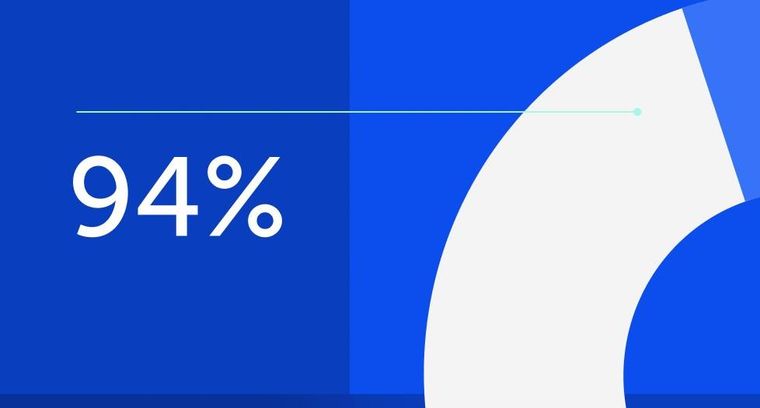
94% of researchers rate our articles as excellent or good
Learn more about the work of our research integrity team to safeguard the quality of each article we publish.
Find out more
ORIGINAL RESEARCH article
Front. Microbiol., 13 January 2022
Sec. Antimicrobials, Resistance and Chemotherapy
Volume 12 - 2021 | https://doi.org/10.3389/fmicb.2021.785411
This article is part of the Research TopicEcology, Environment, and Human Microbiome Interaction with InfectionView all 10 articles
During winter, a large number of rooks gather and defecate at the park of a university clinic. We investigated the prevalence of extended-spectrum beta-lactamase (ESBL)–producing Escherichia coli in these birds and compared recovered isolates with contemporary human isolates. In 2016, fecal samples were collected from 112 trap-captured rooks and investigated for presence of ESBL producers using eosin methylene blue agar supplemented by 2 mg/L cefotaxime; 2,455 contemporary human fecal samples of patients of the clinics sent for routine culturing were tested similarly. In addition, 42 ESBL-producing E. coli isolates collected during the same period from inpatients were also studied. ESBL genes were sought for by PCR and were characterized by sequencing; E. coli ST131 clones were identified. Epidemiological relatedness was determined by pulsed-field gel electrophoresis and confirmed using whole genome sequencing in selected cases. Thirty-seven (33%) of sampled rooks and 42 (1.7%) of human stools yielded ESBL-producing E coli. Dominant genes were blaCTX–M–55 and blaCTX–M–27 in corvid, blaCTX–M–15 and blaCTX–M–27 in human isolates. ST162 was common among rooks. Two rook-derived E. coli belonged to ST131 C1-M27, which was also predominant (10/42) among human fecal and (15/42) human clinical isolates. Another potential link between rooks and humans was a single ST744 rook isolate grouped with one human fecal and three clinical isolates. Despite possible contact, genotypes shared between rooks and humans were rare. Thus, rooks are important as long-distance vectors and reservoirs of ESBL-producing E. coli rather than direct sources of infections to humans in our setting.
Antibiotic resistance is a global problem impacting both human and animal health. The One Health concept sets forth that the health of people, animals, and the environment is interconnected, which fully applies to antibiotic resistance as well, as exemplified by the relationship between avoparcin usage and the spread of vancomycin-resistant Enterococci in Europe (Bager et al., 1997). Besides spread of resistant strains, gene flow between bacteria of human and animal origin drives the dissemination of resistance genes (Graham et al., 2019). Zoonotic or environmental reservoirs served as sources for emerging resistance genes, e.g., Kluyvera spp. as source for blaCTX–M genes, Shewanella algae as source for blaOXA–48, or Acinetobacter radioresistens as source for blaOXA–23–like genes (Livermore et al., 2007; Poirel et al., 2008; Tacão et al., 2018). Resistant bacteria can spread between humans and their households involving their companion animals, and the environment and wildlife. International travel, trade of animal food products, and wildlife migration further contribute to the global dissemination of antibiotic resistance (Guenther et al., 2011; Hussain et al., 2017, 2019; Zendri et al., 2020).
Escherichia coli is a characteristic example linking One Health and antibiotic resistance, being a frequent and abundant member of both human and animal gut microbiome as well as an important pathogen of humans and animals. The massive usage of antibiotics both in human medicine and animal industry led to contamination of natural environments with antimicrobials, antibiotic resistance genes, and resistant human pathogens (Graham et al., 2014, 2019). Wildlife living in contaminated habitats such as landfills, wastewater, sewage sludge of farms, or exposed directly to feces from livestock and companion animals can acquire resistant bacteria or resistance genes (Graham et al., 2014, 2019). These animals, particularly the highly mobile species, may scatter the resistant bacteria. Wild birds were shown to carry antibiotic resistant bacteria; typical carriers are crows (Loncaric et al., 2013; Jamborova et al., 2015) and gulls (Báez et al., 2015; Zendri et al., 2020), which often utilize human waste as food source. These birds are frequently urbanized, and their droppings pollute the cities, potentially reintroducing strains into the human environment. Because of their migration and/or vagrant behavior, these birds may serve as reservoirs and long-distance vectors both for antibiotic-resistant strains and antibiotic resistance genes (Wang et al., 2017).
Our aim was to investigate the prevalence of ESBL-producing E. coli carried by rooks (Corvus frugilegus ssp. frugilegus, Linnaeus 1758) gathering in a university clinic and to compare these isolates with contemporary and geographically related human-derived isolates.
Cloacal swabs were taken from 112 trap-captured rooks wintering in a suburban environment close to the clinical campus of the University of Debrecen between October 2016 and March 2017. The trapping and capturing process was conducted as previously described (Kövér et al., 2018); recapturing did not occur. In parallel, we screened all 2,455 contemporary human fecal samples of the patients of the university clinics sent for routine fecal culture during the study period to assess human asymptomatic fecal carriage of ESBL-producing bacteria using the same culture methodology. Third-generation cephalosporin (3GC)–resistant isolates were recovered using eosin–methylene blue media supplemented with 2 mg/L cefotaxime. One to three colonies per different morphologies were processed further from each sample and identified by matrix-assisted laser desorption ionization (MALDI)–time of flight (TOF) mass spectrometry (Bruker, Bremen, Germany). We also characterized 42 contemporary extended-spectrum beta-lactamase (ESBL)–producing E. coli isolates from various samples of inpatients of the university clinics sent for microbiological diagnostic purposes for comparison with isolates carried by rooks and humans. Production of ESBL was examined by double-disk synergy test using cefotaxime, ceftazidime, and cefepime. Susceptibility to ertapenem, ciprofloxacin, trimethoprim–sulfamethoxazole, amikacin, gentamicin, and tobramycin was determined by disk diffusion method following EUCAST guidelines.
Each isolate showing ESBL phenotype was screened by PCR for blaSHV, blaCTX–M, and for CTX-M-1, 2, 8, and 9 subgroups (Ebrahimi et al., 2014, 2016a,b). All amplicons were purified by QIAquick Gel Extraction Kit (Qiagen, Hilden, Germany) and further characterized by sequencing (Macrogen Europe, Amsterdam, Netherlands). Sequences were analyzed by CLC Main Workbench (CLC Bio, Aarhus, Denmark).
To investigate the presence of plasmid-mediated colistin resistance genes mcr-1, mcr-2, mcr-3, mcr-4, and mcr-5, a multiplex PCR assay was used (Rebelo et al., 2018).
We determined the different E. coli phylogenetic groups by the multiplex PCR method developed by Clermont et al. (2013). A multiplex PCR assay was performed to detect the presence of virulence factor genes characteristic for enterovirulent E. coli pathotypes (Persson et al., 2007). To identify the E. coli sequence type (ST) 131 clonal lineage and its members [clades A, B, C, and C subclades (C1-M27, C1-non-M27, and C2)], we used the multiplex PCR developed by Matsumura et al. (2017).
To analyze the epidemiological relationship, we used pulsed-field gel electrophoresis (PFGE) as previously described (Ebrahimi et al., 2014). The threshold for probable genetic relatedness was set to a similarity of >85%.
Based on the results of the PFGE, 20 isolates were selected for whole genome sequencing (WGS) to represent major pulsotypes carried by birds as well as pulsotypes that contained both human and bird isolates to reveal possible connections. Genomic DNA was extracted using Zixpress-32 Bacterial DNA Extraction Kit on Zixpress-32 Automated Nucleic Acid Purification Instrument (Zinexts Life Science Corporation) following the manufacturer’s instructions. WGS was performed using Nextera XT DNA Library Preparation Kit followed by 150-bp single-end sequencing on Illumina NextSeq500 platform. FASTQ files were quality trimmed then assembled de novo using Velvet (v1.0.0.); these are available under BioProject ID PRJNA693168. ResFinder (Camacho et al., 2009; Bortolaia et al., 2020; Zankari et al., 2020), PlasmidFinder (Camacho et al., 2009; Carattoli et al., 2014), and VirulenceFinder (Joensen et al., 2014; Malberg Tetzschner et al., 2020) available from the Center for Genomic Epidemiology1 were used to identify resistance genes, plasmid replicon types, and virulence factors. Multi-locus sequence typing (MLST) and core genome MLST (cgMLST) were performed using SeqSphere + (Ridom, Münster, Germany) according to the “E. coli MLST Warwick v1.0” and “E. coli cgMLST” version 1.0 scheme.
Extended-spectrum beta-lactamase-producing bacteria were carried by 37 (33%) of 112 sampled birds and a total of 43 isolates have been recovered, all of which were E. coli; six samples (8544, 8551, 8557, 8578, 8583, and HOR3) yielded two different morphologies and during further analysis they turned to be pheno- and genotypically different ESBL-producing E. coli isolates (Supplementary Figure 2). The predominant ESBL genes were blaCTX–M–55 (16/43) followed by blaCTX–M–27 (n = 15/43) (Supplementary Figure 1). Fluoroquinolone (17/43) and sulfonamide (23/43) resistance was frequent whereas all isolates were susceptible to aminoglycosides; 40% (17/43) of the isolates were susceptible to examined non-beta-lactam antibiotics including all blaCTX–M–15 producers. The majority of the isolates carried by birds belonged to commensal phylogroups A (2.3%, 1/43), B1 (51.2%, 22/43), and C (7%, 3/43), B1 being the dominant phylogroup. However, a high proportion (40%, 17/43) of rook isolates belonged to phylogroups associated with human disease, B2 (34.9%, 15/43) and D (4.7%, 2/43) (Supplementary Figure 1). Two of B2 CTX-M-27-producing E. coli isolates proved to belong to the pandemic ST131 clonal lineage, to the recently emerged C1-M27 subclone. In addition, 21% (9/43) of the isolates carried the intimin coding eae gene.
In 2,455 human fecal samples, 42 ESBL-producing E. coli were found corresponding to a fecal carriage rate of 1.7%. The dominant ESBL genotypes were blaCTX–M–15 (20/42) followed by blaCTX–M–27 (10/42) (Supplementary Figure 1). Resistance to fluoroquinolones (24/42), sulfonamides (29/42), amikacin (14/42), gentamicin (12/42), and to tobramycin (14/42) was common. Isolates of commensal phylogroups were more prevalent; four, three, eight, and eight isolates belonged to phylogroup A, B1, C, and E, but overall B2 (17/42) was the dominant phylogroup. Of the B2 isolates, two, one, one, and ten belonged to ST131 clade A, B, subclade C2, and subclade C1-M27, respectively.
The dominant ESBL genes were blaCTX–M–15 (18/42) and blaCTX–M–27 (13/42) (Supplementary Figure 1). As sole ESBL gene, blaSHV–12 was present in two isolates. Co-resistance rates were high; 60% (25/42) of isolates were resistant to fluoroquinolones, sulfonamides, and aminoglycosides, mostly the blaCTX–M–15 producers. The majority (74%, 31/42) of the isolates belonged to B2 phylogroup with high prevalence (62%, 26/42) of ST131 clones. Among ST131 isolates, one, nine, and 16 belonged to clade B, subclade C2, and subclade C1-M27, respectively. Curiously, three ST131 C1-M27 isolates were blaCTX–M–15 producers.
In rooks, blaCTX–M–55 was the dominant ESBL gene while in humans it was blaCTX–M–15; blaCTX–M–27 was the second most common ESBL gene in all three isolate collections (Supplementary Figure 1). Group 2 and group 8 CTX-M types were not detected. Rook-derived isolates showed lower co-resistance rates to non-beta-lactam antibiotics than human clinical isolates. Isolates resistant to aminoglycosides, fluoroquinolones, and trimethoprim–sulfamethoxazole tend to carry CTX-M-1 group, particularly blaCTX–M–15, except for rooks where blaCTX–M–15 producers were susceptible; blaCTX–M–27 producers were resistant to fluoroquinolones and to trimethoprim–sulfamethoxazole but not to aminoglycosides. Carbapenem resistance was not detected in the recovered isolates. The majority of rook and human fecal isolates belonged to commensal phylogroups while B2 was dominant among human clinical isolates. The pandemic ST131 E. coli clonal lineage was present in isolates of rooks and humans with the dominance of C1-M27 subclade. All isolates were negative for plasmid-mediated colistin resistance genes tested.
Pulsed-field gel electrophoresis revealed that human clinical and fecal isolates clustered frequently together whereas the vast majority of rook isolates tended to cluster separately from human isolates (Supplementary Figure 2), although clusters containing both rook and human isolates were also found. Out of these, a cluster of eight human fecal, ten human clinical, and two rook isolates (EC069) was the largest, which belonged to the ST131 clone. Most isolates of the ST131 clone were grouped into three clusters (EC003, EC069, and EC380) although a few other ST131 isolates were randomly distributed. The EC003 cluster exclusively consisted of human clinical ST131 isolates while EC380 cluster contained human fecal ST131 isolates. In the EC069 cluster, the two corvid ST131 isolates showed PFGE profiles indistinguishable from human clinical and human fecal isolates. A smaller cluster of three human clinical, one human fecal, and one rook isolate (EC088) was also detected. In addition, clusters EC147 and EC183 each comprised one rook and one human fecal isolate.
A total of eleven, four, and five of rook, human clinical, and human fecal isolates, respectively, were characterized by WGS (Table 1). The results of the cgMLST are shown in Figure 1. The relatedness of isolates in PFGE clusters of EC183 and EC399 were not supported by the results of the WGS (Table 1 and Supplementary Figure 2). Both ST24 and ST162 rook isolates were highly uniform genetically; distance based on allele presence was ≤1, although these isolates have been recovered from various birds in November and December. Human-derived ST744 isolates were closely related, but the rook one was relatively distant from this cluster; ST131 C1-M27 rook isolates were identical and in close connection with human strains (≤7 alleles) (Figure 1).
Figure 1. Minimum spanning tree based on cgMLST allelic profiles of 20 sequenced E. coli isolates. Each circle represents an allelic profile based on sequence analysis of 2,513 cgMLST target genes. The numbers on the connecting lines illustrate the numbers of target genes with different alleles. Closely related genotypes (<10 alleles difference) are shaded.
An increasing number of studies reported high prevalence of ESBL-producing E. coli in wild animals (Wang et al., 2017). Birds are the most studied hosts, where the most frequently found genes are blaCTX–M–1 and blaCTX–M–15 (Wang et al., 2017). Birds may serve as long distance vectors of strains/genes of human origin. Franklin’s gulls (Leucophaeus pipixcan) sampled in Chile frequently carried pandemic ST131 CTX-M-15-producing strains, which are highly prevalent in humans in the United States but rarely found in Chile (Báez et al., 2015). Similarly, blaCTX–M–1 and blaCTX–M–15 were previously dominant in rooks wintering in Europe (Loncaric et al., 2013; Jamborova et al., 2015). A few recent European studies also reported a low prevalence of blaCTX–M–55 and blaCTX–M–24 in rooks (Jamborova et al., 2015; Söderlund et al., 2019). Importantly, an earlier study from 2005 reported lack of ESBL producers in wintering rooks in the Czech Republic (Literak et al., 2007).
Rooks wintering in Hungary and in neighboring countries belong to a large population migrating from Russia and Western Asia, belonging to subspecies Western Rook (Corvus frugilegus frugilegus) with a breeding area stretching from the East European Plain to the West Siberian Plain. These populations migrate through the Black Sea–Mediterranean flyway and usually winter in European countries (Madge, 2020). The other rook subspecies Eastern Rook (Corvus frugilegus pastinator) nests in the Central Siberian Plateau and the Manchurian Plain and migrates to China and to Japan through the East Asian–Australasian flyway. On the border of West Siberian Plain and Central Siberian Plateau, there is a hybrid zone of the two subspecies, where birds may intermingle (Madge, 2020).
In the present work, blaCTX–M–55 and blaCTX–M–27 were predominant in rooks; blaCTX–M–55 is rarely reported in Europe from humans but highly prevalent in Southeast Asia (Lupo et al., 2018). It has been suggested that blaCTX–M–55 in humans in Asia arose from food animal sources, highlighting the importance of One Health (Bevan et al., 2017). Previously, blaCTX–M–14 and, to a lesser extent, blaCTX–M–15 were dominant ESBL genes in Asia; recently, blaCTX–M–55 emerged as the most common ESBL gene in human and animal isolates, while blaCTX–M–27 started to outcompete blaCTX–M–14 (Bevan et al., 2017). As blaCTX–M–55 is dominant in livestock in Asia (Bevan et al., 2017), and manure is often used to fertilize crop fields and may contain ESBL-producing E. coli, rooks foraging in these may acquire blaCTX–M–55 producers. This shift in the epidemiology of ESBL genes in Asia may be the cause of the alteration of ESBL genes in rooks as compared with earlier studies (Loncaric et al., 2013; Jamborova et al., 2015).
We hypothesized that Eastern Rooks acquire strains carrying resistance genes prevalent in animals and humans in China and transmit them to Western Rook individuals interacting with carrier Eastern Rooks in the hybrid area. Thus, intermingling rooks may become long-distance vectors mediating spread of strains/genes from Asia to Europe. Similarly, this may have been the route for clade C1-M27 described first in Japan in 2006 then in Korea in 2008 spreading since to Europe and to America (Matsumura et al., 2016). Similar spread routes of H5N1 avian influenza virus was reported extensively in different bird species (Gauthier-Clerc et al., 2007). High similarity between ST131 C1-M27 isolates of rook and human origin may also be explained by acquisition of these strains by rooks in Hungary, suggesting bidirectional transfer. Food importation may be another potential pathway where blaCTX–M–55 can spread from animals to humans toward Europe as exemplified by the dissemination mcr-1 resistance gene since a lot of poultry and pork are imported from China to Europe (Hasman et al., 2015) and the detection of these genes can often be traced back there (Lupo et al., 2018).
The majority of rook isolates of phylogroup B2 had indistinguishable macrorestriction profile (Supplementary Figure 2) identified as ST24 carrying blaCTX–M–27. ST24 was reported rarely, mainly from diarrheic rabbits, cattle, and humans (Moura et al., 2009; Xiong et al., 2012). All ST24 isolates carried the eae gene (Supplementary Table 1), which encodes a major virulence gene of enteropathogenic E. coli (EPEC); lack of the bfp gene indicates that these isolates are atypical EPEC strains, which are reported both from humans and animals (Moura et al., 2009). A study found that atypical EPEC strains of animal origin have potential to cause diarrhea in humans and revealed a close clonal relationship between human and animal isolates (Moura et al., 2009).
The main phylogroup was B1 among rook isolates, forming a large cluster belonging to ST162 and carrying blaCTX–M–55 (Supplementary Table 1). ST162 was found previously in rooks wintering in Europe with low occurrence (Loncaric et al., 2013; Jamborova et al., 2015). This emerging multiresistant E. coli lineage is now found worldwide colonizing different hosts including livestock, wild animals, humans, rivers, and sewage (Fuentes-Castillo et al., 2020). ST162 was reported from dairy cows with mastitis (Tahar et al., 2020) and from human clinical samples, even associated with blaNDM–5 in humans (Yoon et al., 2018). These associations raise the concern of dissemination of commensal multiresistant strains in human populations and the diffusion of the antibiotic resistance carried by these strains to other non-commensal, pathogenic strains (Zhuge et al., 2019). Moreover, ST162 E. coli recovered from poultry was identified as a highly virulent clone, despite belonging to phylogroup B1, capable of causing bloodstream infections and meningitis in animal models (Zhuge et al., 2019). In our study, ST162 and ST24 isolates seemed to have clonally expanded in rooks, which is notable as the clonal spread of ESBL-producing E. coli is scarcely documented in wild animals (Lupo et al., 2018).
Sequence types with human importance ST744 and ST131 C1-M27 were found seldom. ST744 is an international high-risk clone identified in our rook, human fecal, and clinical isolates (Table 1). ST744 carrying blaCTX–M–55 was previously reported from diseased pigs in the United States and from healthy and diseased bovines in France, from wastewater, birds of prey (Guenther et al., 2012; Lupo et al., 2018; Hayer et al., 2020) as well as from healthy and diseased companion animals, and humans (Tacão et al., 2017; Zhong et al., 2017; Zogg et al., 2018). Besides ESBLs, ST744 was sporadically associated with mcr-1, mcr-3, blaKPC–3, and blaNDM genes from patients, healthy individuals, and livestock worldwide (Tacão et al., 2017; Zhong et al., 2017; Lupo et al., 2018; Zogg et al., 2018; Li et al., 2019). ST744 isolates of phylogroup A are not as virulent as those belonging to phylogroup B2 and D, but our ST744 rook isolate carried 15 virulence factors (Supplementary Table 1) commonly found in extraintestinal pathogenic E. coli (ExPEC) isolates.
Presence of key virulence genes (iss, iroN, hlyF and ompT, iutA and cvaC) and phylogroup A indicates that our rook ST744 is an avian pathogenic E. coli (APEC) strain, which is the main cause of avian colibacillosis (Johnson et al., 2008). Therefore, wild birds carrying APEC strains might pose a potential economic risk toward poultry; these genes are also frequently found among human ExPEC strains, raising the possibility of zoonotic transmission (Johnson et al., 2008; Zhuge et al., 2019). These suggest that ST744 may be a zoonotic strain capable of colonizing and infecting multiple host species including humans. Moreover, its potential to carry multiple plasmids predisposes it to be involved in transmission of resistance plasmids to other E. coli STs.
Subclade ST131 C1-M27 is associated with clonal spread in humans, and was also reported from great cormorants (Phalacrocorax carbo), mallards (Anas platyrhynchos) (Tausova et al., 2012), gulls (Zendri et al., 2020), companion animals, freshwater, and wastewater (Bevan et al., 2017). Similarly, it occurred in rooks and was also prevalent in human isolates in our study.
Although blaCTX–M–15 remained the predominant ESBL gene among asymptomatically carried human isolates in this study, the prevalence of ST131-CTX-M-15 E. coli was lower compared with earlier findings (Ebrahimi et al., 2014, 2016a,b), suggesting a slow replacement of C2 subclade carrying blaCTX–M–15 by the C1-M27 subclade (Merino et al., 2018). ST131 C1-M27 had a higher transmission rate than CTX-M-15-producing ST131 C2 (Merino et al., 2018). C1-M27 isolates often show lower co-resistance to other antimicrobial agents than C2 isolates (Jamborova et al., 2018), which may be advantageous in an antibiotic landscape dominated by beta-lactams as seen in many European countries including Hungary, and particularly the setting where this study was conducted (Tóth et al., 2019).
Both blaCTX–M–27 and blaCTX–M–55 are associated with a wide range of plasmid replicons in animal isolates and certain plasmids showed epidemic spread in Asia in humans (Bevan et al., 2017; Lupo et al., 2018). Unlike blaCTX–M–27 associated with ST131 C1-M27, horizontal transmission is considered to be the main factor driving the dissemination of blaCTX–M–55 in China (Ho et al., 2013). In our work, blaCTX–M–55 have been associated with IncN replicon type (Table 1), which often harbors various ESBL genes but rarely blaCTX–M–552. This association of blaCTX–M–55 with IncN plasmids carried by ST162 may open a new way to the dissemination of blaCTX–M–55 in and from Asia toward Europe by bird migration or vagrancy as it may have earlier happened in the case of ST131 C1-M27 (Matsumura et al., 2016).
In summary, increased carriage of ESBL-producing E. coli was found in rooks than reported in previous years. Despite the possibilities for contact, birds and humans shared a low proportion of genotypes. The presence of high-risk clones (ST131 and ST744), high prevalence of Asia-related ESBL genes (blaCTX–M–55 and blaCTX–M–27) together with the epidemiological history of ST131 C1-M27 clone suggests that rooks are among the potential vectors for the dissemination of antibiotic resistance genes and resistant strains.
The datasets presented in this study can be found in online repositories. The names of the repository/repositories and accession number(s) can be found in the article/Supplementary Material.
Ethical review and approval was not required for the animal study because the birds were captured for the purpose of bird ringing. Bird ringing permission number 309 (Birdlife Hungary MME).
GK, LK, ÁL-K, and ÁT: conceptualization. BN, BB, and PG: data curation. BN, EK, KBl, and ÁT: formal analysis. BN, BB, IB, ID, and KBl: investigation. PG, LK, ÁL-K, ID, BN, LM, ÁT, and KBn: methodology. LK, ÁT, KBn, and GK: resources. EK, KBl, ID, and ÁT: software. ÁT and GK: supervision. PG, ÁL-K, ID, LM, ÁT, and KBn: validation. ID and ÁT: visualization. BN and GK: writing—original draft. BN, ÁT, and GK: writing—review and editing. All authors contributed to the article and approved the submitted version.
GK was supported by a Bolyai Scholarship of the Hungarian Academy of Sciences. BB was supported by the New National Excellence Program of the Ministry of Human Capacities (ÚNKP-19-3-I).
The authors declare that the research was conducted in the absence of any commercial or financial relationships that could be construed as a potential conflict of interest.
All claims expressed in this article are solely those of the authors and do not necessarily represent those of their affiliated organizations, or those of the publisher, the editors and the reviewers. Any product that may be evaluated in this article, or claim that may be made by its manufacturer, is not guaranteed or endorsed by the publisher.
The help of the technicians of Medical Microbiology during screening work and National Public Health Center with PFGE are gratefully acknowledged.
The Supplementary Material for this article can be found online at: https://www.frontiersin.org/articles/10.3389/fmicb.2021.785411/full#supplementary-material
Báez, J., Hernández-García, M., Guamparito, C., Díaz, S., Olave, A., Guerrero, K., et al. (2015). Molecular characterization and genetic diversity of ESBL-producing Escherichia coli colonizing the migratory Franklin’s gulls (Leucophaeus pipixcan) in Antofagasta, North of Chile. Microb. Drug Resist. 21, 111–116. doi: 10.1089/mdr.2014.0158
Bager, F., Madsen, M., Christensen, J., and Aarestrup, F. M. (1997). Avoparcin used as a growth promoter is associated with the occurrence of vancomycin-resistant Enterococcus faecium on Danish poultry and pig farms. Prev. Vet. Med. 31, 95–112. doi: 10.1016/s0167-5877(96)01119-1
Bevan, E. R., Jones, A. M., and Hawkey, P. M. (2017). Global epidemiology of CTX-M β-lactamases: temporal and geographical shifts in genotype. J. Antimicrob. Chemother. 72, 2145–2155. doi: 10.1093/jac/dkx146
Bortolaia, V., Kaas, R. F., Ruppe, E., Roberts, M. C., Schwarz, S., Cattoir, V., et al. (2020). ResFinder 4.0 for predictions of phenotypes from genotypes. J. Antimicrob. Chemother. 75, 3491–3500. doi: 10.1093/jac/dkaa345
Camacho, C., Coulouris, G., Avagyan, V., Ma, N., Papadopoulos, J., Bealer, K., et al. (2009). BLAST+: architecture and applications. BMC Bioinformatics 10:421. doi: 10.1186/1471-2105-10-421
Carattoli, A., Zankari, E., Garcia-Fernandez, A., Voldby Larsen, M., Lund, O., Villa, L., et al. (2014). In silico detection and typing of plasmidsusing PlasmidFinder and plasmid multilocus sequence typing. Antimicrob. Agents Chemother. 58, 3895–3903. doi: 10.1128/AAC.02412-14
Clermont, O., Christenson, J. K., Denamur, E., and Gordon, D. M. (2013). The Clermont Escherichia coli phylo-typing method revisited: improvement of specificity and detection of new phylo-groups. Environ. Microbiol. Rep. 5, 58–65. doi: 10.1111/1758-2229.12019
Ebrahimi, F., Mózes, J., Mészáros, J., Juhász, Á., and Kardos, G. (2014). Carriage rates and characteristics of Enterobacteriaceae producing extended-spectrum beta-lactamases in healthy individuals: comparison of applicants for long-term care and individuals screened for employment purposes. Chemotherapy 60, 239–249. doi: 10.1159/000375407
Ebrahimi, F., Mózes, J., Monostori, J., Gorácz, O., Fésűs, A., Majoros, L., et al. (2016a). Comparison of rates of fecal colonization with extended-spectrum beta-lactamase-producing enterobacteria among patients in different wards, outpatients and medical students. Microbiol. Immunol. 60, 285–294. doi: 10.1111/1348-0421.12373
Ebrahimi, F., Mózes, J., Mészáros, J., Juhász, Á., Majoros, L., Szarka, K., et al. (2016b). Asymptomatic fecal carriage of ESBL producing Enterobacteriaceae in Hungarian healthy individuals and in long-term care applicants: a shift towards CTX-M producers in the community. Infect. Dis. 48, 557–559. doi: 10.3109/23744235.2016.1155734
Fuentes-Castillo, D., Esposito, F., Cardoso, B., Dalazen, G., Moura, Q., Fuga, B., et al. (2020). Genomic data reveal international lineages of critical priority Escherichia coli harboring wide resistome in Andean condors (Vultur gryphus Linnaeus, 1758). Mol. Ecol. 29, 1919–1935. doi: 10.1111/mec.15455
Gauthier-Clerc, M., Lebarbenchon, C., and Thomas, F. (2007). Recent expansion of highly pathogenic avian influenza H5N1: a critical review. Ibis 149, 202–214. doi: 10.1111/j.1474-919X.2007.00699.x
Graham, D. W., Bergeron, G., Bourassa, M. W., Dickinson, J., Gomes, F., Howe, A., et al. (2019). Complexities in understanding antimicrobial resistance across domesticated animal, human, and environmental systems. Ann. N. Y. Acad. Sci. 1441, 17–30. doi: 10.1111/nyas.14036
Graham, D. W., Collignon, P., Davies, J., Larsson, D. G. J., and Snape, J. (2014). Underappreciated role of regionally poor water quality on globally increasing antibiotic resistance. Environ. Sci. Technol. 48, 11746–11747. doi: 10.1021/es504206x
Guenther, S., Aschenbrenner, K., Stamm, I., Bethe, A., Semmler, T., Stubbe, A., et al. (2012). Comparable high rates of extended-spectrum-beta-lactamase-producing Escherichia coli in birds of prey from Germany and Mongolia. PLoS One 7:e53039. doi: 10.1371/journal.pone.0053039
Guenther, S., Ewers, C., and Wieler, L. H. (2011). Extended-spectrum beta-lactamases producing E. coli in wildlife, yet another form of environmental pollution? Front. Microbiol. 2:246. doi: 10.3389/fmicb.2011.00246
Hasman, H., Hammerum, A. M., Hansen, F., Hendriksen, R. S., Olesen, B., Agersø, Y., et al. (2015). Detection of mcr-1 encoding plasmid-mediated colistin-resistant Escherichia coli isolates from human bloodstream infection and imported chicken meat, Denmark. Euro Surveill. 20:30085. doi: 10.2807/1560-7917.ES.2015.20.49.30085
Hayer, S. S., Lim, S., Hong, S., Elnekave, E., Johnson, T., Rovira, A., et al. (2020). Genetic Determinants of Resistance to Extended-Spectrum Cephalosporin and Fluoroquinolone in Escherichia coli Isolated from Diseased Pigs in the United States. mSphere 5:e00990-20. doi: 10.1128/mSphere.00990-20
Ho, P. L., Chan, J., Lo, W. U., Law, P. Y., Li, Z., Lai, E. L., et al. (2013). Dissemination of plasmid-mediated fosfomycin resistance fosA3 among multidrug-resistant Escherichia coli from livestock and other animals. J. Appl. Microbiol. 114, 695–702. doi: 10.1111/jam.12099
Hussain, A., Shaik, S., Ranjan, A., Nandanwar, N., Tiwari, S. K., Majid, M., et al. (2017). Risk of transmission of antimicrobial resistant Escherichia coli from commercial broiler and free-range retail chicken in India. Front. Microbiol. 8:2120. doi: 10.3389/fmicb.2017.02120
Hussain, A., Shaik, S., Ranjan, A., Suresh, A., Sarker, N., Semmler, T., et al. (2019). Genomic and functional characterization of poultry Escherichia coli from India revealed diverse Extended-spectrum β-lactamase-producing lineages with shared virulence profiles. Front. Microbiol. 10:2766. doi: 10.3389/fmicb.2019.02766
Jamborova, I., Dolejska, M., Vojtech, J., Guenther, S., Uricariu, R., Drozdowska, J., et al. (2015). Plasmid-mediated resistance to cephalosporins and fluoroquinolones in various Escherichia coli sequence types isolated from rooks wintering in Europe. Appl. Environ. Microbiol. 81, 648–657. doi: 10.1128/AEM.02459-14
Jamborova, I., Johnston, B. D., Papousek, I., Kachlikova, K., Micenkova, L., Clabots, C., et al. (2018). Extensive genetic commonality among wildlife, wastewater, community, and nosocomial isolates of Escherichia coli sequence type 131 (H30R1 and H30Rx Subclones) that carry blaCTX–M–27 or blaCTX–M–15. Antimicrob Agents Chemother. 62:e00519-18. doi: 10.1128/AAC.00519-18
Joensen, K. G., Scheutz, F., Lund, O., Hasman, H., Kaas, R. S., Nielsen, E. M., et al. (2014). Real-time whole-genome sequencing for routine typing, surveillance, and outbreak detection of verotoxigenic Escherichia coli. J. Clin. Micobiol. 52, 1501–1510. doi: 10.1128/JCM.03617-13
Johnson, T. J., Wannemuehler, Y., Johnson, S. J., Stell, A. L., Doetkott, C., Johnson, J. R., et al. (2008). Comparison of extraintestinal pathogenic Escherichia coli strains from human and avian sources reveals a mixed subset representing potential zoonotic pathogens. Appl. Environ. Microbiol. 74, 7043–7050. doi: 10.1128/AEM.01395-08
Kövér, L., Tóth, N., Lengyel, S. Z., and Juhász, L. (2018). Corvid control in urban environments: a comparison of trap types. North West. J. Zool. 14, 85–90.
Li, J., Bi, Z., Ma, S., Chen, B., Cai, C., He, J., et al. (2019). Inter-host transmission of carbapenemase-producing Escherichia coli among humans and backyard animals. Environ. Health Perspect. 127:107009. doi: 10.1289/EHP5251
Literak, I., Vanko, R., Dolejska, M., Cízek, A., and Karpísková, R. (2007). Antibiotic resistant Escherichia coli and Salmonella in Russian rooks (Corvus frugilegus) wintering in the Czech Republic. Lett. Appl. Microbiol. 45, 616–621. doi: 10.1111/j.1472-765X.2007.02236.x
Livermore, D. M., Canton, R., Gniadkowski, M., Nordmann, P., Rossolini, G. M., Arlet, G., et al. (2007). CTX-M: changing the face of ESBLs in Europe. J. Antimicrob. Chemother. 59, 165–174. doi: 10.1093/jac/dkl483
Loncaric, I., Stalder, G. L., Mehinagic, K., Rosengarten, R., Hoelzl, F., Knauer, F., et al. (2013). Comparison of ESBL–and AmpC producing Enterobacteriaceae and methicillin-resistant Staphylococcus aureus (MRSA) isolated from migratory and resident population of rooks (Corvus frugilegus) in Austria. PLoS One 8:e84048. doi: 10.1371/journal.pone.0084048
Lupo, A., Saras, E., Madec, J. Y., and Haenni, M. (2018). Emergence of blaCTX-M-55 associated with fosA, rmtB and mcr gene variants in Escherichia coli from various animal species in France. J. Antimicrob. Chemother. 73, 867–872. doi: 10.1093/jac/dkx489
Madge, S. (2020). “Rook (Corvus frugilegus), version 1.0,” in Birds of the World, eds J. del Hoyo, A. Elliott, J. Sargatal, D. A. Christie, and E. de Juana (Ithaca, NY: Cornell Lab of Ornithology). doi: 10.2173/bow.rook1.01
Malberg Tetzschner, A. M., Johnson, J. R., Johnston, B. D., Lund, O., and Scheutz, F. (2020). In Silico Genotyping of Escherichia coli Isolates for Extraintestinal Virulence Genes by Use of Whole-Genome Sequencing Data. J. Clin. Microbiol. 58:e01269-20. doi: 10.1128/JCM.01269-20
Matsumura, Y., Pitout, D. D. J., Peirano, G., DeVinney, R., Noguchi, T., Yamamoto, M., et al. (2017). Rapid identification of different Escherichia coli sequence type 131 clades. Antimicrob. Agents Chemother. 61:e00179-17. doi: 10.1128/AAC.00179-17
Matsumura, Y., Pitout, J. D., Gomi, R., Matsuda, T., Noguchi, T., Yamamoto, M., et al. (2016). Global Escherichia coli sequence type 131 clade with blaCTX-M-27 gene. Emerg. Infect. Dis. 22, 1900–1907. doi: 10.3201/eid2211.160519
Merino, I., Hernández-García, M., Turrientes, M. C., Pérez-Viso, B., López-Fresneña, N., Diaz-Agero, C., et al. (2018). Emergence of ESBL-producing Escherichia coli ST131-C1-M27 clade colonizing patients in Europe. J. Antimicrob. Chemother. 73, 2973–2980. doi: 10.1093/jac/dky296
Moura, R. A., Sircili, M. P., Leomil, L., Matté, M. H., Trabulsi, L. R., Elias, W. P., et al. (2009). Clonal relationship among atypical enteropathogenic Escherichia coli strains isolated from different animal species and humans. Appl. Environ. Microbiol. 75, 7399–7408. doi: 10.1128/AEM.00636-09
Persson, S., Olsen, K. E. P., Scheutz, F., Krogfelt, K. A., and Gerner-Smidt, P. (2007). A method for fast and simple detection of major diarrhoeagenic Escherichia coli in the routine diagnostic laboratory. Clin. Microbiol. Infect. 13, 516–524. doi: 10.1111/j.1469-0691.2007.01692
Poirel, L., Figueiredo, S., Cattoir, V., Carattoli, A., and Nordmann, P. (2008). Acinetobacter radioresistens as a silent source of carbapenem resistance for Acinetobacter spp. Antimicrob. Agents Chemother. 52, 1252–1256. doi: 10.1128/AAC.01304-07
Rebelo, A. R., Bortolaia, V., Kjeldgaard, J. S., Pedersen, S. K., Leekitcharoenphon, P., Hansen, I. M., et al. (2018). Multiplex PCR for detection of plasmid-mediated colistin resistance determinants, mcr-1, mcr-2, mcr-3, mcr-4 and mcr-5 for surveillance purposes. Eurosurveillance 23, 17–00672. doi: 10.2807/1560-7917.ES.2018.23.6.17-00672
Söderlund, R., Skarin, H., Börjesson, S., Sannö, A., Jernberg, T., Aspán, A., et al. (2019). Prevalence and genomic characteristics of zoonotic gastro-intestinal pathogens and ESBL/pAmpC producing Enterobacteriaceae among Swedish corvid birds. Infect. Ecol. Epidemiol. 9:1701399. doi: 10.1080/20008686.2019.1701399
Tacão, M., Araújo, S., Vendas, M., Alves, et al. (2018). Shewanella species as the origin of blaOXA-48 genes: insights into gene diversity, associated phenotypes and possible transfer mechanisms. Int. J. Antimicrob. Agents 51, 340–348. doi: 10.1016/j.ijantimicag.2017.05.014
Tacão, M., dos Santos Tavares, R., Teixeira, P., Roxo, I., Ramalheira, E., Ferreira, S., et al. (2017). mcr-1 and blaKPC-3 in Escherichia coli sequence type 744 after meropenem and colistin therapy, Portugal. Emerg. Infect. Dis. 23, 1419–1421. doi: 10.3201/eid2308.170162
Tahar, S., Nabil, M. M., Safia, T., Ngaiganam, E. P., Omar, A., Hafidha, C., et al. (2020). Molecular Characterization of Multidrug-Resistant Escherichia coli Isolated from Milk of Dairy Cows with Clinical Mastitis in Algeria. J. Food Prot. 83, 2173–2178. doi: 10.4315/JFP-20-198
Tausova, D., Dolejska, M., Cizek, A., Hanusova, L., Hrusakova, J., Svoboda, O., et al. (2012). Escherichia coli with extended-spectrum β-lactamase and plasmid-mediated quinolone resistance genes in great cormorants and mallards in Central Europe. J. Antimicrob. Chemother. 67, 1103–1107. doi: 10.1093/jac/dks017
Tóth, H., Fésűs, A., Kungler-Gorácz, O., Balázs, B., Majoros, L., Szarka, K., et al. (2019). Utilization of vector autoregressive and linear transfer models to follow up the antibiotic resistance spiral in gram-negative bacteria from cephalosporin consumption to colistin resistance. Clin. Infect. Dis. 69, 1410–1421. doi: 10.1093/cid/ciy1086
Wang, J., Ma, Z. B., Zeng, Z. L., Yang, X. W., Huang, Y., and Liu, J. H. (2017). The role of wildlife (wild birds) in the global transmission of antimicrobial resistance genes. Zool. Res. 38, 55–80. doi: 10.24272/j.issn.2095-8137.2017.003
Xiong, Y., Wang, P., Lan, R., Ye, C., Wang, H., Ren, J., et al. (2012). A novel Escherichia coli O157: H7 clone causing a major hemolytic uremic syndrome outbreak in China. PLoS One 7:e36144. doi: 10.1371/journal.pone.0036144
Yoon, E. J., Kang, D. Y., Yang, J. W., Kim, D., Lee, H., Lee, K. J., et al. (2018). New Delhi metallo-beta-lactamase-producing Enterobacteriaceae in South Korea between 2010 and 2015. Front. Microbiol. 9:571. doi: 10.3389/fmicb.2018.00571
Zankari, E., Allesøe, R., Joensen, K. G., Cavaco, L. M., Lund, O., and Aarestrup, F. M. (2020). PointFinder: a novel web tool for WGS-based detection of antimicrobial resistance associated with chromosomal point mutations in bacterial pathogens. J. Antimicrob. Chemother. 72, 2764–2768. doi: 10.1093/jac/dkx217
Zendri, F., Maciuca, I. E., Moon, S., Jones, P. H., Wattret, A., Jenkins, R., et al. (2020). Occurrence of ESBL-Producing Escherichia coli ST131, Including the H30-Rx and C1-M27 Subclones, Among Urban Seagulls from the United Kingdom. Microb. Drug Resist. 26, 697–708. doi: 10.1089/mdr.2019.0351
Zhong, L. L., Zhang, Y. F., Doi, Y., Huang, X., Zhang, X. F., Zeng, K. J., et al. (2017). Coproduction of MCR-1 and NDM-1 by colistin-resistant Escherichia coli isolated from a healthy individual. Antimicrob. Agents Chemother. 61:e01962-16. doi: 10.1128/AAC.01962-16
Zhuge, X., Jiang, M., Tang, F., Sun, Y., Ji, Y., Xue, F., et al. (2019). Avian-source mcr-1-positive Escherichia coli is phylogenetically diverse and shares virulence characteristics with E. coli causing human extra-intestinal infections. Vet. Microbiol. 239:108483. doi: 10.1016/j.vetmic.2019.108483
Zogg, A. L., Zurfluh, K., Schmitt, S., Nüesch-Inderbinen, M., and Stephan, R. (2018). Antimicrobial resistance, multilocus sequence types and virulence profiles of ESBL producing and non-ESBL producing uropathogenic Escherichia coli isolated from cats and dogs in Switzerland. Vet. Microbiol. 216, 79–84. doi: 10.1002/mbo3.845
Keywords: ESBL carriage, E. coli ST131, E. coli ST162, E. coli ST744, long-distance dispersal, bird migration, CTX-M-55
Citation: Nagy BJ, Balázs B, Benmazouz I, Gyüre P, Kövér L, Kaszab E, Bali K, Lovas-Kiss Á, Damjanova I, Majoros L, Tóth Á, Bányai K and Kardos G (2022) Comparison of Extended-Spectrum Beta-Lactamase-Producing Escherichia coli Isolates From Rooks (Corvus frugilegus) and Contemporary Human-Derived Strains: A One Health Perspective. Front. Microbiol. 12:785411. doi: 10.3389/fmicb.2021.785411
Received: 29 September 2021; Accepted: 09 December 2021;
Published: 13 January 2022.
Edited by:
Alain Hartmann, Institut National de Recherche pour l’Agriculture, l’Alimentation et l’Environnement (INRAE), FranceReviewed by:
Arif Hussain, International Centre for Diarrhoeal Disease Research, Bangladesh (icddr,b), BangladeshCopyright © 2022 Nagy, Balázs, Benmazouz, Gyüre, Kövér, Kaszab, Bali, Lovas-Kiss, Damjanova, Majoros, Tóth, Bányai and Kardos. This is an open-access article distributed under the terms of the Creative Commons Attribution License (CC BY). The use, distribution or reproduction in other forums is permitted, provided the original author(s) and the copyright owner(s) are credited and that the original publication in this journal is cited, in accordance with accepted academic practice. No use, distribution or reproduction is permitted which does not comply with these terms.
*Correspondence: Gábor Kardos, a2dAbWVkLnVuaWRlYi5odQ==
Disclaimer: All claims expressed in this article are solely those of the authors and do not necessarily represent those of their affiliated organizations, or those of the publisher, the editors and the reviewers. Any product that may be evaluated in this article or claim that may be made by its manufacturer is not guaranteed or endorsed by the publisher.
Research integrity at Frontiers
Learn more about the work of our research integrity team to safeguard the quality of each article we publish.