuhan Xiongfeng Technology Co., Ltd., China. Group IV received BD supplemented with 150 mg/kg NSPases + 25 mg/kg Bacillus probiotics. The BD was prepared in accordance with the Nutrient Requirements of Ducks (National Research Council, 1994) and the Nutrient Requirements of Meat-type Duck (China, NY/T 2122-2012) (Ministry of Agriculture of the People’s Republic of China, 2012; Table 1).
Growth Performance
Birds were weighed at the beginning (day 1) and the end (day 28) of the trial for calculation of growth performance. Average daily weight gain (ADG), average daily feed intake (ADFI), and feed/gain ratio (F/G) were calculated according to the data from each cage for the whole experimental period. The birds were fasted for 12 h before weighting and sampling.
Sample Collection
At the end of the trail, one bird per cage with a live weight close to the mean (10 birds per group) were selected for sampling. Blood samples from the wing vein were collected into vacuum blood collection tubes and were centrifuged at 3,000 × g for 10 min to collect the serums. Birds were slaughtered by exsanguination from the jugular vein. Samples of the small intestine were immediately removed and then divided into three parts: Duodenum, jejunum, and ileum. Intestinal tissue samples were cut from the medial of the duodenum, jejunum, and ileum with a segment of 1.5 cm, and lightly flushed using physiological saline (154 mmol/L) and drained on filter paper. Then these fresh samples were fixed into 10% neutral buffered formalin for further analysis of intestinal mucosal morphology (Watkins et al., 2004; Applegate et al., 2005). Samples of chyme in each duck’s ileum and cecum were collected separately into 2 mL EP tubes and flash-frozen using liquid N2 and stored at –80°C until analysis.
Serum Antioxidant Capacity
Samples of serum were analyzed for malonaldehyde (MDA), antioxidant biomarkers including glutathione (GSH), activities of superoxide dismutase (SOD),glutathione peroxidase (GSH-Px), total antioxidant capacity (T-AOC), and Catalase (CAT) were determined by the commercial assay kits (Nanjing Jiancheng Bioengineering Institute, China) with an automated fluorescence instrument (MultiskanM™ SkyHigh, Thermo Fisher Scientific, Waltham, MA, United States).
Measurement of Intestinal Mucosal Morphology
The intestinal morphological measurement of the duodenum, jejunum, and ileum was based on the method reported in our previous research (Lin Q. et al., 2017). Briefly, 1.5 cm-intestinal tissue samples of the duodenum, jejunum, and ileum were fixed and embedded in paraffin, sectioned at a thickness of 5 or 6 μm using a microtome (RM-2235, Leica microsystems AG., Hessen, Germany), then mounted on glass slides and subsequently stained with hematoxylin and eosin (HE staining). We observed the finished slides and chose the typical microscopic fields to take photos of under an Olympus Van-Ox S microscope (Opelco, Washington, DC). Visual measurements of the villus height, crypt depth, and intestinal wall thickness from each slide were made on 10 readings at 40 × and 100 × magnifications using an image analysis system (Image-Pro, Media Cybernetics, Inc., Silver Springs, MD, United States). Then the ratios of villus height to crypt depth (V/C) can be calculated.
Gut Microbiota Composition by 16S rRNA Gene Sequencing
The genomic DNA of the ileum and cecum microbial community were extracted according to the manufacturer’s instructions by the E.Z.N.A. DNA kits (Omega Bio-tech, Norcross, GA, United States). Ten ileum/cecum digesta samples were mixed in pairs for the 16S rDNA sequence determination. The 16S rRNA gene V3 + V4 regions of the bacteria were amplified with the primer pairs 806R (5′-GGACTACHVGGGTWTCTAAT-3′) and 338F (5′-ACTCCTACGGGAGGCAGCAG-3′) combined with adapter sequences and barcode sequences. Next, the DNA was purified by AxyPrep DNA Gel Extraction Kits (Axygen Bioscience, Union City, CA, United States). Finally, the sequencing of 16S rRNA gene was performed on an Illumina HiSeq 2500 platform (Illumina, San Diego, CA, United States) with purified amplicons were paired.
Reads with complete (length > 300 bp) sequence barcodes were screened for the following analysis. Obtained sequences of samples were filtered by using QIIME (version 1.17) software and the number of operational taxonomic units (OTUs) with a cut of 97% sequence similarity were determined by using UPARSE (version 7.1). Each OTU represented sequence was analyzed by Ramer-Douglas-Peucker (RDP) Classifier version 2.2 via the 16S rRNA database.
The alpha diversities of the ileal and cecal microbiota, including abundance-based coverage estimator (ACE), Chao1 estimator, Simpson and Shannon diversity index were calculated to investigate the richness and diversity of the community, respectively. Principal coordinate analysis (PCoA), and Non-MetricMulti- Dimensional Scaling (NMDS) were calculated to evaluate the difference of the microbial community based on the Bray-Curtis dissimilarity matrix. The analysis procedures of ileal and cecal microbiota were processed on the free online platform of BMK Cloud Platform (BioMarker Technologies Co., Ltd., Beijing, China).
Statistical Analysis
One-way ANOVA model was performed to identify significant differences in growth performance, serum antioxidant profiles, intestinal mucosal morphology, and richness and community diversity of bacteria. All the results were presented as means plus standard errors of the means (SEM). Differences between means of all groups were considered significant at P-value less than 0.05. The analysis of statistical comparison was conducted on the basis of Student’s t-test to declare the difference of the relative abundance of the ileal and cecal microbiota between two groups. Spearman correlation analysis was performed to identify the relationship between ileum and cecum microbial community and measured parameters. Heatmap was constructed using Prism 9.0 (GraphPad Software, San Diego, CA). All the statistical analysis was done with SPSS 19.0 (IBM, Armonk, New York).
Results
Growth Performance
Growth performances are shown in Table 2. Compared to the control group I, the values of average final weight and ADG showed a significant increase in Group III, whereas Group II and Group IV exhibited an increasing trend with no significant difference (P > 0.05). The ADFI and F/G of ducks among any groups did not change significantly (P > 0.05) during the entire experimental period.
Serum Antioxidant Profiles
Six serum antioxidant indicators were presented in Table 3. GSH-Px (P = 0.878), T-AOC (P = 0.411) and CAT (P = 0.282) in different treatment group showed similar levels. But significant differences were told among groups in the serum levels of GSH, SOD, and MDA (P < 0.01). Compared to Group I, Groups III, and IV showed a significant increase in the serum level of SOD and GSH (P < 0.01). In contrast, the level of MDA showed a significant decrease in Groups II, III, and IV compared to that of Group I.
Intestinal Mucosal Morphology
According to Table 4, no significant difference (P > 0.05) was observed on any intestinal morphology parameters of all groups. But for the duodenum, jejunum, and ileum, an increasing trend in villus height and V/C, and a decreasing trend in Crypt depth has been shown in Groups II, III, or IV when compared to Group I.
Modulation of Intestinal Microbiota
Shannon, Simpson, ACE, and Chao, are important indexes of the α diversity of a bacterial community. The results (as shown in Table 5) were obtained through the 16S rRNA sequencing. For ileum, the values of ACE (P = 0.007) and Chao (P = 0.006) showed a significant increasing trend in Group IV, and they also increased in Group II and Group III. But instead, the α diversity parameters of the cecum showed no change (P > 0.05) among groups. PCoA and NMDS revealed the variation between microbiome profiles based upon Bray-Curtis dissimilarity. For cecum, PCoA plot (Figure 1A) and NMDS plot (Figure 1B) revealed no differences in microorganism distributions between the four groups at the genera level. However, in the ileum, samples from Group I and Group II were clearly distributed in both clusters, on the left and right parts of both PC1 (Figure 1C) and NMDS1 (Figure 1D), respectively.
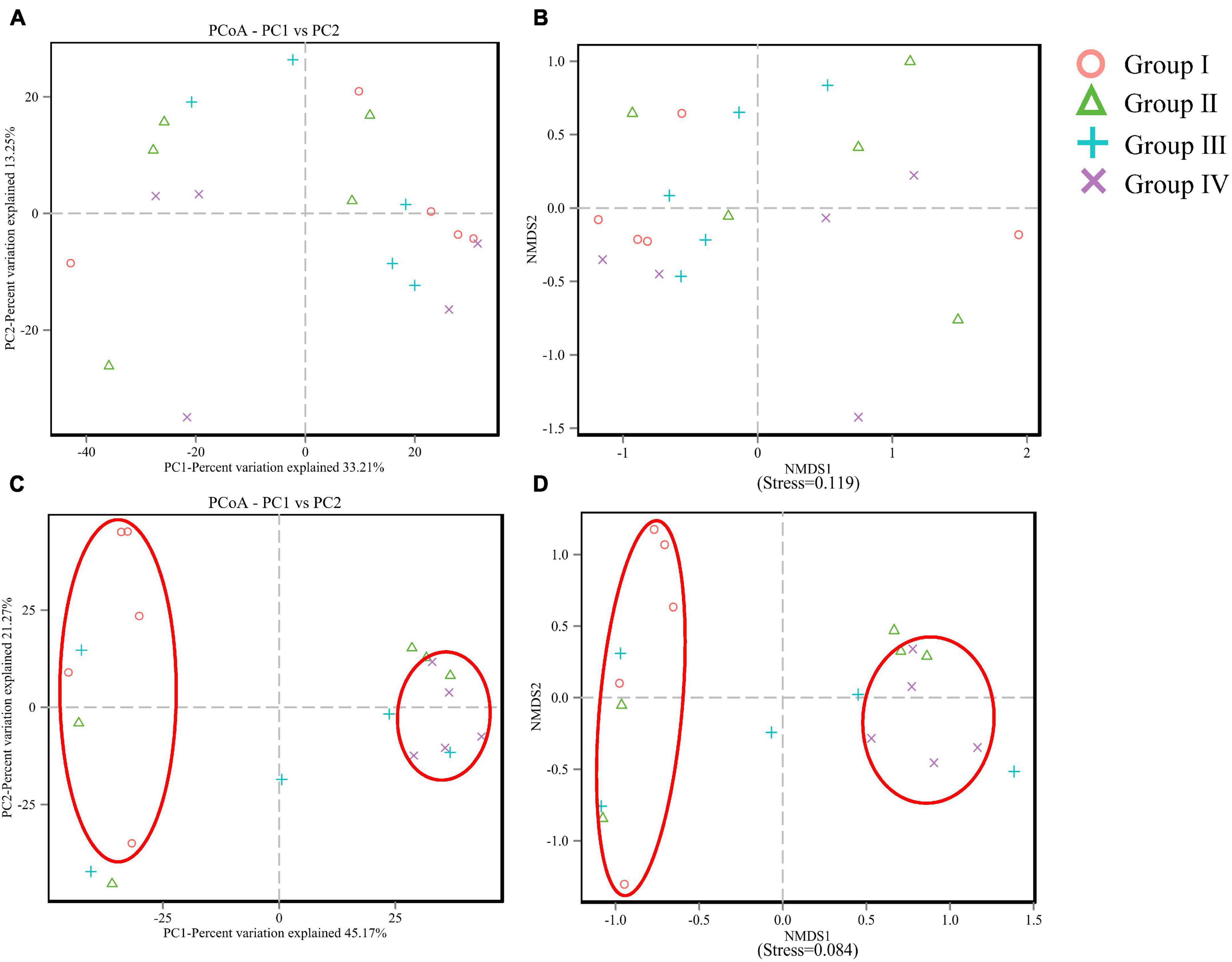
Figure 1. PCoA and NMDS analysis of cecum (A,B) and ileum (C,D) microbial community compositions based on information of operational taxonomic units (OTU).
In cecum, microbial communities among different groups at the genus level are shown in Figure 2A. Faecalibacterium, Ruminococcaceae, Lachnospiraceae, Torques group, and UCG-014 were the dominant microbes and occupied approximately 50% of the total genera. Faecalibacterium was detected in the highest abundance in Group I, whereas the Ruminococcaceae and Torques group showed lower abundance than other groups. As for Lachnospiraceae, higher abundance was observed in Group II and IV. For the microbial composition analysis, pair-wise comparisons with Student’s T approach were used between experimental groups and the control group. Significant differences were found in Ruminococcaceae (P < 0.01) (Figure 2B) and vadinBB60 group (P < 0.05) (Figure 2C) between Group I and IV, Ruminococcaceae (P < 0.05) (Figure 2B) between Group I and IV.
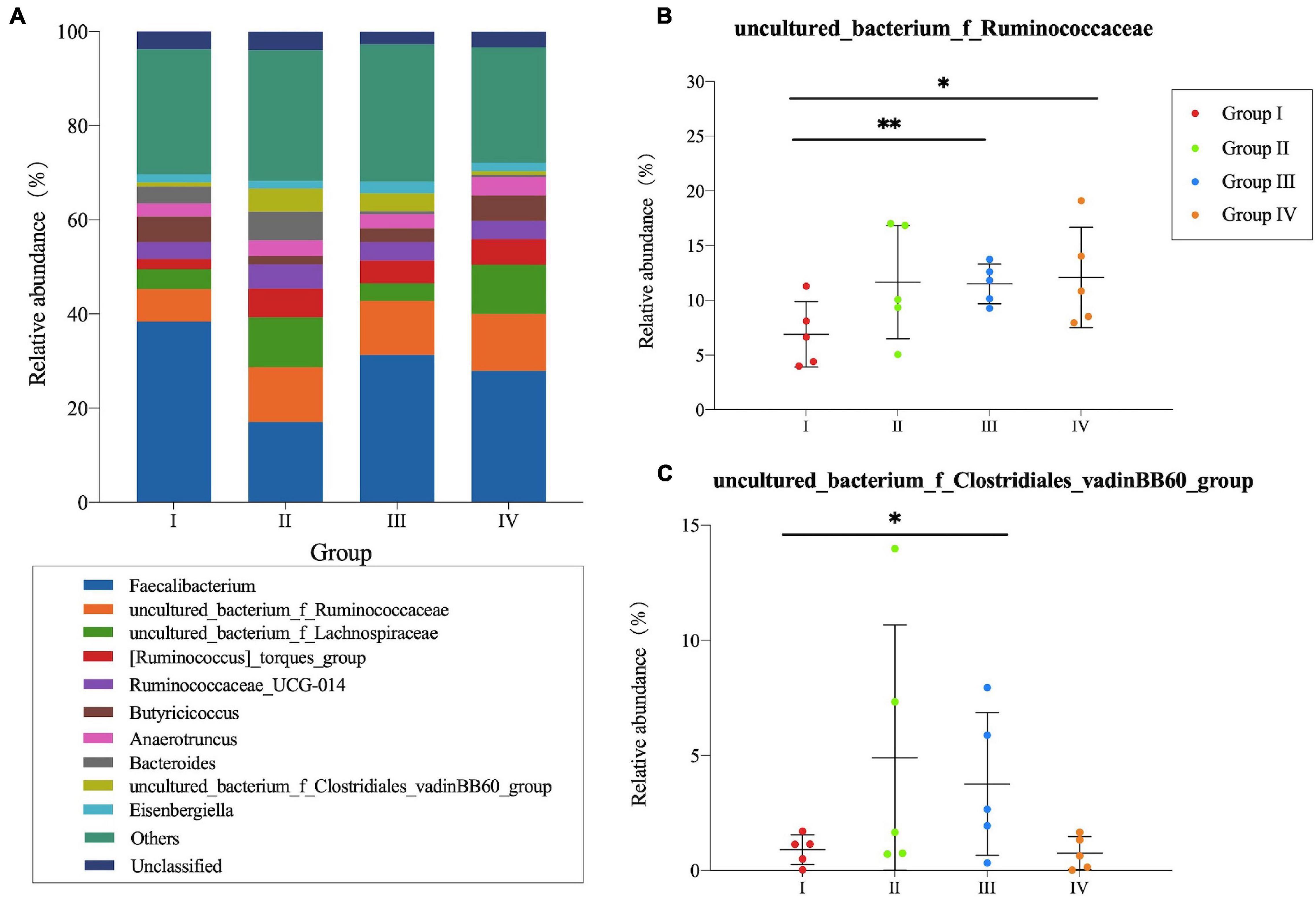
Figure 2. Community structure of bacteria at genus level in the cecum of Zhijiang ducks. Histogram (A) of the top 10 genera in each group. Significant difference relative abundance of Ruminococcaceae (B) and vadinBB60_group (C) at the genera level. (*P < 0.05 and **P < 0.01 compared with control group).
In the ileum (Figure 3A), Candidatus Arthromitus and Bacteroides were dominant genera in Groups I, II, and III which represented more than 30% of the genus type. However, they were detected in Group IV with low abundance. As for Barnesiella, Intestinibacter, and Faecalibacterium, higher abundance was observed in Group I. Additionally, the relative abundance of S24-7 group, Lactobacillus, and Subgroup 2 in Group IV were higher compared with other groups. Through the Student’s T-test, there were significant differences of Bacteroides, S24-7 group, Lactobacillus, and Subgroup 2 (P < 0.05) (Figures 3B–E) between Group I and test groups.
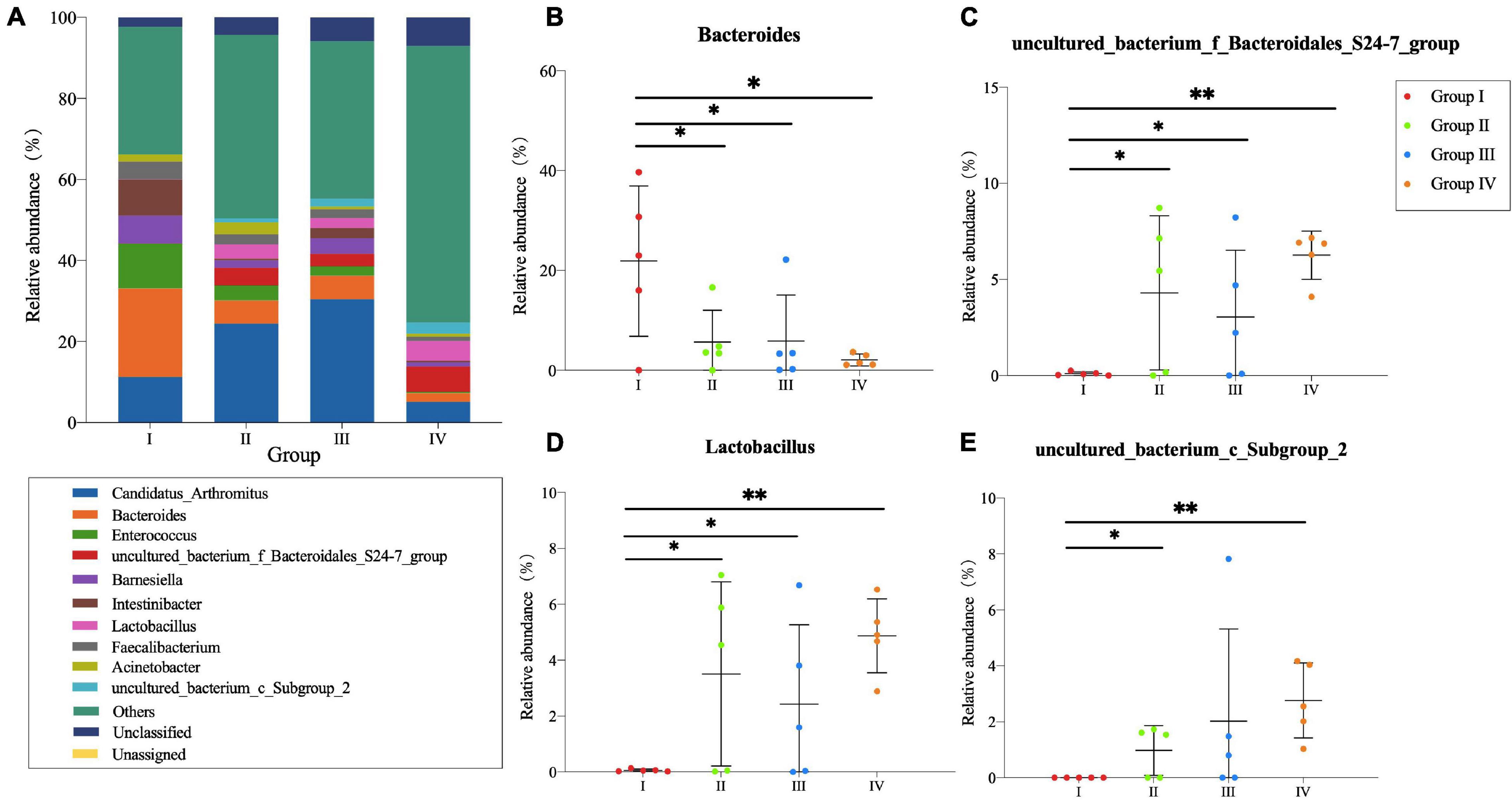
Figure 3. Community structure of bacteria at genus level in the ileum of Zhijiang ducks. Histogram (A) of the top 10 genera in each group. Significant difference relative abundance of Bacteroides (B), S24-7_group (C), Lactobacillus (D), and Subgroup_2 (E) at the genera level. (*P < 0.05 and **P < 0.01 compared with the control group).
Furthermore, the correlation between the microbiota composition of ceacum/ileum and the indices of growth performance and antioxidative capacity were shown in Figure 4. After Spearman’s correlation analysis in ceacum, ADFI and F/G were found to have significant positive correlations with Faecalibacterium (P = 0.018 and P = 0.031), while negative correlations were examined between GSH and the Bacteroides (P = 0.039), and ADFI and the Eisenbergiella (P = 0.027). In ileum (Figure 4B), significantly positive results were observed between SOD and S24-7 group (P = 0.013), Lactobacillus (P = 0.024), Subgroup 2 (P = 0.013), Subgroup 1 (P = 0.010), Kitasatospora (P = 0.002), Candidatus Solibacter (P = 0.009), and Akkermansia (P = 0.040). Meanwhile, SOD was significantly negative in the Bacteroides (P = 0.024) and Alistipes (P = 0.009). In addition, significant positive correlations were existed between T-AOC and Akkermansia (P = 0.015),GSH and Subgroup 1 (P = 0.042),and GSH and Kitasatospora (P = 0.042).
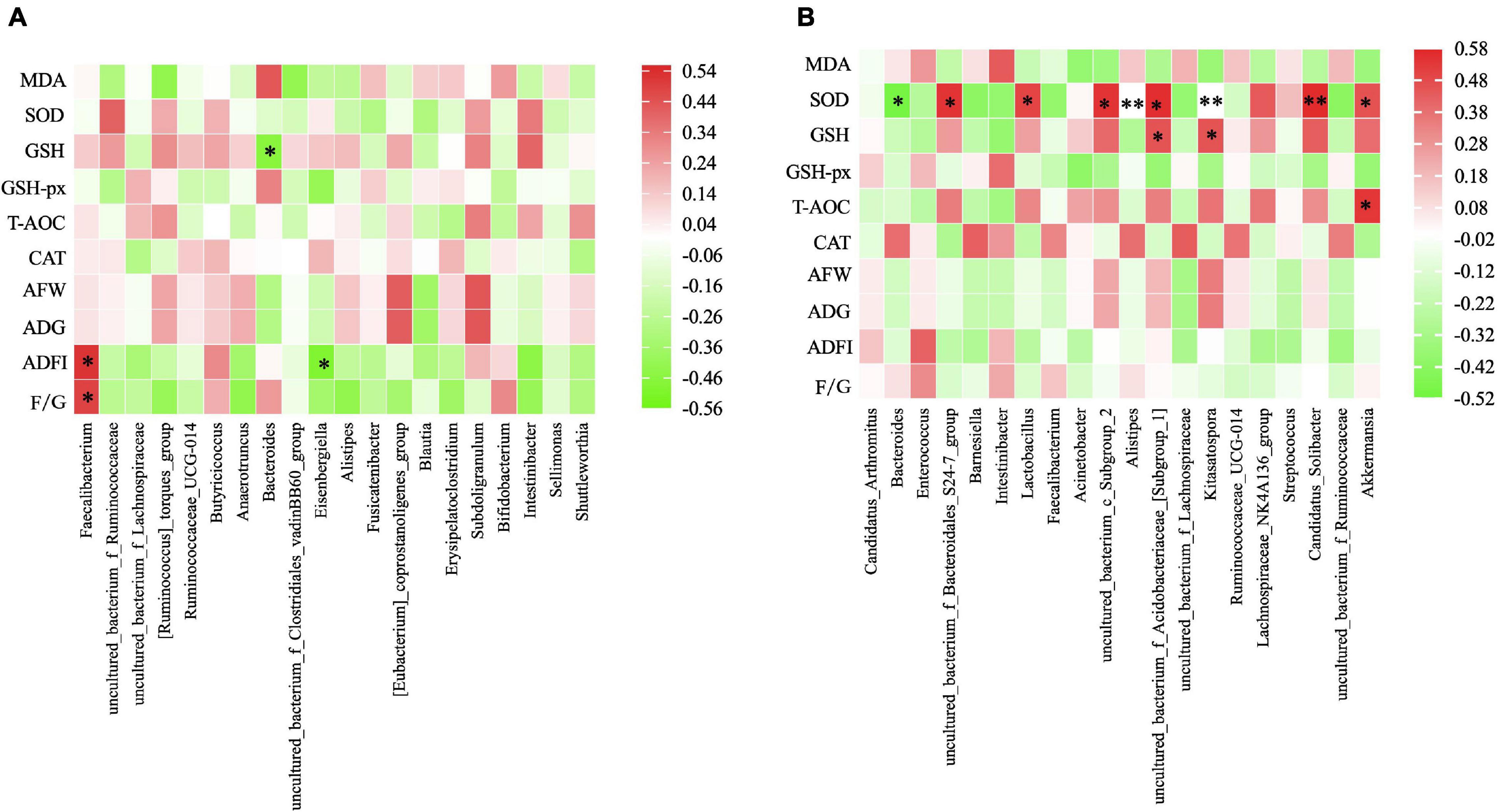
Figure 4. Heatmap of Spearman rank correlation between the cecal microbiota. (A) /ileum microbiota (B) and the measured parameters under different treatment. The genus with relative abundance in the top 10 are presented and the intensity of the colors performed the degree of association. Green, negative correlation; Red, positive correlation. AFW, average final weight; ADG, average daily weight gain; ADFI, average daily feed intake; F/G, feed to gain ratio. MDA, malonaldehyde; GSH, glutathione; SOD, activities of superoxide dismutase; GSH-Px, glutathione peroxidase; T-AOC, total antioxidant capacity; CAT, catalase. *P ≤ 0.05, **P ≤ 0.01.
Discussion
Several lines of studies demonstrated that Bacillus Probiotics could improve the growth performance of poultry (Jeong and Kim, 2014; Lee et al., 2015; Lin Y. et al., 2017). In the current study, we found that Bacillus probiotics supplementation could increase the average final weight and ADG by modulating antioxidative status and intestinal microflora. In fact, the antioxidant effect of Bacillus Probiotics has been verified in rats before (Paik et al., 2005). In our study, we found Bacillus probiotics have the efficacy of significantly decreasing the serum level of MDA and increasing the level of SOD and GSH. These results revealed that feeding ducks with Bacillus Probiotics can also improve the antioxidant status. Meanwhile, there were a higher relative proportion of the genera Ruminococcaceae, vadinBB60 group, and S24-7 group in the Bacillus-fed group in the cecal or ileal microbial community. Many species belonging to these genera are able to produce short-chain fatty acid (SCFA), especially butyrate (Hooda et al., 2012; Ridlon et al., 2015), which serves as a preferred energy source for enterocytes and a known regulator of cellular differentiation and proliferation within the intestinal mucosa (Sikandar et al., 2017; Bedford and Gong, 2018). This can contribute to improving the morphological development of the intestines and to reinforce the intestinal defense barrier, for instance, strengthening tight junctions, thereby promoting animal growth (Le Blay et al., 2000; Fukunaga et al., 2003; Bordin et al., 2004; Peng et al., 2007). Additionally, a significant increasing trend was also observed for Lactobacillus of the ileum in the Bacillus-treated group. This point deserves further investigation as it might be of interest, as Lactobacillus may provide nutrients to the host and help defend against opportunistic pathogens (Cross, 2002; LeBlanc et al., 2013). Interestingly, the Bacteroides genus was largely decreased with the presence of Bacillus, which conflicts with the findings of a very recent broiler study (Wang et al., 2017). However, studies using other species, such as swine, have shown that Bacillus subtilis supplementation decreased the copy numbers and percentage of Bacteroidetes while it increased the percentage of Firmicutes in the cecal contents (Cui et al., 2013). Similarly, a significant decrease trend was also revealed in Bacteroidetes of the ileum in our study. Given the fact that the decrease in Bacteroidetes and the increase in the Firmicutes/Bacteroidetes ratio are positively correlated with body mass index in humans (Koliada et al., 2017) and associated with an increase in ADG (Salaheen et al., 2017), our data revealed that Bacillus Probiotics promotes duck growth by improving the intestinal flora.
Nowadays, poultry feed with higher levels of NSP may reduce the digestibility of nutrients in their diets (Salim et al., 2010), which can lead to poorer growth and performance of birds (Annison and Choct, 1991). One solution to this issue accounting for feed cost and variability is to use exogenous enzymes (Woyengo et al., 2019), including NSPases. In our study, the addition of NSPases increased the average final weight and ADG of ducks, but insignificantly. A similar result was also obtained by Uthai et al. (2004). They found NSPase type mixture supplementation could improve the growth performances (average total weight gained, average daily gain, and average feed intake) of pigs also with non-statistically significant differences. Besides, Ao et al. (2010) reported that 2% NSPase supplementation to based diets for weaned piglets did not significantly improve performance. But interestingly, the average daily gain and gain/feed were increased 4.40 and 6.26% when fed with 1% NSPase supplementation. Variation in the results is likely associated with the content of substrates (Ao et al., 2010). However, a significant increase was found in the proportions of the S24-7 group, Lactobacillus, and Subgroup 2, while a significant decrease was found in the proportion of Bacteroides in the NSPase-treated group at the ileal level. Considering the results that the decrease in Bacteroidetes and the increase in S24-7 group, Lactobacillus, and Subgroup 2 are positively correlated with SOD activity. Thus, ducks fed with NSPases showed improved growth performance, and increased antioxidant capacity might be linked to the microbial changes. In many studies, the change of intestinal microflora was beneficial for the health and welfare of poultry. Naumova et al. (2021) found that the beneficial effect on the production of ducks was associated with the changes in gut microbiota due to Bacillus-feed probiotic supplementation. Dietary probiotics can enhance growth performance by the regulation of intestinal microbial composition, the immune system, and maintenance of intestinal integrity and barrier function, as described by Tuohy et al. (2003). In our study, the major changes were found within the foregut area and the predominant role of Lactobacillus was enhanced. Lactobacillus consists of gram-positive and facultative anaerobes that produce lactic acid, which can create a low pH environment and inhibit the growth of pathogens (Rodriguez-Cabezas et al., 2010). In addition, Lactobacillus was proven to be of antioxidant activity (Lin and Chang, 2000), and having the capacity of scavenging free radicals to alleviate damages induced by oxidative stress (Xin et al., 2014). It indicated that the increase of the genus Lactobacillus probably enhances the antioxidant activity of duck. However, other bacterial species and their interaction (significantly positively associated with SOD) were still unclear.
In the present study, Bacillus probiotics and NSPases supplementation have been proven to be a potential method to improve the average final weight and ADG of ducks. Our findings are consistent with the result reported by Lin Q. et al. (2012), who demonstrated that Bacillus probiotics and NSPases showed obvious synergistic growth-promoting effects on yellow-feathered broilers. Most noteworthily, compared to the basal diet group, the diversity index of ACE and Chao increased when the treatment of NSPase or Bacillus Probiotics alone or combined, especially, the significantly increased (P < 0.05) of ACE and Chao revealed in group IV. The PCoA and NMDS results further indicated that the group of diet NSPases + Bacillus was far from the control group, which was consistent with the above ACE and Chao analysis results. Our results were consistent with Klein et al. (2015) and Liu et al. (2018). They reported that the beneficial effects may be observed from the use of multiple enzymes or mixed-use of Bacillus subtilis and photosynthetic bacteria. Moreover, a significant increase was found in proportions of Ruminococcaceae, S24-7 group, Lactobacillus, and Subgroup 2, and a significant decrease was found in the proportion of Bacteroides in NSPase + Bacillus Probiotics-treated group at the cecal or ileal level. An obvious change in ACE or Chao index would suggest the deep changes in microbial diversity. On one hand, NSPase might affect the intestinal microbiota by reducing the undigested substrates and some short-chain oligosaccharides with potential prebiotic effects were created (in situ) from cell wall NSP (Kiarie et al., 2013). On the other hand, Bacillus probiotics could influence the distribution and colonization of the innate microflora along the gastrointestinal tract, reduce the competition for nutrients between microbes and the host, promote the growth and proliferation of other good symbiotic bacteria (Grant et al., 2018). To summarize, it has been suggested that Bacillus Probiotics and NSPases have a significant synergistic effect on improving the intestinal microflora of ducks.
Conclusion
Including Bacillus (25 mg/kg) and NSPases (150 mg/kg) in the diet could efficiently enhance growth performance via altering gut microbiota composition at the genera level and antioxidant indices of ducks. By comparison, the supplementation combining both Bacillus and NSPases showed the best effect for microbial abundances and diversities. Thus, using an additive of both Bacillus and NSPases could be recommended in diets during the early stage of Zhijiang ducks.
Data Availability Statement
The data analyzed in this study is subject to the following licenses/restrictions: The data used to support the findings of this study are available from the corresponding author upon request. Requests to access these datasets should be directed to QL, bGlucWlhbkBjYWFzLmNu.
Ethics Statement
The animal study was reviewed and approved by the experimental procedures of this study were approved by the Institutional Animal Care and Use Committee of Institute of Bast Fiber Crops, Chinese Academy of Agricultural Sciences.
Author Contributions
HQ, QL, and QD conceived and designed the experiments. XW, YZW, TL, SP, and HZ prepared the samples. XW completed the serum indices measurement. YYW detected the growth performance index. YYW and QL performed the sequencing of the 16S rRNA gene. SP and SZ completed the data analysis. SP and QL wrote the manuscript. QL and JZ refined the article. All authors contributed to the article and approved the submitted version.
Funding
The authors gratefully acknowledge the support from the Key R&D Program of Hunan Province (Grants: 2020NK2061 and 2020NK2031), Hunan Natural Science Foundation (Grant: 2019JJ50710), Agricultural Science and Technology Innovation Program (ASTIP-IBFC), Central Public-interest Scientific Institution Basal Research Fund (Grants: 1610242021005 and 1610242021006), and Modern Agricultural Technical System Foundation of China (CARS-42).
Conflict of Interest
The authors declare that the research was conducted in the absence of any commercial or financial relationships that could be construed as a potential conflict of interest.
Publisher’s Note
All claims expressed in this article are solely those of the authors and do not necessarily represent those of their affiliated organizations, or those of the publisher, the editors and the reviewers. Any product that may be evaluated in this article, or claim that may be made by its manufacturer, is not guaranteed or endorsed by the publisher.
References
Alagawany, M., Elnesr, S. S., and Farag, M. R. (2018). The role of exogenous enzymes in promoting growth and improving nutrient digestibility in poultry. Iranian J. Vet. Res. 19:157.
Amerah, A. M. (2015). Interactions between wheat characteristics and feed enzyme supplementation in broiler diets. Anim. Feed Sci. Technol. 199, 1–9. doi: 10.1016/j.anifeedsci.2014.09.012
Annison, G., and Choct, M. (1991). Anti-nutritive activities of cereal non-starch polysaccharides in broiler diets and strategies minimizing their effects. World’s Poultry Sci. J. 47, 232–242. doi: 10.1079/wps19910019
Ao, X., Meng, Q. W., Yan, L., Kim, H. J., Hong, S. M., Cho, J. H., et al. (2010). Effects of non-starch polysaccharide-degrading enzymes on nutrient digestibility, growth performance and blood profiles of growing pigs fed a diet based on corn and soybean meal. Asian Austral. J. Anim. Sci. 23, 1632–1638. doi: 10.5713/ajas.2010.10123
Applegate, T. J., Karcher, D. M., and Lilburn, M. S. (2005). Comparative development of the small intestine in the turkey poult and Pekin duckling. Poult. Sci. 84, 426–431. doi: 10.1093/ps/84.3.426
Bedford, A., and Gong, J. (2018). Implications of butyrate and its derivatives for gut health and animal production. Anim. Nutr. 4, 151–159. doi: 10.1016/j.aninu.2017.08.010
Bordin, M., D’Atri, F., Guillemot, L., and Citi, S. (2004). Histone deacetylase inhibitors up-regulate the expression of tight junction proteins11swiss cancer league, swiss national science foundation, Ministry for Italian University and Research, ERASMUS Program (M. Bordin), and Roche Research Foundation fellowship (L. Guillemot). Mol. Cancer Res. 2, 692–701.
Coppedge, J. R., Oden, L. A., Ratliff, B., Brown, B., Ruch, F., and Lee, J. T. (2012). Evaluation of nonstarch polysaccharide-degrading enzymes in broiler diets varying in nutrient and energy levels as measured by broiler performance and processing parameters. J. Appl. Poult. Res. 21, 226–234. doi: 10.3382/japr.2011-00329
Cross, M. L. (2002). Microbes versus microbes: immune signals generated by probiotic lactobacilli and their role in protection against microbial pathogens. FEMS Immunol. Med. Microbiol. 34, 245–253. doi: 10.1111/j.1574-695X.2002.tb00632.x
Cui, C., Shen, C. J., Jia, G., and Wang, K. N. (2013). Effect of dietary Bacillus subtilis on proportion of Bacteroidetes and Firmicutes in swine intestine and lipid metabolism. Genet. Mol. Res. 12, 1766–1776. doi: 10.4238/2013.May.23.1
Fukunaga, T., Sasaki, M., Araki, Y., Okamoto, T., Yasuoka, T., Tsujikawa, T., et al. (2003). Effects of the soluble fibre pectin on intestinal cell proliferation, fecal short chain fatty acid production and microbial population. Digestion 67, 42–49. doi: 10.1159/000069705
Gadde, U., Kim, W. H., Oh, S. T., and Lillehoj, H. S. (2017). Alternatives to antibiotics for maximizing growth performance and feed efficiency in poultry: a review. Anim. Health Res. Rev. 18, 26–45. doi: 10.1017/S1466252316000207
Grant, A. Q., Gay, C. G., and Lillehoj, H. S. (2018). Bacillus spp. as direct-fed microbial antibiotic alternatives to enhance growth, immunity, and gut health in poultry. Avian Pathol. 47, 339–351. doi: 10.1080/03079457.2018.1464117
Guo, M., Hao, G., Wang, B., Li, N., Li, R., Wei, L., et al. (2016). Dietary administration of Bacillus subtilis enhances growth performance, immune response and disease resistance in Cherry Valley ducks. Front. Microbiol. 7:1975. doi: 10.3389/fmicb.2016.01975
Han, X. Y., Yan, F. Y., Nie, X. Z., Wei, X. I. A., Sha, C. H. E. N., Zhang, X. X., et al. (2017). Effect of replacing antibiotics using multi-enzyme preparations on production performance and antioxidant activity in piglets. J. Integr. Agric. 16, 640–647. doi: 10.1016/s2095-3119(16)61425-9
Hooda, S., Boler, B. M. V., Serao, M. C. R., Brulc, J. M., Staeger, M. A., Boileau, T. W., et al. (2012). 454 pyrosequencing reveals a shift in fecal microbiota of healthy adult men consuming polydextrose or soluble corn fiber. J. Nutr. 142, 1259–1265. doi: 10.3945/jn.112.158766
Hu, Y. J., and Cowling, B. J. (2020). Reducing antibiotic use in livestock, China. Bull. World Health Organ. 98:360. doi: 10.2471/blt.19.243501
Jeong, J. S., and Kim, I. H. (2014). Effect of Bacillus subtilis C-3102 spores as a probiotic feed supplement on growth performance, noxious gas emission, and intestinal microflora in broilers. Poult. Sci. 93, 3097–3103. doi: 10.3382/ps.2014-04086
Kiarie, E., Romero, L. F., and Nyachoti, C. M. (2013). The role of added feed enzymes in promoting gut health in swine and poultry. Nutr. Res. Rev. 26, 71–88. doi: 10.1017/S0954422413000048
Klein, J., Williams, M., Brown, B., Rao, S., and Lee, J. T. (2015). Effects of dietary inclusion of a cocktail NSPase and β-mannanase separately and in combination in low energy diets on broiler performance and processing parameters. J. Appl. Poult. Res. 24, 489–501. doi: 10.3382/japr/pfv055
Koliada, A., Syzenko, G., Moseiko, V., Budovska, L., Puchkov, K., Perederiy, V., et al. (2017). Association between body mass index and Firmicutes/Bacteroidetes ratio in an adult Ukrainian population. BMC Microbiol. 17:120. doi: 10.1186/s12866-017-1027-1
Kumar, R. R., Lee, J. T., and Cho, J. Y. (2012). Fate, occurrence, and toxicity of veterinary antibiotics in environment. J. Korean Soc. Appl. Biol. Chem. 55, 701–709. doi: 10.1007/s13765-012-2220-4
Le Blay, G., Blottière, H. M., Ferrier, L., Le Foll, E., Bonnet, C., Galmiche, J. P., et al. (2000). Short-chain fatty acids induce cytoskeletal and extracellular protein modifications associated with modulation of proliferation on primary culture of rat intestinal smooth muscle cells. Dig. Dis. Sci. 45, 1623–1630. doi: 10.1023/a:1005529414765
LeBlanc, J. G., Milani, C., De Giori, G. S., Sesma, F., Van Sinderen, D., and Ventura, M. (2013). Bacteria as vitamin suppliers to their host: a gut microbiota perspective. Curr. Opin. Biotechnol. 24, 160–168. doi: 10.1016/j.copbio.2012.08.005
Lee, K. W., Kim, D. K., Lillehoj, H. S., Jang, S. I., and Lee, S. H. (2015). Immune modulation by Bacillus subtilis-based direct-fed microbials in commercial broiler chickens. Anim. Feed Sci. Technol. 200, 76–85. doi: 10.1016/j.anifeedsci.2014.12.006
Lee, S. H., Ingale, S. L., Kim, J. S., Kim, K. H., Lokhande, A., Kim, E. K., et al. (2014). Effects of dietary supplementation with Bacillus subtilis LS 1–2 fermentation biomass on growth performance, nutrient digestibility, cecal microbiota and intestinal morphology of weanling pig. Anim. Feed Sci. Technol. 188, 102–110. doi: 10.1016/j.anifeedsci.2013.12.001
Lin, M. Y., and Chang, F. J. (2000). Antioxidative effect of intestinal bacteria Bifidobacterium longum ATCC 15708 and Lactobacillus acidophilus ATCC 4356. Dig. Dis. Sci. 45, 1617–1622. doi: 10.1023/A:1005577330695
Lin, Q., Dai, Q., Bin, S., Jiang, G. T., and Zhang, J. H. (2012). Probiotics and enzyme preparation: effects on growth performance of yellow-feathered broilers and its mechanism. Chinese J. Anim. Nutr. 24, 1955–1965.
Lin, Q., Zhao, J., Xie, K., Wang, Y., Hu, G., Jiang, G., et al. (2017). Magnolol additive as a replacer of antibiotic enhances the growth performance of Linwu ducks. Anim. Nutr. 3, 132–138. doi: 10.1016/j.aninu.2017.03.004
Lin, Y., Xu, S., Zeng, D., Ni, X., Zhou, M., Zeng, Y., et al. (2017). Disruption in the cecal microbiota of chickens challenged with Clostridium perfringens and other factors was alleviated by Bacillus licheniformis supplementation. PLoS One 12:e0182426. doi: 10.1371/journal.pone.0182426
Liu, J., Xia, B., Du, X., Zeng, T., Liu, Y., Chen, L., et al. (2018). Effects of water supplemented with Bacillus subtilis and photosynthetic bacteria on egg production, egg quality, serum immunoglobulins and digestive enzyme activity of ducks. J. Appl. Anim. Res. 46, 322–326. doi: 10.1080/09712119.2017.1299741
Ministry of Agriculture of the People’s Republic of China (2012). Nutrient requirements of Meat-Type Duck. NY/T 2122–2012. Beijing: China Agriculture Press.
National Research Council (1994). Nutrient Requirements of Poultry: 1994. Washington, DC: National Academies Press.
Naumova, N. B., Alikina, T. Y., Zolotova, N. S., Konev, A. V., Pleshakova, V. I., Lescheva, N. A., et al. (2021). Bacillus-based probiotic treatment modified bacteriobiome diversity in duck feces. Agriculture 11:406. doi: 10.3390/agriculture11050406
Paik, H. D., Park, J. S., and Park, E. (2005). Effects of Bacillus polyfermenticus SCD on lipid and antioxidant metabolisms in rats fed a high-fat and high-cholesterol diet. Biol. Pharm. Bull. 28, 1270–1274. doi: 10.1248/bpb.28.1270
Peng, L., He, Z., Chen, W., Holzman, I. R., and Lin, J. (2007). Effects of butyrate on intestinal barrier function in a Caco-2 cell monolayer model of intestinal barrier. Pediatr. Res. 61, 37–41. doi: 10.1203/01.pdr.0000250014.92242.f3
Ravindran, V. (2013). Feed enzymes: the science, practice, and metabolic realities. J. Appl. Poult. Res. 22, 628–636. doi: 10.3382/japr.2013-00739
Ridlon, J. M., Kang, D. J., Hylemon, P. B., and Bajaj, J. S. (2015). Gut microbiota, cirrhosis, and alcohol regulate bile acid metabolism in the gut. Dig. Dis. 33, 338–345. doi: 10.1159/000371678
Rodriguez-Cabezas, M. E., Camuesco, D., Arribas, B., Garrido-Mesa, N., Comalada, M., Bailon, E., et al. (2010). The combination of fructooligosaccharides and resistant starch shows prebiotic additive effects in rats. Clin. Nutr. 29, 832–839. doi: 10.1016/j.clnu.2010.05.005
Salaheen, S., Kim, S. W., Haley, B. J., Van Kessel, J. A. S., and Biswas, D. (2017). Alternative growth promoters modulate broiler gut microbiome and enhance body weight gain. Front. Microbiol. 8:2088. doi: 10.3389/fmicb.2017.02088
Salim, H. M., Kruk, Z. A., and Lee, B. D. (2010). Nutritive value of corn distillers dried grains with solubles as an ingredient of poultry diets: a review. World’s Poult. Sci. J. 66, 411–432. doi: 10.1017/s0043933910000504
Sen, S., Ingale, S. L., Kim, Y. W., Kim, J. S., Kim, K. H., Lohakare, J. D., et al. (2012). Effect of supplementation of Bacillus subtilis LS 1-2 to broiler diets on growth performance, nutrient retention, caecal microbiology and small intestinal morphology. Res. Vet. Sci. 93, 264–268. doi: 10.1016/j.rvsc.2011.05.021
Sikandar, A., Zaneb, H., Younus, M., Masood, S., Aslam, A., Khattak, F., et al. (2017). Effect of sodium butyrate on performance, immune status, microarchitecture of small intestinal mucosa and lymphoid organs in broiler chickens. Asian Austral. J. Anim. Sci. 30:690. doi: 10.5713/ajas.16.0824
Stolker, A. A. M., Zuidema, T., and Nielen, M. W. F. (2007). Residue analysis of veterinary drugs and growth-promoting agents. TrAC Trends Anal. Chem. 26, 967–979. doi: 10.1016/j.trac.2007.09.008
Tuohy, K. M., Probert, H. M., Smejkal, C. W., and Gibson, G. R. (2003). Using probiotics and prebiotics to improve gut health. Drug Discov. Today 8, 692–700. doi: 10.1016/S1359-6446(03)02746-6
Uthai, K., Jattupornpong, S., Vandepitte, W., and Vanderbeke, E. (2004). Effects of dietary supplementation of enzymes in soybean meal rich diet on performance of growing-finishing (20-100 kg) pigs. Agric. Nat. Resour. 38, 125–131.
Wang, H., Wang, Y., and Yang, R. (2017). Recent progress in Bacillus subtilis spore-surface display: concept, progress, and future. Appl. Microbiol. Biotechnol. 101, 933–949. doi: 10.1007/s00253-016-8080-9
Watkins, E. J., Butler, P. J., and Kenyon, B. P. (2004). Posthatch growth of the digestive system in wild and domesticated ducks. Br. Poult. Sci. 45, 331–341. doi: 10.1080/00071660410001730824
Wealleans, A. L., Walsh, M. C., Romero, L. F., and Ravindran, V. (2017). Comparative effects of two multi-enzyme combinations and a Bacillus probiotic on growth performance, digestibility of energy and nutrients, disappearance of non-starch polysaccharides, and gut microflora in broiler chickens. Poult. Sci. 96, 4287–4429. doi: 10.3382/ps/pex226
Woyengo, T. A., Bogota, K. J., Noll, S. L., and Wilson, J. (2019). Enhancing nutrient utilization of broiler chickens through supplemental enzymes. Poult. Sci. 98, 1302–1309. doi: 10.3382/ps/pey452
Xin, J., Zeng, D., Wang, H., Ni, X., Yi, D., Pan, K., et al. (2014). Preventing nonalcoholic fatty liver disease through Lactobacillus johnsonii BS15 by attenuating inflammation and mitochondrial injury and improving gut environment in obese mice. Appl. Microbiol. Biotechnol. 98, 1–13. doi: 10.1007/s00253-014-5752-1
Keywords: gut microbiota, Bacillus, non-starch polysaccharidase, growth performance, serum antioxidant profiles, intestinal morphology, duck
Citation: Peng S, Wang X, Wang Y, Lv T, Zhao H, Wang Y, Zhu S, Qiu H, Zeng J, Dai Q and Lin Q (2021) Effects of Dietary Bacillus and Non-starch Polysaccharase on the Intestinal Microbiota and the Associated Changes on the Growth Performance, Intestinal Morphology, and Serum Antioxidant Profiles in Ducks. Front. Microbiol. 12:786121. doi: 10.3389/fmicb.2021.786121
Received: 29 September 2021; Accepted: 10 November 2021;
Published: 08 December 2021.
Edited by:
Jia Yin, Hunan Normal University, ChinaReviewed by:
Zeying Huang, Institute of Food and Nutrition Development, Chinese Academy of Agricultural Sciences (CAAS), ChinaFaizul Hassan, University of Agriculture, Faisalabad, Pakistan
Dunxue Chen, Guizhou University, China
Lu Ma, Institute of Animal Sciences, Chinese Academy of Agricultural Sciences (CAAS), China
Copyright © 2021 Peng, Wang, Wang, Lv, Zhao, Wang, Zhu, Qiu, Zeng, Dai and Lin. This is an open-access article distributed under the terms of the Creative Commons Attribution License (CC BY). The use, distribution or reproduction in other forums is permitted, provided the original author(s) and the copyright owner(s) are credited and that the original publication in this journal is cited, in accordance with accepted academic practice. No use, distribution or reproduction is permitted which does not comply with these terms.
*Correspondence: Jianguo Zeng, emVuZ2ppYW5ndW9AaHVuYXUuZWR1LmNu; Qiuzhong Dai, ZGFpcWl1emhvbmdAMTYzLmNvbQ==; Qian Lin, bGlucWlhbkBjYWFzLmNu
†These authors have contributed equally to this work and share first authorship